- 1School of Construction and Environment Engineering, Shenzhen Polytechnic, Shenzhen, China
- 2School of Environment & Natural Resource, Renmin University of China, Beijing, China
- 3Department of Military Installations, Army Logistics University of PLA, Chongqing, China
An efficient Fenton-like catalyst CuO/CeO2 was synthesized using ultrasonic impregnation and used to remove diclofenac from water. The catalyst was characterized by N2 adsorption-desorption, SEM-EDS, XRD, HRTEM, Raman, and XPS analyses. Results showed that CuO/CeO2 possessed large surface area, high porosity, and fine elements dispersion. Cu was loaded in CeO2, which increased the oxygen vacancies. The exposed crystal face of CeO2 (200) was beneficial to the catalytic activity. The diclofenac removal experiment showed that there was a synergistic effect between CuO and CeO2, which might be caused by more oxygen vacancies generation and electronic interactions between Cu and Ce species. The experimental conditions were optimized, including pH, catalyst and H2O2 dosages, and 86.62% diclofenac removal was achieved. Diclofenac oxidation by ·OH and adsorbed oxygen species was the main mechanism for its removal in this Fenton-like system.
Highlights
- CuO/CeO2 was prepared with ultrasound to remove diclofenac in Fenton-like system.
- Ultrasound made CuO/CeO2 had large surface area, high porosity and fine elements dispersion.
- More oxygen vacancies caused by Cu doping were in favor of the catalytic reaction.
- 86.62% diclofenac removal was achieved under the optimal conditions.
- ·OH and adsorbed oxygen species were responsible for diclofenac degradation.
Introduction
Advanced oxidation processes (AOPs) for wastewater treatment have attracted extensive attention due to the quick and efficient pollutant removal by strong oxidizing free radicals, like hydroxyl radical (Silveira et al., 2015; Zhu et al., 2019). The most widely used AOP is Fenton reaction (Fe2+ activate H2O2), which is a homogeneous reaction that requires low pH (2.0–4.0) and forms large amount of iron sludge (Blanco et al., 2016). To overcome these disadvantages, heterogeneous catalysts have been adopted as the alternative (Lei et al., 2015), forming heterogeneous Fenton-like systems (Nidheesh, 2015).
The frequently used catalysts in heterogeneous Fenton-like systems are transition metal-based catalysts (Anipsitakis and Dionysiou, 2004; Bokare and Choi, 2014), due to the good catalytic activity, low cost, easily available materials, and abundant reserves. Among them, catalysts containing Cu2+ are in the limelight (Gu et al., 2019), because of the similar redox properties of Cu2+/Cu+ to Fe3+/Fe2+, broad pH workable range, few sludge production and easily decomposition of Cu2+ complexes by ·OH (Bokare and Choi, 2014; Peng et al., 2019). As one of the most common and simplest copper compounds, CuO has successfully activated H2O2 to form ·OH to remove pollutants (Sehati and Entezari, 2017). However, CuO nanoparticles tend to agglomerate in water which is unfavorable for catalytic reaction. Besides, the leached Cu ions are poisonous. Loading CuO on support is an effective way to overcome the above problems.
Some metal oxides (such as CeO2, Al2O3), clays, zeolites and carbon materials have been used as supports (Rao et al., 2018; Xu et al., 2018). The support increases the specific surface area of catalyst and reduces the leaching of metal ions (Du et al., 2016), which are beneficial to the adsorption property and stability of catalyst. Especially, CeO2 can enhance the catalytic property of catalyst for its unique structure and redox property. CeO2 has abundant oxygen vacancy defects and Ce4+/Ce3+ redox couple (Chong et al., 2016), so it has high oxygen storage capacity, which is beneficial for catalytic reaction.
Doping transition metal ion into CeO2 can create more oxygen vacancies due to the different ionic radii between Ce ion and transition metal ion, and the number of oxygen vacancies depends on the ionic radius and electrons of transition metal ions (Raj et al., 2017). Thus, the catalytic activity is improved by enhancing the oxygen storage and redox capacity (Parvas et al., 2014). The catalytic performance improvement by Cu doped into CeO2 has been reported before (Wang et al., 2006; Yang et al., 2009; Lin et al., 2011).
The traditional impregnation method takes a long doping time and metal species agglomerate easily, due to the small mass transfer force between CeO2 and the doping ions (Wang et al., 2006; Yang et al., 2009; Lin et al., 2011). Ultrasound has been used in the impregnation stage to overcome these disadvantages. Ultrasound waves induce the cavitation effect in water, which is related to the formation, growth, and rapid collapse of cavitation bubbles (Mirtamizdoust et al., 2017; Zhang et al., 2017). The ultrasonic cavitation can significantly accelerate the mass transfer velocity and provide thermal effects (Zhu and Zhang, 2008; Li et al., 2018). Therefore, ultrasound impregnation increases active components (transition metal ions) doped onto the surface of support (like CeO2). Meanwhile, some active components may be introduced into the structure of support under the power of ultrasound, thus the catalyst prepared by ultrasound impregnation would have better catalytic performance (Chong et al., 2016). Moreover, ultrasound can significantly shorten the preparation time of catalyst by providing faster mass transfer rate.
In this paper, CuO was doped into CeO2 by ultrasonic impregnation to form CuO/CeO2, which was then applied as a catalyst in Fenton-like process. Diclofenac, a typical pharmaceutical and emerging contaminant, was used as the target pollutant to check the activity of the catalyst. The catalyst was characterized by N2 adsorption-desorption, scanning electron microscope (SEM), X-ray powder diffraction (XRD), X-ray photoelectron spectra (XPS), high resolution transmission electron microscope (HRTEM), and Raman analyses. Influences of experimental parameters including pH, catalyst dosage, and H2O2 dosage on diclofenac removal were investigated. The potential reaction mechanism was proposed. The objective was to obtain a highly active Fenton-like catalyst with facial synthesis.
Materials and Methods
Materials
All chemicals used were analytical grade. Cu(NO3)2·4H2O and Ce(NO3)3·6H2O were bought from Tianjin Guangfu Fine Chemical Co. (China). Thirty percent of H2O2 was obtained from Beijing Chemical (China). Diclofenac (98%) was bought from Tokyo Chemical (Japan). HPLC grade acetonitrile was purchased from Fisher Scientific (USA).
Synthesis of Catalysts
CuO was prepared by precipitation method (Ghasemi and Karimipour, 2018). One hundred and fifty milliliter NaOH solution (16.67 mol/L) was slowly injected into 150 mL of Cu(NO3)2 solution (0.67 mol/L). The mixture was stirred at ~1,000 rpm at a constant temperature of 80°C for 3 h. The precipitate was separated by centrifugation, in which the solution was centrifuged for 20 min at 3,500 rpm. The precursor of CuO was dried for 3 h at 105°C and then calcined for 3 h at 700°C. The CuO powder was obtained after grinding.
CeO2 was prepared by precipitation method (Zhao et al., 2014) and CuO/CeO2 was prepared by ultrasonic impregnation method. As such, 2 g prepared CeO2 powder was added to 20 mL 1 mol/L Cu(NO3)2 solution and impregnated for 30 min under 0.5 W/cm3 ultrasound, and then the filtered solid particulars were calcined for 2 h at 450°C in muffle furnace to obtain CuO/CeO2.
Characterization of CuO/CeO2
The porosity and specific surface area of CuO/CeO2 were characterized by N2 adsorption-desorption test using 3H-2000PS2, BeiShiDe Instrument-S&T Company. CuO/CeO2 morphology was recorded by SEM with energy dispersive spectroscopy (EDS) (Hitachi S 4700 SEM analyzer). The XRD patterns were carried out by Rigaku D/maxrc diffractometer. The HRTEM measurement was investigated by Thermo Scientific Dionex UltiMate 3000 instrument. The oxidation state of CuO/CeO2 was characterized by XPS analysis (EScalab250Xi spectrometer), and the binding energies were calibrated by C 1s peak at 284.8 eV.
Diclofenac Removal Experiments
The operation processes of reactions were as follows: 50 mL diclofenac solution (20 mg/L) was added to a 150 mL beaker and adjusted to the desired pH using 1 mol/L NaOH and H2SO4 solutions. After that, certain dosages of CuO/CeO2 and H2O2 were poured into the solution under continuous magnetic stirring. Finally, a small sample of the mixture was taken out by syringe at certain times and filtered by a 0.45 μm membrane filter. The filtered solution was measured by HPLC. The experiments were done in triplicate.
The diclofenac concentration in the experiment was analyzed by Thermo Scientific Dionex UltiMate 3000 liquid chromatographt with a C18 column. The operation parameters were as follows: the flowing phases were 70% acetonitrile and 30% acetic acid solution (volume fraction was 0.2%), the flow rate was 1 mL/min, the injection volume was 10 μL, and the detection wavelength was 275 nm. The leached metal ions were detected by inductively coupled plasma optical emission spectrometer (ICP-OES) (IRIS Intrepid II XSP, ThermoFisher).
Results and Discussion
Catalyst Characterization
N2 Adsorption-Desorption Analysis
Figure 1 shows the N2 adsorption-desorption isotherm of CuO-CeO2, which was typical type-IV isotherm with a H1-type hysteresis loop. This implied that mesoporous structure existed in the obtained CuO/CeO2. The pore diameter distribution of CuO/CeO2 was calculated from the adsorption branch and suggested the existence of mesopores at ~10 nm with the average diameter 3.9 nm (the embedded diagram of Figure 1). Table 1 summarized the basic structural parameters of CuO, CeO2, and CuO/CeO2. The specific surface area of CuO/CeO2 reached 114.8 m2/g which was higher than that of CuO and CeO2, and was also much higher than that of other CuO-containing catalysts such as CuO/rGO (56.1 m2/g) (Du et al., 2019) and CuO/Ti6O13 (6.93 m2/g) (Sehati and Entezari, 2017). The high specific surface area allowed the rapid electron transfer (Prathap et al., 2012) and would benefit the catalytic performance of CuO/CeO2. These results indicated that ultrasonic preparation might facilitate catalytic reaction by changing the surface structure of the catalyst. For one thing, the mechanical effect of ultrasound could cut CeO2 into smaller particles increasing specific surface area. Furthermore, the ultrasonic cavitation effect made CuO more uniformly distributed on the surface of CeO2. The decreases of pore volume and pore diameter after ultrasonic impregnation of CeO2 (Table 1) exactly indicated that CuO was indeed loaded on CeO2.
SEM Analysis
SEM was used to characterize the morphology of CuO/CeO2, and the results were shown in Figure 2. Figure 2A shows an overview of CuO/CeO2 in which the size of particles was uniform. CuO was finely loaded on CeO2 (Figure 2B). Element mapping results of Cu, Ce, and O (Figures 2D–F) indicated that the three elements were all well-dispersed in the catalyst. To investigate how ultrasound affected the structure of catalyst, Mahdiani et al. (2018) prepared PbFe12O19 with and without ultrasound, and found that ultrasound could decompress the large structures and form fine and homogeneous structures. Thus, the fine dispersion of elements and uniform size of CuO/CeO2 benefitted from the cavitation effect of ultrasound which created an intense environment in the reaction mixture (Shende et al., 2018). Moreover, Hočevar et al. (2000) showed that the high dispersion of Cu on CeO2 had positive effect on the activity and selectivity of copper cerium oxide. The Cu, Ce, and O atomic percentages confirmed by EDS were 10.56, 18.07, and 71.37%, respectively. The lower content of Cu showed that the dispersion of Cu was relatively sparse.
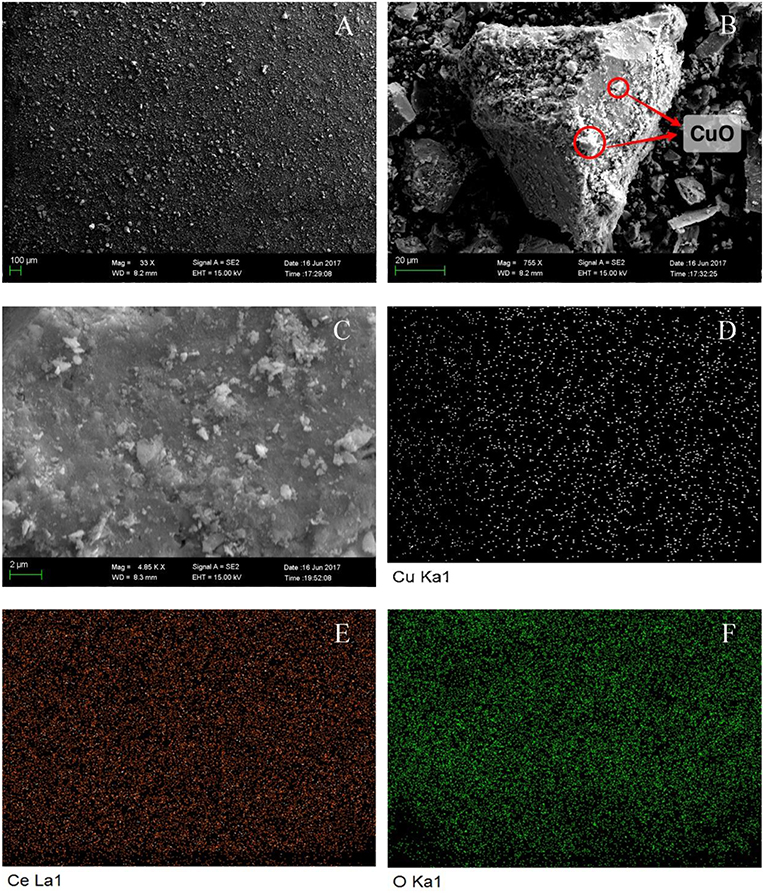
Figure 2. SEM analysis of CuO/CeO2: (A) general view, (B) detailed image, (C) mapping region, (D) Cu mapping image, (E) Ce mapping image, and (F) O mapping image.
XRD Analysis
XRD analysis was applied to identify the component and crystallography of the catalyst. The results were shown in Figure 3. The diffraction peaks at 28.46°, 32.9°, 47.44°, 56.24°, 76.260°, and 79.22° could be attributed to the cubic fluorite CeO2 (JCPDS No. 48-1548), which are characteristics of the (111), (200), (220), (311), (400), and (331) crystal faces. There were also characteristic diffraction peaks owing to CuO crystal, and 35.46°, 38.66° were assigned to the (002) and (111) faces (JCPDS No. 04-0593). The characteristic diffraction peaks of CeO2 in CuO/CeO2 were slightly broadened compared with that in CeO2. This might be associated with the incorporation of Cu2+ ions with a smaller ionic radius (0.73 Å) compared with Ce4+ (0.97 Å) (Cau et al., 2014). These phenomena indicated that lattice constriction occurred in CuO/CeO2.
TEM and HRTEM Analyses
The TEM images of CuO/CeO2 and CeO2 were shown in Figures 4A,C. The particle size of CuO/CeO2 was uniform, and was generally smaller than that of CeO2, which was a favorable result of ultrasonic impregnation method and was good for catalytic reaction. The lattice fringes of CuO/CeO2 were measured at 2.77 and 3.14 Å (Figure 4B), corresponding to (200) and (111) crystal faces. Compared with the two crystal faces of CeO2 (Figure 4D), the lattice fringe spacing in CuO/CeO2 had a slight increase. This phenomenon might be caused by some Cu atoms doped into CeO2 structure. CuO/CeO2 had lattice constriction and generated a solid solution structure. The XRD analysis showed similar results.
The crystal face (111) of CeO2 was the most stable crystal face in CeO2 for its minimal surface energy. Thus, the stability of the catalyst would be enhanced with the increase of exposed crystal face (111). The catalytic activity could be improved by the crystal face (200) for its high surface energy. Moreover, oxygen vacancies were more favored to form on unstable face (200) than on (111). Thus, exposed (200) and (111) faces were beneficial to the catalytic performance and stability of CuO/CeO2.
Raman Analysis
To investigate the effect of CuO doping on the quantities of oxygen vacancies over CuO/CeO2, CuO/CeO2, and CeO2 were both characterized by Raman spectra. As shown in Figure 5, a strong peak at 462 cm−1 was observed, which was attributed to the vibration mode of the F2 g symmetry in cubic fluorite CeO2 lattice. This peak of CuO/CeO2 presented a red shift compared to pure CeO2 because of the reduction in the lattice parameter as a result of shorter M-O bond length (Cau et al., 2014). The weak band at 1,172 cm−1 was assigned to the second-order phonon mode of fluorite structure. The band at 593 cm−1 was attributed to oxygen vacancies in CeO2. With Cu doping into CeO2, more oxygen vacancies were generated, which would promote the catalytic property of CuO/CeO2.
XPS Analysis
The valence states of Cu, Ce, and O in CuO/CeO2 were investigated by XPS analysis. Figure 6A shows the XPS spectrum of Cu 2p of CuO/CeO2. The peak at 935.09 eV was the main peak of Cu 2p3/2, which was related to CuO (Jawad et al., 2018). CuO had a satellite peak accompanied by the main peak, which was approximately 9 eV higher binding energy than the main peak (Zeng et al., 2013). Thus, 944.27 eV was the satellite peak of CuO.
The XPS spectrum of Ce 3d is shown in Figure 6B. The four main 3d5/2 features appeared at 883.23, 885.2, 889.68, and 899.19 eV, while the 3d3/2 features appeared at 901.68, 903.38, 908.39, and 917.64 eV. Among them, the peaks at 883.23 and 901.68 eV were assigned to characteristic of Ce3+, indicating that there were two valence states of Ce (+3 and +4) existed in CuO/CeO2.
The O 1s XPS spectrum was shown in Figure 6C. O 1s existed in two groups, O2− and OH−, which were fitted with peaks at 529.89 and 532.12 eV, respectively. O2− groups represented the lattice oxygen (Olatt) in metal oxides, generating from CuO and CeO2. The peak at 532.12 eV was the characteristic of adsorbed oxygen species or surface OH species. The chemical adsorbed oxygen (Oads) on the surface of CuO/CeO2 might be transformed from Olatt through oxygen vacancies, which was a reactive oxygen specie to attack organics (Chong et al., 2017). This kind of conversion demonstrated that Olatt species participated in the degradation process of diclofenac, which was consistent with the study by Sedmak et al. (2003). Furthermore, XPS results indicated that CuO doped in CeO2, which could improve the formation of Olatt in CuO/CeO2 (Zou et al., 2011).
Diclofenac Removal in CuO/CeO2-H2O2 Fenton-Like System
The comparison of diclofenac removal efficiency by H2O2, CuO/CeO2, CuO-H2O2, CeO2-H2O2, and CuO/CeO2-H2O2 was shown in Figure 7. Single H2O2 and single CuO/CeO2 could hardly remove diclofenac, the same for CuO-H2O2 and CeO2-H2O2 system. However, CuO/CeO2 could effectively catalyze H2O2 to remove diclofenac. The highest removal efficiency was 81.05, 30.01, and 19.67% for CuO/CeO2-H2O2, CuO-H2O2 and CeO2-H2O2 processes, respectively. Compared with CuO-H2O2 and CeO2-H2O2 systems, diclofenac removal efficiency after 60 min in CuO/CeO2-H2O2 system was increased by 63.99 and 65.05%, respectively.
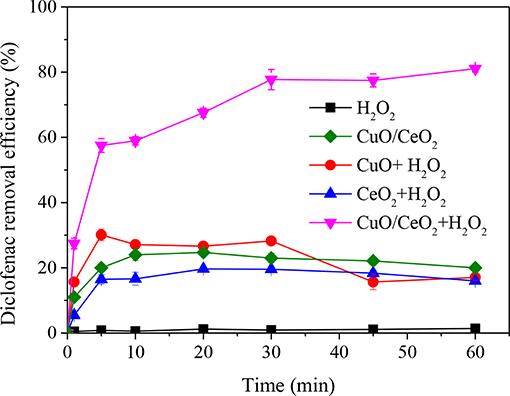
Figure 7. Removal efficiency of diclofenac in different systems. Reaction conditions: pH = 5, H2O2 = 200 mg/L, catalyst = 1 g/L.
Clearly, CuO/CeO2-H2O2 system had higher diclofenac removal than the sum of CuO-H2O2 system and CeO2-H2O2 system, showing a synergistic effect between CuO and CeO2. The synergistic effect might be caused by two reasons. Firstly, more oxygen vacancies were formed for Cu doped in CeO2, which was shown in the above characterization analyses. Lu et al. (2011) found that the formation energy of oxygen vacancy in Cu-doped ceria was lower than bare ceria, demonstrating that Cu dopant could serve as a seed for the formation of oxygen vacancies. Secondly, there were electronic interactions between metal and the support, i.e., the facilitation of redox interactions between Cu2+/Cu+ and Ce4+/Ce3+ redox couples, which would favor the CuO reduction (Konsolakis, 2016). Studies (Szabová et al., 2010, 2013) also reported that Cu doped on CeO2 surface accompanied electron transfer process between Cu and neighboring Ce4+, generating more Ce3+.
Optimization of Operation Parameters for Diclofenac Removal
pH is an important parameter in AOPs. An advantage of Fenton-like process over Fenton reaction is avoiding of too acidic condition (pH < 3.5). As known, diclofenac was more likely to dissociate to ionic structure when the pH was higher than its pKa (4.2), which was favorable to its adsorption and degradation. Thus, the effect of pH above 5 was investigated. As shown in Figure 8A, pH had a significant effect on diclofenac removal in CuO/CeO2-H2O2 system, which decreased by 79.31% when the pH changed from 5 to 11. The result might attribute to two reasons: (1) the oxidation potential of ·OH decreased, and more H2O2 decomposed to O2 and H2O at high pH value; (2) deprotonation of the catalyst gradually increased, which was unfavorable to the electrostatic attraction between diclofenac and CuO/CeO2 (Hassani et al., 2018).
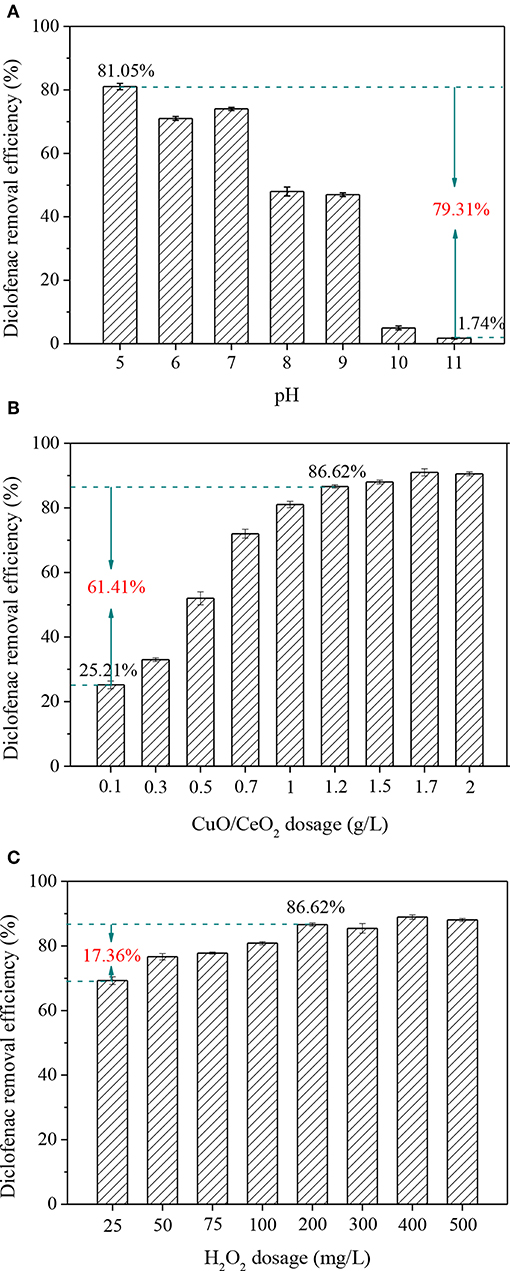
Figure 8. Influencing factors of diclofenac removal in CuO/CeO2-H2O2 system: (A) pH, (B) CuO/CeO2 dosage, (C) H2O2 dosage. Reaction conditions: (A) H2O2 = 200 mg/L, catalyst = 1 g/L; (B) pH = 5, H2O2 = 200 mg/L; (C) pH = 5, catalyst = 1.2 g/L.
Different dosages of CuO/CeO2 were added to the Fenton-like system. Figure 8B shows that the diclofenac removal efficiency was improved from 25.21 to 86.62% when the catalyst dosage increased from 0.1 to 1.2 g/L. The active sites were mostly on the surface of the catalyst, so more catalyst meant that more active sites exposed. But when the active sites were adequate in CuO/CeO2-H2O2 system, further addition of catalyst would bring little improvement. Thus, the optimum CuO/CeO2 dosage was 1.2 g/L.
The effect of H2O2 on diclofenac removal was studied within the dosage of 25–500 mg/L. Figure 8C shows that with the continuous increase of H2O2 dosage, the removal efficiency of diclofenac reached 86.62% at 200 mg/L H2O2, then the reaction tended to stabilize. Excessive H2O2 reacted with ·OH, thus go against the diclofenac removal (Hassani et al., 2018). Taking into account the removal efficiency and cost, 200 mg/L was considered as the optimum dosage of H2O2.
Lee et al. (2014) found only about 24% diclofenac could be removed in 60 min by Cu(II)/H2O2 system. Xu et al. (2016) used Cu-doped α-FeOOH as Fenton-like catalyst to degrade diclofenac, <50% diclofenac was removed in 60 min. Zhou et al. (2018) found 80% diclofenac was removed in a magnetic field enhanced Fe0/EDTA Fenton-like system. In comparsion, CuO/CeO2 was a high-efficiency Fenton-like catalyst for diclofenac removal. Moreover, leaching test showed that there was almost no Ce leached (<0.05 mg/L) under the above optimal reaction conditions. The leached Cu concentration in the solution was 1.7 mg/L, which met the wastewater discharge standard of China (Cu < 2 mg L−1) (Wang et al., 2016) and was only 9‰ of the catalyst dosage. Besides, the removal of diclofenac still reached 70.12% in the third run of CuO/CeO2-H2O2 system. These results showed a good stability and reusability of the CuO/CeO2 catalyst.
Potential Mechanism of Diclofenac Degradation in CuO/CeO2-H2O2 System
Potential mechanism of diclofenac degradation in CuO/CeO2-H2O2 system was proposed in Figure 9. CuO could decompose H2O2 to form highly active ·OH (Drijvers et al., 1999), and the reaction was represented by Equations (1) and (2) (Yamaguchi et al., 2018). ·OH radical could also be produced by Ce3+ with H2O2, which was achieved by the division of O-O bond of H2O2, as shown in Equation (3) (Chong et al., 2017). Moreover, the synergistic copper and ceria interaction (Equation 4) facilitated the redox cycles of Cu2+/Cu+ and further was conducive to ·OH generation (Konsolakis, 2016). Sehati and Entezari (2017) found that ·OH on the catalyst surface (·OHads) was the major reactive species, while ·OH in the solution had little effect on pollutant removal in Fenton-like system. During diclofenac degradation, diclofenac molecules were adsorbed on the surface of the catalyst, meanwhile, H2O2 was decomposed to ·OH by Cu and Ce species on the catalyst surface. Then, the adsorbed diclofenac molecules reacted with ·OHads and transformed into smaller molecules (intermediate products), H2O or CO2. The process continued until the complete decomposition of diclofenac was achieved (Ziylan et al., 2013).
More oxygen vacancies were generated by Cu doped into CeO2, which was proven by the Raman spectra (Figure 5). The oxygen storage capacity of a ceria-based catalyst benefited from oxygen vacancies (Soler et al., 2016). In the degradation process, the adsorbed O2 on the surface of CuO/CeO2 and bulk Olatt could replenish oxygen vacancies by diffusion from the surface and inner of the catalyst (Balcaen et al., 2010), then transformed to active oxygen (Oads) accompanied by valence state transformation of CeO2 and CuO (Ce3+/Ce4+ and Cu+/Cu2+) (Dong et al., 2019). Oads could also participate in the oxidation of diclofenac (Xian et al., 2019). Besides, large specific surface area and high porosity of ultrasonically prepared CuO/CeO2 catalyst allowed rapid electron transfer, which improved the catalytic property (Prathap et al., 2012).
Conclusions
An efficient heterogeneous Fenton-like catalyst CuO/CeO2 was synthesized by ultrasonic impregnation method and used to remove diclofenac from water. The prepared CuO/CeO2 had large specific surface area, high porosity and fine elements dispersion. CuO and CeO2 crystals coexisted in CuO/CeO2 with a lattice constriction. HRTEM analysis demonstrated that the main exposed crystal faces of CeO2 contained (200) face which readily formed oxygen vacancies and improved the catalytic property of CuO/CeO2. Oxygen vacancies in CeO2 were increased by Cu doping. The optimal operating conditions of CuO/CeO2-H2O2 system were pH = 5, CuO/CeO2 dosage = 1.2 g/L, and H2O2 dosage = 200 mg/L, with 86.62% diclofenac removal. The synergistic effect between CuO and CeO2 on diclofenac removal might be caused by more oxygen vacancies generation and electronic interactions between Cu and Ce species in CuO/CeO2. The degradation of diclofenac was mainly by ·OH and adsorbed oxygen species which were enhanced by oxygen vacancies.
Data Availability Statement
All datasets generated for this study are included in the article/supplementary material.
Author Contributions
GZ, GX, and JZ designed the experiments. GX, NZ, and JL performed the experiments. GZ and GX wrote the paper. GZ, GX, NZ, and JZ discussed the results and analyzed the data.
Conflict of Interest
The authors declare that the research was conducted in the absence of any commercial or financial relationships that could be construed as a potential conflict of interest.
Acknowledgments
The authors thank for the financial support from the National Natural Science Foundation of China (51778374) and National Water Pollution Control and Treatment Science and Technology Major Project of China (2018ZX07110003).
References
Anipsitakis, G. P., and Dionysiou, D. D. (2004). Radical generation by the interaction of transition metals with common oxidants. Environ. Sci. Technol. 38, 3705–3712. doi: 10.1021/es035121o
Balcaen, V., Roelant, R., Poelman, H., Poelman, D., and Marin, G. B. (2010). TAP study on the active oxygen species in the total oxidation of propane over a CuO-CeO2/γ-Al2O3 catalyst. Catal. Today 157, 49–54. doi: 10.1016/j.cattod.2010.02.048
Blanco, L., Hermosilla, D., Merayo, N., and Blanco, Á. (2016). Assessing the use of zero-valent iron microspheres to catalyze Fenton treatment processes. J. Taiwan Inst. Chem. E 69, 54–60. doi: 10.1016/j.jtice.2016.08.014
Bokare, A. D., and Choi, W. (2014). Review of iron-free Fenton-like systems for activating H2O2 in advanced oxidation processes. J. Hazard. Mater. 275, 121–135. doi: 10.1016/j.jhazmat.2014.04.054
Cau, C., Guari, Y., Chave, T., Larionova, J., and Nikitenko, S. I. (2014). Thermal and sonochemical synthesis of porous (Ce,Zr)O2 mixed oxides from metal β-diketonate precursors and their catalytic activity in wet air oxidation process of formic acid. Ultrason. Sonochem. 21, 1366–1373. doi: 10.1016/j.ultsonch.2014.01.006
Chong, S., Zhang, G., Zhang, N., Liu, Y., Huang, T., and Chang, H. (2017). Diclofenac degradation in water by FeCeOx catalyzed H2O2: Influencing factors, mechanism and pathways. J. Hazard. Mater. 334, 150–159. doi: 10.1016/j.jhazmat.2017.04.008
Chong, S., Zhang, G., Zhang, N., Liu, Y., Zhu, J., Huang, T., et al. (2016). Preparation of FeCeOx by ultrasonic impregnation method for heterogeneous Fenton degradation of diclofenac. Ultrason. Sonochem. 32, 231–240. doi: 10.1016/j.ultsonch.2016.03.019
Dong, C.-D., Huang, C. P., Nguyen, T.-B., Hsiung, C.-F., Wu, C.-H., Lin, Y.-L., et al. (2019). The degradation of phthalate esters in marine sediments by persulfate over iron-cerium oxide catalyst. Sci. Total Environ. 696:133973. doi: 10.1016/j.scitotenv.2019.133973
Drijvers, D., Langenhove, H. V., and Beckers, M. (1999). Decomposition of phenol and trichloroethylene by the ultrasound/H2O2/CuO process. Water Res. 33, 1187–1194. doi: 10.1016/S0043-1354(98)00308-X
Du, X., Zhang, Y., Si, F., Yao, C., Du, M., Hussain, I., et al. (2019). Persulfate non-radical activation by nano-CuO for efficient removal of chlorinated organic compounds: reduced graphene oxide-assisted and CuO (001) facet-dependent. Chem. Eng. J. 356, 178–189. doi: 10.1016/j.cej.2018.08.216
Du, Y., Ma, W., Liu, P., Zou, B., and Ma, J. (2016). Magnetic CoFe2O4 nanoparticles supported on titanate nanotubes (CoFe2O4/TNTs) as a novel heterogeneous catalyst for peroxymonosulfate activation and degradation of organic pollutants. J. Hazard. Mater. 308, 58–66. doi: 10.1016/j.jhazmat.2016.01.035
Ghasemi, S., and Karimipour, A. (2018). Experimental investigation of the effects of temperature and mass fraction on the dynamic viscosity of CuO-paraffin nanofluid. Appl. Therm. Eng. 128, 189–197. doi: 10.1016/j.applthermaleng.2017.09.021
Gu, T., Dong, H., Lu, T., Han, L., and Zhan, Y. (2019). Fluoride ion accelerating degradation of organic pollutants by Cu(II)-catalyzed Fenton-like reaction at wide pH range. J. Hazard. Mater. 377, 365–370. doi: 10.1016/j.jhazmat.2019.05.073
Hassani, A., Celikdag, G., Eghbali, P., Sevim, M., Karaca, S., and Metin, O. (2018). Heterogeneous sono-Fenton-like process using magnetic cobalt ferrite-reduced graphene oxide (CoFe2O4-rGO) nanocomposite for the removal of organic dyes from aqueous solution. Ultrason. Sonochem. 40, 841–852. doi: 10.1016/j.ultsonch.2017.08.026
Hočevar, S., Krašovec, U. O., Orel, B., Aricó, A. S., and Kim, H. (2000). CWO of phenol on two differently prepared CuO-CeO2 catalysts. Appl. Catal. B Environ. 28, 113–125. doi: 10.1016/S0926-3373(00)00167-3
Jawad, A., Lang, J., Liao, Z., Khan, A., Ifthikar, J., Lv, Z., et al. (2018). Activation of persulfate by CuOx@Co-LDH: A novel heterogeneous system for contaminant degradation with broad pH window and controlled leaching. Chem. Eng. J. 335, 548–559. doi: 10.1016/j.cej.2017.10.097
Konsolakis, M. (2016). The role of Copper-Ceria interactions in catalysis science: Recent theoretical and experimental advances. Appl. Catal. B Environ. 198, 49–66. doi: 10.1016/j.apcatb.2016.05.037
Lee, H.-J., Lee, H., and Lee, C. (2014). Degradation of diclofenac and carbamazepine by the copper(II)-catalyzed dark and photo-assisted Fenton-like systems. Chem. Eng. J. 245, 258–264. doi: 10.1016/j.cej.2014.02.037
Lei, Y., Chen, C. S., Tu, Y. J., Huang, Y. H., and Zhang, H. (2015). Heterogeneous degradation of organic pollutants by persulfate activated by CuO-Fe3O4: mechanism, stability, and effects of pH and bicarbonate ions. Environ. Sci. Technol. 49, 6838–6845. doi: 10.1021/acs.est.5b00623
Li, J. X., Qin, Z. B., Li, Y. H., and Cui, G. H. (2018). Sonochemical synthesis and properties of two new nanostructured silver(I) coordination polymers. Ultrason. Sonochem. 48, 127–135. doi: 10.1016/j.ultsonch.2018.05.016
Lin, K.-S., Chowdhury, S., Yeh, H.-P., Hong, W.-T., and Yeh, C.-T. (2011). Preparation and characterization of CuO/ZnO–Al2O3 catalyst washcoats with CeO2 sols for autothermal reforming of methanol in a microreactor. Catal. Today 164, 251–256. doi: 10.1016/j.cattod.2010.11.038
Lu, Z., Yang, Z., He, B., Castleton, C., and Hermansson, K. (2011). Cu-doped ceria: oxygen vacancy formation made easy. Chem. Phys. Lett. 510, 60–66. doi: 10.1016/j.cplett.2011.03.091
Mahdiani, M., Soofivand, F., and Salavati-Niasari, M. (2018). Investigation of experimental and instrumental parameters on properties of PbFe12O19 nanostructures prepared by sonochemical method. Ultrason. Sonochem. 40, 271–281. doi: 10.1016/j.ultsonch.2017.06.023
Mirtamizdoust, B., Travnicek, Z., Hanifehpour, Y., Talemi, P., Hammud, H., and Joo, S. W. (2017). Synthesis and characterization of nano-peanuts of lead(II) coordination polymer [Pb(qcnh)(NO3)2]n with ultrasonic assistance: a new precursor for the preparation of pure-phase nano-sized PbO. Ultrason. Sonochem. 34, 255–261. doi: 10.1016/j.ultsonch.2016.05.041
Nidheesh, P. V. (2015). Heterogeneous Fenton catalysts for the abatement of organic pollutants from aqueous solution: a review. RSC Adv. 5, 40552–40577. doi: 10.1039/C5RA02023A
Parvas, M., Haghighi, M., and Allahyari, S. (2014). Degradation of phenol via wet-air oxidation over CuO/CeO2-ZrO2 nanocatalyst synthesized employing ultrasound energy: physicochemical characterization and catalytic performance. Environ. Technol. 35, 1140–1149. doi: 10.1080/09593330.2013.863952
Peng, J., Zhang, C., Zhang, Y., Miao, D., Zhang, Y., Liu, H., et al. (2019). Enhanced Cu(II)-mediated fenton-like oxidation of antimicrobials in bicarbonate aqueous solution: kinetics, mechanism and toxicity evaluation. Environ. Pollut. 252, 1933–1941. doi: 10.1016/j.envpol.2019.05.148
Prathap, M. U., Kaur, B., and Srivastava, R. (2012). Hydrothermal synthesis of CuO micro-/nanostructures and their applications in the oxidative degradation of methylene blue and non-enzymatic sensing of glucose/H2O2. J. Colloid Interf. Sci. 370, 144–154. doi: 10.1016/j.jcis.2011.12.074
Raj, A. K. V., Prabhakar Rao, P., Sreena, T. S., and Aju Thara, T. R. (2017). Influence of local structure on photoluminescence properties of Eu(3+) doped CeO2 red phosphors through induced oxygen vacancies by contrasting rare earth substitutions. Phys. Chem. Chem. Phys. 19, 20110–20120. doi: 10.1039/C7CP02741A
Rao, W., Lv, G., Wang, D., and Liao, L. (2018). Enhanced degradation of Rh 6G by zero valent iron loaded on two typical clay minerals with different structures under microwave irradiation. Front. Chem. 6:463. doi: 10.3389/fchem.2018.00463
Sedmak, G., Hočevar, S., and Levec, J. (2003). Kinetics of selective CO oxidation in excess of H2 over the nanostructured Cu0.1Ce0.9O2-y catalyst. J. Catal. 213, 135–150. doi: 10.1016/S0021-9517(02)00019-2
Sehati, S., and Entezari, M. H. (2017). Sono-incorporation of CuO nanoparticles on the surface and into the mesoporous hexatitanate layers: enhanced Fenton-like activity in degradation of orange-G at its neutral pH. Appl. Surf. Sci. 399, 732–741. doi: 10.1016/j.apsusc.2016.12.079
Shende, T. P., Bhanvase, B. A., Rathod, A. P., Pinjari, D. V., and Sonawane, S. H. (2018). Sonochemical synthesis of Graphene-Ce-TiO2 and Graphene-Fe-TiO2 ternary hybrid photocatalyst nanocomposite and its application in degradation of crystal violet dye. Ultrason. Sonochem. 41, 582–589. doi: 10.1016/j.ultsonch.2017.10.024
Silveira, J. E., Zazo, J. A., Pliego, G., Bidóia, E. D., and Moraes, P. B. (2015). Electrochemical oxidation of landfill leachate in a flow reactor: optimization using response surface methodology. Environ. Sci. Pollut. Res. 22, 5831–5841. doi: 10.1007/s11356-014-3738-2
Soler, L., Casanovas, A., Escudero, C., Perez-Dieste, V., Aneggi, E., Trovarelli, A., et al. (2016). Ambient pressure photoemission spectroscopy reveals the mechanism of carbon soot oxidation in ceria-based catalysts. ChemCatChem 8, 2748–2751. doi: 10.1002/cctc.201600615
Szabová, L., Camellone, M. F., Huang, M., Matolin, V., and Fabris, S. (2010). Thermodynamic, electronic and structural properties of Cu/CeO2 surfaces and interfaces from first-principles DFT+U calculations. J. Chem. Phys. 133:234705. doi: 10.1063/1.3515424
Szabová, L., Skála, T., Matolínová, I., Fabris, S., Farnesi Camellone, M., and Matolín, V. (2013). Copper-ceria interaction: a combined photoemission and DFT study. Appl. Surf. Sci. 267, 12–16. doi: 10.1016/j.apsusc.2012.04.098
Wang, C., Wan, J., Ma, Y., and Wang, Y. (2016). Insights into the synergy of zero-valent iron and copper oxide in persulfate oxidation of Orange G solutions. Res. Chem. Intermed. 42, 481–497. doi: 10.1007/s11164-015-2035-0
Wang, X., Rodriguez, J. A., Hanson, J. C., Gamarra, D., Martínez-Arias, A., and Fernández-García, M. (2006). In situ studies of the active sites for the water gas shift reaction over Cu-CeO2 catalysts: complex interaction between metallic copper and oxygen vacancies of ceria. J. Phys. Chem. B 110, 428–434. doi: 10.1021/jp055467g
Xian, G., Zhang, G., Chang, H., Zhang, Y., Zou, Z., and Li, X. (2019). Heterogeneous activation of persulfate by Co3O4-CeO2 catalyst for diclofenac removal. J. Environ. Manage. 234, 265–272. doi: 10.1016/j.jenvman.2019.01.013
Xu, D., Lv, H., and Liu, B. (2018). Encapsulation of metal nanoparticle catalysts within mesoporous zeolites and their enhanced catalytic performances: a review. Front. Chem. 6:550. doi: 10.3389/fchem.2018.00550
Xu, J., Li, Y., Yuan, B., Shen, C., Fu, M., Cui, H., et al. (2016). Large scale preparation of Cu-doped α-FeOOH nanoflowers and their photo-Fenton-like catalytic degradation of diclofenac sodium. Chem. Eng. J. 291, 174–183. doi: 10.1016/j.cej.2016.01.059
Yamaguchi, R., Kurosu, S., Suzuki, M., and Kawase, Y. (2018). Hydroxyl radical generation by zero-valent iron/Cu (ZVI/Cu) bimetallic catalyst in wastewater treatment: heterogeneous Fenton/Fenton-like reactions by Fenton reagents formed in-situ under oxic conditions. Chem. Eng. J. 334, 1537–1549. doi: 10.1016/j.cej.2017.10.154
Yang, W., Li, D., Xu, D., and Wang, X. (2009). Effect of CeO2 preparation method and Cu loading on CuO/CeO2 catalysts for methane combustion. J. Nat. Gas Chem. 18, 458–466. doi: 10.1016/S1003-9953(08)60141-3
Zeng, S., Zhang, W., Śliwa, M., and Su, H. (2013). Comparative study of CeO2/CuO and CuO/CeO2 catalysts on catalytic performance for preferential CO oxidation. Int. J. Hydrogen Energ. 38, 3597–3605. doi: 10.1016/j.ijhydene.2013.01.030
Zhang, N., Zhao, H., Zhang, G., Chong, S., Liu, Y., Sun, L., et al. (2017). Preparation of a magnetic N-Fe/AC catalyst for aqueous pharmaceutical treatment in heterogeneous sonication system. J. Environ. Manage. 187, 201–211. doi: 10.1016/j.jenvman.2016.11.043
Zhao, H., Zhang, G., and Zhang, Q. (2014). MnO2/CeO2 for catalytic ultrasonic degradation of methyl orange. Ultrason. Sonochem. 21, 991–996. doi: 10.1016/j.ultsonch.2013.12.002
Zhou, T., Feng, K., Xiang, W., Lv, Y., Wu, X., Mao, J., et al. (2018). Rapid decomposition of diclofenac in a magnetic field enhanced zero-valent iron/EDTA Fenton-like system. Chemosphere 193, 968–977. doi: 10.1016/j.chemosphere.2017.11.090
Zhu, L., and Zhang, H. (2008). A novel method for the modification of zinc powder by ultrasonic impregnation in cerium nitrate solution. Ultrason. Sonochem. 15, 393–401. doi: 10.1016/j.ultsonch.2007.09.016
Zhu, Y., Zhu, R., Xi, Y., Zhu, J., Zhu, G., and He, H. (2019). Strategies for enhancing the heterogeneous Fenton catalytic reactivity: a review. Appl. Catal. B Environ. 255, 117739. doi: 10.1016/j.apcatb.2019.05.041
Ziylan, A., Koltypin, Y., Gedanken, A., and Ince, N. H. (2013). More on sonolytic and sonocatalytic decomposition of Diclofenac using zero-valent iron. Ultrason. Sonochem. 20, 580–586. doi: 10.1016/j.ultsonch.2012.05.005
Keywords: CuO/CeO2, ultrasonic impregnation, Fenton-like system, OH, oxygen vacancies
Citation: Zhu J, Zhang G, Xian G, Zhang N and Li J (2019) A High-Efficiency CuO/CeO2 Catalyst for Diclofenac Degradation in Fenton-Like System. Front. Chem. 7:796. doi: 10.3389/fchem.2019.00796
Received: 01 September 2019; Accepted: 05 November 2019;
Published: 19 November 2019.
Edited by:
Renato Falcão Dantas, Campinas State University, BrazilCopyright © 2019 Zhu, Zhang, Xian, Zhang and Li. This is an open-access article distributed under the terms of the Creative Commons Attribution License (CC BY). The use, distribution or reproduction in other forums is permitted, provided the original author(s) and the copyright owner(s) are credited and that the original publication in this journal is cited, in accordance with accepted academic practice. No use, distribution or reproduction is permitted which does not comply with these terms.
*Correspondence: Jia Zhu, 7566874@qq.com
†These authors have contributed equally to this work and share first authorship