- GICOM Research Group, Department of Chemical, Biological and Environmental Engineering, School of Engineering, Edifici Q, Universitat Autònoma de Barcelona, Barcelona, Spain
Biorefineries are emerging facilities that are planned to transform existing waste treatment plants based on composting and/or anaerobic digestion into complex installations where biological and physicochemical processes coexist to maximize the recovery of renewable energy and valuable bioproducts. Biorefineries are mainly based on the use of several typologies of organic waste as starting material. This feature, together with the fact that biorefineries are supposed to be carbon neutral, has provoked that two biotechnologies arise as the key to have a successful biorefinery in economic and environmental terms: anaerobic digestion, a consolidated renewable energy provider, and solid-state fermentation, an emerging technology, derived from composting, to act as a supplier of a new generation of biorefinery products that include enzymes, bioplastics, biopesticides, biosurfactants and bioestimulants, among others. This review explores the importance of the role of anaerobic digestion and solid-state fermentation in novel biorefineries, highlighting their obvious advantages and their main challenges, in a specific moment where anaerobic digestion and, in consequence, digestate, are undergoing an exponential increase as a renewable energy source and a predominant organic waste.
Highlights
• Biorefineries are emerging new facilities to use organic waste as feedstock.
• The main resulting products from biorefineries are renewable energy and biomaterials.
• Anaerobic digestion is the main actor in biorefineries to provide renewable energy.
• Solid-state fermentation is one of the main emerging technologies to obtain bioproducts.
• Both technologies have advantages and challenges to overcome.
1 Introduction
In response to the classical linear economic procedures, which have eventually led to an emergence related to critical environmental issues such as global warming and climate change, new strategies are being developed to break this linearity in favour of circular approaches by reducing the dependence on fossil fuels and introducing new ways of economic development. The objective of these novel paradigm is to implement sustainable production processes and development, according to the Sustainable Development Goals (SDG) promoted by the United Nations (United Nations, 2017). Some of these strategies include Circular Economy as a trend specifically designed to increase process sustainability.
In this framework, biorefineries are installations for the design of products and processes that reduce or eliminate the generation of substances that are hazardous for humans and/or the environment, replacing them by biomaterials (US Environmental Protection Agency, 2024). Biorefineries’ performance must be analysed across the life cycle of any obtained bioproduct (design, manufacture, use and disposal) to be really sustainable; therefore, Life Cycle Assessment (LCA) is an essential tool for their evaluation in terms of environmental impact. On the other hand, Circular Economy draws from concepts derived from Industrial Ecology, Industrial Symbiosis and Smart cities. Circular Economy is defined by the European Commission as: “A model of production and consumption, which involves sharing, leasing, reusing, repairing, refurbishing and recycling existing materials and products as long as possible”. In this way, the life cycle of products is extended (European Union, 2015), although other definitions can be found (Ellen MacArthur Foundation, 2024). Nevertheless, in all cases, Circular Economy is focused on the recovery of what is now considered waste to increase sustainability, developing a novel economy based on the paradigm of materials circularity within the production circle, avoiding the increasing generation of waste.
An important point when considering the environmental sustainability of new configurations of biorefineries is their analysis using powerful tools as Life Cycle Assessment (LCA). Although its application to complex biorefineries is still in an incipient point, several examples can be already found in literature. For instance, Gaffey et al. (2024) presents an excellent review of 59 preliminary LCA performed in different biorefineries. Since some of them are quite simple, it is evident that the amount of data necessary to perform rigorous analysis in more complex installations is still not available. Being some of these complex biorefineries in the designing stage, to obtain reliable and consistent conclusions about the environmental benefits of them will be one of the main lacks to cover in future research (Martínez-Arce et al., 2024).
The use of renewable materials and biodegradable products, the energy efficiency and the use of renewable sources of energy, coupled with pollution prevention, are some of the principles that Circular Economy and biomaterials have in common. In this context, the concept of biorefinery appears as a new installation including several processes to maximise energy and biomaterials recovery. A biorefinery is defined as “an industrial facility (or a network of facilities) that utilizes biomass as raw material for the sustainable production of a wide range of marketable products and energy” (Cherubini, 2010). More specifically, if energy and bioproducts are simultaneously produced, biorefineries achieve a high level of sustainability (Molina-Peñate et al., 2022). Additionally, it is noteworthy to note that biorefineries also present an excellent opportunity to recycle waste and by-products from industrial processes, which gives biorefineries the possibility of using locally available materials, being this point another important advantage. This review highlights the importance of two technologies for organic waste-based biorefineries: on one hand, the consolidation of anaerobic digestion (AD) as a provider of renewable energy and, on the other hand, the emerging role of solid-state fermentation (SSF) as a novel strategy to obtain a wide variety of bioproducts of added-value from organic waste and side products. Besides, in second term, AD is as a producer of digestate, an organic material that can be further processed in the biorefinery. The exponential presence that AD (European Biogas Association, 2022) will have in the overall picture of renewable energy, as a source of locally available raw materials, will result in an increasing amount of digestate, being an excellent starting material for SSF in the production of new biomaterials. Therefore, the success in the complementarity of both biotechnologies is a critical factor for the development of a future generation of biorefineries, which maximize the production of renewable energy and bioproducts substituting its chemically-produced twins.
2 Classification of biorefineries
Biorefineries use organic waste and biomass as input material. The heterogeneity of these materials according to its seasonal (quality and quantity) and geographical variations and source diversity, makes impossible to have a unique classification of biorefineries. This fact, jointly with the continuous evolution of biorefinery definition, has resulted in a classification that is periodically reviewed. The International Energy Agency proposes four categories to classify biorefineries (Annevelink et al., 2022):
1) Related to feedstock: it can be classified as primary biomass and secondary biomass. Primary biomass corresponds to feedstock from agriculture, marine, forestry and nature management. Secondary biomass corresponds to the residues produced from the use of primary biomass. Table 1 presents the main subcategories included in primary and secondary biomass, according to this classification. It is important to clarify that biorefineries can work on these two different types of biomass, being primary biomass raw materials whereas secondary biomass are biomass-derived waste (Lago et al., 2019; Anvari et al., 2024). Considering these different types, it is also worthwhile to mention that the technologies used in the corresponding biorefineries should be adapted for a proper performance.
2) Related to the conversion processes: probably the most relevant classification in technological terms. It refers to the transformations of input materials, which can include one or more of the following processes (Figure 1): biochemical (aerobic conversion, anaerobic digestion, enzymatic process, fermentation and insect-based bioconversion), chemical (catalytic esterification, hydrogenation, hydrolysis, methanation, chemical pulping, steam reforming, water electrolysis and water gas shift), mechanical and thermomechanical (blending extraction, mechanical and thermomechanical disruption and fractionation, mechanical pulping and separation processes), or thermochemical (combustion, gasification, hydrothermal liquefaction, pyrolysis, supercritical conversion, torrefaction and carbonization) (Annevelink et al., 2022). In this sense, it is important to consider that all these conversion processes require energy and, sometimes, auxiliary materials. In consequence, Circular Economy principles must be applied to increase the sustainability of the entire biorefinery process, which gives AD a critical importance (Font and Sánchez, 2021).
3) Related to the platforms: the term platform chemicals is used to design those intermediates that are subsequently transformed into various other higher value-added products. Several materials have been proposed as platform chemicals, although this list is again continuously updated, it includes: biochar, bio-coal, bio-crude, biogas, bio-oils, bio-hydrogen, bio-Naphtha, C5/C6 sugars, carbon dioxide, lignin, oils, organic fibres, organic juice, protein, pyrolytic liquid, starch, syngas, and others (Takkellapati et al., 2018).
4) Related to the final products: as biorefinery outputs include marketable products and energy, they categorize biorefineries as producers of chemicals, energy products, material products, food and animal feed, among others.
As a result of this classification, lignocellulosic materials are the main feedstock, sugars are the dominant platform, and bioethanol is the main targeted product, although this situation is quickly evolving in accordance to bioproducts that are more interesting, especially those coming from SSF (Oiza et al., 2022).
3 Key technologies in biorefineries
3.1 AD as a source of renewable energy
AD is a series of biological processes in which microorganisms break down biodegradable material in the absence of oxygen. The products of this process are biogas, mainly composed of methane and carbon digestate, liquid digestate and solid digestate (Lora-Granado et al., 2017). AD is a well-known process, implemented at full-scale from decades in a large number of facilities for the treatment of a wide variety of organic waste and mixtures (co-digestion), in which the main objective is the production of biogas as a source of renewable energy (Esposito et al., 2012).
In the context of modern biorefineries, anaerobic digestion has a main role: the transformation of substrates and the production of biogas as energy source. In essence, AD is a key technology to decrease the carbon footprint of any biorefinery, and it is the responsible for having a positive energy balance because of the production of biogas (Hagman et al., 2018). Due to the versatility of biogas, anaerobic digestion is an essential key technology for Circular Bioeconomy, by reducing energy dependence, promoting organic waste circularity and increasing local economy (Font and Sánchez, 2021). The relationship between biogas and biorefineries indicates that several specific added values can result from this association, thus making the system more resilient and versatile.
As commented, biogas plays an important role in the configuration in most of biorefinery schemes. Actually, biogas is the main source of energy to cover the needs of these installations. In this sense, biorefineries rely on anaerobic digestion to be feasible from the technical, environmental and economic point of view. Recently, some examples can be found in which heat is also used to cover some external energy needs as district heating schemes (Weinand et al., 2019; Millati et al., 2023).
As a source of renewable energy for the biorefinery, biogas is typically pre-treated to remove some pollutants such as hydrogen sulphide or siloxanes (Vali et al., 2023) and burned on-site through co-generation devices to produce two types of energy: electricity and heat, which can be internally or externally used (Picardo et al., 2019). More recently, several technologies are being studied to transform the carbon dioxide contained in biogas to methane with the use of green hydrogen, to have a highly concentrated methane that can be compressed and pumped to the existing gas natural grids. This is the case of biogas upgrading, using membranes or biological processes as biomethanation (Paniagua et al., 2022; Gkotsis et al., 2023).
Besides, biogas, can be used as raw material to obtain valuable products instead of energy. Indeed, it has been demonstrated, up to pilot scale, the bioconversion of the CH4 present in biogas into bulk commodities such as polyhydroxybutyrate (PHA), methanol, single cell protein or ectoine (Pérez et al., 2022).
In summary, the role of AD in biorefineries and, by extension, in Circular Bioeconomy is crucial in terms of technical, economic and environmental performance. The flexibility of AD to treat a multitude of organic feedstock, while producing renewable energy, make it an essential biotechnology for a large number of biorefinery typologies and configurations (Fagerström et al., 2018).
3.2 SSF as a source of bioproducts
3.2.1 Solid-state vs. submerged fermentation
The classical scientific definition of SSF was presented by Pandey (2003) as: “the fermentation involving solids in absence (or near absence) of free water; however, substrate must possess enough moisture to support growth and metabolism of microorganisms”. However, in practical terms, a new definition including the paradigm of Circular Bioeconomy can be also considered: “The biological solid-state aerobic transformation of organic biodegradable waste into bioproducts that permits the substitution of raw sources of materials and energy” (Oiza et al., 2022). In both cases, it is evident that SSF and SmF diverge in critical points, which in some cases may be considered advantages and disadvantages depending on the bioproduct to be obtained, and its formulation. In fact, formulation is of especial relevance with bioproducts that need complex down-stream processes such as biosurfactants or enzymes to be further commercialized (Al-Ghamdi et al., 2022; Amador Morilla et al., 2023). Table 2 summarizes some of these items according to some of the most significant works published on SSF in the literature treating the challenges of this biotechnology (Pandey, 2003; Mitchell et al., 2006; Casciatori and Thomeo, 2018; Oiza et al., 2022). In the current scenario, probably the use of organic waste and the minimal water requirements are the main advantages of SSF, while mass and heat transfer are its main challenges when comparing SSF and SmF (Oiza et al., 2022). Nevertheless, an important point often neglected in both fermentation typologies is down-stream. This is an evident flaw in research, as sometimes this part is neither assessed nor discussed and it can suppose more than half of the costs and environmental impacts of the global process, which is highly relevant when biorefineries are designed (Catalán and Sánchez, 2020).
3.2.2 Main features of SSF
Among bio-based technologies, SSF plays an important role, and feedstock properties and composition are key parameters for the process performance. In fact, the solid matrix can have two different roles in SSF: it can be the source of nutrients for the target microorganisms or an inert material with a proper structure to support the growth of microorganisms, which retains a solution containing the required nutrients.
Regarding microorganisms, several strategies are possible and its application depends on specific technical and logistic issues. In some cases, a consortium of microorganisms can be used, which can be autochthonous or externally enriched (Krishna, 2005). However, SSF is mainly conducted by a specific strain selected and inoculated according to the desired product, under sterile or near-to sterile conditions (Sánchez, 2022). Down-stream can be also a critical point in SSF. Therefore, the extraction of some bioproducts from the solid matrix can be considerably complex, and it usually contributes to process cost and environmental impact to a large extent (Catalán et al., 2019). This step can be avoided in some cases where the bioproduct can be used embedded in the solid matrix. Figure 2 presents the main inputs and outputs of the SSF process. Factors influencing the process and the main types of SSF reactors are also included, being packed-bed reactors the most commonly used (Mitchell et al., 2023).
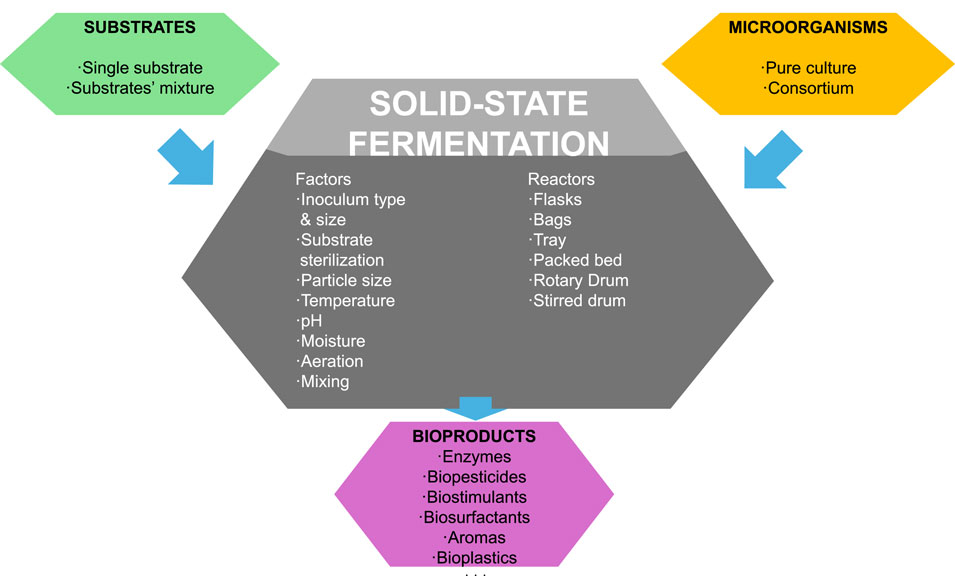
Figure 2. Solid-state fermentation: influencing factors, bioreactor typologies, organic substrates and microorganisms.
3.2.3 Substrates, microorganisms and bioproducts
Microorganisms (including bacteria, yeast and fungi) and organic substrates are the most important factors to consider in the SSF process (Soccol et al., 2017). In the case of the target bioproduct it is especially relevant to determine the process economic viability and environmental performance.
Among the different microorganisms used in SSF, filamentous fungi have gained relevance as solid substrates reproduce their natural habitat (Gowthaman et al., 2001). Nevertheless, yeasts and some species of bacteria have been also successfully cultivated in solid matrices. Regarding substrates, probably the most commonly used are agricultural, agro-industrial, forestry biomass and food-processing waste. Similarly to other biotechnologies as composting and anaerobic digestion, different typologies of waste can be combined to balance nutrients or to fix the lacks that a single residual material may present. The porosity of the solid matrix is also a critical property in SSF as it governs the oxygen transfer and it often results in the requirement of additional bulking agents (Sala et al., 2021). Table 3 summarizes some very recent representative examples of SSF processes and their role in selected biorefineries. This table must be considered a snapshot of a specific time, since the variety of bioproducts obtained through SSF is continually increasing (Cuadrado-Osorio et al., 2022; Oiza et al., 2022; Prabhu et al., 2022).
From Table 3 and other existing literature, some conclusions can be summarized regarding the incipient role of SSF in recently designed biorefineries:
a) There is a clear absence of full-scale studies, being this one of the main challenges for SSF implementation, as commented later.
b) SSF works are focused on the process itself, with a high level of detail in the fermentation conditions, strain screening and optimization. However, the integration of SSF in the entire biorefinery scheme is usually commented from a theoretical perspective.
c) SSF down-stream to obtain the bioproduct of interest is rarely considered. This is an important gap in scientific literature, although the complexity of this process is highly dependent on the nature of the obtained bioproduct and its potential use.
d) Biorefineries are supposed to be environmentally friendly installations, but this is only occasionally studied using accepted tools as Life Cycle Assessment. Their carbon footprint, which should be decreased with the use of AD is only theoretically considered (Gnansounou and Pandey, 2017).
e) Similarly, only few studies have some kind of economic assessment. This is surprising in the sense that biorefineries are closely related to Circular Economy.
f) A large number of studies are reviews or perspective papers. Although useful and somewhat convincing, they confirm the need of experimental data to develop realistic process, with their advantages and disadvantages.
g) Biofuels and enzymes are still the most studied products to involve SSF in a biorefinery scheme, typically for the hydrolysis of lignocellulosic waste leading to fermentable sugars.
3.2.4 SSF challenges
Heat and mass transfer limitations are probably the most important challenges that SSF is currently facing for its successful implementation in full-scale biorefineries, being this a well know problem from the very beginning of SSF, when practically its unique application was in the food industry (Lonsane et al., 1992). Since then, several strategies have been presented in scientific literature to overcome this problem.
Bioreactors design is one of the most commonly used approaches to move SSF towards the scale of few kilograms. Accordingly, several novel reactor configurations have been proposed (Mitchell et al., 2006) to overcome typical problems of SSF such as temperature increase due to an inefficient heat and mass transfer in solid matrices (Casciatori and Thomeo, 2018). Among these configurations, probably tray reactors are gaining attention as they perform properly with fungi (Sentís-Moré et al., 2023). Tray reactors present improved heat and mass transfer when compared to typical packed-bed reactors (Mitchell et al., 2023). However, their implementation at full-scale is scarcely reported (Sala et al., 2022), at least when considering scientific publications. In the case of patents, some of them can be found, with different approaches to overcome some problems related to SSF (Lüth and Eiben, 2003; Vitanen et al., 2011; Ranganathan, 2014; Andersen et al., 2015; Granucci and Granato Billas Boas, 2019; Bobbiesi, 2019). Unfortunately, it is not possible to know if these patents have been transformed into real industrial SSF bioreactors. Another potential indirect strategy to increase the production without changing the reactor volume is the use of sequential batch reactors. In this case, positive results have been obtained in the production of biopesticides, aromas and enzymes through SSF at pilot scale (Cerda et al., 2017; Martínez et al., 2018; Sala et al., 2021). However, the integration of this strategy in a full-scale biorefinery seems again complicated, as it should coexist with other purely continuous process such as anaerobic digestion. In close relationship with all the problems related to SSF scale-up is the absence of robust mathematical models that permit the simulation and prediction of processes at any scale. In this sense, some attempts have been published with the use of simplified models (Casciatori et al., 2016; Fonseca et al., 2017), but the complexity of SSF is enough to consider other approaches such as data driven modelling, which already has been applied in solid-state biological processes such as composting (Aidyn Temel et al., 2023; Gupta et al., 2024).
However, and despite these efforts, it is evident that scale-up is still the main problem to solve in SSF, especially when coupled with other technologies in complex biorefinery schemes.
Although less studied, another potential main challenge of SSF regarding its implementation in biorefineries is the down-stream and purification of the target bioproduct once the biological process is considered finished. This point presents a significant difference with SmF, as the time when the maximum concentration is reached in the SSF of some bioproducts does not coincide with the fermentation end point, that is, the point at which substrate is completely depleted. For instance, this is the case of the SSF production of biopesticides and biostimulants (Rodríguez et al., 2019; Ghoreishi et al., 2023). This fact can result in complex multi-step down-stream processes, whose cost can reach up to 70% of the entire SSF process cost (Catalán et al., 2019). In some cases, the extraction of some products, such as biosurfactants, can involve the use of organic environmentally unfriendly solvents (Dolman et al., 2019), which is against the biorefinery concept.
This notwithstanding, in some applications, the fermented exhaust solid can be directly used as the final product, thus avoiding expensive and highly environmental impact down-stream and purification process. There are some excellent examples in which this situation occurs, as the process of biodiesel synthesis using lipases produced through the SSF process of organic waste rich in fats or oils (Aguieiras et al., 2018).
4 Composting and SSF in zero-waste biorefineries
One of the main objectives of modern biorefineries is to have a zero-waste process. Achieving this goal has large benefits in technical, environmental and economic terms (Sachdeva et al., 2022). In this framework, composting is a suitable technology as it permits a wide variety of raw materials to end up in an organic amendment. It is important to note that, sometimes, it is forgotten that composting was one of the first approaches to circular bioeconomy and this role remains intact (European Compost Network, 2024). Recently, composting has gain more interest when complemented with AD and SSF. Both processes result in organic exhaust material that is neither stable nor mature for some soil applications or for most of the horticultural uses. In this case, composting seems the most adequate strategy, especially for SSF exhaust matrices and the solid fraction of digestate.
In case of AD digested materials, composting has been largely studied to transform digestate into compost with enough stability (Colón et al., 2017). Besides, other advantages of composting have been highlighted to obtain an improved organic amendment with a better environmental performance: nutrient mineralization and humification, decrease of ammonia emissions and, obviously, a considerable shortening in the composting process and a decrease of aeration requirements (Chen et al., 2023; Czekała et al., 2023).
In the case of SSF, its complementarity with AD is beyond energy or compost production. However, the research on the use of exhaust solid waste after obtaining the product of interest is much scarcer. Anyway, two main trends can be observed in recent literature. On one hand, if the bioproduct has application to soil, SSF and composting simultaneously occur and the resulting product is stable enough, as in the case of some biopesticides and biostimulants (Ballardo et al., 2017; Ghoreishi et al., 2023). On the other hand, when a product is extracted from the fermented solid, composting can be a proper technology to obtain a compost-like product, to finally reach a zero waste process. In other cases, digestate can act as raw material to produce hydrolytic enzymes or biosurfactants from organic waste through SSF (Marín et al., 2018; Cerda et al., 2019). Some of these bioproducts can also act as additives to improve AD biogas yields, which is a novel concept of biorefinery that maximises the energy production without using external materials such as biochar or nutrients (Romero-Güiza et al., 2016; Parra-Orobio et al., 2023). For instance, in the case of biosurfactants obtained from municipal solid waste through SSF, the strategy is highly beneficial, as some of these bioproducts are reported to enhance the production of biogas in AD, as the case of sophorolipids (Xu et al., 2019; Oiza et al., 2024). In the case of certain enzymes, they are also reported to act as enzymatic cocktails to shorten the hydrolytic limiting step of AD when treating complex substrates as the source-selected organic fraction of municipal solid waste (Martínez-Valdez et al., 2017).
5 Conclusion
Biorefineries are called to be advanced substitutes for waste and wastewater treatment plants, in a context where Circular Economy paradigms may be implemented to the detriment of linear strategies, which result in poor recovery of energy and material resources and a high generation of waste to be disposed of. In this framework, modern biorefineries should implement novel biotechnologies, such as SSF, which is in line with the basic principle of circularity: transform waste into raw and profitable materials. SSF needs research and reliable strategies of scaling-up to be a reality in the biorefineries of the next future, to take profit of its huge potential. However, the role of traditional technologies as AD or composting must not be diminished. Particularly, AD will be the main process to provide renewable energy to these facilities, while reducing their carbon footprint. Composting, as a way to produce a stable organic amendment for soil application, must be considered complementary to AD and SSF, with a high relevance in achieving zero-waste processes. In any case, it is evident that these new configurations of biorefineries should be environmentally evaluated with specific tools such as LCA. In summary, the exponential increase of AD (and inherently digestate) will result in a considerable change in the waste management overall picture, which will place AD, SSF and its complementarity in a key position regarding the success of future organic waste-based biorefineries, which should be optimized in terms of renewable energy generation and the variety of bioproducts that may be substitutes of their chemical alternatives.
Author contributions
AA: Writing–review and editing, Investigation, Conceptualization. XF: Writing–review and editing, Validation, Investigation, Conceptualization. JM-V: Writing–review and editing, Validation, Investigation, Conceptualization. AS: Writing–review and editing, Writing - original draft, Validation, Supervision, Investigation, Conceptualization.
Funding
The author(s) declare that financial support was received for the research, authorship, and/or publication of this article. This research was funded by the Spanish Ministerio de Ciencia, Innovación y Universidades, grant number PID 2023-146978OB-I00 (Project Solstice).
Conflict of interest
The authors declare that the research was conducted in the absence of any commercial or financial relationships that could be construed as a potential conflict of interest.
The author(s) declared that they were an editorial board member of Frontiers, at the time of submission. This had no impact on the peer review process and the final decision.
Publisher’s note
All claims expressed in this article are solely those of the authors and do not necessarily represent those of their affiliated organizations, or those of the publisher, the editors and the reviewers. Any product that may be evaluated in this article, or claim that may be made by its manufacturer, is not guaranteed or endorsed by the publisher.
References
Aguieiras, E. C. G., Cavalcanti-Oliveira, E. D., Cammarota, M. C., and Freire, D. M. G. (2018). “Chapter 8 - solid-state fermentation for the production of lipases for environmental and biodiesel applications,” in Current developments in biotechnology and bioengineering. Editors A. Pandey, C. Larroche, and C. R. Soccol (Netherlands: Elsevier), 123–168. doi:10.1016/B978-0-444-63990-5.00008-6
Aguieiras, E. C. G., Greco-Duarte, J., Pires de Souza, C., Oliveira, R. A., de, O., Pinto, T., et al. (2024). Integrated process for a new sequential valorization of corn ethanol production by-products: production of fermented solid with lipase activity, ethyl esters, and animal feed. Ind. Crop. Prod. 209, 118028. doi:10.1016/j.indcrop.2024.118028
Al-Ghamdi, A., Haq, B., Al-Shehri, D., Muhammed, N. S., and Mahmoud, M. (2022). Surfactant formulation for green enhanced oil recovery. Energy Rep. 8, 7800–7813. doi:10.1016/j.egyr.2022.05.293
Amador Morilla, E., Mutti Stegmann, P., and Tubio, G. (2023). Enzymatic cocktail production by a co-cultivation Solid-State Fermentation for detergent formulation. Food Bioprod. process. 140, 110–121. doi:10.1016/j.fbp.2023.05.001
Andersen, C., Korsholm, L., Rezaei, F., Chang, F., and Saadat, A. P. (2015). Solid state bioreactor adapted for automation. Bagsværd, Denmark: Assigned to Novozymes Bioag A/S. US patent 20150166945.
Annevelink, B., Garcia Chavez, L., van Ree, R., and Vural Gursel, I. (2022). IEA Bioenergy, Global biorefinery status report 2022. Available at: https://www.ieabioenergy.com/wp-content/uploads/2022/09/IEA-Bioenergy-Task-42-Global-biorefinery-status-report-2022-220712.pdf (Accessed June, 2024).
Anvari, S., Aguado, R., Jurado, F., Fendri, M., Zaier, H., Larbi, A., et al. (2024). Analysis of agricultural waste/byproduct biomass potential for bioenergy: the case of Tunisia. Energy sustain. Dev. 78, 101367. doi:10.1016/j.esd.2023.101367
Aydin Temel, F., Cagcag Yolcu, O., and Gamze Turan, N. (2023). Artificial intelligence and machine learning approaches in composting process: a review. Bioresour. Technol. 370, 128539. doi:10.1016/j.biortech.2022.128539
Ballardo, C., Barrena, R., Artola, A., and Sánchez, A. (2017). A novel strategy for producing compost with enhanced biopesticide properties through solid-state fermentation of biowaste and inoculation with Bacillus thuringiensis. Waste Manage 70, 53–58. doi:10.1016/j.wasman.2017.09.041
Bobbiesi, G. (2019). Dynamic solid-state fermentation apparatus. Milano, Italy: Assigned to Gibob S.R.L. European patent EP3561046A1.
Bühlmann, C. H., Mickan Bede, S., Tait, T., Batstone, D. J., and Bahri Parisa, A. (2023). Lactic acid production from food waste at an anaerobic digestion biorefinery: effect of digestate recirculation and sucrose supplementation. Front. Bioeng. Biotechnol. 11, 1177739. doi:10.3389/fbioe.2023.1177739
Bulgari, D., Renzetti, S., Messgo-Moumene, S., Monti, E., and Gobbi, E. (2023). Optimization of esterase production in solid-state fermentation of agricultural digestate. Fermentation 9, 524. doi:10.3390/fermentation9060524
Casciatori, F. P., Bück, A., Thoméo, J. C., and Tsotsas, E. (2016). Two-phase and two-dimensional model describing heat and water transfer during solid-state fermentation within a packed-bed bioreactor. Chem. Eng. J. 287, 103–116. doi:10.1016/j.cej.2015.10.108
Casciatori, F. P., and Thomeo, J. C. (2018). Heat transfer in packed-beds of agricultural waste with low rates of air flow applicable to solid-state fermentation. Chem. Eng. Sci. 188, 97–111. doi:10.1016/j.ces.2018.05.024
Catalán, E., Komilis, D., and Sánchez, A. (2019). Environmental impact of cellulase production from coffee husks by solid-state fermentation: a life-cycle assessment. J. Clean. Prod. 233, 954–962. doi:10.1016/j.jclepro.2019.06.100
Catalán, E., and Sánchez, A. (2020). Solid-state fermentation (SSF) versus submerged fermentation (SmF) for the recovery of cellulases from coffee husks: a life cycle assessment (LCA) based comparison. Energies 13, 2685. doi:10.3390/en13112685
Cecconet, D., and Capodaglio, A. G. (2022). Sewage sludge biorefinery for circular economy. Sustainability 14, 14841. doi:10.3390/su142214841
Cerda, A., Gea, T., Vargas-García, M. C., and Sánchez, A. (2017). Towards a competitive solid state fermentation: cellulases production from coffee husk by sequential batch operation and role of microbial diversity. Sci. Total Environ. 589, 56–65. doi:10.1016/j.scitotenv.2017.02.184
Cerda, A., Mejias, L., Rodríguez, P., Rodríguez, A., Artola, A., Font, X., et al. (2019). Valorisation of digestate from biowaste through solid-state fermentation to obtain value added bioproducts: a first approach. Bioresour. Technol. 271, 409–416. doi:10.1016/j.biortech.2018.09.131
Chen, L., Chen, Y., Li, Y., Liu, Y., Jiang, H., Li, H., et al. (2023). Improving the humification by additives during composting: a review. Waste Manage 158, 93–106. doi:10.1016/j.wasman.2022.12.040
Cherubini, F. (2010). The biorefinery concept: using biomass instead of oil for producing energy and chemicals. Energy Convers. manage. 51, 1412–1421. doi:10.1016/j.enconman.2010.01.015
Colón, J., Ponsá, S., Álvarez, C., Vinot, M., Lafuente, F. J., Gabriel, D., et al. (2017). Analysis of MSW full-scale facilities based on anaerobic digestion and/or composting using respiration indices as performance indicators. Bioresour. Technol. 236, 87–96. doi:10.1016/j.biortech.2017.03.172
Cuadrado-Osorio, P. D., Ramírez-Mejía, J. M., Mejía-Avellaneda, L. F., Mesa, L., and Bautista, E. J. (2022). Agro-industrial residues for microbial bioproducts: a key booster for bioeconomy. Bioresour. Technol. Rep. 20, 101232. doi:10.1016/j.biteb.2022.101232
Czekała, W., Nowak, M., and Piechota, G. (2023). Sustainable management and recycling of anaerobic digestate solid fraction by composting: a review. Bioresour. Technol. 375, 128813. doi:10.1016/j.biortech.2023.128813
da Costa, R. A. M., Rubio-Ribeaux, D., Carneiro, B. C., Franco, P. M., de Azevedo Mendes, G., da Silva, I. L., et al. (2023). Sugarcane bagasse pretreated by different technologies used as support and carbon source in solid-state fermentation by Aureobasidium pullulans LB83 to produce bioemulsifier. Biomass Conv. bioref. 14, 18579–18592. doi:10.1007/s13399-023-03896-5
Dolman, B. M., Wang, F., and Winterburn, J. B. (2019). Integrated production and separation of biosurfactants. Proc. Biochem. 83, 1–8. doi:10.1016/j.procbio.2019.05.002
Ellen MacArthur Foundation (2024). Circular economy introduction. Available at: https://www.europarl.europa.eu/topics/en/topic/circular-economy (Accessed June, 2024).
Esposito, G., Frunzo, L., Giordano, A., Liotta, F., Panico, A., and Pirozzi, F. (2012). Anaerobic co-digestion of organic wastes. Rev. Environ. Sci. Biotechnol. 11, 325–341. doi:10.1007/s11157-012-9277-8
European Biogas Association (2022). A way out of the EU gas price crisis with biomethane. Available at: https://www.europeanbiogas.eu/a-way-out-of-the-eu-gas-price-crisis-with-biomethane/(Accessed August, 2024).
European Compost Network (2024). Bio-waste management plays a keyrole in bioeconomy. Available at: https://www.compostnetwork.info/policy/circular-economy/bio-waste-management/(Accessed August, 2024).
European Union (2015). Circular economy. Available at: https://www.europarl.europa.eu/topics/en/topic/circular-economy (Accessed June, 2024).
Fagerström, A., Al Seadi, T., Rasi, S., and Briseid, T. (2018). The role of anaerobic digestion and biogas in the circular economy. Cork, Ireland: IEA Bioenergy Task 37. Available at: https://www.ieabioenergy.com/blog/publications/the-role-of-anaerobic-digestion-and-biogas-in-the-circular-economy/(Accessed June, 2024).
Farahmandpour, R., Karimi, K., Denayer, J. F. M., and Shafiei, M. (2022). Innovative biorefineries for cleaner waste textile management towards circular economy: techno-economic analysis. J. Clean. Prod. 378, 134500. doi:10.1016/j.jclepro.2022.134500
Fonseca, R. F., Melo, C. B., Sanches, C. P., Bertucci-Neto, V., Farinas, C. S., and Kwong, W. H. (2017). Modelling of solid-state fermentation over wide operational range for application in process optimization. Can. J. Chem. Eng. 96, 1723–1734. doi:10.1002/cjce.23088
Font, X., and Sánchez, A. (2021). “Significance of anaerobic digestion in circular bioeconomy,” in Biomass, Biofuels, biochemicals. Editors A. Pandey, R. Dayal Tyagi, and S. Varjani (Netherlands: Elsevier), 269–289. doi:10.1016/B978-0-12-821878-5.00020-9
Gaffey, J., Collins, M. N., and Styles, D. (2024). Review of methodological decisions in life cycle assessment (LCA) of biorefinery systems across feedstock categories. J. Environ. Manage. 358, 120813. doi:10.1016/j.jenvman.2024.120813
Ghoreishi, G., Barrena, R., and Font, X. (2023). Using green waste as substrate to produce biostimulant and biopesticide products through solid-state fermentation. Waste Manage 159, 84–92. doi:10.1016/j.wasman.2023.01.026
Gkotsis, P., Kougias, P., Mitrakas, M., and Zouboulis, A. (2023). Biogas upgrading technologies – recent advances in membrane-based processes. Int. J. Hydrog. Energy 48, 3965–3993. doi:10.1016/j.ijhydene.2022.10.228
Gnansounou, E., and Pandey, A. (2017). Life-cycle assessment of biorefineries. Netherlands: Elsevier. doi:10.1016/B978-0-444-63585-3.09989-7
Gowthaman, M. K., Krishna, C., and Moo-Young, M. (2001). Fungal solid state fermentation - an overview. Appl. Mycol. Biotechnol. 1, 305–352. doi:10.1016/S1874-5334(01)80014-9
Granucci, N., and Granato Billas Boas, S. (2019). Process and production system for large scale solid-state fermentation. Toulouse, France: Assigned to Green Spot Technologies SAS. European patent EP3805358A1.
Gupta, R., Ouderji, Z. H., Uzma, Yu, Z., Sloan, W. T., and You, S. (2024). Machine learning for sustainable organic waste treatment: a critical review. Mater. Sustain. 2, 5. doi:10.1038/s44296-024-00009-9
Hagman, L., Blumenthal, A., Eklund, M., and Svensson, N. (2018). The role of biogas solutions in sustainable biorefineries. J. Clean. Prod. 172, 3982–3989. doi:10.1016/j.jclepro.2017.03.180
Johnravindar, D., Wong, J. W. C., Dharma Patria, R., Uisan, K., Kumar, R., and Kaur, G. (2022). Bioreactor-scale production of rhamnolipids from food waste digestate and its recirculation into anaerobic digestion for enhanced process performance: creating closed-loop integrated biorefinery framework. Bioresour. Technol. 360, 127578. doi:10.1016/j.biortech.2022.127578
Khoshnevisan, B., Tabatabaei, M., Tsapekos, P., Rafiee, S., Aghbashlo, M., Lindeneg, S., et al. (2020). Environmental life cycle assessment of different biorefinery platforms valorizing municipal solid waste to bioenergy, microbial protein, lactic and succinic acid. Renew. Sustain. Energy Rev. 117, 109493. doi:10.1016/j.rser.2019.109493
Krishna, C. (2005). Solid-state fermentation systems - an overview. Crit. Rev. Biotechnol. 25, 1–30. doi:10.1080/07388550590925383
Lago, C., Caldés, N., and Lechón, Y. (2019). The role of bioenergy in the emerging bioeconomy (resources, technologies, sustainability and policy). 1st Edition. Netherlands: Elsevier. doi:10.1016/C2016-0-03740-3
Lonsane, B. K., Saucedo-Castaneda, G., Raimbault, M., Roussos, S., Viniegra-Gonzalez, G., Ghildyal, N. P., et al. (1992). Scale-up strategies for solid state fermentation systems. Process Biochem. 27, 259–273. doi:10.1016/0032-9592(92)85011-P
Lora-Granado, R., de Souza-Antune, A. D., da Fonseca, F. V., Sánchez, A., Barrena, R., and Font, X. (2017). Technology overview of biogas production in anaerobic digestion plants: a European evaluation of research and development. Renew. Sustain. Energy Rev. 80, 44–53. doi:10.1016/j.rser.2017.05.079
Lüth, P., and Eiben, U. (2003). Solid-state fermenter and method for solid-state fermentation. Malchow, Germany: Assigned to Bayer Cropscience Biologics GMBH. US patent US6620614B1.
Marín, M., Artola, A., and Sánchez, A. (2018). Production of proteases from organic wastes by solid-state fermentation: downstream and zero waste strategies. 3 Biotech. 8, 205. doi:10.1007/s13205-018-1226-y
Martínez, O., Sánchez, A., Font, X., and Barrena, R. (2018). Enhancing the bioproduction of value-added aroma compounds via solid-state fermentation of sugarcane bagasse and sugar beet molasses: operational strategies and scaling-up of the process. Bioresour. Technol. 263, 136–144. doi:10.1016/j.biortech.2018.04.106
Martínez-Arce, A., O'Flaherty, V., and Styles, D. (2024). State-of-the-art in assessing the environmental performance of anaerobic digestion biorefineries. Resour. Conserv. Recy. 207, 107660. doi:10.1016/j.resconrec.2024.107660
Martínez-Valdez, F., Komilis, D., Saucedo-Castañeda, G., Barrena, R., and Sánchez, A. (2017). The effect of a short term aerobic pretreatment step on the anaerobic Co-digestion of the organic fraction of municipal solid wastes: liquid extract addition versus solid phase addition. Waste Biomass. Valor. 8, 1793–1801. doi:10.1007/s12649-016-9743-6
Millati, R., Wikandari, R., Ariyanto, T., Hasniah, N., and Taherzadeh, M. J. (2023). Anaerobic digestion biorefinery for circular bioeconomy development. Bioresour. Technol. Rep. 21, 101315. doi:10.1016/j.biteb.2022.101315
Mitchell, D. A., Krieger, N. A., and Berovič, M. (2006). Solid-state fermentation bioreactors: fundamentals of design and operation. Germany: Springer-Verlag Berlin Heidelberg.
Mitchell, D. A., Ruiz, H. A., and Krieger, N. A. (2023). A critical evaluation of recent studies on packed-bed bioreactors for solid-state fermentation. Processes 11, 872. doi:10.3390/pr11030872
Molina-Peñate, E., Artola, A., and Sánchez, A. (2022). Organic municipal waste as feedstock for biorefineries: bioconversion technologies integration and challenges. Rev. Environ. Sci. Biotechnol. 21, 247–267. doi:10.1007/s11157-021-09605-w
Molina-Peñate, E., Artola, A., and Sánchez, A. (2024). Exploring biorefinery alternatives for biowaste valorization: a techno-economic assessment of enzymatic hydrolysis coupled with anaerobic digestion or solid-state fermentation for high-value bioproducts. Bioengineered 15, 2307668. doi:10.1080/21655979.2024.2307668
Oiza, N., Moral-Vico, J., Sánchez, A., and Gea, T. (2024). Enhanced anaerobic digestion of food waste using purified lactonic sophorolipids produced by solid-state fermentation of molasses and oil waste: a circular approach. J. Clean. Prod. 468, 143062. doi:10.1016/j.jclepro.2024.143062
Oiza, N., Moral-Vico, J., Sánchez, A., Oviedo, E. R., and Gea, T. (2022). Solid-state fermentation from organic wastes: a new generation of bioproducts. Processes 10, 2675. doi:10.3390/pr10122675
Outeiriño, D., Costa-Trigo, I., Ochogavias, A., de Souza Oliveira, R. P., Pérez Guerra, N., Salgado, J. M., et al. (2024). Biorefinery of brewery spent grain to obtain bioproducts with high value-added in the market. New Biotechnol. 79, 111–119. doi:10.1016/j.nbt.2023.12.010
Pandey, A. (2003). Solid-state fermentation. Biochem. Eng. J. 13, 81–84. doi:10.1016/S1369-703X(02)00121-3
Paniagua, S., Lebrero, R., and Muñoz, R. (2022). Syngas biomethanation: current state and future perspectives. Bioresour. Technol. 358, 127436. doi:10.1016/j.biortech.2022.127436
Parra-Orobio, A., Soto-Paz, J., Oviedo-Ocaña, R., Vali, S. A., and Sánchez, A. (2023). Advances, trends and challenges in the use of biochar as an improvement strategy in the anaerobic digestion of organic waste: a systematic analysis. Bioengineered 14. doi:10.1080/21655979.2023.2252191
Pérez, V., Moltó, J. L., Lebrero, R., and Muñoz, R. (2022). Ectoine production from biogas: a sensitivity analysis. Effect of local commodity prices, economy of scale, market trends and biotechnological limitations. J. Clean. Prod. 369, 133440. doi:10.1016/j.jclepro.2022.133440
Picardo, A., Soltero, V. M., Peralta, E., and Chacartegui, R. (2019). District heating based on biogas from wastewater treatment plant. Energy 180, 649–664. doi:10.1016/j.energy.2019.05.123
Prabhu, G., Bhat, D., Bhat, R. M., and Selvaraj, S. (2022). A critical look at bioproducts Co-cultured under solid state fermentation and their challenges and industrial applications. Waste Biomass Valor 13, 3095–3111. doi:10.1007/s12649-022-01721-0
Ranganathan, L. (2014). A solid state fermentation method. Bagsværd, Denmark: Assigned to Novozymes A/S. European patent WO2014082847A1.
Rodríguez, P., Cerda, A., Font, X., Sánchez, A., and Artola, A. (2019). Valorisation of biowaste digestate through solid state fermentation to produce biopesticides from Bacillus thuringiensis. Waste Manage 93, 63–71. doi:10.1016/j.wasman.2019.05.026
Romero-Güiza, M. S., Vila, J., Mata-Alvarez, J., Chimenos, J. M., and Astals, S. (2016). The role of additives on anaerobic digestion: a review. Renew. Sustain. Energy Rev. 58, 1486–1499. doi:10.1016/j.rser.2015.12.094
Sachdeva, S., Garg, V. K., Labhsetwar, N. K., Singh, A., and Yogalakshmi, K. N. (2022). “Zero waste biorefinery: a comprehensive outlook,” in Zero waste biorefinery, energy, environment, and sustainability series. Editors Y. Kadapakkam Nandabalan, V. K. Garg, N. K. Labhsetwar, and A. Singh (Singapore: Springer), 3–22. doi:10.1007/978-981-16-8682-5_1
Sahu, P. K., Singh, R., Shrivastava, M., Darjee, S., Mageshwaran, V., Phurailtpam, L., et al. (2024). Microbial production of α-amylase from agro-waste: an approach towards biorefinery and bio-economy. Energy Nexus 14, 100293. doi:10.1016/j.nexus.2024.100293
Sala, A., Barrena, R., Sánchez, A., and Artola, A. (2021). Fungal biopesticide production: process scale-up and sequential batch mode operation with Trichoderma harzianum using agro-industrial solid wastes of different biodegradability. Chem. Eng. J. 425, 131620. doi:10.1016/j.cej.2021.131620
Sala, A., Echegaray, T., Palomas, G., Boggione, M. J., Tubio, G., Barrena, R., et al. (2022). Insights on fungal solid-state fermentation for waste valorization: conidia and chitinase production in different reactor configurations. Sustain. Chem. Pharm. 26, 100624. doi:10.1016/j.scp.2022.100624
Sambusiti, C., Monlau, F., and Barakat, A. (2016). Bioethanol fermentation as alternative valorization route of agricultural digestate according to a biorefinery approach. Bioresour. Technol. 212, 289–295. doi:10.1016/j.biortech.2016.04.056
Sánchez, A. (2022). Adding circularity to organic waste management: from waste to products through solid-state fermentation. Resour. Environ. Sustain. 8, 100062. doi:10.1016/j.resenv.2022.100062
Sentís-Moré, P., Romero-Fabregat, M. P., Rodríguez-Marca, C., Guerra-Sánchez, A. J., and Ortega-Olivé, N. (2023). Design optimization of a tray bioreactor for solid-state fermentation: study of process parameters through protein modification of by-products. Fermentation 9, 921. doi:10.3390/fermentation9100921
Singh, P., Srivastava, N., Mohammad, A., Lal, B., Singh, R., Syed, A., et al. (2023). Facile pretreatment strategies to biotransform Kans grass into nanocatalyst, cellulolytic enzymes, and fermentable sugars towards sustainable biorefinery applications. Bioresour. Technol. 386, 129491. doi:10.1016/j.biortech.2023.129491
Soccol, C. R., Ferreira da Costa, E. S., Junior Letti, L. A., Grace Karp, S., Woiciechowski, A. L., and de Souza Vandenberghe, L. P. (2017). Recent developments and innovations in solid state fermentation. Biotechnol. Res. Innov. 1, 52–71. doi:10.1016/j.biori.2017.01.002
Takkellapati, S., Li, T., and Gonzalez, M. A. (2018). An overview of biorefinery-derived platform chemicals from a cellulose and hemicellulose biorefinery. Clean. Techn. Environ. Policy 20, 1615–1630. doi:10.1007/s10098-018-1568-5
United Nations (2017). The 17 sustainable development goals (SDGs). Available at: https://sdgs.un.org/goals (Accessed June, 2024).
U.S. Environmental Protection Agency (2024). Basics of green chemistry. Available at: https://www.epa.gov/greenchemistry/basics-green-chemistry (Accessed June, 2024).
Vali, S. A., Moral-Vico, J., Font, X., and Sánchez, A. (2023). Adsorptive removal of siloxanes from biogas: recent advances in catalyst reusability and water content effect. Biomass Conv. bioref. 14, 23259–23273. doi:10.1007/s13399-023-04478-1
Virtanen, V., Jääskeläinen, S., and Seiskari, P. (2011). Reactor and method for solid state fermentation. Luoma, Finland: Assigned to Verdera Oy. US patent 20080057576.
Weinand, J. M., McKenna, R., Karner, K., Braun, L., and Herbes, C. (2019). Assessing the potential contribution of excess heat from biogas plants towards decarbonising residential heating. J. Clean. Prod. 238, 117756. doi:10.1016/j.jclepro.2019.117756
Xu, Q., Liu, X., Wang, D., Liu, Y., Wang, Q., Ni, B.-J., et al. (2019). Enhanced short-chain fatty acids production from waste activated sludge by sophorolipid: performance, mechanism, and implication. Bioresour. Technol. 284, 456–465. doi:10.1016/j.biortech.2019.03.121
Keywords: organic waste, solid-state bioconversion, biomaterials, bioproducts, renewable energy, zero-waste strategy
Citation: Artola A, Font X, Moral-Vico J and Sánchez A (2024) The role of solid-state fermentation to transform existing waste treatment plants based on composting and anaerobic digestion into modern organic waste-based biorefineries, in the framework of circular bioeconomy. Front. Chem. Eng. 6:1463785. doi: 10.3389/fceng.2024.1463785
Received: 12 July 2024; Accepted: 07 October 2024;
Published: 17 October 2024.
Edited by:
Madan L. Verma, Indian Institute of Information Technology, Una, IndiaReviewed by:
Jéssica Mulinari, The University of Passo Fundo, BrazilArturo F. Chica, University of Cordoba, Spain
Simone Kubeneck, Federal University of the Southern Frontier, Brazil
Copyright © 2024 Artola, Font, Moral-Vico and Sánchez. This is an open-access article distributed under the terms of the Creative Commons Attribution License (CC BY). The use, distribution or reproduction in other forums is permitted, provided the original author(s) and the copyright owner(s) are credited and that the original publication in this journal is cited, in accordance with accepted academic practice. No use, distribution or reproduction is permitted which does not comply with these terms.
*Correspondence: Antoni Sánchez, YW50b25pLnNhbmNoZXpAdWFiLmNhdA==