- 1Key Laboratory for Organic Electronics and Information Displays & Jiangsu Key Laboratory for Biosensors, Jiangsu National Synergetic Innovation Center for Advanced Materials (SICAM), Institute of Advanced Materials (IAM), Nanjing University of Posts and Telecommunications, Nanjing, China
- 2Institute of Flexible Electronics, Northwestern Polytechnical University, Xi'an, China
The exploitation of thermally activated delayed fluorescence (TADF) emitters with aggregation-induced emission is highly prerequisite for the construction of highly efficient electroluminescent devices in materials science. Herein, two asymmetric TADF emitters of SFCOCz and SFCODPAC with charming aggregation-induced emission are expediently designed and prepared based on highly twisted strong electron-withdrawing acceptor (A) of sulfurafluorene (SF)-modified ketone (CO) and arylamine donor (D) in D1−A–D2 architecture by simple synthetic procedure in high yields. High photoluminescence quantum yields up to 73% and small singlet–triplet splitting of 0.03 eV; short exciton lifetimes are obtained in the resultant molecules. Strikingly, efficient non-doped and doped TADF organic light-emitting diodes (OLEDs) facilitated by these emitters show high luminance of 5,598 and 11,595 cd m−2, current efficiencies (CEs) of 16.8 and 35.6 cd/A, power efficiencies (PEs) of 9.1 and 29.8 lm/W, and external quantum efficiencies (EQEs) of 7.5 and 15.9%, respectively. This work furnishes a concrete instance in exploring efficient TADF emitter, which is highly conducive and encouraging in stimulating the development of TADF OLEDs with high brightness and excellent efficiencies simultaneously.
Introduction
Luminescent materials that are capable of thermally activated delayed fluorescent (TADF) have been widely investigated not only because of their great potential in utilizing theoretically 100% internal quantum efficiency (IQE) through the back transfer of non-radiative triplet exactions (75%) into radiative singlet excitons conferred by small single–triplet energy splitting (ΔEST) for efficient reverse intersystem crossing (RISC), but also due to their fundamental significance both in scientific investigations and technological applications of organic electronics (Uoyama et al., 2012; Tao et al., 2014; Etherington et al., 2016; Guo et al., 2018; Han et al., 2018; Kotadiya et al., 2019; Pershin et al., 2019; Zhang Y. L. et al., 2019). With flourish developments over the past few years, considerable attention has been devoted to designing and exploiting excellent TADF materials with the consideration of the following rational metrics (Park et al., 2016; Chen et al., 2017; Im et al., 2017; Wong and Zysman-Colman, 2017; Yang Z. et al., 2017; Zhang Y. et al., 2019): (i) the separated highest occupied molecular orbital (HOMO) and lowest unoccupied molecular orbital (LUMO) distributions for enabling small ΔEST; (ii) slight overlap of frontier molecular orbital (FMO) for maintaining high photoluminescence quantum yield (PLQY); (iii) short exciton lifetimes to eliminate the concentration-induced quenching effect; (iv) reduced intermolecular interactions to alleviate aggregation quenching processes; and (v) acceptable thermal stability for long-term device operation and ease synthesis procedure for mass productions. Nonetheless, most of the reported TADF materials suffer from serious aggregation caused quenching (ACQ) phenomenon that obviously hinders their practical applications (Einzinger et al., 2017; Wei et al., 2017, 2019; Wong and Zysman-Colman, 2017; Gan et al., 2019). Therefore, it remains forbidden issues to construct prominent TADF materials because of the great challenge in obtaining such aforementioned features in a molecule simultaneously, especially for alleviating the serious ACQ (Aydemir et al., 2017).
Aggregation-induced emission (AIE) is a fascinating optical phenomenon with greatly enhanced luminescent efficiency in solid state, which has been increasingly emerging as a promising candidate in organic electronics, bio-electronics, and photonics (Huang et al., 2017; Mao et al., 2017; Tsujimoto et al., 2017; Yang J. et al., 2017; Chen et al., 2019). The implantation of AIE properties into TADF materials has demonstrated a possibility to suppress the ACQ of solid films (Lee et al., 2017; Zheng et al., 2019). Generally, the linkage of donor and acceptor units through the spiro- and/or twist structure to lessen the overlap of FMO and to inhibit the molecular aggregation has been proven to be a perspective strategy for achieving AIE-TADF materials. Following this guideline, an extensive collection of AIE-TADF materials has been designed and explored in fabricating efficient TADF organic light-emitting diodes (OLEDs). Recently, diphenyl ketone, which can not only serve as an electron-deficient core to construct charge transfer (CT) molecule with spatially separated HOMO and LUMO distributions through the incorporation of varieties of donor units for achieving a small ΔEST but can also be used as the twist and rotation center to reduce the interactions for alleviating the self-quenching effect of multiple molecules and to incorporate AIE for boosting emission of the resultant materials in solid state, has been regarded as one of the most key building blocks in constructing TADF materials with the AIE character (Guo et al., 2017; Huang et al., 2017). These impressive advantages have stimulated us to explore new diphenyl ketone-based TADF derivatives for fabricating high-performance OLEDs.
Herein, to achieve the AIE-TADF materials, we designed and synthesized two emitters of SFCOCz and SFCODPAC with asymmetric D1−A–D2 architecture (Figure 1A) through the direct linkage of sulfurafluorene (SF)-modified ketone (CO) and arylamine of carbazole (Cz) or 9,9-diphenyl-9,10-dihydroacridine (DPAC). In this moiety, the D1−A–D2 can effectively render separated HOMO and LUMO distributions to guarantee a small ΔEST for the promotion of RISC process under thermal activation; moreover, the twisted and asymmetric molecular configuration can endow AIE and restrain intermolecular interaction of π-π stacking and/or aggregation to reduce ACQ in solid state (Aydemir et al., 2017; Wang et al., 2017; Zeng et al., 2018; Liu et al., 2019). These newly constructed AIE-TADF materials in D1−A–D2 skeleton can be easily prepared with high yields up to 68%. Strikingly, SFCOCz and SFCODPAC exhibited excellent TADF with small ΔEST of ~0.03 eV, high PLQY (ΦPL) of ~73%, and efficient RISC rate and relatively short delayed fluorescence lifetime. High-performance non-doped and doped TADF OLEDs endowed by these emitters were fabricated, showing high luminance of 5,598 and 11,595 cd m−2 and external quantum efficiencies (EQEs) of 7.5 and 15.9%, respectively. The ease of synthetic route, excellent optoelectronics, and high device performance make the SFCO-based asymmetric TADF emitters promising candidates in practical applications, conferring a new paradigm for next-generation organoelectronics.
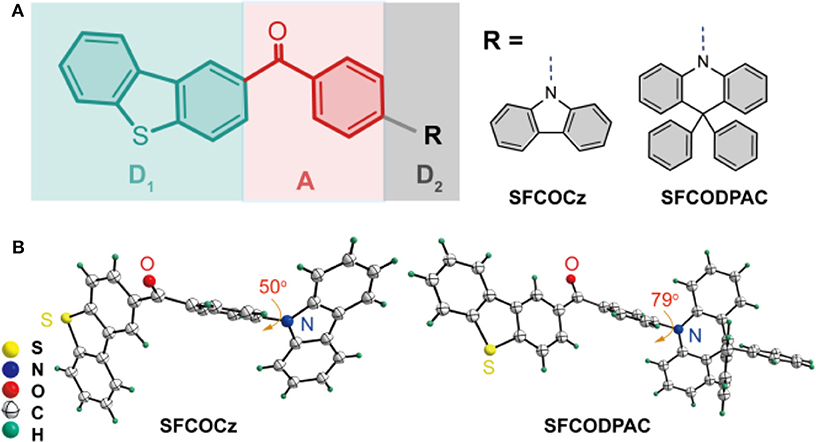
Figure 1. (A) Schematical drawing of designed SFCO-based thermally activated delayed fluorescence (TADF) materials with asymmetric D1−A–D2 architecture. (B) ORTEP single-crystal structures of SFCOCz (CCDC 1964730) and SFCODPAC (CCDC 1964747).
Results and Discussion
Design, Synthesis, and Characterization
To prepare the asymmetric TADF materials exhibiting AIE trait, two molecules, namely (4-(9H-carbazol-9-yl)phenyl)(dibenzo[b,d]thiophen-2-yl)methanone (SFCOCz) and dibenzo[b,d]thiophen-2-yl(4-(9,9-diphenylacridin-10(9H)-yl)phenyl)methanone (SFCODPAC), were designed and synthesized in D1−A–D2 architecture through the direct connection of sulfurafluorene-tailored diphenyl ketone and donor of Cz (SFCOCz) or DPAC (SFCODPAC) by a conventional two-step procedure composed of Friedel–Crafts acylation and C–N coupling reaction (Figure 1A and Scheme S1, Figures S1–S4). As revealed by single-crystal X-ray diffraction (XRD) analysis, the dihedral angle between arylamine and SFCO in these two molecules is up to 79° (Figure 1B and Table S1). Such a highly twisted molecular conformation would not only be beneficial to reduce electron communications, ensuring an optimized HOMO and LUMO separation for acquiring a small ΔEST, but can also effectively suppress the molecular aggregations in solid state for eliminating ACQ (Wang et al., 2017). SFCOCz and SFCODPAC display high thermal stabilities (Figure S5), exposing the decomposition temperatures (Td) of 386 and 429°C as revealed by the thermogravimetric (TGA) measurements and melting temperature (Tm) of 197 and 251°C as measured by differential scanning calorimetry (DSC) analyses. The slightly higher Td and Tm of SFCODPAC than those of SFCOCz could be well-explained by its high molecular weight and rigid structure. In addition, the vacuum-evaporated thin films on glass substrates are amorphous and uniform with quite small root-mean-square roughness (RMS) of 0.307 and 0.173 nm for (Figure S6) SFCOCz and SFCODPAC. The excellent thermal and morphology stabilities of resultant AIE-TADF materials would be favored for vacuum-deposited device fabrication and long-term operation stability.
Photophysical Properties
The photophysical profiles of asymmetric TADF molecules SFCOCz and SFCODPAC in dilute dichloromethane solution (CH2Cl2, 1 × 10−5 mol L−1) and neat and doped films (Figure 2 and Figures S7–S11) were detailedly investigated by UV–visible absorption and photoluminescence (PL) spectra. SFCOCz and SFCODPAC imply that the n–π* transition dominated absorption band peaked ~300 nm, and the CT band at ~350 nm originated from intramolecular CT (ICT) from arylamine to CO (Table 1 and Table S2) (Lee et al., 2017). The PL spectra of SFCOCz and SFCODPAC exhibit typical structureless ICT fluorescence bands located at 484 and 558 nm in CH2Cl2, respectively. The ICT characters were also demonstrated by the red-shifted emission bands with the increasing in solvent polarities (Figure S8). In film states, these two molecules reveal nearly the same absorption profiles to those of the solution, showing absorption peaks at 295 and 346 nm for SFCOCz and 297 and 359 nm for SFCODPAC. These findings verify the effective suppressing of molecule aggregation in the films. The optical bandgaps (°ptEgs), calculated by the onset edge of the absorption spectra, were 3.04 and 2.88 eV for SFCOCz and SFCODPAC, respectively. For the PL spectra, SFCOCz and SFCODPAC also show structureless ICT bands at ~450 and 492 nm with corresponding ΦPL of ~22 and 73%, respectively. Notably, the ΦPLs of SFCOCz and SFCODPAC in the solid state are up to ~3.7-fold that in the solution, indicating the obvious AIE characteristics. To further demonstrate the AIE properties of these two TADF molecules, the PL spectra in tetrahydrofuran (THF) with varied water fractions (fw) were performed. SFCOCz and SFCODPAC exhibit intense emissions as the formation of nanoaggregates upon injecting a large amount of poor solvent of H2O (fraction >80%) into THF solutions (Figures 2B–D), suggesting again the AIE features. This significantly enhanced photoluminescence in nanoaggregates should be due to the suppressed molecule rotation and motion that are highly active in the solution, thus blocking the non-radiative decay of excitons. In addition, we also explore the PL properties of SFCOCz (30 wt%) and SFCODPAC (30 wt%) doped films using 2,8-bis(diphenyl-phosphoryl)-dibenzo[b,d]thiophene (PPT) as the host material (Figure S9). Because of the strong polarity of PPT, the doped films showcase the red-shifted emission peaks with lower ΦPL compared to their corresponding neat films (Méhes et al., 2014).
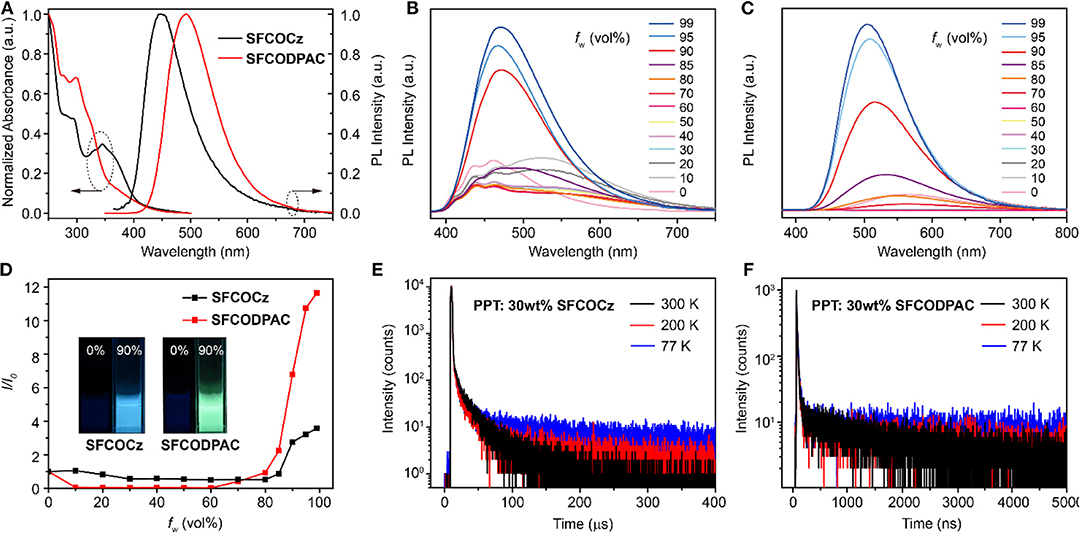
Figure 2. (A) Absorption and photoluminescence (PL) (excited at 320 nm) spectra of asymmetric thermally activated delayed fluorescence (TADF) molecules in films. PL spectra of (B) SFCOCz and (C) SFCODPAC in tetrahydrofuran (THF)/water mixtures with varied water fractions (fw). (D) Plots of I/I0 values vs. water fractions (fw). I0 is representing the integrated intensity in THF. Inset: photographs of the TADF molecules in THF/water mixture. (E,F) Temperature-dependent decay profiles of (E) SFCOCz and (F) SFCODPAC in doped film (30 wt% in PPT).
To demonstrate their TADF properties, a set of experiments was carried out. We attempted to estimate the ΔEST of SFCOCz and SFCODPAC on the basis of the fluorescence spectra and phosphorescence spectra at 77 K. As shown in Figure S10, the ΔESTs of SFCOCz and SFCODPAC in neat and (30 wt%) doped films were 0.17 and 0.03 eV in the neat films, and 0.21 and 0.02 eV in the doped films, respectively, potentially enabling the process of RISC by thermal activation. The transient photoluminescence profiles were also performed to understand the photophysical process of SFCOCz and SFCODPAC (Figures 2D,E and Figure S11). The double exponential lifetime decay curve was observed in both of SFCOCz and SFCODPAC in neat and doped films, showing a short nanosecond lifetime of prompt fluorescence (τPF) and a microsecond lifetime (τDF) of delayed emission (Table S2). Contributed by the small ΔEST, τDF of SFCODPAC were 0.22 and 0.84 μs in the neat and doped films, respectively, which is 1,513- and 24-fold smaller than those of SFCOCz. The temperature-dependent lifetime measurements of the doped films further present efficient proof of the TADF characteristics of these two materials. The delayed component afforded by the RISC was increased gradually, with the temperature increasing from 77 to 300 K (Figures 2E,F), obviously indicating the TADF trait. By means of the PLQY and lifetime, the calculated rate of RISC (Supplementary Material) are 0.1 × 105 and 6.16 × 106 s−1 in neat films, and 1.3 × 105 and 2.16 × 106 s−1 in doped films for SFCOCz and SFCODPAC, respectively. The greatly improved RISC of SFCODPAC could be attributed to its smaller ΔEST.
Theoretical and Electrochemical Investigations
As shown in Figure 3, the spatial distributions of HOMOs and LUMOs of SFCOCz and SFCODPAC were clearly observed (Leitl et al., 2014). The HOMOs are largely located on the electron-donating (D2) unit of Cz for SFCOCz and DPAC for SFCODPAC, while the LUMOs are mainly concentrated on CO and slightly located on the SF unit, showing an overlap extent (IH/L) of 32.2 and 10.9%, respectively (Chen et al., 2015). The discrete HOMOs and LUMOs distribution in the asymmetric D1−A–D2 materials would be favorable for realizing small ΔEST. The simulated ΔESTs were 0.38 and 0.01 eV for SFCOCz and SFCODPAC in the monomeric state. The decreased ΔEST of SFCODPAC may be originated from the combined effect of the strong electron-donating ability of DPAC and large dihedral angle between CO and DPAC for more separated FMO distributions. The FMO energy levels of these two molecules were analyzed by the cyclic voltammetric (CV) curves. According to the oxidation onset at 0.94 and 0.76 V, the HOMOs were evaluated to be −5.70 and −5.52 eV for SFCOCz and SFCODPAC, respectively. By means of optical bandgaps and HOMOs, the LUMOs were speculated to be −2.66 and −2.64 eV. To reveal the electron transition components of excited states in SFCOCz and SFCODPAC, the natural transition orbital (NTO) analyses were also carried out (Li et al., 2018). The highest occupied NTO (HONTO) and the lowest unoccupied NTO (LUNTO) distributions of SFCOCz and SFCODPAC at excited states are almost identical to their corresponding ground-state FMOs. The HONTOs of these two molecules were primarily dominated by the donor moiety; the LUNTO were largely assigned on the CO core with slight extension on the SF unit. The overlap extent of HONTO and LUNTO is 41.5 and 16.2% on singlet state (Is), and 65.7 and 22.2% on triplet state (IT) for SFCOCz and SFCODPAC, respectively. The overlap at the excited state should play an important role in guaranteeing high PLQY.
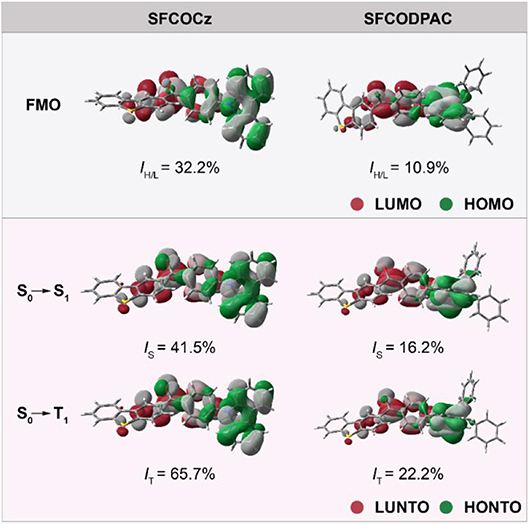
Figure 3. Density functional theory (DFT) calculated frontier molecular orbital (FMO) distributions, natural transition orbital (NTO) analyses, and IH/L, IS, and IT of asymmetric thermally activated delayed fluorescence (TADF) molecules of SFCOCz and SFCODPAC.
Electroluminescent Performance of OLEDs
To further elucidate the feasibility of asymmetric D1−A–D2 molecules in constructing high-performance thermal-evaporated devices, the non-doped (TA-TB) and doped (TC-TD) TADF OLED of SFCOCz (TA, TC) and SFCODPAC (TB, TD) were successfully fabricated using the following configurations (Figure 4A): ITO/MoO3 (30 nm)/4,4′-bis[N-(1-naphthyl)-N-phenylamino]-1,1′-biphenyl (NPB) (50 nm)/1,3-bis(carbazol-9-yl)benzene (mCP) (5 nm)/EML (20 nm)/PPT (5 nm)/bathophenanthroline (BPhen) (5 nm)/LiF (1 nm)/Al (100 nm). In these devices, NPB and BPhen were hole- and electron-transporting layers, and mCP and PPT were exciton-blocking layers. TA-TD exhibited pure electroluminescence (EL) spectra inherited from their neat and doped films at different driving voltages, showing maximum emission peaks at 451, 482, 468, and 485 nm with corresponding Commission International de l'Eclairage (CIE) coordinates of (0.16, 0.11), (0.20, 0.37), (0.15, 0.18), and (0.19, 0.37), respectively (Table S3). Compared to SFCOCz-based TA and TC, SFCODPAC-endowed TADF OLEDs of TB and TD revealed slightly decreased driving voltages (Vons) of 4.4 and 3.8 V, respectively. In addition to the decreased Von, TB and TD also demonstrated improved luminance up to 5,598 and 11,595 cd m−2 than those of TA and TC. The low Vons and high luminance of TB and TD should be attributed to its high HOMO and PLQYs of SFCODPAC for the promotion of hole injection from the adjacent layer and the generation of excitons. Not surprisingly, TADF OLEDs based on SFCODPAC show much better device efficiencies with current efficiencies (CEs) of 16.8 and 35.6 cd/A, power efficiencies (PEs) of 9.1 and 29.8 lm/W, and EQEs of 7.5 and 15.9% for TB and TD, respectively (Figures 4B,C and Table S3). There values are comparable to the best results of non-doped and doped TADF OLEDs based on AIE-type TADF emitters (Table S4). In addition, TD displays acceptable device performance with 29.1 and 21.0 cd A−1 for CE, 11.8 and 5.6 lm W−1 for PE, and 13.1 and 9.4% for EQE at 100 and 1,000 cd m−2, respectively. Although the non-doped films suggest enhanced PLQYs, the poor charge transport properties in neat films induced by highly twisted asymmetric molecular skeleton should be responsible for the decreased device performance of non-doped TADF OLEDs.
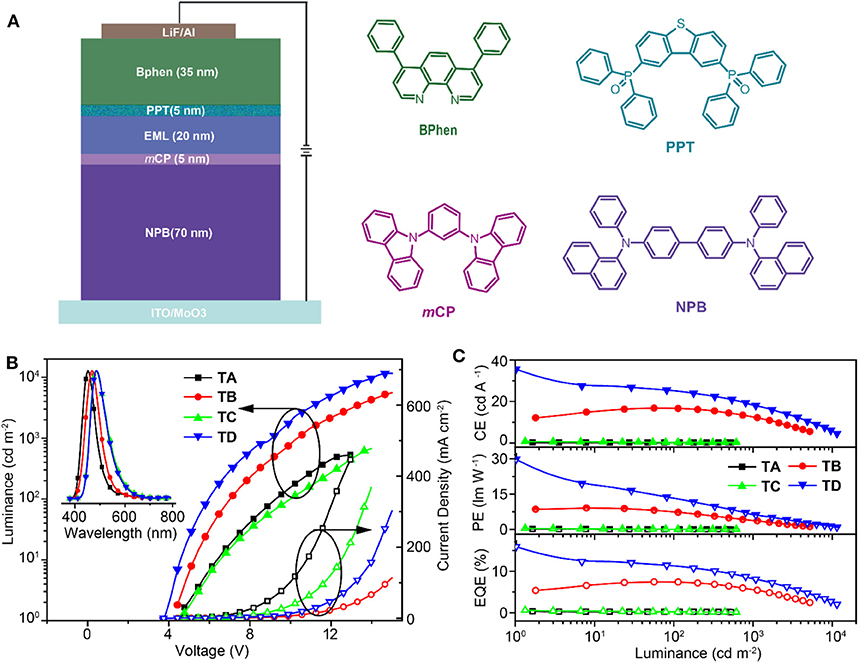
Figure 4. (A) Device configuration and chemical structures of the adopted materials, (B) luminance (solid symbols)–current density (open symbols)–voltage inset: electroluminescence (EL) spectra of thermally activated delayed fluorescence organic light-emitting diodes (TADF OLEDs) at 6.0 V, and (C) efficiencies–luminescence curves of the non-doped and doped TADF OLEDs.
Conclusions
In summary, we have succeeded in designing and developing two asymmetric TADF molecules of SFCOCz and SFCODPAC in the D1−A–D2 skeleton through a simple and effective procedure in high yields. The resultant TADF molecules exhibit small ΔEST, short delayed lifetimes, and robust AIE characteristics with high PLQY up to 73%. More impressively, benefiting from the excellent optoelectronic properties, the non-doped and doped TADF OLEDs conferred by SFCODPAC display high efficiencies with peak EQEs of 7.5 and 15.9%, accompanied with the CIE coordinates of (0.20, 0.37) and (0.19, 0.37), respectively. Our work here provides a delicate molecular design strategy for the construction of asymmetric AIE-type TADF emitters, and clearly manifests the significant advance of the combined TADF and AIE features in exploiting high-performance organic emitters.
Data Availability Statement
The datasets generated for this study can be found in the Cambridge Structural Database under the following identifiers: CCDC/1964730, CCDC/1964747.
Author Contributions
HuaL, YZ, YT, WH, and RC conceived the experiment. HuaL, YZ, YJ, and HuiL performed the characterizations of optoelectronic properties. YD, YT, and ML designed and fabricated the devices. QY and PL contributed the theoretical simulations. HuaL, YZ, YJ, and YT wrote the manuscript. All authors contributed to the scientific discussion.
Funding
This work was financially supported in part by the National Natural Science Foundation of China (21604039, 21704042, 61875090, 91833306, and 51873159), the Six Talent Plan of Jiangsu Province (XCL-049), 1311 Talents Program of Nanjing University of Posts and Telecommunications (Dingshan), China Postdoctoral Science Foundation project (2018M642284), and Nanjing University of Posts and Telecommunications Start-up Fund (NUPTSF) (NY219007 and NY217140).
Conflict of Interest
The authors declare that the research was conducted in the absence of any commercial or financial relationships that could be construed as a potential conflict of interest.
Supplementary Material
The Supplementary Material for this article can be found online at: https://www.frontiersin.org/articles/10.3389/fchem.2020.00049/full#supplementary-material
References
Aydemir, M., Xu, S., Chen, C., Bryce, M. R., Chi, Z., and Monkman, A. P. (2017). Photophysics of an asymmetric donor-acceptor-donor' TADF molecule and reinterpretation of aggregation-induced TADF emission in these materials. J. Phys. Chem. C. 121, 17764–17772. doi: 10.1021/acs.jpcc.7b06299
Chen, F., Hu, J., Wang, X., Shao, S., Wang, L., and Jing, X. (2019). Synthesis and electroluminescent properties of through-space charge transfer polymers containing acridan donor and triarylboron acceptors. Front. Chem. 7:854. doi: 10.3389/fchem.2019.00854
Chen, T., Zheng, L., Yuan, J., An, Z., Chen, R., and Tao, Y. (2015). Understanding the control of singlet-triplet splitting for organic exciton manipulating: a combined theoretical and experimental approach. Sci. Rep. 5:10923. doi: 10.1038/srep10923
Chen, X. K., Tsuchiya, Y., Ishikawa, Y., Zhong, C., Adachi, C., and Brédas, J. L. (2017). A new design strategy for efficient thermally activated delayed fluorescence organic emitters: from twisted to planar structures. Adv. Mater. 29:1702767. doi: 10.1002/adma.201702767
Einzinger, M., Zhu, T., de Silva, P., Belger, C., Swager, T. M., Van Voorhis, T., et al. (2017). Shorter exciton lifetimes via an external heavy-atom effect: alleviating the effects of bimolecular processes in organic light-emitting diodes. Adv. Mater. 29:1701987. doi: 10.1002/adma.201701987
Etherington, M. K., Gibson, J., Higginbotham, H. F., Penfold, T. J., and Monkman, A. P. (2016). Revealing the spin–vibronic coupling mechanism of thermally activated delayed fluorescence. Nat. Commun. 7:13680. doi: 10.1038/ncomms13680
Gan, L., Xu, Z., Wang, Z., Li, B., Li, W., Cai, X., et al. (2019). Utilizing a spiro TADF moiety as a functional electron donor in TADF molecular design toward efficient “multichannel” reverse intersystem crossing. Adv. Funct. Mater. 29:1808088. doi: 10.1002/adfm.201808088
Guo, J., Li, X., Nie, H., Luo, W., Gan, S., Hu, S., et al. (2017). Achieving high-performance nondoped OLEDs with extremely small efficiency roll-off by combining aggregation-induced emission and thermally activated delayed fluorescence. Adv. Funct. Mater. 27:1606458. doi: 10.1002/adfm.201606458
Guo, J., Zhao, Z., and Tang, B. Z. (2018). Purely organic materials with aggregation-induced delayed fluorescence for efficient nondoped OLEDs. Adv. Opt. Mater. 6:1800264. doi: 10.1002/adom.201800264
Han, C., Zhang, Z., Ding, D., and Xu, H. (2018). Dipole-dipole interaction management for efficient blue thermally activated delayed fluorescence diodes. Chem 4, 2154–2167. doi: 10.1016/j.chempr.2018.06.005
Huang, J., Nie, H., Zeng, J., Zhuang, Z., Gan, S., Cai, Y., et al. (2017). Highly efficient nondoped OLEDs with negligible efficiency roll-off fabricated from aggregation-induced delayed fluorescence luminogens. Angew. Chem. Int. Ed. 56, 12971–12976. doi: 10.1002/anie.201706752
Im, Y., Kim, M., Cho, Y. J., Seo, J., Yook, K. S., and Lee, J. Y. (2017). Molecular design strategy of organic thermally activated delayed fluorescence emitters. Chem. Mater. 29, 1946–1963. doi: 10.1021/acs.chemmater.6b05324
Kotadiya, N. B., Blom, P. W. M., and Wetzelaer, G. A. H. (2019). Efficient and stable single-layer organic light-emitting diodes based on thermally activated delayed fluorescence. Nat. Photon. 13, 765–769. doi: 10.1038/s41566-019-0488-1
Lee, J., Aizawa, N., Numata, M., Adachi, C., and Yasuda, T. (2017). Versatile molecular functionalization for inhibiting concentration quenching of thermally activated delayed fluorescence. Adv. Mater. 29:1604856. doi: 10.1002/adma.201604856
Leitl, M. J., Krylova, V. A., Djurovich, P. I., Thompson, M. E., and Yersin, H. (2014). Phosphorescence versus thermally activated delayed fluorescence controlling singlet–triplet splitting in brightly emitting and sublimable Cu(I) compounds. J. Am. Chem. Soc. 136, 16032–16038. doi: 10.1021/ja508155x
Li, C., Duan, C., Han, C., and Xu, H. (2018). Secondary acceptor optimization for full-exciton radiation: toward sky-blue thermally activated delayed fluorescence diodes with external quantum efficiency of≈30%. Adv. Mater. 30:1804228. doi: 10.1002/adma.201804228
Liu, J., Zhou, K., Wang, D., Deng, C., Duan, K., Ai, Q., et al. (2019). Pyrazine-based blue thermally activated delayed fluorescence materials: combine small singlet–triplet splitting with large fluorescence rate. Front. Chem. 7:312. doi: 10.3389/fchem.2019.00312
Mao, D., Wu, W., Ji, S., Chen, C., Hu, F., Kong, D., et al. (2017). Chemiluminescence-guided cancer therapy using a chemiexcited photosensitizer. Chem 3, 991–1007. doi: 10.1016/j.chempr.2017.10.002
Méhes, G., Goushi, K., Potscavage, W. J., and Adachi, C. (2014). Influence of host matrix on thermally-activated delayed fluorescence: effects on emission lifetime, photoluminescence quantum yield, and device performance. Org. Electron. 15, 2027–2037. doi: 10.1016/j.orgel.2014.05.027
Park, I. S., Lee, S. Y., Adachi, C., and Yasuda, T. (2016). Full-color delayed fluorescence materials based on wedge-shaped phthalonitriles and dicyanopyrazines: systematic design, tunable photophysical properties, and oled performance. Adv. Funct. Mater. 26, 1813–1821. doi: 10.1002/adfm.201505106
Pershin, A., Hall, D., Lemaur, V., Sancho-Garcia, J., Muccioli, L., Zysman-Colman, E., et al. (2019). Highly emissive excitons with reduced exchange energy in thermally activated delayed fluorescent molecules. Nat. Commun. 10:597. doi: 10.1038/s41467-019-08495-5
Tao, Y., Yuan, K., Chen, T., Xu, P., Li, H., Chen, R., et al. (2014). Thermally activated delayed fluorescence materials towards the breakthrough of organoelectronics. Adv. Mater. 26, 7931–7958. doi: 10.1002/adma.201402532
Tsujimoto, H., Ha, D., Markopoulos, G., Chae, H. S., Baldo, M. A., and Swager, T. M. (2017). Thermally activated delayed fluorescence and aggregation induced emission with through-space charge transfer. J. Am. Chem. Soc. 139, 4894–4900. doi: 10.1021/jacs.7b00873
Uoyama, H., Goushi, K., Shizu, K., Nomura, H., and Adachi, C. (2012). Highly efficient organic light-emitting diodes from delayed fluorescence. Nature 492, 234–238. doi: 10.1038/nature11687
Wang, K., Zheng, C., Liu, W., Liang, K., Shi, Y., Tao, S., et al. (2017). Avoiding energy loss on TADF emitters: controlling the dual conformations of D-A structure molecules based on the pseudoplanar segments. Adv. Mater. 29:1701476. doi: 10.1002/adma.201701476
Wei, X., Chen, Y., Duan, R., Liu, J., Wang, R., Liu, Y., et al. (2017). Triplet decay-induced negative temperature dependence of the transient photoluminescence decay of thermally activated delayed fluorescence emitter. J. Mater. Chem. C. 5, 12077–12084. doi: 10.1039/C7TC04025C
Wei, X., Liu, Y., Hu, T., Li, Z., Liu, J., Wang, R., et al. (2019). Design of efficient exciplex emitters by decreasing the energy gap between the local excited triplet (3LE) state of the acceptor and the charge transfer (CT) states of the exciplex. Front. Chem. 7:188. doi: 10.3389/fchem.2019.00188
Wong, M. Y., and Zysman-Colman, E. (2017). Purely organic thermally activated delayed fluorescence materials for organic light-emitting diodes. Adv. Mater. 29:1605444. doi: 10.1002/adma.201605444
Yang, J., Ren, Z., Xie, Z., Liu, Y., Wang, C., Xie, Y., et al. (2017). AIEgen with fluorescence-phosphorescence dual mechanoluminescence at room temperature. Angew. Chem. Int. Ed. 56, 880–884. doi: 10.1002/anie.201610453
Yang, Z., Mao, Z., Xie, Z., Zhang, Y., Liu, S., and Zhao, J. (2017). Recent advances in organic thermally activated delayed fluorescence materials. Chem. Soc. Rev. 46, 915–1016. doi: 10.1039/c6cs00368k
Zeng, W., Lai, H., Lee, W., Jiao, M., Shiu, Y., Zhong, C., et al. (2018). Achieving nearly 30% external quantum efficiency for orange-red organic light emitting diodes by employing thermally activated delayed fluorescence emitters composed of 1,8-naphthalimide-acridine hybrids. Adv. Mater. 30:1704961. doi: 10.1002/adma.201704961
Zhang, Y., Li, Z., Li, C., and Wang, Y. (2019). Suppressing efficiency roll-off of TADF based OLEDs by constructing emitting layer with dual delayed fluorescence. Front. Chem. 7:302. doi: 10.3389/fchem.2019.00302
Zhang, Y. L., Ran, Q., Wang, Q., Liu, Y., Hänisch, C., Reineke, S., et al. (2019). High-efficiency red organic light-emitting diodes with external quantum efficiency close to 30% based on a novel thermally activated delayed fluorescence emitter. Adv. Mater. 31:1902368. doi: 10.1002/adma.201902368
Keywords: thermally activated delayed fluorescence, asymmetric structure, aggregation-induced emission, charge-transfer, electroluminescence
Citation: Li H, Zhi Y, Dai Y, Jiang Y, Yang Q, Li M, Li P, Tao Y, Li H, Huang W and Chen R (2020) Asymmetric Thermally Activated Delayed Fluorescence Materials With Aggregation-Induced Emission for High-Efficiency Organic Light-Emitting Diodes. Front. Chem. 8:49. doi: 10.3389/fchem.2020.00049
Received: 13 December 2019; Accepted: 15 January 2020;
Published: 26 February 2020.
Edited by:
Guohua Xie, Wuhan University, ChinaReviewed by:
Kai Wang, Soochow University, ChinaShiyang Shao, Changchun Institute of Applied Chemistry (CAS), China
Copyright © 2020 Li, Zhi, Dai, Jiang, Yang, Li, Li, Tao, Li, Huang and Chen. This is an open-access article distributed under the terms of the Creative Commons Attribution License (CC BY). The use, distribution or reproduction in other forums is permitted, provided the original author(s) and the copyright owner(s) are credited and that the original publication in this journal is cited, in accordance with accepted academic practice. No use, distribution or reproduction is permitted which does not comply with these terms.
*Correspondence: Ye Tao, iamytao@njupt.edu.cn; Runfeng Chen, iamrfchen@njupt.edu.cn
†These authors have contributed equally to this work