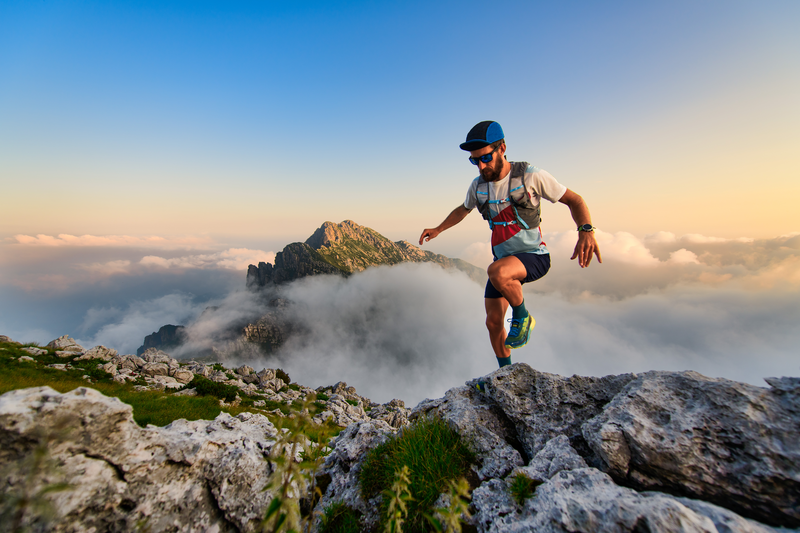
94% of researchers rate our articles as excellent or good
Learn more about the work of our research integrity team to safeguard the quality of each article we publish.
Find out more
ORIGINAL RESEARCH article
Front. Chem. , 21 November 2018
Sec. Catalysis and Photocatalysis
Volume 6 - 2018 | https://doi.org/10.3389/fchem.2018.00569
This article is part of the Research Topic Key Electrochemical Energy Reactions Catalyzed by Nanomaterials View all 6 articles
It is extremely meaningful to develop cheap, highly efficient, and stable bifunctional electrocatalysts for both hydrogen and oxygen evolution reactions (HER and OER) to promote large-scale application of water splitting technology. Herein, we reported the preparation of CoS2 nanoparticles supported on nitrogen-doped graphene (CoS2@N-GN) by one-step hydrothermal method and the enhanced electrochemical efficacy for catalyzing hydrogen and oxygen in water electrolysis. The CoS2@N-GN composites are composed of nitrogen-doped graphene and CoS2 nanocrystals with the average size of 73.5 nm. Benefitting from the improved electronic transfer and synergistic effect, the as-prepared CoS2@N-GN exhibits remarkable OER and HER performance in 1.0 M KOH, with overpotentials of 243 mV for OER and 204 mV for HER at 10 mA cm−2, and the corresponding Tafel slopes of 51.8 and 108 mV dec−1, respectively. Otherwise, the CoS2@N-GN hybrid also presents superior long-term catalytic durability. Moreover, an alkaline water splitting device assembled by CoS2@N-GN as both anode and cathode can achieve a low cell voltage of 1.53 V at 60 °C with a high faraday efficiency of 100% for overall water splitting. The tremendously enhanced electrochemical behaviors arise from favorable factors including small sized, homogenously dispersed novel CoS2 nanocrystals and coupling interaction with the underlying conductive nitrogen-doped graphene, which would provide insight into the rational design of transition metal chalcogenides for highly efficient and durable hydrogen and oxygen-involved electrocatalysis.
The large demand of clean and sustainable energy stimulated intensive research on the development of efficient and robust electrochemical energy conversion systems such as water splitting that can produce hydrogen and oxygen (Wang J. et al., 2016; Jia et al., 2017; Li H. et al., 2017; Zheng et al., 2017). It consists of two half reactions: the oxygen evolution reaction (OER) and hydrogen evolution reaction (HER) (Dong et al., 2015; Menezes et al., 2015; Sun et al., 2017; Han et al., 2018). However, slow reaction dynamics and high overpotential limit the wide application of water electrolyzer (Cheng et al., 2017a; Ma et al., 2017; Huang et al., 2018). High-performance electrocatalysts are urgently needed to reduce the overpotential and improve the energy efficiency (Fang et al., 2017; Yin et al., 2017; Shit et al., 2018). Currently, Pt-based materials and noble metal oxides (RuO2, IrO2) are the best catalysts for HER and OER (Han et al., 2014; Lee et al., 2015; Wang et al., 2015), respectively. However, high price and limited reserves restrict the wide application of these noble metal-based materials (Cheng et al., 2017b; Su et al., 2017). Moreover, these catalysts are still faced the problems of inferior long-term stability and unsatisfied bifunctional activity. Therefore, it is of great significance to design and develop high-abundant, high active and stable precious metal-free electrocatalysts (Forgie et al., 2010; Mccrory et al., 2015).
Recently, transition metal sulfides, especially cobalt sulfide, have attracted much research attention because of their earth-abundance, environmentally green, and significant chemical/electrochemical stability (Wang et al., 2009; Zheng et al., 2015; Han et al., 2017; Liu et al., 2017; Wu et al., 2017). However, the limited active sites and intrinsically low conductivity of traditional cobalt sulfide materials hinder their activity enhancement for catalyzing HER and OER (Liu et al., 2013). Therefore, the combination of metal sulfides and conductive materials is supposed to increase the electrical conductivity and meanwhile synergistically promoting the catalytic activity (Zhou et al., 2019). For example, Zn–Co-mixed sulfide nanostructure on carbon fiber paper has been synthesized for efficient rechargeable zinc–air batteries and water electrolysis (Wu et al., 2017); Co9S8 nanoparticles were anchored on nitrogen and sulfur dual-doped carbon nanosheets for bifunctional oxygen evolution and reduction reactions (Gulzar et al., 2017), CoSx/Ni3S2 heterostructure supported on nickel foam was developed as an efficient catalytic electrode for accelerating HER and OER (Wang et al., 2018). Previous investigations demonstrated that, among various cobalt sulfides (i.e., Co3S4, Co9S8, CoS2, etc.), the pyrite-type CoS2 exhibited the superior intrinsic performance owning to the abundant cobalt active sites and more proton-acceptor centers as a results of the unique crystal structure and S-rich nature (Kumar et al., 2018). Accordingly, it appears to be a smart strategy to design CoS2 nanostructure/conductive supported hybrid material to further optimize the HER and OER capability to replace the precious metal-based catalysts, thereby promoting the large-scale implementation of overall water splitting technologies (Wang J. et al., 2016; Xia et al., 2017).
In this work, we prepared the composite material of CoS2 uniformly supported on nitrogen-doped graphene (CoS2@N-GN) by one-step hydrothermal method and its application as bifunctional electrocatalytic catalyst for OER/HER and overall water-splitting in alkaline media. The average size of CoS2 is about 73.5 nm, homogeneously dispersed on the N-doped reduced graphene oxide nanosheet. Electrochemical tests reveal that the CoS2@N-GN hybrid exhibits superior catalytic performance than bare CoS2 and physically mixture sample (CoS2/N-GN), delivering low overpotentials of 243 and 204 mV at current density of 10 mA cm−2 for OER and HER, respectively. The in-situ grown composite electrode also presents remarkable long-term catalytic durability with negligible activity decay after 12 h period. Furthermore, the alkaline electrolyzer using CoS2@N-GN loaded on carbon fiber paper as both anode and cathode electrodes can achieve 10 mA cm−2 at a low cell voltage of 1.53 V at 60°C with a faradaic efficiency of 100% for overall water splitting. Our research will provide insights on the development of low cost and efficient hybrid electrocatalysts for next-generation energy conversion devices.
Nitrogen doped graphene was purchased from Aladdin. Cobalt (II) acetate tetrahydrate (Co(AC)2·4H2O, 99.5%) and Potassium hydroxide (KOH, 99.99%) were purchased from Beijing Chemicals (Beijing, China). Carbon disulfide (CS2, 99%) and ethylenediamine (EN, 99%) were obtained from Tianjin Yuanli Chemical Co. Ltd. (Tianjin, China). The carbon paper (CP) was purchased from Phychemi Company Limited and used as the substrate of active substance. The deionized water (18.2 MΩ·cm−1) was obtained via Millipore, an ultrapure water system. High purity nitrogen (Air Product, purity 99.995%) gas was used to deaerate the 1 M KOH solution. All the reagents were of analytical grade and used as received without further purification.
The CoS2@N-GN hybrid was synthesized by one step hydrothermal method. Typically, 30 mg of nitrogen doped graphene was put into a beaker with 100 mL and then 35 mL of deionized water was added followed by 30 min of ultrasonic agitation. Then, 0.4 mmol of Co(CH3COO)2·4H2O was added into the above mixed solution, followed by magnetic stirring under 800 rounds per minute (rpm) for 10 min. Then 0.16 mL of EN was added into the solution with magnetic stirring for 20 min. Afterwards, 0.16 mL of CS2 was dropped into the solution to form a uniform mixture after magnetic stirring for another 20 min. The above aqueous solution was then transferred into a 50 mL Teflon-lined stainless autoclave and then maintained at 200°C for 9 h. After cooling naturally to room temperature, the product was separated by centrifugal force, washed by distilled water for three times, and then lyophilized. The preparation of pure CoS2 was the same as the above route without adding 30 mg nitrogen doped graphene. The synthesis of physical mixture of CoS2 and N-doped graphene was achieved by mechanically mixed two components at mass ratio of 1:1.
The phase purity of the as-prepared samples was characterized by X-ray diffraction (XRD, Bruker/D8 Advanced with Cu Kα radiation) at a scanning rate of 2° min−1. The morphological micro-nanostructures were characterized by field-emission scanning electron microscopy (SEM, Hitachi S4800, 30 kV) and transmission electron microscopy (TEM, JEOL JEM-2100F, 200 kV) equipped with an energy-dispersive spectrometer (EDS). The Brunauer-Emmett-Teller (BET) specific surface area was determined by N2 adsorption/desorption isotherms at 77 K using the AutosorbiQ instrument (Quantachrome U.S.) with a 6 h outgas at 80°C. X-ray photoelectron spectroscopy (XPS) was conducted by using a Perkin Elmer PHI 1600 ECSA system.
The electrocatalytic properties of the synthesized catalysts were tested on an IviumStat workstation using a three-electrode configuration. All the electrochemical data was performed in 1.0 M KOH electrolyte, which was saturated with high-purity N2 (Air Product, purity 99.995 %) for OER and HER for at least 30 min before each test and maintained under the corresponding atmosphere during the whole experiment. In addition, a saturated calomel electrode (SCE), and a platinum foil electrode were employed as reference electrode, and counter electrode. The linear sweeping voltammetry (LSV) of ORR and OER were scanning at a same scan rate of 5 mV s−1. The HER and OER potentials were corrected to the reversible hydrogen electrode (RHE) on the basis of following equation:
The electrochemical data of HER and OER was measured from −0.8 to −1.6 V vs. SCE, and 0.2 to 1.0 V vs. SCE, respectively. Electrochemical impedance spectra was carried out in a frequency range of from 100 to100 mHz at a potential of 0.6 V vs. RHE. The obtained HER and OER linear sweeping voltammetry (LSV) data was treated with iR-compensation according to the equation: Ec = Em - Im × Rs, where Ec, Em, Im, and Rs stand for compensated voltage, measured voltage, measured voltage and electrolyte resistance, respectively. The mass loading of synthesized electrocatalysts was about 1.5 mg cm−2. The RuO2 and Pt/C electrodes were also prepared for comparison with the same mass loading.
The synthetic procedure of the composite materials follows a facile, one-step, and hydrothermal method. As shown in Figure 1a, the power X-ray diffraction (XRD) pattern of CoS2@N-GN can be assigned to cubic CoS2 (JCPDS no. 41-1471), suggesting the successful formation of pyrite CoS2 phase in the hybrid. The morphological structures were characterized by scanning electron microscope (SEM) and transmission electron microscope (TEM) techniques. SEM image in Figure 1b revealed that CoS2 nanoparticles were uniformly anchored on nitrogen-doped graphene nanosheets. The average crystallize size is around 73.5 nm (Figure S1), which coincides with the Scherrer analysis based on the XRD pattern. The homogeneous distribution of CoS2 nanocrystals on graphene is further confirmed by the TEM imaging (Figure 1c). Moreover, in the high-resolution TEM (HRTEM) of inset in Figure 1c, an observed lattice spacing of 0.28 nm matches the (200) lattice plane of CoS2 (Ma et al., 2018), further proving the XRD analysis. Otherwise, the elemental mapping reveal the homogeneous dispersion of Co, S, C and N in the composite (Figure 1d), indicating the uniform distribution of CoS2 nanoparticles on the N-doping graphene substance. These observations collectively demonstrate the successful synthesis of CoS2@N-GN hybrid through stepwise controlled strategies. The Brunner–Emmet–Teller (BET) surface area of CoS2@N-GN hybrid was characterized to be 79.8 m2 g−1 (Figure S2). Thus, the CoS2@N-GN shows a significantly high BET area, which can provide more electroactive sites and facilitate electrolyte infiltration during the catalytic process (Li et al., 2018). The content of CoS2 in the hybrid is determined to be around 51 wt% (Figure S3) by thermal gravimetric analysis (TGA) (Liang et al., 2012).
Figure 1. (a) XRD, (b) SEM, (c) TEM image, and (d) elemental mapping of CoS2@N-GN hybrid. Inset in (a) shows the crystal structure of pyrite-type CoS2. Inset in (c) shows the HRTEM image.
The chemical valence states of N, Co, O and S in CoS2@N-GN were characterized by X-ray photoelectron spectroscopy (XPS, Figure 2). The XPS survey spectrum in Figure 2A shows the existence of C, N, Co, S, as well as O. The determined stoichiometric ratio is consistent with the result from energy-dispersive spectroscopy (Figure S4). The existence of O is due to exposure to air (Zhang et al., 2017). Additionally, the high-resolution N 1s spectrum (Figure 2B) can be deconvoluted into three sub-peaks located at 400.30 eV (pyridinic N), 402.20 eV (pyrrolic N), and 403.38 eV (graphitic N) (Fu et al., 2016; Li G. et al., 2016; Wang Z. et al., 2016). It is well known that doped N into carbon framework can potentially increase the active sites and thereby benefit the electrochemical catalytic activity enhancement (Ma et al., 2014; Li et al., 2018). The Co 2p spectra (Figure 2C) can be de-convoluted into six species, including pairs of fitting peaks for Co2+ and Co3+, and their shakeup satellites (Xiao et al., 2013; Ma et al., 2016; Zhu et al., 2016). The Co 2p3/2 peaks at 778.9 and 782.4 eV can be assigned to Co atoms in CoS2@N-GN and surface oxidized cobalt species coordinated with oxide or hydroxyl groups, respectively (Li C. et al., 2017). The main peak centered at 798.9 eV corresponds to spin–orbit characteristic peak of Co 2p1/2 in CoS2 compounds. For the high-resolution S 2p spectrum (Figure 2D), a weak doublet situated at 163.3 eV corresponds to S 2p. The peak located at 163.7 eV is assigned to the S 2p1/2 of S2− ions that matched with metal ions (Du et al., 2015). The peak at 169.7 eV indicates the presence of a oxygen-sulfur (O-S) bond in the CoS2@N-GN compound, which may be ascribed to the surface oxidation, as confirmed by previous observations (Sivanantham et al., 2016).
The electrocatalytic OER performance of synthesized CoS2@N-GN, together with CoS2/N-GN (the mechanical mixtures of pure CoS2 and nitrogen-doped graphene) and pure CoS2, was assessed by a three-electrode configuration in 1.0 M KOH. As shown in the polarization curves (Figure 3A and Table 1), CoS2@N-GN hybrid displays an overpotential of 204 mV to achieve an OER current density of 10 mA cm−2, which is much lower than those of CoS2/N-GN (278 mV) and pure CoS2 (297 mV), highlighting the superior OER activity of in-situ fabricated CoS2@N-GN. The fitted Tafel value of the CoS2@N-GN catalyst is 51.8 mV dec−1, which is lower than those of CoS2/N-GN (71.6 mV dec−1) and pure CoS2 (81.4 mV dec−1) (Figure 3B), indicating more favorable OER kinetics over the CoS2@N-GN surface. Otherwise, the corresponding charge transfer resistance (Rct) values of CoS2@N-GN, CoS2/N-GN and pure CoS2 are fitted to be 1.85, 3.2, 4.02 Ω, respectively (Figure 3C), suggesting that the efficient charge transfer contributes the superior activity of CoS2@N-GN electrode (Li P. et al., 2016). The presented OER performance parameters here are among the non-noble metal-based OER electrocatalysts reported in literatures (Table S1). In addition to good catalytic activity, long-term stability is also a critical factor in evaluating its practical performance. As we can see, the OER polarization curve of CoS2@N-GN after 1000 OER cycles at a scanning rate of 200 mV s−1 almost overlays the initial one (Figure 3D), evidencing that the activity is considerably maintained after the long-term continuous cycles. This is also corroborated by the chronopotentiometric response (Figure 3E), in which that the overpotential does not increase after 12 h period at an anodic current density of 10 mA cm−2. The SEM image of CoS2@N-GN further shows that the morphology is substantially unchanged after the 500 and 1000 OER cycles (Figure S5 and Figure 3f), signaling the remarkable structure durability of the composite catalyst. This may be mainly ascribed to the intrinsic crystal stability of CoS2 and firm attachment between the in situ grown sulfide nanostructures and the graphene support. The possible reaction mechanism of Co-based materials for catalyzing OER in alkaline media follows the sequence (Chen et al., 2015; Ma et al., 2018):
As shown in Figure S6a, after 1000 OER cycles, it can be clearly seen that the proportion of Co3+ is significantly increased. Meanwhile, the proportion of S-O is also significantly increased (Figure S6b), suggesting the considerable oxidation, consistent with previous observations (Han et al., 2018; Ma et al., 2018).
Figure 3. (A) OER LSVs of CoS2@N-GN, CoS2/N-GN, pure CoS2 in 1.0 M KOH at a scanning rate of 5 mV s−1. (B) Corresponding Tafel curves. (C) Nyquist plots of EIS at the potential of 1.56 V. (D) Polarization curves of CoS2@N-GN before and after 1000 cycles. (E) Chronopotentiometric response of CoS2@N-GN at a constant current density of 10 mA cm−2. (F) SEM image of the CoS2@N-GN catalyst after OER for 1000 cycles.
Table 1. Summary of the electrochemical activities of CoS2@N-GN, CoS2/N-GN and pure CoS2 electrodes.
In the context of developing bifunctional catalysts for overall water oxidation, the HER performance of synthesized samples was tested in the same electrolyte. All the linear sweeping voltammetry (LSVs) measurements were collected at a scan rate of 5 mV s−1 while the electrolyte is saturated with N2 during the experiment. From the HER LSVs in Figure 4A, it is clear that the pure CoS2 shows weak electrocatalytic HER activity. The in-situ fabricated CoS2@N-GN achieves a current density of 10 mA cm−2 at an overpotential of 204 mV, which is much lower than that of physical mixture of CoS2/N-GN (278 mV) and pure CoS2 (297 mV).
Figure 4. (A) HER LSV curves of CoS2@N-GN, CoS2/N-GN, pure CoS2 in 1.0 M KOH at a scanning rate of 5 mV s−1. (B) Corresponding Tafel slopes. (C) HER polarization curves of CoS2@N-GN before and after 1,000 cycles. (D) Chronopotentiometric response of CoS2@N-GN at a constant current density of 10 mA cm−2.
The corresponding Tafel slopes of N-GP@CoS2, N-GP/CoS2, and pure CoS2 are 108.5, 139.1, 144.7 mV dec−1, respectively, which is comparable to those of transition metal sulfide catalysts reported recently (Figure 4B) (Liang et al., 2016; Sivanantham et al., 2016). In particular, N-GP@CoS2 shows the lowest Tafel slope of 108.5 mV dec−1 among three synthesized catalysts. The disparity in Tafel slopes further corroborates the advantage of the synergistic effect between N-GN and CoS2. The double-layer capacitance (Cdl) values are determined to be 76.7, 20.1 and 13.6 mF cm−2 for CoS2@N-GN, CoS2/N-GN, and pure CoS2 (Figure S7), demonstrated that the CoS2@N-GN could provide larger electrochemical active area for electrolyte soakage and water adsorption, which is another favorable factor in enhancing the electrocatalytic capability. The HER typically occurs through two reactions in alkaline solution (Li H. et al., 2017; Ma et al., 2018) Volmer reaction (H2O + e− + M → M-H + OH−, where H is a reactive intermediate and M is the catalytically active site) and Heyrovsky reaction (H2O + M-H + e− → M + H2 + OH−). The key HER performance parameters presented here are among the best non-noble metal HER electrocatalysts reported in literatures (Table S2). Moreover, after 1000 CV cycles, the polarization curve of CoS2@N-GN is remarkably maintained in comparison with the initial state (Figure 4C). The morphology of CoS2@N-GN electrode was still essentially preserved (Figure S8), further confirming the structural durability, which is mainly due to the intrinsic stability of CoS2 and the strong chemical interaction between two components. In a continuous polarization period of 12 h (Figure 4D), the HER current retention of CoS2@N-GN again confirms its remarkable long-term stability for catalyzing the HER in alkaline media.
As shown in Figure 5A, to further investigate its practical application for overall water-splitting, CoS2@N-GN electrode was applied as both anode and cathode to assemble an electrochemical device, which presents low cell voltages of 1.70 V at 25°C and 1.53 V at 60°C to achieve 10 mA cm−2 in 1 M KOH. The generated oxygen and hydrogen bubbles on both anode and cathode surface can be obviously seen at 10 mA cm−2 (Figure 5B). In addition, the stability of the electrolyzer device was tested with sustained polarization for 12 h at 1.90 V. The activity degradation of CoS2@N-GN (8.1%) is even lower than that of the combined catalyst of precious Pt/C + RuO2 (17.6%, Figure 5C), further demonstrating the considerable durability of the CoS2@N-GN electrode in a practical water splitting device. As displayed in Figure 5D, the amounts of collected H2 and O2 match well with the calculated values at a constant current density of 10 mA cm−2, which shows advanced concept of efficient energy conversion from electric energy to chemical fuel gas. The relationship between time and gas quantity is calculated according to the following formula:
I, t, e, V and NA are current (mA), time (s), 1.6 × 10−19 C, volume (L) and avogadro constant (6.02 × 1023).
Figure 5. (A) Overall water splitting performance of N-GP@CoS2 at different temperatures. (B) The photograph of gas evolution at 10 mA cm−2. (C) Chronoamperometric response of two overall water splitting systems at 1.9 V. (D) The volumes of H2 and O2 experimentally measured and theoretically calculated vs. time at 10 mA cm−2.
In summary, a novel CoS2@N-GN hybrid was successfully prepared through a facile and one-step hydrothermal strategy. Compared with CoS2/N-GN and pure CoS2 catalyst, CoS2@N-GN hybrid exhibits remarkable overall electrocatalytic activity toward OER and HER in alkaline electrolyte as well as the enhanced long-term stability. Moreover, the CoS2@N-GN enables the assembled water splitting device with low cell voltage, high efficiency and prolonged operational life. The remarkable electrochemical properties are attributed to the high intrinsic activity of CoS2, efficient electron transfer provided by N-doped graphene and the synergetic coupling interaction between two components. This work establishes the low cost and earth-abundant metal sulfide-based composite catalyst as a promising and high performance functional electrode for promoting large-scale water electrolyzer technologies.
WZ conducted the experiments and helped write the manuscript. XM helped with operating the experiments and data analysis. CZ and YD interpreted the results. XH, TM, and WH supervised the research. All authors read and approved the final manuscript.
This work was supported by the National Natural Science Foundation of China (51602216, U1601216), Tianjin Natural Science Foundation (17JCQNJC02100), and Shen-zhen Science and Technology Foundation (JCYJ20170307145703486).
The authors declare that the research was conducted in the absence of any commercial or financial relationships that could be construed as a potential conflict of interest.
The Supplementary Material for this article can be found online at: https://www.frontiersin.org/articles/10.3389/fchem.2018.00569/full#supplementary-material
Chen, P. Z., Xu, K., Fang, Z. W., Tong, Y., Wu, J. C., Lu, X. L., et al. (2015). Metallic Co4N porous nanowire arrays activated by surface oxidation as electrocatalysts for the oxygen evolution reaction. Angew. Chem. Int. Ed. 54,14710–14714. doi: 10.1002/anie.201506480
Cheng, H., Li, M. L., Su, C. Y., Li, N., and Liu, Z. Q. (2017a). Cu-Co bimetallic oxide quantum dot decorated nitrogen-doped carbon nanotubes: a high-efficiency bifunctional oxygen electrode for zn-air batteries. Adv. Funct. Mater. 27:1701833. doi: 10.1002/adfm.201701833
Cheng, H., Su, C.-Y., Tan, Z.-Y., Tai, S.-Z., and Liu, Z.-Q. (2017b). Interacting ZnCo2O4 and Au nanodots on carbon nanotubes as highly efficient water oxidation electrocatalyst. J. Power Sources 357, 1–10. doi: 10.1016/j.jpowsour.2017.04.091
Dong, U. L., Park, M. G., Park, H. W., Min, H. S., Ismayilov, V., Ahmed, R., et al. (2015). Highly active Co-doped LaMnO3 perovskite oxide and N-doped carbon nanotube hybrid bi-functional catalyst for rechargeable zinc–air batteries. Electrochem. Commun. 60, 38–41. doi: 10.1016/j.elecom.2015.08.001
Du, Y., Zhu, X., Zhou, X., Hu, L., Dai, Z., and Bao, J. (2015). Co3S4 porous nanosheets embedded in graphene sheets as high-performance anode materials for lithium and sodium storage. J. Mater. Chem. A 3, 6787–6791. doi: 10.1039/c5ta00621j
Fang, L., Li, W., Guan, Y., Feng, Y., Zhang, H., Wang, S., et al. (2017). Tuning Unique Peapod Like Co(SxSe1−−x)2 nanoparticles for efficient overall water splitting. Adv. Funct. Mater. 27:1701008. doi: 10.1002/adfm.201701008
Forgie, R., Bugosh, G., Neyerlin, K. C., Liu, Z., and Strasser, P. (2010). Bimetallic Ru electrocatalysts for the OER and electrolytic water splitting in acidic media. Electrochem. Solid ST 13, 36–39. doi: 10.1149/1.3290735
Fu, J., Hassan, F. M., Li, J., Lee, D. U., Ghannoum, A. R., Lui, G., et al. (2016). Flexible rechargeable zinc-air batteries through morphological emulation of human hair array. Adv. Mater. 28, 6421–6428. doi: 10.1002/adma.201600762
Gulzar, A., Xu, J., Yang, P., He, F., and Xu, L. (2017). Upconversion processes: versatile biological applications and biosafety. Nanoscale 9, 12248–12282. doi: 10.1039/c7nr01836c
Han, X., Cheng, F., Zhang, T., Yang, J., Hu, Y., and Chen, J. (2014). Hydrogenated uniform Pt Clusters supported on porous CaMnO3 as a bifunctional electrocatalyst for enhanced oxygen reduction and evolution. Adv. Mater. 26, 2047–2051. doi: 10.1002/adma.201304867
Han, X., Wu, X., Deng, Y., Liu, J., Lu, J., Zhong, C., et al. (2018). Ultrafine Pt nanoparticle-decorated pyrite-Type CoS2 nanosheet arrays coated on carbon cloth as a bifunctional electrode for overall water splitting. Adv. Energy Mater. 8:1800935. doi: 10.1002/aenm.201800935
Han, X., Wu, X., Zhong, C., Deng, Y., Zhao, N., and Hu, W. (2017). NiCo2S4 nanocrystals anchored on nitrogen-doped carbon nanotubes as a highly efficient bifunctional electrocatalyst for rechargeable zinc-air batteries. Nano Energy 31, 541–550. doi: 10.1016/j.nanoen.2016.12.008
Huang, C., Ouyang, T., Zou, Y., Li, N., and Liu, Z.-Q. (2018). Ultrathin NiCo2Px nanosheets strongly coupled with CNTs as efficient and robust electrocatalysts for overall water splitting. J. Mater. Chem. A. 6, 7420–7427. doi: 10.1039/C7TA11364A
Jia, Y., Zhang, L., Gao, G., Chen, H., Wang, B., Zhou, J., et al. (2017). A Heterostructure coupling of exfoliated Ni–Fe hydroxide nanosheet and defective graphene as a bifunctional electrocatalyst for overall water splitting. Adv. Mater. 29:1700017. doi: 10.1002/adma.201700017
Kumar, N., Su, W., Veselý, M., Weckhuysen, B. M., Pollard, A. J., and Wain, A. J. (2018). Nanoscale chemical imaging of solid-liquid interfaces using tip-enhanced Raman spectroscopy. Nanoscale 10, 1815–1824. doi: 10.1039/c7nr08257f
Lee, Y., Jin, S., May, K. J., Perry, E. E., and Yang, S. H. (2015). Synthesis and activities of rutile IrO2 and RuO2 nanoparticles for oxygen evolution in acid and alkaline solutions. J. Phys. Chem. Lett. 3, 399–404. doi: 10.1021/jz2016507
Li, C., Du, Y., Wang, D., Yin, S., Tu, W., Chen, Z., et al. (2017). Unique P-Co-N surface bonding states constructed on g-C3N4 nanosheets for drastically enhanced photocatalytic activity of H2 evolution. Adv. Funct. Mater. 27:1604328. doi: 10.1002/adfm.201604328
Li, G., Wang, X., Fu, J., Li, J., Park, M. G., Zhang, Y., et al. (2016). Pomegranate-inspired design of highly active and durable bifunctional electrocatalysts for rechargeable metal-air batteries. Angew. Chem. Int. Ed. 55, 4977–4982. doi: 10.1002/anie.201600750
Li, H., Wen, P., Li, Q., Dun, C., Xing, J., Lu, C., et al. (2017). Earth-abundant iron diboride (FeB2) nanoparticles as highly active bifunctional electrocatalysts for overall water splitting. Adv. Energy Mater. 7:1700513. doi: 10.1002/aenm.201700513
Li, P., Yang, Z., Shen, J., Nie, H., Cai, Q., Li, L., et al. (2016). Subnanometer molybdenum sulfide on carbon nanotubes as a highly active and stable electrocatalyst for hydrogen evolution reaction. ACS Appl. Mater. Interfaces 8, 3543–3550. doi: 10.1021/acsami.5b08816
Li, Y., Zhong, C., Liu, J., Zeng, X., Qu, S., Han, X., et al. (2018). Atomically thin mesoporous Co3O4 layers strongly coupled with N-rGO nanosheets as high-performance bifunctional catalysts for 1D knittable zinc-air batteries. Adv. Mater. 30:1703657. doi: 10.1002/adma.201703657
Liang, Y., Liu, Q., Luo, Y., Sun, X., He, Y., and Asiri, A. M. (2016). Zn0.76Co0.24S/CoS2 nanowires array for efficient electrochemical splitting of water. Electrochim. Acta 190, 360–364. doi: 10.1016/j.electacta.2015.12.153
Liang, Y., Wang, H., Zhou, J., Li, Y., Wang, J., Regier, T., et al. (2012). Covalent hybrid of spinel manganese-cobalt oxide and graphene as advanced oxygen reduction electrocatalysts. J. Am. Chem. Soc. 134, 3517–3523. doi: 10.1021/ja210924t
Liu, J., Wang, J., Zhang, B., Ruan, Y., Lv, L., Ji, X., et al. (2017). Hierarchical NiCo2S4@NiFe LDH Heterostructures Supported on Nickel Foam for Enhanced Overall-Water-Splitting Activity. ACS Appl. Mater. Interfaces 9, 15364–15372. doi: 10.1021/acsami.7b00019
Liu, Q., Jin, J., and Zhang, J. (2013). NiCo2S4@graphene as a bifunctional electrocatalyst for oxygen reduction and evolution reactions. ACS Appl. Mater. Interfaces 5, 5002–5008. doi: 10.1021/am4007897
Ma, F. X., Yu, L., Xu, C. Y., and Lou, X. W. (2016). Self-supported formation of hierarchical NiCo2O4 tetragonal microtubes with enhanced electrochemical properties. Energy Environ. Sci. 9, 862–866. doi: 10.1039/c5ee03772g
Ma, T. Y., Dai, S., Jaroniec, M., and Qiao, S. Z. (2014). Graphitic carbon nitride nanosheet–carbon nanotube three-dimensional porous composites as high-performance oxygen evolution electrocatalysts. Angew. Chem. Int. Ed. 53, 7281–7285. doi: 10.1002/anie.201403946
Ma, X., Wang, J., Liu, D., Kong, R. M., Hao, S., Du, G., et al. (2017). Hydrazine-assisted electrolytic hydrogen production: CoS2 nanoarray as a superior bifunctional electrocatalyst. New J. Chem. 41, 4754–4757. doi: 10.1039/C7NJ00326A
Ma, X., Zhang, W., Deng, Y., Zhong, C., Hu, W., and Han, X. (2018). Phase and composition controlled synthesis of cobalt sulfide hollow nanospheres for electrocatalytic water splitting. Nanoscale 10, 4816–4824. doi: 10.1039/c7nr09424h
Mccrory, C. C. L., Jung, S., Ferrer, I. M., Chatman, S. M., Peters, J. C., and Jaramillo, T. F. (2015). Benchmarking hydrogen evolving reaction and oxygen evolving reaction electrocatalysts for solar water splitting devices. J. Am. Chem. Soc. 137, 4347–4357. doi: 10.1021/ja510442p
Menezes, P. W., Indra, A., Littlewood, P., Schwarze, M., Göbel, C., Schomäcker, R., et al. (2015). Nanostructured manganese oxides as highly active water oxidation catalysts: a boost from manganese precursor chemistry. Chemsuschem 7, 2202–2211. doi: 10.1002/cssc.201402169
Shit, S., Chhetri, S., Jang, W., Murmu, N. C., Koo, H., Samanta, P., et al. (2018). Cobalt sulfide/nickel sulfide heterostructure directly grown on nickel foam: an efficient and durable electrocatalyst for overall water splitting application. ACS Appl. Mater. Interfaces 10, 27712–27722. doi: 10.1021/acsami.8b04223
Sivanantham, A., Ganesan, P., and Shanmugam, S. (2016). Hierarchical NiCo2S4 nanowire arrays supported on ni foam: an efficient and durable bifunctional electrocatalyst for oxygen and hydrogen evolution reactions. Adv. Funct. Mater. 26, 4661–4672. doi: 10.1002/adfm.201600566
Su, C. Y., Cheng, H., Li, W., Liu, Z. Q., Li, N., Hou, Z., et al. (2017). Atomic modulation of FeCo–nitrogen–carbon bifunctional oxygen electrodes for rechargeable and flexible all soli-state zinc-air battery. Adv. Energy Mater. 7:1602420. doi: 10.1002/aenm.201602420
Sun, Y., Hang, L., Shen, Q., Zhang, T., Li, H., Zhang, X., et al. (2017). Mo doped Ni2P nanowire arrays: an efficient electrocatalyst for the hydrogen evolution reaction with enhanced activity at all pH values. Nanoscale 9, 16674–16679. doi: 10.1039/c7nr03515b
Wang, H., Lee, H. W., Deng, Y., Lu, Z., Hsu, P. C., Liu, Y., et al. (2015). Bifunctional non-noble metal oxide nanoparticle electrocatalysts through lithium-induced conversion for overall water splitting. Nat. Commun. 6, 7261–7269. doi: 10.1038/ncomms8261
Wang, J., Zhong, H., Wang, Z., Meng, F., and Zhang, X. (2016). Integrated three-dimensional carbon paper/carbon tubes/cobalt-sulfide sheets as an efficient electrode for overall water splitting. ACS Nano 10, 2342–2348. doi: 10.1021/acsnano.5b07126
Wang, K., Liu, X. K., Chen, X. H., Yu, D. G., Yang, Y. Y., and Liu, P. (2018). Electrospun hydrophilic janus nanocomposites for the rapid onset of therapeutic action of helicid. ACS Appl. Mater. Interfaces 10, 2859–2867. doi: 10.1021/acsami.7b17663
Wang, X. Z., Liu, S. S., Sun, Y., Wu, J. Y., Zhou, Y. L., and Zhang, J. H. (2009). Beta-cypermethrin impairs reproductive function in male mice by inducing oxidative stress. Theriogenology 72, 599–611. doi: 10.1016/j.theriogenology.2009.04.016
Wang, Z., Li, B., Ge, X., Goh, F. W. T., Zhang, X., Du, G., et al. (2016). Co@Co3O4@PPD Core@bishell nanoparticle-based composite as an efficient electrocatalyst for oxygen reduction reaction. Small 12, 2580–2587. doi: 10.1002/smll.201503694
Wu, X., Han, X., Ma, X., Zhang, W., Deng, Y., Zhong, C., et al. (2017). Morphology-controllable synthesis of Zn-Co-mixed sulfide nanostructures on carbon fiber paper toward efficient rechargeable Zinc-air batteries and water electrolysis. ACS Appl. Mater. Interfaces 9, 12574–12583. doi: 10.1021/acsami.6b16602
Xia, C., Liang, H., Zhu, J., Schwingenschlögl, U., and Alshareef, H. N. (2017). Active edge sites engineering in nickel cobalt selenide solid solutions for highly efficient hydrogen evolution. Adv. Energy Mater. 7:1602089. doi: 10.1002/aenm.201602089
Xiao, J., Kuang, Q., Yang, S., Xiao, F., Wang, S., and Guo, L. (2013). Surface structure dependent electrocatalytic activity of Co3O4anchored on graphene sheets toward oxygen reduction reaction. Sci. Rep. 3, 2300–2308. doi: 10.1038/srep02300
Yin, J., Li, Y., Lv, F., Lu, M., Sun, K., Wang, W., et al. (2017). Oxygen vacancies dominated NiS2/CoS2 interface porous nanowires for portable Zn–air batteries driven water splitting devices. Adv. Mater. 29:1704681. doi: 10.1002/adma.201704681
Zhang, G., Wang, P., Lu, W. T., Wang, C. Y., Li, Y. K., Ding, C., et al. (2017). Co nanoparticles/Co, N, S tri-doped graphene templated from in-situ-formed Co, S Co-doped g-C3N4 as an active bifunctional electrocatalyst for overall water splitting. ACS Appl. Mater. Interfaces 9, 28566–28576. doi: 10.1021/acsami.7b08138
Zheng, G., Peng, Z., Jia, D., Al-Enizi, A. M., and Elzatahry, A. A. (2015). From water oxidation to reduction: Homologous Ni-Co based nanowires as complementary water splitting electrocatalysts. Adv. Energy Mater. 5:1402031. doi: 10.1002/aenm.201402031
Zheng, M., Ding, Y., Yu, L., Du, X., and Zhao, Y. (2017). In Situ grown pristine cobalt sulfide as bifunctional photocatalyst for hydrogen and oxygen evolution. Adv. Funct. Mater. 27:1605846. doi: 10.1002/adfm.201605846
Zhou, S., Zhou, Q.-X., Su, H., Wang, Y., Dong, Z., Dai, X., et al. (2019). Hybrid of Fe3C@N, S co-doped carbon nanotubes coated porous carbon derived from metal organic frameworks as an efficient catalyst towards oxygen reduction. J. Colloid Inter. Sci. 533, 311–318. doi: 10.1016/j.jcis.2018.06.091
Keywords: water splitting, cobalt sulfide, nanoparticle, graphene, HER/OER, composite
Citation: Zhang W, Ma X, Zhong C, Ma T, Deng Y, Hu W and Han X (2018) Pyrite-Type CoS2 Nanoparticles Supported on Nitrogen-Doped Graphene for Enhanced Water Splitting. Front. Chem. 6:569. doi: 10.3389/fchem.2018.00569
Received: 05 October 2018; Accepted: 01 November 2018;
Published: 21 November 2018.
Edited by:
Wee-Jun Ong, Xiamen University Malaysia, MalaysiaReviewed by:
Zhao-Qing Liu, Guangzhou University, ChinaCopyright © 2018 Zhang, Ma, Zhong, Ma, Deng, Hu and Han. This is an open-access article distributed under the terms of the Creative Commons Attribution License (CC BY). The use, distribution or reproduction in other forums is permitted, provided the original author(s) and the copyright owner(s) are credited and that the original publication in this journal is cited, in accordance with accepted academic practice. No use, distribution or reproduction is permitted which does not comply with these terms.
*Correspondence: Tianyi Ma, dGlhbnlpLm1hQG5ld2Nhc3RsZS5lZHUuYXU=
Xiaopeng Han, eHBoYW5AdGp1LmVkdS5jbg==
Disclaimer: All claims expressed in this article are solely those of the authors and do not necessarily represent those of their affiliated organizations, or those of the publisher, the editors and the reviewers. Any product that may be evaluated in this article or claim that may be made by its manufacturer is not guaranteed or endorsed by the publisher.
Research integrity at Frontiers
Learn more about the work of our research integrity team to safeguard the quality of each article we publish.