- 1State Key Laboratory for Artificial Microstructures and Mesoscopic Physics, Department of Physics, Peking University, Beijing, China
- 2Department of Chemistry, National Taiwan University, Taipei, Taiwan
- 3Department of Chemistry, King Saud University, Riyadh, Saudi Arabia
- 4New Display Device and System Integration Collaborative Innovation Center of the West Coast of the Taiwan Strait, Fuzhou, China
Two novel small molecules DTRDTQX and DTIDTQX, based on ditolylaminothienyl group as donor moiety and quinoxaline as middle acceptor moiety with different terminal acceptor groups were synthesized and characterized in this work. In order to study the photovoltaic properties of DTRDTQX and DTIDTQX, bulk-heterojunction solar cells with the configuration of FTO/c-TiO2/DTRDTQX(or DTIDTQX):C70/MoO3/Ag were fabricated, in which DTRDTQX and DTIDTQX acted as the donors and neat C70 as the acceptor. When the weight ratio of DTRDTQX:C70 reached 1:2 and the active layer was annealed at 100°C, the optimal device was realized with the power conversion efficiency (PCE) of 1.44%. As to DTIDTQX:C70-based devices, the highest PCE of 1.70% was achieved with the optimal blend ratio (DTIDTQX:C70 = 1:2) and 100°C thermal annealing treatment. All the experimental data indicated that DTRDTQX and DTIDTQX could be employed as potential donor candidates for organic solar cell applications.
Introduction
Recently, organic solar cells (OSCs) based on bulk-heterojunction structure have attracted much attention due to the distinctive characteristics of low cost, easy fabrication, flexibility and light weight, etc. (Gustafsson et al., 1992; Shaheen et al., 2001; Chen and Cao, 2009). Compared with polymers employed in solar cells, small molecule donors have the advantage of less batch-to-batch variation, well-defined molecular structure, easier purification, etc. (You et al., 2013; Chen et al., 2014, 2015; He et al., 2015; Zhou et al., 2015). Therefore, much work focused on small molecule donors and the photovoltaic performance of OSCs was improved accordingly (Sun et al., 2011; Liu et al., 2013; Love et al., 2013; Coughlin et al., 2014). In general, the active layers of the solar cells consisted of small molecule donors and fullerene/fullerene derivative acceptors (Chen et al., 2012; Huang et al., 2016). In order to optimize the photovoltaic characteristics of OSCs, narrow band-gap and deep highest occupied molecular orbital (HOMO) of small molecule donors should be considered, which resulted in broad absorption and high open-circuit voltage (Voc) of devices. Then, various small molecules composed of electron rich moieties (donor, “D”) and electron deficient moieties (acceptor, “A”), have been reported with the molecular configuration such as D-A (Roquet et al., 2006), A-D-A (Schulze et al., 2006), D-A-A (Lin et al., 2011) and D-A-D conjugated structures. In this regard, the HOMO and lowest unoccupied molecular orbital (LUMO) of the small molecules were effectively tuned, mainly due to the intramolecular charge transfer (ICT) between donors and acceptors (Zhang et al., 2011).
Herein, the photovoltaic properties of two novel small molecule donors (named DTRDTQX and DTIDTQX, Figure 1) based on D-A-A structure were studied in this work. DTIDTQX or DTRDTQX consisted of ditolylaminothienyl group as the donor moiety, quinoxaline as middle acceptor moiety with different terminal acceptor groups such as 1,3-indandione or 3-ethylrhodanine, respectively. To investigate the photovoltaic properties of the small molecules, bulk-heterojunction (BHJ) solar cells based on DTRDTQX or DTIDTQX as the donor together with C70 as the acceptor were fabricated and the optimal cells showed PCE of 1.44 and 1.70%, respectively.
Experimental
Materials and Characterization
All materials in this work were purchased commercially, except for the tailor made DTRDTQX and DTIDTQX donors. The commercial materials were used without further purification.
Scheme 1 depicts the synthesis of DTIDTQX and DTRDTQX. By following the protocols established by Krebs et al. (Jorgensen and Krebs, 2005) and Janssen et al. (Bijleveld et al., 2009), we could get 4-bromo-7-methyl-2,1,3-benzo-thiadiazole (3). Then the hetereocyclic 3 was converted to diamine intermediate 4 by treating Fe/HCl, which was then followed by condensation with glyoxal to afford 5-bromo-8-methylquinoxaline (5) without further purification. The 8-bromoquinoxaline-5-carbaldehyde (7) was synthesized by benzylic bromination with N-bromosuccinimide (NBS) initiated by azobisisobutyronitrile (AIBN) and followed by hydrolysis with CaCO3 in H2O/acetonitrile (Lin et al., 2011). Aldehyde 7 was reacted with N,N-di-p-tolyl-5-(tri-n-butylstannyl)-thiophen-2-amine (8) through Stille coupling reaction and gave key intermediate 9. Finally, the condensation of 9 with 1,3-indandione and 3-ethylrhodanine via Knöevenagel reaction afforded DTIDTQX and DTRDTQX, respectively. The absorption spectra were measured with JASCO V-670 spectrophotometer. Themogravimetric analysis (TGA) was determined on a TA Instruments Model TGA Q500 V20.13 (build 39) with a heating rate of 10°C/min. Differential Scanning Calorimeter (DSC) was carried out at a heating rate of 10°C/min on a TA Instruments Model DSC Q100 V9.9 (build 303). The thickness of the films was evaluated using a surface profilometer. The electrochemical cyclic voltammetry (CV) was recorded by a CHI619B potentiostat with glassy carbon electrode, Pt wire and Ag/AgCl which were used as the working electrode, counter electrode, and reference electrode, respectively, further calibrated with the ferrocene/ferrocenium (Fc/Fc+) redox couple. The oxidation waves were recorded in CH2Cl2 (for 1.0 mM) with 0.1 M tetrabutylammonium hexafluorophosphate (nBuNPF6) as supporting electrolyte, while reductive waves were recorded in THF (for 1.0 mM) with 0.1 M tetrabutylammonium perchlorate (nBuNClO4) as supporting electrolyte.
Solar Cell Fabrication and Characterization
In order to investigate the photovoltaic properties of DTRDTQX and DTIDTQX, the OSCs with the configuration of FTO/c-TiO2/DTRDTQX(or DTIDTQX):C70/MoO3/Ag were fabricated as shown in Figure 1. The compact TiO2 layer in OSCs acted as the electron transporting layer (Heo et al., 2015) and MoO3 as the hole buffer layer. As to the photoactive layers, DTRDTQX and DTIDTQX served as the donors and C70 as the acceptor, respectively. The FTO cathode was pre-cleaned in an ultrasonic cleaner with deionized water, acetone and alcohol for 15 min respectively and then treated with oxygen plasma for 15 min. The TiO2 films were fabricated according to the literatures (Kim et al., 2012; Zhang et al., 2016) and sintered at 500°C for 15 min in a muffle furnace. And then, the TiO2 films were naturally cooled to room temperature. Blended solutions (total concentration: 20 mg/ml) of DTRDTQX(or DTIDTQX):C70 in ortho-dichlorobenzene (oDCB) were spin-coated (700 rpm, 18 s) onto FTO/TiO2 substrates in a glove box and then thermal annealed at 100°C or 150°C. The effect of thermal annealing on the photovoltaic properties of the active layers was also studied in this work. Finally, 7 nm MoO3 buffer layers and 100 nm Ag anodes were thermal evaporated successively below 10−6 Torr. The photovoltaic performance of the OSCs were evaluated by current density-bias voltage (J-V) measurement (using a Keithley 2400 source meter) under AM 1.5G simulated solar illumination (Newport model 94021A, 100 mW cm−2).
Results and Discussion
Thermal Property
Thermal properties of the two small molecules were investigated by TGA measurement as shown in Figure 2 and the thermal decomposition temperatures (Td, 5% weight loss) were evaluated to be 362°C and 312°C for DTRDTQX and DTIDTQX respectively, indicating the good thermal stability of the small molecules. According to the DSC plots shown in Figure 3, the melting temperatures (Tm) were evaluated to be 187.8°C and 263.3°C for DTRDTQX and DTIDTQX, respectively. Moreover, the glass transition temperatures (Tg) were measured to be 94.0°C and 149.7°C for DTRDTQX and DTIDTQX, respectively. Therefore, both DTRDTQX and DTIDTQX were stable donors for OSCs due to their decent thermal stability.
Absorption Properties
The UV-Vis absorption of DTIDTQX and DTRDTQX in CH2Cl2 were shown in Figure 4 and the corresponding data were summarized in Table 1. The compounds showed broad band absorption from 480 to 750 nm with high extinction coefficient (3.3–3.5 × 104 M−1cm−1) in the visible range (450–700 nm). DTIDTQX absorbed longer wavelength than DTRDTQX (631 vs. 588 nm), mainly due to the stronger electron withdrawing ability of 1,3-indanedione group than that of N-ethylrhodanine group.
Electrochemical Properties
The electrochemical properties of DTRDTQX and DTIDTQX were studied with cyclic voltammetry (CV) as shown in Figure 5. In addition, the energy levels as well as the band gaps of DTRDTQX and DTIDTQX were summarized in Table 1. With the oxidation and reduction potentials recorded, the HOMO and LUMO levels of the two materials could be calculated (HOMO = −5.1 eV – , LUMO = −5.1 eV – ), which were −5.33 eV, −3.96 eV for DTIDTQX and −5.29 eV, −3.59 eV for DTRDTQX respectively. Interestingly, the HOMO and LUMO levels of DTIDTQX were both deeper than those of DTRDTQX. The phenomenon implied that the electron withdrawing ability of 1,3-indanedione group was stronger than that of N-ethylrhodanine group, which was consistent with the observation of UV-Vis absorption. The energy levels of the materials used in the OSCs were depicted in Figure 6. The large gap between the low-lying HOMO level (−5.33 eV) of DTIDTQX and LUMO (−4.20 eV) of C70 was evaluated to be 1.13 eV, which resulted in the large Voc (0.71 V) of the optimal DTIDTQX-based OSCs in this work. Furthermore, the electrochemical energy band gap (ΔECV) of DTIDTQX was 0.33 eV lower than that of DTRDTQX and strong absorption of DTIDTQX active layer in red region could be realized, which was matched well with the UV-Vis absorption spectrum shown in Figure 4. Therefore, the light-harvesting capability as well as the photovoltaic performance of DTIDTQX-based devices could be superior to that of DTRDTQX-based counterparts, which will be discussed further in following.
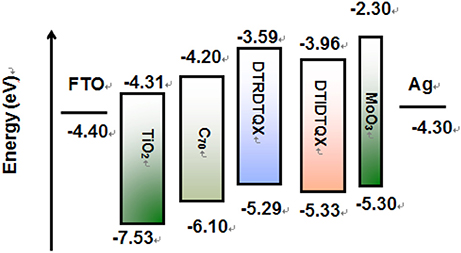
Figure 6. The energy levels of the materials used in the OSCs (Lau et al., 2013; Zhang et al., 2013; Xiao et al., 2016).
Photovoltaic Properties
To study the photovoltaic properties of the small molecules, OSCs with the structure of FTO/c-TiO2/donor:C70/MoO3/Ag were fabricated. The weight ratios of DTRDTQX:C70 and DTIDTQX:C70 varied from 1:1 to 1:3 and the corresponding J-V curves of the OSCs were shown in Figures 7, 8. All the photovoltaic data of OSCs were summarized in Table 2. When the weight ratio of DTRDTQX:C70 reached 1:2 and the photoactive layer was thermal annealed at 100°C, the best DTRDTQX-based OSC was realized with the short-circuit current density (Jsc) and PCE of 5.66 mA/cm2 and 1.44%, respectively. The champion DTRDTQX-based OSC exhibited almost the same open-circuit voltage (Voc) of ~0.65 V as other OSCs with different weight ratios (1:1 and 1:3) of DTRDTQX:C70. Moreover, for the devices based on DTRDTQX:C70 with the weight ratios of 1:1 and 1:3, the decreased Jsc was mainly ascribed to the imbalanced electron and hole diffusion in the OSCs (Kim et al., 2009). The photovoltaic data in Table 2 implied that the weight ratio (DTRDTQX:C70) of 1:2 was advantageous to the photovoltaic performance of DTRDTQX:C70-based OSCs. The photovoltaic properties of DTRDTQX:C70(1:2)-based OSCs with 150°C thermal annealing and without thermal annealing were also studied and compared. The Voc and PCE of the OSC with 150°C thermal annealing were decreased to 0.51 V and 1.19%, respectively. As to the OSC without thermal annealing, the PCE was decreased to 1.14% and Voc (~0.66V) was almost unchanged compared with the champion DTRDTQX-based OSC. Therefore, 100°C thermal annealing treatment was necessary for the reasonable photovoltaic performance of DTRDTQX:C70(1:2)-based OSCs according to the experimental data.
As to DTIDTQX-based OSCs, the photovoltaic performance was modulated by the weight ratios of DTIDTQX:C70 from 1:1 to 1:3. When the blend ratio of DTIDTQX:C70 reached 1:2, the best DTIDTQX-based OSC was realized as shown in Table 2. The Voc, Jsc, FF, and PCE of the champion device were 0.71V, 6.24 mA/cm2, 0.38 and 1.70%, respectively. It was worthy to note that the Voc of DTIDTQX:C70(1:2)-OSC was 0.06 V higher than that of DTRDTQX:C70(1:2)-OSC, mainly due to the low-lying HOMO (−5.33 eV) of DTIDTQX as shown in Figure 6. Moreover, the Jsc and PCE of DTIDTQX:C70(1:2)-OSC were both higher than those of DTRDTQX:C70(1:2)-OSC. Therefore, the photovoltaic properties of DTIDTQX-based devices were superior to those of DTRDTQX-based counterparts, which was mainly ascribed to the narrow band gap (~1.37 eV) of DTIDTQX and the consequent effective absorption in solar spectrum. The photovoltaic performance of DTIDTQX:C70(1:2)-OSC was deteriorated when the active layer was treated with 150°C thermal annealing as shown in Table 2. And when DTIDTQX:C70(1:2)-OSC was fabricated without thermal annealing, the PCE decreased to 1.26%. Therefore, 100°C thermal annealing was favorable to DTIDTQX:C70(1:2)-OSC and a decent PCE of 1.70% was obtained accordingly. However, the FF values of the OSCs were relatively low in this work and much work should be required to further increase FF as well as PCE of the OSCs, such as inserting buffer layers (Ji et al., 2016; Li et al., 2016; Mbuyise et al., 2016), introducing optical spacers (Ben Dkhil et al., 2014), employing solvent annealing (Sun et al., 2014; Li et al., 2015), chemical treatments (Bai et al., 2015), etc.
The morphology of DTRDTQX:C70(1:2) and DTIDTQX:C70(1:2) films was studied by atomic force microscopy (AFM) (Agilent Series 5500) as shown in Figure 9. The root-mean-square roughness (RMS) of DTIDTQX:C70 (1:2) film was 2.94 nm, which was a little higher than that of DTRDTQX:C70 (1:2) film (2.58 nm), The relatively low RMS of DTRDTQX:C70(1:2) and DTIDTQX:C70(1:2) facilitated the reasonable photovoltaic performance of the corresponding devices. Besides, the external quantum efficiency (EQE) spectra of the champion devices were measured with a lock-in amplifier (model SR830 DSP) as shown in Figure 10. The EQE of DTIDTQX-based device was higher than that of DTRDTQX-based counterpart and the integrated photocurrent was 5.47 and 4.71 mA/cm2, respectively, which was consistent with the photovoltaic properties of the corresponding OSCs. In order to further study the charge transporting properties of the p-type small molecules, hole mobility was measured by using the space-charge-limited current (SCLC) method and the structure of the hole-only devices was ITO/PEDOT:PSS/donor/Au. The J1/2-V curves were measured as shown in Supplementary Material. The relation of J and V could be described by J = 9ε0εμ(Vapp-Vs-Vbi)2/8L3, where J was the current density, ε0 was the permittivity of free space, ε was the relative permittivity of the p-type small molecules, μ was the hole mobility, Vapp was the applied voltage, Vs was the voltage drop from series resistance of the substrate, Vbi was the built-in voltage and L was the thickness of the active layers (Qu et al., 2017). The hole mobilities were calculated with the fitted slope of the J1/2-V curves, which were 3.62*10−6 cm2 V−1 s−1 and 2.27*10−5 cm2 V−1 s−1 for DTRDTQX and DTIDTQX, respectively. The hole mobility of DTIDTQX was higher than that of DTRDTQX, which contributed to the decent photovoltaic performance of DTIDTQX-based OSCs. All the experimental data showed that DTIDTQX and DTRDTQX were promising donor candidates for small molecule OSCs and improved photovoltaic performance of OSCs based on DTIDTQX and DTRDTQX would be foreseen in the future.
Conclusions
Two small molecules DTRDTQX and DTIDTQX with the D-A-A structure were studied in this work. DTRDTQX and DTIDTQX were used as the donors in bulk-heterojunction solar cells. The optimal OSCs based on DTRDTQX:C70(1:2) and DTIDTQX:C70(1:2) were achieved with the PCE of 1.44% and 1.70%, respectively. The photovoltaic properties of DTIDTQX were superior to those of DTRDTQX, which was attributed to the narrow band gap (1.37 eV) and the high hole mobility (2.27*10−5 cm2 V−1 s−1) of DTIDTQX. Therefore, DTRDTQX and DTIDTQX would be promising donor materials for organic solar cells in future.
Author Contributions
All authors listed have made a substantial, direct and intellectual contribution to the work, and approved it for publication.
Funding
This work was supported by the National Natural Science Foundation of China under grant Nos 11574013, U1605244, and 11527901, the National Fund for Fostering Talents of Basic Science (NFFTBS) with grant No. J1030310 and J1103205, the authors also extend their appreciation for the support from the International Scientific Partnership Program ISPP at King Saud University, ISPP#0112.
Conflict of Interest Statement
The authors declare that the research was conducted in the absence of any commercial or financial relationships that could be construed as a potential conflict of interest.
The reviewer XZ and handling Editor declared their shared affiliation.
Supplementary Material
The Supplementary Material for this article can be found online at: https://www.frontiersin.org/articles/10.3389/fchem.2018.00260/full#supplementary-material
References
Bai, S., Jin, Y. Z., Liang, X. Y., Ye, Z. Z., Wu, Z. W., Sun, B. Q., et al. (2015). Ethanedithiol treatment of solution-processed ZnO thin films: controlling the intragap states of electron transporting interlayers for efficient and stable inverted organic photovoltaics. Adv. Energy Mater. 5:1401606. doi: 10.1002/aenm.201401606
Ben Dkhil, S., Duche, D., Gaceur, M., Thakur, A. K., Aboura, F. B., Escoubas, L., et al. (2014). Interplay of optical, morphological, and electronic effects of ZnO optical spacers in highly efficient polymer solar cells. Adv. Energy Mater. 4:1400805. doi: 10.1002/aenm.201400805
Bijleveld, J. C., Shahid, M., Gilot, J., Wienk, M. M., and Janssen, R. A. J. (2009). Copolymers of cyclopentadithiophene and electron-deficient aromatic units designed for photovoltaic applications. Adv. Funct. Mater. 19, 3262–3270. doi: 10.1002/adfm.200900412
Cardona, C. M., Li, W., Kaifer, A. E., Stockdale, D., and Bazan, G. C. (2011). Electrochemical considerations for determining absolute frontier orbital energy levels of conjugated polymers for solar cell applications. Adv. Mater. 23, 2367–2371. doi: 10.1002/adma.201004554
Chen, C. C., Chang, W. H., Yoshimura, K., Ohya, K., You, J. B., Gao, J., et al. (2014). An efficient triple-junction polymer solar cell having a power conversion efficiency exceeding 11%. Adv. Mater. 26 5670–5677. doi: 10.1002/adma.201402072
Chen, J., and Cao, Y. (2009). Development of novel conjugated donor polymers for high-efficiency bulk-heterojunction photovoltaic devices. Acc. Chem. Res. 42, 1709–1718. doi: 10.1021/ar900061z
Chen, J. D., Cui, C., Li, Y. Q., Zhou, L., Ou, Q. D., Li, C., et al. (2015). Single-junction polymer solar cells exceeding 10% power conversion efficiency. Adv. Mater. 27, 1035–1041. doi: 10.1002/adma.201404535
Chen, Y. H., Lin, L. Y., Lu, C. W., Lin, F., Huang, Z. Y., Lin, H. W., et al. (2012). Vacuum-deposited small-molecule organic solar cells with high power conversion efficiencies by judicious molecular design and device optimization. J. Am. Chem. Soc. 134, 13616–13623. doi: 10.1021/ja301872s
Coughlin, J. E., Henson, Z. B., Welch, G. C., and Bazan, G. C. (2014). Design and synthesis of molecular donors for solution-processed high-efficiency organic solar cells. Acc. Chem. Res. 47, 257–270. doi: 10.1021/ar400136b
Gustafsson, G., Cao, Y., Treacy, G. M., Klavetter, F., Colaneri, N., and Heeger, A. J. (1992). Flexible light-emitting-diodes made from soluble conducting polymers. Nature 357, 477–479. doi: 10.1038/357477a0
He, Z. C., Xiao, B., Liu, F., Wu, H. B., Yang, Y. L., Xiao, S., et al. (2015). Single-junction polymer solar cells with high efficiency and photovoltage. Nat. Photonics 9, 174–179. doi: 10.1038/nphoton.2015.6
Heo, J. H., Song, D. H., Han, H. J., Kim, S. Y., Kim, J. H., Kim, D., et al. (2015). Planar CH3NH3PbI3 perovskite solar cells with constant 17.2% average power conversion efficiency irrespective of the scan rate. Adv. Mater. 27, 3424–3430. doi: 10.1002/adma.201500048
Huang, J., Zhang, S., Jiang, B., Chen, Y., Zhang, X., Fan, Z., et al. (2016). Terminal moiety-driven electrical performance of asymmetric small-molecule-based organic solar cells. J. Mater. Chem. A 4, 15688–15697. doi: 10.1039/C6TA07450B
Ji, C. H., Jang, J. M., and Oh, S. Y. (2016). Performance of organic photovoltaics using an ytterbium trifluoride n-type buffer layer. Electr. Mater. Lett. 12, 301–307. doi: 10.1007/s13391-016-5451-4
Jorgensen, M., and Krebs, F. C. (2005). Stepwise unidirectional synthesis of oligo phenylene vinylenes with a series of monomers. Use in plastic solar cells. J. Org. Chem. 70, 6004–6017. doi: 10.1021/jo0506783
Kim, B. J., Miyamoto, Y., Ma, B. W., and Frechet, J. M. J. (2009). Photocrosslinkable polythiophenes for efficient, thermally stable, organic photovoltaics. Adv. Funct. Mater. 19, 2273–2281. doi: 10.1002/adfm.200900043
Kim, H. S., Lee, C. R., Im, J. H., Lee, K. B., Moehl, T., Marchioro, A., et al. (2012). Lead iodide perovskite sensitized all-solid-state submicron thin film mesoscopic solar cell with efficiency exceeding 9%. Sci. Rep. 2:591. doi: 10.1038/srep00591
Lau, X. B. C., Wang, Z. Q., and Mitra, S. (2013). A C70-carbon nanotube complex for bulk heterojunction photovoltaic cells. Appl. Phys. Lett. 103:243108. doi: 10.1063/1.4847376
Li, J. S., Jiu, T. G., Li, B. R., Kuang, C. Y., Chen, Q. S., Ma, S. S., et al. (2016). Inverted polymer solar cells with enhanced fill factor by inserting the potassium stearate interfacial modification layer. Appl. Phys. Lett. 108:181602. doi: 10.1063/1.4948585
Li, M., Liu, F., Wan, X., Ni, W., Kan, B., Feng, H., et al. (2015). Subtle balance between length scale of phase separation and domain purification in small-molecule bulk-heterojunction blends under solvent vapor treatment. Adv. Mater. 27, 6296–6302. doi: 10.1002/adma.201502645
Lin, L. Y., Chen, Y. H., Huang, Z. Y., Lin, H. W., Chou, S. H., Lin, F., et al. (2011). A low-energy-gap organic dye for high-performance small-molecule organic solar cells. J. Am. Chem. Soc. 133, 15822–15825. doi: 10.1021/ja205126t
Liu, Y., Chen, C. C., Hong, Z., Gao, J., Yang, Y. M., Zhou, H., et al. (2013). Solution-processed small-molecule solar cells: breaking the 10% power conversion efficiency. Sci. Rep. 3:3356. doi: 10.1038/srep03356
Love, J. A., Proctor, C. M., Liu, J. H., Takacs, C. J., Sharenko, A., van der Poll, T. S., et al. (2013). Film morphology of high efficiency solution-processed small-molecule solar cells. Adv. Funct. Mater. 23, 5019–5026. doi: 10.1002/adfm.201300099
Mbuyise, X. G., Tonui, P., and Mola, G. T. (2016). The effect of interfacial layers on charge transport in organic solar cell. Phys. B Condens. Matter 496, 34–37. doi: 10.1016/j.physb.2016.05.021
Qu, B., Wu, H., Zhao, B., Liu, H., Gao, C., Qi, X., et al. (2017). An alternating polymer with fluorinated quinoxaline and 2,7-carbazole segments for photovoltaic devices. RSC Adv. 7, 16041–16048. doi: 10.1039/C6RA28128A
Roquet, S., Cravino, A., Leriche, P., Aleveque, O., Frere, P., and Roncali, J. (2006). Triphenylamine-thienylenevinylene hybrid systems with internal charge transfer as donor materials for heterojunction solar cells. J. Am. Chem. Soc. 128, 3459–3466. doi: 10.1021/ja058178e
Schulze, K., Uhrich, C., Schuppel, R., Leo, K., Pfeiffer, M., Brier, E., et al. (2006). Efficient vacuum-deposited organic solar cells based on a new low-bandgap oligothiophene and fullerene C-60. Adv. Mater. 18, 2872–2875. doi: 10.1002/adma.200600658
Shaheen, S. E., Radspinner, R., Peyghambarian, N., and Jabbour, G. E. (2001). Fabrication of bulk heterojunction plastic solar cells by screen printing. Appl. Phys. Lett. 79, 2996–2998. doi: 10.1063/1.1413501
Sun, K., Xiao, Z., Hanssen, E., Klein, M. F. G., Dam, H. H., Pfaff, M., et al. (2014). The role of solvent vapor annealing in highly efficient air-processed small molecule solar cells. J. Mater. Chem. A 2, 9048–9054. doi: 10.1039/c4ta01125b
Sun, Y., Welch, G. C., Leong, W. L., Takacs, C. J., Bazan, G. C., and Heeger, A. J. (2011). Solution-processed small-molecule solar cells with 6.7% efficiency. Nat. Mater. 11, 44–48. doi: 10.1038/nmat3160
Xiao, Y. M., Han, G. Y., Wu, J. H., and Lin, J. Y. (2016). Efficient bifacial perovskite solar cell based on a highly transparent poly(3,4-ethylenedioxythiophene) as the p-type hole-transporting material. J. Pow. Sour. 306, 171–177. doi: 10.1016/j.jpowsour.2015.12.003
You, J., Dou, L., Yoshimura, K., Kato, T., Ohya, K., Moriarty, T., et al. (2013). A polymer tandem solar cell with 10.6% power conversion efficiency. Nat. Commun. 4:1446. doi: 10.1038/ncomms2411
Zhang, H., Xu, M. F., Cui, R. L., Guo, X. H., Yang, S. Y., Liao, L. S., et al. (2013). Enhanced performance of inverted organic photovoltaic cells using CNTs-TiOX nanocomposites as electron injection layer. Nanotechnology 24:355401. doi: 10.1088/0957-4484/24/35/355401
Zhang, S. D., Lei, L., Yang, S. W., Li, X. M., Liu, Y., Gao, Q. Q., et al. (2016). Influence of TiO2 blocking layer morphology on planar heterojunction perovskite solar cells. Chem. Lett. 45, 592–594. doi: 10.1246/cl.160059
Zhang, Z. G., Yang, Y., Zhang, S. Y., Min, J., Zhang, J., Zhang, M. J., et al. (2011). Effect of acceptor substituents on photophysical and photovoltaic properties of triphenylamine-carbazole alternating copolymers. Synth. Met. 161, 1383–1389. doi: 10.1016/j.synthmet.2011.05.005
Keywords: bulk-heterojunction, small molecule, donor, solar cell, ditolylaminothienyl, quinoxaline
Citation: Qi X, Lo Y-C, Zhao Y, Xuan L, Ting H-C, Wong K-T, Rahaman M, Chen Z, Xiao L and Qu B (2018) Two Novel Small Molecule Donors and the Applications in Bulk-Heterojunction Solar Cells. Front. Chem. 6:260. doi: 10.3389/fchem.2018.00260
Received: 09 March 2018; Accepted: 11 June 2018;
Published: 02 July 2018.
Edited by:
Chuanlang Zhan, Institute of Chemistry (CAS), ChinaReviewed by:
Xiaozhang Zhu, Institute of Chemistry (CAS), ChinaDaniel Glossman-Mitnik, Centro de Investigación en Materiales Avanzados, Mexico
Copyright © 2018 Qi, Lo, Zhao, Xuan, Ting, Wong, Rahaman, Chen, Xiao and Qu. This is an open-access article distributed under the terms of the Creative Commons Attribution License (CC BY). The use, distribution or reproduction in other forums is permitted, provided the original author(s) and the copyright owner(s) are credited and that the original publication in this journal is cited, in accordance with accepted academic practice. No use, distribution or reproduction is permitted which does not comply with these terms.
*Correspondence: Ken-Tsung Wong, a2Vud29uZ0BudHUuZWR1LnR3
Bo Qu, YnF1QHBrdS5lZHUuY24=