- 1Departamento de Quimica Organica, Universidad de Cordoba, Cordoba, Spain
- 2Scientific Center for Molecular Design and Synthesis of Innovative Compounds for the Medical Industry, Peoples Friendship University of Russia (RUDN), Moscow, Russia
A novel, environmentally friendly, and sustainable ultrasound-assisted methodology in the valorization of valeric acid to alkyl valerate using a biosilicified lipase from Candida antarctica is reported. This one-pot room temperature methodology of enzyme biosilicification leads to biosilicified lipases with improved activity and reaction efficiency as compared to free enzymes. Yields in the ultrasound-promoted esterification of valeric acid was ca. 90% in 2 h with 15% m/v of biosilicified lipase (Bio-lipase; 616 U/g biocatalyst enzymatic activity) and a molar ratio 1:2 (valeric acid:ethanol), slightly superior to that observed by the free enzyme (75% conversion, 583U/g biocatalyst enzymatic activity). The reuse of enzymes in these conditions was tested and the results show a relatively good reusability of these biosilicified enzymes under the investigated conditions, particularly preserving fairly stable specific activities (616 vs. 430 U/g biocatalyst after four reuses).
Introduction
The valorization of waste generated in various industrial activities worldwide becomes fundamental for the development of a sustainable planet and a more sustainable society. Esters of carboxylic acids are generally recognized natural flavors and fragrances with numerous applications (Dhake et al., 2013). Ethyl valerate, with a typical green apple fragrance, arouses great interest since it is widely used in the food, cosmetic, and pharmaceutical industries (Karra Chaabouni et al., 2006; Padilha et al., 2013). Importantly, alkyl valerates have also been recently demonstrated to have excelling properties to be employed in biofuel blends (Hari Krishna et al., 2001). These components, commonly extracted from natural resources, are however very limited in nature (Hari Krishna et al., 2001; Ben Akacha, 2014). Additionally, the chemical synthesis of esters generally employ not particularly green methods (harsh reaction conditions, use of mineral acids, etc.), with most systems exhibiting low selectivity (Ben Akacha, 2014; Brault et al., 2014). Lipases (triacylglycerol ester hydrolases—EC 3.1.1) are a promising alternative in esterification reactions, particularly for valeric acid, under moderate conditions (Adlercreutz, 2013; Dhake et al., 2013; Ben Akacha, 2014). Furthermore, the production of these esters via enzymatic route have been considered as more environmentally friendly (Brault et al., 2014; Corradini et al., 2017).
Biocatalytic processes, mediated by enzymes, have attracted keen interest in the valorization of biomass (Himmel et al., 2007). They represent a more efficient and ecological alternative to the traditional inorganic catalysts due to the fact that they take place under environmentally friendly conditions (room temperature, neutral pH), with often greater yields because of their regioselectivity and stereoselectivity (Patel et al., 2017). On the other hand, the use of these biocatalysts is not well established in industry because they have two main disadvantages: their limited stability, not compatible with continuous production (Itabaiana et al., 2013); and the difficulty of separating the substrates and products in the reaction medium, which prevents their reuse (Corradini et al., 2017). The immobilization of enzymes was proposed to partially overcome these problems, and allowed to date a number of biocatalytic industrial processes to be economically profitable (Güven et al., 2002).
A proposed enzymatic immobilization implemented by our group is the use of silica (SiO2) as encapsulating material, the proposed process denoted biosilicification. Enzyme biosilicification is based on a simple sol-gel synthetic procedure in which a SiO2 precursor (e.g., tetraethylorthosilicate) is added dropwise to a buffer solution of the commercial enzyme to generate a partially encapsulated enzyme in a SiO2 material upon hydrolysis of the SiO2 precursor (Itabaiana et al., 2013). The biosilicification was proved to give the enzyme a greater stability (at room temperature and neutral pH) in organic and aqueous media and to increase the catalytic efficiency at the same time. This is due to an observed partial encapsulation of the enzyme by the porous SiO2 that provides a mechanical/thermal stability to the enzyme. Additionally, it favors recovery and reuse, in comparison to the free enzyme, reducing the final costs of production (Pistone et al., 2016).
One of the novel methodologies that attract significant attention in biocatalyzed esterification reactions is the use of ultrasonic systems, alternative greener processes with high yields, and a greater selectivity as compared to other methodologies (Adak and Banerjee, 2016; Gupta S. et al., 2017; Gupta S. M. et al., 2017; Javad Safaei Ghomi, 2018). By comparison with conventional heating in carousel reactors, where mass transfer in esterification reactions is very limited, acoustic cavitation, and ultrasound hydrodynamics can solve mass transfer limitations at short times of reaction (Singh et al., 2007; Mahamuni and Adewuyi, 2009; Thanh le et al., 2010; Batistella et al., 2012; Veljković et al., 2012; Bhangu et al., 2017). This methodology also increases the yield in the reaction mediated by biocatalyst under the pressure and temperature conditions required for lipases (Suslick, 1990; Eddingsaas and Suslick, 2006). In summary, this methodology shows low reaction times, does not require the use of toxic solvents (Xu et al., 2013), being low energy intensive and cost-competitive.
Following previous efforts from the group in the design of biosilicified enzymes (Itabaiana et al., 2013; Henriquez et al., 2014; Pistone et al., 2016) the objective of this study was to evaluate the performance and stability of biosilicified lipases in the esterification of valeric acid under ultrasonic conditions. Parameters including type of alcohol, acid: alcohol ratio and temperature were investigated. The immobilized lipase was characterized using different BET, TGA, and XPS techniques and subsequently tested for stability and reuses under the investigated ultrasound-assisted conditions.
Results
Esterification Activity
Esterification experiments (Scheme 1) were conducted at room temperature via ultrasounds at different acid: alcohol molar relations and diverse concentrations of biocatalyst.
Table 1 summarizes the main set of experiments under optimized reaction conditions. Blank runs (in the absence of biocatalyst) or in the presence of a SiO2 material (without enzyme synthesized using an identical methodology in the absence of enzyme buffer solution) did not provide any valeric acid conversion under the investigated ultrasonic-promoted conditions despite the use of different alcohols and acid:alcohol ratios (Table 1, entries 1–8).
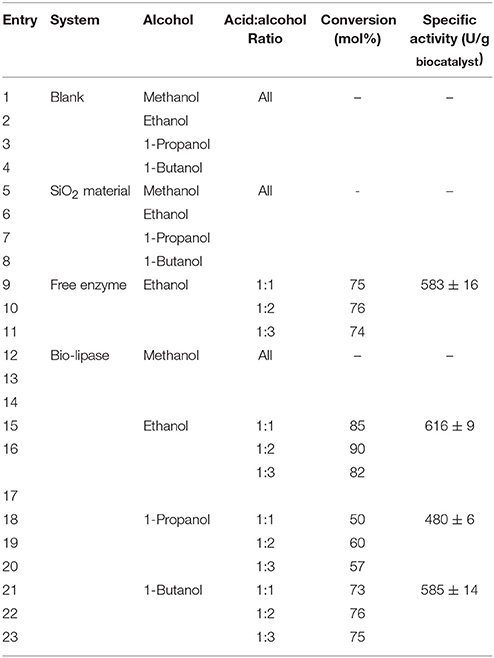
Table 1. Ultrasound-assisted esterification of valeric acid with various alcohols (MeOH, EtOH, iPrOH, BuOH) and different acid: alcohol ratios.
Reaction conditions: 0.01 mol valeric acid (1.09 mL), 0.01–0.03 mol EtOH (MeOH, i-PrOH, BuOH), 30 mg Bio-lipase (15% m/v, equal quantity for free enzyme in buffer solution—corresponding to 83.3 μL—), 2 h ultrasonic irradiation. Specific activities calculated for 1:2 acid:alcohol ratio (selected optimum conditions).
The enzymatic activity measured in all experiments (Table 1) provided comparable specific activities for the free enzyme (even slightly reduced, Table 1, entry 16) to Bio-lipase. Taking into consideration the quantity of free enzyme in the systems, the calculated percentage of protein loading in biosilicified lipase was 86%. These results are in good agreement with previous reports which related this comparable/improved activities at slightly reduced protein loadings to the stability of the encapsulated/immobilized lipase (Itabaiana et al., 2013; Pistone et al., 2016). Comparably, the highest conversion (90% conversion, 100% selectivity to ethyl valerate) was obtained using a 15% m/v of biosilicified enzyme and a 1:2 acid: alcohol molar ratio after 2 h (Table 2, entry 16).
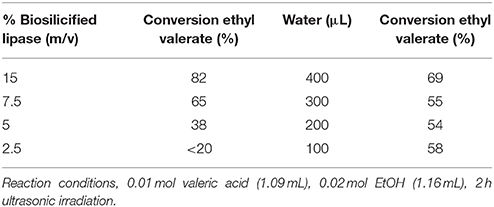
Table 2. Effect of the quantity of biosilicified lipase and water content in the conversion of valeric acid to ethyl valerate.
Additional investigations on different acid:alcohol ratios (Table 1, entries 15–17, 18–20, and 21–23) indicated that the acid-to-alcohol ratio did not have a significant influence in the specific activities and/or in the conversion in the systems under the investigated reaction conditions. In any case, a 1:2 acid:alcohol ratio was selected as optimum in all experiments. Interestingly, the use of other alcohols (e.g., 1-Propanol and 1-Butanol) could provide conversions to alkyl valerate between 50 and 70% (Table 1, entries 18–23), with minor differences also between more acid/alcohol concentrated solutions.
Conversions in the 70–82% range at complete selectivity could be also obtained keeping the quantity of enzyme to a maximum of 15% m/v and/or in aqueous solution (400 μL water added, Table 2). The presence of water in the reaction has been reported to favor enzyme-substrate interaction in biocatalyzed reactions (Bradford, 1976; Garcia-Galan et al., 2014), which in this case was not significantly influenced for biosilicified lipases under the investigated reaction conditions (Table 2, water results). A decrease in catalyst loading was found to decrease, as expected, ethyl valerate yields and specific activities of Bio-lipase and the free enzyme in the investigated reactions (Table 2, %biosilicified lipase results).
A probe was employed in the ultrasound system for temperature control and to determine the increase in temperature in the medium during the first hour of reaction. After this time period, cool water replaced the original solution to start a new reaction time. Over the hour reaction period, the temperature increases by ca. 20°C (max. 40–45°C), with such observed temperature increase being reproducible and consistent per hour for several cycles.
The stability and reusability of the enzyme under the investigated ultrasonic irradiation conditions was subsequently tested (Table 3). Results pointed out that the biosilicified lipase gradually deactivates with reuses in ethanol (from 90 to 60% after four uses) but importantly Bio-lipase was not fully denaturalised after several cycles under the investigated conditions. Reused biosilicified enzymes, as expected, provided after equal ultrasound treatment reduced specific activities (300-400 U/mg biocat., reuses R2-R4) as well as lower protein loading ratios (70-53% protein content in reused R2, R3, and R4 biosilicified lipase vs. 86% protein present in the fresh Bio-lipase) related to the loss of protein with reuses which seems to account for deactivation. Indeed, additional experiments on the potential activity/conversion of leached enzyme (reduced protein content after reuses, Table 3) indicate that such enzyme loss after reuses has exclusively residual activity (21–15% conversion, almost negligible specific activity) indicating (1) enzyme loss after reuses does not account for any relevant activity in the systems; (2) while it does certainly account for the observed deactivation after each cycle (Table 3, conversion values).
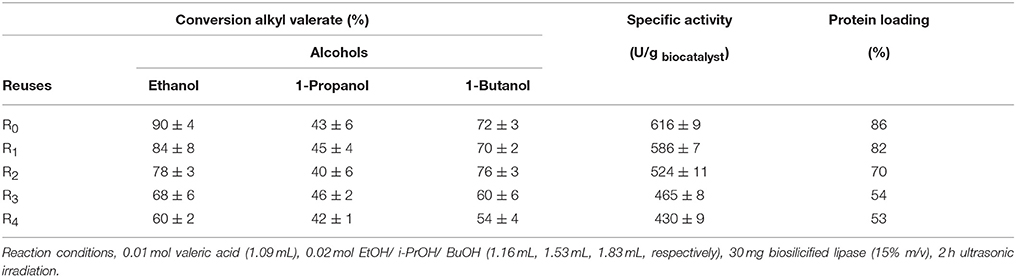
Table 3. Reusability studies of biosilicified lipase in the esterification reactions using ultrasound.
Interestingly, Bio-lipase was found to be increasingly stable in 1-Propanol and 1-Butanol. Selectivity to alkyl valerate was fully preserved after reuses in each case. Comparably, the free enzyme provided a 72% conversion at complete selectivity in the first run, followed by a significant reduction in specific activity after 2 reuses (<20% conversion, <150 U/mg biocat., run R2), most probably due to denaturalisation under ultrasonic conditions.
Characterization of the Biosilicified Enzyme Materials
The characterization of SiO2 material (Bio-lipase, same protocol in the absence of the enzyme buffer solution) showed a very similar surface area, exhibiting characteristics of non-porous materials prior to template removal [pores blocked with n-dodecylamine template, see also Figure 1 for Thermogravimetric (TG) analysis results]. Upon template removal (soxhlet extraction in ethanol under reflux for 8 h), the SiO2 material exhibited large surface areas (ca. 500 m2 g−1), with a pore diameter of 3.6 nm, typical of organo-templated mesoporous SiO2 materials (Macquarrie et al., 2005).
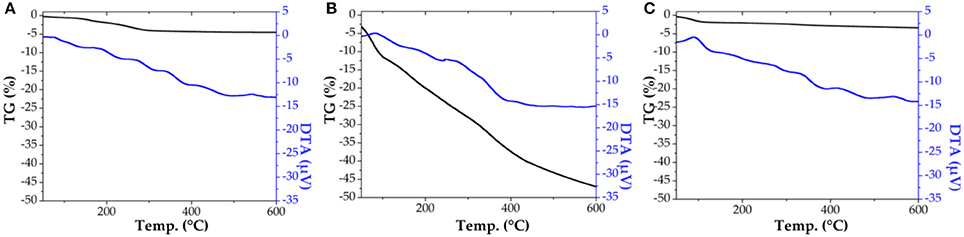
Figure 1. Thermogravimetric (TG) analysis for SiO2 support (A), biosilicified lipase R0 (B), and biosilicified lipase R3 (C).
Comparably, the textural properties of the fresh as-synthesized biosilicified enzyme (R0) show BET surface values below 5 m2/g−1, with negligible pore volumes (Table 4). These results indicate that the biosilicifed enzyme is essentially non-porous. However, these new biological catalysts do not have to meet the typical surface-specific standards normally common in inorganic solid catalysts. These materials may not have a direct relationship between catalytic surface/biocatalyst activity as illustrated in the biocatalytic activity results. Interestingly, thermogravimetrically treated Bio-lipase materials exhibited an interesting behavior worth discussing. The total loss of organics from biosilicified lipase R0 after TG (Figure 1, middle graph, 47.5% weight loss in the 110–600°C range) rendered a nicely porous material that still contains certain quantities of lipase (ca. 35–45% protein loading) and remains active in the investigated esterification reactions. Comparably, the third reused Bio-lipase (R3) material after thermogravimetric treatment (heating up to 600°C) exhibited a largely similar surface area (443 m2/g−1) as well as textural properties (pore volume and diameter) for which a minimum mass loss was observed in the 100–600°C range (ca. 4% from its original weight), accounting for ca. 45–50% protein loading left in the material. Taking into account the measurements errors and observed similarities, these relevant results further support the high stability of the enzyme in the biosilicified form as compared to the free enzyme, particularly under the investigated ultrasonic irradiation conditions.
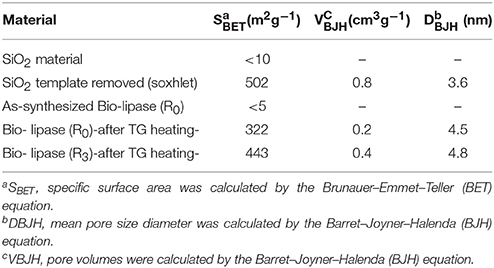
Table 4. BET surface area and pore volumes of biosilicified enzymes (as-synthesized and after TG treatment) as compared to analogously prepared silica materials (without enzyme).
The broad and intense XPS bands of N and C1s demonstrate the presence of C and Ni on the surface of the biosilicified material (Figure 2). These results may indicate a potential availability of the enzyme on the surface of the biosilicified material in spite of the biosilification process which rendered highly active encapsulated enzymes.
Conclusions
A simple and efficient methodology is reported for the highly selective esterification of valeric acid to alkyl valerate using synthesized encapsulated lipases (via biosilicification) under ultrasonic irradiation. Biosilicified enzymes exhibited slightly improved specific activities and product yields in the systems as compared to the free enzyme in ethanol (90% conversion and 616 U/g biocatalyst vs. 75% conversion and 583 U/g biocatalyst at complete ethyl valerate selectivity after 2 h reaction under ultrasounds). Importantly, biosilicified lipase materials were active in the esterification using longer chain alcohols (e.g., 1-Propanol, 1-Butanol) also exhibiting a moderate to good reusability after several uses, with a stable specific activity despite the significant protein loss (616 vs. 430 U/g biocatalyst with a reduction in protein content from 86 to 53% after four reuses). The observed protein loss proved to be responsible for the slight deactivation observed after reuses but clearly had a minor contribution in the activity of the systems. Comparatively, biosilicified lipases were remarkably more stable than the free enzyme that fully deactivates after two reaction runs.
Materials and Methods
Materials
All reagents were purchased in Sigma Aldrich and were used without further purification. The enzyme lipase B of the species Candida antartica (CalB) was purchased from Sigma Aldrich as a buffer solution.
Enzyme Biosilicification
According to the protocol of Henriquez et al. (2014), Tetraethoxysilane (TEOS) (20.80 g, 0.10 mol) is added to a stirred solution composed of n-dodecylamine (5.10 g, 0.03 mol), 50 g acetonitrile, and 50 g buffer solution of Cal-B at room temperature. The solution containing a visible solid precipitate after a few minutes and was stirred for another 3 h, then filtered, washed with ethanol, and dried at room temperature for at least 24 h. The SiO2 material was synthesized using the same protocol without the addition of the buffer solution of Cal-B.
Protein Concentration and Enzymatic Activity
Protein concentration was defined by Bradford method (Bradford, 1976), using as standard protein bovine serum albumin. All solutions were prepared by employing ultrapure water.
Enzymatic activity was determined by hydrolysis of p-nitrophenyl butyrate (pNPB), in acetonitrile, pH 7 and 25°C according to the protocol described by Garcia-Galan et al. (2014). P-nitrophenol, released to the reaction medium as hydrolysis product of pNPB, was measured at 400 nm. In the present work, a unit (U) is the amount of enzyme capable of hydrolyzing 1 μmol of substrate per minute at pH 7 and 25°C.
Immobilization Efficiency
Immobilization efficiency was evaluated with two parameters: Protein loading ratio and activity yield. Protein loading ratio (%) was used to compare the amount of enzyme that finally remains into the biocatalyst with respect to the free enzyme (for which a value of 100% is given). Activity yield (%) equally determined the difference between specific activity in case of biosilicified enzyme and free form.
Materials Characterization
The immobilized enzymes were characterized using N2 physisorption and powder X-ray diffraction (XRD). N2 adsorption measurements were performed at 77 K by using a Micromeritics ASAP 2000 volumetric adsorption analyzer. The samples were degassed at 35°C for 24 under vacuum (p < 10¢2 Pa) before the adsorption measurements. Surface areas were calculated according to the BET (Brunauer–Emmet–Teller) equation. Mean pore size diameter and pore volumes were measured from porosimetry data by using the BJH (Barret–Joyner–Halenda) method.
TGA measurements were performed using a System Setaram Setsys 12 TGA instrument. Samples were heated at a heating rate of 10°C min−1, in Nitrogen (50 mL min−1) at the temperature range 30–800°C.
XPS (aka ESCA) measurements were performed in a ultra-high vacuum (UHV) multipurpose surface analysis system (SpecsTM model, Germany) operating at pressures <10−10 mbar using a conventional X-Ray source (XR-50, Specs, Mg-Kα, 1253.6 eV) in a “stop-and-go” mode to reduce potential damage due to sample irradiation. The survey and detailed Fe and Cu high-resolution spectra (pass energy 25 and 10 eV, step size 1 and 0.1 eV, respectively) were recorded at room temperature with a Phoibos 150-MCD energy analyzer. Powdered samples were deposited on a sample holder using double- sided adhesive tape and subsequently evacuated under vacuum (<10−6 Torr) overnight. Eventually, the sample holder containing the degassed sample was transferred to the analysis chamber for XPS studies. Binding energies were referenced to the C1s line at 284.6 eV from adventitious carbon. The curve deconvolution of the obtained XPS spectra were obtained using the Casa XPS program.
Esterification Activity Assay
Valeric acid and ethanol were used as reaction substrates. In a typical esterification reaction, 30 mg of biosilicified lipase (15% Lipase m/v) was weighted and added to a solution of the alcohol (0.58, 1.16, 1.75 mL EtOH; 0.76, 1.53, 2.29 mL i-PrOH; 0.92, 1.83, 2.75 mL BuOH; corresponding to 1:1, 1:2, and 1:3 molar ratios respective to valeric acid), followed by the addition of 0.01 mol valeric acid (1.09 mL). The mixture was submerged in an ultrasonic bath (Ultrasound Midi II, P-seddlecta) and ultrasonicated for 2 h, with continuous temperature measurement. Reaction runs using the free enzyme were performed under identical conditions using comparable enzyme quantities (15% Lipase m/v), corresponding to 83.3 μL of lipase buffer solution (CAL-B, Sigma-Aldrich. Products were analyzed at different time intervals by GC in an Agilent 7890 GC model fitted with a capillary column Petrocol 100 m × 0.25 nm × 0.5 μm and a flame ionization detector (FID). The GC program was as follows: oven from RT to 220°C (40 min), inlet 250°C (split mode), Detector 300°C, N2 carrier flow: 30 mL min−1.
Author Contributions
SC-G conducted all experiments in the lab and wrote the first draft of the manuscript. AB and RL planned and programmed all experiments and wrote the final manuscript including the discussion.
Conflict of Interest Statement
The authors declare that the research was conducted in the absence of any commercial or financial relationships that could be construed as a potential conflict of interest.
Acknowledgments
RL gratefully acknowledges funding from MINECO under project CTQ2016-78289-P, co-financed with FEDER funds. SC-G is grateful to Junta de Andalucía for a contract European Social Fund-Program Youth Employment-PTA. The publication has been prepared with support of RUDN University Program 5-100.
References
Adak, S., and Banerjee, R. (2016). A green approach for starch modification: esterification by lipase and novel imidazolium surfactant. Carbohydr. Polym. 150, 359–368. doi: 10.1016/j.carbpol.2016.05.038
Adlercreutz, P. (2013). Immobilisation and application of lipases in organic media. Chem. Soc. Rev. 42, 6406–6436. doi: 10.1039/c3cs35446f
Batistella, L., Lerin, L. A., Brugnerotto, P., Danielli, A. J., Trentin, C. M., Popiolski, A., et al. (2012). Ultrasound-assisted lipase-catalyzed transesterification of soybean oil in organic solvent system. Ultrason. Sonochem. 19, 452–458. doi: 10.1016/j.ultsonch.2011.11.018
Ben Akacha, N. (2014). Microbial and enzymatic technologies used for the production of natural aroma compounds: synthesis, recovery modeling, and bioprocesses. Food Bioproducts Process. 94, 675–706. doi: 10.1016/j.fbp.2014.09.011
Bhangu, S. K., Gupta, S., and Ashokkumar, M. (2017). Ultrasonic enhancement of lipase-catalysed transesterification for biodiesel synthesis. Ultrason. Sonochem. 34, 305–309. doi: 10.1016/j.ultsonch.2016.06.005
Bradford, M. M. (1976). A rapid and sensitive method for the quantitation of microgram quantities of protein utilizing the principle of protein-dye binding. Anal. Biochem. 72, 248–254. doi: 10.1016/0003-2697(76)90527-3
Brault, G., Shareck, F., Hurtubise, Y., Lépine, F., and Doucet, N. (2014). Short-chain flavor ester synthesis in organic media by an E. coli whole-cell biocatalyst expressing a newly characterized heterologous lipase. PLoS ONE 9:e91872. doi: 10.1371/journal.pone.0091872
Corradini, M. C., Costa, B. M., Bressani, A. P., Garcia, K. C., Pereira, E. B., and Mendes, A. A. (2017). Improvement of the enzymatic synthesis of ethyl valerate by esterification reaction in a solvent system. Prep. Biochem. Biotechnol. 47, 100–109. doi: 10.1080/10826068.2016.1181084
Dhake, K. D., Thakare, D. M., and Bhanage, B. (2013). Lipase: a potential biocatalyst for the synthesis of valuable flavour and fragrance ester compounds Flavour Fragr. J. 28, 71–83. doi: 10.1002/ffj.3140
Eddingsaas, N. C., and Suslick, K. S. (2006). Mechanoluminescence: light from sonication of crystal slurries. Nature 444:163. doi: 10.1038/444163a
Garcia-Galan, C., Barbosa, O., Hernandez, K., dos Santos, J. C., Rodrigues, R. C., and Fernandez-Lafuente, R. (2014). Evaluation of styrene-divinylbenzene beads as a support to immobilize lipases. Molecules 19, 7629–7645. doi: 10.3390/molecules19067629
Gupta, S. M., Kamble, M. P., and Yadav, G. D. (2017). Insight into microwave assisted enzyme catalysis in process intensification of reaction and selectivity: kinetic resolution of (R,S)-flurbiprofen with alcohols. Mol. Catal. 440, 50–56. doi: 10.1016/j.mcat.2017.06.020
Gupta, S., Scott, D., Prabha, C. R., and Ashokkumar, M. (2017). Biodiesel synthesis assisted by ultrasonication using engineered thermo-stable Proteus vulgaris lipase. Fuel 208, 430–438. doi: 10.1016/j.fuel.2017.07.041
Güven, Ç., Afife, K., and Nurcan Mehmetoğlu, Ü. (2002). The production of isoamyl acetate using immobilized lipases in a solvent-free system. Process Biochem. 38, 379–386. doi: 10.1016/S0032-9592(02)00099-7
Hari Krishna, S., Divakar, S., Prapulla, S. G., and Karanth, N. G. (2001). Enzymatic synthesis of isoamyl acetate using immobilized lipase from Rhizomucor miehei. J. Biotechnol. 87, 193–201. doi: 10.1016/S0168-1656(00)00432-6
Henriquez, C., Junior, I., de Souza, R., and Luque, R. (2014). Novel nanoparticle/enzyme biosilicified nanohybrids for advanced heterogeneously catalyzed protocols Catal. Sci. Tech. 5. doi: 10.1039/C4CY01313A
Himmel, M. E., Ding, S.-Y., Johnson, D. K., Adney, W. S., Nimlos, M. R., Brady, J. W., et al. (2007). Biomass recalcitrance: engineering plants and enzymes for biofuels production. Science 315, 804–807. doi: 10.1126/science.1137016
Itabaiana, I., Sutili, F. K., Leite, S. G. F., Goncalves, K. M., Cordeiro, Y., Leal, I. C. R., et al. (2013). Continuous flow valorization of fatty acid waste using silica-immobilized lipases. Green Chem. 15, 518–524. doi: 10.1039/c2gc36674f
Javad Safaei Ghomi, Z. A. (2018). Ultrasonic accelerated Knoevenagel condensation by magnetically recoverable MgFe2O4 nanocatalyst: a rapid and green synthesis of coumarins under solvent-free conditions. Ultrason. Sonochem. 40, 78–83. doi: 10.1016/j.ultsonch.2017.06.022
Karra Chaabouni, M., Ghamgui, H., Sofiane, B., Rekik, A., and Gargouri, Y. (2006). Production of flavour esters by immobilized Staphylococcus simulans lipase in a solvent-free system. Process Biochem. 41, 1692–1698. doi: 10.1016/j.procbio.2006.02.022
Macquarrie, D. J., Gilbert, B. C., Gilbey, L. J., Caragheorgheopol, A., Savonea, F., Jackson, D. B., et al. (2005). Structured mesoporous organosilicas from an acetonitrile-water template system. J. Mater. Chem. 15, 3946–3951, doi: 10.1039/B505695K
Mahamuni, N. N., and Adewuyi, Y. G. (2009). Optimization of the synthesis of biodiesel via ultrasound-enhanced base-catalyzed transesterification of soybean oil using a multifrequency ultrasonic reactor. Energy Fuels 23, 2757–2766. doi: 10.1021/ef900047j
Padilha, G. S., de Barros, M. M., Alegre, R., and Tambourgi, E. (2013). Production of ethyl valerate from Burkholderia cepacia lipase immobilized in alginate. Chem. Eng. Trans. 32, 1063–1068. doi: 10.3303/CET1332178
Patel, V., Deshpande, M., and Madamwar, D. (2017). Increasing esterification efficiency by double immobilization of lipase-ZnO bioconjugate into sodium bis (2-ethylhexyl) sulfosuccinate (AOT)- reverse micelles and microemulsion based organogels. Biocatal. Agric. Biotechnol. 10, 182–188. doi: 10.1016/j.bcab.2017.03.009
Pistone, L., Ottolina, G., De, S., Romero, A. A., Martins, L. O., and Luque, R. (2016). Encapsulated laccases for the room-temperature oxidation of aromatics: towards synthetic low-molecular-weight lignins. Chem. Sus. Chem. 9, 756–762. doi: 10.1002/cssc.201501427
Singh, A. K., Fernando, S. D., and Hernandez, R. (2007). Base-catalyzed fast transesterification of soybean oil using ultrasonication. Energy Fuels 21, 1161–1164. doi: 10.1021/ef060507g
Thanh le, T., Okitsu, K., Sadanaga, Y., Takenaka, N., Maeda, Y., and Bandow, H. (2010). A two-step continuous ultrasound assisted production of biodiesel fuel from waste cooking oils: a practical and economical approach to produce high quality biodiesel fuel. Bioresour. Technol. 101, 5394–5401. doi: 10.1016/j.biortech.2010.02.060
Veljković, V. B., Avramović, J. M., and Stamenković, O. S. (2012). Biodiesel production by ultrasound-assisted transesterification: state of the art and the perspectives. Renew. Sustain. Energy Rev. 16, 1193–1209. doi: 10.1016/j.rser.2011.11.022
Keywords: biosilicification, esterification, ultrasounds, biocatalysis, lipases
Citation: Cebrián-García S, Balu AM and Luque R (2018) Ultrasound-Assisted Esterification of Valeric Acid to Alkyl Valerates Promoted by Biosilicified Lipases. Front. Chem. 6:197. doi: 10.3389/fchem.2018.00197
Received: 02 February 2018; Accepted: 15 May 2018;
Published: 07 June 2018.
Edited by:
Florent Allais, AgroParisTech Institut des Sciences et Industries du Vivant et de L'environnement, FranceReviewed by:
Alexei Lapkin, University of Cambridge, United KingdomVictor Sans Sangorrin, University of Nottingham, United Kingdom
Copyright © 2018 Cebrián-García, Balu and Luque. This is an open-access article distributed under the terms of the Creative Commons Attribution License (CC BY). The use, distribution or reproduction in other forums is permitted, provided the original author(s) and the copyright owner are credited and that the original publication in this journal is cited, in accordance with accepted academic practice. No use, distribution or reproduction is permitted which does not comply with these terms.
*Correspondence: Rafael Luque, cmFmYWVsLmx1cXVlQHVjby5lcw==