- 1School of Physics and Technology, University of Jinan, Jinan, China
- 2School of Mathematics and Physics, Anhui University of Technology, Ma'anshan, China
Energy and environmental issues raise higher demands on the development of a sustainable energy system, and the electrocatalytic hydrogen evolution is one of the most important ways to realize this goal. Two-dimensional (2D) materials represented by molybdenum disulfide (MoS2) have been widely investigated as an efficient electrocatalyst for the hydrogen evolution. However, there are still some shortcomings to restrict the efficiency of MoS2 electrocatalyst, such as the limited numbers of active sites, lower intrinsic catalytic activity and poor interlayer conductivity. In this review, the application of monolayer MoS2 and its composites with 0D, 1D, and 2D nanomaterials in the electrocatalytic hydrogen evolution were discussed. On the basis of optimizing the composition and structure, the numbers of active sites, intrinsic catalytic activity, and interlayer conductivity could be significantly enhanced. In the future, the study would focus on the structure, active site, and interface characteristics, as well as the structure-activity relationship and synergetic effect. Then, the enhanced electrocatalytic activity of monolayer MoS2 can be achieved at the macro, nano and atomic levels, respectively. This review provides a new idea for the structural design of two-dimensional electrocatalytic materials. Meanwhile, it is of great significance to promote the study of the structure-activity relationship and mechanism in catalytic reactions.
Introduction
The continuous growth of the population and the development of the industrialization process have accelerated the consumption of fossil energy, and brought serious environmental problems. Therefore, the development of sustainable energy system is one of the most important challenges today (Wang and Mi, 2017; Chi and Yu, 2018). At present, a promising method is to produce renewable energy through the electrochemically catalytic reaction, which converts the common materials, such as water, carbon dioxide, and nitrogen, into the high-energy carriers (hydrogen, oxygen, hydrocarbons, ammonia, etc.). After years of research and practice, many important advances have been made in electrochemical energy conversion (Gu et al., 2018; Mao et al., 2018; Xiong J. et al., 2018). Among them, hydrogen energy is considered as the most powerful candidate to alternate fossil energy due to its clean, renewable, and environmentally friendly properties and high energy density (Lin et al., 2017; Zhang S. et al., 2017). Among various methods of hydrogen energy production, electrocatalytic water splitting has attracted tremendous attention because of its advantages of low cost, non-pollution and high efficiency (Wang et al., 2016). Moreover, the electrocatalytic cathode in this method is the key to determine the efficiency of water decomposition. So far, the Pt cathode possessing the near zero overpotential is considered to be the most effective catalytic cathode. However, it is difficult to be practically applied or industrialized due to its high cost and scarce resource (Eftekhari, 2017; Hou et al., 2017). Therefore, seeking for low-cost, abundant, high efficient, and environmentally friendly catalytic cathode materials has become a research hotspot. In the view of this point, many materials have been extensively explored, such as carbides, nitrides, sulfides, selenides, phosphides, and Mo-based non-noble metal electrocatalysts (Xie et al., 2014; Pu et al., 2016a,b,c, 2017, 2018; Voiry et al., 2016; Wei et al., 2016; Xie and Xie, 2016; Kou et al., 2017, 2018a,b; Jin et al., 2018). Among these materials, molybdenum disulfide (MoS2) has attracted much more attention due to its low cost, high catalytic activity, high stability, large in-plane carrier mobility and good mechanical properties (Tan et al., 2017; Li et al., 2018; Wang et al., 2018a). Studies have shown that monolayer MoS2 has higher electrocatalytic activity for hydrogen evolution. However, there are still some shortcomings, such as the limited numbers of active sites, lower intrinsic catalytic activity and poor interlayer conductivity. In order to further improve the electrocatalytic activity of monolayer MoS2, researchers usually composite them with other materials. In this paper, the composite of monolayer MoS2 with 0D, 1D, and 2D materials and its application in electrocatalytic hydrogen evolution are reviewed in order to provide guidance for related research. At present, there are two kinds of methods for preparing monolayer MoS2. The first method is top-down approach, including mechanical stripping (Li et al., 2012), ion intercalation (Nurdiwijayanto et al., 2018) and liquid phase stripping (Zhao et al., 2016), and the second one is bottom-up approach, including chemical vapor deposition(CVD) (Liu et al., 2018) and wet chemical stripping (Zeng et al., 2017). The development strategy of sustainable energy pattern and catalyst based on the electrocatalysis are shown in Figure 1.
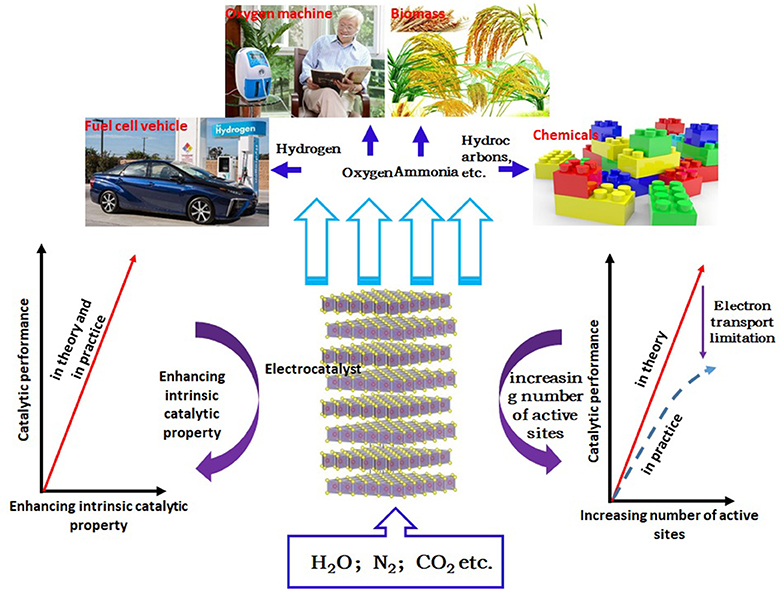
Figure 1. Schematic diagram of sustainable energy pattern and catalyst development strategies based on the electrocatalysis.
Strategies for Electrocatalytic Hydrogen Evolution
As the electrocatalyst plays an important role in improving conversion efficiency in energy conversion process, the research of electrocatalyst is a crucial part in these conversion technologies. Up to now, the electrocatalysts suffer from the lack of types and low efficiency. What's more, the high expense leads them difficult to be practically used on a large scale. Many efforts have been made to solve these problems. For example, in order to improve the electrocatalytic activity, three strategies are usually proposed: one is to increase the number of active sites (from the view of the “quantity” aspect); the other is to increase the intrinsic activity of active sites (which belongs to the “quality” aspect); the third is to improve the conductivity of electrocatalysts by forming composites. These strategies are not mutually exclusive, but can be mutually complementary to improve the activity of catalysts simultaneously (Seh et al., 2017; Tang C. et al., 2018).
Two-dimensional materials such as MoS2 have been extensively studied in the electrocatalytic hydrogen evolution due to their promising potential application prospect. However, there is still a big gap compared with Pt catalyst. Therefore, great efforts have been made to improve the electrocatalytic activity of MoS2, including phase transformation (Tang and Jiang, 2016; Jiao et al., 2018; Wang J. et al., 2018), defect engineering (Xie et al., 2013a, 2017, 2019; Xie and Yi, 2015), nanocrystallization (Yun et al., 2017), doping (Xie et al., 2013b, 2016; Sun et al., 2014, 2018; Xiong Q. et al., 2018), modification (Benson et al., 2017; Wang Q. et al., 2018) and compounding (Jayabal et al., 2017; Zhai et al., 2018), etc.
The bulk phase MoS2 is inert for the electrocatalytic hydrogen evolution, and the free energy of hydrogen adsorption on the base surface of MoS2 is 1.92 eV. However, the theoretical results show that the ΔGH of Mo (1010) is 0.08 eV at 50% hydrogen coverage, which is close to the optimum value (≈0 eV) and exhibits the good electrocatalytic activity (Hinnemann et al., 2005). In addition, this propose is confirmed by the experimental results (Jaramillo et al., 2007). Theoretical and experimental studies have proved that the edge of MoS2 is active. Therefore, exposing more edge sites of MoS2 is an important method to enhance its electrocatalytic activity. Thus, the way to improve the electrocatalytic performance is classified to increase the “quantity” of active sites (Zhang J. et al., 2017). The electrocatalytic hydrogen evolution reaction is a two-electron transfer process, and the reaction rate depends largely on ΔGH. If the bonding between H2 and the surface is too weak, the adsorption (Volmer) step will limit the overall reaction rate; if the bonding is too strong, the desorption (Heyrovsky/Tafel) step will limit the reaction rate (Parsons, 1958; Wang et al., 2018b). Therefore, a highly active catalyst should have neither too strong nor too weak bonding intermediates. According to these points, by controlling the surface/interface properties of MoS2, the surface electronic properties, surface adsorption behavior and hydrogen evolution reaction path can be optimized, which can promote the kinetic process of the electrocatalytic hydrogen evolution and enhance the intrinsic catalytic ability (Otyepková et al., 2017; Chen et al., 2018). The research in this field is to improve the electrocatalytic activity by optimizing the “quality” of the active site. It has been proved that the transport of electrons between MoS2 layers needs to overcome certain barriers. The electron transport is dominated by the jump transport mode leading to the low transport efficiency, which limits the improvement of their electrocatalytic activities. So, the acceleration of the electron transport between layers is also an effective way to enhance the catalytic activity (Yu et al., 2013).
Electrocatalytic Hydrogen Evolution
Monolayer MoS2
Monolayer MoS2 exhibits relatively high electrocatalytic activity due to the exposure of more active sites, which can enhance the “quantity” of active sites. Zhang et al. prepared monolayer MoS2 by low-voltage CVD method (Shi et al., 2014). By changing the growth temperature or the distance between source and substrate, the controllable boundary length of MoS2 was successfully realized. The electrocatalytic hydrogen evolution results showed that the exchange current density increased linearly with the increase of boundary length. By changing the morphology of monolayer MoS2, the boundary length could be further extended. The dendritic morphology enriched the boundary of monolayer MoS2 to a great extent, which contributed greatly to the enhancement of the electrocatalytic activity (Zhang et al., 2014; Xu et al., 2018). Fractal monolayer MoS2 can also promote the efficiency of the electrocatalytic hydrogen evolution reaction. The fractal monolayer MoS2 synthesized on the surface of fused quartz can expose a large number of active sites at its edge. Besides, the existence of large internal stresses in the fractal monolayer MoS2, causes more electrons to migrate to the edge active sites, further improving the electrocatalytic performance (Wan et al., 2018). This study also manifests that there is a linear relationship between the electrocatalytic hydrogen evolution activity and the number of marginal active sites of MoS2. The inert surface of MoS2 can be tuned into ordered porous structure by using template. The porous structure can increase the proportion of edge atoms (the number of active sites), resulting in the enhanced electrocatalytic performance (Su et al., 2018). MoS2 nanosheets with rich 1T phase content can be prepared by the chemical peeling. These nanosheets possess many defects, which benefit to the good catalytic activity in the electrocatalytic hydrogen evolution (Voiry et al., 2013; Chang et al., 2016). Doping monolayer MoS2 can activate the activity of the base surface and improve the catalytic activity. For example, the doping of transition metal element Co atoms can change the surface electronic structure of MoS2 and the adsorption energy of hydrogen atoms, improving the catalytic performance (Hai et al., 2017; Lau et al., 2018). The post treatment on monolayer MoS2 is also a strategy to enhance catalytic capacity. Processing with oxygen plasma can increase defects and interfaces in a large extent, which play a certain role in increasing active sites and enhancing intrinsic catalytic activity (Ye et al., 2016).
However, the electrocatalytic hydrogen evolution of monolayer MoS2 is still limited. The number of active sites, intrinsic catalytic activity and interlayer conductance limit the further improvement of the electrocatalytic hydrogen evolution performance of monolayer MoS2. The process for improving the electrocatalytic properties of monolayer MoS2 is complicated or needs special equipments which may limit its practical application. In order to overcome the defects of monolayer MoS2, it is necessary to composite monolayer MoS2 with other low-dimensional materials.
Composition With 0D Materials
The electronic structure of the surface and the binding energy of the active intermediates can be modulated by compositing the single layer MoS2 and 0D, 1D, and 2D materials, leading to the improvement of the electrocatalytic performance by means of the active sites on the “quality” aspect. At the same time, the interlayer conductance of MoS2 can be enhanced by forming the composite, which further improves the electrocatalytic activity from another aspect. Zhang et al. composited Pd, Pt, and Ag nanoparticles with monolayer MoS2 by wet chemical method (Huang et al., 2013). The Pt nanoparticles with the size of 1-3 nm are successfully composited on the surface of monolayer MoS2. The Pt-modified monolayer MoS2 showed the excellent electrocatalytic performance with the neglected overpotential and the comparable Tafel slope of 40 mV/dec compared with pure MoS2 and Pt, which could be ascribed to the effective collection and transport of electrons in the presence of Pt.
Polyoxometallates (POMs) possess excellent performance in catalysis, which is attributed to the abundant oxygen on the surfaces and rich negative charges (Huang J. et al., 2017; Huang et al., 2018b). Polyoxometallates have the abilities to accept multiple electrons and reversible redox properties, which means that they have the outstanding electronic transport properties (Ammam, 2013). The MoS2 nanosheets were successfully exfoliated using the liquid phase exfoliation method assisted by formamide solvothermal treatment (Huang et al., 2018a). The monolayer MoS2 and POM were stacked into a multilayer heterostructure by the layer-by-layer (LBL) method, and the process for buildup of multilayer films is shown in Figure 2A. The electrocatalytic performance was improved due to the high electron transport performance of POMs and the electrochemical test results were plotted in Figures 2B–E.
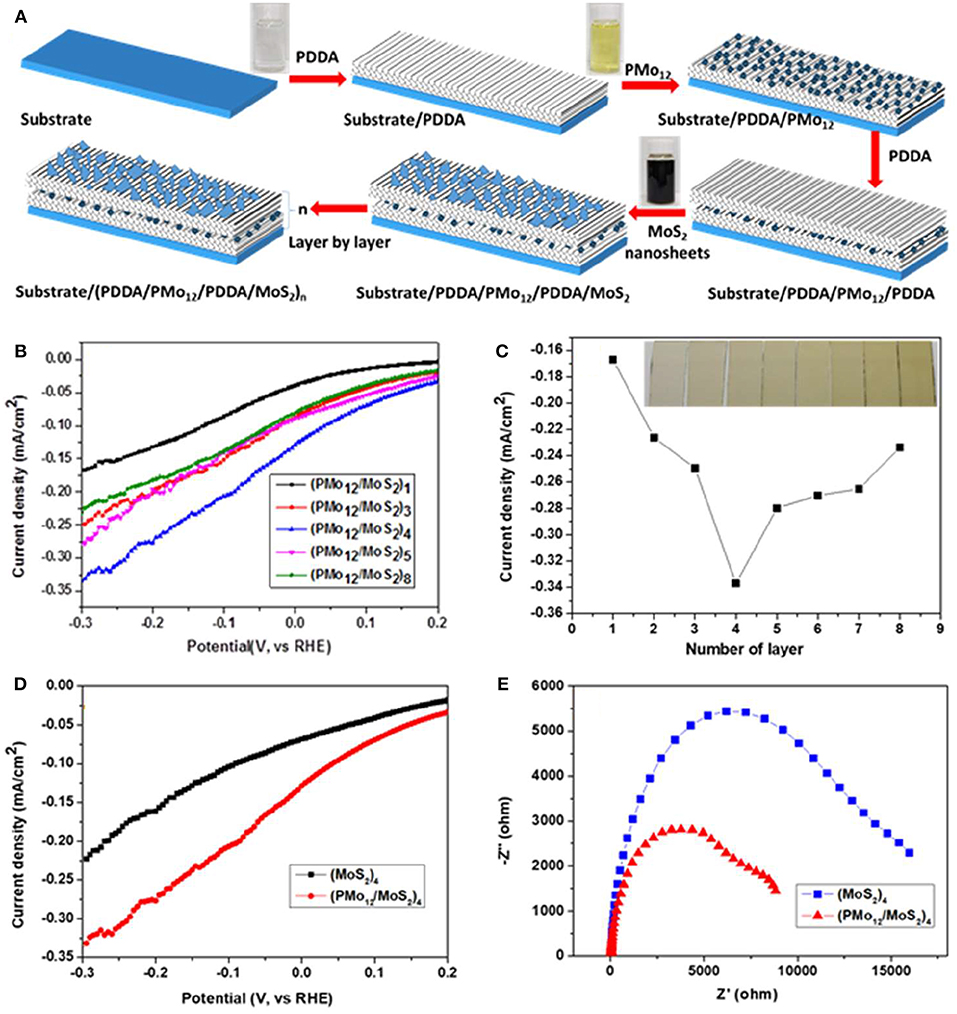
Figure 2. (A) Process for building up the multilayer films (PMo12/MoS2)n, (B) Polarization curves of multilayer (PMo12/MoS2)n, (C) Current density as a function of layer number (inset of (c) Photograph of thin films of (PMo12/MoS2)n deposited on ITO with different number of layer), (D) Polarization curves of multilayer (PMo12/MoS2)4 and (MoS2)4, (E) The EIS spectra of multilayer (PMo12/MoS2)4 and (MoS2)4 (Huang et al., 2018a).
Composition With 1D Materials
One-dimensional (1D) nanostructures offer the unique electronic transport channels. By compositing them with monolayer MoS2, the carrier transport capacity can be improved and the carrier recombination can be reduced. At the same time, the composite structure can bring the modification of the interface and the change of electronic structure, then the electrocatalytic performance can be further improved. Kim group prepared monolayer MoS2 by Li intercalation method (Ahn and Kim, 2017). 1D carbon nanotubes and MoS2 nanosheets were composited by LBL method to form a multilayer structure. The fabrication process was shown in Figure 3A. The hydrogen evolution performance reached the optimum value with the Tafel slope of 62.7 mV/dec for the number of layers of 14. The enhanced catalytic performance was attributed to the high conductivity of carbon nanotubes, which increased the conductivity of interlayer of MoS2, as shown in Figures 3B,C.
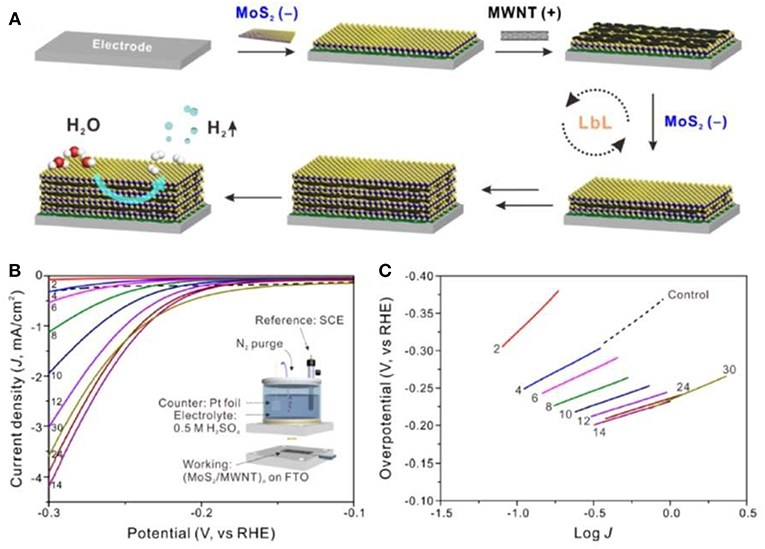
Figure 3. (A) Schematic of the LbL assembly of (MoS2/MWNT)n multilayer electrode, (B) Polarization curves of hybrid multilayer (MoS2/MWNT)n electrodes (Inset of B is the experimental setup of the three-electrode system), (C) Corresponding Tafel plots (Ahn and Kim, 2017).
Xia et al. combined Au nanorods with MoS2 to achieve surface plasmon resonance under auxiliary illumination, which increased the carrier concentration. Moreover, the improved carrier injection and carrier separation efficiency benefited from 1D structure can enhance the electrocatalytic efficiency (Shi et al., 2015).
Composition With 2D Materials
The calculation results pointed out that the combination of graphene oxide and MoS2 could change the interface electronic structure, improve electron transport, and enhance electrocatalytic performance (Tang S. et al., 2018). The combination of graphene and monolayer MoS2 could increase the number of active sites, accelerate the desorption rate of H2 and enhance the efficiency of electron injection, and thus greatly boosted the electrocatalytic activity (Huang H. et al., 2017).
Sasaki team successfully exfoliated bulk MoS2 to obtain monolayer by Li intercalation method, and the monolayer MoS2 was verified to be 1T phase structure (Xiong P. et al., 2018). Then, it can be seen that the monolayer MoS2 was successfully restacked with graphene to form composite structure by the flocculation method (Figures 4A–E). The electrochemical measurements (Figures 4F–J) showed that this structure exhibited excellent electrocatalytic hydrogen evolution performance with the overpotential of 88 mV and Tafel slope of 48.7 mV/dec. The long-term stability was also manifested at 10 mA/cm2 for 10,00,00 s. The outstanding electrochemical properties could be originated from the enhanced electron transport and reduced Gibbs free energy of this unique structure.
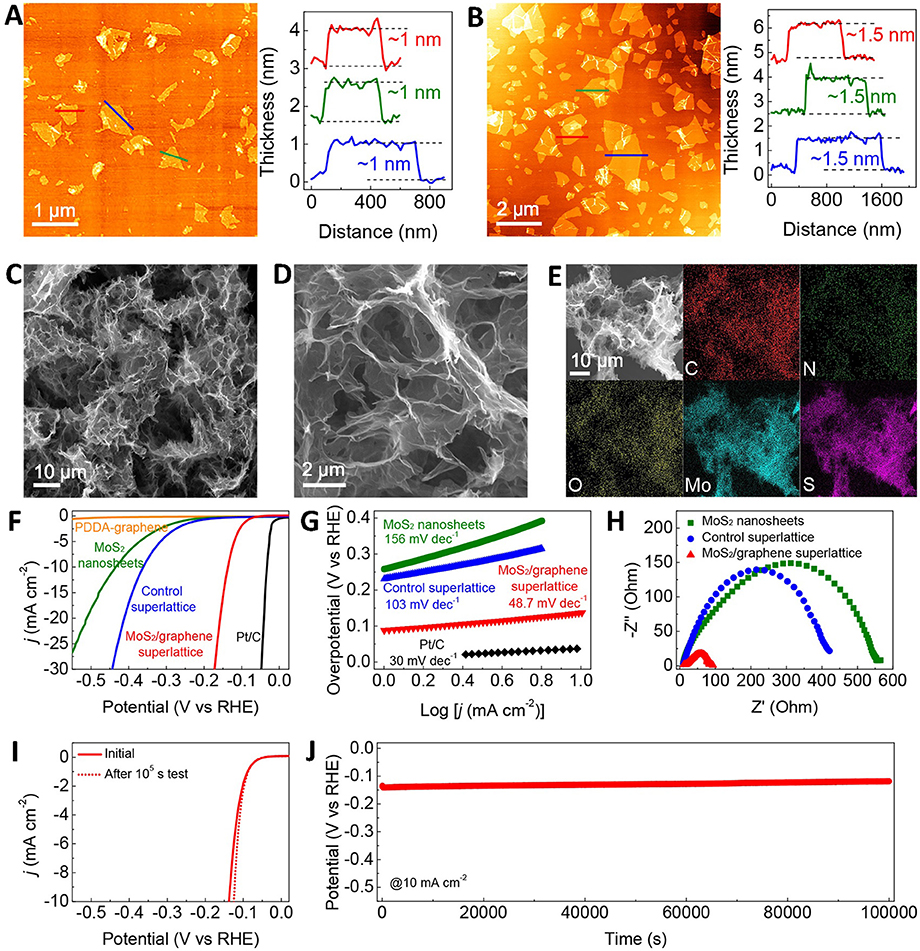
Figure 4. (A) AFM images and height profiles for the exfoliated metallic MoS2 nanosheets, (B) AFM images and height profiles for the PDDA-graphene nanosheets, (C,D) SEM images of the MoS2/graphene superlattice with different magnifications, (E) SEM image and corresponding elemental mapping images of the MoS2/graphene, (F) Polarization curves, (G) Tafel plots, (H) The EIS spectra, (I) The polarization curves of the MoS2/graphene superlattice before and after the 105 s test, (J) Long-term stability measurement (Xiong P. et al., 2018).
According to the characteristics of 0D, 1D, 2D materials, it can play different roles in the composite structure, which can serve as an enhanced electron transport function, as well as to increase the active site, or to activate the in-plane properties. In the actual operation, the electrocatalytic performance can be improved with diverse composite structure in different aspects, such as intrinsic catalysis, increasing the number of active sites, and improving conductivity.
Outlook
Monolayer MoS2 has attracted extensive attention for the electrocatalytic hydrogen evolution. In order to overcome the limitations of active sites, low intrinsic catalytic activity and poor interlayer conductivity, surface modification and composite structure are carried out to improve the electrocatalytic performance. However, there are still some challenges to be worthy of further investigation. Firstly, the properties of composite structure, active site and interface of composite materials are not clear, and need to be studied by more detailed characterization methods; secondly, the comprehensive utilization of monolayer MoS2 and its composite structures at the macro, nano and atomic levels will improve the efficiency of the electrocatalytic hydrogen evolution in principal, but involving the preparation, test and mechanism explanation of materials and devices. It belongs to the multi-disciplinary frontier field and can be studied through in-depth research. In-depth research on these issues can provide the clue for the improvement of the efficiency of the electrocatalytic hydrogen evolution and the deep insight of catalytic mechanism.
Author Contributions
All authors listed have made a substantial, direct and intellectual contribution to the work, and approved it for publication.
Funding
This work was supported by the Natural Science Foundation of Shandong Province (Grant No. ZR2016FM30).
Conflict of Interest Statement
The authors declare that the research was conducted in the absence of any commercial or financial relationships that could be construed as a potential conflict of interest.
References
Ahn, E., and Kim, B. S. (2017). Multidimensional thin film hybrid electrodes with MoS2 multilayer for electrocatalytic hydrogen evolution reaction. ACS Appl. Mater. Inter. 9, 8688–8695. doi: 10.1021/acsami.6b15251
Ammam, M. (2013). Polyoxometalates: formation, structures, principal properties, main deposition methods and application in sensing. J. Mater. Chem. 1, 6291–6312. doi: 10.1039/C3TA01663C
Benson, E. E., Zhang, H., Schuman, S. A., Nanayakkara, S. U., Bronstein, N. D., Ferrere, S., et al. (2017). Balancing the hydrogen evolution reaction, surface energetics, and stability of metallic MoS2 nanosheets via covalent functionalization. J. Am. Chem. Soc. 140, 441–450. doi: 10.1021/jacs.7b11242
Chang, K., Hai, X., Pang, H., Zhang, H., Shi, L., Liu, G., et al. (2016). Targeted synthesis of 2H- and 1T-phase MoS2 monolayers for catalytic hydrogen evolution. Adv. Mater. 28, 10033–10041. doi: 10.1002/adma.201603765
Chen, S., Chen, X., Wang, G., Liu, L., He, Q., Li, X., et al. (2018). Reaction mechanism with thermodynamic structural screening for electrochemical hydrogen evolution on monolayer 1T' phase MoS2. Chem. Mater. 30, 5404–5411. doi: 10.1021/acs.chemmater.8b02236
Chi, J., and Yu, H. (2018). Water electrolysis based on renewable energy for hydrogen production. Chinese J. Catal. 39, 390–394. doi: 10.1016/S1872-2067(17)62949-8
Eftekhari, A. (2017). Electrocatalysts for hydrogen evolution reaction. Int. J. Hydrog. Energ. 42, 11053–11077. doi: 10.1016/j.ijhydene.2017.02.125
Gu, M., Choi, J., Lee, T., Park, M., Shin, I., Hong, J., et al. (2018). Diffusion controlled multilayer electrocatalysts via graphene oxide nanosheets of varying sizes. Nanoscale 10, 16159–16168. doi: 10.1039/c8NR02883d
Hai, X., Zhou, W., Wang, S., Pang, H., Chang, K., Ichihara, F., et al. (2017). Rational design of freestanding MoS2 monolayers for hydrogen evolution reaction. Nano Energy 39, 409–417. doi: 10.1016/j.nanoen.2017.07.021
Hinnemann, B., Moses, P. G., Bonde, J., Jørgensen, K. P., Nielsen, J. H., Horch, S., et al. (2005). Biomimetic hydrogen evolution: MoS2 nanoparticles as catalyst for hydrogen evolution. J. Am. Chem. Soc. 36, 5308–5309. doi: 10.1002/chin.200525015
Hou, Y., Zhuang, X., and Feng, X., (2017). Recent advances in earth-abundant heterogeneous electrocatalysts for photoelectrochemical water splitting. Small Methods 1:1700090. doi: 10.1002/smtd.201700090
Huang, H., Huang, J., Liu, W., Fang, Y., and Liu, Y., (2017). Ultradispersed and single-layered MoS2 nanoflakes strongly coupling with graphene: an optimized structure with high kinetics for the hydrogen evolution reaction. ACS Appl. Mater. Inter. 9, 39380–39390. doi: 10.1021/acsami.7b12038
Huang, J., Deng, X., Wan, H., Chen, F., Lin, Y., Xu, X., et al. (2018a). Liquid phase exfoliation of MoS2 assisted by formamide solvothermal treatment and enhanced electrocatalytic activity based on (H3Mo12O40P/MoS2)n multilayer structure. ACS Sustain. Chem. Eng. 6, 5227–5237. doi: 10.1021/acssuschemeng.7b04873
Huang, J., Yao, N., Fu, K., and Deng, X., (2017). Low cost and high catalytic efficiency compo-site counter electrode NiS-H3Mo12O40P for dye-sensitized solar cells. Mater. Lett. 198, 65–68. doi: 10.1016/j.matlet.2017.03.176
Huang, J., Yao, N., Fu, K., Deng, X., Zhang, S., Hou, P., et al. (2018b). Enhanced dye-sensitized solar cell efficiency by insertion of a H3PW12O40 layer between the transparent conductive oxide layer and the compact TiO2 layer. Sci. Adv. Mater. 10, 867–871. doi: 10.1166/sam.2018.3241
Huang, X., Zeng, Z., Bao, S., Wang, M., Qi, X., Fan, Z., et al. (2013). Solution-phase epitaxial growth of noble metal nanostructures on dispersible single-layer molybdenum disulfide nanosheets. Nat. Commun. 4, 1444. doi: 10.1038/ncomms2472
Jaramillo, T. F., Jørgensen, K. P., Bonde, J., Nielsen, J. H., Horch, S., and Chorkendorff, I., (2007). Identification of active edge sites for electrochemical H2 evolution from MoS2 nanocatalysts. Science 317, 100–102. doi: 10.1126/science.1141483
Jayabal, S., Saranya, G., Wu, J., Liu, Y., Geng, D., and Meng, X., (2017). Understanding the high-electrocatalytic performance of two-dimensional MoS2 nanosheets and their composite materials. J. Mater. Chem. A 5, 24540–24563. doi: 10.1039/C7TA08327K
Jiao, Y., Hafez, A. M., Cao, D., Mukhopadhyay, A., Ma, Y., and Zhu, H. (2018). Metallic MoS2 for high performance energy storage and energy conversion. Small 14, 1800640. doi: 10.1002/smll.201800640
Jin, H., Guo, C., Liu, X., Liu, J., Vasileff, A., Jiao, Y., et al. (2018). Emerging two-dimensional nanomaterials for electrocatalysis. Chem. Rev. 118, 6337–6408. doi: 10.1021/acs.chemrev.7b00689
Kou, Z., Kai, X., Pu, Z., and Mu, S. (2017). Constructing carbon-cohered high-index (222) faceted tantalum carbide nanocrystals as a robust hydrogen evolution catalyst. Nano Energy 36, 374–380. doi: 10.1016/j.nanoen.2017.04.057
Kou, Z., Wang, T., Pu, Z., Wu, L., Xi, K., and Mu, S. (2018a). Realizing the extraction of carbon from WC for in situ formation of W/WC heterostructures with efficient photoelectrochemical hydrogen evolution. Nanoscale Horiz. 4, 196–201. doi: 10.1039/C8NH00275D
Kou, Z., Wang, T., Yi, C., Cao, G., Pu, Z., Zhu, C., et al. (2018b). Ultrafine molybdenum carbide nanocrystals confined in carbon foams via a colloid-confinement route for efficient hydrogen production. Small Methods 2:1700396. doi: 10.1002/smtd.201700396
Lau, T., Lu, X., Kulhavý, J., Wu, S., Lu, L., Wu, T. S., et al. (2018). Transition metal atom doping of the basal plane of MoS2 monolayer nanosheets for electrochemical hydrogen evolution. Chem. Sci. 9, 4769–4776. doi: 10.1039/C8SC01114A
Li, H., Jia, X., Zhang, Q., and Wang, X. (2018). Metallic transition-metal dichalcogenide nanocatalysts for energy conversion. Chem 4, 1510–1537. doi: 10.1016/j.chempr.2018.03.012
Li, H., Yin, Z., He, Q., Li, H., Huang, X., Lu, G., et al. (2012). Fabrication of single- and multilayer MoS2 film-based field-effect transistors for sensing NO at room temperature. Small 8, 63–67. doi: 10.1002/smll.201101016
Lin, L., Zhou, W., Gao, R., Yao, S., Zhang, X., Xu, W., et al. (2017). Low-temperature hydrogen production from water and methanol using Pt/α-MoC catalysts. Nature 544, 80–83. doi: 10.1038/nature21672
Liu, L., Wu, J., Wu, L., Ye, M., Liu, X., Wang, Q., et al. (2018). Phase-selective synthesis of 1T′ MoS2 monolayers and heterophase bilayers. Nat. Mater. 17, 1108–1114. doi: 10.1038/s41563-018-0187-1
Mao, J., Wang, Y., Zheng, Z., and Deng, D. (2018). The rise of two-dimensional MoS2 for catalysis. Front. Phys. 13, 138118. doi: 10.1007/s11467-018-0812-0
Nurdiwijayanto, L., Wu, J., Sakai, N., Ma, R., Ebina, Y., and Sasaki, T. (2018). Monolayer attachment of metallic MoS2 on restacked titania nanosheets for efficient photocatalytic hydrogen generation. ACS Appl. Energ. Mater. 1, 6912–6918. doi: 10.1021/acsaem.8b01319
Otyepková, E., Lazar, P., and Luxa, J. (2017). Surface properties of MoS2 probed by inverse gas chromatography and their impact on electrocatalytic properties. Nanoscale 9, 19236–19244. doi: 10.1039/c7nr07342a
Parsons, R. (1958). The rate of electrolytic hydrogen evolution and the heat of adsorption of hydrogen. Faraday Soc. 54, 1603–1611. doi: 10.1039/tf9585401053
Pu, Z., Amiinu, I. S., Gao, F., Xu, Z., Zhang, C., Li, W., et al. (2018). Efficient strategy for significantly decreasing overpotentials of hydrogen generation via oxidizing small molecules at flexible bifunctional CoSe electrodes. J. Power Sources 401, 238–244. doi: 10.1016/j.jpowsour.2018.08.085
Pu, Z., Amiinu, I. S., Kou, Z., Li, W., and Mu, S. (2017). RuP2-based catalysts with platinum-like activity and higher durability for the hydrogen evolution reaction at all pH values. Angew. Chem. Int. Ed. 56, 11559–11564. doi: 10.1002/anie.201704911
Pu, Z., Amiinu, I. S., Wang, M., Yang, Y., and Mu, S. (2016a). Semimetallic MoP2: an active and stable hydrogen evolution electrocatalyst over the whole pH range. Nanoscale 8, 8500–8504. doi: 10.1039/c6nr00820h
Pu, Z., Wang, M., Kou, Z., Amiinu, I. S., and Mu, S. (2016b). Mo2C quantum dot embedded chitosan-derived nitrogen-doped carbon for efficient hydrogen evolution in a broad pH range. Chem. Commun. 52, 12753–12756. doi: 10.1039/c6cc06267a
Pu, Z., Wei, S., Chen, Z., and Mu, S. (2016c). Flexible molybdenum phosphide nanosheet array electrodes for hydrogen evolution reaction in a wide pH range. Appl. Catal. B-Environ. 196, 193–198. doi: 10.1016/j.apcatb.2016.05.027
Seh, Z. W., Kibsgaard, J., Dickens, C. F., Chorkendorff, I., Nørskov, J. K., and Jaramillo, T. F. (2017). Combining theory and experiment in electrocatalysis: insights into materials design. Science 355:eaad4998. doi: 10.1126/science.aad4998
Shi, J., Ma, D., Han, G. F., Zhang, Y., Ji, Q., Gao, T., et al. (2014). Controllable growth and transfer of monolayer MoS2 on Au foils and its potential application in hydrogen evolution reaction. ACS Nano 8, 10196–10204. doi: 10.1021/nn503211t
Shi, Y., Wang, J., Wang, C., Zhai, T. T., Bao, W. J., Xu, J. J., et al. (2015). Hot electron of Au nanorods activates the electrocatalysis of hydrogen evolution on MoS2 nanosheets. J. Am. Chem. Soc. 137, 7365–7370. doi: 10.1021/jacs.5b01732
Su, S., Zhou, Q., Zeng, Z., Hu, D., Wang, X., Jin, M., et al. (2018). Ultra-thin alumina masks assisted nanopore patterning on monolayer MoS2 for highly catalytic efficiency in hydrogen evolution reaction. ACS Appl. Mater. Inter. 10, 8026–8035. doi: 10.1021/acsami.7b19197
Sun, T., Wang, J., Chi, X., Lin, Y., Chen, Z., Ling, X., et al. (2018). Engineering the electronic structure of MoS2 nanorods by N and Mn dopants for ultra-efficient hydrogen production. ACS Catal. 8, 7585–7592. doi: 10.1021/acscatal.8b00783
Sun, X., Dai, J., Guo, Y., Wu, C., Hu, F., Zhao, J., et al. (2014). Semimetallic molybdenum disulfide ultrathin nanosheets as an efficient electrocatalyst for hydrogen evolution. Nanoscale 6, 8359–8367. doi: 10.1039/c4nr01894j
Tan, C., Cao, X., Wu, X. J., He, Q., Yang, J., Zhang, X., et al. (2017). Recent advances in ultrathin two-dimensional nanomaterials. Chem. Rev. 117, 6225–6331. doi: 10.1021/acs.chemrev.6b00558
Tang, C., Wang, H. F., and Zhang, Q. (2018). Multiscale principles to boost reactivity in gas-involving energy electrocatalysis. Acc. Chem. Res. 51, 881–889. doi: 10.1021/acs.accounts.7b00616
Tang, Q., and Jiang, D. (2016). Mecha nism of hydrogen evolution reaction on 1T-MoS2 from first principles. ACS Catal. 6, 4953–4961. doi: 10.1021/acscatal.6b01211
Tang, S., Wu, W., Zhang, S., Ye, D., Zhong, P., Li, X., et al. (2018). Tuning the activity of the inert MoS2 surface via graphene oxide support doping towards chemical functionalization and hydrogen evolution: a density functional study. Phys. Chem. Chem. Phys. 20, 1861–1871. doi: 10.1039/C7CP06636H
Voiry, D., Salehi, M., Silva, R., Fujita, T., Chen, M., Asefa, T., et al. (2013). Conducting MoS2 nanosheets as catalysts for hydrogen evolution reaction. Nano Lett. 13, 6222–6227. doi: 10.1021/nl403661s
Voiry, D., Yang, J., and Chhowalla, M., (2016). Recent strategies for improving the catalytic activity of 2D TMD nanosheets toward the hydrogen evolution reaction. Adv. Mater. 28, 6197–6206. doi: 10.1002/adma.201505597
Wan, Y., Zhang, Z., Xu, X., Zhang, Z., Li, P., Fang, X., et al. (2018). Engineering active edge sites of fractal-shaped single-layer MoS2 catalysts for high-efficiency hydrogen evolution. Nano Energy 51, 786–792. doi: 10.1016/j.nanoen.2018.02.027
Wang, H., Li, C., Fang, P., Zhang, Z., and Zhang, J. Z. (2018a). Synthesis, properties, and optoelectronic applications of two-dimensional MoS2 and MoS2-based heterostructures. Chem. Soc. Rev. 47, 6101–6127. doi: 10.1039/C8CS00314A
Wang, H., Ouyang, L., Zou, G., Sun, C., Hu, J., Xiao, X., et al. (2018b). Optimizing MoS2 edges by alloying isovalent W for robust hydrogen evolution activity. ACS Catal. 8, 9529–9536. doi: 10.1021/acscatal.8b02162
Wang, J., Cui, W., Liu, Q., Xing, Z., Asiri, A. M., and Sun, X. (2016). Recent progress in cobalt-based heterogeneous catalysts for electrochemical water splitting. Adv. Mater. 28, 215–230. doi: 10.1002/adma.201502696
Wang, J., Wang, N., Guo, Y., Yang, J., Wang, J., Wang, J., et al. (2018). Metallic-phase MoS2 nanopetals with enhanced electrocatalytic activity for hydrogen evolution. ACS Sustain. Chem. Eng. 6, 13435–13442. doi: 10.1021/acssuschemeng.8b03324
Wang, Q., Zhao, Z., Dong, S., He, D., Lawrence, M. J., Han, S., et al. (2018). Design of active nickel single-atomdecorated MoS2 as a pH-universal catalyst for hydrogen evolution reaction. Nano Energy 53, 458–467. doi: 10.1016/j.nanoen.2018.09.003
Wang, Z., and Mi, B. (2017). Environmental applications of 2D molybdenum disulfide (MoS2) nanosheets. Environ. Sci. Technol. 51, 8229–8244. doi: 10.1021/acs.est.7b01466
Wei, G., Shi, Y., Zhang, Y., Zuo, L., Lu, H., Huang, Y., et al. (2016). Molybdenum carbide anchored on graphene nanoribbons as highly efficient all-pH hydrogen evolution reaction electrocatalyst. Acs Sustain. Chem. Eng. 4, 6313–6321. doi: 10.1021/acssuschemeng.6b00859
Xie, J., Gao, L., Jiang, H., Zhang, X., Lei, F., Hao, P., et al. (2019). Platinum nanocrystals decorated on defect-rich MoS2 nanosheets for pH-universal hydrogen evolution reaction. Cryst. Growth Des. 19, 60–65. doi: 10.1021/acs.cgd.8b01594
Xie, J., Li, S., Zhang, X., Zhang, J., Wang, R., Zhang, H., et al. (2014). Atomically-thin molybdenum nitride nanosheets with exposed active surface sites for efficient hydrogen evolution. Chem. Sci. 5, 4615–4620. doi: 10.1039/C4SC02019G
Xie, J., Qu, H., Xin, J., Zhang, X., Cui, G., Zhang, X., et al. (2017). Defect-rich MoS2 nanowall catalyst for efficient hydrogen evolution reaction. Nano Res. 10, 1178–1188. doi: 10.1007/s12274-017-1421-x
Xie, J., and Xie, Y. (2016). Transition metal nitrides for electrocatalytic energy conversion: opportunities and challenges. Chem. Eur. J. 22, 3588–3598. doi: 10.1002/chem.201501120
Xie, J., Xin, J., Cui, G., Zhang, X., Zhou, L., Wang, Y., et al. (2016). Vertically aligned oxygen-doped molybdenum disulfide nanosheets grown on carbon cloth realizing robust hydrogen evolution reaction. Inorg. Chem. Front. 3, 1160–1166. doi: 10.1039/C6QI00198J
Xie, J., and Yi, X. (2015). Structural engineering of electrocatalysts for the hydrogen evolution reaction: order or disorder? Chemcatchem 7, 2568–2580. doi: 10.1002/cctc.201500396
Xie, J., Zhang, H., Li, S., Wang, R., Sun, X., Zhou, M., et al. (2013a). Defect-rich MoS2 ultrathin nanosheets with additional active edge sites for enhanced electrocatalytic hydrogen evolution. Adv. Mater. 25, 5807–5813. doi: 10.1002/adma.201301794
Xie, J., Zhang, J., Li, S., Grote, F., Zhang, X., Zhang, H., et al. (2013b). Controllable disorder engineering in oxygen-incorporated MoS2 ultrathin nanosheets for efficient hydrogen evolution. J. Am. Chem. Soc. 136, 17881–17888. doi: 10.1021/ja4129636
Xiong, J., Di, J., and Li, H. (2018). Atomically thin 2D multinary nanosheets for energy-related photo. Adv. Sci. 5:180024. doi: 10.1002/advs.201800244
Xiong, P., Ma, R., Sakai, N., Nurdiwijayanto, L., and Sasaki, T. (2018). Unilamellar metallic MoS2/graphene superlattice for efficient sodium storage and hydrogen evolution. ACS Energy Lett. 3, 997–1005. doi: 10.1021/acsenergylett.8b00110
Xiong, Q., Zhang, X., Wang, H., Liu, G., Wang, G., Zhang, H., et al. (2018). One-step synthesis of cobalt-doped MoS2 nanosheets as bifunctional electrocatalysts for overall water splitting under both acidic and alkaline conditions. Chem. Commun. 54, 3859–3862. doi: 10.1039/C8CC00766G
Xu, W., Li, S., Zhou, S., Lee, J. K., Wang, S., Sarwat, S. G., et al. (2018). Large dendritic monolayer MoS2 grown by atmospheric pressure chemical vapor deposition for electrocatalysis. ACS Appl. Mater. Inter. 10, 4630–4639. doi: 10.1021/acsami.7b14861
Ye, G., Gong, Y., Lin, J., Li, B., He, Y., Pantelides, S. T., et al. (2016). Defects engineered monolayer MoS2 for improved hydrogen evolution reaction. Nano Lett. 16, 1097–1103. doi: 10.1021/acs.nanolett.5b04331
Yu, Y., Huang, S. Y., Li, Y., Steinmann, S. N., Yang, W., and Cao, L. (2013). Layer-dependent electrocatalysis of MoS2 for hydrogen evolution. Nano Lett. 14, 553–558. doi: 10.1021/nl403620g
Yun, Q., Lu, Q., Zhang, X., Tan, C., and Zhang, H. (2017). Three-dimensional architectures constructed from transition metal dichalcogenide nanomaterials for electrochemical energy storage and conversion. Angew. Chem. 57, 626–646. doi: 10.1002/ange.201706426
Zeng, X., Hirwa, H., Wagner, V., Ortel, M., Nicolosi, V., and Nerl, H. C. (2017). Growth of large size two-dimensional MoS2 flakes in aqueous solution. Nanoscale 9, 6575–6580. doi: 10.1039/C7NR00701A
Zhai, Z., Li, C., Zhang, L., Zhang, L., Wu, H. C., Zhang, L., et al. (2018). Dimensional construction and morphological tuning of heterogeneous MoS2/NiS electrocatalysts for efficient overall water splitting. J. Mater. Chem. A 6, 9833–9838. doi: 10.1039/C8TA03304H
Zhang, J., Wu, J., Guo, H., Chen, W., Yuan, J., Martinez, U., et al. (2017). Unveiling active sites for the hydrogen evolution reaction on monolayer MoS2. Adv.Mater. 29, 1701955. doi: 10.1002/adma.201701955
Zhang, S., Yang, H., Gao, H., Cao, R., Huang, J., and Xu, X. (2017). One-pot synthesis of CdS irregular nanospheres hybridized with oxygen-incorporated defect-rich MoS2 ultrathin nanosheets for efficient photocatalytic hydrogen evolution. ACS Appl. Mater. Inter. 9, 23635–23646. doi: 10.1021/acsami.7b03673
Zhang, Y., Ji, Q., Han, G. F., Ju, J., Shi, J., Ma, D., et al. (2014). Dendritic, transferable, strictly monolayer MoS2 flakes synthesized on SrTiO3 single drystals for efficient electrocatalytic applications. ACS Nano 8, 8617–8624. doi: 10.1021/nn503412w
Keywords: monolayer MoS2, electrocatalytic hydrogen evolution, active sites, intrinsic catalysis, composite structure
Citation: Wang C, Huang J, Chen J, Xi Z and Deng X (2019) Progress in Electrocatalytic Hydrogen Evolution Based on Monolayer Molybdenum Disulfide. Front. Chem. 7:131. doi: 10.3389/fchem.2019.00131
Received: 16 January 2019; Accepted: 20 February 2019;
Published: 19 March 2019.
Edited by:
Tianyou Zhai, Huazhong University of Science and Technology, ChinaCopyright © 2019 Wang, Huang, Chen, Xi and Deng. This is an open-access article distributed under the terms of the Creative Commons Attribution License (CC BY). The use, distribution or reproduction in other forums is permitted, provided the original author(s) and the copyright owner(s) are credited and that the original publication in this journal is cited, in accordance with accepted academic practice. No use, distribution or reproduction is permitted which does not comply with these terms.
*Correspondence: Jinzhao Huang, c3NfaHVhbmdqaW56aGFvQHVqbi5lZHUuY24=
Xiaolong Deng, ZGVuZ3hsODRAYWh1dC5lZHUuY24=