- Catalysis Group, Advanced Material Division, Mintek, Randburg, South Africa
The emerging field of using titanium dioxide (TiO2)-based photosensitizers for enhancing photocatalytic removal of thiazine dyes such as methylene blue (MB) from water has long been recognized for its exceptional photocatalytic properties, making it an attractive material for environmental remediation and energy conversion. However, its wide bandgap limits its responsiveness to visible light. As such, the utilization of TiO2-based photosensitizers for the removal of thiazine dyes, presents a promising avenue for diverse applications. In addressing the dual challenges of environmental pollution and harnessing sustainable energy sources, this review focuses on the removal of thiazine dyes from water and their subsequent application as photosensitizers for TiO2 materials. Thiazine dyes, ubiquitous in industrial effluents, pose environmental concerns due to their persistence and potential toxicity. Conversely, this innovative approach involves employing TiO2 materials as photocatalysts, utilizing the unique properties of thiazine dyes to enhance light absorption. Studies have shown that beyond the conventional role of thiazine dyes as colorants, they can serve as effective photosensitizers when coupled with TiO2. This tandem not only facilitates the elimination of thiazine dyes, such as MB, from water but also augments the improvement of the photocatalytic performance of TiO2 materials. The synergy between dye sensitizers and TiO2 enhances the overall efficiency of processes like dye degradation and water splitting. Dye sensitizers, acting as light energy absorbers, can efficiently transfer this energy to TiO2, thereby promoting electron transfer and generating reactive oxygen species (ROS). These ROS, in turn, initiate chemical reactions, rendering dye sensitizers valuable in applications such as wastewater treatment, solar energy conversion, and environmental remediation. As such, it is crucial to acknowledge the potential drawbacks associated with thiazine dyes, including toxicity and non-biodegradability. Consequently, careful consideration must be given to thiazine dye application and disposal. Therefore, this review manuscript delves into the comprehensive exploration of TiO2-based photosensitizers, shedding light on their efficacy in various photocatalytic processes for thiazine dye removal.
1 Introduction to TiO2 as a photocatalyst
Photocatalysis, a phenomenon that harnesses the power of light to drive chemical reactions on the surface of semiconductors, has garnered substantial attention in the realm of environmental and energy-related research (Magalhães et al., 2017; Tahir et al., 2020; Li et al., 2020). Among the diverse array of photocatalysts, titanium dioxide (TiO2) has emerged as a paradigmatic and widely studied material due to its exceptional photochemical properties and versatile applications (AlSalka et al., 2023). This review delves into the multifaceted aspects of TiO2 photocatalysis, emphasizing its significance, its inherent limitations concerning visible light utilization, and the pivotal role played by photosensitizers such as thiazines in augmenting TiO2 photocatalytic efficacy.
TiO2 photocatalysis is a photochemical process that exploits the semiconducting properties of this material to catalyze a myriad of chemical reactions when exposed to ultraviolet (UV) or visible light irradiation (Schneider et al., 2014; Pendergast et al., 2010). Its significance lies in the potential to address a wide spectrum of global challenges, ranging from environmental remediation to renewable energy technologies (Zhang et al., 2022). The pioneering work of Akira Fujishima and Kenichi Honda in the late 1960s brought TiO2 photocatalysis to the forefront by showcasing its ability to split water into hydrogen and oxygen when illuminated by UV light, thereby initiating the exploration of TiO2 as a photocatalyst for water splitting and pollutant degradation (Kenichi Honda, 2023; Fujishima and Honda, 1972). TiO2 photocatalysis has since emerged as a sustainable and eco-friendly strategy for mitigating environmental pollution. Its ability to mineralize a variety of organic pollutants into harmless by-products has made it an invaluable tool for wastewater treatment and air purification (Schneider et al., 2014; Dharma et al., 2022). The ability to harness solar energy for these processes has the potential to revolutionize the energy landscape by offering an environmentally friendly alternative to conventional energy sources (AlSalka et al., 2023; Esrafili et al., 2022; Peiris et al., 2021). As a result, TiO2 materials hold immense promise for the generation of clean energy through processes such as photocatalytic hydrogen production and photovoltaic applications.
Despite the myriad advantages of TiO2, one of the most significant drawbacks as a semiconductor is the limited responsiveness to visible light, which constitutes a substantial portion of the solar spectrum. The intrinsic wide bandgap of TiO2 (∼3.2 eV for anatase and ∼3.0 eV for rutile) only utilise UV light energy for the excitation of electrons from the valence band (VB) to the conduction band (CB) to enable photocatalytic reactions, which was reported by Anucha et al. (2022) and Rafique et al. (2020) in their studies (Anucha et al., 2022; Rafique et al., 2020). This limitation restricts the overall efficiency and practicality of TiO2-based photocatalysis, as UV light comprises only a small fraction of the solar radiation reaching the Earth’s surface (Anucha et al., 2022; Dong et al., 2015). This reliance on UV light not only limits the range of applications but also results in increased energy consumption for artificial UV light sources, diminishing the overall sustainability of the photocatalytic processes. To address these challenges and maximize the utilization of solar energy for photocatalysis, there is a compelling need to extend TiO2 photoresponsiveness into the visible light region (Rafique et al., 2020; Dong et al., 2015). Through expanded light absorption, efficient charge separation, and improved catalytic activity, photosensitizers contribute to making TiO2-based photocatalysis more versatile and effective for applications such as environmental remediation and solar energy conversion. A study by Tinoco et al. (2023) showed that TiO2 is a widely used photocatalyst known for its ability to harness light energy to drive chemical reactions, particularly in the degradation of pollutants or the generation of clean energy (Tinoco Navarro and Jaroslav, 2023). As a result, incorporation of photosensitizers could play a crucial role in enhancing the photocatalytic activity of materials like TiO2.
Anucha et al. (2022) have exhibited that the incorporation of photosensitizers is a strategy employed to address these limitations and improve the photocatalytic performance of TiO2. The following are the key reasons why photosensitizers are important in the context of TiO2 photocatalysis (Anucha et al., 2022). The TiO2 primarily absorbs UV light due to its wide bandgap. Photosensitizers, on the other hand, can absorb light in a broader range, including visible light (Tinoco Navarro and Jaroslav, 2023; Yu et al., 2022). Through coupling a photosensitizer with TiO2, the composite photocatalyst becomes capable of utilizing a wider spectrum of sunlight, making it more efficient under natural light conditions. The photosensitizers can facilitate the separation of photoinduced electron-hole pairs more effectively (Pawar et al., 2018). When light is absorbed by the photosensitizer, it generates an excited state with an electron at a higher energy level. This electron can then can be transfered to the conduction band of TiO2, leaving behind a positive hole in the photosensitizer (Carella et al., 2018). This separation of charges helps to reduce electron-hole recombination rates, a common issue in TiO2 photocatalysis that can limit its overall efficiency. In this regard, the presence of photosensitizers can boost the photocatalytic activity of TiO2 by promoting specific reactions or pathways (Kang et al., 2019). For example, Shin et al. (2023) in their study showed that certain photosensitizers have been found to enable the generation of reactive oxygen species (ROS), which are highly effective in the degradation of organic pollutants (Shin et al., 2023). Such photosensitizers can also be engineered to have enhanced ROS generation by controlling their excitation wavelength. The formation of excited triplet states through intersystem crossing (ISC) plays a crucial role in the generation of ROS by organic photosensitizers (Wang et al., 2022). This showed that enhanced catalytic activity could contributes to the overall efficacy of TiO2-based photocatalysts. Lastly, photosensitizers can modify the redox potential of TiO2, making it more favorable for specific reactions. This modification can enhance the ability of TiO2 to participate in oxidation-reduction reactions, which are often involved in photocatalytic processes (Kang et al., 2019; Al-Nuaim et al., 2022; Jiang et al., 2021).
2 TiO2-based photosensitizers
Photosensitizers have emerged as a strategic solution to circumvent photocatalysts limitations such as wide band gap of TiO2, ZnO, etc., regarding visible light utilization. These molecular entities, which can absorb visible light, subsequently transfer their photoexcited electrons to TiO2, extending its absorption spectrum into the visible region (Hamza et al., 2023; Escudero et al., 2021). This energy transfer mechanism facilitates the excitation of TiO2 electrons from the valence band to the conduction band, enabling the photocatalytic process to occur under visible light irradiation. Incorporating photosensitizers into TiO2-based photocatalytic systems has become a pivotal strategy to enhance the efficiency and versatility of TiO2 photocatalysis. Photosensitizers may encompass a diverse range of compounds, including organic dyes, metal complexes, and semiconductor quantum dots, each possessing unique optical and electronic properties that can be tailored to specific photocatalytic applications (AlSalka et al., 2023; Esrafili et al., 2022; Anucha et al., 2022; Sakar et al., 2019). TiO2 is a widely used semiconductor material in the field of dye-sensitized solar cells (DSSCs) where it serves as the photoanode, and its surface is sensitized with a dye to enhance light absorption and electron injection. Various dyes have been developed over the years for this purpose. Table 1 generally summaries typical dye photosensitizers used to modify TiO2 material.
As shown on Table 1, dye photosensitizers exhibited a remarkable performance by efficiently harnessing light energy to initiate intricate photochemical processes, facilitating enhanced charge carrier generation and separation for applications ranging from photocatalysis to sustainable environmental technologies. As a result, researchers continue to explore novel dye structures and sensitization strategies to improve the efficiency and stability of TiO2-based photosensitizers. The judicious selection and design of photosensitizers have thus opened up new horizons for harnessing visible light and expanding the scope of TiO2 photocatalysis, allowing for more sustainable and energy-efficient processes (Liu et al., 2023; Wategaonkar et al., 2021). Therefore, TiO2 photocatalysis represents a powerful and versatile approach for addressing environmental and energy challenges. However, its limited responsiveness to visible light has prompted the integration of photosensitizers as a strategic means to enhance its photocatalytic activity. This comprehensive review explores the multifaceted aspects of TiO2-based photosensitizers for the removal and reuse of thiazine dyes such as MB from water, shedding light on their synthesis, characterization, and applications in diverse photocatalytic processes, thereby advancing the field of sustainable and efficient photocatalysis.
3 Thiazine dyes and their properties
Thiazines are classified as organic molecules, which can be structured in many different ways, as shown in Figure 1. In organic synthesis, thiazines are produced from the reaction of thiourea and alkyl propiolates compounds (Danilkina et al., 2006; Begum Sri Padmavati Mahila Visvavidyalayam et al., 2016; Mohlala et al., 2021; Pirillo et al., 2018). These groups of organic compounds have been extensively explored in the literature for diverse applications, spanning biological activities and material utilization. Although thiazine dyes are considered toxic, they find application as photosensitizers in pharmaceutical applications.
In the absence of light, thiazine dyes remain non-toxic. However, upon exposure to an appropriate amount of light, they undergo a transformation, becoming highly reactive (Mohlala et al., 2021; Pirillo et al., 2018; Fabio et al., 2016). Thiazine dyes are among the most significant organic dyes with established uses in technology and science. They are employed in a variety of chemical, biological, and medical research studies (Gilani et al., 2017). Because of their acceptable biological, chemical, photochemical, and photophysical properties, these cationic dyes are utilized as phototherapeutic agents. The physiological and physicochemical characteristics of the thiazine dyes influences the effectiveness of the photodynamic therapy (PDT). To date the generation of thiazine dyes as photosensitizers in the presence of light is driven to improve properties such as cytotoxicity, chemical purity and composition (Montes De Oca et al., 2013). These set of compounds are also derived to be effective in the production of singlet oxygen, photochemical reactivity, high extinction coefficient and referential retention by the target tissue (Robertson et al., 2009; Detty et al., 2004; Triesscheijn et al., 2006). The high singlet oxygen quantum yields of thiazine dyes contribute to their efficacy in inducing photodynamic reactions. This feature is particularly advantageous for the degradation of organic pollutants present in wastewater.
Global production of various dyes amounts to over 700,000 tons per year, and the textile dye sector generates significant amounts of highly coloured wastewater from dye processes (Eslami et al., 2015). Since all of this wastewater is poisonous and has the potential to be dangerous, it should be cleaned up before being dumped into rivers, streams, or the ocean (Cunico et al., 2015). Table 2 provides an overview of various thiazine dyes along with key properties relevant to their application in photocatalysis and environmental processes. The included dyes encompass a range of well-known compounds, each distinguished by its unique chemical structure, absorption wavelength, photocatalytic activity, and environmental impact.
The presented table offers a concise overview of various thiazine dyes. Methylene Blue, with its high photocatalytic activity at a 665 nm absorption wavelength, emerges as a promising candidate (jie Song et al., 2023; Khan et al., 2022; Tardivo et al., 2005). Rhodamine B and Azure A exhibit moderate photocatalytic activity, while Toluidine Blue and Janus Green B show varying degrees of effectiveness. The choice of thiazine dye in photocatalysis is multifaceted, considering factors like absorption wavelength, environmental impact, and overall performance. Rhodamine B and Toluidine Blue, despite moderate photocatalytic activity, may find applications in scenarios where a balance between performance and environmental impact is critical (Hamza et al., 2023; Valadez-Renteria et al., 2022). Furthermore, continuous exploration and understanding of the chemical structures and properties of thiazine dyes will contribute to the optimization of photocatalytic processes and the development of sustainable environmental technologies.
The majority of dyes have stable chemical structures that ae challenging to break down; in particular, azo and cationic thiazine variants, which are produced in vast quantities, and are most challenging (Navarro et al., 2017). Treatments for the degradation of azo and cationic thiazine dyes employing several advanced oxidation processes (AOPs) have garnered a lot of attention lately (Luo et al., 2017). Research on wastewater treatment for the degradation of household wastes, agricultural discharges, and industrial effluents has been conducted for a long time. To identify and illuminate the hazardous emergence from the pollutants, the plant needs its initial detection system. The sludge extraction process reverses osmosis, membrane filtration, photocatalysis, and flocculation are some of the cutting-edge techniques for treating wastewater introduced by nanotechnology (Asahi et al., 2001). According to a report, TiO2 and ZnO nanostructures are employed as efficient photocatalysts for the treatment of wastewater because they possess many advantages, which include high activity, affordability, stability, and nontoxicity (Bhatkhande et al., 2002).
Photocatalysis has emerged as a well-organized method for air and water purification through the degradation of contaminants that are commonly used in organic colorants on paper, plastics, food, leather, textiles, and cosmetics. Because the effluents maximize chemical oxygen demand, they are highly dangerous to aquatic environments and species (Murray and Parsons, 2004). Since the majority of organic dyes are both stable and resistant to photodegradation, currently research is being done on the removal of these released dyes. However, in typical wastewater treatment, thiazine dye removal or incorporation into the photocatalysts can in turn be tailored to selectively and further target specific contaminants commonly found in wastewater, such as organic matter, microbes, and other impurities. This selectivity could minimize the impact on non-target components, ensuring a more focused and efficient treatment process as well as the reuse of thiazine dyes. As a result, the use of thiazine dyes as photosensitizers in wastewater treatment presents a future prospect that can lead to their reduced environmental footprint. Their low toxicity to healthy organisms and tissues could make them a more sustainable alternative compared to traditional water treatment chemicals (Zhang et al., 2023).
In addition, the thiazine compounds ability to self-assemble has significant implications for a variety of applications, including photo-medicine, fluorescence depolarization diagnostics, tunable lasers, molecular optoelectronics, and photographic technologies (Arik and Onganer, 2003). Thiazine dyes are more stable in aqueous solutions as they do not undergo demethylation (Abbott, 1962) and they have low ionization potential due to the presence of two heteroatoms, which could limit their toxicity in water (Havelcovaâ et al., 2023). Intrinsically thiazine set of compounds are ideal candidates for photodynamic treatment because they exhibit selectivity for cancer cells in vivo (Paul and Suresh Kumar, 2013) and have high singlet oxygen quantum yields (Ronzani et al., 2014). Thiazine dyes as photosensitizers have also shown to have antibacterial effects on a variety of harmful microbes, which could be also beneficial in wastewater treatment (Pinto et al., 2017; Decker et al., 2017; Monteiro et al., 2017). However, it effectiveness as a dye photosensitizer for wastewater treatment is still an unproven hypothesis. Meanwhile, despite these benefits of thiazine dyes, it is well known that their planar and even ionic structures tend to congregate in diluted fluids, producing dimers and occasionally higher-order aggregates (Chakraborty et al., 2010; Dar and Ankari, 2022). This phenomenon is mainly associated with the hydrophobic character of the basic structure of thiazine dyes (Gilani et al., 2017).
On the other prospect, the removal of thiazine dyes, particularly methylene blue, from wastewater has gained significant attention, and TiO2-based photosensitizers have emerged as effective agents. When combined with TiO2, thiazine dyes like MB can serve as potent photosensitizers, enhancing the photocatalytic activity of TiO2 and enabling the efficient removal of these dyes from water (Khan et al., 2022; Kohle et al., 2020; Basumatary et al., 2022). However, the interaction between thiazine dyes and TiO2 involves the absorption of light energy by the dyes, which is then transferred to TiO2. This process promotes electron transfer and the generation of ROS, which play a pivotal role in initiating chemical reactions (Sarfraz et al., 2023; Rao et al., 2022; Lima and Reis, 2023). The photocatalytic activity induced by TiO2-based photosensitizers proves valuable in the degradation of thiazine dyes, contributing to the remediation of wastewater. This environmentally friendly approach not only addresses the removal of pollutants but also aligns with the broader goals of sustainable water treatment technologies. The integration of TiO2-based photosensitizers for thiazine dye removal and reuse holds promise for advancing efficient and eco-friendly wastewater treatment strategies.
4 Light absorption and energy transfer by photosensitizers
The mechanisms of light absorption and energy transfer between dye sensitizers and TiO2 involve a series of intricate steps that facilitate the enhancement of photocatalytic activity. For example, organic dye, as a photosensitizer, absorbs light energy, typically in the visible spectrum (Zani et al., 2021). This absorbed energy excites the electrons within the dye molecules to higher energy states. Subsequently, the excited electrons in organic dye are transferred to the TiO2 surface through a process known as energy transfer. TiO2, being a semiconductor, effectively captures and utilizes the transferred electrons (Zani et al., 2021; Zhao et al., 2021).
The interaction between dye sensitizers and TiO2 is governed by the creation of electron-hole pairs on the TiO2 surface upon absorbing the transferred electrons. This process promotes efficient charge separation, where electrons move through the TiO2 lattice while holes are left behind (Elmorsy et al., 2023; Zani et al., 2021). The excited electrons on the TiO2 surface can participate in redox reactions, leading to the generation of ROS, such as superoxide ions and hydroxyl radicals. These ROS play a crucial role in initiating chemical reactions and promoting the degradation of organic pollutants, including the thiazine dyes. The synergistic interaction between photosensitizers and TiO2 amplifies the photocatalytic activity of TiO2, making it a powerful approach for applications such as wastewater treatment and dye degradation (Elmorsy et al., 2023; Ananthakumar et al., 2019).
4.1 Photosulfitochemistry mechanism of MB dye
A study by Luo et al. (2021) reported the photosulfitochemistry mechanism of MB dye as demonstrated in Figure 2. The mechanism outlines a sequence of chemical reactions involved in the wastewater treatment process using MB as a photosensitizer involving five processes namely;
(i) Photo-excitation of MB+: where the ground state MB+ becomes excited to the single state 1MB⁺* after absorbing visible light. This process is followed by the generation of triplet state 3MB⁺* from 1MB⁺* after going through fast intersystem crossing (ISC). For the sake of simplicity in this process, the ISC reaction is not included here, and MB⁺* stands for the excited MB+'s triplet state.
(ii) Electron transfer between MB⁺* and sulfite: When MB+* removes an electron from sulfite, primary radicals MB⁺ and SO3⁻ are created. At this point, the oxysulfur radical chain reaction is currently at its starting phase.
(iii) Radical propagation: After reacting with an oxygen molecule to generate secondary highly oxidative SO5, SO3⁻ next interacts with sulfite to form SO4⁻.
(iv) Recovery of MB+: MB+ recovery is achieved by the radical termination of MB⁺ via disproportionation or radical transfer to oxygen. As an intermediate from the disproportionation of MB⁺, the colourless dye Leuco methylene blue (LMB) is readily reoxidized by O2 back to MB+. When compared to transition metals as a catalyst for sulfite activation, MB+/LMB exhibits a substantially faster redox turnover, which facilitates a significant As(III) oxidation and requires significantly less MB+ concentration.
(v) As(III) oxidation: The primary radical oxidant that causes As(III) oxidation is SO5⁻.
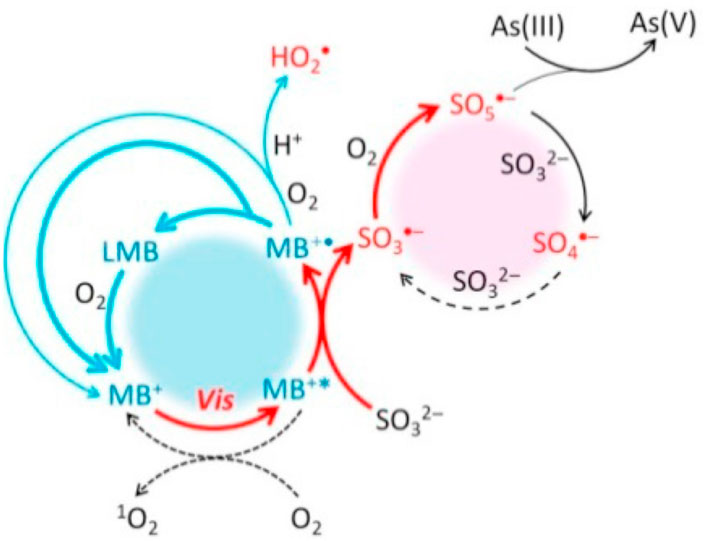
FIGURE 2. The As(III) oxidation mechanism in the photo-MB⁺-sulfite system at neutral pH (Luo et al., 2021).
As a result, the use of MB⁺ and its recovery in this process is advantageous due to its faster redox turnover compared to transition metals as catalysts for sulfite activation. This efficiency facilitates significant As(III) oxidation and requires lower MB⁺ concentrations (Luo et al., 2021). Intrinsically, it is worth noting that the overall success of this wastewater treatment process depends on factors such as reaction kinetics, efficiency of radical generation, and the ability to recycle thiazine dyes i.e., MB effectively. Additionally, the specifics of the ISC reaction and any possible side reactions not mentioned may influence the overall performance of the system. Notably, MB has been widely employed in the photooxidation of both synthetic and natural compounds (Tardivo et al., 2005; Luo et al., 2021; Yhon et al., 2023). As shown on Scheme 1, two main types of photochemical process can be observed, which can be explained as follows:
Type I, is where reducing agents gives an electron to the MB triplet, generating the semi-reduced radical MB, Reaction (3), in Scheme 1, and Type II, is where the triplet energy is transferred to oxygen, forming singlet oxygen (1O2, Reaction (2)) (Scheme 1). The ground state MB molecules can function as reducing agents by themselves at high dye concentrations (D − D* mechanism) (Junqueira et al., 2002; Severino et al., 2003). As such, the schematic representation demonstrates the effectiveness of using thiazines such as MB as photosensitizers.
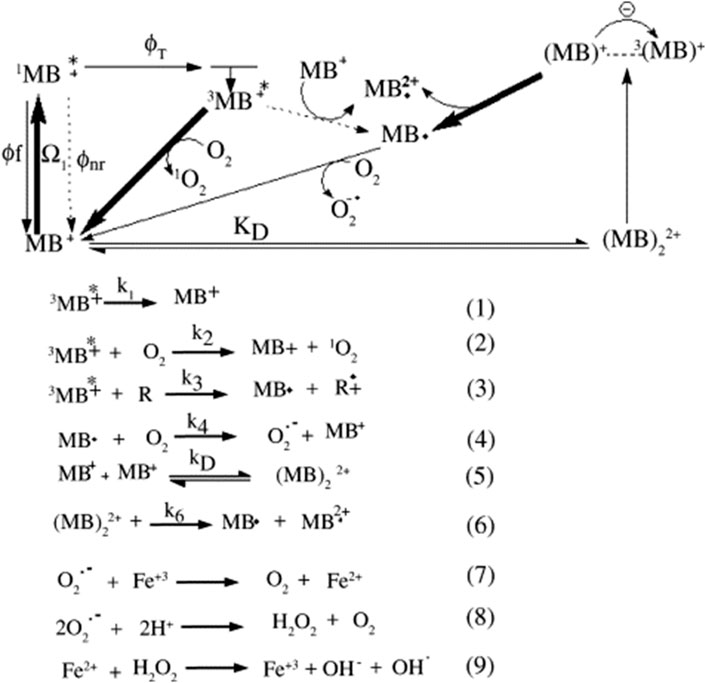
SCHEME 1. The photochemical reaction pathways of methylene blue (Tardivo et al., 2005).
Scheme 1 also shows the photochemical pathways for MB in which MB+, 1MB+*, 3MB+* represent the ground state, singlet, and triplet excited states of MB, respectively, which was reported by Tardivo et al. (2005) (Tardivo et al., 2005). In here, MB⋅ and MB⋅2+ represent the semi-reduced and semi-oxidized radicals of methylene blue, respectively; Ω1 denotes light absorption; Φf, Φnr, ΦT are fluorescence, non-radiative, and triplet quantum yield.
4.2 The mechanism of dye-sensitization process
The light that is typically applied in the visible and near-infrared wavelengths must stimulate photosensitizers in order to start the photodynamic process. The initial state of the photosensitizer is a singlet state in which paired electrons in frontier molecular orbitals have opposing spins (Zhao et al., 2021; Li and Pu, 2019). Upon exposure to light of the appropriately designated wavelength, the photosensitizers absorb the light, resulting in the excitation of a single electron from the highest occupied molecular orbital (HOMO) to the lowest unoccupied molecular orbital (LUMO), while the spin remains unchanged during the process (Rossi, 2023; Kenry and Liu, 2022). When the photosensitizer is exposed to light with the right wavelength, it absorbs the light and one electron is excited from HOMO to the LUMO, with the spin staying unaltered throughout. This excited state, which lasts for only a few nanoseconds at most, is referred to as the excited singlet state (Rossi, 2023; Kenry and Liu, 2022; Huang et al., 2014). The photosensitization mechanism, as illustrated in Figure 3, involves electrons from the dyes HOMO excited to their LUMO and then injected to the CB of TiO2. This process occurs once the dyes absorb photons in the visible light energy range of the solar spectrum (Li et al., 2020).
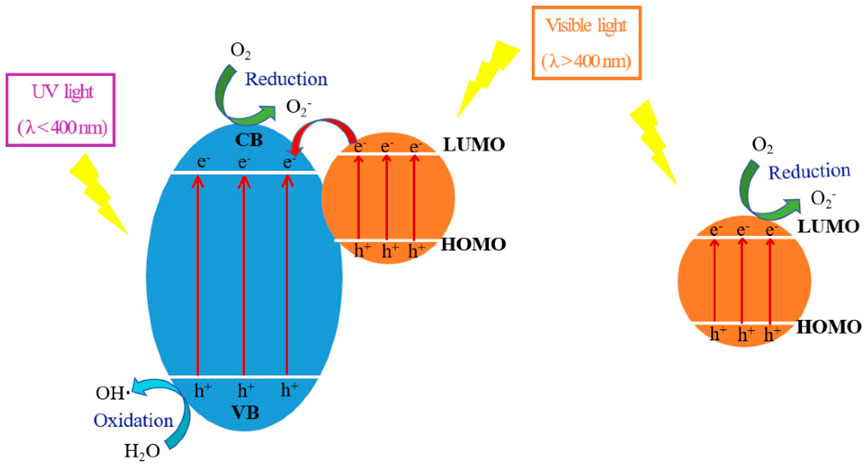
FIGURE 3. The mechanism of the dye sensitization process (Li et al., 2020).
The occurrence of this excited singlet state is pivotal in initiating subsequent photochemical reactions and is fundamental to the overall functionality of photosensitizers in diverse applications such as photodynamic therapy, solar energy conversion, and photocatalysis (Zhao et al., 2021; Li and Pu, 2019; Xu et al., 2022; Monro et al., 2019). In this context, the singlet-excited state of photosensitizers can manifest in different pathways. It may either emit light in the form of fluorescence or undergo radiationless relaxation, converting the excitation energy into heat internally (Ortiz-Rodríguez et al., 2021). Alternatively, the excited electron has the option to reverse its spin, a process that, for most organic compounds, is relatively slow and requires a singlet state with an extended lifetime. The majority of organic compounds exhibit a prolonged lifetime for the singlet state due to the sluggish nature of the spin reversal process (Ortiz-Rodríguez et al., 2021; Stoll and Schweiger, 2006; Li et al., 2022). It is noteworthy that the excited electron, having a parallel spin relative to its unexcited, paired electron, transitions into the triplet state, which boasts a considerably longer lifetime. However, the return of the excited electron to the ground state in the triplet state is constrained by the Pauli exclusion principle, as this process is considered “spin forbidden” (Stoll and Schweiger, 2006; Li et al., 2022; Minaev et al., 2022). Consequently, the excited electron in the triplet state cannot simply revert to the ground level, leading to a substantially prolonged lifetime for the triplet state (Minaev et al., 2022). These intricate dynamics play a pivotal role in determining the fate of the excited state and influence the subsequent photochemical and photophysical processes in which the photosensitizer is involved. The triplet state is much longer lived because the excited electron possesses a parallel spin to its paired, unexcited electron. In the triplet state, the excited electron cannot easily fall back to ground level (a “spin forbidden” process such as this would violate the Pauli Exclusion Principle) (Ortiz-Rodríguez et al., 2021; Lynch et al., 2019).
4.3 Dynamics of photodynamic processes
The PDT exhibits its distinctive oxidative damage through the capability of photosensitizers to interact with oxygen during their prolonged triplet state. Exposure to light triggers electron-hole pairs in TiO2, generating redox reactions that produce ROS, such as superoxide ions and hydroxyl radicals, which can damage organic pollutants in wastewater (Gilson et al., 2017). The extended triplet state of photosensitizers in PDT and the resulting oxidative damage pathways are analogous to the generation of ROS during the photocatalytic activity of TiO2 in wastewater treatment (Tanielian et al., 2003). The corresponding pathways are illustrated in Figure 4, delineating the ensuing photochemistry and potential mechanisms of damage to various targets. In this process, the photosensitizer, residing in its triplet state with an extended lifetime, engages in interactions with molecular oxygen. This interaction initiates a cascade of events leading to the generation of ROS, such as singlet oxygen.
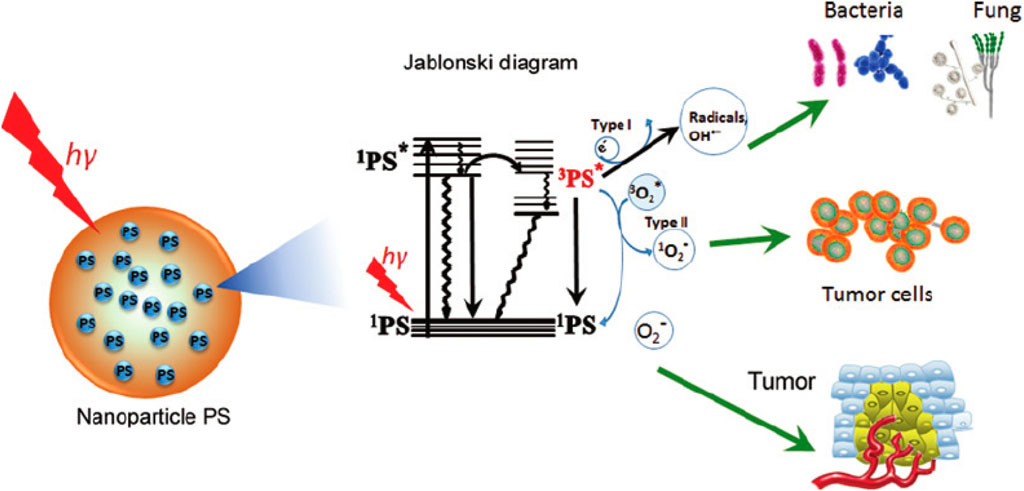
FIGURE 4. Photochemistry depicted in the Jablonski diagram (Das and Roychoudhury, 2014).
The ROS, particularly singlet oxygen, is highly reactive and can induce oxidative damage to biomolecules and cellular structures. These damaging effects are the basis for the therapeutic efficacy of PDT, as the targeted generation of ROS selectively damages cancer cells or other pathogenic targets (Das and Roychoudhury, 2014; Zapata et al., 2022; Abdal Dayem et al., 2017). The pathways depicted in Figure 4 provide a visual representation of the intricate photochemical processes and the subsequent mechanisms by which PDT induces oxidative damage to its intended targets (Das and Roychoudhury, 2014). Understanding these pathways is crucial for optimizing PDT protocols and expanding its applications in medical treatments and other fields. In the context of wastewater treatment, the oxidative damage caused by the extended triplet state of photosensitizers aligns with the oxidative degradation of pollutants facilitated by TiO2 photocatalysis (Lima and Reis, 2023; Zhao et al., 2021). The pathways illustrated in Figure 4, along with the resulting photochemistry and potential mechanisms of damage to various targets, could be conceptually linked to the intricate processes involved in the photocatalytic degradation of contaminants in water using TiO2 (Lima and Reis, 2023; Monro et al., 2019). Understanding these mechanisms aids in optimizing TiO2-based photocatalysis for effective wastewater treatment and pollutant removal.
4.4 Mechanism of TiO2-based photosensitization
The elucidation of photocatalytic mechanisms involving TiO2-based dye photosensitizers is crucial for a comprehensive understanding of how these systems work and for optimizing their performance in various applications (Schneider et al., 2014; Sakar et al., 2019; Chen et al., 2020; Serpone, 2018). Researchers have employed a combination of experimental techniques and theoretical modelling to uncover these mechanisms. Figure 5 shows the mechanism of the dye sensitization process with the dye adsorbed onto the catalyst surface and dye in the bulk.
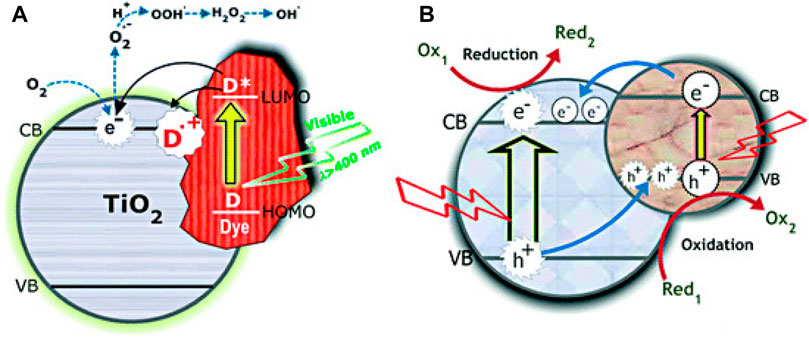
FIGURE 5. Mechanism of charge carrier transfer in semiconductor-coupled (A) narrow band gap TiO2 and (B) dye-sensitized TiO2 (Vinu and Madras, 2011).
Thiazine dyes play a critical role in extending the light absorption range of TiO2 (Rochkind et al., 2015; Bindhu et al., 2022). When exposed to light, thiazine dyes can absorb photons in the visible region, promoting electron excitation from their HOMO to their LUMO ground state to higher energy levels (Luo et al., 2021; Rochkind et al., 2015; Senthilkumar et al., 2010; Vara and Ortiz, 2016). Upon excitation, the thiazine dye molecules transfer electrons to the conduction band of TiO2. This injection of photoexcited electrons into TiO2 is facilitated by the difference in energy levels of the dye LUMO and the bandgap of TiO2, particularly its CB position, and this is a key step in the photocatalytic process (Khan et al., 2022; Rochkind et al., 2015; Senthilkumar et al., 2010; Parrino et al., 2022; Ronca et al., 2012; Mezyen et al., 2023). The injected electrons can then participate in redox reactions on the surface of TiO2 with adsorbed species or in the reduction of oxygen to form superoxide radicals. Simultaneously, when electrons are injected into the TiO2 conduction band, holes (positive charge carriers) are created in the valence band of TiO2 (Ronca et al., 2012; Elg et al., 2021). These holes can participate in oxidative reactions, such as the oxidation of water or organic pollutants, which is crucial for overall photocatalytic activity.
The efficiency of a TiO2-based dye sensitizer system depends on the extent of charge separation and minimization of electron-hole recombination. Photogenerated electrons in the conduction band and holes in the valence band should be efficiently separated to avoid recombination, which would lead to a decrease in photocatalytic activity (Elmorsy et al., 2023; Zani et al., 2021). Photogenerated electrons and holes can interact with adsorbed species, leading to the formation of intermediate radicals. These radicals can participate in the degradation of organic pollutants or in other chemical reactions. For example, superoxide radicals formed from oxygen reduction can contribute to the degradation of organic compounds. Oxygen and water are often present in photocatalytic reactions. Oxygen can accept electrons from the conduction band, forming superoxide or other oxygen radicals. Water can serve as an electron donor to reduce holes in the valence band (Chakhtouna et al., 2021; Goodarzi et al., 2023; Humayun et al., 2022; Li et al., 2013). These reactions are crucial for pollutant degradation and hydrogen production.
4.5 Probing photocatalytic dynamics
The interaction of thiazine dye-sensitized TiO2 with target molecules or pollutants on the surface is essential for the photocatalytic process (Zani et al., 2021; Senthilkumar et al., 2010; Barakat et al., 2022). Surface adsorption, electron transfer, and radical reactions play a significant role in the degradation or transformation of target compounds. Researchers often study the quantum yields of photocatalytic reactions to understand the efficiency of the process. Additionally, reaction kinetics are examined to determine rate constants, reaction pathways, and the role of intermediates (Senthilkumar et al., 2010; Araujo et al., 2022; Liu et al., 2014). To elucidate these mechanisms, researchers use techniques like transient absorption spectroscopy, electron paramagnetic resonance (EPR) spectroscopy, surface science experiments, and computational modelling, including density functional theory (DFT) calculations (Qin et al., 2023). These methods provide valuable insights into the dynamic processes occurring during photocatalysis, helping to optimize TiO2-based thiazine dye systems for various applications, including water treatment, pollutant degradation, and hydrogen production (Luo et al., 2021; Senthilkumar et al., 2010; Acar et al., 2015; Yang and Wang, 2018). Figure 6 demonstrates the application of EPR spectroscopy in TiO2and Nb2O5 photocatalysis.
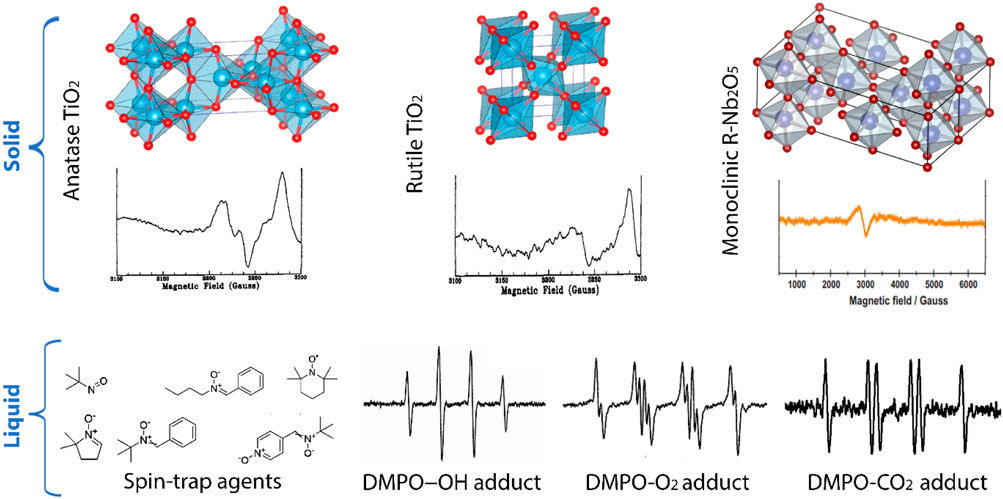
FIGURE 6. Application of EPR spectroscopy in TiO2 and Nb2O5 photocatalysis (Al-Madanat et al., 2021).
As shown in Figure 6, the application of EPR spectroscopy in the observation of paramagnetic centres formed upon irradiation of TiO2 and niobium oxide (Nb2O5) photocatalysts was reported by Al-Madanat et al. (2021). They showed that electron paramagnetic resonance spectroscopy is a powerful technique that can be used to monitor the photoinduced phenomena occurring in semiconductors like TiO2 and Nb2O5 photocatalysts. As such, the EPR spectroscopy provides precise insights into the dynamic and reactivity of the photocatalyst under different experimental conditions (Al-Madanat et al., 2021). It can characterize paramagnetic centers formed upon irradiation of these photocatalysts. The application of EPR in the observation of paramagnetic centers formed upon irradiation of TiO2 and Nb2O5 photocatalysts has been studied. The EPR spectroscopy differentiates itself from other techniques by providing exceptional sensitivity and specificity towards species with unpaired electrons, including organic and inorganic radicals, crystal defects, dopant atoms, and both free and trapped charge carriers (Al-Madanat et al., 2021; Hurum et al., 2003). The results obtained from EPR spectroscopy can help in understanding the electron-hole recombination, the nature of trapped charge carriers, and the reactions involved in the photocatalytic process.
4.6 Electron and energy transfer processes
Electron and energy transfer processes are fundamental mechanisms that play a critical role in a wide range of scientific and technological applications, including photophysics, photochemistry, and photobiology (Parrino et al., 2022). Understanding these processes is essential for optimizing various technologies and advancing our knowledge of chemical and physical systems. Electron transfer refers to the movement of an electron from one molecular entity (donor) to another (acceptor). This process can be categorized into two types such as chemical electron transfer, where the transfer of electrons is accompanied by changes in the chemical structure of the donor and acceptor (Beranek, 2011; Bayard et al., 2021). This process is often observed in redox reactions, where the donor loses an electron (oxidation), and the acceptor gains an electron (reduction). Meanwhile, photoinduced electron transfer (PET) refers to a special case of electron transfer that occurs upon absorption of light by a photosensitizer (Beranek, 2011; Speirs et al., 2023). In this process, the excited photosensitizer (electron donor) transfers an electron to an acceptor molecule. The PET is essential in various photophysical and photochemical reactions, such as fluorescence quenching and charge separation in photovoltaic devices (Speirs et al., 2023; Buglak et al., 2022; El-Khouly et al., 2004). The photosensitization mechanism and the role of electron energy transfer in photosensitizers, including the generation of ROS and the potential for tuning ROS generation through anionization are shown in Figure 7.
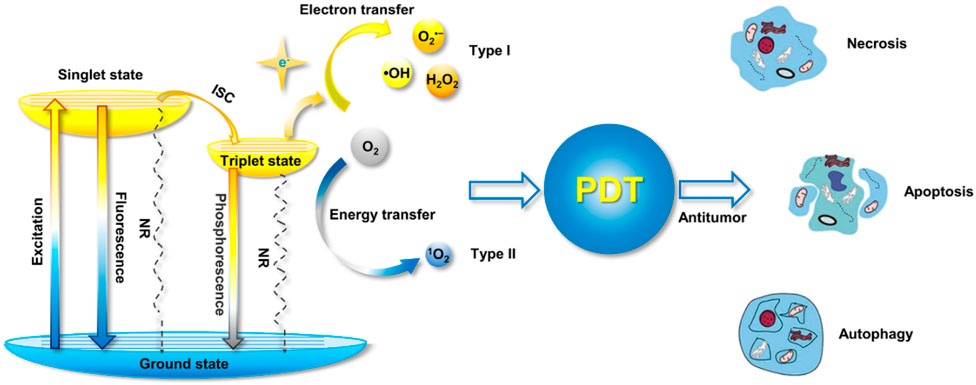
FIGURE 7. The Jablonski diagram shows the mechanism of ROS formation from photosensitizers, as well as the mechanisms underlying PDT-induced cell death (Yu et al., 2022).
As shown in Figure 7, the photosensitizers undergo excitation upon absorbing a photon, transitioning from the singlet ground state to a higher-energy state. In this regard, the energy is transferred from one molecular entity to another without an actual transfer of electrons. Hence, in photosensitizers, energy transfer occurs through the process of intersystem crossing (Yu et al., 2022). There are two main types of energy transfer, which are Radiative Energy Transfer (Forster Resonance Energy Transfer, (FRET)) and Non-Radiative Energy Transfer (Dexter Energy Transfer (DET)) (Calçada et al., 2019; Enhanced Photodynamic et al., 2022; van der Meer, 2013; Tavakkoli Yaraki et al., 2022). The FRET is an Radiative Energy Transfer process that occurs between two molecules (usually chromophores) nearby. When the energy levels of the donor and acceptor molecules are appropriately matched, energy can be transferred from the excited donor to the acceptor (Calçada et al., 2019; van der Meer, 2013). FRET is widely used in applications like fluorescence microscopy, molecular biology, and energy transfer-based sensors. While the dexter energy transfer is a non-radiative process, in which energy is transferred between molecules through electron-electron interactions without the emission of a photon (Chou and Dennis, 2015), (Sekar and Periasamy, 2003). As a result, this process is more distance-dependent than FRET and typically requires closer proximity between donor and acceptor molecules.
The rate and efficiency of both electron and energy transfer processes depends on various factors, such as the degree to which the electronic states of the donor and acceptor molecules overlap the distance between donor and acceptor, the solvent polarity, temperature, and surrounding conditions. The quantum yield represents the probability of a transfer event occurring and is a measure of the efficiency of the process (Sekar and Periasamy, 2003), (DeVine et al., 2015; Kubas, 2021; Roduner and Krüger, 2022). Electron and energy transfer processes are essential in many applications, such as light-harvesting systems in organic photovoltaic cells and solar panels, photoluminescence and fluorescent labelling techniques, sensors and probes for detecting molecular interactions and electron transport in biological systems, including respiration and photosynthesis (DeVine et al., 2015; Kaushal et al., 2023; Wang and Lu, 2022; Chen et al., 2015). Therefore, understanding and controlling electron and energy transfer processes have led to numerous technological advancements and continue to be areas of active research in chemistry, physics, and biology. These processes are crucial for the development of sustainable energy technologies, improved sensors and imaging techniques, and a deeper understanding of biological and chemical systems.
5 Advantages and disadvantages of using thiazine dyes as photosensitizers
5.1 Thiazine dye toxicity in water
Thiazine dyes are a class of synthetic organic dyes that contain a thiazine ring structure as shown in Figure 1. These dyes are commonly used in various industrial applications, including textile dyeing, paper colouring, and as biological stains. The toxicity of thiazine dyes in water can vary depending on the specific dye and its chemical composition (Handbook of Green and Sustainable Nanotechnology, 2023; Rani and Shanker, 2023; Abilaji et al., 2023). In general, some thiazine dyes may pose environmental and health risks due to their potential toxicity. Many synthetic dyes, including MB dyes, are known to be persistent in the environment and may be resistant to biodegradation. Consequently, they can accumulate in water bodies, leading to potential adverse effects on aquatic ecosystems (Selvaraj et al., 2021; Zosenko et al., 2022). Scheme 2 outlines general considerations regarding the potential toxic effects of thiazine dyes.
To assess the toxicity of a specific thiazine dye, it is necessary to consider factors such as its chemical structure, concentration in water, and the sensitivity of the organisms present in the aquatic environment. Toxicity studies and risk assessments are typically conducted to evaluate the potential harm of specific chemicals, including dyes, to aquatic organisms and human health (Oladoye et al., 2022; Khan et al., 2023). It is important to note that regulations and guidelines exist to limit the release of certain dyes into water bodies to mitigate potential environmental and health risk.
5.2 Applications of thiazine dyes in water treatment
Thiazine dyes, particularly exemplified by methylene blue, are recognized as versatile organic dyes with well-established applications in various scientific and technological domains. Their significance extends across chemical, biological, and medical applications, making them indispensable in these fields. In the realm of wastewater treatment, thiazine dyes such as methylene blue play a crucial role in addressing environmental concerns (Paul and Suresh Kumar, 2013). Notably, thiazine dyes exhibit unique properties, including solva-tochromism and metachrosis, making them highly sensitive to changes in their surroundings. Additionally, their high singlet oxygen quantum yields contribute to their efficacy in specific applications. In the context of wastewater treatment, these dyes can be strategically modified to create derivatives with enhanced functionality, allowing for the introduction of new substituents at the nitrogen or CH groups (Montalti et al., 2006; Talman and Atun, 2006). As such, thiazine dyes, like many other synthetic dyes, have both advantages and disadvantages in various applications, including their use in water-based systems. Scheme 3 outlines of some potential advantages and disadvantages of thiazine dyes in water.
The majority of dye producers and consumers, especially those in the textile industry, discharge large amounts of wastewater that contains dye in amounts between 0.001 and 0.7% w/v (see Figure 8) (Langhals and Zollinger, 2004). A review of papers on textile industry wastewater treatment for the removal of different dyes revealed that advanced oxidation processes, such as photocatalysis and Fenton reactions, showed the highest colour removal efficiency (GilPavas et al., 2018; Rashid et al., 2021). However, the research did not clearly demonstrate the impact of green technologies on energy consumption, carbon footprint, and waste generation. New technologies need to be developed and evaluated in a sustainable context with real wastewater. The denim textile industry generates wastewater with persistent pollutants, which can be toxic and carcinogenic. Wastewater treatment is important to reduce risks to aquatic life and public health (GilPavas et al., 2018; Rashid et al., 2021; Kishor et al., 2021).
As shown in Figure 8, the textile dyeing and finishing processes involve the extensive use of dyes, and the wastewater generated during these processes often contains significant amounts of dye residues. The most widely used thiazine dyes i.e., MB is applied in dye silk, cotton, and wood. The wastewater containing dyes is characterized by elevated alkalinity, low biodegradability, total dissolved solids (TDS), chemical oxygen demand (COD), and biochemical oxygen demand (BOD) (Srinivasan and Viraraghavan, 2010). Since dye is colourful, it is simple to detect its presence in water bodies, and it easily blocks sunlight and eventually the water bodies ability to reoxygenate thereby interfering with aquatic life's biological processes. Industrial dye effluent can be colored-free using a variety of techniques. However, due to the wide variety of dyes on the market and the presence of additional chemicals in industrial effluent, many treatment techniques may not be effective when used alone and may need to be combined with other techniques to remove most of the colouring. There are three types of dye wastewater treatment methods viz., chemical, biological, and physical treatments (Crini and Lichtfouse, 2019; Hung et al., 2017; Hung et al., 2020). When not properly treated, this wastewater can be discharged into rivers and other water bodies, causing environmental pollution thiazine dye derivatives, when employed as photosensitizers in PDT processes, offer several advantages. These include high selectivity, resulting in reduced toxicity to healthy tissue, improved cosmetic outcomes, dual functionality during application, a lower risk of infection, absence of organ damage, diminished likelihood of inducing resistance, and minimal side effects such as infertility. Moreover, thiazine photosensitizers in PDT hold promise for combination therapies due to their unique method of action, which makes them resistant to many common chemotherapeutic resistance mechanisms (Callaghan and Senge, 2018; Senge and Radomski, 2013; Spring et al., 2015). Despite these strengths, PDT faces challenges in becoming the primary treatment choice for certain tumours, primarily due to limitations in tissue penetration, light dosage management, and photosensitivity. Nevertheless, PDT remains a clinically useful therapy, and there is a pressing need for innovative approaches to enhance existing therapies. This involves accelerating the development of the third or fourth generation of thiazine photosensitizers to overcome current limitations and potentially revolutionize treatment modalities in relevant fields. Efforts should focus on refining existing methodologies, exploring novel techniques, and fostering collaboration between disciplines to drive transformative advancements in the application of thiazine dyes, such as methylene blue, in wastewater treatment and related areas (Spring et al., 2015).
5.3 Application of thiazine dyes in dye-sensitized solar cells
Thiazine dyes, such as phenothiazine dyes, have limitations that restrict their further optimization for high-efficiency DSSCs (Buene and Almenningen, 2023). However, recent studies have shown the importance of these dyes in achieving higher photovoltaic conversion efficiency (Han et al., 2023). For example, N-phenylphenothiazine dyes with different π-bridges have demonstrated improved JSC and VOC, leading to higher overall efficiency in DSSCs (Hirakawa and Mori, 2021). Additionally, the inclusion of auxiliary acceptors, such as benzothiadiazole, in phenothiazine-based dyes has been shown to broaden their spectral response range and improve device performance (Li et al., 2021). These findings suggest that thiazine dyes can still play a role in sustainable environmental solutions, particularly in the development of highly efficient DSSCs (Wang et al., 2022). However, it is important to consider the potential secondary pollution caused by these dyes and explore alternative options for sustainable environmental solutions. Thiazine dyes have garnered considerable attention for their unique properties, making them promising candidates in various aspects of solar energy conversion. From enhancing light absorption to serving as sensitizers in DSSCs, thiazine dyes play a crucial role in advancing the efficiency and versatility of solar cell technologies (Buene and Almenningen, 2023; Han et al., 2023; Li et al., 2021). Table 3 provides an overview of key applications, showcasing the potential of thiazine dyes across different solar cell architectures and technologies.
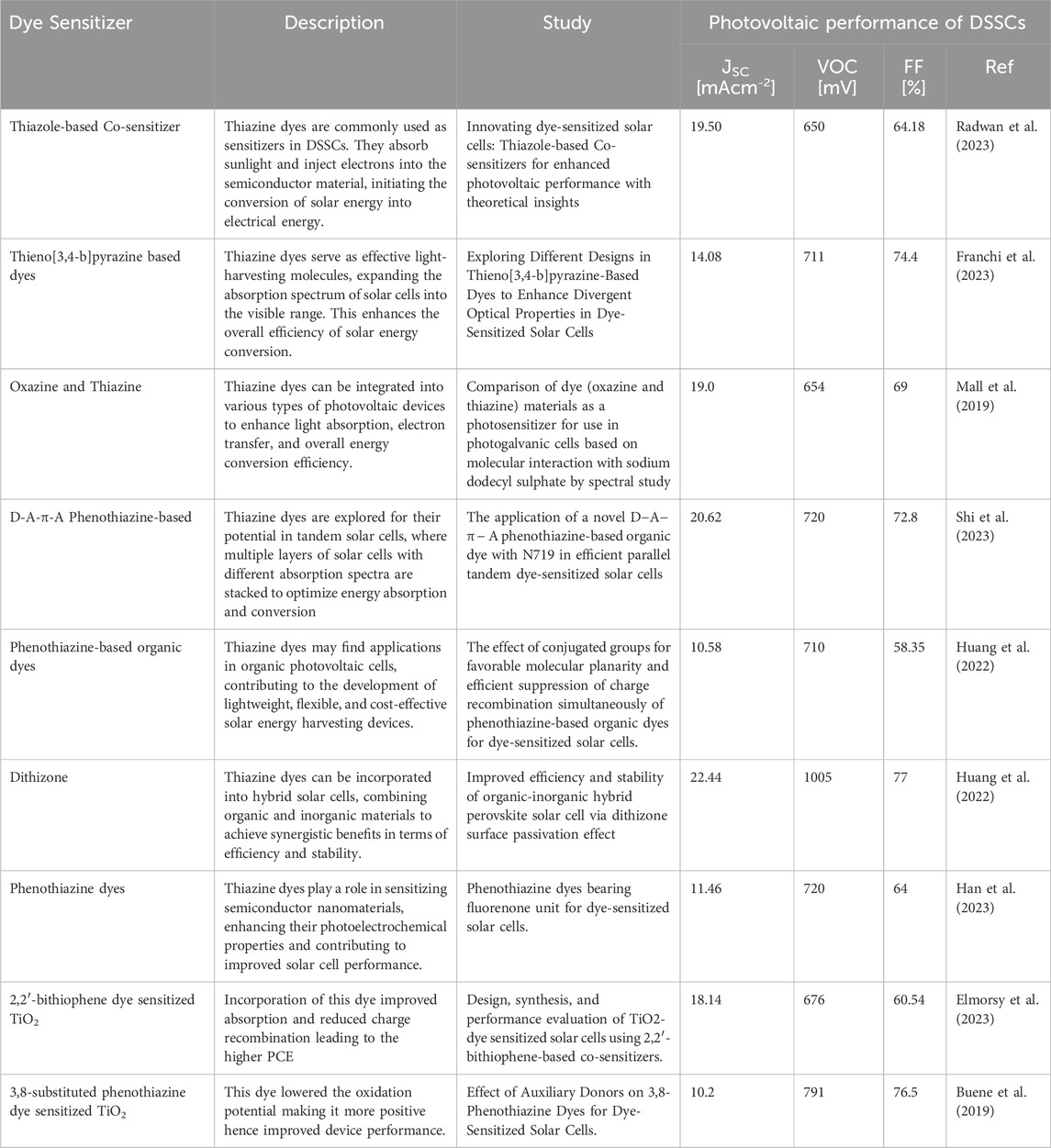
TABLE 3. Summary of different types of dye sensitizers used in various photovoltaic solar cell devices
As shown on the table, different types of dye sensitizers used in various photovoltaic solar cell devices underscores the diverse strategies employed to harness solar energy for electricity generation. Organic dyes, inorganic dyes, and perovskite materials represent distinct categories of sensitizers, each with unique advantages and challenges (Li et al., 2022; Rani and Shanker, 2023; Takahashi et al., 2023). Organic dyes, characterized by their tunable chemical structures, offer versatility and ease of modification. They have been extensively used in DSSCs, demonstrating good light absorption and electron injection capabilities. However, challenges related to stability and limited absorption in the red and near-infrared regions have prompted exploration into alternative sensitizers (Liu et al., 2023; Li et al., 2021; Lee et al., 2017).
Inorganic dyes, particularly metal complexes like ruthenium-based compounds, exhibit remarkable photostability and extended absorption spectra (Roy et al., 2023). Their utilization in DSSCs has shown significant efficiency improvements, yet concerns regarding the scarcity and cost of some metal components stimulate ongoing research for more sustainable alternatives. Perovskite materials have emerged as a groundbreaking class of sensitizers, demonstrating rapid advancements in photovoltaic technology (Takahashi et al., 2023). Perovskite solar cells exhibit high efficiency, low-cost fabrication, and a broad absorption spectrum. However, issues related to stability, toxicity, and scalability remain focal points of research efforts.
6 Synthesis and modification of TiO2-based dye photosensitizers
6.1 TiO2-based dye photosensitizers
The TiO2 structure, morphology, and particle size are known to have a significant impact on its optical properties and, consequently, its photoactivity. There are several methods for achieving titanium dioxide photosensitizers, including surface modification using the right species (Moma and Baloyi, 2018). A visible-light-induced electron or hole injection into the conduction or valence band, respectively, is necessary for the photosensitization process (Hamza et al., 2023; Moma and Baloyi, 2018; Dlamini et al., 2021). The photosensitizer moiety's (surface complex) electrical interaction with the TiO2 particle determines the process's efficiency. Quantum yields of photoinduced electron transfer processes are also impacted by surface modification of TiO2 (Hamza et al., 2023; Elmorsy et al., 2023; Monika et al., 2023). Specifically, TiO2 photosensitization attracts a lot of interest because this material is used as a photoactive material in photovoltaics, optoelectronics, and photocatalysis (Hagfeldt and Grätzel, 2000; Moser et al., 1998; Szaciłowski et al., 2005).
At the surface of TiO2, several Ti(IV) complexes are generated in situ. There may be correlations between the application of TiO2 phonium and the structures of Ti(IV) complexes, particularly those involving oxygen-ligands (Hug and Bahnemann, 2006). In addition, there are bidentate bridging structures made up of chelating ligand binding two nearby Ti(IV) centres, which is the bidentate chelating structure with the ligand occupying two coordination sites. One oxo ligand must be substituted for other titanium centers coupled with five ligands to create a bidentate chelating complex, although monodentate or bridging complexes can be formed by any of the five surfaces Ti(IV) sites. Meanwhile, the monodentate complexes with coordination between aliphatic alcohols and the surface of titanium dioxide is extensively researched, primarily due to its significant impact on photocatalytic reactions that take place when these molecules are present. The hydroxyl group on the surface of TiO2 facilitates the physical (molecular) and chemical adsorption of simple aliphatic alcohols, including methanol, ethanol, and 1- and 2-propanol (Kim et al., 1988; Lusvardi et al., 1995). On the other hand, both phenol and 4-chlorophenol bind to the TiO2 surface with a moderate affinity (Lusvardi et al., 1995; Al-ekabi et al., 1989). The complexes that are produced exhibit poor stability constants. The incapacity of phenol and 4-chlorophenol to form ring-structured surface complexes could be the cause of the observed weak chemisorption.
As summarised in Table 1, bidentate complexes are formed only when the ligand has two donor group photosensitizers or one group with two donor atoms. Physisorption and the monodentate complexation mode are not excluded by the potential for bidentate structures to be formed. Polynuclear complexes results through anchoring of the group photosensitizers, in which different organometallic complexes of transition metals may be attached to the titanium surface centers. In this regards, the carboxyl group photosensitizers can act as anchors leading to the formation of a polynuclear complex with bridging ligands that can coordinate with Ti(IV) and other metal ions. There are many methods used to prepare TiO2-based material to be used as sensitizers reported in the literature coated on fluorine-doped tin oxide (FTO) glass substrate as thin films. Vinaayak et al. (2022), used a doctor-blade approach to coat TiO2 nanoparticles on an FTO substrate as a photoanode. Meanwhile, Dhanasekaran and Marimuthu (2023), reported the synthesis of TiO2 paste from TiO2 nanopowders, actylacetone, and nitric acid. It was Alaya et al. (2023), who prepared TiO2 thin films on FTO glass substrates using spray pyrolysis deposition and annealed them at different temperatures for at least 3 hours per thin film. The preparation of these TiO2 materials was followed by treatment with organic materials such as thiazine dyes, or plant extracts to generate TiO2-based sensitizers.
6.2 Thiazine and methylene blue-based photosensitizers
Thiazine dyes known as phenothiazines have two benzene rings condensed to the thiazine ring in addition to a central thiazine core. These dyes have lately been explored as photosensitizers due to their biological, photochemical, and photophysical properties (Padnya et al., 2023) with an emphasis on the inactivation of bacterial, viral, and parasite strains (Vara and Ortiz, 2016; Almeida et al., 2020; Shen et al., 2020). Methylene blue and toluidine blue O are two of the most well-known phenothiazines. Methylene blue has been used in clinical settings to treat bacterial infections, including dental conditions, and has shown to be safe, effective, and aesthetically pleasing (Shen et al., 2020; Theodoro et al., 2021). Moreover, methylene blue derivatives, or 3,7-disubstituted dyes of the phenothiazine nucleus, have been produced and functionalized at these sites with a variety of amines for further research into photobiological and optoelectronic uses (Lusvardi et al., 1995). Some compounds derived from phenothiazines are still developed within the purview of their application in cancer PDT, albeit being less prevalent than their antibacterial action. One of the most appealing instances happens when the conjugation of methylene blue with camptothecin via an activatable linker containing a disulfide bond that is prone to breakage by GHS (Yang et al., 2022). When administered in vivo, no physiological toxicity was observed, indicating safety and biocompatibility.
Thiazine-based photosensitizers hold significant promise for innovative and effective wastewater treatment strategies. These photosensitizers, derived from thiazine dyes like methylene blue, exhibit unique characteristics that can be harnessed for environmental remediation purposes (Vara and Ortiz, 2016; Bindhu et al., 2022). As such, thiazine dyes are well known for their versatility and well-established applications in various scientific fields. When applied as photosensitizers in wastewater treatment processes, these compounds can demonstrate exceptional photodynamic properties (Bindhu et al., 2022). The key attributes that make thiazine and methylene blue-based photosensitizers advantageous in this context include high sensitivity to environmental changes, which thiazine dyes, with their solva-tochromism and metachrosis properties, become highly responsive to alterations in the surrounding environment. This sensitivity is crucial for detecting and targeting contaminants in wastewater (Luo et al., 2021; Acar et al., 2015; Bindhu et al., 2022). The high singlet oxygen quantum yields of methylene blue, contribute to their efficacy in inducing photodynamic reactions. This property is particularly beneficial for the degradation of organic pollutants in wastewater.
In this regard, the thiazine-based photosensitizers can also be tailored to selectively target specific contaminants in wastewater (Thandu et al., 2015). This selectivity minimizes the impact on non-target organisms and reduces the overall environmental toxicity of the treatment process. As such, the use of thiazine and methylene blue-based photosensitizers ensures low toxicity in water, which is a critical factor for sustainable and environmentally friendly wastewater treatment practices (Kenry and Liu, 2022; Thandu et al., 2015). They also offer the potential for combination therapies, allowing for synergistic approaches to enhance wastewater treatment effectiveness. This can involve coupling photodynamic processes with other treatment methods to address a broader range of contaminants. The unique method of action of thiazine photosensitizers makes them less prone to common chemotherapeutic resistance mechanisms (Kenry and Liu, 2022; Barakat et al., 2022; Thandu et al., 2015). This characteristic enhances their reliability and effectiveness in the treatment of wastewater. While thiazine-based photosensitizers present these advantages, it is essential to acknowledge the existing challenges in wastewater treatment, such as the need for optimized dosages, penetration depth, and potential photosensitivity issues (Barakat et al., 2022; Anjum et al., 2019). Ongoing research and development efforts should focus on refining the application methodologies, exploring novel delivery systems, and addressing these challenges to maximize the potential of thiazine-based photosensitizers in wastewater treatment technologies. Through harnessing the unique properties of these compounds, we can advance towards more sustainable and efficient approaches for treating wastewater and safeguarding our environment (Barakat et al., 2022). This led to the conclusion thiazine dye-sensitized TiO2 could overcome the limits of both camptothecin and methylene blue in terms of their uniqueness.
7 Enhanced photocatalytic applications
Photocatalysis, the process of using a semiconductor material to harness light energy and drive chemical reactions, has gained significant attention in recent years due to its potential to address environmental and energy-related challenges (Jing et al., 2023). Titanium dioxide has emerged as one of the most widely studied photocatalysts, owing to its stability, low cost, and excellent photoactivity in the ultraviolet (UV) region (Magalhães et al., 2017; Yang and Wang, 2018; Lakhera and Neppolian, 2021). The incorporation of MB dyes with TiO2 for photocatalytic applications has been explored for its ability to enhance light absorption and promote specific chemical reactions (Gonuguntla et al., 2023). However, MB dye remains a health hazard to the environment. Figure 9 demonstrates the efficiency of photocatalytic degradation of organic dyes by AgNP/TiO2/Ti3C2Tx MXene composites under UV and solar light.
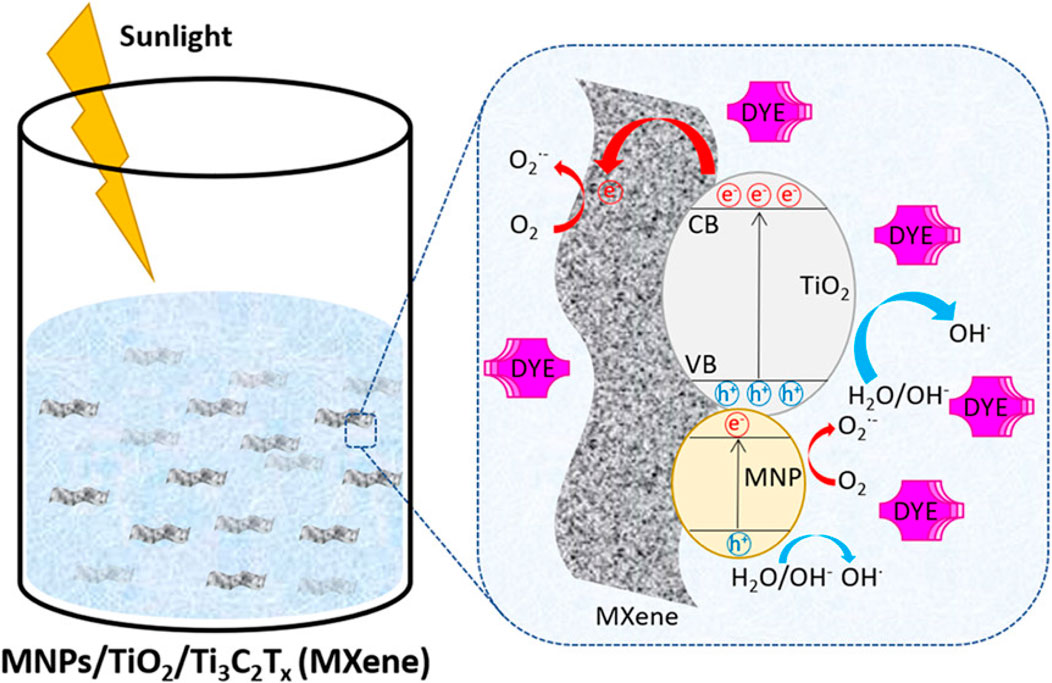
FIGURE 9. Efficient photocatalytic degradation of organic dyes by Silver nanaoparticles/TiO2/Ti3C2Tx MXene composites under UV and visible light (Othman et al., 2021).
The degradation of organic pollutants in water is a crucial aspect of water treatment. Thiazine dyes, which are often used in the textile industry and can be harmful to the environment, can be effectively removed through photocatalysis using TiO2-based systems. Studies have focused on the degradation of methylene blue, rhodamine B, and other thiazine dyes (Vara and Ortiz, 2016; Acar et al., 2015). Researchers have investigated the optimal conditions for photocatalytic water treatment, including dye concentration, pH, and TiO2 catalyst loading. The process not only degrades the dyes but can also lead to the formation of less harmful or non-toxic intermediates.
Beyond thiazine dye removal, TiO2-thiazine dye systems have been extended to the degradation of other organic pollutants. Barakat et al. (2022) successfully used methylene blue dye as a photosensitizer in the water photo-splitting process, which exhibited enhancement in the hydrogen and oxygen production rates under visible light radiation. As such, the hydrogen and oxygen obtained were in good stoichiometric rates (Barakat et al., 2022). The SiO2 nanoparticles incorporated into nanostructured TiO2 was observed to greatly enhance dye photodegradation (Barakat et al., 2022; Senthilkumar et al., 2010). The use of use of methylene blue as a photosensitizer in the form of a methylene blue derivate MB2, which was encapsulated or grafted onto ultrasmall silica nanoparticles for photodynamic therapy applications was reported by Kohle et al. (2020). They found out that singlet oxygen quantum yields improved with particle designs while the encapsulation improved photostability.
Various organic compounds, such as pharmaceuticals, pesticides, and industrial effluents, have been studied in the context of AOP. The synergistic effect of the dye sensitizer with TiO2 enhances the degradation efficiency of these pollutants under UV or visible light irradiation. Figure 10 demonstrates advanced oxidation processes in the removal of organic substances from produced wastewater: potential, configurations, and research needs
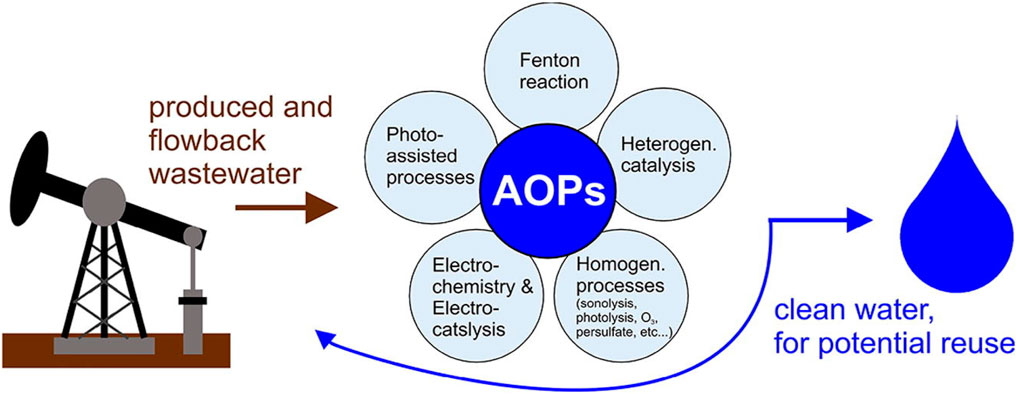
FIGURE 10. Advanced oxidation processes in the removal of organic substances from produced water: Potential, configurations, and research needs (Coha et al., 2021).
Photocatalytic water splitting is a promising method for hydrogen production, which is a clean and renewable energy source. TiO2-based photocatalysts, with or without dye sensitizer, have been investigated for their ability to generate hydrogen gas through water splitting (Tentu and Basu, 2017; Villa et al., 2021). The presence of dye sensitizer can extend the absorption range of TiO2 into the visible region, increasing the overall efficiency of the process. Understanding the mechanisms underlying TiO2-based dye sensitizer systems is essential for optimizing their performance (Elmorsy et al., 2023; Zani et al., 2021). Studies have employed various techniques, including transient absorption spectroscopy, EPR, and DFT calculations, to gain insights into the charge transfer processes, radical formation, and energy levels of the materials involved (Qian et al., 2019; Bonke et al., 2021).
TiO2 nanoparticles have been extensively studied for their photocatalytic activity in the degradation of toxic dyes and typical application are summarised on Table 4. As shown on the table, the addition of fluorine and tin dopants to TiO2 nanoparticles has been shown to enhance their photocatalytic activity (Bindhu et al., 2022). The doped TiO2 nanoparticles exhibit a reduced crystallite size and an increased band gap, indicating improved photochemical activity (Bindhu et al., 2022; Zioui et al., 2022). The morphologies of TiO2 nanoparticles also change significantly with the addition of fluorine and tin dopants (Bindhu et al., 2022). These doped TiO2 nanoparticles have been found to be effective in the degradation of methylene blue dye under both visible and UV light irradiation. Additionally, nanocomposite membranes based on chitosan biopolymer containing TiO2 nanoparticles have been developed and shown to have high photocatalytic activity in the degradation of tartrazine dye under solar light irradiation (Zioui et al., 2022). Overall, TiO2 nanoparticles, especially when doped with fluorine and tin, have shown promise in the photocatalytic degradation of thiazine dyes.
Table 4 presents a comprehensive summary of TiO2-based photocatalytic applications in dye removal, highlighting key studies and outcomes in the realm of environmental remediation. This compilation offers a concise overview of the diverse approaches and methodologies employed in utilizing TiO2 as a photocatalyst for the degradation and removal of various dyes from aqueous solutions. The studies included cover a spectrum of dyes, showcasing the versatility and effectiveness of TiO2-based photocatalysis in addressing environmental challenges associated with dye pollutants.
As shown on the table, the ZnO-TiO2 and ZnO-TiO2-reduced graphene oxide (ZT-rGO) nanocomposites were synthesized using a pulse laser ablation protocol (Manda et al., 2023). The effect of reduced graphene oxide (rGO) loading on the crystalline nanostructures, thermal stability, and removal of methylene blue dye was investigated by Manda et al. (2023). The ZT-rGO 5 nanocomposite showed the best photocatalytic activity, with a preferential rate constant of 0.149 min-1 and 98.5% methylene blue removal within 30 minutes. The rGO component in the nanocomposites acts as an electron trap, enhancing the formation of holes and contributing to the photocatalytic process (Manda et al., 2023). Chaudhari et al. (2022) conducted a novel TiO2CaIn2S4rGO composites (rGO-C1 to rGO-C3) synthesis for rapid degradation of organic dye. In their study, the rGO-C2 exhibited significant photocatalytic degradation activity for MB CR within 15-30 min. Analysis by the LC-MS technique discovered the degradation pathway of both dyes. As such, the rGO-C2 catalyst showed excellent degradation activity for real wastewater from the textile industry and landfill leachate (Chaudhari et al., 2022). A study by Song et al. (2023) reported that the direct band gap of the titanium dioxide carbon (TiO2C-550) composite was 2.7 eV, which lead to the improved photodegradation performance of MB under visible light irradiation. As such, the degradation ratio of MB aqueous solution reached nearly 100% within 30 minutes in the presence of TiO2C-550 composites, and the efficiency was maintained at about 95% after 5 cycles (jie Song et al., 2023). The Au-TiO2 nanospindles were found to be the most efficient catalyst for photocatalytic degradation of MB, with a pseudo-first order reaction rate of 0.1570 min-1 by Khalil et al. (2019). They showed that TiO2 nanospindles exhibited superior photocatalytic performance compared to TiO2 nanocubes, which was primarily due to the exposure of the (001) crystal facet (Khalil et al., 2019).
It is clear that researchers have explored different approaches to modify TiO2 and thiazine dyes to enhance their photocatalytic properties. Other modifications include doping TiO2 with various elements, such as nitrogen or metal ions, and designing new thiazine dyes with tailored properties. Such modifications aim to extend the absorption range, improve charge separation and transportation, and increase the overall efficiency of the photocatalytic systems (Chakhtouna et al., 2021; Humayun et al., 2018). The exploration of photocatalytic processes using TiO2-based dye sensitizer systems has shown great promise in addressing various environmental and energy-related challenges. The field continues to evolve, with researchers focusing on optimizing materials and conditions, gaining a deeper understanding of the mechanisms involved, and expanding the range of applications (AlSalka et al., 2023; Anucha et al., 2022; Zani et al., 2021; Tentu and Basu, 2017). Further research is needed to bridge the gap between laboratory-scale experiments and practical, large-scale implementations for water treatment, pollutant degradation, and hydrogen production. Comparison of the performance of thiazine dye-sensitized TiO2 with other sensitization strategies still require extensive exploration.
8 Future directions and prospects TiO2-based thiazine dye photosensitizers
This comprehensive review meticulously delves into the current challenges and future prospects of TiO2-based thiazine dye sensitizers, aiming to significantly enhance their performance and broaden their applicability. Overcoming the formidable challenge of achieving optimal photocatalytic efficiency in TiO2-based thiazine dye systems necessitates a nuanced understanding of the intricate dynamics involved in charge carrier generation, separation, transportation, and the reduction of electron-hole recombination. Innovative strategies, including fine-tuning the chemical composition of thiazine dyes, optimizing TiO2 morphology, and integrating co-catalysts, offer avenues for addressing existing drawbacks. Elucidating the detailed mechanisms and dynamics of TiO2-based thiazine dye photosensitization is crucial for successful optimization, although the complexity of these processes poses a significant challenge.
The stability and longevity of TiO2-based thiazine dye systems emerge as critical factors for sustained photocatalytic activity. Effectively addressing challenges such as dye desorption, catalyst radiation, and the loss of active sites over time requires exploration into surface modification techniques and material engineering. This involves the development of new materials or coatings to mitigate issues related to catalyst degradation and dye desorption. Despite the efficacy of thiazine dyes in photocatalysis, concerns regarding potential toxicity necessitate rigorous toxicity studies and risk assessments. Concurrently, researchers actively explore alternative dye structures or modifications to mitigate environmental and health risks associated with these dyes.
The scalability of TiO2-based thiazine dye photosensitizers for practical applications introduces challenges related to cost, efficiency, and feasibility. Therefore, collaboration between researchers and industry partners is essential to work towards scalable synthesis methods, cost-effective production, and exploring viable applications, ensuring the practical implementation of TiO2-based thiazine dye systems. Addressing these multifaceted challenges demands a multidisciplinary approach encompassing materials science, chemistry, and engineering. Ongoing research efforts are dedicated to overcoming these challenges, propelling TiO2-based thiazine dye systems towards their full potential for sustainable and effective environmental applications. This interdisciplinary journey holds the promise of delivering innovative solutions and ushering in a new era of environmental technologies.
9 Conclusion
TiO2-based dye photosensitizers have shown potential to enhance the performance of TiO2-based photocatalysis, particularly in applications such as water splitting to produce hydrogen and pollutant degradation in wastewater. The presence of thiazine dye-sensitizer extends the absorption range of TiO2 into the visible region, increasing overall efficiency as a photocatalyst or a light adsorbing material in photovoltaic cells. Various modifications, including doping TiO2 with elements like nitrogen or metal ions, and designing tailored thiazine dyes, aim to extend adsorption range, which inherently leads to improved charge carrier generation, separation, and transportation, and increase overall efficiency of the photocatalytic systems. Mechanistic insights into charge transfer processes, radical formation, and energy levels of the materials involved have been gained through techniques such as transient absorption spectroscopy, electron paramagnetic resonance, and density functional theory calculations. The exploration of TiO2-based dye sensitizer systems continues to evolve, with researchers focusing on optimizing materials and conditions, expanding the range of applications, and bridging the gap between laboratory-scale experiments and large-scale implementations. In this regards, the use of TiO2-based photosensitizers shows promise in enhancing the photocatalytic removal of thiazine dyes, such as methylene blue, from wastewater, thereby addressing environmental remediation and energy conversion needs. Thiazine dyes, when coupled with TiO2, act as effective photosensitizers, promoting electron transfer and generating reactive oxygen species that initiate chemical reactions, making them valuable in applications like wastewater treatment and solar energy conversion. However, it is important to acknowledge the potential drawbacks of thiazine dyes, including toxicity and non-biodegradability. Non-radiative energy transfer processes, such as Förster resonance energy transfer and dexter energy transfer, play a significant role in fluorescence microscopy, molecular biology, and energy transfer-based sensors. Electron Paramagnetic Resonance spectroscopy provides exceptional sensitivity and specificity in characterizing paramagnetic centers formed upon irradiation of photocatalysts like TiO2, aiding in understanding electron-hole recombination and the nature of trapped charge carriers. Efforts should focus on refining existing methodologies, exploring novel techniques, and fostering collaboration between disciplines to enhance TiO2-based thiazine photosensitizers and revolutionize treatments modalities in wastewater and related areas. Therefore, TiO2-based thiazine dye photosensitizers hold promise for improving the efficiency and expanding the applications of TiO2-based photocatalysis, with ongoing research aimed at further optimization and practical implementations.
NB: This review aims to provide a comprehensive understanding of TiO2-based dye photosensitizers and their role in enhancing the photocatalytic activity of TiO2, with a focus on applications in environmental remediation and renewable energy generation. As such, it will be valuable for researchers, chemists, and engineers interested in harnessing visible light for efficient and sustainable photocatalytic processes.
Author contributions
NC: Conceptualization, Formal Analysis, Funding acquisition, Investigation, Project administration, Resources, Writing–original draft, Writing–review & editing. RM: Conceptualization, Writing–review & editing. SN: Conceptualization, Writing–review & editing. MR: Funding acquisition, Project administration, Supervision, Writing–review & editing.
Funding
The author(s) declare financial support was received for the research, authorship, and/or publication of this article. The work was supported by Advance Materials Division-Catalysis Group through Mintek Science Vote Funding Program. The funder was not involved in the study design, collection, analysis, interpretation of data, the writing of this article, or the decision to submit it for publication.
Acknowledgments
The authors would like to thank Advanced Materials Division (AMD), Mintek for financial support.
Conflict of interest
Authors NC, RM, SN, and MR were employed by company Mintek. The editor TM declared a past co-authorship with the author MCR.
Publisher’s note
All claims expressed in this article are solely those of the authors and do not necessarily represent those of their affiliated organizations, or those of the publisher, the editors and the reviewers. Any product that may be evaluated in this article, or claim that may be made by its manufacturer, is not guaranteed or endorsed by the publisher.
References
Abbott, D. C. (1962). The colorimetric determination of anionic surface-active materials in water. Analyst 87 (1033), 286–293. doi:10.1039/AN9628700286
Abdal Dayem, A., Hossain, M. K., Lee, S. B., Kim, K., Saha, S. K., Yang, G. M., et al. (2017). The role of reactive oxygen species (ROS) in the biological activities of metallic nanoparticles. Int. J. Mol. Sci. 18 (1). doi:10.3390/IJMS18010120
Abilaji, S., Narenkumar, J., Das, B., S, S., Rajakrishnan, R., Sathishkumar, K., et al. (2023). Electrochemical oxidation of azo dyes degradation by RuO2–IrO2–TiO2 electrode with biodegradation Aeromonas hydrophila AR1 and its degradation pathway: an integrated approach. Chemosphere 345, 140516. doi:10.1016/J.CHEMOSPHERE.2023.140516
Acar, E. T., Ortaboy, S., and Atun, G. (2015). Adsorptive removal of thiazine dyes from aqueous solutions by oil shale and its oil processing residues: characterization, equilibrium, kinetics and modeling studies. Chem. Eng. J. 276, 340–348. doi:10.1016/J.CEJ.2015.04.089
Alaya, Y., Souissi, R., Toumi, M., Madani, M., El Mir, L., Bouguila, N., et al. (2023). Annealing effect on the physical properties of TiO2 thin films deposited by spray pyrolysis. RSC Adv. 13 (32), 21852–21860. doi:10.1039/D3RA02387G
Al-ekabi, H., Serpone, N., Pelizzetti, E., Minero, C., Fox, M. A., and Draper, R. B. (1989). Kinetic studies in heterogeneous photocatalysis. 2. TiO2-Mediated degradation of 4-chlorophenol alone and in a three-component mixture of 4-chlorophenol, 2,4-dichlorophenol, and 2,4,5-trichlorophenol in air-equilibrated aqueous media. Langmuir 5(1), 250–255. doi:10.1021/LA00085A048/ASSET/LA00085A048.FP.PNG_V03
Al-Madanat, O., Nunes, B. N., AlSalka, Y., Hakki, A., Curti, M., Patrocinio, A. O. T., et al. (2021). Application of EPR spectroscopy in TiO2 and Nb2O5 photocatalysis. Catalysts 11 (12), 1514. doi:10.3390/CATAL11121514
Almeida, A., Faustino, M. A. F., and Neves, M. G. P. M. S. (2020). Antimicrobial photodynamic therapy in the control of COVID-19. Antibiotics 9 6, 1–10. doi:10.3390/ANTIBIOTICS9060320
Al-Nuaim, M. A., Alwasiti, A. A., and Shnain, Z. Y. (2022). The photocatalytic process in the treatment of polluted water. Chem. Pap. 77 (2), 677–701. doi:10.1007/S11696-022-02468-7
AlSalka, Y., Al-Madanat, O., and Hakki, A. (2023). TiO2-based photocatalytic hydrogen production: how to transfer it to an applicable approach? Appl. Catal. A Gen. 662, 119287. doi:10.1016/J.APCATA.2023.119287
Amogne, N. Y., Ayele, D. W., and Tsigie, Y. A. (2020). Recent advances in anthocyanin dyes extracted from plants for dye sensitized solar cell. Mater Renew. Sustain Energy 9 (4), 23–16. doi:10.1007/s40243-020-00183-5
Ananthakumar, S., Balaji, D., Ram Kumar, J., and Moorthy Babu, S. (2019). Role of co-sensitization in dye-sensitized and quantum dot-sensitized solar cells. SN Appl. Sci. 1 (2), 4897–4919. doi:10.1007/s42452-018-0054-3
Anjum, M., Miandad, R., Waqas, M., Gehany, F., and Barakat, M. A. (2019). Remediation of wastewater using various nano-materials. Arabian J. Chem. 12 (8), 4897–4919. doi:10.1016/J.ARABJC.2016.10.004
Anucha, C. B., Altin, I., Bacaksiz, E., and Stathopoulos, V. N. (2022). Titanium dioxide (TiO₂)-based photocatalyst materials activity enhancement for contaminants of emerging concern (CECs) degradation: in the light of modification strategies. Chem. Eng. J. Adv. 10, 100262. doi:10.1016/J.CEJA.2022.100262
Araujo, R. B., Rodrigues, G. L. S., dos Santos, E. C., and Pettersson, L. G. M. (2022). Adsorption energies on transition metal surfaces: towards an accurate and balanced description. Nat. Commun. 13 (1), 6853. 1–14. doi:10.1038/s41467-022-34507-y
Arik, M., and Onganer, Y. (2003). Molecular excitons of Pyronin B and Pyronin Y in colloidal silica suspension. Chem. Phys. Lett. 375 (1–2), 126–133. doi:10.1016/S0009-2614(03)00848-0
Asahi, R., Morikawa, T., Ohwaki, T., Aoki, K., and Taga, Y. (2001). Visible-light photocatalysis in nitrogen-doped titanium oxides. Science 293 (5528), 269–271. doi:10.1126/SCIENCE.1061051
Barakat, N. A. M., Tolba, G. M. K., and Khalil, K. A. (2022). Methylene blue dye as photosensitizer for scavenger-less water photo splitting: new insight in green hydrogen technology. Polym. (Basel) 14 3, 523. doi:10.3390/POLYM14030523
Basumatary, B., Basumatary, R., Ramchiary, A., and Konwar, D. (2022). Evaluation of Ag@TiO2/WO3 heterojunction photocatalyst for enhanced photocatalytic activity towards methylene blue degradation. Chemosphere 286, 131848. doi:10.1016/J.CHEMOSPHERE.2021.131848
Bayard, B. J., Zarrabi, N., Seetharaman, S., Karr, P., van der Est, A., D'Souza, F., et al. (2021). Photoinduced energy and electron transfer in a cofacial aluminum(III) porphyrin – phosphorus(V) porphyrin heterodimer. J. Photochem Photobiol. 8, 100069. doi:10.1016/J.JPAP.2021.100069
Begum Sri Padmavati Mahila Visvavidyalayam, S., Begum, S., and Begum, A. (2016). Thiazines-mini review Saudi journal of medical and pharmaceutical sciences therapeutic utility of 1, 3-thiazines-mini review. Saudi J. Med. Pharm. Sci. Ther. Util. 1, 3. doi:10.21276/sjmps.2016.2.12.2
Beranek, R. (2011). (Photo)electrochemical methods for the determination of the band edge positions of TiO 2-based nanomaterials. Adv. Phys. Chem. 2011, 2011–2020. doi:10.1155/2011/786759
Bhatkhande, D. S., Pangarkar, V. G., and Beenackers, A. A. C. M. (2002). Photocatalytic degradation for environmental applications – a review. J. Chem. Technol. Biotechnol. 77 (1), 102–116. doi:10.1002/JCTB.532
Bindhu, M. R., Willington, T. D., Hatshan, M. R., Chen, S. M., and Chen, T. W. (2022). Environmental photochemistry with Sn/F simultaneously doped TiO2 nanoparticles: UV and visible light induced degradation of thiazine dye. Environ. Res. 207, 112108. doi:10.1016/J.ENVRES.2021.112108
Bonke, S. A., Risse, T., Schnegg, A., and Brückner, A. (2021). In situ electron paramagnetic resonance spectroscopy for catalysis. Nat. Rev. Methods Prim. 1 (1), 33–20. doi:10.1038/s43586-021-00031-4
Buene, A. F., and Almenningen, D. M. (2023). Phenothiazine and phenoxazine sensitizers for dye-sensitized solar cells – an investigative review of two complete dye classes. J. Mater Chem. C Mater 9 (36), 11974–11994. doi:10.1039/D1TC03207K
Buene, A. F., Christensen, M., and Ho, B. H. (2019). Effect of auxiliary donors on 3,8-phenothiazine dyes for dye-sensitized solar cells. Molecules 24. doi:10.3390/MOLECULES24244485
Buglak, A. A., Kapitonova, M. A., Vechtomova, Y. L., and Telegina, T. A. (2022). Insights into molecular structure of pterins suitable for biomedical applications. Int. J. Mol. Sci. 23, 15222. doi:10.3390/IJMS232315222
Calçada, M., Lunardi, J. T., Manzoni, L. A., Monteiro, W., and Pereira, M. (2019). A distributional approach for the one-dimensional hydrogen atom. Front. Phys. 7, 475419. doi:10.3389/fphy.2019.00101
Callaghan, S., and Senge, M. O. (2018). The good, the bad, and the ugly – controlling singlet oxygen through design of photosensitizers and delivery systems for photodynamic therapy. Photochem. Photobiological Sci. 17 (11), 1490–1514. doi:10.1039/C8PP00008E
Carella, A., Borbone, F., and Centore, R. (2018). Research progress on photosensitizers for DSSC. Front. Chem. 6, 481. doi:10.3389/FCHEM.2018.00481
Chakhtouna, H., Benzeid, H., Zari, N., el kacem Qaiss, A., and Bouhfid, R. (2021). Recent progress on Ag/TiO2 photocatalysts: photocatalytic and bactericidal behaviors. Environ. Sci. Pollut. Res. Int. 28 (33), 44638–44666. doi:10.1007/S11356-021-14996-Y
Chakraborty, A., Ali, M., and Saha, S. K. (2010). Molecular interaction of organic dyes in bulk and confined media. Spectrochim. Acta A Mol. Biomol. Spectrosc. 75 (5), 1577–1583. doi:10.1016/J.SAA.2010.02.022
Chaudhari, S. D., Deshpande, A., Kularkar, A., Tandulkar, D., Hippargi, G., Rayalu, S. S., et al. (2022). Engineering of heterojunction TiO2/CaIn2S4@rGO novel nanocomposite for rapid photodegradation of toxic contaminants. J. Industrial Eng. Chem. 114, 305–316. doi:10.1016/J.JIEC.2022.07.020
Chen, D., Cheng, Y., Zhou, N., Chen, P., Wang, Y., Li, K., et al. (2020). Photocatalytic degradation of organic pollutants using TiO2-based photocatalysts: a review. J. Clean. Prod. 268, 121725. doi:10.1016/J.JCLEPRO.2020.121725
Chen, L., Shenai, P., Zheng, F., Somoza, A., and Zhao, Y. (2015). Optimal energy transfer in light-harvesting systems. Molecules 20(8), 15224–15272. doi:10.3390/MOLECULES200815224
Chou, K. F., and Dennis, A. M. (2015). Förster resonance energy transfer between quantum dot donors and quantum dot acceptors. Sensors (Basel) 15 (6), 13288–13325. doi:10.3390/S150613288
Coha, M., Farinelli, G., Tiraferri, A., Minella, M., and Vione, D. (2021). Advanced oxidation processes in the removal of organic substances from produced water: potential, configurations, and research needs. Chem. Eng. J. 414, 128668. doi:10.1016/J.CEJ.2021.128668
Crini, G., and Lichtfouse, E. (2019). Advantages and disadvantages of techniques used for wastewater treatment. Environ. Chem. Lett. 17, 145–155. doi:10.1007/s10311-018-0785-9
Cunico, P., Kumar, A., and Fungaro, D. (2015). Adsorption of dyes from simulated textile wastewater onto modified nanozeolite from coal fly ash.
Danilkina, N. A., Mikhailov, L. E., and Ivin, B. A. (2006). Condensations of thioamides with acetylenecarboxylic acid derivatives. Russ. J. Org. Chem. 42 (6), 783–814. doi:10.1134/s1070428006060017
Dar, N., and Ankari, R. (2022). Theoretical models, preparation, characterization and applications of cyanine J-aggregates: a minireview. ChemistryOpen 11 (11), e202200103. doi:10.1002/OPEN.202200103
Das, K., and Roychoudhury, A. (2014). Reactive oxygen species (ROS) and response of antioxidants as ROS-scavengers during environmental stress in plants. Front. Environ. Sci. 2 (12), 121942. doi:10.3389/fenvs.2014.00053
Decker, E. M., Bartha, V., and Von Ohle, C. (2017). Improvement of antibacterial efficacy through synergistic effect in photodynamic therapy based on thiazinium chromophores against planktonic and biofilm-associated periodontopathogens. Photomed. Laser Surg. 35, 195–205. doi:10.1089/PHO.2016.4152
Detty, M. R., Gibson, S. L., and Wagner, S. J. (2004). Current clinical and preclinical photosensitizers for use in photodynamic therapy. J. Med. Chem. 47 (16), 3897–3915. doi:10.1021/JM040074B
DeVine, J. A., Labib, M., Harries, M. E., Rached, R. A. M., Issa, J., Wishart, J. F., et al. (2015). Electron-transfer dynamics for a donor-bridge-acceptor complex in ionic liquids. J. Phys. Chem. B 119 (34), 11336–11345. doi:10.1021/acs.jpcb.5b03320.PDF
Dhanasekaran, P., and Marimuthu, R. (2023). Efficiency enhancement of natural cocktail dyes in a TiO2-based dye-sensitized solar cell and performance of electron kinetics on the TiO2 surface. Front. Energy Res. 10, 998038. doi:10.3389/FENRG.2022.998038
Dharma, H. N. C., Jaafar, J., Widiastuti, N., Matsuyama, H., Rajabsadeh, S., Othman, M. H. D., et al. (2022). A review of titanium dioxide (TiO2)-based photocatalyst for oilfield-produced water treatment. Membr. (Basel) 12 (3), 345. doi:10.3390/MEMBRANES12030345
Diaz-Angulo, J., Gomez-Bonilla, I., Jimenez-Tohapanta, C., Mueses, M., Pinzon, M., and Machuca-Martinez, F. (2019). Visible-light activation of TiO 2 by dye-sensitization for degradation of pharmaceutical compounds. Photochem. Photobiological Sci. 18 (4), 897–904. doi:10.1039/C8PP00270C
Dlamini, M. C., Maubane-Nkadimeng, M. S., and Moma, J. A. (2021). The use of TiO2/clay heterostructures in the photocatalytic remediation of water containing organic pollutants: a review. J. Environ. Chem. Eng. 9 (6), 106546. doi:10.1016/J.JECE.2021.106546
Dong, H., Zeng, G., Tang, L., Fan, C., Zhang, C., He, X., et al. (2015). An overview on limitations of TiO2-based particles for photocatalytic degradation of organic pollutants and the corresponding countermeasures. Water Res. 79, 128–146. doi:10.1016/J.WATRES.2015.04.038
Elg, D. T., Delgado, H. E., Martin, D. C., Sankaran, R. M., Rumbach, P., Bartels, D. M., et al. (2021). Recent advances in understanding the role of solvated electrons at the plasma-liquid interface of solution-based gas discharges. Spectrochim. Acta Part B A. T. Spectrosc. 186, 106307. doi:10.1016/J.SAB.2021.106307
El-Khouly, M. E., Ito, O., Smith, P. M., and D’Souza, F. (2004). Intermolecular and supramolecular photoinduced electron transfer processes of fullerene–porphyrin/phthalocyanine systems. J. Photochem. Photobiol. C Photochem. Rev. 5 (1), 79–104. doi:10.1016/J.JPHOTOCHEMREV.2004.01.003
Elmorsy, M. R., Abdelhamed, F. H., Badawy, S. A., Abdel-Latif, E., Abdel-Shafi, A. A., and Ismail, M. A. (2023). Design, synthesis, and performance evaluation of TiO2-dye sensitized solar cells using 2,2′-bithiophene-based co-sensitizers. Sci. Rep. 13 (1), 1–18. doi:10.1038/s41598-023-40830-1
Enhanced Photodynamic, J., Mammano, F., Varchi, G., Rodrigues, J. A., and Correia, J. H. (2022). Enhanced photodynamic therapy: a review of combined energy sources. Cells 11 (11), 3995. doi:10.3390/CELLS11243995
Escudero, A., Carrillo-Carrión, C., Castillejos, M. C., Romero-Ben, E., Rosales-Barrios, C., and Khiar, N. (2021). Photodynamic therapy: photosensitizers and nanostructures. Mater Chem. Front. 5 10, 3788–3812. doi:10.1039/D0QM00922A
Eslami, A., Yazdanbakhsh, A. R., and Momayyezi, M. H. (2015). Removal of reactive dyes from textile wastewater using sonochemical process: effective parameters study. J. Res. Health Soc. Dev. Health Promot. Res. Cent. 5 (2), 184–192.
Esrafili, A., Salimi, M., jonidi jafari, A., Reza Sobhi, H., Gholami, M., and Rezaei Kalantary, R. (2022). Pt-based TiO2 photocatalytic systems: a systematic review. J. Mol. Liq. 352, 118685. doi:10.1016/J.MOLLIQ.2022.118685
Fabio, C. A., Yolanda, M., Carmen, G. M., Francisco, C., Antonio Julián, B., Leonor, P., et al. (2016). Use of photodynamic therapy and chitosan for inactivacion of Candida albicans in a murine model. J. Oral Pathol. Med. 45 (8), 627–633. doi:10.1111/JOP.12435
Franchi, D., Bartolini, M., D’Amico, F., Calamante, M., Zani, L., Reginato, G., et al. (2023). Exploring different designs in thieno[3,4-b]pyrazine-based dyes to enhance divergent optical properties in dye-sensitized solar cells. Processes 11 (5), 1542. doi:10.3390/PR11051542
Fujishima, A., and Honda, K. (1972). Electrochemical photolysis of water at a semiconductor electrode. Nature 238, 37–38. doi:10.1038/238037a0
Ghosh, M., Chowdhury, P., and Ray, A. K. (2020). Photocatalytic activity of aeroxide TiO2 sensitized by natural dye extracted from mangosteen peel. Catalysts 10 (8), 917. doi:10.3390/CATAL10080917
Gilani, A. G., Poormohammadi-Ahandani, Z., and Kian, R. (2017). Additive-induced aggregate changes of two structurally similar dyes in aqueous solutions: a comparative photophysical study. doi:10.1016/j.saa.2017.08.048
GilPavas, E., Dobrosz-Gómez, I., and Gómez-García, M. Á. (2018). Optimization of solar-driven photo-electro-Fenton process for the treatment of textile industrial wastewater. J. Water Process Eng. 24, 49–55. doi:10.1016/J.JWPE.2018.05.007
Gilson, R. C., Black, K. C. L., Lane, D. D., and Achilefu, S. (2017). Hybrid TiO2–ruthenium nano-photosensitizer synergistically produces reactive oxygen species in both hypoxic and normoxic conditions. Angew. Chem. - Int. Ed. 56 (36), 10717–10720. doi:10.1002/anie.201704458
Gonuguntla, S., Kamesh, R., Pal, U., and Chatterjee, D. (2023). Dye sensitization of TiO2 relevant to photocatalytic hydrogen generation: current research trends and prospects. J. Photochem. Photobiol. C Photochem. Rev. 57, 100621. doi:10.1016/J.JPHOTOCHEMREV.2023.100621
Goodarzi, N., Ashrafi-Peyman, Z., Khani, E., and Moshfegh, A. Z. (2023). Recent progress on semiconductor heterogeneous photocatalysts in clean energy production and environmental remediation. Catalysts 13 (7), 1102. doi:10.3390/CATAL13071102
Hagfeldt, A., and Grätzel, M. (2000). Molecular photovoltaics. Acc. Chem. Res. 33 (5), 269–277. doi:10.1021/AR980112J
Hamza, M. A., Rizk, S. A., Ezz-Elregal, E. E. M., El-Rahman, S. A. A., Ramadan, S. K., and Abou-Gamra, Z. M. (2023). Photosensitization of TiO2 microspheres by novel Quinazoline-derivative as visible-light-harvesting antenna for enhanced Rhodamine B photodegradation. Sci. Rep. 13 (1), 1–15. doi:10.1038/s41598-023-38497-9
Han, L., Dai, S., Gao, Y., Zhu, J., Zhao, J., Li, Y., et al. (2023). Phenothiazine dyes bearing fluorenone unit for dye-sensitized solar cells. J. Photochem. Photobiol. A-chemistry 440, 114691. doi:10.1016/J.JPHOTOCHEM.2023.114691
Handbook of Green and Sustainable Nanotechnology (2023). Handbook of green and sustainable nanotechnology. doi:10.1007/978-3-031-16101-8
Havelcovaâ, M., Kubaâ, P., Neï Mcovaâ, I., and Heyrovskyâ, J. (2023). Photophysical properties of thiazine dyes in aqueous solution and in micelles.
Hegde, V., O, S. C., Kulkarni, N. V., and Mathew, J. (2023). Synthesis and characterization of cobalt (II) pincer complexes and their application as dyes in dye-sensitized solar cells. J. Mol. Struct. 1286, 135508. doi:10.1016/J.MOLSTRUC.2023.135508
Herath, I., Perera, I., and Hettiarachchi, C. (2020). One-pot synthesis of highly dye impregnated TiO2 nanoparticles for improved white light photo-degradation. Pigment Resin Technol. 49 (1), 1–10. doi:10.1108/prt-03-2019-0031
Hirakawa, K., and Mori, M. (2021). Phenothiazine dyes induce NADH photooxidation through electron transfer: kinetics and the effect of copper ions. ACS Omega 6 (12), 8630–8636. doi:10.1021/ACSOMEGA.1C00484
Hu, L., Zhai, T., Li, H., and Wang, Y. (2019). Redox-Mediator-enhanced electrochemical capacitors: recent advances and future perspectives. ChemSusChem 12 (6), 1118–1132. doi:10.1002/CSSC.201802450
Huang, S., Shao, W., Shi, S., Kong, X., Wang, G., Hu, Z., et al. (2022). The effect of conjugated groups for favourable molecular planarity and efficient suppression of charge recombination simultaneously of phenothiazine-based organic dyes for dye-sensitized solar cells. Synth. Met. 290, 117137. doi:10.1016/J.SYNTHMET.2022.117137
Huang, Y. Y., Sharma, S. K., Yin, R., Agrawal, T., Chiang, L. Y., and Hamblin, M. R. (2014). Functionalized fullerenes in photodynamic therapy. J. Biomed. Nanotechnol. 10 (9), 1918–1936. doi:10.1166/JBN.2014.1963
Hug, S. J., and Bahnemann, D. (2006). Infrared spectra of oxalate, malonate and succinate adsorbed on the aqueous surface of rutile, anatase and lepidocrocite measured with in situ ATR-FTIR. J. Electron Spectros Relat. Phenom. 150 (2–3), 208–219. doi:10.1016/J.ELSPEC.2005.05.006
Humayun, M., Raziq, F., Khan, A., and Luo, W. (2018). Modification strategies of TiO2 for potential applications in photocatalysis: a critical review. Green Chem. Lett. Rev. 11 (2), 86–102. doi:10.1080/17518253.2018.1440324
Humayun, M., Wang, C., and Luo, W. (2022). Recent progress in the synthesis and applications of composite photocatalysts: a critical review. Small Methods 6 (2), 2101395. doi:10.1002/SMTD.202101395
Hung, Y., Aziz, H. A., Zainal, S. F. F. S., Yeh, R. Y., Liu, L., Paul, H. H., et al. (2017). Chemical waste and allied products. Water Environ. Res. 89 (10), 1349–1359. doi:10.2175/106143017X15023776270359
Hung, Y. T., Aziz, H. A., Ramli, S. F., Paul, H. H., Huhnke, C. R., and Adesanmi, B. M. (2020). Chemical waste and allied products. Water Environ. Res. 92 (10), 1504–1509. doi:10.1002/WER.1399
Hurum, D. C., Agrios, A. G., Gray, K. A., Rajh, T., and Thurnauer, M. C. (2003). Explaining the enhanced photocatalytic activity of Degussa P25 mixed-phase TiO2 using EPR. J. Phys. Chem. B 107 (19), 4545–4549. doi:10.1021/jp0273934
Jiang, D., Otitoju, T. A., Ouyang, Y., Shoparwe, N. F., Wang, S., Zhang, A., et al. (2021). A review on metal ions modified TiO2 for photocatalytic degradation of organic pollutants. Catalysts 11 (9), 1039. doi:10.3390/CATAL11091039
jie Song, Y., Li, H. c., Xiong, Z. w., Cheng, L., Du, M., Liu, Z. q., et al. (2023). TiO2/carbon composites from waste sawdust for methylene blue photodegradation. Diam. Relat. Mater 136, 109918. doi:10.1016/J.DIAMOND.2023.109918
Jilakian, M., and Ghaddar, T. H. (2022). Eco-friendly aqueous dye-sensitized solar cell with a copper(I/II) electrolyte system: efficient performance under ambient light conditions. ACS Appl. Energy Mater 5 (1), 257–265. doi:10.1021/acsaem.1c02789
Jing, M., Zhao, H., Jian, L., Pan, C., Dong, Y., and Zhu, Y. (2023). Coral-like B-doped g-C3N4 with enhanced molecular dipole to boost photocatalysis-self-Fenton removal of persistent organic pollutants. J. Hazard Mater 449, 131017. doi:10.1016/J.JHAZMAT.2023.131017
Junqueira, H. C., Severino, D., Dias, L. G., Gugliotti, M. S., and Baptista, M. S. (2002). Modulation of methylene blue photochemical properties based on adsorption at aqueous micelle interfaces. Phys. Chem. Chem. Phys. 4 (11), 2320–2328. doi:10.1039/B109753A
Justin Raj, C., Karthick, S., Park, S., Hemalatha, K., Kim, S. K., Prabakar, K., et al. (2014). Improved photovoltaic performance of CdSe/CdS/PbS quantum dot sensitized ZnO nanorod array solar cell. J. Power Sources 248, 439–446. doi:10.1016/J.JPOWSOUR.2013.09.076
Kang, X., Liu, S., Dai, Z., He, Y., Song, X., and Tan, Z. (2019). Titanium dioxide: from engineering to applications. Catalysts 9 (2), 191. doi:10.3390/CATAL9020191
Kaushal, J. B., Raut, P., and Kumar, S. (2023). Organic electronics in biosensing: a promising frontier for medical and environmental applications. Biosensors 13 (13 11), 976. doi:10.3390/BIOS13110976
Kenichi Honda (2023). Wikipedia. Available at: https://en.wikipedia.org/wiki/Kenichi_Honda.
Kenry, , and Liu, B. (2022). Enhancing the theranostic performance of organic photosensitizers with aggregation-induced emission. Acc. Mater Res. 3 (7), 721–734. doi:10.1021/ACCOUNTSMR.2C00039
Khalil, M., Anggraeni, E. S., Ivandini, T. A., and Budianto, E. (2019). Exposing TiO2 (001) crystal facet in nano Au-TiO2 heterostructures for enhanced photodegradation of methylene blue. Appl. Surf. Sci. 487, 1376–1384. doi:10.1016/J.APSUSC.2019.05.232
Khan, I., Saeed, K., Zekker, I., Zhang, B., Hendi, A. H., Ahmad, A., et al. (2022). Review on methylene blue: its properties, uses, toxicity and photodegradation. Water 14 (2), 242. doi:10.3390/W14020242
Khan, K. A., Shah, A., Nisar, J., Haleem, A., and Shah, I. (2023). Photocatalytic degradation of food and juices dyes via photocatalytic nanomaterials synthesized through green synthetic route: a systematic review. Molecules 28 (12), 4600. doi:10.3390/MOLECULES28124600
Kim, K. S., Barteau, M. A., and Farneth, W. E. (1988). Adsorption and decomposition of aliphatic alcohols on titania. Langmuir 4 (3), 533–543. doi:10.1021/la00081a007
Kishor, R., Purchase, D., Saratale, G. D., Saratale, R. G., Ferreira, L. F. R., Bilal, M., et al. (2021). Ecotoxicological and health concerns of persistent coloring pollutants of textile industry wastewater and treatment approaches for environmental safety. J. Environ. Chem. Eng. 9 (2), 105012. doi:10.1016/J.JECE.2020.105012
Kiwaan, H. A., Atwee, T. M., Azab, E. A., and El-Bindary, A. A. (2020). Photocatalytic degradation of organic dyes in the presence of nanostructured titanium dioxide. J. Mol. Struct. 1200, 127115. doi:10.1016/J.MOLSTRUC.2019.127115
Kohle, F. F. E., Li, S., Turker, M. Z., and Wiesner, U. B. (2020). Ultrasmall PEGylated and targeted core-shell silica nanoparticles carrying methylene blue photosensitizer. ACS Biomater. Sci. Eng. 6 (1), 256–264. doi:10.1021/acsbiomaterials.9b01359
Krishnan, S., and Shriwastav, A. (2021). Application of TiO2 nanoparticles sensitized with natural chlorophyll pigments as catalyst for visible light photocatalytic degradation of methylene blue. J. Environ. Chem. Eng. 9 (1), 104699. doi:10.1016/J.JECE.2020.104699
Kubas, A. (2021). How the donor/acceptor spin states affect the electronic couplings in molecular charge-transfer processes? J. Chem. Theory Comput. 17 (5), 2917–2927. doi:10.1021/ACS.JCTC.1C00126/ASSET/IMAGES/LARGE/CT1C00126_0006.JPEG
Lakhera, S. K., and Neppolian, B. (2021). Visible light photocatalysis: case study (process). Handb. Nanomater. Wastewater Treat. Fundam. Scale up Issues, 1101–1123. doi:10.1016/B978-0-12-821496-1.00001-5
Langhals, H. (2004). “Color chemistry. Synthesis, properties and applications of organic dyes and pigments,” in Angewandte chemie international edition. Editor H. Zollinger 3rd revised edition, 5291–5292. doi:10.1002/ANIE.200385122
Lee, C. P., Li, C. T., and Ho, K. C. (2017). Use of organic materials in dye-sensitized solar cells. Mater. Today 20 (5), 267–283. doi:10.1016/J.MATTOD.2017.01.012
Lee, H., Kim, J., Kim, D. Y., and Seo, Y. (2018). Co-sensitization of metal free organic dyes in flexible dye sensitized solar cells. Org. Electron 52, 103–109. doi:10.1016/J.ORGEL.2017.10.003
Li, J., Li, Z., Liu, H., Gong, H., Zhang, J., Yao, Y., et al. (2022). Organic molecules with inverted singlet-triplet gaps. Front. Chem. 10, 2022. doi:10.3389/FCHEM.2022.999856
Li, J., and Pu, K. (2019). Development of organic semiconducting materials for deep-tissue optical imaging, phototherapy and photoactivation. Chem. Soc. Rev. 48 (1), 38–71. doi:10.1039/C8CS00001H
Li, R., Li, T., and Zhou, Q. (2020b). Impact of titanium dioxide (TiO2) modification on its application to pollution treatment—a review. Catalysts 10 (7), 804. doi:10.3390/CATAL10070804
Li, R., Zhang, F., Wang, D., Yang, J., Li, M., Zhu, J., et al. (2013). Spatial separation of photogenerated electrons and holes among {010} and {110} crystal facets of BiVO4. Nat. Commun. 4 (1), 1432. doi:10.1038/ncomms2401
Li, S., He, J., Jiang, H., Mei, S., Hu, Z., Kong, X., et al. (2021). Comparative studies on the structure-performance relationships of phenothiazine-based organic dyes for dye-sensitized solar cells. ACS Omega 6 (10), 6817–6823. doi:10.1021/ACSOMEGA.0C05887
Li, X., Yu, J., and Jiang, C. (2020a). Principle and surface science of photocatalysis. Interface Sci. Technol. 31, 1–38. doi:10.1016/B978-0-08-102890-2.00001-4
Lima, E., and Reis, L. V. (2023). Photodynamic therapy: from the basics to the current progress of N-Heterocyclic-Bearing dyes as effective photosensitizers. Molecules 28 (13), 5092. doi:10.3390/MOLECULES28135092
Liu, T., Yang, C., and Li, Y. (2023). Enhanced efficiency of DSSCs by co-sensitizing dyes with complementary absorption spectra. J. Photochem Photobiol. A Chem. 442, 114758. doi:10.1016/J.JPHOTOCHEM.2023.114758
Liu, W., Tkatchenko, A., and Scheffler, M. (2014). Modeling adsorption and reactions of organic molecules at metal surfaces. Acc. Chem. Res. 47 (11), 3369–3377. doi:10.1021/ar500118y
Luo, C., Wang, H., Dong, W., and Zhang, X. (2017). Theoretical investigation on the mechanism of the OH-initiated degradation process of reactive red 2 azo dye. RSC Adv. 7 (66), 41799–41811. doi:10.1039/C7RA05727J
Luo, T., Wang, H., Chen, L., Li, J., Wu, F., and Zhou, D. (2021). Visible light-driven oxidation of arsenite, sulfite and thiazine dyes: a new strategy for using waste to treat waste. J. Clean. Prod. 280, 124374. doi:10.1016/J.JCLEPRO.2020.124374
Lusvardi, V. S., Barteau, M. A., and Farneth, W. E. (1995). The effects of bulk titania crystal structure on the adsorption and reaction of aliphatic alcohols. J. Catal. 153 (1), 41–53. doi:10.1006/JCAT.1995.1106
Lynch, P. G., Richards, H., and Wustholz, K. L. (2019). Unraveling the excited-state dynamics of eosin Y photosensitizers using single-molecule spectroscopy. J. Phys. Chem. A 123 (13), 2592–2600. doi:10.1021/ACS.JPCA.9B00409
Magalhães, P., Andrade, L., Nunes, O. C., and Mendes, A. (2017). Titanium Dioxide photocatalysis: fundamentals and application on photoinactivation.
Mall, C., Tiwari, S., and Solanki, P. P. (2019). Comparison of dye (oxazine and thiazine) materials as a photosensitizer for use in photogalvanic cells based on molecular interaction with sodium dodecyl sulphate by spectral study. J. Saudi Chem. Soc. 23 (1), 83–91. doi:10.1016/J.JSCS.2018.04.007
Manda, A. A., Haladu, S. A., Elsayed, K. A., Ibrahim Gaya, U., Alheshibri, M., Al Baroot, A., et al. (2023). Fast one-pot laser-based fabrication of ZnO/TiO2-reduced graphene oxide nanocomposite for photocatalytic applications. Opt. Laser Technol. 160, 109105. doi:10.1016/J.OPTLASTEC.2022.109105
Mezyen, M., El Fidha, G., Bitri, N., Harrathi, F., Ly, I., and Llobet, E. (2023). Visible light activated SnO 2:Dy thin films for the photocatalytic degradation of methylene blue. RSC Adv. 13, 31151–31166. doi:10.1039/D3RA05424A
Minaev, B. F., Panchenko, O. O., Minaeva, V. A., and Ågren, H. (2022). Triplet state harvesting and search for forbidden transition intensity in the nitrogen molecule. Front. Chem. 10, 1005684. doi:10.3389/fchem.2022.1005684
Mohlala, R. L., Coyanis, E. M., Fish, M. Q., Fernandes, M. A., and Bode, M. L. (2021). Synthesis of 6-membered-ring fused thiazine-dicarboxylates and thiazole-pyrimidines via one-pot three-component reactions. Molecules 26 (18), 5493. doi:10.3390/MOLECULES26185493
Moma, J., and Baloyi, J. (2018). Modified titanium dioxide for photocatalytic applications. Photocatal. - Appl. Attributes. doi:10.5772/INTECHOPEN.79374
Monika, S., Mahalakshmi, M., and Pandian, M. S. (2023). TiO2/CdS/CdSe quantum dots co-sensitized solar cell with the staggered-gap (type-II) heterojunctions for the enhanced photovoltaic performance. Ceram. Int. 49 (6), 8820–8826. doi:10.1016/J.CERAMINT.2022.11.034
Monro, S., Colón, K. L., Yin, H., Roque, J., Konda, P., Gujar, S., et al. (2019). Transition metal complexes and photodynamic therapy from a tumor-centered approach: challenges, opportunities, and highlights from the development of TLD1433. Chem. Rev. 119 (2), 797–828. doi:10.1021/ACS.CHEMREV.8B00211
Montalti, M., Credi, A., Prodi, L., and Gandolfi, M. T. (2006). Handbook of photochemistry. Handb. Photochem. doi:10.1201/9781420015195
Monteiro, J. S. C., de Oliveira, S. C. P. S., Pires Santos, G. M., Pires Sampaio, F. J., Pinheiro Soares, L. G., and Pinheiro, A. L. B. (2017). Effectiveness of antimicrobial photodynamic therapy (AmPDT) on Staphylococcus aureus using phenothiazine compound with red laser. Lasers Med. Sci. 32 (1), 29–34. doi:10.1007/S10103-016-2079-4
Montes De Oca, M. N., Vara, J., Milla, L., Rivarola, V., and Ortiz, C. S. (2013). Physicochemical properties and photodynamic activity of novel derivatives of triarylmethane and thiazine. Arch. Pharm. Weinh. 346 (4), 255–265. doi:10.1002/ARDP.201200437
Moser, J. E., Bonnôte, P., and Grätzel, M. (1998). Molecular photovoltaics. Coord. Chem. Rev. 171 (1), 245–250. doi:10.1016/S0010-8545(98)90037-6
Murray, C. A., and Parsons, S. A. (2004). Advanced oxidation processes: flowsheet options for bulk natural organic matter removal. Water Supply 4 (4), 113–119. doi:10.2166/WS.2004.0068
Navarro, P., Antonio Gabald on, J., and opez, V. M. G. (2017). Degradation of an azo dye by a fast and innovative pulsed light/H 2 O 2 advanced oxidation process. Dyes Pigments 136, 887–892. doi:10.1016/j.dyepig.2016.09.053
Oladoye, P. O., Ajiboye, T. O., Omotola, E. O., and Oyewola, O. J. (2022). Methylene blue dye: toxicity and potential elimination technology from wastewater. Results Eng. 16, 100678. doi:10.1016/J.RINENG.2022.100678
Ortiz-Rodríguez, L. A., Hoehn, S. J., Loredo, A., Wang, L., Xiao, H., and Crespo-Hernández, C. E. (2021). Electronic relaxation pathways in heavy-atom-free photosensitizers absorbing near-infrared radiation and exhibiting high yields of singlet oxygen generation. J. Am. Chem. Soc. 143 (7), 2676–2681. doi:10.1021/JACS.0C13203
Othman, Z., Sinopoli, A., MacKey, H. R., and Mahmoud, K. A. (2021). Efficient photocatalytic degradation of organic dyes by AgNPs/TiO2/Ti3C2Tx MXene composites under UV and solar light. ACS Omega 6 49, 33325–33338. doi:10.1021/ACSOMEGA.1C03189/ASSET/IMAGES/LARGE/AO1C03189_0014.JPEG
Padnya, P. L., Khadieva, A. I., and Stoikov, I. I. (2023). Current achievements and perspectives in synthesis and applications of 3,7-disubstituted phenothiazines as Methylene Blue analogues. Dyes Pigments 208, 110806. doi:10.1016/J.DYEPIG.2022.110806
Parrino, F., D’Arienzo, M., Mostoni, S., Dirè, S., Ceccato, R., Bellardita, M., et al. (2022). Electron and energy transfer mechanisms: the double nature of TiO2 heterogeneous photocatalysis. Top. Curr. Chem. 380 (1), 2–15. doi:10.1007/s41061-021-00358-2
Paul, P., and Suresh Kumar, G. (2013). Spectroscopic studies on the binding interaction of phenothiazinium dyes toluidine blue O, azure A and azure B to DNA. Spectrochim. Acta A Mol. Biomol. Spectrosc. 107, 303–310. doi:10.1016/J.SAA.2013.01.063
Pawar, M., Sendoǧdular, S. T., and Gouma, P. (2018). A brief overview of TiO2 photocatalyst for organic dye remediation: case study of reaction mechanisms involved in Ce-TiO2 photocatalysts system. J. Nanomater 2018, 1–13. doi:10.1155/2018/5953609
Peiris, S., de Silva, H. B., Ranasinghe, K. N., Bandara, S. V., and Perera, I. R. (2021). Recent development and future prospects ofTiO2photocatalysis. J. Chin. Chem. Soc. 68 (5), 738–769. doi:10.1002/JCCS.202000465
Pendergast, M. T. M., Nygaard, J. M., Ghosh, A. K., and Hoek, E. M. V. (2010). Using nanocomposite materials technology to understand and control reverse osmosis membrane compaction. Desalination 261, 255–263. doi:10.1016/j.desal.2010.06.008
Pinto, A. P., Bueno Rosseti, I., Lopes Carvalho, M., Marques Da Silva, B. G., Alberto-Silva, C., and Silva Costa, M. (2017). Photodynamic Antimicrobial Chemotherapy (PACT), using Toluidine blue O inhibits the viability of biofilm produced by Candida albicans at different stages of development. doi:10.1016/j.pdpdt.2017.12.001
Pirillo, J., Mazzone, G., and Russo, N. (2018). Theoretical insights into the switching off/on of 1O2 photosensitization in chemicontrolled photodynamic therapy. Chem. – A Eur. J. 24 (14), 3512–3519. doi:10.1002/CHEM.201704768
Qian, R., Zong, H., Schneider, J., Zhou, G., Zhao, T., Li, Y., et al. (2019). Charge carrier trapping, recombination and transfer during TiO2 photocatalysis: an overview. Catal. Today 335, 78–90. doi:10.1016/J.CATTOD.2018.10.053
Qin, Z., Wang, Z., Kong, F., Su, J., Huang, Z., Zhao, P., et al. (2023). In situ electron paramagnetic resonance spectroscopy using single nanodiamond sensors. Nat. Commun. 14 (1), 6278. doi:10.1038/s41467-023-41903-5
Radwan, A. S., Elmorsy, M. R., Abdel-Latif, E., Makhlouf, M. M., and Badawy, S. A. (2023). Innovating dye-sensitized solar cells: thiazole-based Co-sensitizers for enhanced photovoltaic performance with theoretical insights. Opt. Mater (Amst) 140, 113914. doi:10.1016/J.OPTMAT.2023.113914
Rafique, M. S., Tahir, M. B., Rafique, M., and Khan, M. I. (2020). Recent advances in the development of photocatalysis and future perspectives. Nanotechnol. Photocatal. Environ. Appl. 221, 221–223. doi:10.1016/B978-0-12-821192-2.00013-9
Rani, M., and Shanker, U. (2023). Removal of organic dyes by functionalized nanomaterials. Handb. Green Sustain. Nanotechnol., 1267–1298. doi:10.1007/978-3-031-16101-8_52
Rao, V. N., Ravi, P., Sathish, M., Sakar, M., Yang, B. L., Yang, J. M., et al. (2022). Titanate quantum dots-sensitized Cu2S nanocomposites for superficial H2 production via photocatalytic water splitting. Int. J. Hydrogen Energy 47 (95), 40379–40390. doi:10.1016/J.IJHYDENE.2022.05.091
Rashid, M. M., Simončič, B., and Tomšič, B. (2021). Recent advances in TiO2-functionalized textile surfaces. Surfaces Interfaces 22, 100890. doi:10.1016/J.SURFIN.2020.100890
Robertson, C., and Evans, D.H. A.-J. of P. and, and undefined (2009). Photodynamic therapy (PDT): a short review on cellular mechanisms and cancer research applications for PDT. Elsevier. Available at: https://www.sciencedirect.com/science/article/pii/S1011134409000608.
Rochkind, M., Pasternak, S., and Paz, Y. (2015). Using dyes for evaluating photocatalytic properties: a critical review. Molecules 20 (1), 88–110. doi:10.3390/MOLECULES20010088
Roduner, E., and Krüger, T. P. J. (2022). The origin of irreversibility and thermalization in thermodynamic processes. Phys. Rep. 944, 1–43. doi:10.1016/j.physrep.2021.11.002
Ronca, E., Pastore, M., Belpassi, L., Tarantelli, F., and De Angelis, F. (2012). Influence of the dye molecular structure on the TiO2 conduction band in dye-sensitized solar cells: disentangling charge transfer and electrostatic effects. Energy Environ. Sci. 6 (1), 183–193. doi:10.1039/C2EE23170K
Ronzani, F., Trivella, A., Bordat, P., Blanc, S., and Lacombe, S. (2014). Revisiting the photophysics and photochemistry of methylene violet (MV). J. Photochem Photobiol. A Chem. 284, 8–17. doi:10.1016/J.JPHOTOCHEM.2014.03.019
Rossi, V. M. (2023). A quantum mechanical description of photosensitization in photodynamic therapy using a two-electron molecule approximation. doi:10.48550/ARXIV.2301.03653
Roy, A., Mohamed, M. J. S., Gondal, M. A., Mallick, T. K., Tahir, A. A., and Sundaram, S. (2023). Co-sensitization effect of N719 dye with Cu doped CdS colloidal nanoparticles for dye sensitized solar cells. Inorg. Chem. Commun. 148, 110298. doi:10.1016/J.INOCHE.2022.110298
Sakar, M., Mithun Prakash, R., and Trong-On, D. (2019). Insights into the TiO2-based photocatalytic systems and their mechanisms. Catalysts 9 (8), 680. doi:10.3390/CATAL9080680
Sarfraz, B., Mehran, M. T., Shahzad, F., Hussain, S., Naqvi, S. R., Khan, H. A., et al. (2023). Bifunctional CuS/Cl-terminated greener MXene electrocatalyst for efficient hydrogen production by water splitting. RSC Adv. 13 (32), 22017–22028. doi:10.1039/D3RA02581K
Schneider, J., Matsuoka, M., Takeuchi, M., Zhang, J., Horiuchi, Y., Anpo, M., et al. (2014). Understanding TiO2photocatalysis: mechanisms and materials. Chem. Rev. 114 (19), 9919–9986. doi:10.1021/cr5001892
Sekar, R. B., and Periasamy, A. (2003). Fluorescence resonance energy transfer (FRET) microscopy imaging of live cell protein localizations. J. Cell. Biol. 160 (5), 629–633. doi:10.1083/JCB.200210140
Selvaraj, V., Swarna Karthika, T., Mansiya, C., and Alagar, M. (2021). An over review on recently developed techniques, mechanisms and intermediate involved in the advanced azo dye degradation for industrial applications. J. Mol. Struct. 1224, 129195. doi:10.1016/J.MOLSTRUC.2020.129195
Senge, M. O., and Radomski, M. W. (2013). Platelets, photosensitizers, and PDT. Photodiagnosis Photodyn. Ther. 10, 1–16. doi:10.1016/j.pdpdt.2012.08.004
Senthilkumar, K., Paul, P., Selvaraju, C., and Natarajan, P. (2010). Preparation, characterization, and photophysical study of thiazine dyes within the nanotubes and nanocavities of silicate host: influence of titanium dioxide nanoparticle on the protonation and aggregation of dyes. J. Phys. Chem. C 114 (15), 7085–7094. doi:10.1021/jp912267q
Serpone, N. (2018). Heterogeneous photocatalysis and prospects of TiO2-based photocatalytic DeNOxing the atmospheric environment. Catalysts 8 (11), 553. doi:10.3390/CATAL8110553
Severino, D., Junqueira, H. C., Gugliotti, M., Gabrielli, D. S., and Baptista, M. S. (2003). Influence of negatively charged interfaces on the ground and excited state properties of methylene blue ¶. Photochem Photobiol. 77 (5), 459–468. doi:10.1562/0031-8655(2003)0770459IONCIO2.0.CO2
Shen, X., Dong, L., He, X., Zhao, C., Zhang, W., Li, X., et al. (2020). Treatment of infected wounds with methylene blue photodynamic therapy: an effective and safe treatment method. Photodiagnosis Photodyn. Ther. 32, 102051. doi:10.1016/J.PDPDT.2020.102051
Shi, S., Huang, S., Shao, W., Kong, X., Liu, B., Hu, Z., et al. (2023). The application of a novel D−A− π − A phenothiazine-based organic dye with N719 in efficient parallel tandem dye-sensitized solar cells. Synth. Met. 295, 117344. doi:10.1016/J.SYNTHMET.2023.117344
Shin, J., Kang, D. W., Lim, J. H., An, J. M., Kim, Y., Kim, J. H., et al. (2023). Wavelength engineerable porous organic polymer photosensitizers with protonation triggered ROS generation. Nat. Commun. 14 (1), 1498. doi:10.1038/S41467-023-37156-X
Sinha, D., De, D., Goswami, D., Mondal, A., and Ayaz, A. (2019). ZnO and TiO2 nanostructured dye sensitized solar photovoltaic cell. Mater Today Proc. 11, 782–788. doi:10.1016/J.MATPR.2019.03.043
Speirs, M., Hardman, S. J. O., Iorgu, A. I., Johannissen, L. O., Heyes, D. J., Scrutton, N. S., et al. (2023). Photoinduced electron transfer from a 1,4,5,6-tetrahydro nicotinamide adenine dinucleotide (phosphate) analogue to oxidized flavin in an ene-reductase flavoenzyme. J. Phys. Chem. Lett. 14 13, 3236–3242. doi:10.1021/acs.jpclett.3c00176
Spring, B. Q., Rizvi, I., Xu, N., and Hasan, T. (2015). The role of photodynamic therapy in overcoming cancer drug resistance. Cite this Photochem. Photobiol. Sci. 14, 1476–1491. doi:10.1039/c4pp00495g
Srinivasan, A., and Viraraghavan, T. (2010). Decolorization of dye wastewaters by biosorbents: a review. doi:10.1016/j.jenvman.2010.05.003
Stoll, S., and Schweiger, A. (2006). EasySpin, a comprehensive software package for spectral simulation and analysis in EPR. J. Magnetic Reson. 178 (1), 42–55. doi:10.1016/J.JMR.2005.08.013
Szaciłowski, K., Macyk, W., Drzewiecka-Matuszek, A., Brindell, M., and Stochel, G. (2005). Bioinorganic photochemistry: frontiers and mechanisms. Chem. Rev. 105 (6), 2647–2694. doi:10.1021/CR030707E
Tahir, M. B., Iqbal, T., Rafique, M., Rafique, M. S., Nawaz, T., and Sagir, M. (2020). Nanomaterials for photocatalysis. Nanotechnol. Photocatal. Environ. Appl., 65–76. doi:10.1016/B978-0-12-821192-2.00005-X
Takahashi, S., Uchida, S., Jayaweera, P. V. V., Kaneko, S., and Segawa, H. (2023). Impact of compact TiO2 interface modification on the crystallinity of perovskite solar cells. Sci. Rep. 13 (1), 1–10. doi:10.1038/s41598-023-43395-1
Talman, R. Y., and Atun, G. (2006). Effects of cationic and anionic surfactants on the adsorption of toluidine blue onto fly ash. Colloids Surf. A Physicochem Eng. Asp. 281, 15–22. doi:10.1016/J.COLSURFA.2006.02.006
Tanielian, C., Schweitzer, C., Seghrouchni, R., Esch, M., and Mechin, R. (2003). Polyoxometalate sensitization in mechanistic studies of photochemical reactions: the decatungstate anion as a reference sensitizer for photoinduced free radical oxygenations of organic compounds. Photochem. Photobiological Sci. 2 (3), 297–305. doi:10.1039/b210786b
Tardivo, J. P., Del Giglio, A., de Oliveira, C. S., Gabrielli, D. S., Junqueira, H. C., Tada, D. B., et al. (2005). Methylene blue in photodynamic therapy: from basic mechanisms to clinical applications. Photodiagnosis Photodyn. Ther. 2 (3), 175–191. doi:10.1016/S1572-1000(05)00097-9
Tavakkoli Yaraki, M., Liu, B., and Tan, Y. N. (2022). Emerging strategies in enhancing singlet oxygen generation of nano-photosensitizers toward advanced phototherapy. Nanomicro Lett. 14 (1), 123. doi:10.1007/S40820-022-00856-Y
Tentu, R. D., and Basu, S. (2017). Photocatalytic water splitting for hydrogen production. Curr. Opin. Electrochem 5 1, 56–62. doi:10.1016/J.COELEC.2017.10.019
Thandu, M., Comuzzi, C., and Goi, D. (2015). Phototreatment of water by organic photosensitizers and comparison with inorganic semiconductors. Int. J. Photoenergy 2015, 1–22. doi:10.1155/2015/521367
Theodoro, L. H., da Rocha, T. E., Wainwright, M., Nuernberg, M. A. A., Ervolino, E., Souza, E. Q. M., et al. (2021). Comparative effects of different phenothiazine photosensitizers on experimental periodontitis treatment. Photodiagnosis Photodyn. Ther. 34, 102198. doi:10.1016/J.PDPDT.2021.102198
Tinoco Navarro, L. K., and Jaroslav, C. (2023). Enhancing photocatalytic properties of TiO2 photocatalyst and heterojunctions: a comprehensive review of the impact of biphasic systems in aerogels and xerogels synthesis, methods, and mechanisms for environmental applications. Gels 9 (12), 976. doi:10.3390/GELS9120976
Triesscheijn, M., Baas, P., Schellens, J. H., and Stewart, F. A. (2006). Photodynamic therapy in oncology. Oncologist 11 (9), 1173–1179. doi:10.1634/THEONCOLOGIST.11-9-1034
Ursu, D., Miclau, M., Casut, C., Albulescu, D., Birtok-Baneasa, C., and Vajda, M. (2024). Efficient indoor dye-sensitized solar cells based on TiO2 hollow sphere. J. Alloys Compd. 976, 173134. doi:10.1016/J.JALLCOM.2023.173134
Valadez-Renteria, E., Oliva, J., and Rodriguez-Gonzalez, V. (2022). A sustainable and green chlorophyll/TiO2:W composite supported on recycled plastic bottle caps for the complete removal of Rhodamine B contaminant from drinking water. J. Environ. Manage 315, 115204. doi:10.1016/J.JENVMAN.2022.115204
van der Meer, B. W. (2013). Förster theory. FRET - Förster Reson. Energy Transf. Theory Appl. 23, 23–62. doi:10.1002/9783527656028.CH03
Vara, J., and Ortiz, C. S. (2016). Thiazine dyes: evaluation of monomeric and aggregate forms. Spectrochim. Acta A Mol. Biomol. Spectrosc. 166, 112–120. doi:10.1016/J.SAA.2016.05.005
Villa, K., Galán-Mascarós, J. R., López, N., and Palomares, E. (2021). Photocatalytic water splitting: advantages and challenges. Sustain Energy Fuels 5 (18), 4560–4569. doi:10.1039/D1SE00808K
Vinaayak, S. B., Balasubramani, V., Shkir, M., Aslam Manthrammel, M., and Sreedevi, G. (2022). Enhancing the performance of TiO 2 based N-DSSC using dye extracted from Cladophora Columbiana, Ludwigia repens and mixed sensitizer. doi:10.1016/j.optmat.2022.112968
Vinu, R., and Madras, G. (2011). Photocatalytic degradation of water pollutants using nano-TiO2. Green Energy Technol. 33, 625–677. doi:10.1007/978-0-85729-638-2_19/COVER
Wang, B., and Lu, S. (2022). The light of carbon dots: from mechanism to applications. Matter 5 (1), 110–149. doi:10.1016/J.MATT.2021.10.016
Wang, H., Guan, L., Liu, J., Lei, T., Xue, Y., Qu, Z., et al. (2022b). A thiazolo[5,4-d]thiazole functionalized covalent triazine framework showing superior photocatalytic activity for hydrogen production and dye degradation. J. Mater. Chem. A, Mater. energy Sustain. 10 (30), 16328–16336. doi:10.1039/D2TA04177D
Wang, J., Li, H., Zhu, Y., Yang, M., Huang, J., Zhu, X., et al. (2022a). Unveiling upsurge of photogenerated ROS: control of intersystem crossing through tuning aggregation patterns. Chem. Sci. 14 (2), 323–330. doi:10.1039/D2SC06445F
Wategaonkar, S. B., Parale, V. G., Pawar, R. P., Mali, S. S., Hong, C. K., Powar, R. R., et al. (2021). Structural, morphological, and optical studies of hydrothermally synthesized Nb-added TiO2 for DSSC application. Ceram. Int. 47 (18), 25580–25592. doi:10.1016/J.CERAMINT.2021.05.284
Xu, G., Li, C., Chi, C., Wu, L., Sun, Y., Zhao, J., et al. (2022). A supramolecular photosensitizer derived from an Arene-Ru(II) complex self-assembly for NIR activated photodynamic and photothermal therapy. Nat. Commun. 13 (1), 3064. doi:10.1038/S41467-022-30721-W
Yang, X., and Wang, D. (2018). Photocatalysis: from fundamental principles to materials and applications. ACS Appl. Energy Mater 1 (12), 6657–6693. doi:10.1021/acsaem.8b01345
Yang, Y., Zhang, Y., Wang, R., Rong, X., Liu, T., Xia, X., et al. (2022). A glutathione activatable pro-drug-photosensitizer for combined chemotherapy and photodynamic therapy. Chin. Chem. Lett. 33 (12), 4583–4586. doi:10.1016/J.CCLET.2022.03.040
Yhon, J., Mendoza, J., Osorio, E., and Domínguez, M. P. (2023). Continuous removal of dyes from wastewater using banana-peel bioadsorbent: a low-cost alternative for wastewater treatment. Sustainability 15 (13), 9870. doi:10.3390/SU15139870
Yu, H., Chen, B., Huang, H., He, Z., Sun, J., Wang, G., et al. (2022). AIE-active photosensitizers: manipulation of reactive oxygen species generation and applications in photodynamic therapy. Biosensors 12 (5), 348. doi:10.3390/BIOS12050348
Zani, L., Melchionna, M., Montini, T., and Fornasiero, P. (2021). Design of dye-sensitized TiO2 materials for photocatalytic hydrogen production: light and shadow. J. Phys. Energy 3 (3), 031001. doi:10.1088/2515-7655/ABE04B
Zapata, D., Higgs, J., Wittholt, H., Chittimalli, K., Brooks, A. E., and Mulinti, P. (2022). Nanotechnology in the diagnosis and treatment of osteomyelitis. Pharmaceutics 14 (8), 1563. doi:10.3390/PHARMACEUTICS14081563
Zhang, L., and Cole, J. M. (2015). Anchoring groups for dye-sensitized solar cells. ACS Appl. Mater Interfaces 7 (6), 3427–3455. doi:10.1021/am507334m
Zhang, L., Xu, M., Chen, H., Li, Y., and Chen, S. (2022). Globalization, green economy and environmental challenges: state of the art review for practical implications. Front. Environ. Sci. 10, 870271. doi:10.3389/fenvs.2022.870271
Zhang, Z., Li, Z., Bai, X., Shi, J., Hu, M., Chai, J., et al. (2023). Photosensitive dye as an ideal peroxymonosulfate activator for efficient self-degradation: a novel idea of using waste to treat waste. Molecules 28 (10), 4237. doi:10.3390/MOLECULES28104237
Zhao, X., Liu, J., Fan, J., Chao, H., and Peng, X. (2021). Recent progress in photosensitizers for overcoming the challenges of photodynamic therapy: from molecular design to application. Chem. Soc. Rev. 50 (6), 4185–4219. doi:10.1039/D0CS00173B
Zioui, D., Aoudjit, L., Touahra, F., and Bachari, K. (2022). Preparation and characterization of tio2-chitosan composite films and application for tartrazine dye degradation. Cellul. Chem. Technol. 56 (9–10), 1101–1107. doi:10.35812/CELLULOSECHEMTECHNOL.2022.56.98
Keywords: titanium dioxide (TiO2), thiazine dyes, photosensitizers, photocatalysis, visible light, hybrid materials, photodegradation, water treatment
Citation: Chauke NM, Mohlala RL, Ngqoloda S and Raphulu MC (2024) Harnessing visible light: enhancing TiO2 photocatalysis with photosensitizers for sustainable and efficient environmental solutions. Front. Chem. Eng. 6:1356021. doi: 10.3389/fceng.2024.1356021
Received: 14 December 2023; Accepted: 15 January 2024;
Published: 02 February 2024.
Edited by:
Tshimangadzo Munonde, University of South Africa, South AfricaReviewed by:
Neeraj Kumar, Council for Scientific and Industrial Research (CSIR), South AfricaMasixole Sihlahla, University of Johannesburg, South Africa
Copyright © 2024 Chauke, Mohlala, Ngqoloda and Raphulu. This is an open-access article distributed under the terms of the Creative Commons Attribution License (CC BY). The use, distribution or reproduction in other forums is permitted, provided the original author(s) and the copyright owner(s) are credited and that the original publication in this journal is cited, in accordance with accepted academic practice. No use, distribution or reproduction is permitted which does not comply with these terms.
*Correspondence: Nyiko M. Chauke, bnlpa29jQG1pbnRlay5jby56YQ==