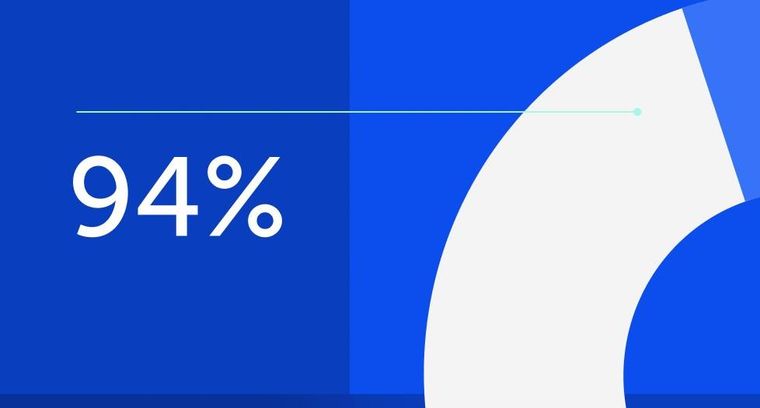
94% of researchers rate our articles as excellent or good
Learn more about the work of our research integrity team to safeguard the quality of each article we publish.
Find out more
REVIEW article
Front. Chem. Eng., 13 January 2025
Sec. Biochemical Engineering
Volume 6 - 2024 | https://doi.org/10.3389/fceng.2024.1514962
Wound healing represents a global public health problem when it is not treated correctly, which can cause complications for the patient, such as functional loss of an organ, amputation, and even death. At a biological level, wound healing involves a complex mechanism in which the immune system and cellular biochemical cascades intervene in a coordinated manner, whose development occurs in stages such as inflammation, proliferation, and remodeling. Therefore, therapies have been developed to accelerate wound healing and have proven effective. However, factors such as diabetes mellitus limit the healing process because it causes alterations in microvascular dysfunction, as well as in the inflammatory response and greater oxidative stress. This is reflected in an abnormal healing process; therefore, the search for healing compounds has become an area of interest. In this regard, medicinal plants have been used for centuries to treat wounds in different cultures in the world. Hence, this review documents the main plant species used in Latin America due to its great biodiversity and numerous species that are potentially important for the development of new active healing compounds. In this review, 62 plant families with wound healing studies were found, highlighting Fabaceae, Asteraceae and Euphorbiaceae family. Additionally, 32 natural compounds with diverse structural nature were found, whose effects have been evaluated in in vivo and in vitro models, which are essential for studying the pathogenesis of the tissue repair mechanism, detecting new biomarkers, and evaluate new treatments. Currently, several models are used to study the wound healing process, including in silico, in vitro, and in vivo models. On the other hand, there is no appropriate model to determine the wound healing effect, and, in many cases, they are combined to provide sufficient scientific evidence. Therefore, this review demonstrates that Latin America is a potential region for research into sources of healing molecules. Nevertheless, other species are still being studied whose scientific findings allow generating viable alternatives for the solution of health problems associated with wound healing.
Wound healing is a natural repair mechanism that aims to restore the affected tissue quickly (Yang et al., 2021). Although literature reports research associated with biological, immune, and biochemical events, they all converge in that healing is a process that occurs in specific stages where perfectly coordinated cells participate. In addition, it has been demonstrated that factors can compromise healing to the extent of making it impossible to recover the function of the affected organ (Järbrink et al., 2017). On the other hand, demonstrating the efficacy of wound-healing therapies is a complex challenge because the available models that demonstrate wound-healing activity allow exploring wound healing in stages, and it is necessary to use several models to determine the level of the wound-healing effect (Singh et al., 2017). Modern medicine applies therapies that promote healing, all of which have a specific basis. On the other hand, there are drugs whose active ingredients are synthetic or natural molecules, and others are still under research and development because there is no special treatment that can resolve all wounds as a general way (Zindle et al., 2021).
Natural molecules are extracted from biological sources, and plants are the most used source. Since the dawn of humanity, man has used plants to treat his health problems, while the WHO reports that 80% of the population uses them to treat their ailments (World Health Organization, 2024). That is why the present review focuses on healing medicinal plants, particularly those from Latin America, since it covers around 15% of the Earth’s surface and contains a wide biodiversity (Quijas et al., 2019; Raven et al., 2020; Alarcon Ruiz et al., 2023). Therefore, the analysis of the reports for these species provides relevant information regarding the use of medicinal plants as a source of secondary metabolites, as well as their validation of popular use by vulnerable populations whose health culture is mainly based on herbal medicine.
In the present review, Latin American scar-healing plants were selected regardless of the year because no similar review exists. The search keywords were: “healing effect of secondary plant metabolites,” “healing medicinal plant,” “healing plant extract,” “healing plants,” “wound healing,” “wound healing,” “wound healing process,” “wound healing mechanism,” “factors influencing wound healing,” “skin and wound healing,” “wound healing therapies,” “wound healing secondary metabolites,” “stages of wound healing,” “wound healing complications,” “wound treatment alternatives,” “wound healing models,” “wound healing effect,” “wound healing activity,” “wound healing biochemistry,” “wound healing effect assays.” The search yielded 477 scientific publications, of which those that were not written in English or did not have precise information were discriminated. Scientific articles on wound-healing plants that were not from Latin America were discriminated; the topics of wound-healing therapies, models of biological wound-healing trials and wound-healing treatments were considered to be 10 years old. Databases were created in order to present relevant information on plants, biological models, and therapies in tabular and graphical formats. The databases were created using Elsevier, SciFinder, PubMed, and Google Scholar, selecting the most representative sources, and a total of 223 references were considered.
Wound care is reported to be a multi-billion-dollar problem worldwide and only in USA it affects 5.7 million people (∼2% of the population) at an annual cost of $20 billion, i.e., nearly 15% of Medicare beneficiaries (8.2 million patients), and a conservative estimate of its annual cost is $28 billion (Järbrink et al., 2017; Nussbaum et al., 2018). In other countries such as the UK, chronic wound treatment and care accounts for 3% of total healthcare expenditure in developed countries (Järbrink et al., 2017). Chronic wounds are expected to remain a major clinical, social, and economic challenge, particularly in low- and middle-income country economies, although there are preclinical studies on scaffolds and dressings with promising results, translational evidence supporting their application (Jodheea-Jutton et al., 2022).
Skin accounts for 16% of the body weight, covering an average surface area of 1.85 m2, representing the human body’s largest organ. It also plays a pivotal role in maintaining homeostasis and as a protective barrier against the external environment to prevent infections and fluid losses (Díaz-García et al., 2021; Ellis et al., 2018). A skin wound results from the breakdown of the epidermal layer integrity with anatomical integrity disruption and functional loss (Jakovija and Chtanova, 2023; Cioce et al., 2024). Since the skin serves as the protective barrier against physical and chemical threats, exposure to radiation or thermal stress, and pathogen entry, a wound radically compromises its functionality (Petkovic et al., 2021), therefore, the ability of an organism to repair or regenerate tissues is a definite advantage for surviving (Díaz-García et al., 2021; Cioce et al., 2024). A wound that has failed to heal in 4 weeks is defined as a chronic wound (Järbrink et al., 2017) however, delayed wound healing in specific populations might be prevented or improved with appropriate therapies to lessen morbidity, loss of limb, and mortality (Falanga et al., 2022; Cioce et al., 2024). Chronic wounds are more susceptible to infections, and poor healing is a challenging problem for both patients and caregivers, affecting them physically, mentally, and socially, causing repercussions on their quality of life (Järbrink et al., 2017).
The skin is the largest organ in the body; anatomically, it has multiple cells that fulfill the role of protection, temperature regulation, water regulation, and aesthetic functions (Cañedo-Dorantes and Cañedo-Ayala, 2019). Therefore, it is the organ most exposed to all external agents, whereby, it has developed defense mechanisms such as healing to avoid compromising its functioning, using the different layers of the skin with their respective cells (Cañedo-Dorantes and Cañedo-Ayala, 2019; Larouche et al., 2018). Likewise, the rest of the body organs have a healing mechanism that is activated when they experience a trauma or stimulus, in general, healing is a set of physiological, dynamic, synchronized, and interdependent mechanisms that try to rebuild the skin, the mucosa or other tissue that has been damaged. It is made up of different phases, depending on the severity of the trauma; the healing time can last days, months, and even years. Figure 1 shows the phases and subphases of healing (Kucharzewski et al., 2019; Díaz-García et al., 2021; Cioce et al., 2024).
The skin has three layers (epidermis, dermis, and hypodermis), in each of which some cells work synchronously to perform the functions of the skin. However, it can have lesions that must be repaired through the healing mechanism to recover its functions (Rodrigues et al., 2019).
This complex mechanism involves numerous biochemical, immunological, and physiological reactions. Healing encompasses the phases of hemostasis, inflammation, proliferation, and remodeling. Each phase simultaneously repairs the injury (Rodrigues et al., 2019; Kucharzewski et al., 2019).
With the rupture of any of the structural levels of the blood vessel such as the external superficial tunica or adventitia, the tunica media made up of muscle fibers or the tunica intima or endothelium, primary hemostasis is activated (Figure 2), defined as the physiological phenomenon that stops bleeding, acts as a defense mechanism, and helps to protect the integrity of the vascular system. The blood that leaves the ruptured endothelium releases von Willebrand factor (vWF), and upon contact with the collagen of the vascular wall, it promotes the activation of platelets, transforming the blood into a solid clot through tissue factor (TF), which seals the injured area and stops the bleeding (Rodrigues et al., 2019; Sorg and Sorg, 2023).
The platelet plug at this stage is weak and matures through secondary hemostasis, involving the coagulation cascade, which is responsible for creating a mature and stable fibrin plug. The coagulation cascade is activated by the extrinsic (first to appear) and intrinsic (amplification of the coagulation process) pathways. The first pathway releases thrombin through tissue factor (TF), which is activated when the blood vessel ruptures, while the intrinsic pathway is triggered by platelet activation; together they generate a provisional matrix that guides fibroblast migration and ultimately repairs the wound (Rodrigues et al., 2019; Sorg and Sorg, 2023; Díaz-García et al., 2021). The process involves coagulation factors that form fibrin and anticoagulant proteins that regulate and control coagulation by preventing factors activated at a specific point from dispersing and producing generalized coagulation (Petkovic et al., 2021; Kucharzewski et al., 2019). Finally, the fibrinolysis phase is triggered. This process eliminates unnecessary fibrin, thus achieving vessel repair and reestablishing vascular flow. This phase is promoted by tissue plasminogen activators and urinary plasminogen activators, whose task is to diffuse from endothelial cells and convert plasminogen absorbed in the fibrin clot into plasmin (Petkovic et al., 2021; Kucharzewski et al., 2019). Plasmin degrades the fibrin polymer into small fragments degraded by the monocyte-macrophage clearance system (Figure 3A).
Following hemostasis, vasodilation subsides and favors circulating cells that reach the wound site. Histamine, complement derivatives, prostaglandins, bacterial peptides, and fibrin degradation products promote this stage by attracting monocytes and neutrophils. Monocytes transform into macrophages, reaching a maximum population between 24 and 48 h, and enter the wound to destroy bacteria and remove harmful agents.
Inflammation is the defensive phase; its objective is to destroy bacteria and eliminate debris, allowing the wound bed to grow new tissue in a clean area. Neutrophils migrate to the injury site, releasing elastase and collagenase enzymes to destroy the damaged tissue and phagocytize the bacteria in the wound. Subsequently, they are trapped in the clot and eliminated by apoptosis (Figure 3B) (Rodrigues et al., 2019; Ellis et al., 2018).
Additionally, macrophages perform several tasks simultaneously; some participate in the debridement of damaged tissue, and at the same time, others undergo a genetic change in their mRNA and secrete cytokines (growth factors and interleukins) that drive the wound healing phases, stimulate cells such as fibroblasts and epidermal cells for wound closure (Ellis et al., 2018; Kimura and Tsuji, 2021). Platelets, macrophages, lymphocytes, and endothelial cells release these growth factors and interleukins into the wound. Macrophages secrete most of the substances that promote healing. The inflammatory phase lasts 4–6 days and manifests as edema, redness, heat, and pain (Rodrigues et al., 2019; Larouche et al., 2018; Leonida et al., 2016).
Growth factors and interleukins secreted by macrophages are released into the wound area, stimulating fibroblast migration to form the extracellular matrix and epithelialization from the wound edges. Fibroblasts form collagen fibers, hyaluronic acid, and proteoglycans; in addition, they are receptors of fibronectin, which allows them to migrate through the clot and synthesize collagen; when the granulation tissue progresses, macrophages reabsorb the clot into the wound bed allowing the passage of collagen fibers type I, II, and III into the connective tissue (Rippon et al., 2022; Kimura and Tsuji, 2021).
Fibroblastic migration is accompanied by neovascularization of the area, and fibroblasts secrete angiogenic factors promoting capillary formation and providing oxygen and nutrients for collagen synthesis. Gradually, the granulation tissue turns reddish in response to the angiogenesis, which proceeds smoothly (Figure 4A). During the resorption of the clot, the provisional extracellular matrix is formed, the main composition of which is fibroblasts, collagen I, II, III, and the fundamental substance formed by hyaluronic acid and proteoglycans (Kimura and Tsuji, 2021; Petkovic et al., 2021).
Subsequently, a more stable secondary matrix is formed, promoted by TGF-b and hyaluronic acid. The fibroblasts change their structure (their Golgi apparatus and endoplasmic reticulum increase in size to produce a greater amount of proteins). A new type of collagen I, III, V, and elastin are synthesized, providing elasticity to the matrix. On the other hand, some fibroblasts acquire smooth muscle properties. These changes promote wound epithelialization (Larouche et al., 2018; Petkovic et al., 2021).
In the epithelialization phase, the stages of migration of epithelial cells from the borders, multiplication, and differentiation of the formed dermis develop. Simultaneously, the synthesis of the dermo-epidermal junction occurs because of the interaction between the dermis and epidermis. Growth factors coordinate this interaction. Keratinocytes proliferate from the edges of the wound towards the center. They are stimulated by growth factors released by epithelial cells (epidermal growth factor, transforming growth factor alpha, fibroblast growth factor, and keratocyte growth factor) (Rippon et al., 2022; Leonida et al., 2016).
Once the granulation tissue matures, the keratinocytes proceed to transit the fibronectin of the extracellular matrix; this fact favors the formation of the basement membrane and binding proteins, which in turn allows for normal epidermal proliferation (Rippon et al., 2022; Leonida et al., 2016).
Next, the collagen fibers undergo remodeling, while the capillaries exhibit necrosis and are reabsorbed by macrophages, and their space is replaced by collagen fibers (Figure 4B). This stage consequently generates a change in the texture of the scarred skin. The wound proceeds to contract and may last from months to years, depending on the wound area and depth (Larouche et al., 2018; Petkovic et al., 2021).
It should be noted that the stages of healing are still being studied, as well as the factors that promote ideal conditions. However, it is important to note that when healing does not flow, and some stages are delayed, tissues can be compromised. The inability of patients to close wounds for prolonged periods drastically affects their quality of life, causing pain, loss of mobility, social isolation, and risk of depression, especially in patients where chronic wounds are associated with a higher risk of amputation, without considering that they can also be associated with life-threatening infection (Rippon et al., 2022; Larouche et al., 2018). For this reason, therapies, medications, and alternatives have been developed to induce healing. Some of them are described below.
Various factors can contribute to impaired wound healing. Broadly, these factors can be divided into local and systemic categories. Local factors directly affect the wound’s specific characteristics, considering systemic factors pertaining to the individual’s overall health or underlying conditions that influence their healing capacity (Guo and DiPietro, 2010).
Local factors include foreign bodies, venous insufficiency, oxygenation (tissue hypoxia), and infections (Guo and DiPietro, 2010). Among these factors, infections are particularly significant as they occur when a skin injury allows microorganisms, normally confined to the surface, to penetrate the underlying tissues. The classification (Khalil et al., 2017) cation of the wound (whether as contaminated, colonized, locally infected/critically colonized, or suffering from spreading invasive infection) depends on the presence and replication status of these microorganisms (Edwards and Harding, 2004). Bacteria and endotoxins can prolong the inflammatory phase by sustaining elevated levels of proinflammatory cytokines. If unresolved, this persistent inflammation can lead to chronic wounds that fail to heal (Menke et al., 2007). Oxygenation also plays a critical role in wound healing. Oxygen supports cellular metabolism, prevents wound infections, promotes angiogenesis, and aids keratinocyte differentiation, migration, and epithelialization (Rodriguez et al., 2008). It further enhances fibroblast proliferation, collagen synthesis, and wound contraction (Bishop, 2008; Cialdai et al., 2022). While temporary hypoxia following an injury can stimulate healing, prolonged or chronic hypoxia impairs the process (Hong et al., 2014).
On the other hand, systematic factors include chemotherapy, nutrition, stress, age, and disease. For example, studies report that chemotherapy drugs and anticoagulants prevent the inflammation phase of the healing process and, therefore, increase the risk of wound infection, increasing healing time. Also, other medications, such as low-dose topical corticosteroids and antibiotics, accelerate wound healing (Khalil et al., 2017; Wagner et al., 2008). However, the authors report that other factors, such as being over 65 years of age, pressure/friction/shear, non-adherence to treatment, infection, obesity, stress, anxiety, and depression, are also important in poor wound healing and increased wound complications significantly affecting healing times, regardless of pharmacological treatment (Ubbink et al., 2015).
On the other hand, reactive oxygen species (ROS) play a crucial role in both the physiological and pathological aspects of wound healing. They are involved in various processes of skin tissue regeneration, including the regulation of inflammation, cell proliferation, angiogenesis, granulation tissue formation, and extracellular matrix development (Dong and Wang, 2023; Xu et al., 2020). Overproduction and accumulation of ROS obstruct the change of wound tissue from the inflammatory stage to the proliferative stage. Consequently, the wound area becomes chronically inflamed, resulting in delayed wound healing (Deng et al., 2019).
As already mentioned, psychological conditions can directly influence wound healing processes. Psychological stress can indirectly modulate the repair process by promoting the adoption of unhealthy behaviors. Furthermore, increased production of glucocorticoids and catecholamines while under stress, or decreased expression of proinflammatory cytokines at the wound site, can directly influence several components of the healing process, such as the initial inflammatory phase (Ebrecht et al., 2004; Gouin and Kiecolt-Glaser, 2011).
This highlights that successful wound healing is a multifactorial process influenced by external factors and each patient’s individual internal conditions.
Chronic wounds are characterized by their inability to heal within an expected timeframe. Over the last several decades, they have emerged as an increasingly important clinical problem due to their higher incidence and greater recognition of associated morbidity and socio-economic burden 3 (Falanga et al., 2022). Chronic wounds are often related to conditions (Figure 5) such as obesity, diabetes, and old age and fall into three categories: pressure ulcers, arterial or venous ulcers, and diabetic ulcers (Nuutila et al., 2014).
Pressure injuries are localized skin and soft tissue injuries that develop due to prolonged pressure exerted over specific areas of the body, typically bony prominences; common locations for these ulcers include over the sacrum, ischial tuberosity, greater trochanter, heel, and lateral malleolus(Gaspar et al., 2019; Mervis and Phillips, 2019). Most people affected by pressure ulcers are those who have mental or physical health conditions that favor immobility, especially those who are confined to bed or chair for prolonged periods (Leblebici et al., 2007). External and internal factors co-occur to form these ulcers; externally, prolonged pressure, friction, shear force, and moisture can lead to tissue deformation and ischemia. Internal factors such as malnutrition, anemia, and endothelial dysfunction can speed up the process of tissue damage (Anders et al., 2010; Bansal et al., 2005).
Venous ulcers are late manifestations of chronic venous insufficiency and venous hypertension. That can cause disability and severe complications (Bonkemeyer Millan et al., 2019). As the global population ages, the disease has shown a worldwide growing incidence (Robles-Tenorio et al., 2024). Most risk factors for developing venous ulcers are non-modifiable, and patients often present more than one. These involve a family history of chronic venous insufficiency, advanced age, female sex, previous thrombosis or pulmonary embolism, multiparity, lipodermatosclerosis, and musculoskeletal and joint disease (Santler and Goerge, 2017). Modifiable risk factors such as obesity and sedentarism are also associated with venous disease (Santler and Goerge, 2017).
Diabetes mellitus is a metabolic endocrine disorder due to an overall deficiency of insulin (Type 1) or defective insulin function (Type 2), which causes hyperglycemia (Meulendijks et al., 2020). A complication of diabetes mellitus is diabetic ulcers, which leads to increased overall morbidity in patients. Patients with diabetes mellitus type 1 or 2 have a total lifetime risk of a diabetic foot ulcer complication as high as 25% (Packer et al., 2023). Diabetic foot ulcers are classified as neuropathic, ischemic, or a combination of both, that is, neuroischemi (Boulton et al., 2008). However, around 90% of diabetic foot ulcerations are diabetic peripheral neuropathy.
Chronic wounds harm health-related quality of life due to the physical injury, the treatment required, the chronicity of the condition, and the likelihood of recurrence. The likelihood of wound healing is highly associated with wound-based and patient-based factors or attributes, such as age and associated diseases, such as diabetes (Guo and DiPietro, 2010).
As described, wound healing is the process of tissue repair involving the tissue response to injury (Steed, 1997). Any failure in the normal wound healing process results in abnormal scar formation and a chronic state more susceptible to infection (Díaz-García et al., 2021; Kolimi et al., 2022). Currently, the therapeutic strategies used for wound healing depend on the type of wound and the intrinsic regenerative capacity (Edmonds et al., 2000; Kolimi et al., 2022). Treatments for wounds, including surgical and non-surgical, are shown in Figure 6.
Growth factors (GFs) are polypeptides that bind to specific receptors on the cell surface, initiating signaling pathways that activate key molecules. These activated molecules may further stimulate cytoplasmic proteins or promote the transcription of new proteins (Singer and Clark, 1999). GFs regulate cell growth, differentiation, and metabolism through these mechanisms across the three phases of wound healing (Steed, 1997). In addition to driving the proliferation, differentiation, and migration of repair cells like keratinocytes, fibroblasts, and vascular endothelial cells, GFs also influence repair cell apoptosis, extracellular matrix composition, DNA, RNA, and protein synthesis, glycolysis, and tissue remodeling (Han et al., 2020).The topical application of growth factors shows promise in treating various skin wounds by accelerating healing or preparing healthy beds for surgical intervention (He et al., 2024). Key growth factors in wound healing and skin regeneration include PDGF, VEGF, EGF, TGF-α, IGF, bFGF, KGF, and TGF-β1‒3 (Park et al., 2017). Growth factors are essential in skin regeneration, highlighting their importance in regenerative medicine and wound treatment (Mitchell et al., 2016).
The wound healing process is also influenced by our skin’s endogenous electric potential, also dubbed the endogenous skin battery (Farber et al., 2014). These endogenous electric fields play a critical role in wound healing, resulting in endogenous currents acting as cues for cellular migration, which concomitantly help heal wounds (Sun, 2017; Zhao, 2009). The therapeutic use of electrical stimulation in medical practice has been established, particularly in pain and wound management (Rajendran et al., 2021). Electrotherapy may have multiple modes of action in wound healing; one possible mechanism is introducing an exogenous electrical signal in the complex cellular and biochemical events within the wound itself. Another method consists of increasing blood circulation through the wound site by stimulating the operation of the peripheral arterial and vascular systems through an electrical signal applied to the muscle motor nerve (Sun, 2017; Zhao, 2009). Commonly used waveforms for electrical stimulation therapy are direct current, alternating current, pulsed current, and degenerate wave (Kloth, 2014).
Vacuum-assisted closure is a non-invasive, negative pressure healing technique to treat chronic, non-healing wounds. This technique uses controlled subatmospheric pressure to remove excess wound fluid from the extravascular space, i2mproving local oxygenation and peripheral blood flow (Enoch et al., 2006; Genecov et al., 1998). This promotes angiogenesis and the formation of granulation tissue, which are particularly useful in deep cavitating wounds to expedite the filling of the wound space (Enoch et al., 2006; Kairinos et al., 2009). This method requires thorough debridement, adequate hemostasis, and application of sterile foam dressing. A fenestrated tube is embedded in the foam, and the wound is sealed to make it airtight. The fenestrate tube is connected to a vacuum pump with a fluid collection container. The machine delivers continuous or intermittent suction, ranging from 50 to 125 mmHg (Timmers et al., 2005). Using vacuum-assisted closure and negative-pressure therapy, it is possible to stabilize the wound, reduce edema and bacterial load, improve tissue perfusion, and stimulate granulation tissue (Agarwal et al., 2019).
Hyperbaric oxygen has been recommended as an adjunctive therapy to treat a variety of non-healing wounds (as many non-healing tissues are hypoxic) (Enoch et al., 2006). It promotes neutrophil-mediated bacterial killing ability in hypoxic tissue. Also, it prevents the release of proteases and free radicals in specific injuries, thereby decreasing vasoconstriction, edema, and cellular damage (De Smet et al., 2017). The treatment is administered by increasing atmospheric pressure in a chamber while the patient is exposed to 100% oxygen. Since wounds need oxygen to heal correctly, exposing one to 100% oxygen can speed healing (Jones and Cooper, 2023).
Ozone therapy has accelerated healing by minimizing inflammation and reducing edema (Naik et al., 2016). Suggested mechanisms of therapeutic enhancement are that it acts as a potent oxidizing agent (Sagai and Bocci, 2011) and enhances tissue repair by removing defective cells, bacteria, and viruses by acting as a powerful disinfectant (Greer et al., 2012). It has also been shown to promote angiogenesis and stimulate fibroblast activity, further accelerating wound healing (Pchepiorka et al., 2020). This therapy has proven particularly effective in treating diabetic foot ulcers, ischemic wounds, and peripheral vascular disease, representing some of its most common applications (Adhikari and Khanal, 2013) (Smith et al., 2017). On the other hand, the route of administration can be by exposure to gaseous ozone within a hyperbaric chamber, application of ozonized oils, or ozonized water (Azarpazhooh and Limeback, 2008; Fitzpatrick et al., 2018).
Plasma, the fourth state of matter, is an ionized gas comprising both stable components (gases) and reactive elements such as ions, energetic particles, and radicals (Adhikari and Khanal, 2013). The use of plasma for wound healing and tissue regeneration has been advanced by developing the “Plazon” system. This system employs a rapidly quenching hot air plasma jet, resulting in a relatively high nitrogen oxide (NO) concentration with significant therapeutic effects (Shekhter et al., 1998). A plasma device operates in two distinct modes: the “hot mode,” in which the plasma jet is used for rapid coagulation, sterilization of wound surfaces, removal and desiccation of dead tissue and pathological growths, as well as tissue dissection, and the “cold mode,” which provides a flow of nitrogen oxide-enriched plasma gas at a temperature of 20°C–40°C to stimulate regenerative processes and enhance wound healing (Fridman et al., 2008). The physical mechanism of plasma-based treatment involves the generation of free radicals and reactive species for the desired wound healing-promoting effects. In contrast, the biological mechanism exploits various cellular processes responsible for DNA and cell membrane damage of bacteria (Kolimi et al., 2022).
Photobiomodulation (PBM) involves the use of nonionizing forms of light from sources including lasers, light-emitting diodes (LEDs), and broadband light in the visible and near-infrared (NIR) spectra to cause physiological changes and therapeutic benefits (Houreld, 2019). Both in vitro and in vivo, it effectively promotes wound healing and cell proliferation. PBM has been used successfully in the healing of diabetic wounds because it reduces oxidative stress and inflammation, accelerates essential cell proliferation and extracellular matrix deposition, and enhances tissue repair (Mgwenya et al., 2024). On the other hand, It has been suggested that laser irradiation in the red light region increases activity in the plasma membrane of cells (Kujawa et al., 2014). The optimal wavelength for treatment is generally considered to be 810 nm. However, wavelengths ranging up to 950 nm are needed to reach cutaneous tissue sites and below the tissue (Wang et al., 2017). It is currently considered that stem cell bioactivity, such as cell migration, proliferation, survival, and overall cellular niche, can be enhanced or positively modulated by PBM (Zamani et al., 2020). Despite this, PBM is not used in mainstream medicine, and this is largely due to a lack of comprehension of the therapy and the molecular mechanisms underlying it (Mgwenya et al., 2024).
Skin grafting is the transfer of cutaneous tissue from one portion of the body to another, often used to cover large wounds. It provides a barrier function that limits wound desiccation, fluid loss, and bacterial contamination and protects underlying viable tissue. In addition, it improves the granulation tissue and becomes incorporated into the recipient tissue bed (Braza and Fahrenkopf, 2023; Hermans, 2011). Autografts are derived from a patient’s healthy skin. Challenges in autograft use include the introduction of a new wound, contracture and scar formation, infection and bleeding risk, and decreased or increased sensation (Shi and Ronfard, 2013). However, autografts provide the advantages of availability and decreased immunogenicity (Chandler et al., 2020).
Skin allografts, or homografts, are tissues harvested from a donor site of the same species with different genetic components (Hermans, 2011). The main lines of attack on the allograft problem are the host’s immune response and the possibility of disease transmission (Megahed et al., 2021).
Skin xenografts may be a temporary, initial treatment that prepares the wound bed for autologous transplantation. When using xenografts, factors such as immunogenicity and disease transmission must be considered (Ma et al., 2008). The most common xenografts used to treat chronic wounds include porcine, bovine, and, more recently, fish (Stubenitsky et al., 2009; Yamamoto et al., 2018).
Hydrogels are intricate three-dimensional structures composed of hydrophilic polymer chains and exhibit a quick swelling response upon contact with water, forming a partially solid material (Ahmed, 2015). They have been proposed as potential remedies because they provide a moist environment that facilitates wound healing (Gounden and Singh, 2024). Hydrogels can be classified according to polymeric composition as homopolymeric hydrogels are referred to polymer networks derived from a single species of monomer, copolymeric hydrogels are comprised of two or more different monomer species with at least one hydrophilic and multipolymer interpenetrating made of two independent cross-linked synthetic and natural polymer components, contained in a network form (Hacker and Mikos, 2011; Maolin et al., 2000). Hydrogels possess biodegradability and biocompatibility, allowing them to serve as a temporary template throughout the re-epithelialization and remodeling of chronic wounds (Maaz Arif et al., 2021).
Cell therapy can be applied for both acute and chronic wounds. In acute treatment wounds, cell therapy can increase the wound healing rate, reduce scar contracture, and minimize donor-site morbidity. On the other hand, in treating chronic wounds, including diabetic ulcers, attempts are made to convert the wound bed into an environment where maximum wound healing can be achieved by transplanting cells with an excellent wound healing capacity to the wound bed (Park et al., 2010; You and Han, 2014). Cell therapy can use autologous or allogeneic cells. Autologous cells accelerate wound healing by reducing the time needed for the host cells to invade the wound tissue and by early synthesis of new skin (Seo et al., 2007). Allogeneic cells promote migration and proliferation of host cells from the wound beds and edges because these cells release growth factors, extracellular matrices, and basement membrane components (You and Han, 2014).
Ultrasound waves have emerged as a promising alternative or adjunctive strategy for chronic wounds (Alkahtani et al., 2017). These waves are delivered to the body and soft tissues and undergo diffusion. The molecules progressively lose their energy through vibrating as the waves pass through the tissue. The range of ultrasonic frequencies that are utilized for therapeutic ultrasound spans ranges from 20 kHz to about 3 MHz (Mason, 2011). In vitro studies have shown that the therapeutic outcomes of ultrasonic waves on tunneling, or debilitation wounds are mainly through killing multi-drug resistant bacteria (Serena et al., 2009). Several in vitro studies have shown that these waves improve cell proliferation, collagen production, bone formation, and angiogenesis (Speed, 2001).
Infections are a worrying complication of skin wounds, which can lead to delayed healing and, in severe cases, sepsis and death. Infected wounds present clinically with erythema, heat, edema, and local pain or tenderness (Yousefian et al., 2023). Usual practice suggests the use of topical or dressing antiseptics, which can be classified into different classes according to their mechanism of action, including emulsifiers, oxidants, acids, heavy metals, alcohols, aldehydes, anilides, bisphenols, and phenols (Cambiaso-Daniel et al., 2018). In clinical practice, the most used are Silver-Based or Iodine-Based Wound Dressings and various classes of antibiotics, including aminoglycosides, beta-lactams, glycopeptides, quinolones, sulfonamides, and tetracyclines, incorporated or not into wound dressings, some examples are listed in Table 1 (Jones and Cooper, 2023; Simões et al., 2018).
Non-steroidal anti-inflammatory drugs (NSAIDs) have been shown to have a depressant effect on wound healing while simultaneously decreasing the granulocytic inflammatory reaction (Su et al., 2010). However, these are prescribed post-soft-tissue injury or post-surgery to assist with pain control management and to diminish inflammation. Short-term use of NSAIDs, such as ibuprofen, after surgery is beneficial for its analgesic effect, but patients with chronic wounds or diabetes could be more dramatically affected by NSAID’s effect on fibroblast inhibition (Legendre et al., 2008). So, it is important that in these conditions, NSAIDs should be used with caution.
All the procedures described above are applied in different cases, depending on the type of wound, the patient’s state of health, allergies, diet, and age. Pharmacological methods have been scientifically developed to determine and measure the efficacy of treatments at different stages of wound healing. These trials are vital since numerous therapies are still under development that could promote the healing process in various stages and contribute to patient treatment alternatives. It should be noted that the population is diverse, and the health system is not the same worldwide. Hence, the availability of treatments for wound healing is an important measure to avoid complications that compromise the survival of individuals due to the complications that can arise from wounds that do not heal progressively.
The characteristics of the wound, its etiological nature, and other factors in the clinical history, together with regular follow-up, help the healthcare team to know at what stage of the healing process the wound is in and to decide the best therapeutic approach. The investigation of any physiological process depends on the use of models; there is no single appropriate model due to the lack of an ideal comprehensive model that approximates humans; a combination of models is a better alternative to studying the complex cellular and molecular interactions that take place during the wound healing process in an appropriate biological environment (Masson-Meyers et al., 2020; Menke et al., 2010; Parnell and Volk, 2019; Sami et al., 2019).
Over the last few decades, efforts to understand the healing process have been redoubled, and experimental models of wound healing have been developed to try to fully explain tissue regeneration and test new treatment strategies (Masson-Meyers et al., 2020; Wilhelm et al., 2017). These models are currently divided into in vitro, in vivo, and in silico (Table 2).
In vitro and in vivo models have provided valuable information for many medical discoveries and advances. In vitro models, such as cell culture, scratch model, and skin explant culture, are essential in several of these studies; in vivo models involve wounding a laboratory animal and observing wound closure over time (Ansell et al., 2012; Grada et al., 2018; Masson-Meyers et al., 2020). However, they are not always adequate and in many cases are unable to support finding the actual etiology of lesions; for example, the intricate biological pathway that takes place during wound healing is not always reflected in animal models, so it is necessary to consider human models, because these models are not always good predictors, but provide helpful insight into acute wound disease pathology. On the other hand, several mathematical equations have been used to evaluate the phases of healing in the in silico models, but one of the disadvantages is that they lack the biophysical characteristics of human skin and remain theoretical until biologically confirmed by in vitro or in vivo models (Sami et al., 2019). The usual course of healing is known, but for a thorough understanding of the kinetics of cell types and subtypes and the signaling pathways or intermediates involved, to cite a few examples, it is necessary to address the problem both in the laboratory and the clinic (Nuutila et al., 2014; Zindle et al., 2021; 2021). Even in the clinical area, it is difficult to understand chronic wound processes because human models are not always good predictors, and it is difficult to have adequate volunteers with chronic wounds, so human models of chronic wounds provide a better opportunity to understand the wound healing process. Several factors can influence wound healing (aging, infections, medications, nutritional status, obesity, diabetes, venous insufficiency, and peripheral arterial disease) (Grada et al., 2018; Wilhelm et al., 2017). The lack of optimal preclinical models capable of adequately recapitulating human wounds remains a significant challenge and is worth further study.
Certainly, the therapies described in this review are widely used and recommended, as are biological assays to determine their efficacy. However, since the beginning of mankind, man has used medicinal plants to solve his health problems, and the treatment of wounds with medicinal plants is no exception.
Numerous reports validate the widespread use of different plant species in traditional medicine with a healing effect. Our review focuses on plant species that grow in the area known as Latin America, which stands out for having about 50% of the planet’s biodiversity, which allows it to sustain natural ecosystems that provide welfare to society. Unsurprisingly, Latin American countries have species used in traditional medicine as healers (Armenteras et al., 2021).
Brazil reports 88 wound-healing plant species, making it the country with the most excellent knowledge of its plants with wound-healing effects. On the other hand, Mexico reports 23 species, while Peru has 9, and the rest of the countries report fewer species. It is worth mentioning that Argentina, Chile, Colombia, and Costa Rica have at least one study on healing plants (Figure 7). Only a few of the plants studied were introduced into the region, which indicates that the research focuses on native plants. These data suggest that the study of medicinal plants with wound-healing uses is fundamental because plants are the primary source of natural products.
The bar chart looks at the seven botanic families most studied in Latin America for their wound-healing effect. However, 62 botanic families are reported in research articles. Despite this, only five families have three or more published articles (Table 3; Supplementary Table S1).
There is a noticeable increase in the number of studied species of Fabaceae. This family has 17 species with scientifically proven research, followed by Asteraceae and Euphorbiaceae, which have 15 and 10, respectively. The other families only have one or two reports (Figure 8).
The chart shows that the three main models used to evaluate the wound-healing capacity in vitro are: Scratch assay is the most common model, with 72.34% of research, followed by MTT assay, with 23.40%. The sum of these two models is approximately 95% of research made (Supplementary Table S2).
On the other hand, Fibroblasts from Swiss mice and HaCaT cells were used in the Scratch assay and MTT assay, and healing effects were shown in both models (Figure 9).
On the other hand, the most popular in vivo assay is the excisional wound model, used in more than 50% of the investigations performed in Latin America. The incisional wound is also frequently used, sometimes in comparison with the excisional wound. These models were mostly tested in Wistar rats or Swiss mice; other biological models were also used (Figure 10).
On the other hand, bioactive compounds from different plant species have been reported; the main ones are shown in Figure 11. These compounds are of different chemical nature, suggesting that there are still compounds with healing potential (Supplementary Table S3).
Figure 11. Reported wound healing compounds. 1: 2ʺ-O-rhamnosylswertisin, 2: 7-O-(β-d-glucopyranosyl)-galactin, 3: (+)-anymol, 4: (+)-epi-α-bisabolol, 5: 1,2,3,4,6-pentagalloylhexose, 6: 3-O-α-L- rhamnopyranosyl- (1→4)-β-D- glucopyranosyl- (1→3)-[β-D- glucopyranosyl-(1→2)]-β-D- fucopyranosyl- 16,23,28-dri, 7: 3-O-α-L- rhamnopyranosyl- (1→4)-β-D- glucopyranosyl- (1→3)-[β-D- glucopyranosyl-(1→2)]-β-D- fucopyranosyl- 16,23,28-trihydroxyoleane- 11,13(18)-diene, 8: 3α-hydroxy masticadienoic acid, 9: 3β, 6β,16β-trihydroxylup- 20(29)-ene, 10: epicatechin3-O-gallate, 11: 4′-O- methylepicatechin-3- O-gallate, 12: 9-palmitamide, 13: octadecenamide, 14: Bornesitol, 15: ellagic acid, 16: gallic acid, 17: isoquercitrin, 18: kaempferol, 19: lenticin, 20: methyl gallate, 21: methyl hexadecanoate, 22: myricetin, 23: oleic acid, 24: phenylalanine-betaine, 25: pinocembrin, 26: quercetin, 27: quercitrin, 28: quinic acid, 29: rosmarinic acid, 30: rutin, 31: salicylic acid, 32: swertisin, 33: taspine hydrochloride.
Among the species worth highlighting from the families shown in Figures 8, 9, is the effect of the alcoholic extract of Achillea asiatica flowers belonging to the Asteraceae family, with an impact on cutaneous wound healing (in vitro and in vivo), demonstrating that the treatment significantly increased epithelialization and accelerated wound healing in a rat model (Dorjsembe et al., 2017). A relevant species of the Fabaceae family is Glycyrrhiza glabra, with a healing effect on full-thickness dermal wounds in a rat model, gastric wound healing, oral mucosal, ulcers, and colitis, and even the extract of this plant was effective in wound healing in guinea pig (Hanafi et al., 2018). Very recently, in a study with Jatropha mollissima (Pohl) Baill. (Euphorbiaceae) which is widely used in traditional medicine to treat skin disorders; a topical gel containing the hydroethanolic extract of its leaves showed potential for wound healing, providing an accelerated wound healing effect (process in 3 and 5 days) in rats (Passos et al., 2024).
Although some studies claim that multiple drugs are necessary to ensure combined pro-regenerative functions, drugs do not follow the wound healing cascade in real time, so it is essential to develop new treatments that can act on pathogenic molecules and cells with multiple targets and intervene in the cascade.
It is, therefore, essential to develop new treatments that can act on pathogenic molecules and cells with multiple targets and intervene in the wound-healing cascade (Cai et al., 2023). Therefore, it is essential to continue searching and testing treatments that either come directly from medicinal plants and are used as herbal medicines or are based on the structural relationship of some bioactive molecules found in plant species, which allow us to have more alternatives that are essential, given the increase in chronic degenerative diseases that lead to the development of wounds such as diabetic foot ulcer.
Wound healing is a global health problem that requires attention because its phases must flow synchronously. When wound healing is prolonged, and its phases do not heal promptly, complications may arise that compromise the recovery of the damaged tissue. Wound healing depends on the patient’s state of health, age, and diet, among other factors. For this reason, it is suggested that treatments that promote healing be incorporated and validated using appropriate trials to guarantee their safe use. On the other hand, medicinal plants are a privileged source of healing compounds. Unfortunately, most of them have not been scientifically validated. Particularly, Latin American countries are geographically favored because they have environmental conditions that allow high biodiversity; taking advantage of natural resources to solve health problems is a valuable contribution that gives back significantly to society. Finally, the species reported now with healing effects come from different families. Therefore, continuing ethnomedical studies of species popularly used as healers is essential.
MS-R: Writing–original draft, Investigation. AR-B: Writing–original draft, Formal Analysis, Investigation. ST-C: Writing–original draft, Formal Analysis, Investigation. AR-G: Writing–review and editing, Validation. MC-P: Writing–original draft, Validation. AG-A: Conceptualization, Writing–review and editing. AB-A: Formal Analysis, Supervision, Writing–original draft. DO-R: Investigation, Writing–review and editing. FC-S: Writing–review and editing, Conceptualization.
The author(s) declare that no financial support was received for the research, authorship, and/or publication of this article.
The authors declare that the review was conducted in the absence of any commercial or financial relationships that could be construed as a potential conflict of interest.
The author(s) declare that no Generative AI was used in the creation of this manuscript.
All claims expressed in this article are solely those of the authors and do not necessarily represent those of their affiliated organizations, or those of the publisher, the editors and the reviewers. Any product that may be evaluated in this article, or claim that may be made by its manufacturer, is not guaranteed or endorsed by the publisher.
The Supplementary Material for this article can be found online at: https://www.frontiersin.org/articles/10.3389/fceng.2024.1514962/full#supplementary-material
Adhikari, B. R., and Khanal, R. (2013). Introduction to the plasma state of matter. Himal. Phys. 4, 60–64. doi:10.3126/hj.v4i0.9430
Agarwal, P., Kukrele, R., and Sharma, D. (2019). Vacuum assisted closure (VAC)/negative pressure wound therapy (NPWT) for difficult wounds: a review. J. Clin. Orthop. Trauma 10, 845–848. doi:10.1016/j.jcot.2019.06.015
Agra, I. K. R., Pires, L. L. S., Carvalho, P. S. M., Silva-Filho, E. A., Smaniotto, S., and Barreto, E. (2013). Evaluation of wound healing and antimicrobial properties of aqueous extract from Bowdichia virgilioides stem barks in mice. An. Acad. Bras. Ciênc. 85, 945–954. doi:10.1590/S0001-37652013005000049
Aguayo-Morales, H., Sierra-Rivera, C. A., Claudio-Rizo, J. A., and Cobos-Puc, L. E. (2023). Horsetail (Equisetum hyemale) extract accelerates wound healing in diabetic rats by modulating IL-10 and MCP-1 release and collagen synthesis. Pharmaceuticals 16, 514. doi:10.3390/ph16040514
Ahmed, E. M. (2015). Hydrogel: preparation, characterization, and applications: a review. J. Adv. Res. 6, 105–121. doi:10.1016/j.jare.2013.07.006
Alarcon Ruiz, C. A., Maguiña, J. L., Apolaya Segura, M., Carhuapoma Yance, M., Aranda Ventrura, J., and Herrera Añazco, P. (2023). Bibliometric analysis of medicinal plants’ original articles from Latin America and the Caribbean Region. J. Scientometr. Res. 12 (1), 79–91. doi:10.5530/jscires.12.1.011
Alerico, G. C., Beckenkamp, A., Vignoli-Silva, M., Buffon, A., and Von Poser, G. L. (2015). Proliferative effect of plants used for wound healing in Rio Grande do Sul state, Brazil. J. Ethnopharmacol. 176, 305–310. doi:10.1016/j.jep.2015.11.001
Alkahtani, S. A., Kunwar, P. S., Jalilifar, M., Rashidi, S., and Yadollahpour, A. (2017). Ultrasound-based techniques as alternative treatments for chronic wounds: a comprehensive review of clinical applications. Cureus 9, e1952. doi:10.7759/cureus.1952
Alves Barros, A. S., Oliveira Carvalho, H., Dos Santos, I. V. F., Taglialegna, T., Dos Santos Sampaio, T. I., Duarte, J. L., et al. (2017). Study of the non-clinical healing activities of the extract and gel of Portulaca pilosa L. in skin wounds in wistar rats: a preliminary study. Biomed. and Pharmacother. 96, 182–190. doi:10.1016/j.biopha.2017.09.142
Amorim, E., Matias, J. E. F., Coelho, J. C. U., Campos, A. C. L., Stahlke Jr, H. J., Timi, J. R. R., et al. (2006). Efeito do uso tópico do extrato aquoso de Orbignya phalerata (babaçu) na cicatrização de feridas cutâneas: estudo controlado em ratos. Acta Cir. Bras. 21, 67–76. doi:10.1590/S0102-86502006000800011
Amorim, J. L., Figueiredo, J. D. B., Amaral, A. C. F., Barros, E. G. D. O., Palmero, C., Mpalantinos, M. A., et al. (2017). Wound healing properties of Copaifera paupera in diabetic mice. PLoS ONE 12, e0187380. doi:10.1371/journal.pone.0187380
Anders, J., Heinemann, A., Leffmann, C., Leutenegger, M., Pröfener, F., and von Renteln-Kruse, W. (2010). Decubitus ulcers: pathophysiology and primary prevention. Dtsch. Arztebl Int. 107, 371–381. doi:10.3238/arztebl.2010.0371
Ansell, D. M., Holden, K. A., and Hardman, M. J. (2012). Animal models of wound repair: are they cutting it? Exp. Dermatol. 21 (8), 581–585. doi:10.1111/j.1600-0625.2012.01540.x
Antoszewska, M., Sokolewicz, E. M., and Barańska-Rybak, W. (2024). Wide use of hyaluronic acid in the process of wound healing—a rapid review. Sci. Pharm. 92, 23. doi:10.3390/scipharm92020023
Andrade, T. A. M., Iyer, A., Das, P. K., Foss, N. T., Garcia, S. B., Coutinho-Netto, J., et al. (2011). The inflammatory stimulus of a natural latex biomembrane improves healing in mice. Brazilian J. Medi. Biologi. Res. 44, 1036–1047. doi:10.1590/s0100-879x2011007500116
Arcaro, G., Koga, A. Y., Carletto, B., Budel, G. M., Rocha Gaspar, M. D. D., Nadal, J. M., et al. (2023). Preclinical trial of Ocotea puberula (rich.) nees (“Canela-Guaicá”) in wound healing: validation of a traditional medicine practice used by indigenous groups in southern Brazil. Evidence-Based Complementary Altern. Med. 2023, 3641383–3641388. doi:10.1155/2023/3641383
Armenteras, D., Dávalos, L. M., Barreto, J. S., Miranda, A., Hernández-Moreno, A., Zamorano-Elgueta, C., et al. (2021). Fire-induced loss of the world’s most biodiverse forests in Latin America. Sci. Advan. 7 (33), eabd3357. doi:10.1126/sciadv.abd3357
Azarpazhooh, A., and Limeback, H. (2008). The application of ozone in dentistry: a systematic review of literature. J. Dent. 36, 104–116. doi:10.1016/j.jdent.2007.11.008
Balachandran, A., Choi, S. B., Beata, M.-M., Małgorzata, J., Froemming, G. R. A., Lavilla, C. A., et al. (2023). Antioxidant, wound healing potential and in silico assessment of naringin, eicosane and octacosane. Molecules 28, 1043. doi:10.3390/molecules28031043
Bansal, C., Scott, R., Stewart, D., and Cockerell, C. J. (2005). Decubitus ulcers: a review of the literature. Int. J. Dermatol 44, 805–810. doi:10.1111/j.1365-4632.2005.02636.x
Batista, E. K. F., Trindade, H. I. D., Farias, I. D. S., Martins, F. M. M., Silva Filho, O. F. D., and Batista, M. D. C. D. S. (2017). Avaliação da atividade cicatrizante de preparados à base de jucá (Caesalpinia ferrea Mart.). AVS 22. doi:10.5380/avs.v22i3.50360
Benvenutti, L., Nunes, R., Venturi, I., Ramos, S. A., Broering, M. F., Goldoni, F. C., et al. (2021). Anti-inflammatory and healing activity of the hydroalcoholic fruit extract of Solanum diploconos (mart.) bohs. J. Immunol. Res. 2021, 1–13. doi:10.1155/2021/9957451
Bishop, A. (2008). Role of oxygen in wound healing. J. Wound Care 17, 399–402. doi:10.12968/jowc.2008.17.9.30937
Bonkemeyer Millan, S., Gan, R., and Townsend, P. E. (2019). Venous ulcers: diagnosis and treatment. Am. Fam. Physician 100, 298–305.
Boulton, A. J., Armstrong, D. G., Albert, S. F., Frykberg, R. G., Hellman, R., Kirkman, M. S., et al. (2008). Reprint—comprehensive foot examination and risk assessment: a report of the task force of the foot care interest group of the American diabetes association, with endorsement by the American association of clinical endocrinologists. Phys. Ther. 88, 1436–1443. doi:10.1093/ptj/88.11.1436
Braza, M. E., and Fahrenkopf, M. P. (2023). “Split-thickness skin grafts,” in StatPearls (Treasure Island (FL): StatPearls Publishing).
Bridi, H., Beckenkamp, A., Ccana-Ccapatinta, G. V., De Loreto Bordignon, S. A., Buffon, A., and Von Poser, G. L. (2017). Characterization of phloroglucinol-enriched fractions of Brazilian Hypericum species and evaluation of their effect on human keratinocytes proliferation. Phytotherapy Res. 31, 62–68. doi:10.1002/ptr.5727
Bueno, F. G., Moreira, E. A., Morais, G. R. D., Pacheco, I. A., Baesso, M. L., Leite-Mello, E. V. D. S., et al. (2016). Enhanced cutaneous wound healing in vivo by standardized crude extract of Poincianella pluviosa. PLoS ONE 11, e0149223. doi:10.1371/journal.pone.0149223
Bueno, F. G., Panizzon, G. P., Mello, E. V. S. D. L., Lechtenberg, M., Petereit, F., Mello, J. C. P. D., et al. (2014). Hydrolyzable tannins from hydroalcoholic extract from Poincianella pluviosa stem bark and its wound-healing properties: phytochemical investigations and influence on in vitro cell physiology of human keratinocytes and dermal fibroblasts. Fitoterapia 99, 252–260. doi:10.1016/j.fitote.2014.10.007
Cai, Y., Chen, K., Liu, C., and Qu, X. (2023). Harnessing strategies for enhancing diabetic wound healing from the perspective of spatial inflammation patterns. Bioact. Mater. 28, 243–254. doi:10.1016/j.bioactmat.2023.04.019
Calero-Armijos, L. L., Herrera-Calderon, O., Arroyo-Acevedo, J. L., Rojas-Armas, J. P., Hañari-Quispe, R. D., and Figueroa-Salvador, L. (2020). Histopathological evaluation of latex of Bellaco-Caspi, Himatanthus sucuuba (Spruce) Woodson on wound healing effect in BALB/C mice. Vet. World 13, 1045–1049. doi:10.14202/vetworld.2020.1045-1049
Cambiaso-Daniel, J., Boukovalas, S., Bitz, G. H., Branski, L. K., Herndon, D. N., and Culnan, D. M. (2018). Topical antimicrobials in burn care: Part 1-topical antiseptics. Ann. Plast. Surg. doi:10.1097/SAP.0000000000001297
Cañedo-Dorantes, L., and Cañedo-Ayala, M. (2019). Skin acute wound healing: a comprehensive review. Int. J. Inflamm. 2019 (1), 1–15. doi:10.1155/2019/3706315
Carmignan, F., Matias, R., Carollo, C. A., Dourado, D. M., Fermiano, M. H., Silva, B. A. K., et al. (2020). Efficacy of application of Equisetum pyramidale Goldm. hydrogel for tissue restoration of induced skin lesions in Wistar rats. Braz. J. Biol. 80, 12–22. doi:10.1590/1519-6984.184409
Carneiro, R. D. S., Canuto, M. R., Ribeiro, L. K., Ferreira, D. C. L., Assunção, A. F. C., Costa, C. A. C. B., et al. (2022). Novel antibacterial efficacy of ZnO nanocrystals/Ag nanoparticles loaded with extract of Ximenia americana L. stem bark for wound healing. South Afr. J. Bot. 151, 18–32. doi:10.1016/j.sajb.2022.09.030
Carvalho, E. G., Soares, C. P., Blau, L., Menegon, R. F., and Joaquim, W. M. (2014). Wound healing properties and mucilage content of Pereskia aculeata from different substrates. Rev. Bras. Farmacogn. 24, 677–682. doi:10.1016/j.bjp.2014.11.008
Carvalho, G. R., Braz, D. S., Gonçalves, T. C. O., Aires, R., Côco, L. Z., Guidoni, M., et al. (2022). Development and evaluation of Virola oleifera formulation for cutaneous wound healing. Antioxidants 11, 1647. doi:10.3390/antiox11091647
Casao, T. D. R. L., Pinheiro, C. G., Sarandy, M. M., Zanatta, A. C., Vilegas, W., Novaes, R. D., et al. (2020). Croton urucurana Baillon stem bark ointment accelerates the closure of cutaneous wounds in knockout IL-10 mice. J. Ethnopharmacol. 261, 113042. doi:10.1016/j.jep.2020.113042
Castro, A. H. F., Braga, K. D. Q., Sousa, F. M. D., Coimbra, M. C., and Chagas, R. C. R. (2016). Callus induction and bioactive phenolic compounds production from Byrsonima verbascifolia (L.) DC. (Malpighiaceae). Rev. ciência agronômica 47. doi:10.5935/1806-6690.20160017
Castro Souza Junior Neto, J. D., Estevão, L. R. D. M., Baratella-Evêncio, L., Vieira, M. G. F., Simões, R. S., Florencio-Silva, R., et al. (2017). Mast cell concentration and skin wound contraction in rats treated with Ximenia americana L. Bras. 32, 148–156. doi:10.1590/s0102-865020170207
Cesca, T. G., Faqueti, L. G., Rocha, L. W., Meira, N. A., Meyre-Silva, C., De Souza, M. M., et al. (2012). Antinociceptive, anti-inflammatory and wound healing features in animal models treated with a semisolid herbal medicine based on Aleurites moluccana L. Willd. Euforbiaceae standardized leaf extract. J. Ethnopharmacol. 143, 355–362. doi:10.1016/j.jep.2012.06.051
Chandler, L. A., Alvarez, O. M., Blume, P. A., Kim, P. J., Kirsner, R. S., Lantis, J. C., et al. (2020). Wound conforming matrix containing purified homogenate of dermal collagen promotes healing of diabetic neuropathic foot ulcers: comparative analysis versus standard of care. Adv. Wound Care (New Rochelle) 9, 61–67. doi:10.1089/wound.2019.1024
Chattopadhyay, S., and Raines, R. T. (2014). Collagen-based biomaterials for wound healing. Biopolymers 101, 821–833. doi:10.1002/bip.22486
Cialdai, F., Risaliti, C., and Monici, M. (2022). Role of fibroblasts in wound healing and tissue remodeling on Earth and in space. Front. Bioeng. Biotechnol. 10, 958381. doi:10.3389/fbioe.2022.958381
Cioce, A., Cavani, A., Cattani, C., and Scopelliti, F. (2024). Role of the skin immune system in wound healing. Cells 13 (7), 624. doi:10.3390/cells13070624
Coco, J. C., Ataide, J. A., Sake, J. A., Tambourgi, E. B., Ehrhardt, C., and Mazzola, P. G. (2022). In vitro antioxidant and wound healing properties of baru nut extract (Dipteryx alata Vog.) in pulmonary epithelial cells for therapeutic application in chronic pulmonary obstructive disease (COPD). Nat. Prod. Res. 36, 4469–4475. doi:10.1080/14786419.2021.1984909
Coriolano, M. C., De Melo, C. M. L., Silva, F. D. O., Schirato, G. V., Porto, C. S., Dos Santos, P. J. P., et al. (2014). Parkia pendula seed lectin: potential use to treat cutaneous wounds in healthy and immunocompromised mice. Appl. Biochem. Biotechnol. 172, 2682–2693. doi:10.1007/s12010-013-0692-2
Corrêa, A. C. L., Oliveira, A. K. M., Dourado, D. M., Facco, G. G., Bento, L. M. A., Rivero-Wendt, C. L. G., et al. (2022). Pouteria ramiflora leaf extract on emulgel in wound healing activity in diabetic rats. Braz. J. Biol. 82, e239378. doi:10.1590/1519-6984.239378
De Almeida, B. M., Dos Santos, I. D. D., De Carvalho, F. M. A., Correa, L. C., Cunha, J. L. S., Dariva, C., et al. (2022). Himatanthus bracteatus-composed in situ polymerizable hydrogel for wound healing. IJMS 23, 15176. doi:10.3390/ijms232315176
De Campos, E. P., Trombini, L. N., Rodrigues, R., Portella, D. L., Werner, A. C., Ferraz, M. C., et al. (2015). Healing activity of Casearia sylvestris Sw. in second-degree scald burns in rodents. BMC Res. Notes 8, 269. doi:10.1186/s13104-015-1251-4
De Gaspi, F. O. D. G., Foglio, M. A., De Carvalho, J. E., Santos, G. M. T., Testa, M., Passarini, J. R., et al. (2011). Effects of the topical application of hydroalcoholic leaf extract of Oncidium flexuosum sims. (Orchidaceae) and microcurrent on the healing of wounds surgically induced in wistar rats. Evidence-Based Complementary Altern. Med. 2011, 1–9. doi:10.1155/2011/950347
De Moura, F., Justino, A., Ferreira, B., Espindola, F., Araújo, F., and Tomiosso, T. (2019). Pro-fibrogenic and anti-inflammatory potential of a polyphenol-enriched fraction from annona crassiflora in skin repair. Planta Med. 85, 570–577. doi:10.1055/a-0733-7151
De Moura, F. B. R., Ferreira, B. A., Deconte, S. R., Landim, B. C., Justino, A. B., Aro, A. A. D., et al. (2021). Wound healing activity of the hydroethanolic extract of the leaves of Maytenus ilicifolia Mart. Ex Reis. J. Traditional Complementary Med. 11, 446–456. doi:10.1016/j.jtcme.2021.03.003
De Moura, F. B. R., Ferreira, B. A., Muniz, E. H., Justino, A. B., Silva, A. G., Santos, R. D. A., et al. (2022). Topic use of Annona crassiflora Mart. contributes to wound healing due to the antioxidant and proliferative effects of fibroblasts. Injury 53, 844–857. doi:10.1016/j.injury.2021.12.007
De Moura Sperotto, N. D., Steffens, L., Veríssimo, R. M., Henn, J. G., Péres, V. F., Vianna, P., et al. (2018). Wound healing and anti-inflammatory activities induced by a Plantago australis hydroethanolic extract standardized in verbascoside. J. Ethnopharmacol. 225, 178–188. doi:10.1016/j.jep.2018.07.012
Deng, Z., Shi, F., Zhou, Z., Sun, F., Sun, M.-H., Sun, Q., et al. (2019). M1 macrophage mediated increased reactive oxygen species (ROS) influence wound healing via the MAPK signaling in vitro and in vivo. Toxicol. Appl. Pharmacol. 366, 83–95. doi:10.1016/j.taap.2019.01.022
De Oliveira, M. L. M., Nunes-Pinheiro, D. C. S., Tomé, A. R., Mota, É. F., Lima-Verde, I. A., Pinheiro, F. G. D. M., et al. (2010). In vivo topical anti-inflammatory and wound healing activities of the fixed oil of Caryocar coriaceum Wittm. seeds. J. Ethnopharmacol. 129, 214–219. doi:10.1016/j.jep.2010.03.014
De Oliveira Rodrigues, R., Yaochite, J. N. U., Sasahara, G. L., Albuquerque, A. A., Da Cruz Fonseca, S. G., De Vasconcelos Araújo, T. D., et al. (2020). Antioxidant, anti-inflammatory and healing potential of ethyl acetate fraction of Bauhinia ungulata L. (Fabaceae) on in vitro and in vivo wound model. Mol. Biol. Rep. 47, 2845–2859. doi:10.1007/s11033-020-05332-7
De Smet, G. H. J., Kroese, L. F., Menon, A. G., Jeekel, J., Van Pelt, A. W. J., Kleinrensink, G., et al. (2017). Oxygen therapies and their effects on wound healing. Wound Repair Regen. 25, 591–608. doi:10.1111/wrr.12561
Díaz-García, D., Filipová, A., Garza-Veloz, I., and Martinez-Fierro, M. L. (2021). A beginner’s introduction to skin stem cells and wound healing. Int. J. Mol. Sci. 22, 11030. doi:10.3390/ijms222011030
Do Nascimento Neto, L. G., Vasconcelos, M. A. D., Pinheiro, A. D. A., Ramos, A. P. M., Silva, R. B. D., Andrade, A. L., et al. (2022). Wound healing activity of lectin isolated from seeds of Centrolobium microchaete Mart. ex Benth. on cutaneous wounds in mice. Nat. Prod. Res. 36, 4734–4739. doi:10.1080/14786419.2021.2005053
Dong, Y., and Wang, Z. (2023). ROS-scavenging materials for skin wound healing: advancements and applications. Front. Bioeng. Biotechnol. 11, 1304835. doi:10.3389/fbioe.2023.1304835
Dorjsembe, B., Lee, H. J., Kim, M., Dulamjav, B., Jigjid, T., and Nho, C. W. (2017). Achillea asiatica extract and its active compounds induce cutaneous wound healing. J. Ethnopharmacol. 206, 306–314. doi:10.1016/j.jep.2017.06.006
Dos Santos, V. L. P., Ribas, J. L. C., De Lima, C. P., Campos, R., Garcia, A. C., Budel, J. M., et al. (2020). The wound healing effect of aqueous extract from Piper amalago L. in diabetic patient. EXPLORE 16, 368–371. doi:10.1016/j.explore.2019.12.001
Ebrecht, M., Hextall, J., Kirtley, L.-G., Taylor, A., Dyson, M., and Weinman, J. (2004). Perceived stress and cortisol levels predict speed of wound healing in healthy male adults. Psychoneuroendocrinology 29, 798–809. doi:10.1016/S0306-4530(03)00144-6
Edmonds, M., Bates, M., Doxford, M., Gough, A., and Foster, A. (2000). New treatments in ulcer healing and wound infection. Diabetes/Metabolism Res. Rev. 16, S51–S54. doi:10.1002/1520-7560(200009/10)16:1+<::AID-DMRR142>3.0.CO;2-S
Edwards, R., and Harding, K. G. (2004). Bacteria and wound healing. Curr. Opin. Infect. Dis. 17, 91–96. doi:10.1097/00001432-200404000-00004
Ellis, S., Lin, E. J., and Tartar, D. (2018). Immunology of wound healing. Curr. Dermatol. Rep. 7, 350–358. doi:10.1007/s13671-018-0234-9
Enoch, S., Grey, J. E., and Harding, K. G. (2006). Non-surgical and drug treatments. BMJ 332, 900–903. doi:10.1136/bmj.332.7546.900
Espinosa-Espinosa, L., Canales-Alvarez, O., Rodríguez-López, M. G., Flores-Tinajero, C. A., Canales-Martinez, M. M., and Rodriguez-Monroy, M. A. (2023). Biological activity of bursera schlechtendalii essential oil and the roles of its chemical components in the wound healing process. IJMS 24, 11040. doi:10.3390/ijms241311040
Espinosa-Espinosa, L., Garduño-Siciliano, L., Rodriguez-Canales, M., Hernandez-Portilla, L. B., Canales-Martinez, M. M., and Rodriguez-Monroy, M. A. (2022). The wound-healing effect of mango peel extract on incision wounds in a murine model. Molecules 27, 259. doi:10.3390/molecules27010259
Falanga, V., Isseroff, R. R., Soulika, A. M., Romanelli, M., Margolis, D., Kapp, S., et al. (2022). Chronic wounds. Nat. Rev. Dis. Prim. 8, 50. doi:10.1038/s41572-022-00377-3
Farber, P. L., Hochman, B., Furtado, F., and Ferreira, L. M. (2014). Electricity and colloidal stability: how charge distribution in the tissue can affects wound healing. Med. Hypotheses 82, 199–204. doi:10.1016/j.mehy.2013.11.038
Fedel-Miyasato, L. E. S., Kassuya, C. A. L., Auharek, S. A., Formagio, A. S. N., Cardoso, C. A. L., Mauro, M. O., et al. (2014). Evaluation of anti-inflammatory, immunomodulatory, chemopreventive and wound healing potentials from Schinus terebinthifolius methanolic extract. Rev. Bras. Farmacogn. 24, 565–575. doi:10.1016/j.bjp.2014.08.004
Ferreira, M. O. G., Ribeiro, A. B., Rizzo, M. S., De Jesus Oliveira, A. C., Osajima, J. A., Estevinho, L. M., et al. (2022). Potential wound healing effect of gel based on chicha gum, chitosan, and Mauritia flexuosa oil. Biomedicines 10, 899. doi:10.3390/biomedicines10040899
Figueiredo, F. D. F., Cechinel Filho, V., Damazo, A. S., Arunachalam, K., Colodel, E. M., Ribeiro, M., et al. (2020). Sorocea guilleminiana Gaudich.: wound healing activity, action mechanisms, and chemical characterization of the leaf infusion. J. Ethnopharmacol. 248, 112307. doi:10.1016/j.jep.2019.112307
Fitzpatrick, E., Holland, O. J., and Vanderlelie, J. J. (2018). Ozone therapy for the treatment of chronic wounds: a systematic review. Int. Wound J. 15, 633–644. doi:10.1111/iwj.12907
Freitas, K. M., Barcelos, L. S., Caliari, M. V., Salas, C. E., and Lopes, M. T. P. (2017). Healing activity of proteolytic fraction (P1G10) from Vasconcellea cundinamarcensis in a cutaneous wound excision model. Biomed. and Pharmacother. 96, 269–278. doi:10.1016/j.biopha.2017.09.109
Fridman, G., Friedman, G., Gutsol, A., Shekhter, A. B., Vasilets, V. N., and Fridman, A. (2008). Applied plasma medicine. Plasma Process. Polym. 5, 503–533. doi:10.1002/ppap.200700154
Fujishima, M. A. T., Sá, D. M. C., Lima, C. M. D. S., Bittencourt, J. A. H. M., Pereira, W. L. A., Muribeca, A. D. J. B., et al. (2020). Chemical profiling of Curatella americana Linn leaves by UPLC-HRMS and its wound healing activity in mice. PLoS ONE 15, e0225514. doi:10.1371/journal.pone.0225514
Galvão, F., Dos Santos, E., Gomes Da Silva Dantas, F., Irlan Da Silva Santos, J., Da Paz Costa Sauda, T., Carvalho Dos Santos, A., et al. (2023). Chemical composition and effects of ethanolic extract and gel of Cochlospermum regium (Schrank) Pilg. Leaves on inflammation, pain, and wounds. J. Ethnopharmacol. 302, 115881. doi:10.1016/j.jep.2022.115881
Gaspar, S., Peralta, M., Marques, A., Budri, A., and Gaspar de Matos, M. (2019). Effectiveness on hospital-acquired pressure ulcers prevention: a systematic review. Int. Wound J. 16, 1087–1102. doi:10.1111/iwj.13147
Geller, F., Schmidt, C., Göttert, M., Fronza, M., Schattel, V., Heinzmann, B., et al. (2010). Identification of rosmarinic acid as the major active constituent in Cordia americana. J. Ethnopharmacol. 128, 561–566. doi:10.1016/j.jep.2010.01.062
Geller, F. C., Teixeira, M. R., Pereira, A. B. D., Dourado, L. P. A., Souza, D. G., Braga, F. C., et al. (2015). Evaluation of the wound healing properties of Hancornia speciosa leaves: Hancornia speciosa. Phytother. Res. 29, 1887–1893. doi:10.1002/ptr.5438
Genecov, D. G., Schneider, A. M., Morykwas, M. J., Parker, D., White, W. L., Argenta, L. C., et al. (1998). A controlled subatmospheric pressure dressing increases the rate of skin graft donor site reepithelialization. Ann. Plastic Surg. 40, 219–225. doi:10.1097/00000637-199803000-00004
Gouin, J.-P., and Kiecolt-Glaser, J. K. (2011). The impact of psychological stress on wound healing: methods and mechanisms. Immunol. Allergy Clin. North Am. 31, 81–93. doi:10.1016/j.iac.2010.09.010
Gounden, V., and Singh, M. (2024). Hydrogels and wound healing: current and future prospects. Gels 10, 43. doi:10.3390/gels10010043
Grada, A., Mervis, J., and Falanga, V. (2018). Research techniques made simple: animal models of wound healing. J. Investigative Dermatology 138, 2095–2105.e1. doi:10.1016/j.jid.2018.08.005
Greer, N., Foman, N., Dorrian, J., Fitzgerald, P., MacDonald, R., Rutks, I., et al. (2012). “Introduction,” in Advanced wound care therapies for non-healing diabetic, venous, and arterial ulcers: a systematic review (Washington (DC): Department of Veterans Affairs) (US).
Guidi, A. C., De Paula, M. N., Mosela, M., Delanora, L. A., Soares, G. C. A., De Morais, G. R., et al. (2020). Stem bark extract of Poincianella pluviosa incorporated in polymer film: evaluation of wound healing and anti-staphylococcal activities. Injury 51, 840–849. doi:10.1016/j.injury.2020.02.027
Guo, S., and DiPietro, L. A. (2010). Factors affecting wound healing. J. Dent. Res. 89, 219–229. doi:10.1177/0022034509359125
Hacker, M. C., and Mikos, A. G. (2011). “Synthetic polymers,” in Principles of regenerative medicine (Elsevier), 587–622. doi:10.1016/B978-0-12-381422-7.10033-1
Han, C.-M., Cheng, B., and Wu, P.writing group of growth factor guideline on behalf of Chinese Burn Association (2020). Clinical guideline on topical growth factors for skin wounds. Burns Trauma 8, tkaa035. doi:10.1093/burnst/tkaa035
Hanafi, N., Talebpour Amiri, F., Shahani, S., Enayatifard, R., and Ghasemi, M. (2018). Licorice cream promotes full-thickness wound healing in Guinea pigs. jrp 22, 84–94. doi:10.12991/jrp.2018.81
He, W., Guan, N., Huang, R., Huang, X., Qu, L., Zhong, Z., et al. (2024). The effects of traditional Chinese medicine on growth factors. Clin. Traditional Med. Pharmacol. 5, 200131. doi:10.1016/j.ctmp.2024.200131
Heil, N., Bravo, K., Montoya, A., Robledo, S., and Osorio, E. (2017). Wound healing activity of Ullucus tuberosus, an Andean tuber crop. Asian Pac. J. Trop. Biomed. 7, 538–543. doi:10.1016/j.apjtb.2017.05.007
Hermans, M. H. E. (2011). Preservation methods of allografts and their (lack of) influence on clinical results in partial thickness burns. Burns 37, 873–881. doi:10.1016/j.burns.2011.01.007
Hernandez-Hernandez, A. B., Alarcon-Aguilar, F. J., Almanza-Perez, J. C., Nieto-Yañez, O., Olivares-Sanchez, J. M., Duran-Diaz, A., et al. (2017). Antimicrobial and anti-inflammatory activities, wound-healing effectiveness and chemical characterization of the latex of Jatropha neopauciflora Pax. J. Ethnopharmacol. 204, 1–7. doi:10.1016/j.jep.2017.04.003
Hernández-Pasteur, G., Silva-Bermúdez, P. S., Reyes-Chilpa, R., Vibrans, H., and Soto-Hernández, M. (2019). Evaluación in vitro de la Actividad Cicatrizante y Antibacteriana de Extractos de Buddleja cordata Kunth y Vismia baccifera (L.) Triana and Planch. Fitotec. Mex. 42, 93–99. doi:10.35196/rfm.2019.2.93
Herrera-Calderón, O., Calero-Armijos, L. L., Cardona-G, W., Herrera-R, A., Moreno, G., Algarni, M. A., et al. (2021). Phytochemical screening of himatanthus sucuuba (spruce) woodson (apocynaceae) latex, in vitro cytotoxicity and incision wound repair in mice. Plants 10, 2197. doi:10.3390/plants10102197
Hofmann, E., Fink, J., Pignet, A.-L., Schwarz, A., Schellnegger, M., Nischwitz, S. P., et al. (2023). Human in vitro skin models for wound healing and wound healing disorders. Biomedicines 11 (4), 1056. doi:10.3390/biomedicines11041056
Hong, W. X., Hu, M. S., Esquivel, M., Liang, G. Y., Rennert, R. C., McArdle, A., et al. (2014). The role of hypoxia-inducible factor in wound healing. Adv. Wound Care (New Rochelle) 3, 390–399. doi:10.1089/wound.2013.0520
Houreld, N. (2019). Healing effects of photobiomodulation on diabetic wounds. Appl. Sci. 9, 5114. doi:10.3390/app9235114
Jakovija, A., and Chtanova, T. (2023). Skin immunity in wound healing and cancer. Front. Immunol. 14, 1060258. doi:10.3389/fimmu.2023.1060258
Järbrink, K., Ni, G., Sönnergren, H., Schmidtchen, A., Pang, C., Bajpai, R., et al. (2017). The humanistic and economic burden of chronic wounds: a protocol for a systematic review. Syst. Rev. 6, 15. doi:10.1186/s13643-016-0400-8
Jodheea-Jutton, A., Hindocha, S., and Bhaw-Luximon, A. (2022). Health economics of diabetic foot ulcer and recent trends to accelerate treatment. Foot (Edinb) 52, 101909. doi:10.1016/j.foot.2022.101909
Jones, M. W., and Cooper, J. S. (2023). “Hyperbaric therapy for wound healing,” in StatPearls (Treasure Island (FL): StatPearls Publishing).
Jorge, M. P., Madjarof, C., Ruiz, A. L. T. G., Fernandes, A. T., Rodrigues, R. A. F., De Oliveira Sousa, I. M., et al. (2008). Evaluation of wound healing properties of Arrabidaea chica Verlot extract. J. Ethnopharmacol. 118, 361–366. doi:10.1016/j.jep.2008.04.024
Kairinos, N., Voogd, A. M., Botha, P. H., Kotze, T., Kahn, D., Hudson, D. A., et al. (2009). Negative-pressure wound therapy II: negative-pressure wound therapy and increased perfusion. Just an illusion? Plastic Reconstr. Surg. 123, 601–612. doi:10.1097/PRS.0b013e318196b97b
Khalil, H., Cullen, M., Chambers, H., and McGrail, M. (2017). Medications affecting healing: an evidence-based analysis. Int. Wound J. 14, 1340–1345. doi:10.1111/iwj.12809
Kimura, S., and Tsuji, T. (2021). Mechanical and immunological regulation in wound healing and skin reconstruction. Int. J. Mol. Sci. 22 (11), 5474. doi:10.3390/ijms22115474
Kloth, L. C. (2014). Electrical stimulation technologies for wound healing. Adv. Wound Care 3, 81–90. doi:10.1089/wound.2013.0459
Kolimi, P., Narala, S., Nyavanandi, D., Youssef, A. A. A., and Dudhipala, N. (2022). Innovative treatment strategies to accelerate wound healing: trajectory and recent advancements. Cells 11, 2439. doi:10.3390/cells11152439
Kreuger, M. R. O., Farias, B. G., Moreira, J., Blind, L. Z., Amoah, S. K. S., Leite, A. S., et al. (2012). Effects of the topical application of an ethyl acetate fraction from Vernonia scorpioides on excisional wounds infected with Staphylococcus aureus in rats. Rev. Bras. Farmacogn. 22, 123–130. doi:10.1590/S0102-695X2011005000192
Kucharzewski, M., Rojczyk, E., Wilemska-Kucharzewska, K., Wilk, R., Hudecki, J., and Los, M. J. (2019). Novel trends in application of stem cells in skin wound healing. Eur. J. Pharmacol. 843, 307–315. doi:10.1016/j.ejphar.2018.12.012
Kujawa, J., Pasternak, K., Zavodnik, I., Irzmański, R., Wróbel, D., and Bryszewska, M. (2014). The effect of near-infrared MLS laser radiation on cell membrane structure and radical generation. Lasers Med. Sci. 29, 1663–1668. doi:10.1007/s10103-014-1571-y
Larouche, J., Sheoran, S., Maruyama, K., and Martino, M. M. (2018). Immune regulation of skin wound healing: mechanisms and novel therapeutic targets. Adv. wound care 7 (7), 209–231. doi:10.1089/wound.2017.0761
Leblebici, B., Turhan, N., Adam, M., and Akman, M. N. (2007). Clinical and epidemiologic evaluation of pressure ulcers in patients at a university hospital in Turkey. J. Wound Ostomy Cont. Nurs. 34, 407–411. doi:10.1097/01.WON.0000281657.63449.1c
Legendre, C., Debure, C., Meaume, S., Lok, C., Golmard, J. L., and Senet, P. (2008). Impact of protein deficiency on venous ulcer healing. J. Vasc. Surg. 48, 688–693. doi:10.1016/j.jvs.2008.04.012
Leite, M. N., Leite, S. N., Caetano, G. F., Andrade, T. A. M. D., Fronza, M., and Frade, M. A. C. (2020). Healing effects of natural latex serum 1% from Hevea brasiliensis in an experimental skin abrasion wound model. An. Bras. Dermatol. 95, 418–427. doi:10.1016/j.abd.2019.12.003
Leonida, M. D., Kumar, I., Leonida, M. D., and Kumar, I. (2016). Wound healing and skin regeneration. Bionanomaterials Skin Regen., 17–25. doi:10.1007/978-3-319-39168-7_3
Lima, I. C., Castro, R. R., Adjafre, B. L., Sousa, S. H. A. F., De Paula, D. S., Alves, A. P. N. N., et al. (2022). Galactomannan of Delonix regia seeds modulates cytokine expression and oxidative stress eliciting anti-inflammatory and healing effects in mice cutaneous wound. Int. J. Biol. Macromol. 203, 342–349. doi:10.1016/j.ijbiomac.2022.01.144
Lopes, G. C., Sanches, A. C. C., Nakamura, C. V., Dias Filho, B. P., Hernandes, L., and Mello, J. C. P. D. (2005). Influence of extracts of Stryphnodendron polyphyllum Mart. and Stryphnodendron obovatum Benth. on the cicatrisation of cutaneous wounds in rats. J. Ethnopharmacol. 99, 265–272. doi:10.1016/j.jep.2005.02.019
Ma, A., Qi, S., and Chen, H. (2008). Antioxidant therapy for prevention of inflammation, ischemic reperfusion injuries and allograft rejection. Cardiovasc Hematol. Agents Med. Chem. 6, 20–43. doi:10.2174/187152508783329966
Maaz Arif, M., Khan, S. M., Gull, N., Tabish, T. A., Zia, S., Ullah Khan, R., et al. (2021). Polymer-based biomaterials for chronic wound management: promises and challenges. Int. J. Pharm. 598, 120270. doi:10.1016/j.ijpharm.2021.120270
Malveira Cavalcanti, J., Henrique Leal-Cardoso, J., Leite Diniz, L. R., Gomes Portella, V., Oliveira Costa, C., Barreto Medeiros Linard, C. F., et al. (2012). The essential oil of Croton zehntneri and trans-anethole improves cutaneous wound healing. J. Ethnopharmacol. 144, 240–247. doi:10.1016/j.jep.2012.08.030
Maolin, Z., Jun, L., Min, Y., and Hongfei, H. (2000). The swelling behavior of radiation prepared semi-interpenetrating polymer networks composed of polyNIPAAm and hydrophilic polymers. Radiat. Phys. Chem. 58, 397–400. doi:10.1016/S0969-806X(99)00491-0
Martín-Aragón, S., and Marcos, E. (2008). Tratamiento de las cicatrices. Revisión. Farm. Prof. 22, 39–43.
Mason, T. J. (2011). Therapeutic ultrasound an overview. Ultrason. Sonochem 18, 847–852. doi:10.1016/j.ultsonch.2011.01.004
Masson-Meyers, D. S., Andrade, T. A. M., Caetano, G. F., Guimaraes, F. R., Leite, M. N., Leite, S. N., et al. (2020). Experimental models and methods for cutaneous wound healing assessment. Int. J. Exp. Pathology 101, 21–37. doi:10.1111/iep.12346
Mazutti Da Silva, S., Rezende Costa, C., Martins Gelfuso, G., Silva Guerra, E., De Medeiros Nóbrega, Y., Gomes, S., et al. (2018). Wound healing effect of essential oil extracted from Eugenia dysenterica DC (myrtaceae) leaves. Molecules 24, 2. doi:10.3390/molecules24010002
Megahed, M. A., Elkashity, S. A., Talaab, A. A., and AboShaban, M. S. (2021). The impact of human skin allograft as A temporary substitute for early coverage of major burn wounds on clinical outcomes and mortality. Ann. Burns Fire Disasters 34, 67–74.
Menke, N. B., Cain, J. W., Reynolds, A., Chan, D. M., Segal, R. A., Witten, T. M., et al. (2010). An in silico approach to the analysis of acute wound healing. Wound Repair Regen. 18, 105–113. doi:10.1111/j.1524-475X.2009.00549.x
Menke, N. B., Ward, K. R., Witten, T. M., Bonchev, D. G., and Diegelmann, R. F. (2007). Impaired wound healing. Clin. Dermatology 25, 19–25. doi:10.1016/j.clindermatol.2006.12.005
Mervis, J. S., and Phillips, T. J. (2019). Pressure ulcers: pathophysiology, epidemiology, risk factors, and presentation. J. Am. Acad. Dermatol 81, 881–890. doi:10.1016/j.jaad.2018.12.069
Meulendijks, A. M., Franssen, W. M. A., Schoonhoven, L., and Neumann, H. a. M. (2020). A scoping review on Chronic Venous Disease and the development of a Venous Leg Ulcer: the role of obesity and mobility. J. Tissue Viability 29, 190–196. doi:10.1016/j.jtv.2019.10.002
Mgwenya, T. N., Abrahamse, H., and Houreld, N. N. (2024). Photobiomodulation studies on diabetic wound healing: an insight into the inflammatory pathway in diabetic wound healing. Wound Repair Regen. n/a 33, e13239. doi:10.1111/wrr.13239
Mitchell, A. C., Briquez, P. S., Hubbell, J. A., and Cochran, J. R. (2016). Engineering growth factors for regenerative medicine applications. Acta Biomater. 30, 1–12. doi:10.1016/j.actbio.2015.11.007
Moura-Letts, G., Villegas, L. F., Marçalo, A., Vaisberg, A. J., and Hammond, G. B. (2006). In vivo wound-healing activity of oleanolic acid derived from the acid hydrolysis of Anredera d iffusa. J. Nat. Prod. 69, 978–979. doi:10.1021/np0601152
Naik, S. V., K, R., Kohli, S., Zohabhasan, S., and Bhatia, S. (2016). Ozone- A biological therapy in dentistry- reality or myth? TODENTJ 10, 196–206. doi:10.2174/1874210601610010196
Nascimento-Neto, L. G. D., Evaristo, F. F. V., Alves, M. F. D. A., Albuquerque, M. R. J. R., Santos, H. S. D., Bandeira, P. N., et al. (2015). Effect of the triterpene 3β, 6β, 16β-trihydroxylup-20(29)-ene isolated from the leaves of Combretum leprosum Mart. on cutaneous wounds in mice. J. Ethnopharmacol. 171, 116–120. doi:10.1016/j.jep.2015.05.037
Nuñez, D., Olavegoya, P., Gonzales, G. F., and Gonzales-Castañeda, C. (2017). Red maca (Lepidium meyenii), a plant from the Peruvian highlands, promotes skin wound healing at sea level and at high altitude in adult male mice. High Alt. Med. and Biol. 18, 372–383. doi:10.1089/ham.2017.0038
Nussbaum, S. R., Carter, M. J., Fife, C. E., DaVanzo, J., Haught, R., Nusgart, M., et al. (2018). An economic evaluation of the impact, cost, and Medicare policy implications of chronic nonhealing wounds. Value Health 21, 27–32. doi:10.1016/j.jval.2017.07.007
Nuutila, K., Katayama, S., Vuola, J., and Kankuri, E. (2014). Human wound-healing research: issues and perspectives for studies using wide-scale analytic platforms. Adv. Wound Care (New Rochelle) 3, 264–271. doi:10.1089/wound.2013.0502
Ogawa, Y., Kinoshita, M., Shimada, S., and Kawamura, T. (2018). Zinc and skin disorders. Nutrients 10, 199. doi:10.3390/nu10020199
Packer, C. F., Ali, S. A., and Manna, B. (2023). “Diabetic ulcer,” in StatPearls (Treasure Island (FL): StatPearls Publishing).
Paiva, L. A. F., De Alencar Cunha, K. M., Santos, F. A., Gramosa, N. V., Silveira, E. R., and Rao, V. S. N. (2002). Investigation on the wound healing activity of oleo-resin from Copaifera langsdorffi in rats. Phytotherapy Res. 16, 737–739. doi:10.1002/ptr.1049
Parente, L. M. L., Lino Júnior, R. D. S., Tresvenzol, L. M. F., Vinaud, M. C., De Paula, J. R., and Paulo, N. M. (2012). Wound healing and anti-inflammatory effect in animal models of Calendula officinalis L. Growing in Brazil. Evidence-Based Complementary Altern. Med. 2012, 1–7. doi:10.1155/2012/375671
Park, D. J., Han, S. K., and Kim, W. K. (2010). Is the foot elevation the optimal position for wound healing of a diabetic foot? J. Plast. Reconstr. Aesthet. Surg. 63, 561–564. doi:10.1016/j.bjps.2008.11.042
Park, J. W., Hwang, S. R., and Yoon, I.-S. (2017). Advanced growth factor delivery systems in wound management and skin regeneration. Molecules 22, 1259. doi:10.3390/molecules22081259
Passos, J. G. R., Gomes, J. A. S., Xavier-Santos, J. B., Yamashita, F. O., Cavalcanti-Cruz, J. V., Siqueira, E. M. S., et al. (2024). Anti-inflammatory, healing and antiophidic potential of Jatropha mollissima (Pohl) Baill. (Euphorbiaceae): from popular use to pharmaceutical formulation in gel. Biomed. and Pharmacother. 173, 116290. doi:10.1016/j.biopha.2024.116290
Parnell, L. K., and Volk, S. W. (2019). The evolution of animal models in wound healing research: 1993–2017. Advan. Wound. Care. 8 (12), 692–702. doi:10.1089/wound.2019.1098
Pchepiorka, R., Moreira, M. S., Lascane, N. A. D. S., Catalani, L. H., Allegrini Jr, S., De Lima, N. B., et al. (2020). Effect of ozone therapy on wound healing in the buccal mucosa of rats. Archives Oral Biol. 119, 104889. doi:10.1016/j.archoralbio.2020.104889
Pereira, L. D. P., Mota, M. R. L., Brizeno, L. A. C., Nogueira, F. C., Ferreira, E. G. M., Pereira, M. G., et al. (2016). Modulator effect of a polysaccharide-rich extract from Caesalpinia ferrea stem barks in rat cutaneous wound healing: role of TNF-α, IL-1β, NO, TGF-β. J. Ethnopharmacol. 187, 213–223. doi:10.1016/j.jep.2016.04.043
Pereira, L. O. M., Vilegas, W., Tangerina, M. M. P., Arunachalam, K., Balogun, S. O., Orlandi-Mattos, P. E., et al. (2018). Lafoensia pacari A. St.-Hil.: wound healing activity and mechanism of action of standardized hydroethanolic leaves extract. J. Ethnopharmacol. 219, 337–350. doi:10.1016/j.jep.2018.02.038
Perez, G. R. M., Vargas, S. R., and Ortiz, H. Y. D. (2005). Wound healing properties of Hylocereus undatus on diabetic rats. Phytotherapy Res. 19, 665–668. doi:10.1002/ptr.1724
Pérez-Contreras, C. V., Alvarado-Flores, J., Orona-Ortiz, A., Balderas-López, J. L., Salgado, R. M., Zacaula-Juárez, N., et al. (2022). Wound healing activity of the hydroalcoholic extract and the main metabolites of Amphipterygium adstringens (cuachalalate) in a rat excision model. J. Ethnopharmacol. 293, 115313. doi:10.1016/j.jep.2022.115313
Perez Gutierrez, R. M., and Vargas, S. R. (2006). Evaluation of the wound healing properties of Acalypha langiana in diabetic rats. Fitoterapia 77, 286–289. doi:10.1016/j.fitote.2006.03.011
Perez-Gutierrez, R. M., and Vargas-Solis, R. (2008). Wound healing properties of triterpenes from Buddleia scordioides in diabetic rats. Pharm. Biol. 46, 647–653. doi:10.1080/13880200802182471
Petkovic, M., Mouritzen, M. V., Mojsoska, B., and Jenssen, H. (2021). Immunomodulatory properties of host defence peptides in skin wound healing. Biomolecules 11 (7), 952. doi:10.3390/biom11070952
Piipponen, M., Li, D., and Landén, N. X. (2020). The immune functions of keratinocytes in skin wound healing. Int. J. Mol. Sci. 21 (22), 8790. doi:10.3390/ijms21228790
Pires, J., Cargnin, S. T., Costa, S. A., Sinhorin, V. D. G., Damazo, A. S., Sinhorin, A. P., et al. (2020). Healing of dermal wounds property of Caryocar brasiliense oil loaded polymeric lipid-core nanocapsules: formulation and in vivo evaluation. Eur. J. Pharm. Sci. 150, 105356. doi:10.1016/j.ejps.2020.105356
Pitz, H. D. S., Pereira, A., Blasius, M. B., Voytena, A. P. L., Affonso, R. C. L., Fanan, S., et al. (2016). In vitro evaluation of the antioxidant activity and wound healing properties of jaboticaba (Plinia peruviana) fruit peel hydroalcoholic extract. Oxidative Med. Cell. Longev. 2016, 3403586–6. doi:10.1155/2016/3403586
Polcz, M. E., and Barbul, A. (2019). The role of vitamin A in wound healing. Nut Clin Prac 34, 695–700. doi:10.1002/ncp.10376
Prado, L.G., Arruda, H. S., Peixoto Araujo, N. M., De Oliveira Braga, L. E., Banzato, T. P., Pereira, G. A., et al. (2020). Antioxidant, antiproliferative and healing properties of araticum (Annona crassiflora Mart.) peel and seed. Food Res. Int. 133, 109168. doi:10.1016/j.foodres.2020.109168
Quijas, S., Romero-Duque, L. P., Trilleras, J. M., Conti, G., Kolb, M., Brignone, E., et al. (2019). Linking biodiversity, ecosystem services, and beneficiaries of tropical dry forests of Latin America: review and new perspectives. Ecosyst. Serv. 36, 100909. doi:10.1016/j.ecoser.2019.100909
Rajendran, S. B., Challen, K., Wright, K. L., and Hardy, J. G. (2021). Electrical stimulation to enhance wound healing. JFB 12, 40. doi:10.3390/jfb12020040
Raven, P. H., Gereau, R. E., Phillipson, P. B., Chatelain, C., Jenkins, C. N., and Ulloa Ulloa, C. (2020). The distribution of biodiversity richness in the tropics. Sci. Adv. 6 (37), eabc6228. doi:10.1126/sciadv.abc6228
Rippon, M. G., Rogers, A. A., Ousey, K., Atkin, L., and Williams, K. (2022). The importance of periwound skin in wound healing: an overview of the evidence. J. wound care 31 (8), 648–659. doi:10.12968/jowc.2022.31.8.648
Robles-Tenorio, A., Lev-Tov, H., and Ocampo-Candiani, J. (2024). “Venous leg ulcer,” in StatPearls (Treasure Island (FL): StatPearls Publishing).
Rodrigues, M., Kosaric, N., Bonham, C. A., and Gurtner, G. C. (2019). Wound healing: a cellular perspective. Physiol. Rev. 99 (1), 665–706. doi:10.1152/physrev.00067.2017
Romana-Souza, B., Pires, T. C., and Monte-Alto-Costa, A. (2015). Mate tea-mediated reduction in catecholamine synthesis improves cutaneous wound healing of chronically stressed mice. Food Res. Int. 71, 32–40. doi:10.1016/j.foodres.2015.02.018
Romero-Cerecero, O., Zamilpa, A., Díaz-García, E. R., and Tortoriello, J. (2014). Pharmacological effect of Ageratina pichinchensis on wound healing in diabetic rats and genotoxicity evaluation. J. Ethnopharmacol. 156, 222–227. doi:10.1016/j.jep.2014.09.002
Romero-Cerecero, O., Zamilpa-Álvarez, A., Ramos-Mora, A., Alonso-Cortés, D., Jiménez-Ferrer, J., Huerta-Reyes, M., et al. (2011). Effect on the wound healing process and in vitro cell proliferation by the medicinal Mexican plant Ageratina pichinchensis. Planta Med. 77, 979–983. doi:10.1055/s-0030-1250743
Rodriguez, P. G., Felix, F. N., Woodley, D. T., and Shim, E. K. (2008). The role of oxygen in wound healing: a review of the literature. Dermatol. Surg. 34, 1159–1169. doi:10.1111/j.1524-4725.2008.34254.x
Sagai, M., and Bocci, V. (2011). Mechanisms of action involved in ozone therapy: is healing induced via a mild oxidative stress? Med. Gas. Res. 1, 29. doi:10.1186/2045-9912-1-29
Salas-Oropeza, J., Jimenez-Estrada, M., Perez-Torres, A., Castell-Rodriguez, A. E., Becerril-Millan, R., Rodriguez-Monroy, M. A., et al. (2020). Wound healing activity of the essential oil of Bursera morelensis, in mice. Molecules 25, 1795. doi:10.3390/molecules25081795
Salas-Oropeza, J., Jimenez-Estrada, M., Perez-Torres, A., Castell-Rodriguez, A. E., Becerril-Millan, R., Rodriguez-Monroy, M. A., et al. (2021). Wound healing activity of α-pinene and α-phellandrene. Molecules 26, 2488. doi:10.3390/molecules26092488
Sami, D. G., Heiba, H. H., and Abdellatif, A. (2019). Wound healing models: a systematic review of animal and non-animal models. Wound Med. 24, 8–17. doi:10.1016/j.wndm.2018.12.001
Santler, B., and Goerge, T. (2017). Chronic venous insufficiency - a review of pathophysiology, diagnosis, and treatment. J. Dtsch. Dermatol Ges. 15, 538–556. doi:10.1111/ddg.13242
Santos, G. J. L., Ferreira, T. C., Rodrigues, A. L. M., Freitas, J. C. C., Morais, S. M., Girão, V. C. C., et al. (2017). Involvement of mast cells, CD68+ and VEGF+ expressions in response to Himatanthus drasticus commercial latex in mice wound healing model. Arq. Bras. Med. Vet. Zootec. 69, 513–522. doi:10.1590/1678-4162-9163
Santos, J. A. A., Silva, J. W. D., Santos, S. M. D., Rodrigues, M. D. F., Silva, C. J. A., Da Silva, M. V., et al. (2020). In vitro and in vivo wound healing and anti-inflammatory activities of babassu oil (Attalea speciosa mart. Ex spreng., arecaceae). Evidence-Based Complementary Altern. Med. 2020, 1–10. doi:10.1155/2020/8858291
Sarandy, M. M., Gusmão, L. J., Purgato, G. A., Píccolo, M. S., Pinto Da Matta, S. L., Pizziolo, V. R., et al. (2022). Hydroalcoholic extract of Remijia ferruginea accelerates the closure of skin wounds by modulating tissue morphology and antioxidant profile: an in vitro and in vivo study. J. Ethnopharmacol. 296, 115464. doi:10.1016/j.jep.2022.115464
Sarandy, M. M., Novaes, R. D., Xavier, A. A., Vital, C. E., Leite, J. P. V., Melo, F. C. S. A., et al. (2017). Hydroethanolic extract of Strychnos pseudoquina accelerates skin wound healing by modulating the oxidative status and microstructural reorganization of scar tissue in experimental type I diabetes. BioMed Res. Int. 2017, 1–11. doi:10.1155/2017/9538351
Schencke, C., Vasconcellos, A., Sandoval, C., Torres, P., Acevedo, F., and Del Sol, M. (2016). Morphometric evaluation of wound healing in burns treated with Ulmo (Eucryphia cordifolia) honey alone and supplemented with ascorbic acid in Guinea pig (Cavia porcellus). Burns and Trauma 4, 25–z. doi:10.1186/s41038-016-0050-z
Schmidt, C., Fronza, M., Goettert, M., Geller, F., Luik, S., Flores, E. M. M., et al. (2009). Biological studies on Brazilian plants used in wound healing. J. Ethnopharmacol. 122, 523–532. doi:10.1016/j.jep.2009.01.022
Schmidt, C. A., Murillo, R., Bruhn, T., Bringmann, G., Goettert, M., Heinzmann, B., et al. (2010). Catechin derivatives from Parapiptadenia rigida with in vitro wound-healing properties. J. Nat. Prod. 73, 2035–2041. doi:10.1021/np100523s
Seo, Y.-K., Song, K.-Y., Kim, Y.-J., and Park, J.-K. (2007). Wound healing effect of acellular artificial dermis containing extracellular matrix secreted by human skin fibroblasts. Artif. Organs 31, 509–520. doi:10.1111/j.1525-1594.2007.00417.x
Serena, T., Lee, S. K., Lam, K., Attar, P., Meneses, P., and Ennis, W. (2009). The impact of noncontact, nonthermal, low-frequency ultrasound on bacterial counts in experimental and chronic wounds. Ostomy Wound Manage 55, 22–30.
Shady, N. H., Mostafa, N. M., Fayez, S., Abdel-Rahman, I. M., Maher, S. A., Zayed, A., et al. (2022). Mechanistic wound healing and antioxidant potential of Moringa oleifera seeds extract supported by metabolic profiling, in silico network design, molecular docking, and in vivo studies. Antioxidants 11, 1743. doi:10.3390/antiox11091743
Shekhter, A. B., Kabisov, R. K., Pekshev, A. V., Kozlov, N. P., and Perov, Yu.L. (1998). Experimental and clinical validation of plasmadynamic therapy of wounds with nitric oxide. Bull. Exp. Biol. Med. 126, 829–834. doi:10.1007/BF02446923
Shi, L., and Ronfard, V. (2013). Biochemical and biomechanical characterization of porcine small intestinal submucosa (SIS): a mini review. Int. J. Burns Trauma 3, 173–179.
Simões, D., Miguel, S. P., Ribeiro, M. P., Coutinho, P., Mendonça, A. G., and Correia, I. J. (2018). Recent advances on antimicrobial wound dressing: a review. Eur. J. Pharm. Biopharm. 127, 130–141. doi:10.1016/j.ejpb.2018.02.022
Singer, A. J., and Clark, R. A. F. (1999). Cutaneous wound healing. N. Engl. J. Med. 341, 738–746. doi:10.1056/NEJM199909023411006
Singh, S., Young, A., and McNaught, C. E. (2017). The physiology of wound healing. Surg. Oxf. 35 (9), 473–477. doi:10.1016/j.mpsur.2017.06.004
Smith, N. L., Wilson, A. L., Gandhi, J., Vatsia, S., and Khan, S. A. (2017). Ozone therapy: an overview of pharmacodynamics, current research, and clinical utility. Med. Gas. Res. 7, 212–219. doi:10.4103/2045-9912.215752
Sorg, H., and Sorg, C. G. (2023). Skin wound healing: of players, patterns, and processes. Eur. Surg. Res. 64 (2), 141–157. doi:10.1159/000528271
Speed, C. A. (2001). Therapeutic ultrasound in soft tissue lesions. Rheumatol. Oxf. 40, 1331–1336. doi:10.1093/rheumatology/40.12.1331
Steed, D. L. (1997). The role of growth factors in wound healing. Surg. Clin. North Am. 77, 575–586. doi:10.1016/s0039-6109(05)70569-7
Stubenitsky, B. M., Brasile, L., Rebellato, L. M., Hawinkels, H., Haisch, C., and Kon, M. (2009). Delayed skin allograft rejection following matrix membrane pretreatment. J. Plast. Reconstr. Aesthet. Surg. 62, 520–525. doi:10.1016/j.bjps.2007.12.001
Su, W.-H., Cheng, M.-H., Lee, W.-L., Tsou, T.-S., Chang, W.-H., Chen, C.-S., et al. (2010). Nonsteroidal anti-inflammatory drugs for wounds: pain relief or excessive scar formation? Mediat. Inflamm. 2010, 1–8. doi:10.1155/2010/413238
Sun, Y.-S. (2017). Electrical stimulation for wound-healing: simulation on the effect of electrode configurations. BioMed Res. Int. 2017, 1–9. doi:10.1155/2017/5289041
Timmers, M. S., Le Cessie, S., Banwell, P., and Jukema, G. N. (2005). The effects of varying degrees of pressure delivered by negative-pressure wound therapy on skin perfusion. Ann. Plastic Surg. 55, 665–671. doi:10.1097/01.sap.0000187182.90907.3d
Ubbink, D. T., Brölmann, F. E., Go, P. M. N. Y. H., and Vermeulen, H. (2015). Evidence-based care of acute wounds: a perspective. Adv. Wound Care (New Rochelle) 4, 286–294. doi:10.1089/wound.2014.0592
Vaisberg, A., Milla, M., Del Carmen Planas, M., Cordova, J., De Agusti, E., Ferreyra, R., et al. (1989). Taspine is the Cicatrizant Principle in Sangre de Grado Extracted from Croton lechleri. Planta Med. 55, 140–143. doi:10.1055/s-2006-961907
Van De Velde, F., Esposito, D., Grace, M. H., Pirovani, M. E., and Lila, M. A. (2019). Anti-inflammatory and wound healing properties of polyphenolic extracts from strawberry and blackberry fruits. Food Res. Int. 121, 453–462. doi:10.1016/j.foodres.2018.11.059
Ventura, A. C. S. S. B., De Paula, T., Gonçalves, J. P., Soley, B. D. S., Cretella, A. B. M., Otuki, M. F., et al. (2021). The oil from Moringa oleifera seeds accelerates chronic skin wound healing. Phytomedicine Plus 1, 100099. doi:10.1016/j.phyplu.2021.100099
Vílchez Cáceda, H. A., Inocente Camones, M. A., and Flores López, O. B. (2020). Actividad cicatrizante de seis extractos hidroalcohólicos de plantas en heridas incisas de Rattus norvegicus albinus. Cub Med. Mil. 49.
Villegas, L. F., Fernández, I. D., Maldonado, H., Torres, R., Zavaleta, A., Vaisberg, A. J., et al. (1997). Evaluation of the wound-healing activity of selected traditional medicinal plants from Perú. J. Ethnopharmacol. 55, 193–200. doi:10.1016/S0378-8741(96)01500-0
Villegas, L. F., Marçalo, A., Martin, J., Fernández, I. D., Maldonado, H., Vaisberg, A. J., et al. (2001). (+)- ep i-α-Bisbolol is the wound-healing principle of peperomia galioides: investigation of the in vivo wound-healing activity of related terpenoids. J. Nat. Prod. 64, 1357–1359. doi:10.1021/np0102859
Vittorazzi, C., Endringer, D. C., Andrade, T. U. D., Scherer, R., and Fronza, M. (2016). Antioxidant, antimicrobial and wound healing properties of Struthanthus vulgaris. Pharm. Biol. 54, 331–337. doi:10.3109/13880209.2015.1040515
Vivcharenko, V., Wojcik, M., Palka, K., and Przekora, A. (2021). Highly porous and superabsorbent biomaterial made of marine-derived polysaccharides and ascorbic acid as an optimal dressing for exuding wound management. Materials 14, 1211. doi:10.3390/ma14051211
Wagner, A. E., Huck, G., Stiehl, D. P., Jelkmann, W., and Hellwig-Bürgel, T. (2008). Dexamethasone impairs hypoxia-inducible factor-1 function. Biochem. Biophys. Res. Commun. 372, 336–340. doi:10.1016/j.bbrc.2008.05.061
Wang, Y., Huang, Y.-Y., Wang, Y., Lyu, P., and Hamblin, M. R. (2017). Photobiomodulation of human adipose-derived stem cells using 810nm and 980nm lasers operates via different mechanisms of action. Biochim. Biophys. Acta Gen. Subj. 1861, 441–449. doi:10.1016/j.bbagen.2016.10.008
Wilhelm, K. P., Wilhelm, D., and Bielfeldt, S. (2017). Models of wound healing: an emphasis on clinical studies. Skin Res. Technol. 23 (1), 3–12. doi:10.1111/srt.12317
World Health Organization (2024). Traditional medicine. Available at: https://www.who.int/news-room/questions-and-answers/item/traditional-medicine.
Xavier-Santos, J. B., Passos, J. G. R., Gomes, J. A. S., Cruz, J. V. C., Alves, J. S. F., Garcia, V. B., et al. (2022). Topical gel containing phenolic-rich extract from Ipomoea pes-capre leaf (Convolvulaceae) has anti-inflammatory, wound healing, and antiophidic properties. Biomed. and Pharmacother. 149, 112921. doi:10.1016/j.biopha.2022.112921
Ximenes, R. M., De Morais Nogueira, L., Cassundé, N. M. R., Jorge, R. J. B., Dos Santos, S. M., Magalhães, L. P. M., et al. (2013). Antinociceptive and wound healing activities of Croton adamantinus Müll. Arg. essential oil. J. Nat. Med. 67, 758–764. doi:10.1007/s11418-012-0740-1
Xu, Z., Han, S., Gu, Z., and Wu, J. (2020). Advances and impact of antioxidant hydrogel in chronic wound healing. Adv. Healthc. Mater. 9, 1901502. doi:10.1002/adhm.201901502
Yamamoto, T., Iwase, H., King, T. W., Hara, H., and Cooper, D. K. C. (2018). Skin xenotransplantation: historical review and clinical potential. Burns 44, 1738–1749. doi:10.1016/j.burns.2018.02.029
Yang, F., Bai, X., Dai, X., and Li, Y. (2021). The biological processes during wound healing. Regen. Med. 16 (4), 373–390. doi:10.2217/rme-2020-0066
You, H.-J., and Han, S.-K. (2014). Cell therapy for wound healing. J. Korean Med. Sci. 29, 311–319. doi:10.3346/jkms.2014.29.3.311
Yousefian, F., Hesari, R., Jensen, T., Obagi, S., Rgeai, A., Damiani, G., et al. (2023). Antimicrobial wound dressings: a concise review for clinicians. Antibiot. (Basel) 12, 1434. doi:10.3390/antibiotics12091434
Zamani, A. R. N., Saberianpour, S., Geranmayeh, M. H., Bani, F., Haghighi, L., and Rahbarghazi, R. (2020). Modulatory effect of photobiomodulation on stem cell epigenetic memory: a highlight on differentiation capacity. Lasers Med. Sci. 35, 299–306. doi:10.1007/s10103-019-02873-7
Zhao, M. (2009). Electrical fields in wound healing-An overriding signal that directs cell migration. Semin. Cell Dev. Biol. 20, 674–682. doi:10.1016/j.semcdb.2008.12.009
Keywords: wound healing, medicinal plants, biological assays, natural products, biodiversity
Citation: Sánchez-Ramos M, Ruiz-Betancourt A, Tadeo-Cuenca SA, Román-Guerrero A, Columba-Palomares MC, Guerrero-Alonso A, Bernabé-Antonio A, Ojeda-Ramírez D and Cruz-Sosa F (2025) The role of Latin America medicinal plants in wound healing. Front. Chem. Eng. 6:1514962. doi: 10.3389/fceng.2024.1514962
Received: 21 October 2024; Accepted: 16 December 2024;
Published: 13 January 2025.
Edited by:
Mohammad Tavakkoli Yaraki, Macquarie University, AustraliaReviewed by:
Hadida Yasmin, Cooch Behar Panchanan Barma University, IndiaCopyright © 2025 Sánchez-Ramos, Ruiz-Betancourt, Tadeo-Cuenca, Román-Guerrero, Columba-Palomares, Guerrero-Alonso, Bernabé-Antonio, Ojeda-Ramírez and Cruz-Sosa. This is an open-access article distributed under the terms of the Creative Commons Attribution License (CC BY). The use, distribution or reproduction in other forums is permitted, provided the original author(s) and the copyright owner(s) are credited and that the original publication in this journal is cited, in accordance with accepted academic practice. No use, distribution or reproduction is permitted which does not comply with these terms.
*Correspondence: Mariana Sánchez-Ramos, marianasan_06@xanum.uam.mx; Francisco Cruz-Sosa, cuhp@xanum.uam.mx
Disclaimer: All claims expressed in this article are solely those of the authors and do not necessarily represent those of their affiliated organizations, or those of the publisher, the editors and the reviewers. Any product that may be evaluated in this article or claim that may be made by its manufacturer is not guaranteed or endorsed by the publisher.
Research integrity at Frontiers
Learn more about the work of our research integrity team to safeguard the quality of each article we publish.