- 1The Key Laboratory of Coal to Ethylene Glycol and Its Related Technology, Fujian Institute of Research on the Structure of Matter, Chinese Academy of Sciences, Fuzhou, China
- 2University of Chinese Academy of Sciences, Beijing, China
- 3Institute of Oceanography, Ocean College, Fujian Provincial Key Laboratory of Information Processing and Intelligent Control, Minjiang University, Fuzhou, China
In this work, a novel method for assigning the absolute configuration of a chiral primary amine has been developed based on the experimental and DFT-calculated 19F NMR chemical shift differences of its derived two fluorinated amides by reacting with two enantiomers of a chiral derivatizing agent FPP (α-fluorinated phenylacetic phenylselenoester) separately. Comparing the experimental chemical shift difference of (R)-FPA-amide/(S)-FPA-amide with the calculated Δδα-FR,S of (R)-FPA-(R)-amide/(S)-FPA-(R)-amide, if the experimental Δδα-FR,S has the same symbol (positive or negative) as one of the theoretical Δδα-FR,S, the assigned configuration of the amine is considered to be consistent with the theoretical one. Our method could be applied to a broad substrate scope avoiding wrong conclusion due to empirical judgment.
Introduction
Over the decade, the increased tremendous demand of optically pure organic compounds in many fields, such as drug discovery, medicinal chemistry, and asymmetric synthesis, has promoted the exploration of practical strategies for determining the absolute configuration of a chiral molecule (Bijvoet et al., 1951; Flack and Bernardinelli, 2000; De Gussem et al., 2012; Burtea and Rychnovsky, 2017; Liu et al., 2017; Sairenji et al., 2017; Yan et al., 2017; Ma et al., 2018). Among varied developed technologies for this purpose, NMR spectroscopic detection of the chemical shift differences of NMR signals of formed diastereomers of chiral guests with chiral agents is one of the most used methods, which is simple and convenient, giving straightforward information for analysis without the need of standard samples (Shvo et al., 1967; Jacobus et al., 1968; Ohtani et al., 1991; Takeuchi et al., 1991, 1993, 2004, 2006; Hanna and Lau-Cam, 1993; Trost et al., 1994; Hoye and Renner, 1996; Kirk, 1998; Yabuuchi and Kusumi, 2000; Fujiwara et al., 2001; Seco et al., 2004; Freire et al., 2005, 2008; Orlov and Ananikov, 2010, 2011; Wenzel and Chisholm, 2011; Kumari et al., 2013, 2015; Pal et al., 2014; Bian et al., 2015; Lakshmipriya et al., 2016; Laskowski et al., 2016; Yan et al., 2017; Burns et al., 2018).
Chiral amines have been ubiquitous in natural products and widely used in the field of medicine, so it is very important to determine their absolute configurations. Up to now, the published research concerning the determination of absolute configuration by NMR is mostly based on 1H NMR. It is very rare to use 19F NMR for this purpose and all are based on empirical models. Determining the absolute configuration of amines in 19F NMR was firstly reported by Mosher using triflurophenyl acetic acid as the chiral derivatizing agent (CDA) who empirically assigned the absolute configurations by comparing the 19F NMR experimental chemical shift differences of the formed amides between a chiral amine and two enantiomers of a CDA based on Mosher's models (Dale and Mosher, 1973; Sullivan et al., 1973) as shown in Figure 1A in which Llarge is the large group of amine and Lsmall is the small group of amine by comparing their inherent stereochemistry. Successful assignment heavily depends on correct judgment of inherent stereochemistry of the groups. However, it is difficult to judge the intrinsic size of groups in many cases, which could result in wrong conclusions. In the 1990s, using alpha-fluorinated phenyl acetic acid (FPA) as a CDA, Hamman observed that correctly constructing the correlation between the absolute configuration of amines and chemical shifts of 19F NMR of formed alpha-fluorinated phenyl acetic amide depends on the property of the L1 and L2 groups of amines. If both groups are alkyl or aryl groups, L1 should be the bulkier group. However, if the formed amide having a CO2Me group attached to the chiral alpha carbon, then the L1 group is always a CO2Me group, no matter how large the other group is (Figure 1B) (Hamman, 1990, 1993; Temperini et al., 2017). In addition to the CO2Me group, our group also observed that if another functional group is attached (such as a hydroxyl group or fluorine atom), this group should be the L1 group in order to give correct configurations (Figure 1B). The interactions between the alpha chiral F atom and the functional groups may cause the judgment complexity of the L1 group. These observations indicate that proper judgment of the L1 group is critical for the correct assignment of absolute configuration. Thus, an empirical model based on inherent stereochemistry simply could not be established for correlating the absolute configurations of amines containing functional groups with the chemical shift values of their corresponding alpha-fluorinated phenyl acetic amides. Interestingly, the international union of pure and applied chemistry (IUPAC) rule for the assignment of the L1 group is more appropriate than inherent stereochemistry with the fluorinated phenyl acetic acid derived amides, but such kind of assignment is arbitrary. How does one establish the correlation between the absolute configuration of varied amines and 19F NMR signal of their corresponding alpha-fluorinated phenyl acetic amides excluding arbitrary assignment? Considering that the assignment of absolute configuration of chiral molecules using a circular dichroism spectrometer by comparing the experimental and calculated CD spectra has been well-established (Dickins et al., 1999; Aamouche et al., 2000; Huang et al., 2000; Pescitelli and Bruhn, 2016), we wonder if we can do the same thing with NMR.
Here, we report a novel method for the assignment of absolute configuration of a chiral amine by comparing the experimental and DFT-calculated 19F NMR chemical shift differences of its corresponding (R)-FPA-amide and (S)-FPA-amide derived from the chiral amine by reacting with two enantiomers of a CDA FPP (α-fluorinated phenylacetic phenylselenoester, Table 1) separately. By comparison, the experimental Δδα-FR,S (Δδα-FR,S = Δδα-FR – Δδα-FS, where Δδα-FR and Δδα-FS are 19F-{1H} NMR values of (R)-FPA-amide and (S)-FPA-amide, respectively) has the same symbol (positive or negative) as one of the theoretical Δδα-FR,S; the assigned configuration of the amine is considered to be consistent with the theoretical one (Figure 1C). The advantages of FPP are as follows: it can react with amines directly in NMR tubes to form amides immediately without the addition of other chemical reagents and give very clean solution avoiding any further handling step. Chiral FPP is stable in varied solvents over weeks and can be stored in a sealed bottle covered with foil for months in a refrigerator.
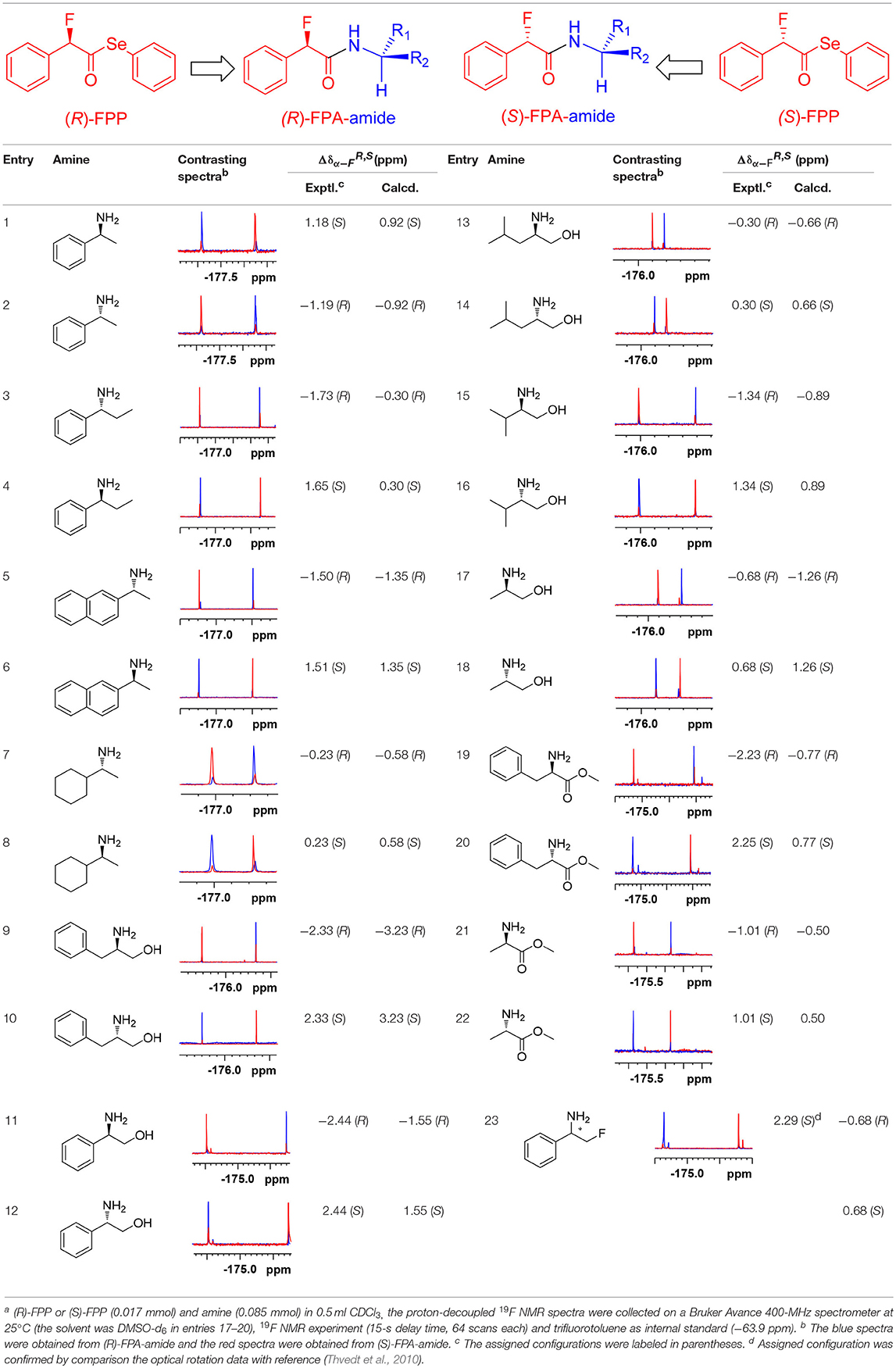
Table 1. Constructing the correlation between the absolute configuration of amines with the experimental measurements and DFT calculations of Δδα-FR,S of their corresponding (R)-FPA-amide and (S)-FPA-amidea.
Materials and Methods
General Information and Materials
All commercial reagents were used as received without further purification unless otherwise stated. All reactions were run under N2 unless otherwise indicated. NMR spectra were recorded using a 400-MHz spectrometer. Chemical shifts were reported in parts per million (ppm), using CDCl3 (δH = 7.26 ppm, δC = 77.16 ppm) and trifluorotoluene (−63.9 ppm) as internal standards. Multiplicities are indicated as s (singlet), d (doublet), t (triplet), q (quartet), and m (multiplet). High-resolution mass spectra (HRMS) were obtained by the ESI ionization sources using the TOF MS technique. The calculated 19F NMR shifts were referenced to trifluorotoluene (σref = 257.58 ppm, δref = −63.9 ppm).
General Synthesis Procedure for Chiral FPP
Chiral FPP was readily prepared by a one-pot procedure reported by Temperini's group (Temperini et al., 2017) using chiral α-fluorinated phenylacetic acid and diphenyl diselenide as the starting materials (the procedure is illustrated in Scheme 1).
To a mixture of (S)-α-fluorobenzeneacetic acid (1.0 g, 6.49 mmol) and N-methylmorpholine (NMM) (722 mg, 7.14 mmol) in 20 ml of dried ethyl acetate, i-BuOCOCl (975 mg, 7.14 mmol) was added dropwise in 30 min under N2 at 0°C. After addition, the mixture was stirred for another 30 min at 0°C. Then, a fresh and white solution of nucleophilic selenium species, prepared by reacting diphenyl diselenide (1.48 g, 3.245 mmol), sodium borohydride (245 mg, 6.49 mmol), and CH3COOH (973.5 mg, 16.225 mmol) in 10 ml of dried ethyl acetate at 40°C for 30 min, was added to the above mixture, and the stirring was continued at room temperature until completion of reaction as detected by TLC. The mixture was quenched by 5 ml of 1 M HCl and extracted by ethyl acetate (20 ml × 2). The organic phase was washed by brine (20 ml), dried over anhydrous sodium sulfate, and concentrated in vacuo. The crude product was purified via flash chromatography on silica gel (petroleum ether/ethyl acetate 10:1 to 5:1), affording the corresponding product (S)-FPP (little yellow oil, total yield up to 69%) (Supplementary Figures S1–S4). [α] = 13.4° (c = 1.0 in CHCl3); 1H NMR (400 MHz, CDCl3) δ 7.54–7.47 (m, 4H), 7.46–7.43 (m, 2H), 7.42–7.37 (m, 4H), 5.81 (d, J = 47.6 Hz, 1H) ppm; 13C NMR (CDCl3, 100 MHz) δ 198.8, 136.2, 135.8, 132.7, 130.1, 129.5, 129.4, 129.0, 128.9, 128.8, 127.9, 126.7, 95.7 (d, J = 189 Hz) ppm; 19F-{1H} NMR (376 MHz, CDCl3) δ −180.8 ppm; HRMS (ESI) Calcd for [C14H11FOSeNa, M+Na]+: 316.9851, found: 316.9849.
Following the same procedure, (R)-FPP was synthesized from (R)-α-fluorobenzeneacetic acid (little yellow oil, total yield up to 67%). [α] = −13.2° (c = 1.0 in CHCl3).
Configurational Assignment of Amines by FPP
FPP [0.017 mmol, (R)-FPP or (S)-FPP] and amine (0.085 mmol) were mixed in 0.5 ml of CDCl3, and the proton-decoupled 19F NMR spectra were collected on a Bruker Avance 400-MHz spectrometer at 25°C (the solvent was DMSO-d6 for guests 17–20) (delay time: 15 s, 64 scans each for 19F-{1H} NMR experiment). The internal standard of fluorine spectrum is 4-trifluorotoluene (−63.9 ppm).
Results and Discussion
First, to examine FPP as in-tube CDA for chiral amines, 1 equiv. of (S)-FPP was added to an NMR tube with 1 equiv. of (S)-α-phenylethanamine in CDCl3 under air at room temperature giving clean solution, and the proton-decoupled 19F(19F-{1H}) NMR spectra were recorded immediately. The corresponding amide was generated within 5 min and the whole derivatizing process was finished within 20 min. Also, the fluorine chemical shift values of the derivatives do not vary with the reaction time and the different ratios of chiral FPP/amine (Supplementary 1), which is crucial to determine the absolute configuration. It is unfortunate that slightly partial racemization happened during the derivatization, which leads to the failure of measuring the enantiomeric purity of chiral amines, but it does not affect the assignment of absolute configuration by using the main enantiomers' signals.
Then, we recorded the 19F-{1H} NMR spectra of a series of amines with known configurations after FPP derivatization (Supplementary Figures S5–S50). The results are listed in Table 1 (entries 1–22). It can be seen that the obtained Δδα-FR,S values are positive for all tested (S)-amines and are negative for all tested (R)-amines, whether the amine is aromatic amine, fatty amine, amino alcohol, or amino acid ester.
How to correlate configurations with Δδα-FR,S reasonably is the key to establishing the method of determining absolute configuration by 19F NMR with FPP. For the amines of entries 1–8, correct configurations could be given by comparing the inherent stereochemistry of groups based on Hamman's model. However, for several amines (Table 1, entries 9–16, 19, and 20), the comparison of the inherent stereochemistry of groups based on Hamman's model gave incorrect assignment of the absolute configuration, which is likely to be caused by the electron effect of the heteroatom (Hamman, 1990, 1993; Apparu et al., 2000). Thus, we need to explore a new strategy to overcome these problems. We propose that δα−F in FPA-amide is mainly influenced by the composite factors of electronic and steric hindrance effects of groups. It is unrealistic to correlate interactions of electronic and steric hindrance effects by a simple empirical model. Nevertheless, in today's highly developed computational chemistry, the combined effects of electronic effect and steric hindrance can be achieved by strict theoretical calculation. By theoretically calculating the chemical shift differences of the amide formed from a chiral amine with two enantiomers of FPP separately and comparing the experiment and calculated values, a new method for assigning the absolute configuration could be well-established.
Our theoretical calculation is as follows. Conformation screening was applied using molecular mechanics methods in our calculations in order to obtain more reliable results. Nine energy favorable conformations of every molecule were selected and geometry optimization was performed based on B3LYP/6-111 G(d,p) level. Vibrational frequency analyses at the same basis sets were used on all optimized structures in order to characterize stationary points as local minima. Then, the Gibbs free energy with zero-point energy (ZPE) corrections was obtained for every conformer. The lowest Gibbs free energy conformers were selected and the 19F NMR parameters for FPA-amines were calculated at the level of B972/cc-PVQZ with the GIAO method. All the calculations were in chloroform solvent and the solvent effects were evaluated by the IEFPCM model. The Gaussian 09 package (Frisch et al., 2010) was used for all of our calculations. All the conformers were local minimum and verified by frequency calculation. Also, two molecules were selected to perform 19F NMR calculation for all conformers according to Boltzmann equations based on free energy data by frequency calculation. It was found that there is a trivial difference in Δδα-FR,S between the data of the most stable conformer and the statistic values (Supplementary 3). Hence, the most stable conformer of each molecule was used to calculate 19F NMR and Δδα-FR,S data. The calculated 19F chemical shifts for the lowest-energy conformers were in agreement with the experimental values (Table 1, entries 1–22). Then, the method is applied to determine the absolute configuration of an amine with unknown configuration (2-fluoro-1-phenylethanamine in Table 1, entry 23). The calculation-predicted Δδα-FR,S of (R)-amide of amine 23 was negative (−0.68), and Δδα-FR,S of (S)-amide of amine 23 was positive (0.68). The experimental measured Δδα-FR,S of the derived amide from amine 23 is positive (2.29), so the assigned configuration of amine 23 was S, which was confirmed by comparison of the optical rotation data with reference (Thvedt et al., 2010) (Supplementary 2). In addition, it should be noted that the present method was limited to be chiral amines, including a chiral tertiary carbon center and sterically more differentiated groups (L1/L2). As to chiral amines containing similar size in space, the obtained chemical shift difference is too small to be suitable for judging absolute configurations (Hamman, 1990).
Conclusions
A novel method for assigning the absolute configuration of chiral primary amines based on experimental and calculated 19F NMR has been developed. The method employs a new type of CDA, chiral α-fluorinated phenylacetic phenylselenoester, which can derivatize a primary amine directly in an NMR tube. Calculating the Δδα-FR,S value of (R)-FPA-(R)-amide and (S)-FPA-(R)-amide and the Δδα-FR,S value of (R)-FPA-(S)-amide and (S)-FPA-(S)-amide, the experimental chemical shift difference Δδα-FR,S of (R)-FPA-amide and (S)-FPA-amide is compared with the calculated values. If the experimental Δδα-FR,S has the same symbol (positive or negative) as one of the theoretical Δδα-FR,S, the assigned configuration of the amine is considered to be consistent with the theoretical one. Since both electronic and steric hindrance effects for the absolute configuration are considered, our method is widely valid for a broad substrate scope and avoids incorrect assignment of absolute configuration by empirical judgment.
Data Availability
All datasets generated for this study are included in the manuscript and the Supplementary Files.
Author Contributions
All authors listed have made a substantial, direct and intellectual contribution to the work, and approved it for publication.
Funding
This work was supported by the Strategic Priority Research Program of the Chinese Academy of Sciences (No. XDB20000000), the Natural Science Foundation of Fujian Province, China (No. 2018J01029), the Open Project Program of the State Key Laboratory of Photocatalysis on Energy and Environment (SKLPEE-KF201813), and the Key Laboratory of Coal to Ethylene Glycol and Its Related Technology, Fujian Institute of Research on the Structure of Matter, Chinese Academy of Sciences.
Conflict of Interest Statement
The authors declare that the research was conducted in the absence of any commercial or financial relationships that could be construed as a potential conflict of interest.
Supplementary Material
The Supplementary Material for this article can be found online at: https://www.frontiersin.org/articles/10.3389/fchem.2019.00318/full#supplementary-material
References
Aamouche, A., Devlin, F. J., Stephens, P. J., Drabowicz, J., Bujnicki, B., and Mikołajczyk, M. (2000). Vibrational circular dichroism and absolute configuration of chiral sulfoxides: tert-butyl methyl sulfoxide. Chem. Eur. J. 6, 4479–4486. doi: 10.1002/1521-3765(20001215)6:24<4479::Aid-Chem4479>3.0.Co;2-2
Apparu, M., Tiba, Y. B., Léo, P. M., Hamman, S., and Coulombeau, C. (2000). Determination of the enantiomeric purity and the configuration of β-aminoalcohols using (R)-2-fluorophenylacetic acid (AFPA) and fluorine-19 NMR: application to β-blockers. Tetrahedron 11, 2885–2898. doi: 10.1016/S0957-4166(00)00254-8
Bian, G., Fan, H., Huang, H., Yang, S., Zong, H., and Song, L., et al. (2015). Highly effective configurational assignment using bisthioureas as chiral solvating agents in the presence of DABCO. Org. Lett. 17, 1369–1372. doi: 10.1021/acs.orglett.5b00030
Bijvoet, J. M., Peerdeman, A. F., and Van Bommel, A. J. (1951). Determination of the absolute configuration of optically active compounds by means of X-rays. Nature 168, 271–272. doi: 10.1038/168271a0
Burns, A. S., Ross, C. C., and Rychnovsky, S. D. (2018). Heteroatom-directed acylation of secondary alcohols to assign absolute configuration. J. Org. Chem. 83, 2504–2515. doi: 10.1021/acs.joc.7b03156
Burtea, A., and Rychnovsky, S. D. (2017). Determination of the absolute configuration of cyclic amines with Bode's chiral hydroxamic esters using the competing enantioselective conversion method. Org. Lett. 19, 4195–4198. doi: 10.1021/acs.orglett.7b01748
Dale, J. A., and Mosher, H. S. (1973). Nuclear magnetic resonance enantiomer regents. Configurational correlations via nuclear magnetic resonance chemical shifts of diastereomeric mandelate, O-methylmandelate, and α-methoxy-α-trifluoromethylphenylacetate (MTPA) esters. J. Am. Chem. Soc. 95, 512–519. doi: 10.1021/Ja00783a034
De Gussem, E., Bultinck, P., Feledziak, M., Marchand-Brynaert, J., Stevens, C. V., and Herrebout, W. (2012). Vibrational circular dichroism versus optical rotation dispersion and electronic circular dichroism for diastereomers: the stereochemistry of 3-(1′-hydroxyethyl)-1-(3′-phenylpropanoyl)-azetidin-2-one. Phys. Chem. Chem. Phys. 14, 8562–8571. doi: 10.1039/c2cp40617a
Dickins, R. S., Howard, J. A., Maupin, C. L., Moloney, J. M., Parker, D., and Riehl, J. P., et al. (1999). Synthesis, time-resolved luminescence, NMR spectroscopy, circular dichroism and circularly polarised luminescence studies of enantiopure macrocyclic lanthanide tetraamide complexes. Chem. Eur. J. 5, 1095–1105. doi: 10.1002/(Sici)1521-3765(19990301)5:3<1095::Aid-Chem1095>3.0.Co;2-C
Flack, H. D., and Bernardinelli, G. (2000). Reporting and evaluating absolute-structure and absolute-configuration determinations. J. Appl. Crystallogr. 33, 1143–1148. doi: 10.1107/S0021889800007184
Freire, F., Quinoa, E., and Riguera, R. (2008). In tube determination of the absolute configuration of α-and β-hydroxy acids by NMR via chiral BINOL borates. Chem. Commun. 35, 4147–4149. doi: 10.1039/b806529b
Freire, F., Seco, J. M., Quinoá, E., and Riguera, R. (2005). Determining the absolute stereochemistry of secondary/secondary diols by 1H NMR: basis and applications. J. Org. Chem. 70, 3778–3790. doi: 10.1021/jo048643a
Frisch, M. J., Trucks, G. W., Schlegel, H. B., Scuseria, G. E., Robb, M. A., and Cheeseman, J. R., et al. (2010). Gaussian 09, Revision b. 01. Vol. 6492. Wallingford, CT: Gaussian. Inc.
Fujiwara, T., Omata, K., Kabuto, K., Kabuto, C., Takahashi, T., and Segawa, M., et al. (2001). Determination of the absolute configurations of α-amino esters from the 19F NMR chemical shifts of their CFTA amide diastereomers. Chem. Commun. 24, 2694–2695. doi: 10.1039/B108842d
Hamman, S. (1990). Acide 2-fluoro-2-phenylacetique. Partie 3 [1]. Correlation entre la configuration de ses amides d'amines chirales et les deplacements chimiques du proton et du fluor. J. Fluorine. Chem. 50, 327–38. doi: 10.1016/S0022-1139(00)84998-2
Hamman, S. (1993). Acide 2-fluoro-2-phényl acétique. Partie 5 [1]. RMN du fluor de ses amides formés avec des hydroxy amines et des fluoro amines. J. Fluorine. Chem. 62, 5–13. doi: 10.1016/S0022-1139(00)80075-5
Hanna, G. M., and Lau-Cam, C. A. (1993). Determination of the optical purity and absolute configuration of threo-methylphenidate by proton nuclear magnetic resonance spectroscopy with chiral solvating agent. J. Pharmaceut. Biomed. 11, 665–670. doi: 10.1016/0731-7085(93)80172-W
Hoye, T. R., and Renner, M. K. (1996). MTPA (Mosher) amides of cyclic secondary amines: conformational aspects and a useful method for assignment of amine configuration. J. Org. Chem. 61, 2056–2064. doi: 10.1021/Jo952043h
Huang, X., Borhan, B., Rickman, B. H., Nakanishi, K., and Berova, N. (2000). Zinc porphyrin tweezer in host–guest complexation: determination of absolute configurations of primary monoamines by circular dichroism. Chem –Eur. J. 6, 216–224. doi: 10.1002/(Sici)1521-3765(20000117)6:2<216::Aid-Chem216>3.0.Co;2-1
Jacobus, J., Raban, M., and Mislow, K. (1968). Preparation of (+)-N-methyl-1-(1-naphthyl) ethylamine and the determination of its optical purity by nuclear magnetic resonance. J. Org. Chem. 33, 1142–1145. doi: 10.1021/Jo01267a048
Kirk, K. (1998). Efficient synthesis of a new, highly versatile chiral derivatizing agent, α-cyano-α-fluoro-p-tolylacetic acid (CFTA). Chem. Commun. 3, 365–366. doi: 10.1039/A707755f
Kumari, D., Bandyopadhyay, P., and Suryaprakash, N. (2013). Discrimination of α-amino acids using green tea flavonoid (–)-epigallocatechin gallate as a chiral solvating agent. J. Org. Chem. 78, 2373–2378. doi: 10.1021/jo3025016
Kumari, D., Sachin, S. L., and Suryaprakash, N. (2015). Enantio sensing property of helicin, the derivative of a natural product: discrimination of amines and amino alcohols. Chem. Phys. Lett. 636, 72–77. doi: 10.1016/j.cplett.2015.07.023
Lakshmipriya, A., Chaudhari, S. R., and Suryaprakash, N. (2016). p-TsOH promotes 2′-amino-[1, 1′-binaphthalene]-2-ol as a chiral solvating agent. New. J. Chem. 40, 8118–8122. doi: 10.1039/c6nj02101h
Laskowski, T., Szwarc, K., Szczeblewski, P., Sowinski, P., Borowski, E., and Pawlak, J. (2016). Monosaccharides as potential chiral probes for the determination of the absolute configuration of secondary alcohols. J. Nat. Prod. 79, 2797–2804. doi: 10.1021/acs.jnatprod.6b00471
Liu, G., Sheng, J., and Zhao, Y. (2017). Chiral covalent organic frameworks for asymmetric catalysis and chiral separation. Sci. China. Chem. 60, 1015–1022. doi: 10.1007/s11426-017-9070-1
Ma, Y., Li, Y., Ma, K., and Wang, Z. (2018). Optical colorimetric sensor arrays for chemical and biological analysis. Sci. China. Chem. 61, 643–655. doi: 10.1007/s11426-017-9224-3
Ohtani, I., Kusumi, T., Kashman, Y., and Kakisawa, H. (1991). High-field FT NMR application of Mosher's method. The absolute configurations of marine terpenoids. J. Am. Chem. Soc. 113, 4092–4096. doi: 10.1021/Ja00011a006
Orlov, N. V., and Ananikov, V. P. (2010). First principles design of derivatizing agent for direct determination of enantiomeric purity of chiral alcohols and amines by NMR spectroscopy. Chem. Commun. 46, 3212–3214. doi: 10.1039/b923359h
Orlov, N. V., and Ananikov, V. P. (2011). NMR analysis of chiral alcohols and amines: development of an environmentally benign “in tube” procedure with high efficiency and improved detection limit. Green Chem. 13, 1735–1744. doi: 10.1039/c1gc15191f
Pal, I., Chaudhari, S. R., and Suryaprakash, N. (2014). A versatile ternary ionic complex for chiral discrimination of molecules with diverse functionalities using 1H NMR. N. J. Chem. 38, 4908–4912. doi: 10.1039/c4nj00417e
Pescitelli, G., and Bruhn, T. (2016). Good computational practice in the assignment of absolute configurations by TDDFT calculations of ECD spectra. Chirality 28, 466–474. doi: 10.1002/chir.22600
Sairenji, S., Kikuchi, T., Abozeid, M. A., Takizawa, S., Sasai, H., and Ando, Y., et al. (2017). Determination of the absolute configuration of compounds bearing chiral quaternary carbon centers using the crystalline sponge method. Chem. Sci. 8, 5132–5136. doi: 10.1039/c7sc01524k
Seco, J. M., Quino,á, E., and Riguera, R. (2004). The assignment of absolute configuration by NMR. Chem. Rev. 104, 17–118. doi: 10.1021/cr000665j
Shvo, Y., Taylor, E. C., Mislow, K., and Raban, M. (1967). Chemical shift nonequivalence of diastereotopic protons due to restricted rotation around aryl-nitrogen bonds in substituted amides. J. Am. Chem. Soc. 89, 4910–4917. doi: 10.1021/Ja00995a015
Sullivan, G. R., Dale, J. A., and Mosher, H. S. (1973). Correlation of configuration and fluorine-19 chemical shifts of α-methoxy-α-trifluoromethylphenyl acetate derivatives. J. Org. Chem. 38, 2143–2147. doi: 10.1021/Jo00952a006
Takeuchi, Y., Fujisawa, H., and Noyori, R. (2004). A very reliable method for determination of absolute configuration of chiral secondary alcohols by 1H NMR spectroscopy. Org. Lett. 6, 4607–4610. doi: 10.1021/ol047989
Takeuchi, Y., Itoh, N., Note, H., Koizumi, T., and Yamaguchi, K. (1991). α-Cyano-α-fluorophenylacetic acid (CFPA): a new reagent for determining enantiomeric excess that gives very large fluorine-19 NMR Δδ values. J. Am. Chem. Soc. 113, 6318–6320. doi: 10.1021/Ja00016a085
Takeuchi, Y., Itoh, N., Satoh, T., Koizumi, T., and Yamaguchi, K. (1993). Chemistry of novel compounds with multifunctional carbon structure. 9. Molecular design, synthetic studies, and NMR investigation of several efficient chiral derivatizing reagents which give very large 19F NMR Δδ values in enantiomeric excess determination. J. Org. Chem. 58, 1812–20. doi: 10.1021/Jo00059a036
Takeuchi, Y., Segawa, M., Fujisawa, H., Omata, K., Lodwig, S. N., and Unkefer, C. J. (2006). The CFTA method: a reliable procedure for the determination of the absolute configuration of chiral primary amines by 1H NMR spectroscopic analysis. Angew. Chem. Int. Edit. 45, 4617–4619. doi: 10.1002/anie.200600157
Temperini, A., Piazzolla, F., Minuti, L., Curini, M., and Siciliano, C. (2017). General, mild, and metal-free synthesis of phenyl selenoesters from anhydrides and their use in peptide synthesis. J. Org. Chem. 82, 4588–4603. doi: 10.1021/acs.joc.7b00173
Thvedt, T. H. K., Fuglseth, E., Sundby, E., and Hoff, B. H. (2010). Enantioenriched 1-aryl-2-fluoroethylamines. Efficient lipase-catalysed resolution and limitations to the Mitsunobu inversion protocol. Tetrahedron 66, 6733–6743. doi: 10.1016/j.tet.2010.06.081
Trost, B. M., Bunt, R. C., and Pulley, S. R. (1994). On the use of O-methylmandelic acid for the establishment of absolute configuration of. α-chiral primary amines. J. Org. Chem. 59, 4202–4205. doi: 10.1021/Jo00094a035
Wenzel, T. J., and Chisholm, C. D. (2011). Using NMR spectroscopic methods to determine enantiomeric purity and assign absolute stereochemistry. Prog. Nucl. Mag. Res. Sp. 59, 1–63. doi: 10.1016/j.pnmrs.2010.07.003
Yabuuchi, T., and Kusumi, T. (2000). Phenylglycine methyl ester, a useful tool for absolute configuration determination of various chiral carboxylic acids. J. Org. Chem. 65, 397–404. doi: 10.1021/Jo991218a
Keywords: absolute configuration assignment, primary amine, 19F nuclear magnetic resonance, DFT calculation, fluorinated phenylacetic phenylselenoester
Citation: Yang S, Bian G, Sa R and Song L (2019) Assigning the Absolute Configurations of Chiral Primary Amines Based on Experimental and DFT-Calculated 19F Nuclear Magnetic Resonance. Front. Chem. 7:318. doi: 10.3389/fchem.2019.00318
Received: 01 March 2019; Accepted: 23 April 2019;
Published: 20 May 2019.
Edited by:
Seiji Mori, Ibaraki University, JapanReviewed by:
Siriporn Jungsuttiwong, Ubon Ratchathani University, ThailandMasahiro Yamanaka, Rikkyo University, Japan
Copyright © 2019 Yang, Bian, Sa and Song. This is an open-access article distributed under the terms of the Creative Commons Attribution License (CC BY). The use, distribution or reproduction in other forums is permitted, provided the original author(s) and the copyright owner(s) are credited and that the original publication in this journal is cited, in accordance with accepted academic practice. No use, distribution or reproduction is permitted which does not comply with these terms.
*Correspondence: Guangling Bian, glb@fjirsm.ac.cn
Rongjian Sa, rjsa@mju.edu.cn
Ling Song, songling@fjirsm.ac.cn