- 1State Key Laboratory of Molecular Engineering of Polymers, Department of Chemistry and Laboratory of Advanced Materials, Collaborative Innovation Center of Chemistry for Energy Materials (2011-iChEM), Fudan University, Shanghai, China
- 2Queensland Micro- and Nanotechnology Centre, Griffith University, Brisbane, QLD, Australia
- 3Department of Orthopedics, Changhai Hospital & Department of Spine Surgery, Changzheng Hospital, Second Military Medical University, Shanghai, China
Mesoporous SiO2 nanoparticles (MSNs) are one of the most promising materials for bio-related applications due to advantages such as good biocompatibility, tunable mesopores, and large pore volume. However, unlike the inorganic nanocrystals with abundant physical properties, MSNs alone lack functional features. Thus, they are not sufficiently suitable for bio-applications that require special functions. Consequently, MSNs are often functionalized by incorporating inorganic nanocrystals, which provide a wide range of intriguing properties. This review focuses on inorganic nanocrystals functionalized MSNs, both their fabrication and bio-applications. Some of the most utilized methods for coating mesoporous silica (mSiO2) on nanoparticles were summarized. Magnetic, fluorescence and photothermal inorganic nanocrystals functionalized MSNs were taken as examples to demonstrate the bio-applications. Furthermore, asymmetry of MSNs and their effects on functions were also highlighted.
Introduction
In the past decades, the impressive advancement of nanotechnology has brought major progress in nano-related biological applications. Nano-size particles can serve as carriers for functional guest molecules, drugs, genes, etc. and effectively accumulate at infection site through the enhanced permeability and retention effect (EPR) (Matsumura and Maeda, 1986; Davis et al., 2008). They also serve as platforms for other in-vivo functions such as bio-sensing and imaging. Commonly reported nano-sized particles, liposomes, polymer, and dendrimers have emerged as fabulous platforms for in-vivo applications. Amongst those particles, mesoporous silica nanoparticles (MSNs) shall be one of the most promising materials for bio-related applications due to their good biocompatibility, tunable mesopores, large volume, simple and scalable fabrication process and easy functionalization (-NH2, -COOH, etc.). Large volume of works has been conducted by loading the mesopores of MSNs with drugs or other bioactive substances to avoid their degradation during blood circulation and raise delivery efficiency (Teng et al., 2015; Moller and Bein, 2017; Yang et al., 2017). Gatekeeper molecules were used to seal drugs within the pores, waiting for environmental changes at a specific in-vivo location, tumor for example, to achieve target and stimuli delivery (Fatieiev et al., 2015; Prasetyanto et al., 2016; Wu et al., 2016). Over the recent years, in-vivo distribution, toxicity, renal clearance, and other properties (He et al., 2011; Du et al., 2016; Chou et al., 2017) of MSNs have been studied thoroughly, making it one of the most understood and favored materials for bio-applications.
However, in contrast to inorganic nanocrystals with abundant optical, magnetic, electric, and other physical properties, MSNs possess no special features, serving merely as a slow release carrier for guest molecules, thus would be insufficient for performing bio-applications which require certain functions. Though loaded molecules can be used for functional applications, for example indocyanine green (ICG) (Li J. et al., 2013; You et al., 2017) and IR-820 (Zhao et al., 2017) for photothermal therapy (PTT), rose bengal (RB) (Lu et al., 2016) for photo dynamic therapy (PDT). Still, the rapid development of nanomedicine and other bio-applications, such as bio-sensing, drug delivery, bio-manufacturing/imaging, diagnosis and therapy, urgently demand for more functions such as magnetic, thermal, and fluorescence properties. Thus, MSNs are often functionalized by incorporating various inorganic nanocrystals, which provide a wide range of intriguing properties, but when used alone they would suffer from low biocompatibility and limited loading capacity. Upon the combination, either the original functions of inorganic nanocrystals are enhanced and more suited for in-vivo applications, or delicate design and employment of mesoporous silica (mSiO2) may bring brand new possibilities in functions. Numerous works have been done in this field, and many outstanding results have been achieved. The most commonly employed methods for incorporation would be the formation of core-shell structure, coating a layer of mSiO2 shell on inorganic nanocrystals. This review focuses on MSNs functionalized with inorganic nanocrystals, both their fabrication and bio-applications.
Core/Shell Structure Based Inorganic Nanocrystals Functionalized Mesoporous Silica Nanocomposites
Fabrication of mSiO2 can be traced back to 1992 (Kresge et al., 1992). Yet, although there have been large volume of works on the synthesis of ordered mSiO2 materials through EISA method (Zhao et al., 1998; Wan and Zhao, 2007), bulk materials are usually not suited for bio-applications. Fabrication of MSNs as well as coating of ordered mSiO2 onto single nanoparticles came later through sol-gel induced assemble of silane with surfactants, and many works have been done by various independent groups. For instance, Deng et al. fabricated core-shell structure with perpendicularly oriented mesoporous channels (Deng et al., 2008). After obtaining Fe3O4@dSiO2, a surfactant-templating approach using cetyltrimethylammonium bromide (CTAB) micelle as template was utilized for coating a uniform layer of CTAB/silica composite. mSiO2 shell with radial mesopores were acquired after extraction of CTAB. This core-shell structure with ordered mSiO2 shell have a uniform pore size of 2.3 nm, surface area of 365 m2/g and a pore volume of 0.29 cm3/g. These accessible uniform mesopores with high surface area and large pore volume are promising for many applications.
Although ordered mesoporous silica can be synthesized easily, it still suffers from the relatively small pore diameter of 2–3 nm, restricting loading guests to small molecules only, limiting its usages. Most recently, Zhao's Group proposed the bi-phase method, using silane and surfactants assembled at the water/oil interface to achieve expanded mesopores (Figures 1A,B; Yue et al., 2015). By changing different oil-phase (cyclohexane, octadecene, etc.) and the precise control of stirring speed, the growth of 3D-dendritic mSiO2 with pores as large as 15–20 nm can be achieved, greatly expanding the species and amount of potential loaded guest molecules. Such large-pore mesoporous silica coating may be used for loading of larger molecules, RNA, proteins, etc. (Shen et al., 2014; Xu et al., 2015; Yang et al., 2015; Meka et al., 2016; Zhang et al., 2016).
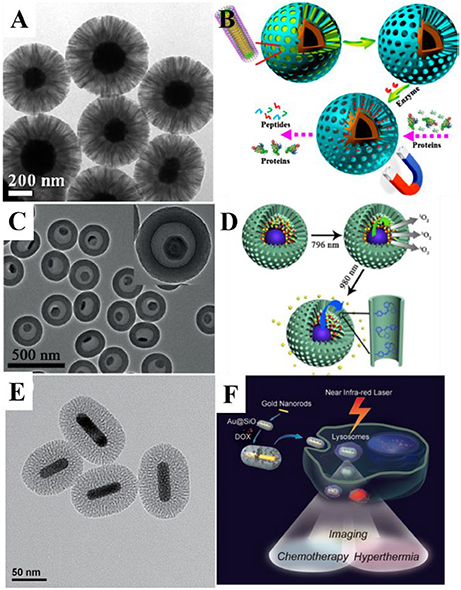
Figure 1. (A) Transmission electron microscope (TEM) image of Fe3O4@RF@mSiO2 nanoparticles and (B) application scheme of the nanocomposite for protein digestion. Reprinted with permission from Yue et al. (2015). Copyright (2015) American Chemical Society. (C) TEM image of the UCNPs@mSiO2 yolk-shell nanocomposites and (D) the scheme for combined photodynamic therapy and chemotherapy using 796 and 980-nm laser, respectively. Reprinted with permission from Li et al. (2016). Copyright 2016 Wiley-VCH Verlag GmbH & Co. KGaA, Weinheim. (E) TEM image of Au@mSiO2 nanoparticles and (F) schematic illustration of mSiO2-coated gold nanorods as a novel multifunctional theranostic platform for cancer treatment. Reprinted with permission from Zhang Z. J. et al. (2012). Copyright 2012 Wiley-VCH Verlag GmbH & Co. KGaA, Weinheim.
While water dispersible nanoparticles can be dispersed in water and ethanol, going through direct coating process, the growth of mSiO2 on oil-dispersed nanoparticles would be rather complicated. Nanoparticles fabricated through high-temperature solvent-thermal method often are capped with oleic acid (OA), oleyl amine, etc., which can only be dispersed in organic solvent such as cyclohexane, hexane, chloroform, etc. The previously mentioned method of coating mSiO2 can be adopted on OA-coordinated nanoparticles after their transfer to aqueous phase through reverse-emulsion method (Garcia et al., 2012; Jayakumar et al., 2012), a typical means for the coating of dense SiO2 on hydrophobic nanoparticles (Hu et al., 2009; Das et al., 2010; Jing et al., 2010). However, in order to form a stabilized emulsion, the amount of water in reverse-emulsion method is often limited to a very low level, resulting in rather low productivity, thus the method is not suitable for mass production. Another easier route is the direct coating of mSiO2, in which CTAB serves both as template for mesopore as well as stabilizer for water disperse. For example, Kim et al. used this method to synthesis core-shell structure with uniform morphologies such as Fe3O4@mSiO2, MnO@mSiO2 etc. (Kim et al., 2008).
By coating a layer of mSiO2 onto inorganic nanocrystals, their biocompatibility is significantly improved. In addition, the mesopores of mSiO2 can also provide a large surface area for loading vast amount of guest molecules, realizing functions such as diagnosis and therapy. Based on the above mentioned core/shell structure engineering, many kinds of inorganic nanoparticle@mSiO2 nanocomposites with different functions were fabricated and applied in bio-related applications.
Magnetic Nanoparticles Functionalized Mesoporous Silica Nanocomposites
Magnetic nanoparticles such as the popular Fe3O4 nanoparticles also include other forms of ferrites and particles doped with Gd3+, Mn2+, etc., possess potential biomedical applications in magnetic resonance imaging (MRI) (Kim et al., 2011; Hu and Zhao, 2012; Lee et al., 2014), and magnetic targeted drug delivery and separation (Giri et al., 2005; Gao et al., 2009; Sahoo et al., 2014; Wang and Gu, 2015). However, pure Fe3O4 (or other iron oxide) nanoparticles have a strong tendency of aggregating and are instable over long periods of internal cycles, limiting their in-vivo biological usages (Liu et al., 2011b). Thus, works on magnetic nanoparticles are often focused on their cooperation with mSiO2.
The cooperation of magnetic nanoparticles and mSiO2 results in multifunctional nanocomposites with both magnetic for MRI and mesopores for drug loading and delivery. Kim et al. achieved discrete encapsulation of one Fe3O4 nanoparticle in one MSN (Kim et al., 2008), the Fe3O4@mSiO2 core-shell nanoparticles with a diameter of 40–90 nm proved to be desirable T2 MR contrast agents. Zhao et al. synthesized rattle-type hollow magnetic mesoporous spheres with a cavity created through post hydrothermal treatment (Zhao et al., 2008), and set the nanocomposite as a drug loading and delivery model. As drug delivery nanocarriers, loading capacity is an important parameter, thus the pursue for large drug loading spaces becomes a quest. Although large pore volume may increase drug loading capacity, there's yet problems such as leakages, researchers seek to fabricated yolk-shell structure with interior vacancy. Chen et al. fabricated ellipsoidal Fe3O4@mSiO2 yolk-shell structure with a large cavity between the functional magnetic core and mesoporous shell (Chen et al., 2010b). In their work, SiO2 and mSiO2 were successively coated on the magnetic core, then the dense SiO2 layer was selectively etched, resulting in the formation of yolk-shell structure. The yolk-shell structured nanocomposite showed an excellent biocompatibility toward all kinds of cells. While the magnetic core served as an in-vivo monitor during the therapy as T2 contrast agent, the vacancy created proved to be an ideal drug loader with high DOX loading capacity. Such selective-etching methodology for creating controllable vacancy has been adopted by many other groups, utilizing the structure on various kinds of inorganic nanocrystals and expanding to applications even beyond drug delivery (Chen et al., 2010a; Zhu et al., 2010; Liu et al., 2011a; Wang et al., 2011).
Apart from magnetic resonance bio-imaging, another advantage of magnetic nanoparticles is the external magnetic field enhanced target drug delivery, a targeting method unnecessary for modifying of targeting molecules such as antibody (Kapse-Mistry et al., 2014). Liu et al. performed magnetic field directed cellular uptake experiment of Fe3O4@mSiO2 nanoparticles and achieved doubled uptake efficiency on A549 cancer cells (Liu et al., 2012). Xiong et al. fabricated Fe3O4@mSiO2 with large 15-nm radial mesopores for the delivery of siRNA (Xiong et al., 2016), a further layer of pH responsive tannic acid was deposed onto the nanoparticle to protect siRNA from degradation. Such design enabled magnetically induced high delivery efficiency and pH responsive release of siRNA. The enhanced gene delivery through magnetic vectors is given the name “magnetofection.” Much research has been conducted in the field, and showed promising future in in-vivo applications (Scherer et al., 2002; Mykhaylyk et al., 2007; Plank et al., 2011).
Current applications of magnetic nanoparticles functionalized MSNs are mostly focused on bio-imaging and targeted delivery of drug. Other functions such as detection and isolation (Song et al., 2011), magnetic hyperthermia (Wang D. W. et al., 2013; Yang et al., 2014), etc. might prefer more attention. Beside superparamagnetic nanoparticles, which at times face problems such as spin-canting, low saturation magnetization, and slow magnetic response, magnetic nanoparticles with vortex domain would also be a promising choice for bio-applications (Fan et al., 2010; Kim et al., 2010).
Fluorescence Nanoparticles Functionalized Mesoporous Silica Nanocomposites
Fluorescence nanoparticles find their usages in almost every field of bio-applications, including diagnosis, luminescence guided therapy (Lim, 2016), light triggered drug delivery, photodynamic therapy (PDT), etc. Quantum dots (QDs) and lanthanide doped upconversion nanoparticles (UCNPs) are the two most frequently used inorganic fluorescence nanoparticles (Li et al., 2013a,b; Li et al., 2014a, 2015a, 2016) However, most fluorescence nanoparticles suffer from bad aqueous dispersity due to the oil-phase solvent-thermal fabrication process, thus naturally leads to the coating of SiO2 to prevent aggregation and to protect the inner core from quenching or harsh environment (Li et al., 2015a).
Many works focused on fluorescence bio-imaging have been done. Li et al. designed CdTe@mSiO2 nanoparticles and realized real-time intracellular luminescence imaging of tumor cells (Li J. et al., 2014). Zhang et al. used NaYF4;Yb;Er@mSiO2 for cell imaging and drug delivery (Zhang et al., 2013). Lanthanide elements such as Gd3+, due to the complex 4f electrons, may also serve as MRI agents. Thus the same group proposed NaYF4;Yb;Er@NaGdF4;Yb core-shell UCNP encapsulated in mSiO2 for fluorescence and MRI dual-model bio-imaging (Li C. et al., 2013). Comparing to fluorescence imaging only, dual-model bio-imaging provide more accurate information toward the target, avoiding problems such as misdiagnose. Beside UV-vis fluorescence, second near-infrared window fluorescent bio-imaging of lanthanide doped nanoparticles also attracted a lot of attention. Both the excitation and emission are in the biological window for such infrared radiation (NIR) excited second NIR window (NIR-II) down-conversion fluorescent bio-imaging nanoparticles, allowing them to avoid many in-vivo disturbance, promoting the bio-imaging ability of the materials. For example, Wang fabricated SiO2-Nd@SiO2@mSiO2 nanoparticles with down-conversion fluorescence that can withstand harsh gastrointestinal conditions, serving as a real-time drug release monitor (Wang et al., 2017).
Stimuli-responsivity is of great significance in drug delivery, light triggered systems offer stimuli control both in time and location, thus would naturally attract attention. While various works have been done on controlled drug delivery under UV light, the near NIR triggering ability of UCNPs tend to be more valuable, since NIR radiation have better penetration and less damage toward tissues. Liu et al. contributed to the foundational work in the field (Liu et al., 2013). The group prepared NaYF4:Tm,Yb@NaYF4 core–shell nanoparticles, which emit UV/blue emission under NIR, and subsequently coated the UCNPs with mSiO2. “Photomechanical” azobenzene groups (azo) were then installed into the mesopores to act as UV/blue responsive “stirrer” to send out loaded guest molecules in the mSiO2. Such delicate design allows almost precise control of the amount of released DOX by varying the intensity or time of NIR light irradiation. This basic concepts were adopted by many other works that followed (Luo et al., 2013; Li W. et al., 2014; Min et al., 2014; Song and Yang, 2015).
Besides chemotherapy, photodynamic therapy (PDT) is another route for light-triggered therapeutic processes. PDT involves activation of photosensitizer (PS) with light to produce single oxygen, killing the tumor cells (Cui et al., 2013; Wang C. et al., 2013). Qian et al. incorporated zinc (II) phthalocyanine (ZnPc) into NaYF4;Yb/Er@mSiO2, using the NIR excited Er3+ green emission to active the photosensitizer to release reactive singlet oxygen for tumor therapy (Qian et al., 2009).
Loading of multiple guests and multifunctional fluorescence inorganic nanocrystals raise the possibility of designing functional MSNs with multiple controllable diagnosis and therapy process, thus the previously mentioned light triggering functionalities could be gathered altogether in one single nanoparticle. Combined therapy and diagnostic, theranostic process, allows more precise treatment toward disease. In order to realize imaging-guided combined PDT and chemotherapy, Li et al. fabricated six-layered UCNPs with orthogonal excitations-emissions upconversion luminescence and further combined them with mSiO2 to form the yolk-shell structured UCNPs@mSiO2 nanocomposites (Figures 1C,D). In this nanocomposite, the 1,050-nm NIR down-conversion luminescence (DCL) under low power density 796-nm excitation is used as a bioimaging signal. UV/blue emission under 980-nm excitation could be used to trigger the release of the chemotherapy drug in the hollow through introduction of azo groups. Independently, the PDT can also be realized under the stimulus of green emission excited by 796 nm (Li et al., 2016). Such design allowed the control of multiple therapy process through different excitation signal.
Photothermal Nanoparticles Functionalized Mesoporous Silica Nanocomposites
Many inorganic materials such as Au, CuS, graphene oxide, etc. can absorb and convert NIR light into thermal energy, causing localized heating which may effectively kill tumor cells. The therapy process of light triggered thermal effect is called photothermal therapy (PTT). Beside directly killing tumor cells through heat generation, the thermal energy can be used as a switch for drug delivery, either by heat-induced molecular movements or combining with thermal sensitive gatekeeper polymers. Yet, similar to other inorganic nanocrystals, this class of nanoparticles also suffer from low drug-loading capacity and easy aggregation.
In order to make up the above mentioned deficiencies, researchers try to combine photothermal materials with the mSiO2. Yang et al. synthesized cubic Ag@mSiO2 yolk shell structure, and then achieved Au nanocage@mSiO2 through galvanic replacement. A layer of thermal responsive polymer poly(nisopropylacrylamide) (PNIPAM) was coated outside the MSNs as a gatekeeper. Upon NIR radiation, heat generated by Au nanocages eventually lead to the collapse of PNIPAM shell, releasing the loaded DOX inside, realizing controlled PTT and chemotherapy (Yang et al., 2013). Such design prevented the leakage of drugs during in-vivo blood cycles, greatly raising the delivery efficiency. Zhang et al. coated mSiO2 with Au nanorods to used them as multifunctional theranostic platforms to serve as all-in-one two-photon imaging (TPI) agents, hyperthermia agent and drug carrier (Figures 1E,F; Zhang Z. J. et al., 2012). Other materials beside Au also possess photothermal ability, Chen et al. used CuS@mSiO2 as theranostic nanomedicine and ablated mice's 4T1 tumor after laser excitation with none regrowth over 2 months (Chen et al., 2015).
Asymmetry Mesoporous Silica Nanocomposites
Most recently, combined therapy with dual-drugs of different therapeutic effects showed excellent performance in treatment of diseases, especially in drug-resistant cancer treatments (Zhang et al., 2010; Zhang F. et al., 2012; Fan et al., 2013; Liu et al., 2015). One of the main challenges in realizing the best therapeutic effects is that the species and doses of drugs should be optimized at different clinical manifestations and periods in the treatment. However, simple drug delivery systems cannot fulfill the requirements of this combined therapy, because the widely used carriers such as MSNs (Deng et al., 2008), hollow structured nanoparticles (Zhang et al., 2016), yolk-shell nanoparticles (Zhu et al., 2010), etc. normally possess symmetrical geometry with limited single storage space available for loading of multiple drug species. When dual-drugs are loaded in a single storage space, release of each drug cannot be controlled independently. Therefore, developing asymmetric multi-compartment carriers with independent storage spaces for loading multiple drugs is critically desired. Nanoparticles with asymmetric nanostructures on geometry, chemical composition, surface property, and functionality, etc. have aroused extensive concern for their unique characteristics and potential applications. Compared with the conventional symmetric hybrid materials, the structural asymmetry is ideally suited for multiple-component conjugations, loading, or designing of smart delivery system on single-particle level. The nanocomposites with geometrical asymmetry determines that the function of each component can work independently without interaction with each other. These characteristics of the asymmetric nanoparticles render them truly “multifunctional entities.”
Most recently, Li et al. first developed a novel anisotropic growth strategy for the fabrication of Janus structured dual-compartment multi-functional UCNP@SiO2@mSiO2&PMO (PMO = periodic mesoporous organosilica) nanocomposites. Start with the core@shell@shell structured UCNP@SiO2@mSiO2 nanospheres, the PMO single-crystal nanocubes can anisotropic island nucleation and growth on the side of the initial nanospheres to the unique Janus morphology (Figures 2A–C; Li et al., 2014b). The obtained nanocomposites are asymmetric not only in morphology, but also in pore size and hydrophobicity/hydrophilicity, which is much important for the multiple guests co-delivery. The distinct chemical properties of the silica sources and different mesostructures of the compartments are two key factors for the formation of the asymmetric nanocomposites. According to the specific designing of the switch at the entrance of the mesopores, the dual-compartment Janus mesoporous SiO2 nanocomposites are used in the dual-drugs co-loading and controllable releasing for high efficiency cancer cell killing. This synthetic strategy can be used in the fabrication of the asymmetry single-hole nanorattles for the co-delivery of dual guests with a great discrepancy in size (Figures 2D–F; Li et al., 2015b). Beside the anisotropic growth of cubic mesostructure, the same group also extended this strategy to the fabrication of 1D asymmetric di- and tri-block mesoporous nanocomposites with ordered hexagonal mesostructures (Figure 2G). Due to the 1D mesopore channels and the asymmetric in the function, the Au nanorod functionalized di-block Au-NR@SiO2&EPMO (EPMO = ethane bridged periodic mesoporous organosilica) nanorods exhibit superior controllable releasing of drug molecules triggered by near-infrared light (Li et al., 2017).
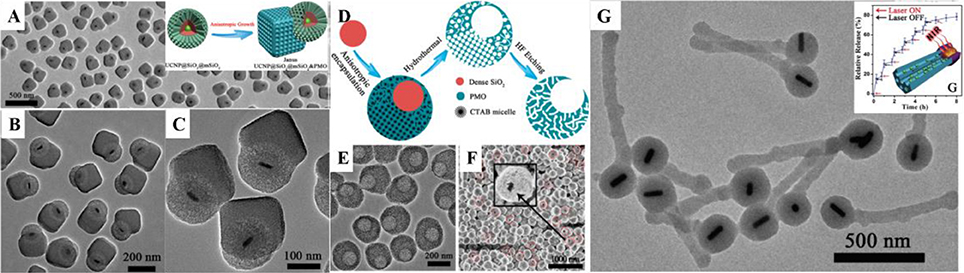
Figure 2. (A–C) TEM image of UCNP@SiO2@mSiO2&PMO Janus nanoparticles and the fabrication scheme (A inset). Reprinted with permission from Li et al. (2014b). Copyright (2014) American Chemical Society. (D) Scheme, (E) TEM, and (F) SEM images of asymmetry single-hole nanorattles. Reprinted with permission from Li et al. (2015b). Copyright (2015) American Chemical Society. (G) TEM image of match-like asymmetric Au-NR@SiO2&EPMO and the photothermal induced drug release (inset). Reprinted with permission from Li et al. (2017). Copyright 2017 Wiley-VCH Verlag GmbH & Co. KGaA, Weinheim.
Conclusion and Perspectives
Functional mesoporous nanoparticles with symmetric and asymmetric architectures possess both unique properties of ordered mesoporous materials and abundant optical, electrical, magnetic properties of inorganic nanomaterials, showing great potential applications on catalysis, adsorption, separation, especially in biology applications.
Despite achieving enormous delightful results, there is still much room for further development. First of all, although many reported works have done intracellular experiments of the fabricated functional MSNs, their in-vivo performances still need further assessment. After incorporation of inorganic nanocrystals, long-term toxicity and pharmacokinetics of the materials used for in-vivo applications require careful monitor, so does biodegradation and side-effects, to ensure bio-safety of MSNs. Future works on functional MSNs call for more thorough design with consideration for not only in-vitro but also in-vivo experiments as well.
Due to the complexity of biological systems, single-functioned nanoparticles often fail to fulfill their assignments, leading to problems such as the danger of misdiagnose and resistance to drugs. Thus, the design and fabrication of multifunctional MSNs would be of great importance for future research. In current fabrications of functional MSNs, mSiO2 mainly serve to improve biocompatibility and load drug molecules, so that the properties of inorganic nanocrystals and MSNs are often isolated. The synergy between the inorganic nanocrystals and mSiO2 should be considered not only in the synthesis but also in the applications.
In many recent works, researchers are not satisfied by only controlling the pore size and overall diameter of MSNs. They seek to precisely tune the configuration of the functional MSNs, surface roughness for example, to render the nanocomposites brand new properties. Such investigations may bring new development to this field.
Furthermore, it still remains a major challenge to prepare MSNs with controlled loading and release of multiple loaded guest molecules, which would be essential for complex theranostic process. Such quest may be achieved through designing asymmetric multi-compartment MSNs and multifunctional inorganic core. While nanocomposites with geometrical asymmetry ensure the function of each component to work independently, multifunctional inorganic core may provide each compartment with the needed stimuli for controlled theranostic process, lifting the properties of functional MSNs to a new level.
In conclusion, inorganic nanocrystals functionalized mSiO2 nanomaterials will continue to be a hotspot and research focus. Further investigations into the system would beyond doubt bring exciting achievements and lead to promising clinic and other bio-applications in the near future.
Author Contributions
All authors listed have made a substantial, direct and intellectual contribution to the work, and approved it for publication.
Conflict of Interest Statement
The authors declare that the research was conducted in the absence of any commercial or financial relationships that could be construed as a potential conflict of interest.
Acknowledgments
The work was supported by the NSFC (grant No. 21701027, 2133003), Shanghai Sailing Program (17YF1401000), Key Basic Research Program of Science and Technology Commission of Shanghai Municipality (17JC1400100), Australia Research Council (ARC) (DP160104089), and Griffith University Postdoctoral Fellowship projects.
References
Chen, F., Hong, H., Goel, S., Graves, S. A., Orbay, H., Ehlerding, E. B., et al. (2015). In vivo tumor vasculature targeting of CuS@MSN based theranostic nanomedicine. ACS Nano 9, 3926–3934. doi: 10.1021/nn507241v
Chen, Y., Chen, H., Guo, L., He, Q., Chen, F., Zhou, J., et al. (2010a). Hollow/rattle-type mesoporous nanostructures by a structural difference-based selective etching strategy. ACS Nano 4, 529–539. doi: 10.1021/nn901398j
Chen, Y., Chen, H., Zeng, D., Tian, Y., Chen, F., Feng, J., et al. (2010b). Core/shell structured hollow mesoporous nanocapsules: a potential platform for simultaneous cell imaging and anticancer drug delivery. ACS Nano 4, 6001–6013. doi: 10.1021/nn1015117
Chou, C. C., Chen, W., Hung, Y., and Mou, C. Y. (2017). Molecular Elucidation of biological response to mesoporous silica nanoparticles in vitro and in vivo. ACS Appl. Mater. Interfaces 9, 22235–22251. doi: 10.1021/acsami.7b05359
Cui, S., Yin, D., Chen, Y., Di, Y., Chen, H., Ma, Y., et al. (2013). In vivo targeted deep-tissue photodynamic therapy based on near-infrared light triggered upconversion nanoconstruct. ACS Nano 7, 676–688. doi: 10.1021/nn304872n
Das, G. K., Heng, B. C., Ng, S. C., White, T., Loo, J. S. C., D'Silva, L., et al. (2010). Gadolinium oxide ultranarrow nanorods as multimodal contrast agents for optical and magnetic resonance imaging. Langmuir 26, 8959–8965. doi: 10.1021/la904751q
Davis, M. E., Chen, Z. G., and Shin, D. M. (2008). Nanoparticle therapeutics: an emerging treatment modality for cancer. Nat. Rev. Drug Discov. 7, 771–782. doi: 10.1038/nrd2614
Deng, Y., Qi, D., Deng, C., Zhang, X., and Zhao, D. (2008). Superparamagnetic high-magnetization microspheres with an Fe3O4@SiO2 core and perpendicularly aligned mesoporous SiO2 shell for removal of microcystins. J. Am. Chem. Soc. 130, 28–29. doi: 10.1021/ja0777584
Du, X., Li, X. Y., Xiong, L., Zhang, X. J., Kleitz, F., and Qiao, S. Z. (2016). Mesoporous silica nanoparticles with organo-bridged silsesquioxane framework as innovative platforms for bioimaging and therapeutic agent delivery. Biomaterials 91, 90–127. doi: 10.1016/j.biomaterials.2016.03.019
Fan, H. M., Olivo, M., Shuter, B., Yi, J. B., Bhuvaneswari, R., Tan, H. R., et al. (2010). Quantum dot capped magnetite nanorings as high performance nanoprobe for multiphoton fluorescence and magnetic resonance imaging. J. Am. Chem. Soc. 132, 14803–14811. doi: 10.1021/ja103738t
Fan, W., Shen, B., Bu, W., Chen, F., Zhao, K., Zhang, S., et al. (2013). Rattle-structured multifunctional nanotheranostics for synergetic chemo-/radiotherapy and simultaneous magnetic/luminescent dual-mode imaging. J. Am. Chem. Soc. 135, 6494–6503. doi: 10.1021/ja312225b
Fatieiev, Y., Croissant, J. G., Alsaiari, S., Moosa, B. A., Anjum, D. H., and Khashab, N. M. (2015). Photoresponsive bridged silsesquioxane nanoparticles with tunable morphology for light-triggered plasmid DNA delivery. ACS Appl. Mater. Interfaces 7, 24993–24997. doi: 10.1021/acsami.5b07365
Gao, J., Gu, H., and Xu, B. (2009). Multifunctional magnetic nanoparticles: design, synthesis, and biomedical applications. Acc. Chem. Res. 42, 1097–1107. doi: 10.1021/ar9000026
Garcia, J. V., Yang, J. P., Shen, D. K., Yao, C., Li, X. M., Wang, R., et al. (2012). NIR-triggered release of caged nitric oxide using upconverting nanostructured materials. Small 8, 3800–3805. doi: 10.1002/smll.201201213
Giri, S., Trewyn, B. G., Stellmaker, M. P., and Lin, V. S. Y. (2005). Stimuli-responsive controlled-release delivery system based on mesoporous silica nanorods capped with magnetic nanoparticles. Angew. Chem. Int. Ed. Engl. 44, 5038–5044. doi: 10.1002/anie.200501819
He, Q., Zhang, Z., Gao, F., Li, Y., and Shi, J. (2011). In vivo biodistribution and urinary excretion of mesoporous silica nanoparticles: effects of particle size and PEGylation. Small 7, 271–280. doi: 10.1002/smll.201001459
Hu, F., and Zhao, Y. S. (2012). Inorganic nanoparticle-based T1 and T1/T2 magnetic resonance contrast probes. Nanoscale 4, 6235–6243. doi: 10.1039/c2nr31865b
Hu, H., Xiong, L., Zhou, J., Li, F., Cao, T., and Huang, C. (2009). Multimodal-luminescence core-shell nanocomposites for targeted imaging of tumor cells. Chemistry 15, 3577–3584. doi: 10.1002/chem.200802261
Jayakumar, M. K. G., Idris, N. M., and Zhang, Y. (2012). Remote activation of biomolecules in deep tissues using near-infrared-to-UV upconversion nanotransducers. Proc. Natl. Acad. Sci. U.S.A. 109, 8483–8488. doi: 10.1073/pnas.1114551109
Jing, L. H., Yang, C. H., Qiao, R. R., Niu, M., Du, M. H., Wang, D. Y., et al. (2010). Highly fluorescent CdTe@SiO2 particles prepared via reverse microemulsion method. Chem. Mater. 22, 420–427. doi: 10.1021/cm9029962
Kapse-Mistry, S., Govender, T., Srivastava, R., and Yergeri, M. (2014). Nanodrug delivery in reversing multidrug resistance in cancer cells. Front. Pharmacol. 5:159. doi: 10.3389/fphar.2014.00159
Kim, B. H., Lee, N., Kim, H., An, K., Park, Y. I., Choi, Y., et al. (2011). Large-scale synthesis of uniform and extremely small-sized iron oxide nanoparticles for high-resolution T1 magnetic resonance imaging contrast agents. J. Am. Chem. Soc. 133, 12624–12631. doi: 10.1021/ja203340u
Kim, D. H., Rozhkova, E. A., Ulasov, I. V., Bader, S. D., Rajh, T., Lesniak, M. S., et al. (2010). Biofunctionalized magnetic-vortex microdiscs for targeted cancer-cell destruction. Nat. Mater. 9, 165–171. doi: 10.1038/nmat2591
Kim, J., Kim, H. S., Lee, N., Kim, T., Kim, H., Yu, T., et al. (2008). Multifunctional uniform nanoparticles composed of a magnetite nanocrystal core and a mesoporous silica shell for magnetic resonance and fluorescence imaging and for drug delivery. Angew. Chem. Int. Ed. Engl. 47, 8438–8441. doi: 10.1002/anie.200802469
Kresge, C. T., Leonowicz, M. E., Roth, W. J., Vartuli, J. C., and Beck, J. S. (1992). Ordered mesoporous molecular-sieves synthesized by a liquid-crystal template mechanism. Nature 359, 710–712. doi: 10.1038/359710a0
Lee, S. H., Kim, B. H., Na, H. B., and Hyeon, T. (2014). Paramagnetic inorganic nanoparticles as T1 MRI contrast agents. Wiley Interdiscip. Rev. Nanomed. Nanobiotechnol. 6, 196–209. doi: 10.1002/wnan.1243
Li, C., Yang, D., Ma, P., Chen, Y., Wu, Y., Hou, Z., et al. (2013). Multifunctional upconversion mesoporous silica nanostructures for dual modal imaging and in vivo drug delivery. Small 9, 4150–4159. doi: 10.1002/smll.201301093
Li, J., Jiang, H., Yu, Z., Xia, H., Zou, G., Zhang, Q., et al. (2013). Multifunctional uniform core-shell Fe3O4@mSiO2 mesoporous nanoparticles for bimodal imaging and photothermal therapy. Chem. Asian J. 8, 385–391. doi: 10.1002/asia.201201033
Li, J., Liu, F., Shao, Q., Min, Y., Costa, M., Yeow, E. K., et al. (2014). Enzyme-responsive cell-penetrating peptide conjugated mesoporous silica quantum dot nanocarriers for controlled release of nucleus-targeted drug molecules and real-time intracellular fluorescence imaging of tumor cells. Adv. Healthc. Mater. 3, 1230–1239. doi: 10.1002/adhm.201300613
Li, W., Wang, J., Ren, J., and Qu, X. (2014). Near-infrared upconversion controls photocaged cell adhesion. J. Am. Chem. Soc. 136, 2248–2251. doi: 10.1021/ja412364m
Li, X. M., Shen, D. K., Yang, J. P., Yao, C., Che, R. C., Zhang, F., et al. (2013a). Successive layer-by-layer strategy for multi-shell epitaxial growth: shell thickness and doping position dependence in upconverting optical properties. Chem. Mater. 25, 106–112. doi: 10.1021/cm3033498
Li, X. M., Wang, R., Zhang, F., Zhou, L., Shen, D. K., Yao, C., et al. (2013b). Nd3+ sensitized up/down converting dual-mode nanomaterials for efficient in-vitro and in-vivo bioimaging excited at 800 nm. Sci. Rep. 3:3536. doi: 10.1038/srep03536
Li, X., Guo, Z., Zhao, T., Lu, Y., Zhou, L., Zhao, D., et al. (2016). Filtration shell mediated power density independent orthogonal excitations-emissions upconversion luminescence. Angew. Chem. Int. Ed. Engl. 55, 2464–2469. doi: 10.1002/anie.201510609
Li, X., Wang, R., Zhang, F., and Zhao, D. (2014a). Engineering homogeneous doping in single nanoparticle to enhance upconversion efficiency. Nano Lett. 14, 3634–3639. doi: 10.1021/nl501366x
Li, X., Zhang, F., and Zhao, D. (2015a). Lab on upconversion nanoparticles: optical properties and applications engineering via designed nanostructure. Chem. Soc. Rev. 44, 1346–1378. doi: 10.1039/C4CS00163J
Li, X., Zhao, T., Lu, Y., Wang, P., El-Toni, A. M., Zhang, F., et al. (2017). Degradation-restructuring induced anisotropic epitaxial growth for fabrication of asymmetric diblock and triblock mesoporous nanocomposites. Adv. Mater. 29, 1701652–1701659. doi: 10.1002/adma.201701652
Li, X., Zhou, L., Wei, Y., El-Toni, A. M., Zhang, F., and Zhao, D. (2014b). Anisotropic growth-induced synthesis of dual-compartment Janus mesoporous silica nanoparticles for bimodal triggered drugs delivery. J. Am. Chem. Soc. 136, 15086–15092. doi: 10.1021/ja508733r
Li, X., Zhou, L., Wei, Y., El-Toni, A. M., Zhang, F., and Zhao, D. (2015b). Anisotropic encapsulation-induced synthesis of asymmetric single-hole mesoporous nanocages. J. Am. Chem. Soc. 137, 5903–5906. doi: 10.1021/jacs.5b03207
Liu, B., Li, C., Ma, P., Chen, Y., Zhang, Y., Hou, Z., et al. (2015). Multifunctional NaYF4:Yb, Er@mSiO2@Fe3O4-PEG nanoparticles for UCL/MR bioimaging and magnetically targeted drug delivery. Nanoscale 7, 1839–1848. doi: 10.1039/C4NR05342G
Liu, J., Bu, W., Pan, L., and Shi, J. (2013). NIR-triggered anticancer drug delivery by upconverting nanoparticles with integrated azobenzene-modified mesoporous silica. Angew. Chem. Int. Ed. Engl. 52, 4375–4379. doi: 10.1002/anie.201300183
Liu, J., Qiao, S. Z., Chen, J. S., Lou, X. W., Xing, X., and Lu, G. Q. (2011a). Yolk/shell nanoparticles: new platforms for nanoreactors, drug delivery and lithium-ion batteries. Chem. Commun. 47, 12578–12591. doi: 10.1039/c1cc13658e
Liu, J., Qiao, S. Z., Hu, Q. H., and Lu, G. Q. (2011b). Magnetic nanocomposites with mesoporous structures: synthesis and applications. Small 7, 425–443. doi: 10.1002/smll.201001402
Liu, Q., Zhang, J., Xia, W., and Gu, H. (2012). Magnetic field enhanced cell uptake efficiency of magnetic silica mesoporous nanoparticles. Nanoscale 4, 3415–3421. doi: 10.1039/c2nr30352c
Lu, F., Yang, L., Ding, Y. J., and Zhu, J. J. (2016). Highly emissive Nd3+-sensitized multilayered upconversion nanoparticles for efficient 795 nm operated photodynamic therapy. Adv. Funct. Mater. 26, 4778–4785. doi: 10.1002/adfm.201600464
Luo, Z., Ding, X., Hu, Y., Wu, S., Xiang, Y., Zeng, Y., et al. (2013). Engineering a hollow nanocontainer platform with multifunctional molecular machines for tumor-targeted therapy in vitro and in vivo. ACS Nano 7, 10271–10284. doi: 10.1021/nn404676w
Matsumura, Y., and Maeda, H. (1986). A new concept for macromolecular therapeutics in cancer chemotherapy: mechanism of tumoritropic accumulation of proteins and the antitumor agent smancs. Cancer Res. 46(12 Pt 1), 6387–6392.
Meka, A. K., Abbaraju, P. L., Song, H., Xu, C., Zhang, J., Zhang, H., et al. (2016). A vesicle supra-assembly approach to synthesize amine-functionalized hollow dendritic mesoporous silica nanospheres for protein delivery. Small 12, 5169–5177. doi: 10.1002/smll.201602052
Min, Y., Li, J., Liu, F., Yeow, E. K., and Xing, B. (2014). Near-infrared light-mediated photoactivation of a platinum antitumor prodrug and simultaneous cellular apoptosis imaging by upconversion-luminescent nanoparticles. Angew. Chem. Int. Ed. Engl. 53, 1012–1016. doi: 10.1002/anie.201308834
Moller, K., and Bein, T. (2017). Talented mesoporous silica nanoparticles. Chem. Mater. 29, 371–388. doi: 10.1021/acs.chemmater.6b03629
Mykhaylyk, O., Antequera, Y. S., Vlaskou, D., and Plank, C. (2007). Generation of magnetic nonviral gene transfer agents and magnetofection in vitro. Nat. Protoc. 2, 2391–2411. doi: 10.1038/nprot.2007.352
Plank, C., Zelphati, O., and Mykhaylyk, O. (2011). Magnetically enhanced nucleic acid delivery. Ten years of magnetofection-progress and prospects. Adv. Drug Deliv. Rev. 63, 1300–1331. doi: 10.1016/j.addr.2011.08.002
Prasetyanto, E. A., Bertucci, A., Septiadi, D., Corradini, R., Castro-Hartmann, P., and De Cola, L. (2016). Breakable hybrid organosilica nanocapsules for protein delivery. Angew. Chem. Int. Ed. Engl. 55, 3323–3327. doi: 10.1002/anie.201508288
Qian, H. S., Guo, H. C., Ho, P. C., Mahendran, R., and Zhang, Y. (2009). Mesoporous-silica-coated up-conversion fluorescent nanoparticles for photodynamic therapy. Small 5, 2285–2290. doi: 10.1002/smll.200900692
Sahoo, B., Devi, K. S., Dutta, S., Maiti, T. K., Pramanik, P., and Dhara, D. (2014). Biocompatible mesoporous silica-coated superparamagnetic manganese ferrite nanoparticles for targeted drug delivery and MR imaging applications. J. Colloid. Interf. Sci. 431, 31–41. doi: 10.1016/j.jcis.2014.06.003
Scherer, F., Anton, M., Schillinger, U., Henke, J., Bergemann, C., Kruger, A., et al. (2002). Magnetofection: enhancing and targeting gene delivery by magnetic force in vitro and in vivo. Gene Ther. 9, 102–109. doi: 10.1038/sj.gt.3301624
Shen, D., Yang, J., Li, X., Zhou, L., Zhang, R., Li, W., et al. (2014). Biphase stratification approach to three-dimensional dendritic biodegradable mesoporous silica nanospheres. Nano Lett. 14, 923–932. doi: 10.1021/nl404316v
Song, E. Q., Hu, J., Wen, C. Y., Tian, Z. Q., Yu, X., Zhang, Z. L., et al. (2011). Fluorescent-magnetic-biotargeting multifunctional nanobioprobes for detecting and isolating multiple types of tumor cells. ACS Nano 5, 761–770. doi: 10.1021/nn1011336
Song, N., and Yang, Y. W. (2015). Molecular and supramolecular switches on mesoporous silica nanoparticles. Chem. Soc. Rev. 44, 3474–3504. doi: 10.1039/C5CS00243E
Teng, Z., Su, X., Zheng, Y., Zhang, J., Liu, Y., Wang, S., et al. (2015). A facile multi-interface transformation approach to monodisperse multiple-shelled periodic mesoporous organosilica hollow spheres. J. Am. Chem. Soc. 137, 7935–7944. doi: 10.1021/jacs.5b05369
Wan, Y., and Zhao, D. (2007). On the controllable soft-templating approach to mesoporous silicates. Chem. Rev. 107, 2821–2860. doi: 10.1021/cr068020s
Wang, C., Cheng, L., and Liu, Z. (2013). Upconversion nanoparticles for photodynamic therapy and other cancer therapeutics. Theranostics 3, 317–330. doi: 10.7150/thno.5284
Wang, D. W., Zhu, X. M., Lee, S. F., Chan, H. M., Li, H. W., Kong, S. K., et al. (2013). Folate-conjugated Fe3O4@SiO2@gold nanorods@mesoporous SiO2 hybrid nanomaterial: a theranostic agent for magnetic resonance imaging and photothermal therapy. J. Mater. Chem. B 1, 2934–2942. doi: 10.1039/c3tb20090f
Wang, R., Zhou, L., Wang, W. X., Li, X. M., and Zhang, F. (2017). In vivo gastrointestinal drug-release monitoring through second near-infrared window fluorescent bioimaging with orally delivered microcarriers. Nat. Commun. 8:14702. doi: 10.1038/ncomms14702
Wang, S. N., Zhang, M. C., and Zhang, W. Q. (2011). Yolk-shell catalyst of single au nanoparticle encapsulated within hollow mesoporous silica microspheres. ACS Catal. 1, 207–211. doi: 10.1021/cs1000762
Wang, Y., and Gu, H. (2015). Core-shell-type magnetic mesoporous silica nanocomposites for bioimaging and therapeutic agent delivery. Adv. Mater. 27, 576–585. doi: 10.1002/adma.201401124
Wu, M., Meng, Q., Chen, Y., Zhang, L., Li, M., Cai, X., et al. (2016). Large pore-sized hollow mesoporous organosilica for redox-responsive gene delivery and synergistic cancer chemotherapy. Adv. Mater. 28, 1963–1969 doi: 10.1002/adma.201505524
Xiong, L., Bi, J., Tang, Y., and Qiao, S. Z. (2016). Magnetic core-shell silica nanoparticles with large radial mesopores for siRNA delivery. Small 12, 4735–4742. doi: 10.1002/smll.201600531
Xu, C., Yu, M., Noonan, O., Zhang, J., Song, H., Zhang, H., et al. (2015). Core-cone structured monodispersed mesoporous silica nanoparticles with ultra-large cavity for protein delivery. Small 11, 5949–5955. doi: 10.1002/smll.201501449
Yang, C., Guo, W., Cui, L., An, N., Zhang, T., Lin, H., et al. (2014). pH-responsive magnetic core-shell nanocomposites for drug delivery. Langmuir 30, 9819–9827. doi: 10.1021/la501833u
Yang, J. P., Shen, D. K., Zhou, L., Li, W., Li, X. M., Yao, C., et al. (2013). Spatially confined fabrication of core-shell gold nanocages@mesoporous silica for near-infrared controlled photothermal drug release. Chem. Mater. 25, 3030–3037. doi: 10.1021/cm401115b
Yang, Y. N., Niu, Y. T., Zhang, J., Meka, A. K., Zhang, H. W., Xu, C., et al. (2015). Biphasic synthesis of large-pore and well-dispersed benzene bridged mesoporous organosilica nanoparticles for intracellular protein delivery. Small 11, 2743–2749. doi: 10.1002/smll.201402779
Yang, Y., Lu, Y., Abbaraju, P. L., Zhang, J., Zhang, M., Xiang, G., et al. (2017). Multi-shelled dendritic mesoporous organosilica hollow spheres: roles of composition and architecture in cancer immunotherapy. Angew. Chem. Int. Ed. Engl. 56, 8446–8450. doi: 10.1002/anie.201701550
You, Q., Sun, Q., Wang, J., Tan, X., Pang, X., Liu, L., et al. (2017). A single-light triggered and dual-imaging guided multifunctional platform for combined photothermal and photodynamic therapy based on TD-controlled and ICG-loaded CuS@mSiO2. Nanoscale 9, 3784–3796. doi: 10.1039/C6NR09042G
Yue, Q., Li, J., Luo, W., Zhang, Y., Elzatahry, A. A., Wang, X., et al. (2015). An interface coassembly in biliquid phase: toward core-shell magnetic mesoporous silica microspheres with tunable pore size. J. Am. Chem. Soc. 137, 13282–13289. doi: 10.1021/jacs.5b05619
Zhang, F., Braun, G. B., Pallaoro, A., Zhang, Y., Shi, Y., Cui, D., et al. (2012). Mesoporous multifunctional upconversion luminescent and magnetic “nanorattle” materials for targeted chemotherapy. Nano. Lett. 12, 61–67. doi: 10.1021/nl202949y
Zhang, F., Braun, G. B., Shi, Y., Zhang, Y., Sun, X., Reich, N. O., et al. (2010). Fabrication of Ag@SiO2@Y2O3:Er nanostructures for bioimaging: tuning of the upconversion fluorescence with silver nanoparticles. J. Am. Chem. Soc. 132, 2850–2851. doi: 10.1021/ja909108x
Zhang, X., Yang, P. P., Dai, Y. L., Ma, P. A., Li, X. J., Cheng, Z. Y., et al. (2013). Multifunctional up-converting nanocomposites with smart polymer brushes gated mesopores for cell imaging and thermo/pH dual-responsive drug controlled release. Adv. Funct. Mater. 23, 4067–4078. doi: 10.1002/adfm.201300136
Zhang, Y., Yue, Q., Jiang, Y. J., Luo, W., Elzatahry, A. A., Alghamdi, A., et al. (2016). A facile biliquid-interface co-assembly synthesis of mesoporous vesicles with large pore sizes. CrystEngComm 18, 4343–4348. doi: 10.1039/C5CE02592C
Zhang, Z. J., Wang, L. M., Wang, J., Jiang, X. M., Li, X. H., Hu, Z. J., et al. (2012). Mesoporous silica-coated gold nanorods as a light-mediated multifunctional theranostic platform for cancer treatment. Adv. Mater. 24, 1418–1423. doi: 10.1002/adma.201104714
Zhao, D., Feng, J., Huo, Q., Melosh, N., Fredrickson, G. H., Chmelka, B. F., et al. (1998). Triblock copolymer syntheses of mesoporous silica with periodic 50 to 300 angstrom pores. Science 279, 548–552. doi: 10.1126/science.279.5350.548
Zhao, Q. F., Wang, X. D., Yan, Y., Wang, D., Zhang, Y., Jiang, T. Y., et al. (2017). The advantage of hollow mesoporous carbon as a near-infrared absorbing drug carrier in chemo-photothermal therapy compared with IR-820. Eur. J. Pharm. Sci. 99, 66–74. doi: 10.1016/j.ejps.2016.11.031
Zhao, W. R., Chen, H. R., Li, Y. S., Li, L., Lang, M. D., and Shi, J. L. (2008). Uniform rattle-type hollow magnetic mesoporous spheres as drug delivery carriers and their sustained-release property. Adv. Funct. Mater. 18, 2780–2788. doi: 10.1002/adfm.200701317
Keywords: mesoporous, bioapplication, nanoparticles, core shell structures, asymmetric structure
Citation: Zhao T, Nguyen N-T, Xie Y, Sun X, Li Q and Li X (2017) Inorganic Nanocrystals Functionalized Mesoporous Silica Nanoparticles: Fabrication and Enhanced Bio-applications. Front. Chem. 5:118. doi: 10.3389/fchem.2017.00118
Received: 04 October 2017; Accepted: 30 November 2017;
Published: 13 December 2017.
Edited by:
Yao He, Soochow University, ChinaReviewed by:
Xiaoyong Huang, Taiyuan University of Technology, ChinaZhenmeng Peng, University of Akron, United States
Yiling Zhong, University of New South Wales, Australia
Copyright © 2017 Zhao, Nguyen, Xie, Sun, Li and Li. This is an open-access article distributed under the terms of the Creative Commons Attribution License (CC BY). The use, distribution or reproduction in other forums is permitted, provided the original author(s) or licensor are credited and that the original publication in this journal is cited, in accordance with accepted academic practice. No use, distribution or reproduction is permitted which does not comply with these terms.
*Correspondence: Qin Li, qin.li@griffith.edu.au
Xiaomin Li, lixm@fudan.edu.cn