- 1Laboratory of Genomics and BioEnergy (LGE), Department of Genetics, Evolution, Microbiology and Immunology, University of Campinas (Unicamp), Campinas, São Paulo, Brazil
- 2Laboratory of Crop Physiology (LCroP), Department of Plant Biology, University of Campinas (Unicamp), Campinas, São Paulo, Brazil
- 3Laboratory of Molecular and Computational Biology of Fungi (LBMCF), Institute of Biological Sciences, Universidade Federal de Minas Gerais (UFMG), Belo Horizonte, Minas Gerais, Brazil
- 4Global Academy of Agriculture and Food Security, University of Edinburgh, Edinburgh, United Kingdom
- 5Center of Agricultural, Environmental and Biological Sciences, Federal University of Recôncavo of Bahia (UFRB), Cruz das Almas, Bahia, Brazil
Sisal bole rot disease is the major phytosanitary problem of Agave plantations in Brazil. The disease is caused by a cryptic species of Aspergillus: A. welwitschiae. To date, the only way to diagnose the disease was to observe external symptoms, visible only when the plant is already compromised, or through the isolation and sequencing of the pathogen, which requires cutting the entire plant for bole tissue sampling. We developed a new primer set based on a unique gene region of A. welwitschiae, which can detect the phytopathogenic strains through PCR directly from sisal leaves. Using the new marker to study the main sisal-producing areas in Brazil, we discovered a troublesome situation. The main producing areas of this crop had a pathogen incidence of 78%–88%. The dispersion index indicates a regular spatial pattern for disease distribution, suggesting that the use of contaminated suckers to establish new fields may be the main disease-spreading mechanism. Altogether, the high incidence of the pathogen, the unavailability of clean plants, the unpredictability of disease progression, and the low investment capacity of farmers reveal the vulnerability of this sector to a potential phytosanitary crisis. By correlating the disease symptomatology with soil nutritional traits, we suggest that higher potassium availability might decrease visual symptoms, while phosphorus may have the opposite effect. Also, we observe a potential cultivar effect, suggesting that common sisal may be more susceptible than hybrid cultivars (especially H400). This new molecular tool is a significant advance for understanding the disease, enabling the implementation of a monitoring program and studies that may lead to pathogen control strategies and changes in the Brazilian production model.
1 Introduction
Sisal is the popular name for a range of hard natural fibers derived from Agave leaves, usually extracted from Agave sisalana (common sisal), A. fourcroydes, or hybrid cultivars, like the H11468 [(A. amaniensis x A. angustifolia) x A. amaniensis] (Raya et al., 2021). In the past, sisal was a very relevant source of hard fibers, which were gradually replaced by the synthetic fibers, such as polypropylene (Davis and Long, 2015). However, given the widespread comprehension of climate change and the need for biorenewables, Agave might experience a resurgence to its former status (Raya et al., 2022), reclaiming global significance not only as a source of hard fibers, but also through profoundly diversified utilization strategies of these resilient plants (Morán et al., 2008; de Paula et al., 2012; Santos et al., 2015; Pérez-Zavala et al., 2020).
Since the 1960s, Brazil is the world’s leading producer of sisal fiber, despite undergoing a significant decline in production. While in 1990 the production was 300,200 Mg annually (Silva and Beltrão, 1999), today Brazil produces around 98,000 Mg per year. This currently constitutes around 36% of the global annual sisal fiber production (FAO, 2023), with the State of Bahia alone accounting for approximately 94% of this output (IBGE, 2021). Sisal cultivation is socioeconomically relevant by providing, directly or indirectly, the main economic driver for a population of over 850,000 people in the Brazilian semiarid, where it is often the only agricultural product available (Broeren et al., 2017). Although other sisal fiber producing countries, such as China and Tanzania, cultivate the H11648 (Monja-Mio et al., 2019), Brazilian fiber production is based on the large-scale cultivation of undefined A. sisalana plants, with lower fiber yield (Azzini et al., 1989; Silva and Beltrão, 1999; Suinaga et al., 2007). One of the main reasons for this scenario is the production model based on a laborious fiber extraction with the utilization of equipment that demands manual operation (the “Paraibana” machine—see Silva and Beltrão, 1999). Therefore, the higher the leaf fiber content, the greater the worker’s effort to accomplish their job. In other countries, such as Tanzania or China, fiber extraction is accomplished by stationary machines, with the leaves being transported to small industries where they are processed (Broeren et al., 2017). In this case, high fiber content becomes an advantage and not a restriction. Nevertheless, some more productive Agave cultivars like H11648 or H400 leaves (“híbrido 400 folhas” in Portuguese, named simply H400 from this point forward) can eventually be found in sisal fields in Brazil (Silva and Beltrão, 1999; Alvarenga Jr, 2012) but at a much lower proportion. The origin of H400, also known as the “white hybrid,” is not clear, but seems to be closely related to the H11648 (Souza et al., 2018).
For the last 2 decades, sisal fiber production in Brazil has significantly declined due to the bole rot disease, which represents the primary phytosanitary issue in the country’s sisal fields (Abreu, 2010; Soares et al., 2020). This disease is caused by Aspergillus welwitschiae, a saprotrophic fungus that switches to a necrotrophic lifestyle when infecting the wounded sisal bole, destroying the parenchymal tissue (Duarte et al., 2018). The primary symptoms of this disease involve the decay of both the bole and leaf base, characterized by the appearance of internal tissue exhibiting hues that span from red to a spectrum of light and dark brown discoloration. Subsequently, secondary indications become apparent through the yellowing and wilting of leaves, eventually leading to the demise of the entire plant (Duarte et al., 2018).
Drawing from extensive field observations and the documented spread of the bole rot after an intense drought period spanning 2011-2012 (both extensively detailed in prior publications by our research group), we believe that the establishment of the necrotrophic phase of the disease hinges on physiological stress. This stress can result from nutritional deficiencies, the pressures induced by drought, or even mechanical damage arising from plant manipulation during leaf removal for fiber extraction or sucker elimination. Notably, the influence of an insect, such as Bemisia tabaci (Quintanilha-Peixoto et al., 2021), or yet by an infestation of pests, such as Dysmicoccus spp. (Wang et al., 2022), could also contribute to this injury-induced phase.
Our research group has recently discussed some molecular characteristics and mechanisms possibly involved in the pathogenicity of A. welwitschiae in sisal (Quintanilha-Peixoto et al., 2019; Quintanilha-Peixoto et al., 2022b), but there are still open questions on the disease cycle and susceptibility of fiber-producing agaves. The identification of the etiological agent, A. welwitschiae, was described only in 2018, along with a comprehensive overview of the global prevalence of sisal bole rot disease and its associated pathogen (Duarte et al., 2018). This is due to the fact that within the Nigri section of the Aspergillus genus, A. welwitschiae is considered a cryptic species of A. niger. This cryptic nature implies that distinguishing between these two species is not feasible using conventional microbiological methods like assessing colony appearance and spore morphology (Varga et al., 2011), or even relying on the primary fungal DNA barcode, nrITS (Susca et al., 2016). Consequently, the current standard for identification and comprehensive examination of the phylogeny and taxonomy of these fungi relies on the meticulous sequencing of the calmodulin (CaM) gene—a secondary marker gene for some fungal clades. This identification method, applied by Duarte et al. (2018), entails cultivating the fungus in a pure culture, extracting its DNA, amplifying the CaM gene via PCR, and subsequently subjecting it to sequencing using the Sanger technique. Efforts have been undertaken to design specific primers targeting the CaM gene for A. welwitschiae (Gherbawy et al., 2015; Palumbo and O’Keeffe, 2015), particularly for discerning strains associated with food contamination. Nevertheless, as elucidated further, these primers might exhibit amplification across other species within the Nigri section.
In this study, our aim was to develop a more reliable and user-friendly molecular marker for accurate A. welwitschiae identification. To achieve this, we diverged from the conventional approach of seeking minor polymorphisms within traditional genes, opting instead to identify exclusive regions within the genomes of A. welwitschiae, which have recently been released. Once this novel marker was validated, we employed the test on samples obtained from Brazil’s main sisal-producing regions, conducting an epidemiological investigation. The new test offers an unprecedented level of insight into bole rot disease in Brazil, shedding light on potential correlations between disease symptomatology and nutritional status, and even revealing potential cultivar-specific effects. This new tool presents novel opportunities for disease management, not only within Brazil but also in sisal-producing regions worldwide.
2 Materials and methods
2.1 A. welwitschiae unique regions detection, primer design, and validation
For the development of a set of primers targeting the detection of A. welwitschiae, we performed an analysis of orthologous genes using the gene-coding sequences (CDS) of 40 Aspergillus strains and two other fungal species, Neurospora crassa and Penicillium rubens. In this analysis, three strains of A. welwitschiae were used, being CBS159.1 isolated from Welwitschia mirabilis and CCMB 663 and CCMB 674 isolated from sisal plants. We used the same set of species (Supplementary Table S1) as seen in Quintanilha-Peixoto et al. (2022b) to find unique genes in A. welwitschiae and design primers that could be used to diagnose the bole rot disease. We used OrthoFinder v. 2.5.2 (Emms and Kelly, 2018) to perform the orthologous analysis, using the parameter “-d” for DNA input. From the results, we selected families that contained only A. welwitschiae genes.
In order to design the primer sequences, we used Primer-BLAST (available at https://www.ncbi.nlm.nih.gov/tools/primer-blast/) under default settings and “A. welwitschiae” as the organism option. Considering the housekeeping function of the gene selected as a marker in A. welwitschiae, we complemented OrthoFinder ortholog designation with a search for paralogs in A. fumigatus Af293, A. nidulans FGSC A4, and A. niger N402, which were also compared against A. welwitschiae CCMB 674 and A. welwitschiae CCMB 663 in the primer set validation.
Selected sets of primers were tested and then validated with ECRA HIFI DNA Polymerase (ECRA Biotec), following manufacturers procedures. After an initial in vitro screening, one primer set (Aw_8 F: CACCTGATCCTGGAGGGAGA, Aw_8 R: AGCCACCTGGAGAAGCAATC) was selected and optimized by adding DMSO to the reaction mix and testing different annealing temperatures to increase specificity. Primers previously described in the literature as capable of segregating A. welwitschiae and A. niger were also used as external controls. One pair of primers described by Palumbo & O’Keeffe (2015) (Aw F: GGGATTTCGACAGCATTTCTCAGAATT and Aw R: GATAAAACCATTGTTGTCGCGGTCA) and the other by Gherbaway et al. (2015) (awaspec: ATTTCGACAGCATTTCTCAGAATTA and cmd6: CCGATAGAGGTCATAACGTGG) were selected.
2.2 Plant material and testing
A total of 179 samples of sisal plants from the main producing areas in Bahia were tested. Samples were collected in August 2019 from three regions known as “identity territories” (Figure 1) demarcated by environmental, economic, and cultural criteria (Secretaria do Planejamento do Estado da Bahia, 2016). The first territory, Piemonte da Diamantina, is in the North of Bahia at an mean altitude of 740 m, has an average annual maximum air temperature of 29.8°C and a minimum of 16.2°C (Fick and Hijmans, 2017), and an average annual rainfall of 492 mm (INMET, 2023). Sampling sites of Piemonte da Diamantina included the municipalities of Jacobina, Várzea Nova, and Ourolândia, which jointly account for 11,457 Mg of sisal fiber produced per year (IBGE, 2021). The second territory, Piemonte Norte do Itapicuru, is farther north at an average altitude of 680 m, with a maximum annual air temperature of up to 30.7°C and a minimum of 16.3°C (Fick and Hijmans, 2017), and average annual rainfall of 580 mm (INMET, 2023). The sampling sites in this territory include only different areas of the municipality of Campo Formoso, the largest sisal-producing municipality in Brazil, with 22,546 Mg of fiber produced per year (IBGE, 2021). The third territory, Sisal, which is farther east than the others, has lower mean altitude (600 m) and an annual maximum air temperature of 30.3°C and a minimum of 17.4°C (Fick and Hijmans, 2017) with an average annual rainfall of 511 mm (INMET, 2023). Samples were collected from the municipalities of Conceição do Coité, Valente, Retirolândia, Itiúba, and Monte Santo, which are responsible for the annual production of 20,397 Mg of fibers (IBGE, 2021). For each territory, 45, 43, and 91 samples were tested from Piemonte da Diamantina, Piemonte Norte do Itapicuru, and Sisal territories respectively. In the Sisal territory, more samples were collected as production areas with hybrid cultivars were found during the survey, specifically in Itiúba. The three sisal cultivars sampled are represented in Figure 2.
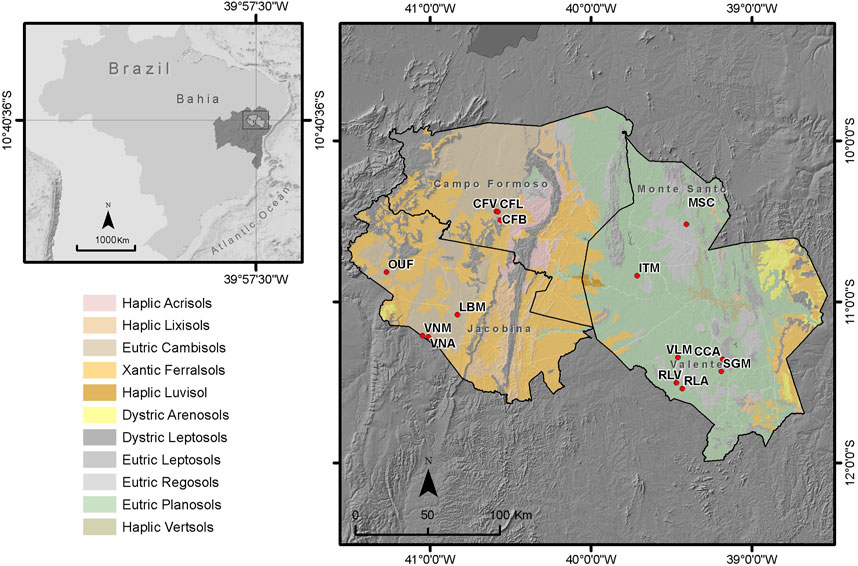
FIGURE 1. Sampling sites and domain map of soils cultivated with sisal in Bahia. On the main map, from left to right are the Piemonte da Diamantina, Piemonte Norte do Itapicuru, and Sisal territories. OUF, Ourolândia; VNM, Várzea Nova—Mulungu; VNA, Várzea Nova—Angicos; LBM, Jacobina—Lages do Batata; CFV, Campo Formoso—A volta; CFL, Campo Formoso—Ladeira do Vivi; CFB, Campo Formoso—Baixão; ITM, Itiúba; MSC, Monte Santo; RLV, Retirolândia—Bela Vista; RLA, Retirolândia—Alto Bonito; VLM, Valente; CCA, Conceição do Coité; SGM, Conceição do Coité.
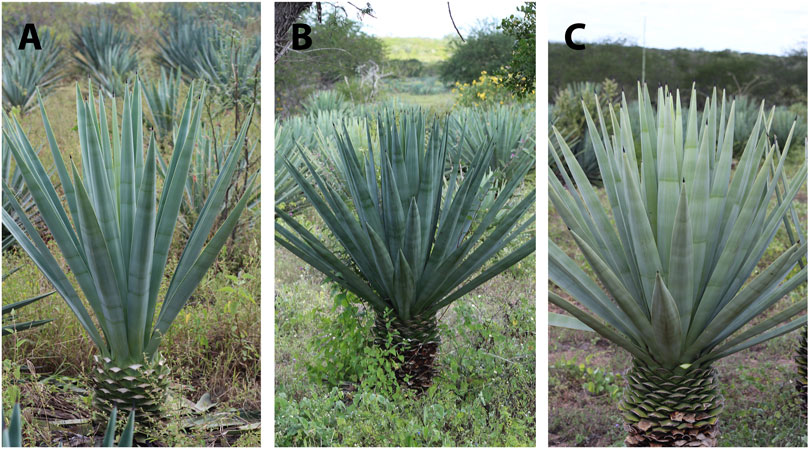
FIGURE 2. The sisal cultivars used in Brazil. (A) Agave sisalana; (B) H11648 [(A. amaniensis x A. angustifolia) x A. amaniensis]; (C) H400 leaves.
Leaf samples were collected and maintained in styrofoam coolers with ice, until arrival at the laboratory, where they were frozen in liquid nitrogen. Total DNA was extracted from leaves using the Sahu et al. (2012) protocol. A. welwitschiae detection was performed as the conditions described in the previous section, but using Agave’s 18S (Martinez-Hernandez et al., 2010) (Atq-rRNA18SF CTGCCGTCCCGTCCTGCCGTCCCGTCCCTTCTGC and Atq-rRNA18SR CCTGGTGGTGCCCTTCCGTCAA) as an endogenous control and DNA extracted from A. welwitschiae strain CCMB 674 was selected as an exogenous control. The results were evaluated according to the presence (positive) or absence (negative) of PCR product at the correct molecular weight.
2.3 Soil analyses
The sisal-producing areas, inserted in the semi-arid region of Bahia, display a great environmental diversity, especially in relation to climate, geology, geomorphology, and local vegetation, exhibiting a high diversity of soil classes. Soils cultivated with sisal range from undeveloped, shallow, fertile, and stony to well-weathered, deep, chemically poor, and clayey soils. According to the updated soil map of Bahia (IBGE, 2018) and the soil reclassification according to FAO/WRB (2015), predominate in the territories of Piemonte da Diamantina and Piemonte Norte do Itapicuru the Xantic Ferralsols (Dystric) and the Eutric Cambisols, with less occurrence of Dystric Leptosols, which is considered of low potential for sisal cultivation.
Soil samples were collected from Eutric Planosols (ITM and RLV), Eutric Cambisols (CFB, CFV, CFL, VNA, VNM, LBM, and OUF) and Eutric Regosols (VLM and CCA). For each site, composite samples were collected, from 0–20 cm, within 1 ha (Raij et al., 2022) and sent to LAGRO—Laboratório Agronônimo S/C Ltda (Indaiatuba, SP) for determination of soil granulometry and fertility (macro and micronutrients), according to the methodology described by Raij et al. (2001). Considering that there are no reference values available for classifying soil nutrients in terms of availability as low, medium and high, the guidelines for Cerrado soils were used as the standard for macronutrients (Lopes and Guimarães, 1989). For micronutrients, the references described for perennial species in the State of São Paulo were used (Raij et al., 2022).
2.4 Data integration and analysis
Epidemiological parameters were calculated according to Vale et al. (2004). The incidence of the pathogen (PI) was calculated through:
where
The prevalence (
where
The dispersion index (
where
In order to understand the potential effect of soil characteristics on the incidence of A. welwitschiae and persistence of bole rot symptoms, we applied multivariate linear regression to the dataset. Because of the small sample of fields (n = 11), the dependent variables set of the regression model had to be reduced, so that the system would not be overdetermined. Therefore, we selected the top nine soil characteristics based on the highest Pearson correlation with pathogen incidence and symptom (Supplementary Figure S1) and possible direct implications on plant health. All selected characteristics had correlation coefficients (r-values) higher than 0.4. With the selected soil characteristics (P, K, Ca, Mg, CEC, V%, Na, Mn, Cu), we used the multivariate model for both (i) pathogen incidence per field and (ii) the proportion of symptomatic individuals in that field:
Where
3 Results
3.1 Detection of unique regions for the diagnosis of the bole rot disease in Agave sisalana
Initial tests with primers described in the literature by Palumbo and O’Keeffe (2015) and Gherbawy et al. (2015) did not excel at differentiating our strains of A. welwitschiae (CCMB 674 and CCMB 663) from A. fumigatus Af293, A. nidulans FGSC A4, and A. niger N402 (Figure 3). Meanwhile, our orthologous analysis using nucleotide sequences initially described 51 orthologous clusters exclusive to our 3 A. welwitschiae strains. 35 orthologous clusters, initially annotated as “hypothetical protein,” were tested on PrimerBLAST for obtaining common PCR products. Among these, genes in four clusters did not share enough similarity for primer design, and 13 produced off-target hits in the A. welwitschiae genome. The top 10 longest PCR products from the remaining clusters were selected for in vitro testing. Among the preselected primers, only one primer set, Aw_08, was able to differentiate A. welwitschiae from A. niger (Supplementary Figure S2), producing a polymorphic PCR product with higher molecular weight. For this primer set, we performed further reaction optimization tests to improve specificity, and subsequently tested against A. niger, A. fumigatus, and A. nidulans (Figure 3). By increasing the annealing temperature to 64°C, Aw_8 was able to identify only the A. welwitschiae phytopathogenic strains (Supplementary Figure S3) (Quintanilha-Peixoto et al., 2022a), and with a sensitivity up to 0.75 ng μL−1 of DNA per sample (Supplementary Figure S3).
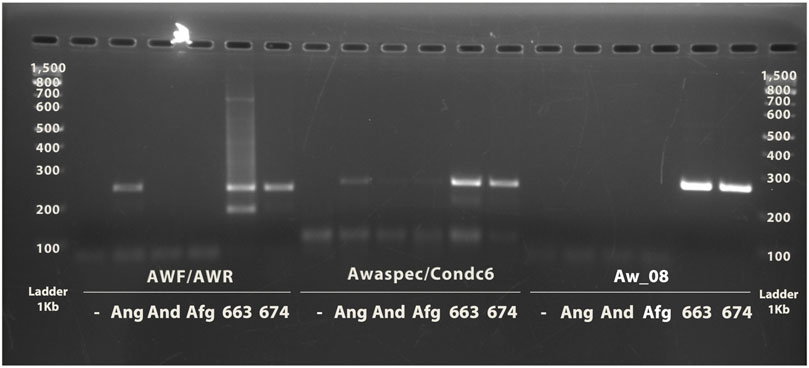
FIGURE 3. In vitro analysis of candidate primers for molecular diagnosis of sisal bole rot disease. -: negative control, Ang: Aspergillus niger N402, And: Aspergillus nidulans FGSC A4, Afg: Aspergillus fumigatus Af293, 663: Aspergillus welwitschiae strain CCMB 663, 674: Aspergillus welwitschiae strain CCMB 674, AWF/AWR: primers set described by Palumbo and O’Keeffe, (2015), Awaspec/Condc6: primers set described by Gherbaway et al. (2015), Aw_08: candidate primers set, and Ladder 1kb: 1Kb KASVI marker.
The full annotation of the proposed marker gene can be found in Supplementary Table S2. To further investigate our primer set specificity we searched for PC5R paralogs in the other genomes used for validation (A. fumigatus Af293, A. nidulans FGSC A4, and A. niger N402). This paralog analysis revealed other PC5R sequences sharing similarity with the A. welwitschiae sequences clustered by OrthoFinder. However, such sequences were highly divergent (Figure 4C), which explains our primer specificity. The closest sequence, XM_677456.1 from A. nidulans FGSC A4, shares a 69% identity region, which covers only 52% of the A. welwitschiae sequence.
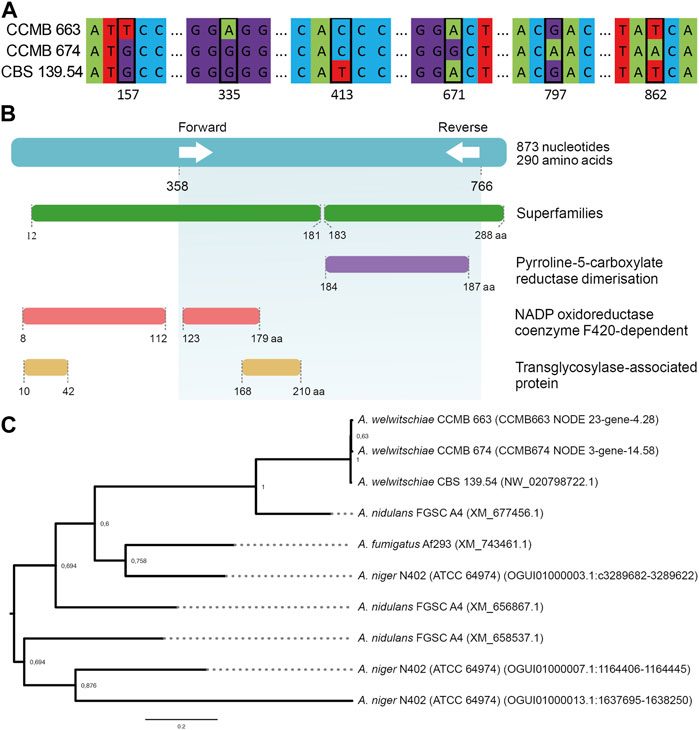
FIGURE 4. (A) SNPs in the PC5R genes of the 3 A. welwitschiae isolates (B) Representation of the flanking regions of the selected primer set (first layer and green shadow), protein superfamilies, and protein domains in the selected gene, according to hmmscan (C) PC5R paralogs phylogeny with bootstrap support.
3.2 Molecular epidemiology
The developed molecular marker successfully identified the presence of A. welwitschiae in sisal leaves. Out of all the collected samples, those showing evident symptoms of bole rot disease consistently tested positive for A. welwitschiae, whereas negative results were obtained from visually healthy plants. Through molecular analysis, we observed a pathogen prevalence of 100% in sisal production areas. A. welwitschiae incidence within the sampling sites varied from 25% to 100% (Table 1). In contrast, symptoms occurrence ranged from 0% to 56%. The territories of Piemonte Norte do Itapicuru and Sisal are traditionally known for the more intense cultivation of sisal, in particular the municipalities of Campo Formoso (largest producer) and Valente (largest fiber industrial units). In these territories, approximately 17% of the sampled plants showed bole rot symptoms. Among all municipalities, Retirolândia (Sisal territory) had the highest occurrence of sisal bole rot symptoms. The territory that presented the higher incidence was Sisal, with 88%. Piemonte da Diamantina had the lowest incidence, with 78%, and it was the territory with the highest asymptomatic rate. The lowest incidence was found in Ourolândia (Piemonte da Diamantina territory), the municipality farthest from the territory with the highest incidence of the disease (Sisal). For all territories, we encountered a dispersion index lower than 1, indicating that this disease has a regular dispersion pattern.
In addition, the incidence of A. welwitschiae and occurrence of symptoms were compared amongst the sisal cultivars (A. sisalana, H11648, and H400) (Table 2). Among them, the common sisal (A. sisalana) showed the highest proportion of symptomatic individuals, while no symptoms were observed for H400. Furthermore, A. welwitschiae was detected in 76% of the H11648 plants analyzed, but only 10% of this population showed symptoms. Nonetheless, it is important to point out that the presence of hybrid cultivars in commercial plantations is uncommon, and 82% of the hybrid cultivars encountered occurred in the same municipality (Itiúba).
3.3 Physical and chemical properties of soil samples
The fertility analysis revealed acid to weak alkaline soils (pH 4.9–7.3), with average organic matter, and rich in Ca and Mg (Table 3). Also, no Al was found, and the potential acidity (H+ + Al³+) was low, which is consistent with the pH. Base saturation (V%) was classified as high to very high (>90), with Ca and Mg levels as major contributors. Accordingly, the cation exchange capacity (CEC) was average to high, indicating soils with overall good natural fertility. Soil P availability varied from 6 to 54 mg dm−3 among the sites, which is considered low for most perennial crops (Raij et al., 2022). Only Retirolândia and Conceição do Coité exhibited P levels above the standard. For K, we found high variations, from 0.6 to 6.9 mmolc dm−3 and CFV, ITM, VLM, and RLV displayed the lowest values. Sulphur ranges were low to medium, ranging from 2.3 to 8.0 mg dm−3.
For micronutrients, soil Mn and B levels were mostly high, while Cu and Zn were low (Table 4). As for Fe, the samples ranged from 4 to 49 mg dm−3. Specifically for the Sisal territory, only high Fe levels were found. It is important to emphasize that pH directly influences the availability of several nutrients, and soils with higher alkalinity have less availability of micronutrients, such as Fe, Zn, Cu, and Mn, and more availability of Ca, Mg, K, P, S, and B. Soil Na varied from 0.2 to 2.4 mmolc dm−3 among the sites. Granulometric analysis of soil samples indicated a predominant texture of sandy to medium, with sandy clay loam textural class being more frequent (Supplementary Table S3).
The PCA grouped the soil samples according to textural class, separating the sandier soils on the surface: Eutric Planosols (ITM), Eutric Regosols (VLM) and some Eutric Cambisols (CFB and VNA), from those Cambisols with medium to clayey texture (Figure 5), showing that the soil granulometry parameters (clay, silt, and sand) were the main factor that contributed to sample segregation followed by CEC and Ca. Despite this grouping, the greater dispersion of soils demonstrates that there is diversity in soil composition within territories and even within the same municipality, as can be seen in the segregation between Várzea Nova samples. The studied Cambisols, associated with the Xantic Ferralsols (Eutrics), with great occurrence in the municipalities of Campo Formoso and Jacobina, are considered soils of medium to high potential for the cultivation of sisal since they are from little to very deep, medium to high fertility, medium to clayey texture and then have reasonable water holding capacity. There are also occurrences of Dystric Leptosols in these territories, which predominate in the Sisal territory, along with Eutric Planosols, Eutric Regosols, and Abruptic or Haplic Solonetzs. These less weathered soils are considered of low potential for the cultivation of sisal, as they present medium to low fertility, reduced effective depth, sandy texture, low water retention capacity, and/or high salinity, factors that, associated with the semi-arid climate of the region, lead to greater stress for cultivated plants.
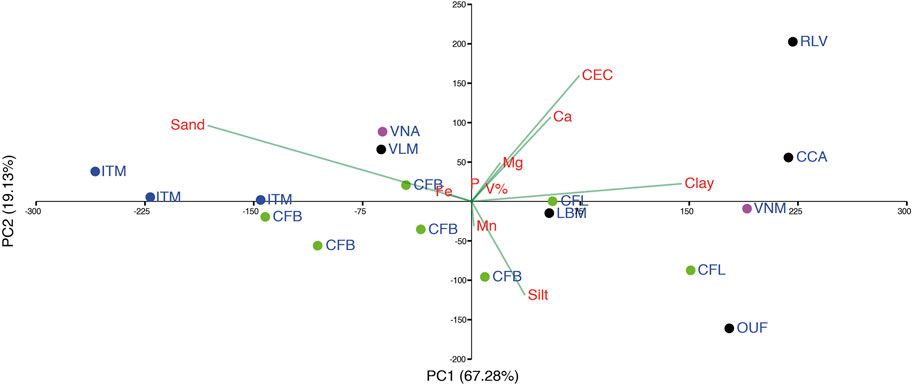
FIGURE 5. Principal component analysis of soil samples. In red are the soil traits that most contribute to the separation of the samples, and the green lines of these represent the factor contribution. OUF, Ourolândia; VNM, Várzea Nova—Mulungu; VNA, Várzea Nova—Angicos; LBM, Jacobina—Lages do Batata; CFV, Campo Formoso—A volta; CFL, Campo Formoso—Ladeira do Vivi; CFB, Campo Formoso—Baixão; ITM, Itiúba; MSC, Monte Santo; RLV, Retirolândia—Bela Vista; RLA, Retirolândia—Alto Bonito; VLM, Valente; CCA, Conceição do Coité; SGM, Conceição do Coité.
Multivariate linear regression analysis revealed significant (p-value ≤0.1) interactions between symptom occurrence and soil characteristics (Table 5). The adjusted model presented multiple R2 of 0.9936 and adjusted R2 of 0.9363. The generated matrix (Supplementary Figure S4) displayed the expected correlations between the nutrient availability and pH (Hartemink and Barrow, 2023), e.g., P, K, Ca, and Mg, demonstrating that the soil data are consistent. The soil traits with significance were P, K, Ca, Mg, CEC, and V%. Among them, K, Ca, and Mg presented negative correlations with symptom occurrence. The analysis summary for interaction with pathogen incidence is depicted in Supplementary Table S4.
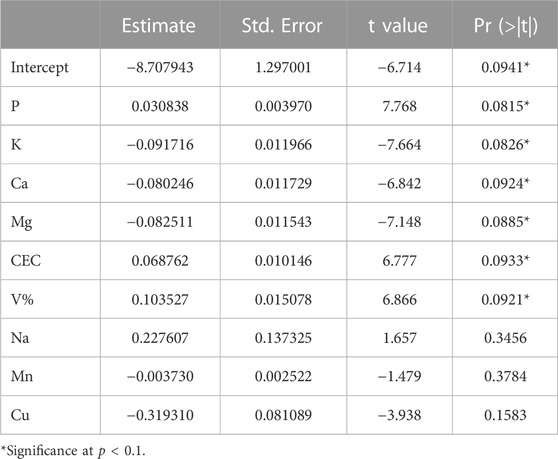
TABLE 5. Multivariate linear regression analysis for the presence of bole rot symptoms against soil characteristics.
4 Discussion
Primer development for the detection and differentiation of Aspergillus species has proven to be a challenge. Even though a couple of studies have tackled this issue (Gherbawy et al., 2015; Palumbo and O’Keeffe, 2015), both studies developed primer sets based on the Calmodulin gene and validated them with local samples. Calmodulin is a housekeeping gene present in all eukaryotes, and its application for phylogenetic studies of the Aspergillus genus is a consensus because it can be easily amplified and a large dataset is available in public databases (Samson et al., 2014; Tam et al., 2014; Alshehri and Palanisamy, 2020). Nonetheless, the proper identification of several Aspergillus species of section Nigri is often based on single-nucleotide polymorphisms (SNPs) of the CaM gene. Relying on such small nuances might decrease PCR specificity, leading to false positive results. When testing those literature primers, we observed PCR amplification for A. nidulans, A. fumigatus, and A. niger (Figure 3), which can be misleading, since these species can behave as saprotrophs (Tekaia and Latgé, 2005) and/or as endophytes (Vijayanandan and Balakrishnan, 2018; Xu et al., 2018; Aziz et al., 2021) and, therefore, can easily be found in complex samples. In this study, we prospected potential targets and designed new primers from orthologous clusters unique for the 3 A. welwitschiae strains within their available genomes. Among the developed primer sets, Aw_08 presented the best outcome, proving to be specific, highly sensitive, and effective in complex samples. This new marker gene codes for a D1-Pyrroline-5-Carboxylate Reductase (P5CR) (Figure 4B), which participates in the proline metabolism (Porcel et al., 2004; Yadav et al., 2020) and has also been associated with pathogenicity in Cryphonectria parasitica (Yao et al., 2013). The PC5R sequences are nearly identical in all three examined strains of A. welwitschiae, differing by only five nucleotides (Figure 4A). These variations do not exert a significant impact on the amino acid sequence or structure. The phylogenetic analysis of Aw-P5CR indicates that the closest sequences present in A. fumigatus Af293, A. nidulans FGSC A4, and A. niger N402 genomes are highly divergent paralogs (Figure 4C), which might contribute to the specificity of this target in comparison with other Aspergillus species.
The new primer set (Aw_8) was able to amplify the Aw-P5CR gene from complex samples. With high sensitivity, the test not only detected A. welwitschiae in leaves of symptomatic plants but also in samples of apparently healthy plants. This is a significant advancement for research with sisal bole rot disease, regarding epidemiological and control studies, since, until date, the pathogen’s proper identification depended on a combination of microbiology techniques with Sanger sequencing (Duarte et al., 2018). The traditional identification methods are also destructive, requiring internal stem samples that implicate cutting and destroying the whole plant. Using leaf samples is an alternative that allows periodic assessments to detect the pathogen at the early stages of plant infection and colonization, before bole rot symptoms become visible. Indeed, our epidemiological study with the Aw_08 primer set detected A. welwitschiae in 81%–89% of A. sisalana asymptomatic plants, as well as in the asymptomatic hybrids H11648 and H400.
The last published research quantifying sisal bole rot disease in Bahia described a 100% prevalence, while the disease incidence varied from 5% to 40% (Coutinho et al., 2006; Abreu, 2010). Despite the gap of over 10 years, our numbers are consistent with those previous results. In our dataset, there is a prevalence of 100%, while symptom occurrence ranges from 0% to 56% (Table 1). Furthermore, the use of molecular epidemiology techniques brought a higher resolution to this analysis. In our data, 81%–89% of sampled sisal plants did not present any visual symptoms but tested positive for the presence of A. welwitschiae. Moreover, this pathogen was also detected in the hybrids H11648 and H400, although only 10% of H11648 plants were symptomatic and all plants of H400 were asymptomatic. According to the dispersion index, the incidence of the disease is regular, indicating, for example, that the spread of the disease may be related to the propagation of contaminated suckers (Duarte et al., 2018; Soares et al., 2020). Appropriately, the location with the lowest disease incidence is the farthest from the territory with the highest incidence. No significant correlation was found between pathogen incidence and soil nutritional analysis, possibly due to the high infection rate, which suggests that the incidence is probably more influenced by the common use of contaminated plant suckers by sisal farmers as planting material.
With the sisal market crisis triggered by the development of synthetic fibers in the late 1960s, investments in research with this crop were either reduced or canceled. In Brazil, the main example of this process was the Agave breeding program. From the 1950s-80s, the Agronomic Institute (IAC) was the country’s leader in the development of new Agave cultivars (Medina, 1954; Medina, 1956; Medina, 1959; Ciaramello et al., 1975; Azzini and Ciaramello, 1977; Salgado et al., 1979; Raya et al., 2023). However, with the lack of investment and the retirement of the leading researchers, there was no continuity of this program (Raya et al., 2021; Raya et al., 2023). Currently, there is no sisal varietal control in Brazil and producers are not concerned with this topic. Nonetheless, with the advancement of bole rot disease, this scenario begins to change. For instance, in Itiúba the high incidence of disease and the susceptibility of the common sisal made many producers lose their entire fields, which pushed those farmers to invest in planting hybrid cultivars, which, according to their popular knowledge, would be more resistant to the disease. Accordingly, we observed a difference in symptomatology between common sisal and hybrid cultivars, especially H400 which did not present any symptoms (Table 2). Still, further experiments need to be performed to confirm this possible resistance or tolerance. Hybrid cultivars are not widespread in Brazil’s sisal farms, mainly because farmers claim that fiber extraction is hampered by leaf rigidity and the foul smell of the bagasse, which could be easily overcome with the adoption of stationary machines.
There is an important parallel between the tequila industry in Mexico and the Brazilian sisal situation that should be pointed out. In the late 1990s, the combination of two pathogens (Fusarium oxysporum and Erwinia spp.) together with a significant weather event wiped out 1/5 of all Agave tequilana planted in Jalisco (Jiménez-Hidalgo et al., 2004; Valenzuela-Zapata and Nabhan, 2004; Vega-Ramos et al., 2013; María de Jesús et al., 2017). In both cases, there was the indiscriminate use of only one clonal line and the presence of opportunistic necrotrophic pathogens. In the Brazilian scenario, this situation could be potentially worse. As the A. sisalana cultivated worldwide originated from plants taken to Florida by Henry Perrine in 1836 (Medina, 1959), A. sisalana may have higher genetic drift than other cultivated agaves. According to our data, 84% of the A. sisalana plants are contaminated with A. welwitschiae, and 16% present visible symptoms of bole rot disease (Table 2). The process by which bole rot disease changes from an asymptomatic to a symptomatic phase is complex and yet to be described. However, disease progression might be triggered by nutritional deficiency and/or other abiotic stresses such as water deficit, or even changes in the microbiome (Medina, 1954; Duarte et al., 2018; Quintanilha-Peixoto et al., 2021).
Based on our analysis, it appears that plant nutrition could play a significant role in this pathosystem. Increased K in soil might inhibit bole rot disease symptoms manifestation. Overall, K supplementation has shown to improve plant resistance to pathogens, decreasing the incidence of fungal diseases by 70% (Perrenoud, 1977), and even disease severity (Williams and Smith, 2001), possibly by modulating either basal susceptibility or constitutive defense mechanisms, such as cell wall architecture or secondary metabolites production (Amtmann et al., 2008; Wang et al., 2013). In contrast, we found that higher P could increase symptoms occurrence. Generally, P tends to improve plant health; however, it can also increase pest infestation or disease severity in several models, possibly by improving pathogen nutrition (Perrenoud, 1990; Develash and Sugha, 1997; Walters and Bingham, 2007).
We also noticed that CEC and V% appear to contribute to an increase in symptom occurrence, whereas Ca and Mg seem to have a mitigating effect. Both Ca and Mg are essential minerals for plants, and their balance has direct effects on pathogen-host interactions. For instance, Fusarium wilt, a necrotrophic pathogen, presents less severe infections when adequate Mg is available (Huber and Jones, 2013) and Ca is not only crucial for early signaling events for plant defense responses but its supplementation has been shown to reduce Phytophthora infections (Sugimoto et al., 2010; Zhang et al., 2014). In our data, soil Ca and Mg were found in concentrations considered to be high for most crops (Raij et al., 2022). CEC and V% measurements are both dependent on Ca, Mg, K, and Al levels. Since no Al was detected, and K varied among soil samples, Ca and Mg were the main contributing factors for CEC and V% correlation to symptom occurrence, which is confirmed by the correlation matrix (Supplementary Figure S4). Ca and Mg might contribute to overall soil salinity, which can decrease biotic stress responses, increasing plant susceptibility to pathogens (Bai et al., 2018). Although Ca-deficiency was previously described as a possible aggravating factor for bole rot disease (Medina, 1954; Duarte et al., 2018), higher soil Ca levels may be detrimental. Besides possible implications on the host health, Ca levels could be influencing the pathogen’s physiology and modulating its virulence. For instance, the recent study of the A. welwitschiae genome revealed that Na+/Ca2+ exchanger genes were under positive selection on phytopathogenic strains (Quintanilha-Peixoto et al., 2022b). Also, Agave accumulates calcium oxalate as a herbivory defense (Salinas et al., 2001; Corbin et al., 2015), but fungal necrotrophic pathogens can use calcium oxalate crystals to improve pathogenicity (Uloth et al., 2015). Nutrient uptake is a complex process, and its relationships can be quite specific to each pathosystem. This challenge becomes even more pronounced when studying semiarid crops that naturally employ different strategies to cope with abiotic stresses, such as salinity and drought. The multivariate regression analysis provides intriguing insights into the nutrient-health relationship. However, the limited data hampers confidence in obtaining more meaningful results. In order to comprehend the interplay between disease progression and mineral nutrition, a comprehensive investigation of the nutritional requirements for sisal cultivars is still necessary and would greatly benefit from a thorough study.
The hybrid cultivars are more productive than the common sisal, producing 30% more leaves per year and heavier leaves (Silva and Beltrão, 1999). Productivity is a multifactor trait being the outcome of several processes like photosynthesis, nutrient absorption, or stress tolerance. Differences between these cultivars’ metabolism are beginning to be described and would be crucial to understand their agronomical performance. For instance, the H11648 biomass was described as more recalcitrant than A. sisalana (Raya et al., 2021), which can enhance resistance to necrotrophic pathogens (Miedes et al., 2014) or abiotic stresses (Moura et al., 2010). Also, cultivar interactions with sisal microbiome might be unique. Even though taxonomically similar microorganisms in A. sisalana and H11648 were found, transcriptomic analysis of these fungi revealed that the same groups might be using different metabolisms in each cultivar (Marone et al., 2022). The combination of these specific mechanisms may contribute to robust hybrids that simply make them healthier and, therefore, more able to deal with pathogenic microorganisms, such as A. welwitschiae.
The sisal market crises have changed the profile of sisal plantations in Brazil. Originally, sisal farms were large-scale operations (Medina, 1954; Silva and Beltrão, 1999). Now, the fiber is mainly produced by smallholders with little to no agricultural management (Medina, 1954; Silva and Beltrão, 1999; Broeren et al., 2017). Although Brazil is still the world’s largest producer, this agro-extractivism system directly reflects in the country’s fiber productivity. Nowadays, Brazil is only the 12th country in fiber productivity (FAO, 2023), being five times lower than the first in the ranking (China). The lack of disease control measures and low level of technology used in sisal field management, particularly the low quality of sisal plants used for planting new fields, have contributed to the spread of bole rot disease (Soares et al., 2020) but also limits farmers investment capacity. In this scenario, a potential phytosanitary crisis arises. The current propagation system of this crop is through rhizomes or suckers that may already be contaminated (Kritzinger et al., 1997; Silva and Beltrão, 1999; Applequist, 2003; Li et al., 2017). Another aggravating factor is that there are no nurseries or biofactories (large-scale tissue culture laboratories) producing sisal plants with high phytosanitary quality. Even with access to healthy plants, currently economic fragility of sisal producers is a barrier that blocks the plantations from being renewed with proper field management technologies.
In order to prevent this possible crisis, it is urgent for the sector to mobilize. Through public policies, it would be possible to restore farmers’ investment capacity and encourage the production of healthy plants through tissue culture techniques, given that this technology is already mastered for Agave (Das, 1992; Bautista-Montes et al., 2022) and is routinely used in other crops (Gerald et al., 2007; Gerald et al., 2011). It is paramount for farmers to be able to count on a broader variety of species/genotypes and affordable clean plants. If the country wishes to maintain the long-term feasibility of sisal production or even correspond to Agave as biorenewables feedstock prospects (Raya et al., 2022), the Agave breeding program must be re-established. In fact, the sisal production system in Brazil needs to be rethought, as the current one depends on decentralized and semi-automatic fiber extraction process, which makes it difficult to introduce and disseminate more robust productive varieties with greater tolerance to the disease. Nonetheless, field removal of plants with bole rot symptoms and the use of healthy plants for sisal plantations are still the main disease control strategies recommended and farmers need to be encouraged (and able) to follow it. Finally, the set of primers developed in our study is an important tool to implement a disease monitoring program using adequate and more precise techniques, such as detection of the pathogen by PCR, before symptoms manifestation. This approach can significantly enhance early detection and management strategies.
Data availability statement
The original contributions presented in the study are included in the article/Supplementary Material, further inquiries can be directed to the corresponding author.
Author contributions
FR: Conceptualization, Methodology, Validation, Formal analysis, Investigation, Data curation, Writing- Original draft preparation, Writing- Review and editing, Visualization. GQ-P: Conceptualization, Methodology, Software, Formal analysis, Investigation, Data curation, Writing- Original draft preparation, Writing- Review and editing, Visualization. AdO: Conceptualization, Methodology, Formal analysis, Investigation. MM: Methodology, Software, Investigation, Data curation, Writing- Original draft preparation, Writing- Review and editing. WdR: Methodology, Validation, Formal analysis, Investigation. JM: Methodology, Software, Formal analysis, Writing–Original Draft, Writing–Review and Editing. RA: Formal analysis, Writing–Original Draft, Writing–Review and Editing. CF: Writing- Original draft preparation, Writing- Review and editing, Visualization. OC: Resources, Writing- Original draft preparation, Writing- Review and editing, Visualization. RR: Writing- Original draft preparation, Writing- Review and editing, Supervision. AS: Conceptualization, Writing- Original draft preparation, Writing- Review and editing, Resources, Project administration, Funding acquisition. AG-N: Conceptualization, Writing- Original draft preparation, Writing- Review and editing, Resources, Project administration, Funding acquisition. GP: Conceptualization, Writing- Original draft preparation, Writing- Review and editing, Resources, Project administration, Funding acquisition. All authors contributed to the article and approved the submitted version.
Funding
This work was supported by Coordenação de Aperfeiçoamento de Pessoal de Nível Superior—Brazil (CAPES) (grant number 88882.329521/2019-01 and 88887.373979/2019-00); Fundação de Amparo à Pesquisa do Estado de São Paulo (FAPESP) (grant number 2020/02524-0); Fundação de Amparo à Pesquisa do Estado de Minas Gerais (FAPEMIG) (grant number 5.18/2022, quote 439); Conselho Nacional de Desenvolvimento Científico e Tecnológico (CNPq)—Nexus Project: Sisal-Caatinga Integration (grant number 441625/2017-7); Agência Nacional do Petróleo, Gás Natural e Biocombustíveis do Brasil (ANP) in association with Shell Brasil Petróleo Ltda, with investments from the PD&I Clauses, for the Brazilian Agave Development Program (BRAVE) (research agreements 5902/5903).
Acknowledgments
We would like to thank all the members of the research groups of Ana Soares, Aristoteles Góes-Neto, and Gonçalo Pereira for their support in the execution of this research, the Funding Agencies for the supporting of this research, and Dr. André Damasio and Natália Wassano of the Laboratory of Enzymology and Molecular Biology of Microorganisms (LEBIMO) from Unicamp for sharing the reference Aspergillus strains.
Conflict of interest
The authors declare that the research was conducted in the absence of any commercial or financial relationships that could be construed as a potential conflict of interest.
The author AG-N declared that they were an editorial board member of Frontiers at the time of submission. This had no impact on the peer review process and the final decision.
Publisher’s note
All claims expressed in this article are solely those of the authors and do not necessarily represent those of their affiliated organizations, or those of the publisher, the editors and the reviewers. Any product that may be evaluated in this article, or claim that may be made by its manufacturer, is not guaranteed or endorsed by the publisher.
Supplementary material
The Supplementary Material for this article can be found online at: https://www.frontiersin.org/articles/10.3389/fceng.2023.1174689/full#supplementary-material
References
Abreu, K. C. L. D. M. (2010). Epidemiologia da podridão vermelha do sisal no estado da Bahia. [Doctorate’s thesis]. Cruz das Almas (BA): Universidade Federal do Recôncavo da Bahia. Available at: http://hdl.handle.net/123456789/547.
Alshehri, B., and Palanisamy, M. (2020). Evaluation of molecular identification of Aspergillus species causing fungal keratitis. Saudi J. Biol. Sci. 27, 751–756. doi:10.1016/j.sjbs.2019.12.030
Alvarenga, E. R. (2012). Cultivo e aproveitamento do Sisal (Agave sisalana). Belo Horizonte, Fundação Centro Tecnológico de Minas Gerais-CETEC.
Amtmann, A., Troufflard, S., and Armengaud, P. (2008). The effect of potassium nutrition on pest and disease resistance in plants. Physiol. Plant. 133, 682–691. doi:10.1111/j.1399-3054.2008.01075.x
Applequist, W. L. (2003). Rhizome and root anatomy of potential contaminants of Actaea racemosa L. (black cohosh). Flora - Morphol. Distrib. Funct. Ecol. Plants 198, 358–365. doi:10.1078/0367-2530-00108
Aziz, L., Hamayun, M., Rauf, M., Iqbal, A., Arif, M., Husssin, A., et al. (2021). Endophytic Aspergillus Niger reprograms the physicochemical traits of tomato under cadmium and chromium stress. Environ. Exp. Bot. 186, 104456. doi:10.1016/j.envexpbot.2021.104456
Azzini, A., and Ciaramello, D. (1977). Dimensões das fibras em Agave. Bragantia 36, 35–38. doi:10.1590/S0006-87051977000100038
Azzini, A., Ciaramello, D., Salgado, A. L. D B., and Zullo, M. A. T. (1989). Caracterização tecnológica de híbridos de sisal. Bragantia 48, 113–124. doi:10.1590/S0006-87051989000100011
Bai, Y., Kissoudis, C., Yan, Z., Visser, R. G. F., and van der Linden, G. (2018). Plant behaviour under combined stress: tomato responses to combined salinity and pathogen stress. Plant J. 93, 781–793. doi:10.1111/tpj.13800
Bautista-Montes, E., Hernández-Soriano, L., and Simpson, J. (2022). Advances in the micropropagation and genetic transformation of agave species. Plants 11, 1757. doi:10.3390/plants11131757
Broeren, M. L. M., Dellaert, S. N. C., Cok, B., Patel, M. K., Worrell, E., and Shen, L. (2017). Life cycle assessment of sisal fibre – exploring how local practices can influence environmental performance. J. Clean. Prod. 149, 818–827. doi:10.1016/j.jclepro.2017.02.073
Ciaramello, D., Castro, G. A. D P., and Petinelli, A. (1975). Estudo comparativo entre espécies de agave. Bragantia 34, 195–201. doi:10.1590/S0006-87051975000100011
Corbin, K. R., Byrt, C. S., Bauer, S., DeBolt, S., Chambers, D., Holtum, J. A. M., et al. (2015). Prospecting for energy-rich renewable raw materials: agave leaf case study. PLoS One 10, e0135382. doi:10.1371/journal.pone.0135382
Coutinho, W. M., Suassuna, N. D., Luz, C. M., Suinaga, F. A., and Silva, O. R. R. F. (2006). Bole rot of sisal caused by Aspergillus Niger in Brazil. Fitopatol. Bras. 31, 605. doi:10.1590/S0100-41582006000600014
Das, T. (1992). Micropropagation of agave sisalana. Plant Cell. Tissue Organ Cult. 31, 253–255. doi:10.1007/BF00036233
Davis, S. C., and Long, S. P. (2015). “Sisal/Agave,” in Handbook of plant breeding. Editors V. M. V. Cruz, and D. A. Dierig (New York, NY: Springer New York), 335–349. doi:10.1007/978-1-4939-1447-0_15
de Paula, M. P., Lacerda, T. M., Zambon, M. D., and Frollini, E. (2012). Adding value to the Brazilian sisal: acid hydrolysis of its pulp seeking production of sugars and materials. Cellulose 19, 975–992. doi:10.1007/s10570-012-9674-8
Develash, R. K., and Sugha, S. K. (1997). Factors affecting development of downy mildew (Peronospora destructor) of onion (Allium cepa). Indian J. Agric. Sci. 67, 71–74.
Duarte, E. A. A., Damasceno, C. L., de Oliveira, T. A. S., Barbosa, L. de O., Martins, F. M., de Silva, J. R. Q., et al. (2018). Putting the mess in order: aspergillus welwitschiae (and not A. niger) is the etiological agent of sisal bole rot disease in Brazil. Front. Microbiol. 9, 1227. doi:10.3389/fmicb.2018.01227
Emms, D. M., and Kelly, S. (2018). OrthoFinder: phylogenetic orthology inference for comparative genomics. bioRxiv, 1–14. doi:10.1101/466201
FAO (2023). FAO statistics division. Available at: http://www.fao.org/faostat/en/ (Accessed February 10, 2023).
Fick, S. E., and Hijmans, R. J. (2017). WorldClim 2: new 1-km spatial resolution climate surfaces for global land areas. Int. J. Climatol. 37, 4302–4315. doi:10.1002/joc.5086
Gerald, L. T. S. G., Bressan, E. A., da Silva, A. D. C., and Lee, L. L. (2007). Implantação de biofábrica de cana-de-açúcar: riscos e sucessos. Ornam. Hortic., 207–229. doi:10.14295/oh.v13i0.1960
Gerald, L. T. S., Lee, L. L., and Gerald, L. T. S. (2011). Biofábrica de plantas: produção industrial de plantas in vitro. 1st Edn. (São Paulo: Aniqua), 207–229.
Gherbawy, Y., Elhariry, H., Kocsubé, S., Bahobial, A., Deeb, B.E., Altalhi, A., et al. (2015). Molecular characterization of black Aspergillus species from onion and their potential for ochratoxin A and fumonisin B2 production. Foodborne Pathog. Dis. 12, 414–423. doi:10.1089/fpd.2014.1870
Hartemink, A. E., and Barrow, N. J. (2023). Soil pH - nutrient relationships: the diagram. Plant Soil 486, 209–215. doi:10.1007/s11104-022-05861-z
Huber, D. M., and Jones, J. B. (2013). The role of magnesium in plant disease. Plant Soil 368, 73–85. doi:10.1007/s11104-012-1476-0
IBGE (2018). BDiA - banco de Informações Ambientais: pedologia Mapa Solos da Estado da Bahia. Available at: https://bdiaweb.ibge.gov.br/#/consulta/ (Accessed February 20, 2023).
IBGE (2021). PAM - produção agrícola municipal. Available at: https://www.ibge.gov.br/estatisticas/economicas/agricultura-e-pecuaria/9117-producao-agricola-municipal-culturas-temporarias-e-permanentes.html (Accessed February 10, 2023).
INMET (2023). Metadados sobre a tipologia climática por município da Bahia dados do INMET, 1977 a 2006. Available at: https://mapas.inmet.gov.br/ (Accessed February 10, 2023).
Jiménez-Hidalgo, I., Virgen-Calleros, G., Mart’inez-de la Vega, O., Vandemark, G., and Olalde-Portugal, V. (2004). Identification and characterisation of bacteria causing soft-rot in agave tequilana. Eur. J. Plant Pathol. 110, 317–331. doi:10.1023/B:EJPP.0000019791.81935.6d
Kritzinger, E. M., van Vuuren, R. J., Woodward, B., Rong, I. H., Spreeth, M. H., and Slabbert, M. M. (1997). “Elimination of external and internal contaminants in rhizomes of zantedeschia aethiopica with commercial fungicides and antibiotics,” in Pathogen and microbial contamination management in micropropagation (Dordrecht: Spinger), 161–167. doi:10.1007/978-94-015-8951-2_19
Li, C., Yang, J., Li, W., Sun, J., and Peng, M. (2017). Direct root penetration and rhizome vascular colonization by Fusarium oxysporum f. sp. cubense are the key steps in the successful infection of Brazil cavendish. Plant Dis. 101, 2073–2078. doi:10.1094/PDIS-04-17-0467-RE
Lopes, A. S., and Guimarães, P. T. G. (1989). Recomendações para o uso de corretivos e fertilizantes em Minas Gerais; 4˚ aproximação. Lavras: CFSEMG.
María de Jesús, R. R., Alejandra Mancilla-Margalli, N., Lucía, M. Á., Ramón, T. T., Doralinda Guzmán-de, P., and Martin Eduardo, A. M. (2017). Epidemiology of Fusarium agave wilt in Agave tequilana Weber var. azul. Plant Prot. Sci. 53, 144–152. doi:10.17221/142/2016-PPS
Marone, M. P., Campanari, M. F. Z., Raya, F. T., Pereira, G. A. G., and Carazzolle, M. F. (2022). Fungal communities represent the majority of root-specific transcripts in the transcriptomes of Agave plants grown in semiarid regions. PeerJ 10, e13252. doi:10.7717/peerj.13252
Martinez-Hernandez, A., Mena-Espino, M. E., Herrera-Estrella, A. H., and Martinez-Hernandez, P. (2010). Construcción de bibliotecas de ADNc y análisis de expresión génica por RT-PCR en agaves. Rev. Latinoam. Quím. 38, 21–44. Available at: http://www.scielo.org.mx/scielo.php?script=sci_arttext&pid=S0370-59432010000100003&lng=es&nrm=iso.
Medina, J. C. (1954). O sisal. Campinas. Campinas: Secretaria da Agricultura do Estado de São Paulo.
Medina, J. C. (1956). Observações preliminares sôbre agave zapupe trelease. Bragantia 15, XXIII. doi:10.1590/S0006-87051956000100037
Medina, J. C. (1959). Plantas Fibrosas da Flora Mundial. Campinas: Instituto Agronômico de Campinas.
Miedes, E., Vanholme, R., Boerjan, W., and Molina, A. (2014). The role of the secondary cell wall in plant resistance to pathogens. Front. Plant Sci. 5, 358. doi:10.3389/fpls.2014.00358
Monja-Mio, K. M., Herrera-Alamillo, M. A., Sánchez-Teyer, L. F., and Robert, M. L. (2019). “Breeding strategies to improve production of agave (agave spp.),” in Advances in plant breeding strategies: industrial and food crops (Cham: Springer International Publishing), 319–362. doi:10.1007/978-3-030-23265-8_10
Morán, J. I., Alvarez, V. A., Cyras, V. P., and Vázquez, A. (2008). Extraction of cellulose and preparation of nanocellulose from sisal fibers. Cellulose 15, 149–159. doi:10.1007/s10570-007-9145-9
Moura, J. C. M. S., Bonine, C. A. V., de Oliveira Fernandes Viana, J., Dornelas, M. C., and Mazzafera, P. (2010). Abiotic and biotic stresses and changes in the lignin content and composition in plants. J. Integr. Plant Biol. 52, 360–376. doi:10.1111/j.1744-7909.2010.00892.x
Palumbo, J. D., and O’Keeffe, T. L. (2015). Detection and discrimination of four <scp>A</scp> spergillus section <scp>N</scp> igri species by PCR. Lett. Appl. Microbiol. 60, 188–195. doi:10.1111/lam.12358
Pérez-Zavala, M. de L., Hernández-Arzaba, J. C., Bideshi, D. K., and Barboza-Corona, J. E. (2020). Agave: a natural renewable resource with multiple applications. J. Sci. Food Agric. 100, 5324–5333. doi:10.1002/jsfa.10586
Porcel, R., Azcón, R., and Ruiz-Lozano, J. M. (2004). Evaluation of the role of genes encoding for Δ1-pyrroline- 5-carboxylate synthetase (P5CS) during drought stress in arbuscular mycorrhizal Glycine max and Lactuca sativa plants. Physiol. Mol. Plant Pathol. 65, 211–221. doi:10.1016/j.pmpp.2005.02.003
Quintanilha-Peixoto, G., Aguiar, E. R. G. R., Góes-Neto, A., Raya, F. T., Marone, M. P., Oliveira, A., et al. (2022a). Iniciadores, kit e método para identificação de Aspergillus welwitschiae e diagnóstico molecular da podridão vermelha de Agave sisalana e seus híbridos, e usos BR 10 2022 018126 8. Rio de Janeiro: Instituto Nacional da Propriedade Industrial.
Quintanilha-Peixoto, G., Fonseca, P. L. C., Raya, F. T., Marone, M. P., Bortolini, D. E., Mieczkowski, P., et al. (2021). The sisal virome: uncovering the viral diversity of agave varieties reveals new and organ-specific viruses. Microorganisms 9, 1704. doi:10.3390/microorganisms9081704
Quintanilha-Peixoto, G., Marone, M. P., Raya, F. T., José, J., Oliveira, A., Fonseca, P. L. C., et al. (2022b). Phylogenomics and gene selection in Aspergillus welwitschiae: possible implications in the pathogenicity in Agave sisalana. Genomics 110517, 110517. doi:10.1016/j.ygeno.2022.110517
Quintanilha-Peixoto, T., Reis, O., Bortolini, D., Oliveira, R., and Bortolini, D. (2019). Calm before the storm: a glimpse into the secondary metabolism of Aspergillus welwitschiae, the etiologic agent of the sisal bole rot. Toxins (Basel). 11, 631. doi:10.3390/toxins11110631
R Core Team (2022). R: a language and environment for statistical computing. Available at: https://www.r-project.org/.
Raij, B. V., Andrade, J. C. D., Cantarella, H., and Quaggio, J. A. (2001). Análise Química para Avaliação da Fertilidade de Solos Tropicais. Campinas: Instituto Agronômico de Campinas.
Raij, B. V., Cantarella, H., and Quaggio, J. A. (2022). “Conceitos de fertilidade de solo aplicados à calagem e adubação,” in Boletim 100: recomendações de adubação e calagem para o Estado de São Paulo. Editors H. Cantarella, J. A. Quaggio, D. Mattos, R. M. Boaretto, and B. van Raij (Campinas: Instituto Agronômico de Campinas), 53–159.
Raya, F. T., de Carvalho, L. M., José, J., da Cruz, L. P., Almeida, R. L., Delevatti, H. A., et al. (2023). Rescuing the Brazilian Agave breeding program: morphophysiological and molecular characterization of a new germplasm. Front. Chem. Eng. 5. doi:10.3389/fceng.2023.1218668
Raya, F. T., de Abreu, L. G. F., Marone, M. P., Salvador, M. de A., Bressiani, J. A., Laborde, J. I. D. R., et al. (2022). “New feedstocks for bioethanol production: energy cane and agave,” in Liquid biofuels: bioethanol. Editors C. R. Soccol, G. A. G. Pereira, C. Dussap, and L. P. S. Vandenberghe (Cham: Springer), 431–455. doi:10.1007/978-3-031-01241-9_18
Raya, F. T., Marone, M. P., Carvalho, L. M., Rabelo, S. C., de Paula, M. S., Campanari, M. F. Z., et al. (2021). Extreme physiology: biomass and transcriptional profiling of three abandoned Agave cultivars. Ind. Crops Prod. 172, 114043. doi:10.1016/j.indcrop.2021.114043
Sahu, S. K., Thangaraj, M., and Kathiresan, K. (2012). DNA extraction protocol for plants with high levels of secondary metabolites and polysaccharides without using liquid nitrogen and phenol. ISRN Mol. Biol. 2012, 1–6. doi:10.5402/2012/205049
Salgado, A. L. D. B., Ciaramello, D., and Azzini, A. (1979). Melhoramento de Agave por hibridação. Bragantia 38, 1–6. doi:10.1590/S0006-87051979000100001
Salinas, M. L., Ogura, T., and Soffchi, L. (2001). Irritant contact dermatitis caused by needle-like calcium oxalate crystals, raphides, in Agave tequilana among workers in tequila distilleries and agave plantations. Contact Dermat. 44, 94–96. doi:10.1034/j.1600-0536.2001.440208.x
Samson, R. A., Visagie, C. M., Houbraken, J., Hong, S. B., Hubka, V., Klaassen, C. H. W., et al. (2014). Phylogeny, identification and nomenclature of the genus Aspergillus. Stud. Mycol. 78, 141–173. doi:10.1016/j.simyco.2014.07.004
Santos, J. D. G., Vieira, I. J. C., Braz-Filho, R., and Branco, A. (2015). Chemicals from Agave sisalana biomass: isolation and identification. Int. J. Mol. Sci. 16, 8761–8771. doi:10.3390/ijms16048761
Secretaria do Planejamento do Estado da Bahia (2016). Política Territorial Territ. Identidade da Bahia - mapas e Informações. Available at: https://www.seplan.ba.gov.br/politica-territorial/ (Accessed April 2, 2023).
Silva, O. R. R. F., and Beltrão, N. E. D. M. (1999). O agronegócio do sisal no Brasil. Brasília, F D: Embrapa.
Soares, A. C. F., Santos, J. S., de Sousa, R. A., Barbosa, L. de O., Duarte, E. A. A., and Góes-Neto, A. (2020). “Sisal: podridão vermelha e o biocontrole,” in Tópicos em microbiologia agrícola. Editors A. C. F. Soares, N. S. Evangelista-Barreto, and P. A. S. Marbach (Cruz das Almas: EDUFRB), 276.
Souza, S. C. D., Cavalcanti, J. J. V., Ramos, J. P. C. R., Alves, I., Santos, R. C. D., and Lima, L. M. D. (2018). Genetic divergence in Agave accessions through ISSR markers and phenotypic traits. Afr. J. Agric. Res. 13, 526–533. doi:10.5897/AJAR2017.12913
Sugimoto, T., Watanabe, K., Yoshida, S., Aino, M., Furiki, M., Shiono, M., et al. (2010). Field application of calcium to reduce Phytophthora stem rot of soybean, and calcium distribution in plants. Plant Dis. 94, 812–819. doi:10.1094/PDIS-94-7-0812
Suinaga, F. A., Silva, O. R. R. F., Coutinho, W. M., Cartaxo, W. V., and Costa, L. B. (2007). Avaliação agronômica de Oito genótipos de Sisal (Agave spp.). Campina Grande: Embrapa Algodão.
Susca, A., Proctor, R. H., Morelli, M., Haidukowski, M., Gallo, A., Logrieco, A. F., et al. (2016). Variation in fumonisin and ochratoxin production associated with differences in biosynthetic gene content in Aspergillus niger and A. welwitschiae isolates from multiple crop and geographic origins. Front. Microbiol. 7, 1412. doi:10.3389/fmicb.2016.01412
Tam, E. W. T., Chen, J. H. K., Lau, E. C. L., Ngan, A. H. Y., Fung, K. S. C., Lee, K. C., et al. (2014). Misidentification of Aspergillus nomius and Aspergillus tamarii as Aspergillus flavus: characterization by internal transcribed spacer, β-tubulin, and calmodulin gene sequencing, metabolic fingerprinting, and matrix-assisted laser desorption ionization–time of flight mass spectrometry. J. Clin. Microbiol. 52, 1153–1160. doi:10.1128/JCM.03258-13
Tekaia, F., and Latgé, J. P. (2005). Aspergillus fumigatus: saprophyte or pathogen? Curr. Opin. Microbiol. 8, 385–392. doi:10.1016/j.mib.2005.06.017
Uloth, M. B., Clode, P. L., You, M. P., and Barbetti, M. J. (2015). Calcium oxalate crystals: an integral component of the Sclerotinia sclerotiorum/Brassica carinata pathosystem. PLoS One 10, e0122362. doi:10.1371/journal.pone.0122362
Vale, F. X. R., de Jesus Junior, W. C., Liberato, J. R., and de Souza, C. A. (2004). “Quantificação de doenças e do crescimento do hospedeiro,” in Epidemiologia aplicada ao manejo de doenças de plantas. Editors F. X. R. Vale, W. C. de Jesus Junior, and L. Zambolin (Belo Horizonte: Perfil), 91–121.
Valenzuela-Zapata, A. G., and Nabhan, G. P. (2004). ¡Tequila!: a natural and cultural history. Tucson: University of Arizona Press.
Varga, J., Frisvad, J. C., Kocsubé, S., Brankovics, B., Tóth, B., Szigeti, G., et al. (2011). New and revisited species in Aspergillus section Nigri. Stud. Mycol. 69, 1–17. doi:10.3114/sim.2011.69.01
Vega-Ramos, K. L., Uvalle-Bueno, J. X., and Gómez-Leyva, J. F. (2013). Molecular variability among isolates of Fusarium oxysporum associated with root rot disease of agave tequilana. Biochem. Genet. 51, 243–255. doi:10.1007/s10528-012-9559-4
Vijayanandan, A. S., and Balakrishnan, R. M. (2018). Biosynthesis of cobalt oxide nanoparticles using endophytic fungus Aspergillus nidulans. J. Environ. Manage. 218, 442–450. doi:10.1016/j.jenvman.2018.04.032
Walters, D. R., and Bingham, I. J. (2007). Influence of nutrition on disease development caused by fungal pathogens: implications for plant disease control. Ann. Appl. Biol. 151, 307–324. doi:10.1111/j.1744-7348.2007.00176.x
Wang, G., Wu, W., Tan, S., Liang, Y., He, C., Chen, H., et al. (2022). Development of a specific nested PCR assay for the detection of 16SrI group phytoplasmas associated with sisal purple leafroll disease in sisal plants and mealybugs. Plants 11, 2817. doi:10.3390/plants11212817
Wang, M., Zheng, Q., Shen, Q., and Guo, S. (2013). The critical role of potassium in plant stress response. Int. J. Mol. Sci. 14, 7370–7390. doi:10.3390/ijms14047370
Williams, J., and Smith, S. G. (2001). Correcting potassium deficiency can reduce rice stem diseases. Better crop. 85, 7–9.
Xu, G., Yang, S., Meng, L., and Wang, B. G. (2018). The plant hormone abscisic acid regulates the growth and metabolism of endophytic fungus Aspergillus nidulans. Sci. Rep. 8, 6504. doi:10.1038/s41598-018-24770-9
Yadav, A., Ansari, W. A., Singh, A. L., and Singh, M. (2020). Transcriptional response of otsA, P5CR, glgX, nodC, and molecular chaperone genes under the PEG-induced drought stress in Mesorhizobium ciceri Ca181. Biocatal. Agric. Biotechnol. 23, 101459. doi:10.1016/j.bcab.2019.101459
Yao, Z., Zou, C., Zhou, H., Wang, J., Lu, L., Li, Y., et al. (2013). Δ1-Pyrroline-5-Carboxylate/Glutamate biogenesis is required for fungal virulence and sporulation. PLoS One 8, e73483. doi:10.1371/journal.pone.0073483
Keywords: Aspergillus, Agave, PCR diagnosis, plant-pathogen interaction, natural fibers
Citation: Raya FT, Quintanilha-Peixoto G, de Oliveira AB, Marone MP, dos Reis WJV, Marques JGO, Almeida RL, Falcão Filho CAT, Costa ODV, Ribeiro RV, Soares ACF, Goés-Neto A and Pereira GAG (2023) Molecular epidemiology of sisal bole rot disease suggests a potential phytosanitary crisis in Brazilian production areas. Front. Chem. Eng. 5:1174689. doi: 10.3389/fceng.2023.1174689
Received: 26 February 2023; Accepted: 10 October 2023;
Published: 01 November 2023.
Edited by:
Sarah C. Davis, Ohio University, United StatesReviewed by:
Awanwee Petchkongkaew, Thammasat University, ThailandAmanda P. De Souza, University of Illinois at Urbana-Champaign, United States
Copyright © 2023 Raya, Quintanilha-Peixoto, de Oliveira, Marone, dos Reis, Marques, Almeida, Falcão Filho, Costa, Ribeiro, Soares, Goés-Neto and Pereira. This is an open-access article distributed under the terms of the Creative Commons Attribution License (CC BY). The use, distribution or reproduction in other forums is permitted, provided the original author(s) and the copyright owner(s) are credited and that the original publication in this journal is cited, in accordance with accepted academic practice. No use, distribution or reproduction is permitted which does not comply with these terms.
*Correspondence: Gonçalo Amarante Guimarães Pereira, Z29uY2Fsb0B1bmljYW1wLmJy