- 1Laboratory of Peptide and Protein Chemistry and Biology (PeptLab), Sesto Fiorentino, Italy
- 2Department of Chemistry “Ugo Schiff”, University of Florence, Sesto Fiorentino, Italy
- 3PeptLab@UCP Platform and Laboratory of Chemical Biology EA4505, Université Paris-Seine, Cergy-Pontoise, France
- 4European Laboratory for Non-Linear Spectroscopy (LENS), Sesto Fiorentino, Italy
- 5Laboratory of Biomolecules, CNRS, Sorbonne University, Ecole Normale Superieure, PSL University, Paris, France
The insertion of azobenzene moiety in complex molecular protein or peptide systems can lead to molecular switches to be used to determine kinetics of folding/unfolding properties of secondary structures, such as α-helix, β-turn, or β-hairpin. In fact, in azobenzene, absorption of light induces a reversible trans ↔ cis isomerization, which in turns generates a strain or a structure relaxation in the chain that causes peptide folding/unfolding. In particular azobenzene may permit reversible conformational control of hairpin formation. In the present work a synthetic photochromic azobenzene amino acid derivative was incorporated as a turn element to modify the synthetic peptide [Pro7,Asn8,Thr10]CSF114 previously designed to fold as a type I β-turn structure in biomimetic HFA/water solution. In particular, the P-N-H fragment at positions 7–9, involved in a β-hairpin, was replaced by an azobenzene amino acid derivative (synthesized ad hoc) to investigate if the electronic properties of the novel peptidomimetic analog could induce variations in the isomerization process. The absorption spectra of the azopeptidomimetic analog of the type I β-turn structure and of the azobenzene amino acid as control were measured as a function of the irradiation time exciting into the respective first ππ* and nπ* transition bands. Isomerization of the azopeptidomimetic results strongly favored by exciting into the ππ* transition. Moreover, conformational changes induced by the cis↔ trans azopeptidomimetic switch were investigated by NMR in different solvents.
Introduction
Azobenzene has been recognized as a potential molecular photoswitch in various fields, such as polymer science, material science, chemistry, and life sciences (Marchi et al., 2012; Goulet-Hanssens and Barrett, 2013; Dong et al., 2015; Bushuyev et al., 2018; Miniewicz et al., 2018). Azobenzene amino acids protected for solid phase synthesis proposed by Sewald et al. opened possibility the for incorporation into photoswitchable peptides (Juodaityte and Sewald, 2004; Renner and Moroder, 2006; Aemissegger and Hilvert, 2007). In particular, compounds containing a methyl spacer between the phenyl ring and the amino group, as [3-(3-aminomethyl)-phenylazo]phenylacetic acid (AMPP) acid or (4-aminomethyl)phenylazobenzoic acid (AMPB), give more flexibility to the chemical structure of a Xaa–AMPB–Yaa fragment than the rigid chromophore, 4-[(4-amino)phenylazo]benzoic acid (APB).
When inserted into larger molecular systems, like protein chains or peptides, azobenzene can be used as a molecular switch and/or as a probe to determine kinetics of folding/unfolding of the corresponding secondary structure, like α-helix, β-turn, or β-hairpin (Behrendt et al., 1999; Kumita et al., 2000; Renner et al., 2000; Spörlein et al., 2002; Komarov et al., 2018). In fact, absorption of light by azobenzene induces a reversible trans ↔ cis isomerization, which in turns generates a strain or a structure relaxation in the chain that causes peptide unfolding/folding. The process can be easily monitored looking at the absorption spectrum of azobenzene itself, that is strongly different in the two forms, trans and cis (Schultz et al., 2003; Satzger et al., 2004; Quick et al., 2014). In particular, the AMPP chromophore incorporation in a peptide sequence led to a β-hairpin structure after irradiation (Dong et al., 2006, 2017; Rampp et al., 2018), and the AMPB was described as a trigger molecule in cyclic peptide structures (Ulysse et al., 1995; Renner et al., 2005).
When a chromophore unit is integrated into a linear or cyclic peptide, the trans ↔ cis isomerization of an azobenzene derivative induced by UV/VIS photo-irradiation, has been demonstrated to induce a reversible change in the peptide structure modulating its biological activity (Ali et al., 2015). For example azobenzene amino acid has been reported for antigen-antibody photocontrol (Beharry et al., 2008; Parisot et al., 2009). In a cyclic polypeptide ligand, azobenzene trans ↔ cis isomerization induced drastic changes in recognition by neural NO synthase leading to reversible photocontrol of muscle fibers (Hoppmann et al., 2011). Finally, in an amyloid azobenzene containing peptide, isomerization plays an active role in self-assembly into β-amyloid fibrils (Deeg et al., 2011).
β-Hairpins are very interesting structures as they are involved in many biological processes i.e., often constitute binding epitopes and are implied in protein–protein or protein–DNA interactions (Hillier et al., 1999; Gajiwala et al., 2000; Wong et al., 2000; Schumacher et al., 2001; Zavala-Ruiz et al., 2004). Thus, the development of highly stable β-hairpins based on introduction of molecules as azobenzene allowed to control the hairpin structure and initiate a folding or unfolding transition with high isomerization yield, remarkable photostability, and ultra-fast kinetics.
In previous studies, the family of structure-based designed β-hairpin peptides termed CSF114(Glc) has been developed to expose aberrant post-translational modifications (PTMs) to characterize antibodies as biomarkers of autoimmune diseases in patient sera (Lolli et al., 2005a,b; Papini, 2009; Pandey et al., 2012). In fact, we demonstrated that the β-turn structure is crucial for the correct exposure of the PTM and allows a specific and high affinity antibody interaction in the context of solid-phase immunoenzymatic assays (Carotenuto et al., 2006, 2008). In this work, a modified sequence of [Pro7,Asn8,Thr10]CSF114 was selected as an optimized type I β-turn structure. Aim of the present work is the design and synthesis of a photocontrolled probe, based on AMPB azobenzene as a turn element in the central part of the amino acid sequence, to investigate if the electronic properties of the new molecule could induce variations in the isomerization process of the azobenzene unit and to study the effect of the photoswitch on its conformation.
Materials and Methods
Reagents
All Fmoc-protected amino acids, Fmoc-Wang resins, DIC (N,N′-Diisopropylcarbodiimide), and Oxyma were purchased from Iris Biotech GmbH (Marktredwitz, Germany). The following amino acid side-chain-protecting groups were used: OtBu (Asp, Glu), tBu (Ser, Thr), Pbf (Arg), Trt (Gln, His), and Boc (Lys). Peptide-synthesis grade N,N-dimethylformamide (DMF) was purchased from Scharlau (Barcelona, Spain); acetonitrile (ACN) from Carlo Erba (Milan, Italy); dichloromethane (DCM), trifluoroacetic acid (TFA), piperidine were purchased from Sigma-Aldrich (Milan, Italy).
MW-Assisted Solid-Phase Peptide Synthesis
The azopeptide 1 was synthesized by microwave-assisted solid-phase peptide synthesis (MW-SPPS) following the Fmoc/tBu strategy, using the Liberty Blue™ automated microwave peptide synthesizer (CEM Corporation, Matthews, NC, USA) following the protocol described elsewhere (Rizzolo et al., 2011). The resin used was a Fmoc-Lys(Boc)-Wang (loading 0.24 mmol/g). Modified amino acids were introduced using the synthesized protected building-blocks suitable for Fmoc/tBu SPPS (Paolini et al., 2007; Rentier et al., 2015). Coupling was performed with the azobenzene amino acid AMPB (2.5 eq), HATU as activator (2.5 eq), and DIPEA (3.5 eq) for 30 min at room temperature. Uncertain peptide coupling steps were checked by the ninhydrin test as described by Kaiser (Kaiser et al., 1970), or micro-cleavages performed with a microwave apparatus CEM Discover™ single-mode MW reactor (CEM Corporation, Matthews, NC, USA). Final cleavage was performed using a mixture of TFA/TIS/H2O (95:2.5:2.5 v:v:v) for 3 h at room temperature.
The crude azopeptide was pre-purified by Reverse Phase Liquid Chromatography (RP-HPLC) using a Li-Chroprep C-18 column on an Armen Instrument (Armen Instrument, Saint-Avé, France) working at 20 ml/min with H2O (MilliQ) and CH3CN as solvent systems. The second step of purification was performed by semipreparative RP-HPLC on a Waters instrument (Separation Module 2,695, detector diode array 2,996) using a Phenomenex (Torrance, CA, USA) Jupiter column C18 (10 μm, 250 × 10 mm), at 4 mL/min with solvent systems A (0.1% TFA in H2O) and B (0.1% TFA in CH3CN).
The azopeptide 1 was characterized by RP-HPLC-ESI-MS, obtaining a final purity ≥ 98% (Figure S1). HPLC: tr = 3.17 min (cis isomer) and 3.78 min (trans isomer), gradient 35–55% of B in 5 min; Mr = calcd. for C116 H170 N29 O25 S1: 2402,88 ESI-MS: m/z: 1202,47 [M+2H]2+; 802,02 [M+3H]3+ RP-HPLC system is an Alliance Chromatography (Waters, Milford Massachusetts, USA) with a Bioshell A160 C18 (Sigma Aldrich, Milano Italy; 1.7 μm 2.1 × 50 mm) column at 35°C, at 0.6 mL/min coupled to a single quadrupole ESI-MS Micromass ZQ (Waters, Milford Massachusetts, USA). The solvent systems used were A (0.1% TFA in H2O) and B (0.1% TFA in CH3CN). Peptides were lyophilized using an Edward Modulyo lyophilizer (Richmond scientific Ldt., Lancashire, Great Britain).
UV/Vis Spectra Experiments
The experimental set up used for the irradiation procedure consists of a Xe “Ozone free” Orion lamp emitting 450 W in the spectral range 200–2,000 nm. The light is focalized through a lens (f = 200 mm) onto the entrance slit of a monochromator. The exit beam, having 1 nm bandwith, is shaped by means of a pin-hole and collimated with a 50 mm lens on the sample cuvette (quartz, 1 cm optical path length). A magnetic stirrer placed inside the cuvette ensures that the irradiating beam always interacts with fresh solution. Incident beam cross section has been estimated 0.8 × 0.8 cm2. Incident power has been measured with a Coherent Field Max II power meter. The power we used for the irradiation was around 500 μW for all the three excitation wavelengths used 313, 440, and 330 nm. Absorption spectra have been obtained with a Varian Cary 5 spectrophotometer, with 2 nm bandwith resolution.
Azobenzene was purchased by Sigma-Aldrich (purity 98%). [(4-aminomethylphenyl)diazenyl] phenylacetic acid was synthesized as described by Juodaityte and Sewald (2004) and azopeptide 1 was synthesized as described. Solution concentrations were all around 10−4 M, using ethanol (Merck, purity grade: Uvasol) as a solvent. Unknown molar extinctions were determined for the new synthetic azopeptide 1 in the trans form (amino derivative εt,(326) = 9,000 cm−1M−1; aminopeptide εt,(330) = 5,090 cm−1M−1). The extinctions of the corresponding cis form was calculated considering still valid the ratio εcis/εtrans in azobenzene 3.
NMR Experiments
NMR experiments were recorded on a 500 MHz Bruker Avance III spectrometer (Wissembourg, France) equipped with a TCI 1H/13C/15N cryoprobe. The lyophilized azopeptide 1 was dissolved in 550 μL of 50% TFE-d3/50% H2O, 50% TFE-d3/50% D2O, or 50% ACN-d3/50% H2O at ~0.5 mM concentration for experiments before irradiation. For induction of azobenzene trans ↔ cis isomerization, the sample was irradiated in 100% TFE-d3 or 50% ACN-d3/50% H2O with a VL-6.L UV-lamp (Vilber, Germany) emitting 6 W at 365 nm for 1–5 h. Suitable volumes of solvents were added after irradiation to recover the same solvent conditions as for experiments before irradiation. 1H and 13C resonance assignments were obtained from the analysis of 2D 1H-1H TOCSY (DIPSI2 isotropic scheme of 66 ms duration), 1H-1H NOESY (200 or 400 ms mixing time), 1H-1H ROESY (250, 300, or 400 ms mixing time), 1H-13C HSQC, and 1H-13C HSQC-TOCSY. NMR experiments were processed with TOPSPIN 3.5 (Bruker) and analyzed with NMRFAM-Sparky program (Lee et al., 2015). NMR chemical shifts were calibrated with respect to the residual protiated solvent signal on 1D 1H or 2D 1H-13C HSQC experiments.
Results and Discussion
In this work we designed, synthesized and studied the reversible cis ↔ trans photoisomerization of the [Pro7,Asn8,Thr10]CSF114 analog peptide 1, where from the original sequence the P-N-H tripeptide was replaced by the photoswitch (4-aminomethyl)phenylazobenzoic acid (AMPB). The photoisomerization of the synthetic azopeptide 1 was explored and its reversibility was compared with more simple systems, the azobenzene amino acid 2 and the azobenzene 3.
Design of the azopeptide 1. In the literature photochromic compounds, i.e., azobenzene, have been reported as molecules able to isomerize reversibly, when exposed to light of appropriate wavelength.
The 21-mer peptide [Pro7,Asn8,Thr10]CSF114, derived from the family of structure-based designed β-turn peptides termed CSF114(Glc), is characterized by a type I β-turn motif (Carotenuto et al., 2006, 2008). In this sequence the β-turn structure was shown around the proline and asparagine residues in positions 7-8. The role of conformation in the recognition and binding of this synthetic antigenic probe to autoantibodies in the context of an immunoenzymatic assay (ELISA) was previously determined to be fundamental.
Because of the importance of the conformation and of the correct exposure of epitopes involved in autoantibody recognition, the light-induced conformational change of the synthetic peptide [Pro7,Asn8,Thr10]CSF114, after the introduction of the azobenzene moiety into the sequence is the object of the present study (Figure 1). Starting from the [Pro7,Asn8,Thr10]CSF114 sequence, the P-N-H segment was targeted for replacement by an AMPB azobenzene amino acid, as a turn element.
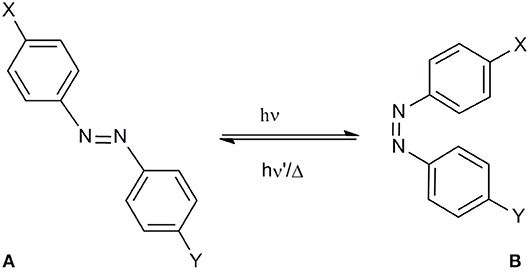
Figure 1. (A) azopeptide 1 (X = T-P-R-V-E-R-;Y = T-V-F-L-A-P-Y-G-W-M-V-K); trans-(4-aminomethyl)phenylazobenzoic (AMPB) acid (X = CH2NH2,Y = CH2COOH) 2; trans-azobenzene (X,Y = H) 3, (B) their possible cis conformation.
The photoswitch (4-aminomethyl)phenylazobenzoic acid (AMPB) was obtained by the condensation of a 4-nitrophenylacetic acid with 4-aminobenzylamine as described previously (Ulysse and Chmielewski, 1994; Juodaityte and Sewald, 2004; Aemissegger et al., 2005). The amino function of 4-aminobenzylamine was protected as Fmoc to obtain 4-[2-[4-[[[(9H-fluorenyl-9-methoxy)carbonyl]amino]methyl]phenyl]diazenyl]benzenacetic acid to be used in Fmoc solid-phase peptide synthesis. Its incorporation into the peptide sequence proceeded into a straightforward manner applying the standard Fmoc-solid phase methodology, using HATU as coupling reagent. The synthesis of the azobenzene-containing peptide 1 was carried out on a 0.1 mmol scale following the standard Fmoc/tBu solid phase peptide synthesis (SPPS) starting from Fmoc-Lys(Boc)-Wang resin. After coupling the amino acids of the sequence protected for SPPS, the peptide was cleaved from the resin and purified and characterized by RP-HPLC coupled to Mass Spectrometry. The ability of AMPB to induce variations in the isomerization process was elucidated by UV-Vis and NMR.
The Azobenzene Peptide as Molecular Switch
In order to propose the synthetic azobenzene as a molecular switch, a deep characterization of its response to light excitation is mandatory to fully understand the effect of substituents on isomerization (Figure 2).
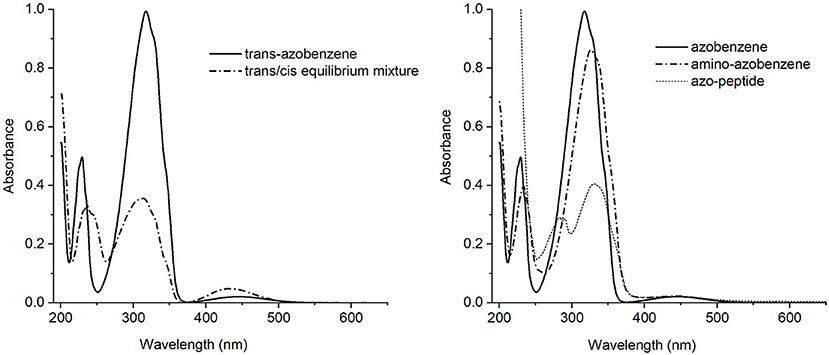
Figure 2. Left side: UV-Vis absorption spectra of the trans-azobenzene 3 (solid line) and the equilibrium mixture trans/cis (dash-dotted line). Right side: UV-Vis absorption spectra of the trans isomers of azobenzene 3 (solid line), aminoazobenzene 2 (dash-dotted line) and azopeptide 1 (short dash line).
The trans-azobenzene 3 spectrum showed in Figure 2 (left), is characterized by a strong absorption centered around 317 nm and a medium one at 230 nm, both assigned as ππ* electronic transitions. At longer wavelength, about 440 nm, a weak band is observed due to the nπ* state. Appearance of the cis form is revealed by the intensity decrease of the main band and a simultaneous growing of a medium intensity absorption at 238 nm.
The absorption spectra of the trans isomers of amino-azobenzene 2 and azopeptide 1 are shown in Figure 2 (right). While the correspondence of the electronic transitions is maintained, a small red shift of the bands is observed in the amino-derivative probably due to a moderate conjugation and/or electron-donor effect induced by the substituents on the aromatic rings. In the azopeptide 1, on the blue side of the aromatic ππ* transition whose maximum is red-shifted at 330 nm, the characteristic absorption of tryptophan is also observed at 290 nm.
Addition of substituents to azobenzene affects the electronic distribution and can also modify the trans-cis isomerization process and its photochemical yields (Crecca and Roitberg, 2006; Bandara and Burdette, 2012). Therefore, the absorption spectra of the three compounds have been measured as a function of the irradiation time, exciting into the respective first ππ* and nπ* transition bands. For all the three examined compounds, the trans→cis conversion is more effective when excitation is performed into the maximum of the ππ* band rather than into the nπ*. For this reason we focused our attention only on the trans→cis conversion obtained exciting into the ππ* absorption band (Figure S2). The resulting absorption spectra of azobenzene, aminoazobenzene and azopeptide are shown in Figures 3A–C, respectively.
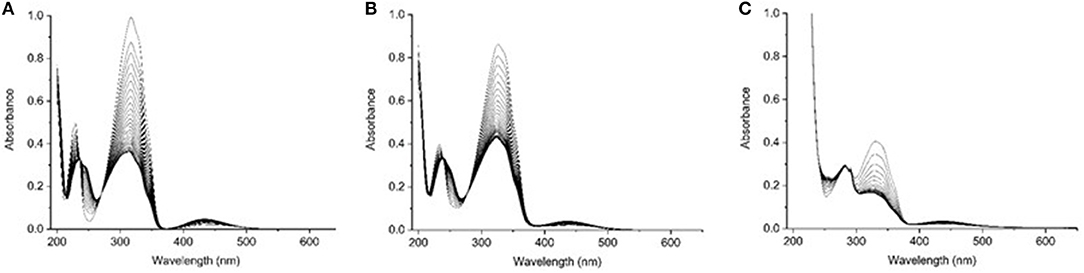
Figure 3. UV-Vis absorption spectra at several irradiation time for (A) azobenzene 3, (B) aminoazobenzene 2, and (C) azopeptide 1, exciting at the wavelength of the absorption maxima (313 nm for azobenzene and aminoazobenzene, and 330 nm for the azopeptide 1).
In the absorption spectra, isosbestic points are observed, indicating the presence of the trans-cis equilibrium in solution. Azobenzene absorption spectrum shows up to four isosbestic points, at 373, 270, 236, and 213 nm. On the opposite the azopeptide 1 shows only one isosbestic point at longer wavelength, due to the overlap in the blue side of the spectrum, with the large tryptophan absorption. In all three cases, after some time, a photostationary state is obtained, where the trans→cis and cis→trans isomerization rates equalize and no further variation is observed in the absorption intensity.
In Figure 4, the absorption maxima are plotted as a function of the irradiation time at those wavelengths. From this graph, quantitative information can be gained about the isomerization kinetics and the relative photochemical yields, following known kinetic procedure (Zimmermann et al., 1958; Bortolus and Monti, 1979).
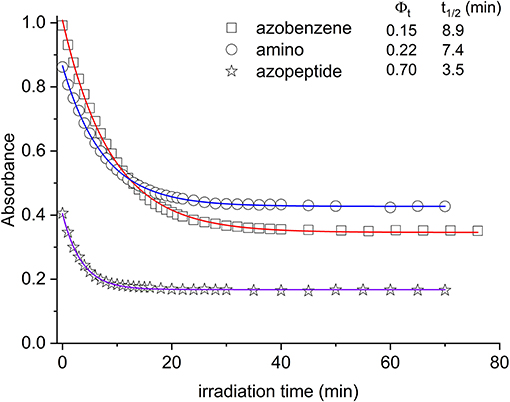
Figure 4. Absorbance decrease of the ππ* transition as a function of the irradiation time (azobenzene, squares; aminoazobenzene, circles; azopeptide 1, stars). The time evolution is due to the conversion between the trans and cis forms. In the inset the photoconversion quantum yields are reported, along with the respective decay times obtained by fitting the absorbance data (solid lines in the graph).
In Figure 4, the absorption maxima are plotted as a function of the irradiation time at those wavelengths. From this graph, quantitative information can be gained about the isomerization kinetics and the relative photochemical yields, following known kinetic procedure (Zimmermann et al., 1958; Bortolus and Monti, 1979). In this respect, we have considered the kinetic equation:
where [cis] = C0·Y, being C0 the initial molar concentration of the trans isomer and Y the cis molar fraction appearing in time. Φt is the photochemical yield of the trans→cis reaction, while Φc of the cis→trans one. k is the constant relative to the thermic isomerization. Since this last mechanism is much slower than the photochemistry, it can be neglected in our experiment (Zimmermann et al., 1958). Iλ is the power density absorbed by the trans/cis isomer. After some manipulations, the kinetic equation assumes the following aspect:
where εt is the extinction coefficient of the trans form, F = A/(1–10−A), being A the absorbance plotted in Figure 4. This last equation may be integrated giving a function whose time dependence is linear. The slope m of this line is related to the photochemical yield of the trans isomer (Zimmermann et al., 1958):
By using this kinetic analysis, the photochemical yield Φt shown in the inset of Figure 4 is obtained.
In agreement with previous data (Bortolus and Monti, 1979; Siampiringue et al., 1987; Satzger et al., 2004), azobenzene photochemical yield in ethanol was found to be 0.15. In the amino derivative 2, the ring substitution has the effect to increase the yield to 0.22. The major change is observed in the case of azopeptide 1, where the isomerization results strongly favored giving a yield of 0.70.
Adding electron-donor or electron-attractor groups to the aromatic rings alters the isomerization process due to the modification of the electron density on the molecule (Crecca and Roitberg, 2006; Bandara and Burdette, 2012). In the amino derivative AMBP, the presence of CH2 spacers reduces the electron-donor effect of the amino groups. Nevertheless, a small change in the isomerization yield is observed. Also in the azopeptide 1, the ring substituents are bonded through CH2 spacers. However a strong increase in the trans–cis quantum yield value is observed according to a faster isomerization kinetics (Yamamura et al., 2014). Such a different behavior can then be ascribed to some effective interactions involving the amino acid residues of the side chains, which stabilize the cis form of the azobenzene moiety. However, from the absorption data, the nature of this interaction cannot be known. The NMR data suggest the presence of interactions between the azobenzene aromatic protons and the side chains of amino acid residues, influencing the isomerization kinetic and the thermodynamic parameters (Renner et al., 2000).
Further investigations on thermal cis→trans reconversion kinetics confirm the stability of the final compound, accordingly to the NMR results. In fact, keeping irradiated solutions of the azopeptide 1 48 h in the dark at 50°C, only half trans form is thermally recovered, while for azobenzene and α-helix short peptide chain derivatives the recovery is complete, on this time scale, also at room temperature (Kumita et al., 2000).
Conformational Analysis
The effects of azobenzene trans ↔ cis isomerization on azopeptide 1 (Figure 5) conformation were investigated by NMR under different solvent conditions. Azopeptide 1 was found to be poorly soluble in water and organic solvents such as methanol and acetonitrile. Azopeptide was soluble in DMSO but exhibited poor spectrum quality with large HN signals. A previous NMR study of CSF114 analogs exhibiting β-hairpin propensity was carried out in HFA:water mixture (1:1) to stabilize folded conformations (Carotenuto et al., 2008). However, in the case of azopeptide 1, this solvent condition led to broad NMR signals that precluded conformational studies. We then turned to trifluoroethanol (TFE) as a different fluorinated cosolvent, which is commonly used to stabilize peptide structures. TFE/water 1:1 mixture yielded satisfactory quality of NMR spectra (Figures S3, S4). The stabilization of secondary structures by TFE is well-known for α-helices (Roccatano et al., 2002) but far less documented for β-hairpin structures (Blanco et al., 1994). Therefore, we also decided to investigate the use of ACN/water 1:1 mixture to analyze the effect of organic cosolvent on azopeptide folding.
Complete 1H assignments of the trans form were obtained both in TFE/water 1:1 and ACN/water 1:1 mixtures, using 2D TOCSY and 2D NOESY (or ROESY) experiments (Tables S1, S3, supporting information). Upon irradiation, the resonances of the cis form was observed on NMR spectra, with a population reaching 75–80% after 5 h irradiation. The cis→trans conversion was slow enough (>2 weeks) to record NMR spectra over several days, enabling to fully assign the cis form (Tables S2, S4). The cis form was characterized by a large (~1 ppm) upfield shift of aromatic meta resonances (with respect to methylene group) of azobenzene moiety, confirming the trans→cis isomerization of the N = N azo-bond (Figure S4).
A comparison of backbone Hα and HN chemical shift differences between cis and trans forms is shown in Figure 6, for both solvents. Large chemical shift variation is observed for the azobenzene group and neighboring residues Arg6 and Thr9. Interestingly, more distant residues in the two peptide arms are also affected, mostly in segment 9-13 and 4-5. Similar trends are observed under both solvent conditions, albeit to a lesser extent in ACN/water. The chemical shift changes can be ascribed to magnetic susceptibility anisotropy effects as the two aromatic groups get closer in space and/or to conformational effects.
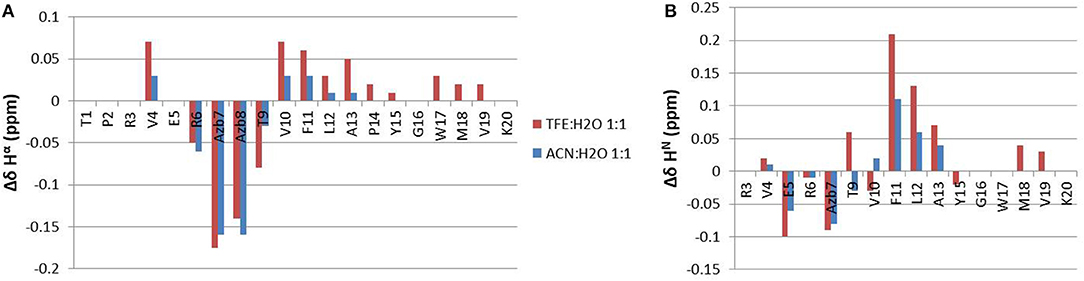
Figure 6. Δδ chemical shift differences between the cis and the trans forms of azopeptide 1, calculated for Hα (A) and HN protons (B).
The analysis of backbone and sequential NOEs reveals the presence of complex equilibria between extended and turn/helical folded conformations, in both cis and trans forms. HN-Hα sequential NOEs are stronger than intraresidual ones, indicating that extended backbone conformations are largely populated. However, the observation of sequential HN-HN NOEs (V4/E5, E5/R6, Y15/G16, G16/W17 in particular) can be ascribed to turn or helical conformations. These folded conformations are further supported by weak NOEs of azobenzene aromatic protons with methyl protons of Val4 and Leu12. Importantly, no NOEs could be detected between the two peptide arms in both forms. This result is in agreement with the observation that the trans→cis isomerization does not stabilize a β-hairpin structure.
Conclusion
In this work photoisomerization of the azopeptide 1 was explored and its reversibility was compared with more simple systems, such as azobenzene amino acid 2 and azobenzene 3. To this aim azopeptide 1 was modified introducing the photoswitch (4-aminomethyl)phenylazobenzoic acid (AMPB) to replace in [Pro7,Asn8,Thr10]CSF114 the P-N-H tripeptide on the tip of the β-hairpin.
The absorption spectra of azobenzene 3, AMPB amino acid 2, and azopeptide 1 were measured as a function of irradiation time, exciting into the ππ* band. The major differences are observed in the case of 1, where the isomerization results favored by exciting into the ππ* transition and the corresponding cis isomer results strongly stabilized.
Detailed NMR structural studies of azopeptide 1 confirmed that the AMPB chromophore insertion into the sequence allowed reversible control of peptide conformation in solution, but the trans→cis isomerization does not stabilize a β-hairpin structure, characteristic of the original sequence. Thus, incorporation of different photocontrolled switches, such as AMPP, will require further investigations to verify their possible role in controlling β-hairpin conformations.
Data Availability
No datasets were generated or analyzed for this study.
Author Contributions
FN designed the experiments, performed the synthesis, interpreted the data, and wrote the manuscript. CG, RC, PRS, and GP designed, performed and interpreted the data of the UV/Vis experiments, wrote the manuscript. ML and OL designed, performed and interpreted the data of the NMR experiments, wrote the manuscript. LS provided technical support to the experiments. AMP developed the project, designed the experiments, interpreted the data, and wrote the manuscript.
Funding
This work was supported by the Fondazione Ente Cassa di Risparmio Firenze (grant n. 2014.0306).
Conflict of Interest Statement
The authors declare that the research was conducted in the absence of any commercial or financial relationships that could be construed as a potential conflict of interest.
Supplementary Material
The Supplementary Material for this article can be found online at: https://www.frontiersin.org/articles/10.3389/fchem.2019.00180/full#supplementary-material
References
Aemissegger, A., and Hilvert, D. (2007). Synthesis and application of an azobenzene amino acid as a light-switchable turn element in polypeptides. Nat. Protoc. 2, 161–167. doi: 10.1038/nprot.2006.488
Aemissegger, A., Kräutler, V., van Gunsteren, W. F., and Hilvert, D. (2005). A photoinducible beta-hairpin. J. Am. Chem. Soc. 127, 2929–2936. doi: 10.1021/ja0442567
Ali, A. M., Forbes, M. W., and Woolley, G. A. (2015). Optimizing the photocontrol of bZIP coiled coils with azobenzene crosslinkers: role of the crosslinking site. Chembiochem 16, 1757–1763. doi: 10.1002/cbic.201500191
Bandara, H. M., and Burdette, S. C. (2012). Photoisomerization in different classes of azobenzene. Chem. Soc. Rev. 41, 1809–1825. doi: 10.1039/c1cs15179g
Beharry, A. A., Sadovski, O., and Woolley, G. A. (2008). Photo-control of peptide conformation on a timescale of seconds with a conformationally constrained, blue-absorbing, photo-switchable linker. Org. Biomol. Chem. 6, 4323–4332. doi: 10.1039/b810533b
Behrendt, R., Renner, C., Schenk, M., Wang, F., Wachtveitl, J., Oesterhelt, D., and Moroder, L. (1999). Photomodulation of the conformation of cyclic peptides with azobenzene moieties in the peptide backbone. Angew. Chem. Int. Ed Engl. 38, 2771–2774.
Blanco, F. J., Jiménez, M. A., Pineda, A., Rico, M., Santoro, J., and Nieto, J. L. (1994) NMR solution structure of the isolated N-terminal fragment of protein-G B1 domain. Evidence of trifluoroethanol induced native-like beta-hairpin formation. Biochemistry 33, 6004–6014
Bortolus, P., and Monti, S. (1979). Cis-trans photoisomerization of azobenzene. Solvent and triplet donors effects. J. Phys. Chem. 83, 648–652. doi: 10.1021/j100469a002
Bushuyev, O. S., Aizawa, M., Shishido, A., and Barrett, C. J. (2018). Shape-shifting azo dye polymers: towards sunlight-driven molecular devices. Macromol. Rapid Commun. 39:1700253. doi: 10.1002/marc.201700253
Carotenuto, A., Alcaro, M. C., Saviello, M. R., Peroni, E., Nuti, F., Papini, A. M., Novellino, E., and Rovero, P. (2008). Designed glycopeptides with different beta-turn types as synthetic probes for the detection of autoantibodies as biomarkers of multiple sclerosis. J. Med. Chem. 51, 5304–5309. doi: 10.1021/jm800391y
Carotenuto, A., D'Ursi, A. M., Mulinacci, B., Paolini, I., Lolli, F., Papini, A. M., Novellino, E., and Rovero, P. (2006). Conformation-activity relationship of designed glycopeptides as synthetic probes for the detection of autoantibodies, biomarkers of multiple sclerosis. J. Med. Chem. 49, 5072–5079. doi: 10.1021/jm060117j
Crecca, C. R., and Roitberg, A. E. (2006). Theoretical study of the isomerization mechanism of azobenzene and disubstituted azobenzene derivatives. J. Phys. Chem. A. 110, 8188–8203. doi: 10.1021/jp057413c
Deeg, A. A., Schrader, T. E., Kempter, S., Pfizer, J., Moroder, L., and Zinth, W. (2011). Light-triggered aggregation and disassembly of amyloid-like structures. Chemphyschem 12, 559–562. doi: 10.1002/cphc.201001012
Dong, M., Babalhavaeji, A., Collins, C. V., Jarrah, K., Sadovski, O., Dai, Q., and Woolley, G. A. (2017). Near-infrared photoswitching of azobenzenes under physiological conditions. J. Am. Chem. Soc. 139, 13483–13486. doi: 10.1021/jacs.7b06471
Dong, M., Babalhavaeji, A., Samanta, S., Beharry, A. A., and Woolley, G. A. (2015). Red-shifting azobenzene photoswitches for in vivo use. Acc. Chem. Res. 48, 2662–2670. doi: 10.1021/acs.accounts.5b00270
Dong, S. L., Löweneck, M., Schrader, T. E., Schreier, W. J., Zinth, W., Moroder, L., and Renner, C. (2006) A photocontrolled b-hairpin peptide. Chem. Eur. J. 12, 1114–1120. doi: 10.1002/chem.200500986
Gajiwala, K. S., Chen, H., Cornille, F., Roques, B. P., Reith, W., Mach, B., and Burley, S. K. (2000). Structure of the winged-helix protein hRFX1 reveals a new mode of DNA binding. Nature 403, 916–921. doi: 10.1038/35002634
Goulet-Hanssens, A., and Barrett, C. J. (2013) Photo-control of biological systems with azobenzene polymers. J. Polym. Sci. Part A Polym. Chem. 51, 3058–3070. doi: 10.1002/pola.26735
Hillier, B. J., Christopherson, K. S., Prehoda, K. E., Bredt, D. S., and Lim, W. A. (1999). Unexpected modes of PDZ domain scaffolding revealed by structure of nNOS-syntrophin complex. Science 284, 812–815. doi: 10.1126/science.284.5415.812
Hoppmann, C., Schmieder, P., Domaing, P., Vogelreiter, G., Eichhorst, J., Wiesner, B., Morano, I., Rück-Braun, K., and Beyermann, M. (2011). Photocontrol of contracting muscle fibers. Angew. Chem. Int. Ed Engl. 50, 7699–7702. doi: 10.1002/anie.201101398
Juodaityte, J., and Sewald, N. (2004). Synthesis of photoswitchable amino acids based on azobenzene chromophores: building blocks with potential for photoresponsive biomaterials. J. Biotechnol. 112, 127–138. doi: 10.1016/j.jbiotec.2004.03.017
Kaiser, E., Colescott, R. L., Bossinger, C. D., and Cook, P. I. (1970). Color test for detection of free terminal amino groups in the solid-phase synthesis of peptides. Anal. Biochem. 34, 595–598. doi: 10.1016/0003-2697(70)90146-6
Komarov, I. V., Afonin, S., Babii, O., Schober, T., and Ulrich, A. S. (2018). Efficiently Photocontrollable or Not? Biological activity of photoisomerizable diarylethenes. Chemistry 24, 11245–11254. doi: 10.1002/chem.201801205
Kumita, J. R., Smart, O. S., and Woolley, G. A. (2000). Photo-control of helix content in a short peptide. Proc. Natl. Acad. Sci. U.S.A. 97, 3803–3808. doi: 10.1073/pnas.97.8.3803
Lee, W., Tonelli, M., and Markley, J. L. (2015). NMRFAM-SPARKY: enhanced software for biomolecular NMR spectroscopy. Bioinformatics 31, 1325–1327. doi: 10.1093/bioinformatics/btu830
Lolli, F., Mazzanti, B., Pazzagli, M., Peroni, E., Alcaro, M. C., Sabatino, G., et al. (2005a). The glycopeptide CSF114(Glc) detects serum antibodies in multiple sclerosis. J. Neuroimmunol. 167, 131–137. doi: 10.1016/j.jneuroim.2005.05.016
Lolli, F., Mulinacci, B., Carotenuto, A., Bonetti, B., Sabatino, G., Mazzanti, B., et al. (2005b). An N-glucosylated peptide detecting disease-specific autoantibodies, biomarkers of multiple sclerosis. Proc. Natl. Acad. Sci. U.S.A. 102, 10273–10278. doi: 10.1073/pnas.0503178102
Marchi, E., Baroncini, M., Bergamini, G., Van Heyst, J., Vögtle, F., and Ceroni, P. (2012). Photoswitchable metal coordinating tweezers operated by light-harvesting dendrimers. J. Am. Chem. Soc. 134, 15277–15280. doi: 10.1021/ja307522f
Miniewicz, A., Orlikowska, H., Sobolewska, A., and Bartkiewicz, S. (2018). Kinetics of thermal cis-trans isomerization in a phototropic azobenzene-based single-component liquid crystal in its nematic and isotropic phases. Phys. Chem. Chem. Phys. 20, 2904–2913. doi: 10.1039/c7cp06820d
Pandey, S., Alcaro, M. C., Scrima, M., Peroni, E., Paolini, I., Di Marino, S., et al. (2012). Designed glucopeptides mimetics of myelin protein epitopes as synthetic probes for the detection of autoantibodies, biomarkers of multiple sclerosis. J. Med. Chem. 55, 10437–10447. doi: 10.1021/jm301031r
Paolini, I., Nuti, F., de la Cruz Pozo-Carrero, M., Barbetti, F., Kolesinska, B., Kaminski, Z. J., Chelli, M., and Papini, A. M. (2007). A convenient microwave-assisted synthesis of N-glycosyl amino acids. Tetrahedron Lett. 48, 2901–2904. doi: 10.1016/j.tetlet.2007.02.087
Papini, A. M. (2009). The use of post-translationally modified peptides for detection of biomarkers of immune-mediated diseases. J. Pept. Sci. 15, 621–628. doi: 10.1002/psc.1166
Parisot, J., Kurz, K., Hilbrig, F., and Freitag, R. (2009). Use of azobenzene amino acids as photo-responsive conformational switches to regulate antibody-antigen interaction. J. Sep. Sci. 32, 1613–1624. doi: 10.1002/jssc.200800698
Quick, M., Dobryakov, A. L., Gerecke, M., Richter, C., Berndt, F., Ioffe, I. N., Granovsky, A. A., Mahrwald, R., Ernsting, N. P., and Kovalenko, S. A. (2014). Photoisomerization dynamics and pathways of trans- and cis-azobenzene in solution from broadband femtosecond spectroscopies and calculations. J. Phys. Chem. B. 118, 8756–8771. doi: 10.1021/jp504999f
Rampp, M. S., Hofmann, S. M., Podewin, T., Hoffmann-Röder, A., Moroder, L., and Zinth, W. (2018). Time-resolved infrared studies of the unfolding of a light triggered β-hairpin peptide. Chem. Phys. 512, 116–121. doi: 10.1016/j.chemphys.2018.02.003
Renner, C., Behrendt, R., Spörlein, S., Wachtveitl, J., and Moroder, L. (2000). Photomodulation of conformational states. I. Mono- and bicyclic peptides with (4-amino)phenylazobenzoic acid as backbone constituent. Biopolymers 54, 489–500. doi: 10.1002/1097-0282(200012)54:7<489::AID-BIP20>3.0.2-F
Renner, C., Kusebauch, U., Löweneck, M., Milbradt, A. G., and Moroder, L. (2005). Azobenzene as photoresponsive conformational switch in cyclic peptides. J. Pept. Res. 65, 4–14. doi: 10.1111/j.1399-3011.2004.00203.x
Renner, C., and Moroder, L. (2006). Azobenzene as conformational switch in model peptides. Chembiochem 7, 868–878. doi: 10.1002/cbic.200500531
Rentier, C., Pacini, G., Nuti, F., Peroni, E., Rovero, P., and Papini, A. M. (2015). Synthesis of diastereomerically pure Lys(Nε-lipoyl) building blocks and their use in Fmoc/tBu solid phase synthesis of lipoyl-containing peptides for diagnosis of primary biliary cirrhosis. J. Pept. Sci. 21, 408–414. doi: 10.1002/psc.2761
Rizzolo, F., Testa, C., Lambardi, D., Chorev, M., Chelli, M., Rovero, P., and Papini, A. M. (2011). Conventional and microwave-assisted SPPS approach: a comparative synthesis of PTHrP(1-34)NH(2). J. Pept. Sci. 17, 708–714. doi: 10.1002/psc.1395
Roccatano, D., Colombo, G., Fioroni, M., and Mark, A. E. (2002) Mechanism by which 2,2,2-trifluoroethanol/water mixtures stabilize secondary-structure formation in peptides: a molecular dynamics study. Proc Natl Acad Sci U.S.A. 99, 12179–12184. doi: 10.1073/pnas.182199699
Satzger, H., Root, C., and Braun, M. (2004). Excited-State dynamics of trans- and cis-azobenzene after UV excitation in the ππ* band. J. Phys. Chem A. 108, 6265–6271. doi: 10.1021/jp049509x
Schultz, T., Quenneville, J., Levine, B., Toniolo, A., Martínez, T. J., Lochbrunner, S., Schmitt, M., Shaffer, J. P., Zgierski, M. Z., and Stolow, A. (2003). Mechanism and dynamics of azobenzene photoisomerization. J. Am. Chem. Soc. 125, 8098–8099. doi: 10.1021/ja021363x
Schumacher, M. A., Hurlburt, B. K., and Brennan, R. G. (2001). Crystal structures of SarA, a pleiotropic regulator of virulence genes in S. aureus. Nature 409, 215–219. doi: 10.1038/35051623
Siampiringue, N., Guyot, G., Monti, S., and Bortolus, P. (1987). The Cis-Trans photoisomerization of azobenzene: an experimental reexamination. J. Photochem. 37, 185–188. doi: 10.1016/0047-2670(87)85039-6
Spörlein, S., Carstens, H., Satzger, H., Renner, C., Behrendt, R., Moroder, L., Tavan, P., Zinth, W., and Wachtveitl, J. (2002). Ultrafast spectroscopy reveals subnanosecond peptide conformational dynamics and validates molecular dynamics simulation. Proc. Natl. Acad. Sci. U.S.A. 99, 7998–8002. doi: 10.1073/pnas.122238799
Ulysse, L., and Chmielewski, J. (1994). The synthesis of a light-switchable amino acid for inclusion into conformationally mobile peptides bioorg. Med. Chem. Lett. 4, 2145–2146. doi: 10.1016/S0960-894X(01)80118-9
Ulysse, L., Cubillos, J., and Chmielewski, J. (1995). Photoregulation of cyclic peptide conformation. J. Am. Chem. Soc. 117, 8466–8467. doi: 10.1021/ja00137a023
Wong, H. C., Mao, J., Nguyen, J. T., Srinivas, S., Zhang, W., Liu, B., Li, L., Wu, D., and Zheng, J. (2000). Structural basis of the recognition of the dishevelled DEP domain in the Wnt signaling pathway. Nat. Struct. Biol. 7, 1178–11784.820477, 1178–1184
Yamamura, M., Yamakawa, K., Okazaki, Y., and Nabeshima, T. (2014). Coordination-driven macrocyclization for locking of photo- and thermal cis → trans isomerization of azobenzene. Chemistry 20, 16258–16265. doi: 10.1002/chem.201404620
Zavala-Ruiz, Z., Strug, I., Walker, B. D., Norris, P. J., and Stern, L. J. (2004). A hairpin turn in a class II MHC-bound peptide orients residues outside the binding groove for T cell recognition. Proc. Natl. Acad. Sci. U.S.A. 101, 13279–13284. doi: 10.1073/pnas.0403371101
Keywords: azobenzene, cis/trans photoisomerization, photoswitchable peptide, optical control, NMR spectroscopy, UV/Vis spectroscopy
Citation: Nuti F, Gellini C, Larregola M, Squillantini L, Chelli R, Salvi PR, Lequin O, Pietraperzia G and Papini AM (2019) A Photochromic Azobenzene Peptidomimetic of a β-Turn Model Peptide Structure as a Conformational Switch. Front. Chem. 7:180. doi: 10.3389/fchem.2019.00180
Received: 10 January 2019; Accepted: 07 March 2019;
Published: 29 March 2019.
Edited by:
Alessandro Contini, University of Milan, ItalyReviewed by:
Junji Zhang, East China University of Science and Technology, ChinaTangxin Xiao, Changzhou University, China
Copyright © 2019 Nuti, Gellini, Larregola, Squillantini, Chelli, Salvi, Lequin, Pietraperzia and Papini. This is an open-access article distributed under the terms of the Creative Commons Attribution License (CC BY). The use, distribution or reproduction in other forums is permitted, provided the original author(s) and the copyright owner(s) are credited and that the original publication in this journal is cited, in accordance with accepted academic practice. No use, distribution or reproduction is permitted which does not comply with these terms.
*Correspondence: Anna Maria Papini, annamaria.papini@unifi.it
†These authors have contributed equally to this work