- Department of Materials and Life Science, Seikei University, Musashino-shi, Japan
The development of efficient redox-photosensitizers based on the earth-abundant metal ions as an alternative toward noble- and/or rare-metal based photosensitizers is very desirable. In recent years, heteroleptic diimine-diphosphine Cu(I) complexes have been well investigated as one of the most remarkable candidates because of their great potentials as efficient photosensitizers. Here, we investigated the effects of the structure of the diphosphine ligands on the photosensitizing abilities using a series of Cu(I) complexes bearing 2,9-dimethyl-4,7-diphenyl-1,10-phenanthroline (dmpp) and various diphosphine ligands in order to explore the suitable structure for the photosensitizing reactions. The number of methylene chains between the two phosphorous atoms in the diphosphine ligands was systematically changed from two to four, and the relationship between the length of the carbon chains and the photosensitizing abilities were investigated by conducting photocatalytic CO2 reduction with the Cu(I) complexes as photosensitizers. Turnover frequencies of the CO2 reduction drastically increased with increasing the length of the carbon chains. The systematic study herein reported suggests that the large P-Cu-P angles should be one of the most important factors for enhancing the photosensitizing abilities.
Introduction
In the last few decades, photocatalytic CO2 reduction has been widely investigated due to emerging concerns about the serious environmental problems, e.g., global warming and depletion of carbon and energy resources. Metal complexes have played an important role both as photosensitizers and as CO2 reduction catalysts in this research area, owing to their suitable photophysical and photochemical properties, high reaction selectivity, and flexibility in the molecular design (Morris et al., 2009; Windle and Perutz, 2012; Sahara and Ishitani, 2015; Yamazaki et al., 2015; Kuramochi et al., 2018). Noble- and/or rare-metal complexes in particular have shown quite high efficiency and durability. For instance, the photocatalytic systems constructed with a Re(I) tricarbonyl complex as a CO2-reduction catalyst and a ring-shaped Re(I) multinuclear complex as a photosensitizer, can trigger CO2 reduction with tremendously high quantum yield (ΦCO = 82%) (Morimoto et al., 2013; Rohacova and Ishitani, 2016, 2017). Another example is a system using a Ru(II)-Re(I) multinuclear complexes, (Gholamkhass et al., 2005; Sato et al., 2007; Koike et al., 2009; Tamaki et al., 2012, 2013a; Kato et al., 2015; Ohkubo et al., 2016; Tamaki and Ishitani, 2017; Yamazaki and Ishitani, 2018) which shows both high efficiency and durability, i.e., up to 50% of ΦCO and more than 3,000 of the turnover number (TONCO) (Tamaki et al., 2013a; Tamaki and Ishitani, 2017; Yamazaki and Ishitani, 2018). Though the fundamental researches using noble- and/or rare-metals must be quite important in order to fully understand the reaction mechanism of the CO2 reduction and to explore strategies of molecular design for efficient components in photocatalytic systems, in the future, such metals which lie under the ground in small amounts should be replaced by earth-abundant elements because the amount of emission of CO2 relating to consumption of fossil fuels is quite large and is increasing year by year (Takeda et al., 2016a).
In recent years, heteroleptic diimine-diphosphine Cu(I) complexes have been reported as an alternative toward noble- and/or rare-metal based photosensitizers (Takeda et al., 2016b, 2018; Heberle et al., 2017; Rosas-Hernández et al., 2017; McCullough et al., 2018; Zhang et al., 2018). Some of them show not only high efficiency but also high durability equal to or higher than those of noble- and/or rare-metal complexes, and the highest ΦCO and TONCO in the photocatalytic systems for CO2 reduction using Cu(I) photosensitizers were 57% and >1,300, respectively (Takeda et al., 2018). These reports clearly indicate that Cu(I) complexes should be powerful candidates not just as an alternative toward photosensitizers based on noble- and/or rare-metal ions, but as one of the most efficient photosensitizers. Therefore, further investigation using series of Cu(I) complexes to clarify the relationship between the molecular structure and photosensitizing abilities should be useful to explore the suitable molecular design for the efficient Cu(I)-complex photosensitizers.
We previously reported photophysical properties of series of diimine-diphosphine Cu(I) complexes bearing 1,10-phenanthroline derivatives and various bidentate phosphine ligands (Saito et al., 2006; Tsubomura et al., 2015; Nishikawa et al., 2017). In particular, a series of the Cu(I) complexes having 2,9-dimethyl-4,7-diphenyl-1,10-phenanthroline (dmpp) showed high molar extinction coefficients and emission quantum yields compared with those without phenyl groups at 4,7 positions (Tsubomura et al., 2015). The photophysical properties of the dmpp complexes were strongly affected by the structure of the bidentate phosphine ligands; wavelength of both absorption bands and emission maxima were blue-shifted and emission lifetimes became longer with increasing the length of the methylene chains between the two phosphorous atoms, likely due to the difference in the bite angles of the chelate-phosphine ligands (P-Cu-P angles). The strong absorption abilities and long emission lifetimes should be useful not only as photo-luminescent materials but also as photosensitizers for photochemical reactions. Therefore, in this study, we examined the effects of diphosphine ligands in detail on the photosensitizing abilities using a series of Cu(I) complexes bearing dmpp ligands shown in Chart 1, i.e., dmpp complexes with 3 types of diphosphine ligands with different length of carbon chains: dppe (1,2-bis(diphenylphosphino)ethane), dppp (1,3-bis(diphenylphosphino)propane), and dppb (1,4-bis(diphenylphosphino)butane). The dmpp complex bearing Xantphos (4,5-Bis(diphenylphosphino)-9,9-dimethylxanthene), which is often used as a diphosphine ligand of Cu(I) photosensitizers, was also investigated for comparison. The systematic study herein reported suggests that the P-Cu-P angles should be one of the most important factors which determines the photosensitizing abilities.
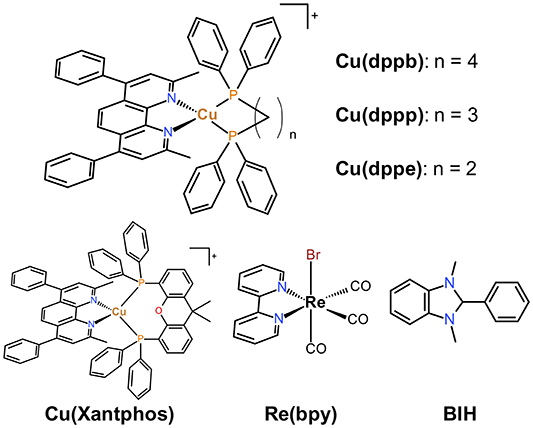
Chart 1. Structures and abbreviations of the Cu(I) complexes, the Re(I) complex and the electron donor used in this study.
Results and Discussion
Photocatalytic CO2 Reduction Using Cu(I) Complexes as Photosensitizers
In a typical run of photocatalytic reactions, a mixed solution of MeCN-TEOA (TEOA = triethanolamine, 4:1 v/v) containing Cu(dppb) (0.5 mM) as a photosensitizer, Re(2,2'-bipyridine)(CO)3Br (Re(bpy), 0.05 mM) as a CO2-reduction catalyst and 1,3-dimethyl-2-phenyl-2,3-dihydro-1H-benzo[d]imidazole (BIH, 0.1 M) as an electron donor was irradiated under a CO2 atmosphere using a high-pressure mercury-lamp equipped with a UV-cut filter (>370 nm, Figure 1). Re(bpy) is well known not only as a photocatalyst for CO2 reduction (Hawecker et al., 1983, 1986; Kutal et al., 1985) but also as a CO2-reduction catalyst in photocatalytic systems, which can produce CO with high selectivity; (Gholamkhass et al., 2005; Sato et al., 2007; Kiyosawa et al., 2009; Koike et al., 2009; Morris et al., 2009; Tamaki et al., 2012, 2013a,b; Kou et al., 2014; Kato et al., 2015; Ohkubo et al., 2016). Therefore, we measured the gaseous products of the photocatalysis using gas-chromatography. CO was selectively produced and no hydrogen was detected over 30-min irradiation. TONCO after 30-min irradiation was 40. Though various other CO2-reduction catalysts, i.e., Re(4,4'-dimethyl-2,2'-bipyridine)(CO)3Br, Re(1,10-phenanthroline)(CO)3Br, and Ru(6,6'-dimethyl-2,2'-bipyridine)(CO)2Cl2, were also used instead of Re(bpy), the system using Re(bpy) showed the highest TONCO in this reaction condition (Figure S1).
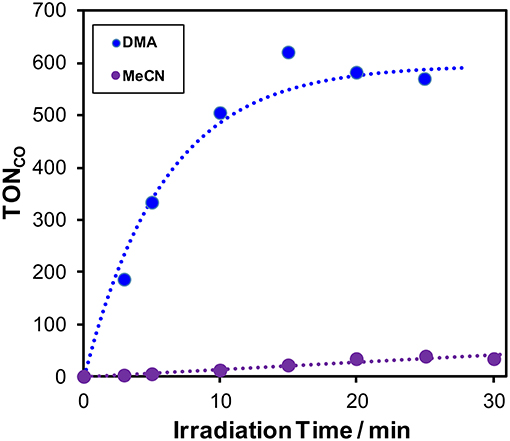
Figure 1. Time courses of the TON of CO formation during photocatalytic reactions (λex > 370 nm) using a mixture of MeCN and TEOA (4:1 v/v, purple) or a mixture of DMA and TEOA (4: 1 v/v, blue) containing 0.5 mM Cu(dppb), 0.05 mM Re(bpy), and 0.1 M BIH.
In the case of using a mixed solvent of DMA-TEOA (DMA = N,N-dimethylacetamide, 4:1 v/v) instead of that of MeCN-TEOA (4:1 v/v), TONCO increased and reached 580 after 25-min irradiation (Figure 1). The turnover frequency (TOF), which was determined from the slopes of the fitting curves of the time course in the initial stage of the photocatalysis, was relatively high (65 min−1, Figure S2). This result suggests that DMA should be more preferable solvent for the efficient photocatalytic reaction using Cu(dppb) and Re(bpy). In the absence of Cu(dppb), TONCO after 1-h irradiation were 20; thus, the photocatalysis by only Re(bpy) should be not sufficient under the condition. In addition, in the absence of Re(bpy) or BIH, TONCO were 0 or 7, respectively. These results clearly indicate that Cu(dppb) has relatively high photosensitizing ability and promoted CO2 reduction on Re(bpy) using BIH as an electron donor. From these results, we concluded the photocatalyses using Re(bpy) as a CO2-reduction catalyst and a mixed solvent of DMA-TEOA as a reaction solvent are suitable for the investigation to clarify the photosensitizing abilities of the series of the Cu(I) complexes shown in Chart 1.
Mixed solutions of DMA-TEOA (4:1 v/v) containing Re(bpy) (0.05 mM), BIH (0.1 M), and various Cu(I) complexes (0.5 mM) were shined under the same condition described above. Figure 2 shows the time-courses of the CO production and both TONCO and TOF are summarized in Table 1 and Figure S2. Interestingly, both TONCO and TOF strongly depended on the structure of the diphosphine ligands. When comparing Cu(dppb), Cu(dppp), and Cu(dppe), TOF drastically increased with increasing the length of the methylene chains between the two phosphorous atoms in the diphosphine ligands. In the case using Cu(Xantphos), TOF was quite high and almost equal to that of Cu(dppb). In contrast, TONCO of Cu(Xantphos) was low, and the time course of CO formation reached plateau within 5-min of irradiation. The absorption spectral changes of the reaction solutions after irradiation are shown in Figure S3. In all cases, the metal-to-ligand charge transfer (MLCT) absorption bands of the Cu(I) complexes decreased gradually; therefore, one of the main reasons for the decreasing of the reaction rate with increasing the irradiation time should be the photo-decomposition of the Cu(I) complexes. Curiously, in the case using Cu(Xantphos), the photocatalytic CO formation stopped within 5 min even though the MLCT absorption band was obviously observed in the absorption spectrum of the reaction solution after 5-min irradiation. Though the reason for the low TONCO when using Cu(Xantphos) is not clear at this stage, the degradation of Re(bpy) possibly proceeded more rapidly than the other cases. As described later, Cu(Xantphos) showed quite small absorption in the longer wavelength region (>430 nm) compared to the other Cu(I) complexes. It has been recently reported that the irradiation with short wavelength light (<450 nm) drastically lowered the photo-stability of Re(I) complexes; (Lang et al., 2019) thus, it is expected that Re(bpy) could absorb the irradiation light in the longer wavelength region, e.g., the emission line at 436 nm from the high-pressure mercury lamp, more frequently and photo-decomposition of Re(bpy) proceeded rapidly. Since the analysis of the Re(I) complexes, e.g., measurement of FT-IR spectra of the reaction solutions, was difficult because of the low concentration of the Re(I) complexes, further investigation is now undergoing.
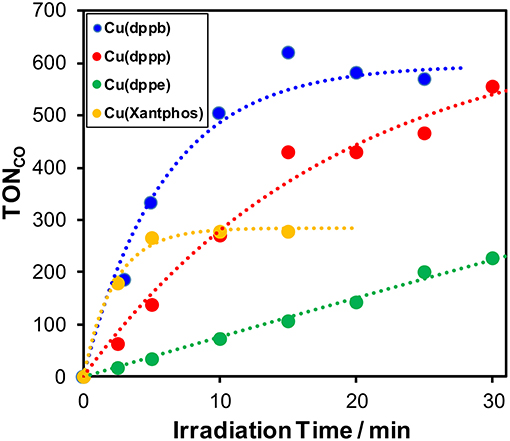
Figure 2. Time courses of the TON of CO formation during photocatalytic reactions (λex > 370 nm) using a mixture of DMA and TEOA (4:1 v/v) containing 0.05 mM Re(bpy), 0.1 M BIH, and various Cu(I) complexes [blue: Cu(dppb), red: Cu(dppp), green: Cu(dppe), orange: Cu(Xantphos)]. The concentration of each Cu(I) complex is 0.5 mM.
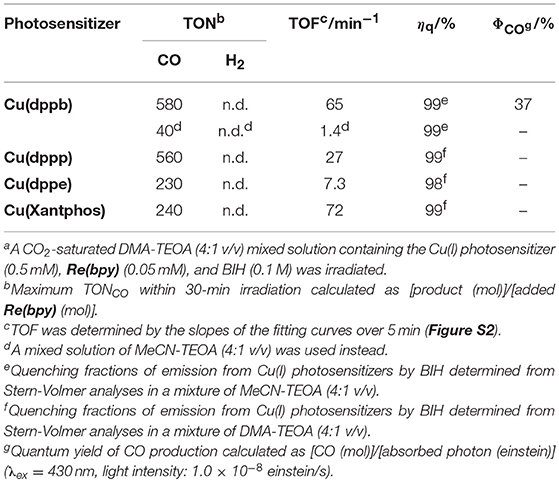
Table 1. Photocatalytic properties using the mixed system of the Cu(I) photosensitizers and Re(bpy)a.
In order to precisely evaluate the efficiency of CO2 reduction, the quantum yield for CO formation (ΦCO) was determined using the system of Cu(dppb) as a typical example. BIH is well known as a two-electron donor, which can induce the second-electron injection even to ground-state of photosensitizers and/or CO2-reduction catalysts owing to the quite strong reducing power of the radical species produced after oxidation by the excited state of photosensitizers and subsequent deprotonation by TEOA (Equation 1); (Tamaki et al., 2013a). Thus, the maximum ΦCO of this system should be 100%. A mixture of DMA and TEOA (4:1 v/v) containing 0.5 mM Cu(dppb), 0.05 mM Re(bpy), and 0.1 M BIH was irradiated with 430-nm monochromic light. CO was linearly produced over 1-h irradiation, and ΦCO was determined to be 37% from the slopes of the fitting curves (Figure S4). This value is relatively high among the photocatalytic systems for CO2 reduction using Cu(I)-complex photosensitizers.

Properties of Cu(I) Complexes
Structure of Cu(I) Complexes in Solutions
As described above, the Cu(I) complexes, in particular Cu(dppb), showed relatively high photosensitizing ability. In order to clarify the reason for the difference in the photosensitizing abilities of the Cu(I) complexes, we firstly investigated the molecular structure of the Cu(I) complexes in the reaction solutions in detail because it is known that Cu(I) complexes often cause structural changes in solutions due to the ligand-exchange reactions. Figure 3 illustrates the UV-vis absorption spectra of Cu(dppb) in various solutions, i.e., dichloromethane, DMA, a mixture of DMA-TEOA and that of MeCN-TEOA. The shapes of the spectra at around 330–450 nm were similar. Except the spectrum in dichloromethane, small absorption bands were observed at around 450–530 nm. This is probably due to the formation of [Cu(dmpp)2]+-type complex produced by the disproportionation reaction after dissolving in solvents having coordination ability (Kaeser et al., 2013). Since the molar extinction coefficient of [Cu(dmpp)2]+ was large (ε484 = 10,300 in a DMA-TEOA mixed solution, Figure S5), the amount of the [Cu(dmpp)2]+ should not be large. 1H NMR analyses of Cu(dppb) using CD2Cl2 or CD3CN also revealed that the structure in CD3CN is almost identical to that in CD2Cl2, though a few mol-percent of [Cu(dmpp)2]+ were observed. The other complexes also showed similar spectrum-pattern in both absorption spectra and 1H NMR spectra regardless of solvents (Figure S6). Therefore, the main species should be the diimine-diphosphine type complexes even in the reaction solutions for the photocatalytic reactions, and the solvent molecules do not strongly affect the structure and the electronic properties of the ground state of the Cu(I) complexes.
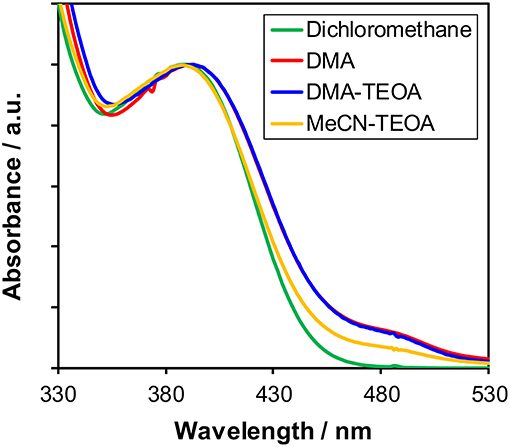
Figure 3. UV-Vis absorption spectra of Cu(dppb) in various solutions: dichloromethane (green), DMA (red), a mixture of DMA-TEOA (4:1 v/v, blue), and a mixture of MeCN-TEOA (4:1 v/v, orange).
We previously clarified that Cu(dppb) is dimerized by bridging with two bidentate phosphine ligands in the solid state using single crystal X-ray structure analyses. The dimerization might be a reason for the higher photosensitizing ability of Cu(dppb); Thus, we measured diffusion-ordered NMR spectroscopy (DOSY-NMR) in order to clarify whether Cu(dppb) is dimerized even in solutions. The obtained DOSY-NMR spectra of Cu(dppb), Cu(dppp), Cu(dppe), Cu(Xantphos), and [Cu(dmpp)2](PF6) measured in CD2Cl2 solutions are illustrated in Figure S7. The diffusion coefficients of Cu(dppb), Cu(dppp), Cu(dppe), Cu(Xantphos), and [Cu(dmpp)2](PF6) in the solutions were determined to be 7.5 × 10−10, 7.9 × 10−10, 8.0 × 10−10, 7.0 × 10−10 and 8.4 × 10−10 [m2/s], respectively. These obtained values were quite similar though they gradually decreased with increasing the molecular weight calculated as mononuclear complexes. Hence, the molecular sizes of the Cu(I) complexes might be almost the same in solutions. Since Cu(dppp) and Cu(dppe) were reported as mononuclear complexes in the solid state, Cu(dppb) in the reaction solutions is regarded as a mononuclear complex in this paper and the dimerization should not be the main reason for the high TOF of the system using Cu(dppb).
Absorption Abilities of the Cu(I) Complexes
Figure 4A shows the UV-vis absorption spectra of Cu(dppb) and Re(bpy) in a DMA-TEOA mixed solution. Both complexes showed MLCT absorption bands at similar region with similar molar extinction coefficients. However, in the reaction condition, Cu(dppb) can absorb visible light more efficiently than Re(bpy) because the used concentration of Cu(dppb) was 10-times higher than that of Re(bpy) (Figure 4B). The light source was a high-pressure mercury lamp equipped with a UV-cut filter (>370 nm); Therefore, the main wavelength of the excitation light should be 405 and 436 nm. At 405 and 436 nm, more than 96% of absorbed photons should excite Cu(dppb) [Abs405(Cu(dppb)): Abs405(Re(bpy)) = 2.3: 0.10, Abs436(Cu(dppb)): Abs436(Re(bpy)) = 1.1: 0.03]. This means Cu(dppb) was selectively excited by the excitation light under the reaction condition.
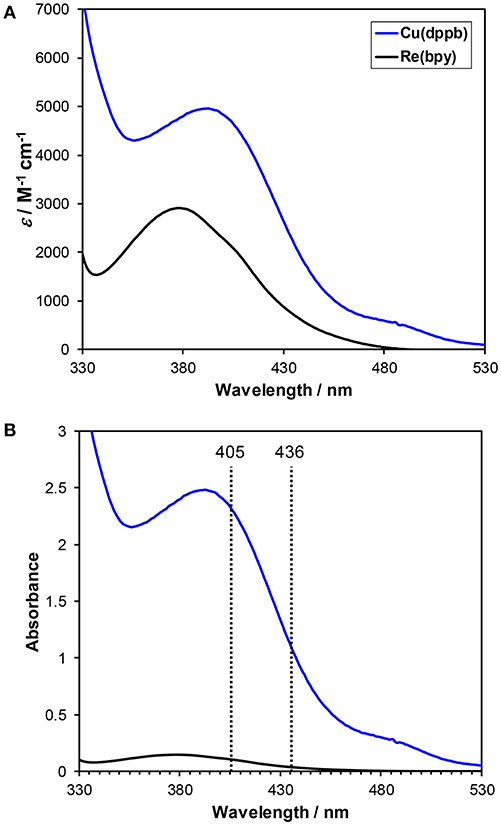
Figure 4. (A) UV-Vis absorption spectra of Cu(dppb) (blue) and Re(bpy) (black). The solvent was DMA-TEOA mixed solutions (4:1 v/v). (B) Normalized spectra by the concentration of the reaction condition for the photocatalytic reactions ([Cu(dppb)] = 0.5 mM, [Re(bpy)] = 0.05 mM).
The UV-Vis absorption spectra of the series of the Cu(I) complexes in DMA-TEOA mixed solutions are illustrated in Figure 5. All of the Cu(I) complexes showed MLCT absorption bands at around 350–450 nm. When comparing Cu(dppb), Cu(dppp), and Cu(dppe), the absorption maxima of the MLCT absorption bands were gradually blue-shifted with increasing the length of the carbon chains [λabs(Cu(dppe)) = 417 nm, λabs(Cu(dppp)) = 410 nm, λabs(Cu(dppb)) = 391 nm]. Similar blue-shift of the absorption maxima was also observed in the reported system using dichloromethane solutions (Tsubomura et al., 2015). It is reported that the shift was strongly related to the bite angles of the diphosphine ligands, which were determined using the single crystal X-ray diffraction, i.e., the absorption energy became larger as bite angle became larger. The wavelength of the absorption maximum of Cu(Xantphos) was the shortest (388 nm); thus, the bite angle of Xantphos in Cu(Xantphos) might be larger than those in the other complexes. It should be noted that the absorbance at the irradiation wavelength (405, 436 nm) are relatively high (Abs > 0.86). Therefore, the total of the absorbed photon number of each reaction solution for the photocatalyses should be almost identical regardless of the structure of the diphosphine ligands, and TOFs should reflect the relative quantum yields for the photocatalytic CO2 reduction.
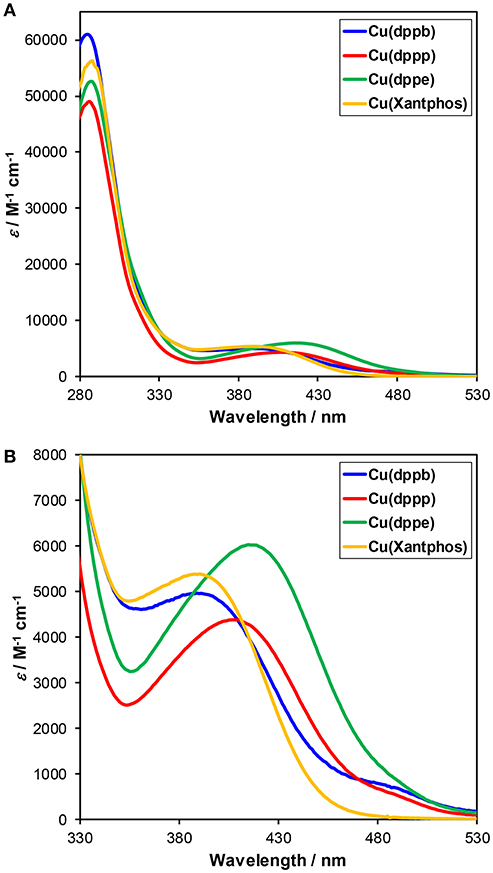
Figure 5. (A) UV-Vis absorption spectra of Cu(I) complexes, i.e., Cu(dppb) (blue), Cu(dppp) (red), Cu(dppb) (green), and Cu(Xantphos) (orange). The solvent was DMA-TEOA mixed solutions (4:1 v/v). (B) Enlarged spectra at λabs = 330–530 nm.
Photophysical and Photochemical Properties
In order to investigate the efficiencies of the initial steps of the photosensitizing reactions, we tried to measure the photophysical properties of Cu(I) complexes in the main solvent for the photocatalyses, i.e., DMA-TEOA mixed solutions. Interestingly, however, the Cu(I) complexes showed quite low luminescence properties in DMA-TEOA mixed solutions, even though the photocatalytic reactions proceeded efficiently in them. In particular, the emission quantum yield (Φem) of Cu(dppb) in the DMA-TEOA solutions was <0.01% and emission lifetime (τem) was shorter than 30 ns in contrast to those in other solvents (Φem in CH2Cl2 = 16%, τem in CH2Cl2 = 10 μs). The reason for the quite low luminescence properties of Cu(dppb) in DMA-TEOA solutions is now under investigation, but it might be caused by an efficient exciplex-quenching by DMA molecules (McMillin and McNett, 1998; Kuang et al., 2002). The detection of the luminescence from the DMA-TEOA solutions containing Cu(dppb) was difficult; therefore, we decided to compare the photophysical and photochemical properties of the series of Cu(I) complexes in the other solvents for the catalyses, i.e., a mixture of MeCN-TEOA. The emission properties of the Cu(I) complexes in a mixture of MeCN-TEOA (4:1 v/v) are summarized in Table 2 and the emission spectra are shown in Figure S8. All of the complexes showed weak emission intensity and short emission lifetimes in a mixture of MeCN-TEOA compared with those in dichloromethane likely due to the exciplex-quenching by the MeCN molecules. In fact, the emission intensity of Cu(dppb) in MeCN solutions was almost identical to that in MeCN-TEOA solutions. Hence, the low emission intensity observed should not be derived from the quenching by TEOA (Figure S9). In accordance with the blue-shift of the MLCT absorption bands in the absorption spectra, the wavelength of the emission maxima of the three complexes, i.e., Cu(dppb), Cu(dppp), and Cu(dppe), gradually decreased as the number of the methylene chains in the diphosphine ligands increased. The emission lifetimes became longer with increasing the length of the carbon chains. This is likely because the steric hindrance of the phenyl groups on the phosphorous atoms became larger with increasing the bite-angle of the diphosphine ligands, which resulted in inhibiting the attack of the solvent molecules in the excited state and decreasing non-radiative decay constants. Cu(Xantphos) showed the shortest wavelength of the emission maximum and the longest emission lifetime.
Since the reactions between the excited state of the Cu(I) complexes and BIH compete with the radiative and non-radiative deactivation processes, the shorter lifetime might lower the efficiencies of the quenching by BIH. Thus, we conducted quenching experiments using BIH to check whether the excited state of the Cu(I) complexes can be quenched by BIH. We measured emission intensity in the presence of various amounts of BIH. As shown in Figure 6A, the emission intensities became smaller with increasing the concentrations of BIH, indicating the excited state of Cu(dppb) was efficiently quenched by BIH. The Stern–Volmer analysis showed linear plots with an intercept at 1 (Figure 6B). From the slope of the fitting curve (KSV = kqτem = 0.71 mM−1) and the emission lifetime τem = 240 ns, the quenching rate constant (kq) was determined to be 3.0 × 109 M−1 s−1. From these values, the quenching fraction (ηq) is estimated to be larger than 98% under the reaction condition (Equation 2) and the excited state of Cu(dppb) is expected to be quenched by BIH almost quantitatively, even though the emission lifetime is not so long. Moreover, in the presence of Re(bpy), the emission intensity was almost identical to that in the absence of Re(bpy) (Figure S10); therefore, oxidative quenching, i.e., the electron transfer from the excited state of Cu(dppb) to Re(bpy) should be negligible. These results suggest that the photosensitizing reaction by Cu(dppb) mainly proceeds via the reductive quenching by BIH.
In the case using the other Cu(I) complexes, the emission intensity also decreased drastically by adding BIH, and emission from the Cu(I) complexes could not be detected in the presence of 0.1 M BIH. The Stern–Volmer analysis using the other Cu(I) complexes not only in a mixture of MeCN-TEOA but also in a mixture of DMA-TEOA could also be conducted owing to the larger emission intensities and the longer emission lifetimes in DMA-TEOA mixed solutions than those of Cu(dppb). The Stern–Volmer analysis showed linear plots with an intercept at 1 in all cases (Figure S11). From the slope of the fitting curve, the quenching fractions of Cu(dppp), Cu(dppe), and Cu(Xantphos) were estimated to be larger than 99, 98, and 99%, respectively. These results clearly indicate that all of the Cu(I) complexes used in this study have relatively strong oxidizing power in the excited state and the efficiencies of the initial stage of the photosensitizing reaction, i.e., reductive quenching, should be almostidentical regardless of the structure of the diphosphine ligands.
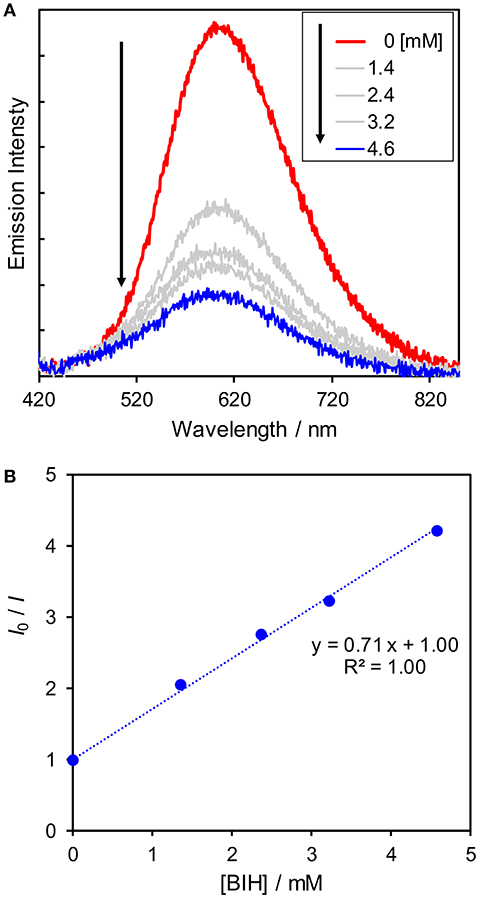
Figure 6. (A) Emission spectra (excitation 410 nm) of Cu(dppb) in MeCN-TEOA under an Ar atmosphere in the presence of various amounts of BIH and (B) the Stern-Volmer plot using emission intensity (I) and the emission intensity without any BIH (I0).
Spectral Changes During Irradiation
As mentioned above, the excited state of the Cu(I) complexes was efficiently quenched by BIH. To clarify the produced species of the quenching, UV-vis absorption spectral changes during photo-irradiation of a DMA-TEOA mixed solution (4:1 v/v) containing Cu(dppb) (0.1 mM) and BIH (0.1 M) under Ar were measured (Figure 7A). After an induction period over a few minutes, a new absorption bands at around 350–450 nm was observed. The new band increased continuously and linearly over 30-min irradiation. After irradiation, the solution was exposed to an ambient air. The solution color was immediately changed from deep-yellow to light-yellow. In the absorption spectrum measured after exposure to air, the new absorption band was completely disappeared and the original spectrum-shape was recovered (Figure 7C). These results clearly indicate that the quenching of the excited state of Cu(dppb) is a reductive quenching process, which gives one-electron reduced species (OERS) of Cu(dppb). In contrast, when using Cu(dppe) the observed spectral changes were much smaller than Cu(dppb) though the shape of the new absorption band was similar to those of Cu(dppb) (Figure 7B). This difference should be derived from the difference of efficiencies for production of OERS between Cu(dppb) and Cu(dppe). Cu(Xantphos) also showed rapid accumulation of OERS under the same condition, and Cu(dppp), in contrast, showed slow accumulation as well as Cu(dppe) (Figure S12). Though the determination of the exact rate of production of OERS requires a spectrum obtained by electrochemical spectroscopy technics, such big difference in spectral changes and similarity of the shapes of the new absorption bands should suggest that the efficiency for production of OERS of Cu(dppb) and Cu(Xantphos) should be much larger than those of Cu(dppp) and Cu(dppe). The tendency of the degree of the spectral changes corresponded reasonably well with that of TOF described above.
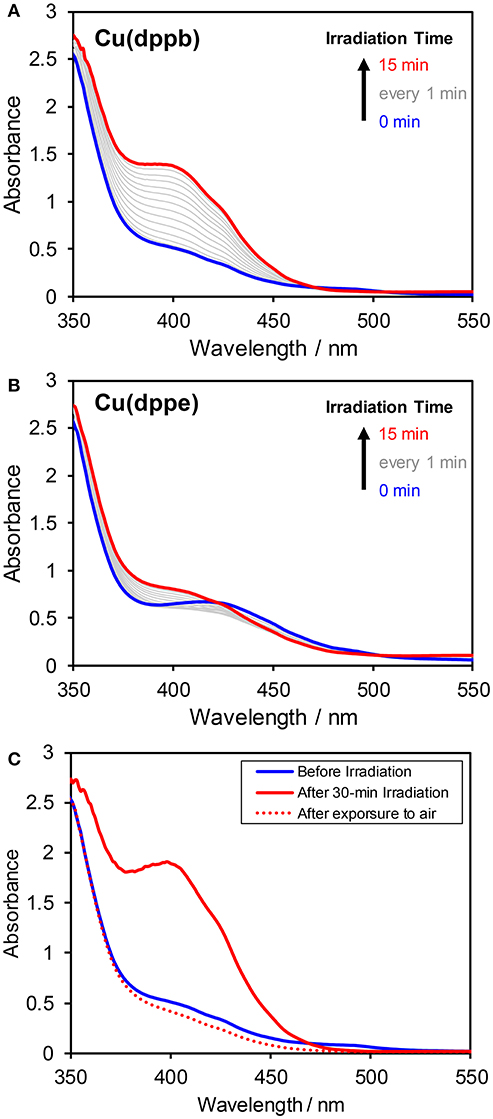
Figure 7. Absorption spectral changes of DMA–TEOA (4:1 v/v) solutions containing BIH (0.1 M) and 0.1 mM of (A) Cu(dppb) or (B) Cu(dppe) under an argon atmosphere. The solution was irradiated with 430-nm monochromic light. (C) Absorption spectral changes of the reaction solution of Cu(dppb) measured before irradiation (blue solid line), just after 30-min irradiation (red solid line), and after irradiation and subsequent exposure to an ambient air (red dotted line).
Electrochemical Properties
Figure 8 shows the cyclic voltammograms of Cu(dppb) and Re(bpy) measured in DMA-TEOA solutions and their redox potentials are summarized in Table 3. Both Cu(dppb) and Re(bpy) exhibited a quasi-reversible reduction wave as a first reduction wave at −1.96 and −1.70 V (vs. Ag/AgNO3), respectively. These waves are attributable to the reduction of the diimine ligands. The reduction potential of Cu(dppb) is more negative than that of Re(bpy); thus, OERS of Cu(dppb) has relatively strong reducing power and the electron transfer process from OERS of Cu(dppb) to Re(bpy) occurs exothermically when using Cu(dppb) as a photosensitizer and Re(bpy) as a catalyst. Interestingly, when using an MeCN-TEOA solution, the first reduction wave of Cu(dppb) became irreversible, though the peak potential was almost identical to that in the DMA-TEOA solution. This means OERS of Cu(dppb) is not stable in an MeCN-TEOA solution and might be one of the reasons for the low TONCO in the case using MeCN-TEOA solutions. Even though the oxidation potentials of the complexes could not be observed due to the potential window narrowed by oxidation of TEOA, the irreversible oxidation wave was observed at +0.80 V when using a DMA solution instead of the DMA-TEOA solutions. The first reduction potential observed in the DMA solution was almost identical to that in the presence of TEOA (−1.91 V). Cu(dppb) showed more negative reduction potential than Ru(II) tris-diimine complexes, which are typical redox-photosensitizers in the photocatalytic systems for CO2 reduction. On the other hand, the oxidation potential was similar to those of the Ru(II) complexes; (Yamazaki et al., 2015) thus, Cu(dppb) should have both high reducing power in the reduced state and relatively high oxidizing power in the excited state.
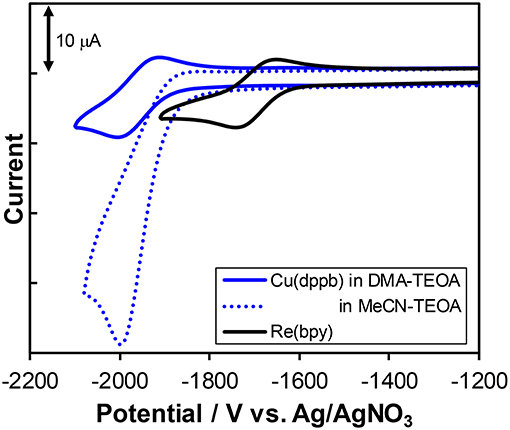
Figure 8. Cyclic voltammograms of Cu(dppb) (blue solid line) and Re(bpy) (black solid line) a mixture of DMA and TEOA (4:1 v/v) containing Et4NPF6 (0.1 M) as a supporting electrolyte with a Ag/AgNO3 (0.01M) reference electrode. The voltammogram of Cu(dppb) measured in a mixture of MeCN and TEOA (4:1 v/v) is also illustrated as a blue dotted line.
The other Cu(I) complexes also showed reversible or quasi-reversible waves in the cyclic voltammograms measured in DMA-TEOA solutions (Figure S13). The reduction potentials were almost identical (−1.93 to −1.96 V); the reduction potentials were not strongly affected by the structure of the diphosphine ligands. These results are reasonable because it is well known that the lowest-unoccupied molecular orbitals (LUMO) of the Cu(I) complexes are mainly derived from the π*-orbitals of diimine ligands and the series of Cu(I) complexes have the same diimine ligands. Therefore, all of the Cu(I) complexes should have high reducing power in the reduced state enough to smoothly trigger the electron transfer from OERS of the Cu(I) complexes to Re(bpy).
Expected Reaction Mechanism and Relationship Between the Molecular Structure and the Photosensitizing Abilities
From the results in the previous sections, the expected reaction mechanism of the photocatalytic systems in this study can be summarized as follows (Figure 9): (1) almost all of the irradiated photons are absorbed by the Cu(I) complexes; (2) the excited states of the Cu(I) complexes are reductively quenched by BIH to give OERS with high efficiencies; (3) the electron transfer from the OERS of the Cu(I) complexes to Re(bpy) proceeds exothermically; (4) CO2 reduction takes place on Re(bpy) using the obtained electrons.
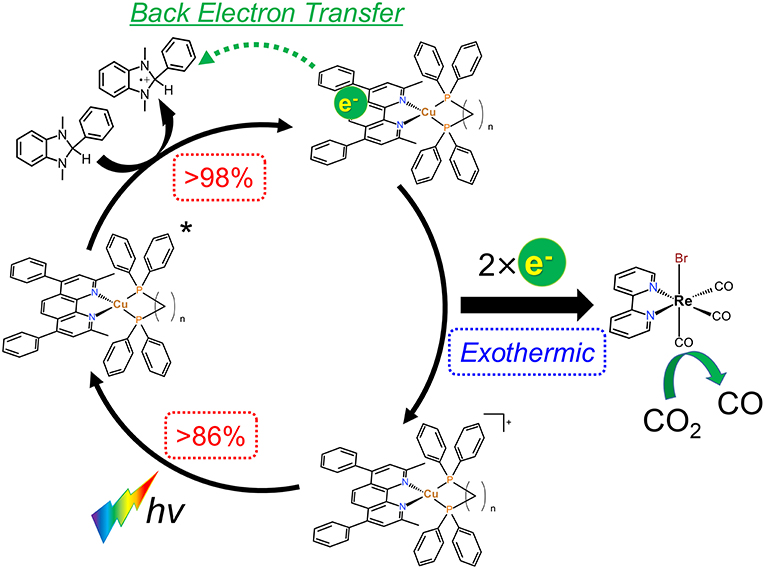
Figure 9. Expected reaction mechanism of the CO2 reduction using the Cu(I) complexes, Re(bpy) and BIH.
As described in section Photocatalytic CO2 reduction using Cu(I) complexes as photosensitizers, TOFs were drastically changed by changing the structure of the diphosphine ligands. In contrast, all of the Cu(I) complexes showed quite similar absorbance, quenching fractions and reduction potentials; therefore, we could not observe a big difference in the efficiency of each step illustrated in Figure 9. However, only the efficiencies of the production of OERS strongly depended on the molecular structure even though the preceding process, i.e., the reductive quenching, proceeded almost quantitatively in all cases. It is reported that the efficiencies for the production of OERS strongly affected not only by the quenching fractions but also by the rate of the back electron transfer processes, i.e., the electron transfer from the OERS of the Cu(I) complexes to the one-electron oxidized species of BIH (BIH·+) produced by the reductive quenching processes (Tamaki et al., 2013a). This charge-recombination process forms the ground states of the Cu(I) complexes and BIH, resulting in wasting absorbed photons. The low efficiencies for the production of OERS in the case using Cu(dppe) should indicate that the back electron transfer proceeded more rapidly than the case using Cu(dppb) (Figure 10). The reason for the great difference in the rate of the back electron transfer is unclear at the moment, but the difference in the bite angles of the diphosphine ligands might be an important factor. The orientation of the four phenyl groups on the two phosphorous atoms is affected by changing the bite angles of the diphosphine ligands. Along with this, the distance between the phenyl groups on the phosphine ligands and the dmpp ligands will decrease by increasing the bite angles. The electrons obtained photochemically should mainly localize on the π* orbital in the dmpp ligands; thus, the back electron transfer process should proceed via the attack of BIH·+ to the π* orbital. If the phenyl groups exist nearby the dmpp ligands and cause the steric hindrance, the access of BIH·+ will be suppressed. As a result, the large P-Cu-P angles might suppress the charge-recombination and enhanced the efficiencies for OERS production. In fact, not only the photophysical properties but also TOFs have good correlation with the reported bite angles determined using the single crystal X-ray diffraction (Table 4); (Tsubomura et al., 2015; Heberle et al., 2017) in other words, not only long emission lifetimes but also high photosensitizing abilities will be obtained by increasing the bite angles of the diphosphine ligands likely due to the steric hindrance of the phenyl groups to suppress the approach of the solvent molecules to the Cu(I) center and BIH·+ to the dmpp ligands.
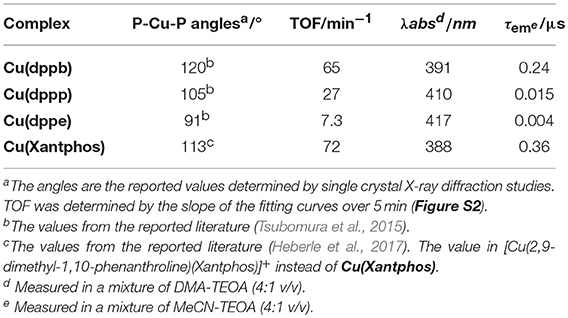
Table 4. Relationship between P-Cu-P angles in the solid state of the Cu(I) complexes and photosensitizing abilitiesa.
Experiments
General Procedure
NMR spectra were recorded on a JEOL ECA500 (500 MHz) spectrometer. The spectra were analyzed by Delta version 5. Transmission UV-vis (ultraviolet-visible light) absorption spectra were recorded on an Agilent 8453 spectrometer. Emission spectra were obtained in a solvent degassed by bubbling with argon. Emission spectra and emission lifetimes were recorded on a laboratory-made apparatus. The samples were excited by a monochromated xenon light source and the emission was analyzed by using a cooled CCD spectrometer. For the lifetime measurement, a nitrogen laser (USHO AN-200) was used to excite the samples, and the emission was detected by a monochromator equipped with a photomultiplier tube, and the signal was analyzed by using a digital oscilloscope. Emission quantum yields in solution were determined a combination of an integrating sphere, a monochromated xenon light source and a cooled CCD spectrometer.
Electrochemical Measurement
Cyclic voltammograms were conducted using an electrochemical analyzer, EC-stat 100 (EC Frontier CO., Ltd), using an electrochemical cell equipped with working (glassy carbon, φ = 3 mm), auxiliary (platinum wire), and reference (Ag/AgNO3, 10 mM) electrodes. The solution containing a Cu(I) complex (0.5 mM) and Et4NPF6 (0.1 M) was bubbled with argon before the measurements.
Materials
Cu(dppb), Cu(dppp), Cu(dppe), Re(bpy), and BIH were prepared according to reported methods with some modifications (Hawecker et al., 1986; Tamaki et al., 2013a; Tsubomura et al., 2015). Cu(Xantphos) were synthesized using dmpp instead of the corresponding diimine ligand according to the method for [Cu(2,2′-biquinoline)(Xantphos)](PF6) (McCullough et al., 2018). Other chemicals were used as purchased without further purification.
Photocatalytic Reactions
The photocatalytic reactions were performed in a 15 mL test tube (i.d. = 11 mm) containing a 2 mL DMA–TEOA (4:1 v/v) solution of Re(bpy) (0.05 mM), BIH (0.1 M), and a Cu(I) complex (0.5 mM) after purging with CO2 for more than 20 min. The solution was irradiated using a laboratory-made merry-go-round irradiation apparatus at λ > 370 nm with a high-pressure mercury lamp (SEN LIGHT Co.) combined with a UV-cut filter (Lintec Commerce. Inc.). During irradiation, the tube was cooled with a thermostatic bath (20°C). The gaseous reaction products (CO and H2) were analyzed using a GC-TCD instrument (Shimadzu GC-8A).
Measurement of the Absorption Spectrum of the OERS
A DMA–TEOA (4:1 v/v) solution (2 mL) containing the Cu(I) complex (0.1 mM) and BIH (0.1 M) in a 7 mL quartz cell (light-pass length: 1 cm) was purged with Ar for over 20 min. The solution was irradiated with 430-nm monochromic light derived from an LED lamp purchased from CELL System Co. UV-Vis absorption spectral changes during irradiation were recorded using a Shimadzu QYM-01 system.
Measurement of Quantum Yields of Photocatalytic CO Formation
A DMA–TEOA (4:1 v/v) solution (2 mL) containing Cu(dppb) (0.5 mM), Re(bpy) (0.05 mM), and BIH (0.1 M) in a quartz cell (light-pass length: 1 cm, volume: 7 mL, light intensity: 1 × 10−8 einstein per s) was purged with CO2 for 30 min. The solution was irradiated at λex = 430 nm derived from an LED lamp purchased from CELL System Co. UV-Vis absorption spectral changes during irradiation were recorded using a Shimadzu QYM-01 system. During irradiation, the cell was cooled at 25°C with a thermostatic bath. The gaseous reaction products (CO and H2) were analyzed using a GC-TCD instrument (Shimadzu GC-8A).
Conclusion
We investigated in detail the effects of the structure of the diphosphine ligands on the photosensitizing abilities of heteroleptic diimine-diphosphine Cu(I) complexes using a series of Cu(I) complexes bearing dmpp ligands and 4 types of diphosphine ligands by conducting photocatalytic CO2 reduction using the Cu(I) complexes as photosensitizers. Turnover frequencies of the CO2 reduction drastically increased up to ~70 min−1 with increase of the P-Cu-P angles, even though all of the Cu(I) complexes showed similar photophysical, photochemical and electrochemical properties. In the case using Cu(dppb), rapid accumulation of OERS was observed. On the contrary, when using Cu(dppe), the apparent rate of the production of the OERS was quite slow. The low efficiency for the production of the OERS should be derived from the rapid charge-recombination with BIH·+. The large bite angles make the orientation of the phenyl groups on the phosphorous atoms toward the dmpp ligands, resulting in suppression of the attack by BIH·+ to the dmpp ligands. This might be one of the reasons for the high efficiencies of both the production of OERS and photocatalytic reactions when using the Cu(I) complexes connecting with the diphosphine ligands with the large bite angles.
Author Contributions
YY and TT designed the study and YY wrote the initial draft of the manuscript. TO and TU investigated the photosensitizing abilities of the Cu(I) complexes. JI contributed to analyses of the structure of the Cu(I) complexes in solutions using NMR spectroscopy. SF measured the photophysical properties of the Cu(I) complexes in detail. The electrochemical properties of the Cu(I) complexes were checked by CT. All authors approved the final version of the manuscript, and agree to be accountable for all aspects of the work.
Funding
This work was supported by JSPS KAKENHI Grant Number 17K05816 and Grant from Seikei University.
Conflict of Interest Statement
The authors declare that the research was conducted in the absence of any commercial or financial relationships that could be construed as a potential conflict of interest.
Supplementary Material
The Supplementary Material for this article can be found online at: https://www.frontiersin.org/articles/10.3389/fchem.2019.00288/full#supplementary-material
References
Gholamkhass, B., Mametsuka, H., Koike, K., Tanabe, T., Furue, M., and Ishitani, O. (2005). Architecture of supramolecular metal complexes for photocatalytic CO2 reduction: ruthenium–rhenium Bi- and tetranuclear complexes. Inorg. Chem. 44, 2326–2336. doi: 10.1021/ic048779r
Hawecker, J., Lehn, J.-M., and Ziessel, R. (1983). Efficient photochemical reduction of CO2 to CO by visible light irradiation of systems containing Re(bipy)(CO)3X or Ru(bipy)-Co2+ combinations as homogeneous catalysts. J. Chem. Soc. Chem. Commun. 536–538. doi: 10.1039/C39830000536
Hawecker, J., Lehn, J.-M., and Ziessel, R. (1986). Photochemical and electrochemical reduction of carbon dioxide to carbon monoxide mediated by (2,2'-Bipyridine)tricarbonylchlororhenium(I) and related complexes as homogeneous catalysts. Helv. Chim. Acta 69, 1990–2012. doi: 10.1002/hlca.19860690824
Heberle, M., Tschierlei, S., Rockstroh, N., Ringenberg, M., Frey, W., Junge, H., et al. (2017). Heteroleptic copper photosensitizers: why an extended p-system does not automatically lead to enhanced hydrogen production. Chem. Eur. J. 23, 312–319. doi: 10.1002/chem.201604005
Kaeser, A., Mohankumar, M., Mohanraj, J., Monti, F., Holler, M., Cid, J. J., et al. (2013). Heteroleptic copper(I) complexes prepared from phenanthroline and bis-phosphine ligands. Inorg. Chem. 52, 12140–12151. doi: 10.1021/ic4020042
Kato, E., Takeda, H., Koike, K., Ohkubo, K., and Ishitani, O. (2015). Ru(II)–Re(I) binuclear photocatalysts connected by –CH2XCH2- (X = O, S, CH2) for CO2reduction. Chem. Sci. 6, 3003–3012. doi: 10.1039/c4sc03710c
Kiyosawa, K., Shiraishi, N., Shimada, T., Masui, D., Tachibana, H., Takagi, S., et al. (2009). Electron transfer from the porphyrin S2 state in a zinc porphyrin-rhenium bipyridyl dyad having carbon dioxide reduction activity. J. Phys. Chem. C 113, 11667–11673. doi: 10.1021/jp901548y
Koike, K., Naito, S., Sato, S., Tamaki, Y., and Ishitani, O. (2009). Architecture of supramolecular metal complexes for photocatalytic CO2 reduction III: effects of length of alkyl chain connecting photosensitizer to catalyst. J. Photochem. Photobiol. A 207, 109–114. doi: 10.1016/j.jphotochem.2008.12.014
Kou, Y., Nakatani, S., Sunagawa, G., Tachikawa, Y., Masui, D., Shimada, T., et al. (2014). Visible light-induced reduction of carbon dioxide sensitized by a porphyrin–rhenium dyad metal complex on p-type semiconducting NiO as the reduction terminal end of an artificial photosynthetic system. J. Catal. 310, 57–66. doi: 10.1016/j.jcat.2013.03.025
Kuang, S.-M., Cuttell, D. G., McMillin, D. R., Fanwick, P. E., and Walton, R. A. (2002). Synthesis and structural characterization of Cu(I) and Ni(II) complexes that contain the Bis[2-(diphenylphosphino)phenyl]ether ligand. Novel emission properties for the Cu(I) species. Inorg. Chem. 41, 3313–3322. doi: 10.1021/ic0201809
Kuramochi, Y., Ishitani, O., and Ishida, H. (2018). Reaction mechanisms of catalytic photochemical CO2 reduction using Re(I) and Ru(II) complexes. Coord. Chem. Rev. 373, 333–356. doi: 10.1016/j.ccr.2017.11.023
Kutal, C., Weber, M. A., Ferraudi, G., and Geiger, D. (1985). A mechanistic investigation of the photoinduced reduction of carbon dioxide mediated by tricarbonylbromo(2,2'-bipyridine)rhenium(I). Organometallics 4, 2161–2166. doi: 10.1021/om00131a016
Lang, P., Giereth, R., Tschierlei, S., and Schwalbe, M. (2019). Unexpected wavelength dependency of the photocatalytic CO2 reduction performance of the well-known (bpy)Re(CO)3Cl complex. Chem. Commun. 55, 600–603. doi: 10.1039/c8cc08742c
McCullough, B. J., Neyhouse, B. J., Schrage, B. R., Reed, D. T., Osinski, A. J., Ziegler, C. J., et al. (2018). Visible-light-driven photosystems using heteroleptic Cu(I) photosensitizers and Rh(III) catalysts to produce H2. Inorg. Chem. 57, 2865–2875. doi: 10.1021/acs.inorgchem.7b03273
McMillin, D. R., and McNett, K. M. (1998). Photoprocesses of copper complexes that bind to DNA. Chem. Rev. 98, 1201–1220. doi: 10.1021/cr9601167
Morimoto, T., Nishiura, C., Tanaka, M., Rohacova, J., Nakagawa, Y., Funada, Y., et al. (2013). Ring-shaped Re(I) multinuclear complexes with unique photofunctional properties. J. Am. Chem. Soc. 135, 13266–13269. doi: 10.1021/ja406144h
Morris, A. J., Meyer, G. J., and Fujita, E. (2009). Molecular approaches to the photocatalytic reduction of carbon dioxide for solar fuels. Acc. Chem. Res. 42, 1983–1994. doi: 10.1021/ar9001679
Nishikawa, M., Kakizoe, D., Saito, Y., Ohishi, T., and Tsubomura, T. (2017). Redox properties of Copper(I) complex bearing 4,7-Diphenyl-2,9-dimethyl-1,10-phenanthroline and 1,4-Bis(diphenylphosphino)butane ligands and effects of light in the presence of chloroform. Bull. Chem. Soc. Jpn. 90, 286–288. doi: 10.1246/bcsj.20160339
Ohkubo, K., Yamazaki, Y., Nakashima, T., Tamaki, Y., Koike, K., and Ishitani, O. (2016). Photocatalyses of Ru(II)–Re(I) binuclear complexes connected through two ethylene chains for CO2 reduction. J. Catal. 343, 278–289. doi: 10.1016/j.jcat.2015.12.025
Rohacova, J., and Ishitani, O. (2016). Rhenium(I) trinuclear rings as highly efficient redox photosensitizers for photocatalytic CO2 reduction. Chem. Sci. 7, 6728–6739. doi: 10.1039/c6sc01913g
Rohacova, J., and Ishitani, O. (2017). Photofunctional multinuclear rhenium(I) diimine carbonyl complexes. Dalton Trans. 46, 8899–8919. doi: 10.1039/c7dt00577f
Rosas-Hernández, A., Steinlechner, C., Junge, H., and Beller, M. (2017). Earth-abundant photocatalytic systems for the visible-light-driven reduction of CO2 to CO. Green Chem. 19, 2356–2360. doi: 10.1039/c6gc03527b
Sahara, G., and Ishitani, O. (2015). Efficient photocatalysts for CO2 reduction. Inorg. Chem. 54, 5096–5104. doi: 10.1021/ic502675a
Saito, K., Arai, T., Takahashi, N., Tsukuda, T., and Tsubomura, T. (2006). A series of luminescent Cu(I) mixed-ligand complexes containing 2,9-dimethyl-1, 10-phenanthroline and simple diphosphine ligands. Dalton Trans. 4444–4448. doi: 10.1039/b608641a
Sato, S., Koike, K., Inoue, H., and Ishitani, O. (2007). Highly efficient supramolecular photocatalysts for CO2 reduction using visible light. Photochem. Photobiol. Sci. 6, 454–461. doi: 10.1039/b613419j
Takeda, H., Cometto, C., Ishitani, O., and Robert, M. (2016a). Electrons, Photons, protons and earth-abundant metal complexes for molecular catalysis of CO2 reduction. ACS Catal. 7, 70–88. doi: 10.1021/acscatal.6b02181
Takeda, H., Kamiyama, H., Okamoto, K., Irimajiri, M., Mizutani, T., Koike, K., et al. (2018). Highly efficient and robust photocatalytic systems for CO2 reduction consisting of a Cu(I) photosensitizer and Mn(I) catalysts. J. Am. Chem. Soc. 140, 17241–17254. doi: 10.1021/jacs.8b10619
Takeda, H., Ohashi, K., Sekine, A., and Ishitani, O. (2016b). Photocatalytic CO2 reduction using Cu(I) photosensitizers with a Fe(II) catalyst. J. Am. Chem. Soc. 138, 4354–4357. doi: 10.1021/jacs.6b01970
Tamaki, Y., and Ishitani, O. (2017). Supramolecular photocatalysts for the reduction of CO2. ACS Catal. 7, 3394–3409. doi: 10.1021/acscatal.7b00440
Tamaki, Y., Koike, K., Morimoto, T., and Ishitani, O. (2013a). Substantial improvement in the efficiency and durability of a photocatalyst for carbon dioxide reduction using a benzoimidazole derivative as an electron donor. J. Catal. 304, 22–28. doi: 10.1016/j.jcat.2013.04.002
Tamaki, Y., Koike, K., Morimoto, T., Yamazaki, Y., and Ishitani, O. (2013b). Red-light-driven photocatalytic reduction of CO2 using Os(II)–Re(I) supramolecular complexes. Inorg. Chem. 52, 11902–11909. doi: 10.1021/ic4015543
Tamaki, Y., Watanabe, K., Koike, K., Inoue, H., Morimoto, T., and Ishitani, O. (2012). Development of highly efficient supramolecular CO2 reduction photocatalysts with high turnover frequency and durability. Faraday Discuss. 155, 115–127. doi: 10.1039/c1fd00091h
Tsubomura, T., Kimura, K., Nishikawa, M., and Tsukuda, T. (2015). Structures and photophysical properties of copper(I) complexes bearing diphenylphenanthroline and bis(diphenylphosphino)alkane: the effect of phenyl groups on the phenanthroline ligand. Dalton Trans. 44, 7554–7562. doi: 10.1039/c5dt00835b
Windle, C. D., and Perutz, R. N. (2012). Advances in molecular photocatalytic and electrocatalytic CO2 reduction. Coord. Chem. Rev. 256, 2562–2570. doi: 10.1016/j.ccr.2012.03.010
Yamazaki, Y., and Ishitani, O. (2018). Synthesis of Os(II)–Re(I)–Ru(II) hetero-trinuclear complexes and their photophysical properties and photocatalytic abilities. Chem. Sci. 9, 1031–1041. doi: 10.1039/c7sc04162d
Yamazaki, Y., Takeda, H., and Ishitani, O. (2015). Photocatalytic reduction of CO2 using metal complexes. J. Photochem. Photobiol. C 25, 106–137. doi: 10.1016/j.jphotochemrev.2015.09.001
Zhang, Y., Schulz, M., Wächtler, M., Karnahl, M., and Dietzek, B. (2018). Heteroleptic diimine–diphosphine Cu(I) complexes as an alternative towards noble-metal based photosensitizers: design strategies, photophysical properties and perspective applications. Coord. Chem. Rev. 356, 127–146. doi: 10.1016/j.ccr.2017.10.016
Keywords: CO2 reduction, photocatalytic reaction, Cu(I) complexes, redox-photosensitizer, diphosphine ligands
Citation: Yamazaki Y, Onoda T, Ishikawa J, Furukawa S, Tanaka C, Utsugi T and Tsubomura T (2019) Photocatalytic CO2 Reduction Using Various Heteroleptic Diimine-Diphosphine Cu(I) Complexes as Photosensitizers. Front. Chem. 7:288. doi: 10.3389/fchem.2019.00288
Received: 30 January 2019; Accepted: 08 April 2019;
Published: 30 April 2019.
Edited by:
Hitoshi Ishida, Kitasato University, JapanReviewed by:
Yi Du, University of Wollongong, AustraliaFrancesco Nastasi, University of Messina, Italy
Copyright © 2019 Yamazaki, Onoda, Ishikawa, Furukawa, Tanaka, Utsugi and Tsubomura. This is an open-access article distributed under the terms of the Creative Commons Attribution License (CC BY). The use, distribution or reproduction in other forums is permitted, provided the original author(s) and the copyright owner(s) are credited and that the original publication in this journal is cited, in accordance with accepted academic practice. No use, distribution or reproduction is permitted which does not comply with these terms.
*Correspondence: Taro Tsubomura, dHN1Ym9tdXJhQHN0LnNlaWtlaS5hYy5qcA==