- 1UGC-Human Resource Development Centre, Pondicherry University, Puducherry, India
- 2Department of Environment Studies, Panjab University, Chandigarh, India
- 3Soil Ecosystem and Restoration Ecology Lab, Department of Botany, Panjab University, Chandigarh, India
- 4Environmental Sciences Department, Faculty of Science, Alexandria University, Alexandria, Egypt
- 5Green Technology Group, Faculty of Science, Alexandria University, Alexandria, Egypt
Global demand for freshwater is rapidly escalating. It is highly essential to keep pace with the necessities of the increasing population. The effluents of wastewater are gradually identified as a reservoir of resources for energy generation and economic boom. Henceforth, most wastewater and sludge have great potential for reuse and recycling. The re-utilization and valorization of wastewater and sludge contribute to accomplishing sustainable development goals, combating water scarcity, and alleviating adverse environmental impacts of wastewater on the environmental components. The present article highlights the most novel approaches for wastewater treatment for the waste valorization of different industrial origins and the generation of value-added products and recovery of biopolymers, vitamins, enzymes, dyes, pigments, and phenolic compounds. We highlighted the life cycle assessment and techno-economic analysis. In addition, we have addressed a critical overview of the barriers to the large-scale application of resource recovery strategies and economic, environmental, and social concerns associated with using waste-derived products.
1 Introduction
An essential requirement for human civilisation is having access to clean and fresh water. The exploitation of non-renewable natural resources has led to their depletion and raised environmental concerns due to the world’s rapid population growth and economic development. Water is one of the natural resources that has been impacted by multiple issues, including poor quality, scarcity, and lack of access to clean water (Scanlon et al., 2023). The shrinking of freshwater resources has emerged as one of the significant global issues in the twenty-first century faced by the human race. The ability of the planet’s natural resources to sustainably meet the rising demand has also grown to be a challenging problem due to population growth, urbanisation, and industrialisation.
In fact, in the 2030 Agenda towards sustainable development goals of the United Nations, the sixth goal was established as a goal for clean water and sanitation to guarantee sanitation and water accessibility and sustainable management worldwide (Mahmoud et al., 2021a). In the past few years, urbanisation has led to the generation of a considerable amount of solid waste and wastewater effluents disposed of in nearby water bodies without complete treatment (Markandeya and Shukla, 2022). The release of such wastes and effluents into the environment has many repercussions like eutrophication, groundwater leaching, spreading of pathogens, increase in waterborne diseases, and deterioration of water body aesthetics (Bashir et al., 2020; Jadon et al., 2022). The current wastewater treatment plants are now being examined as a potential reservoir of resources for nutrient recovery and the generation of value-added products (Singh et al., 2022). Previously, these facilities were designed to treat wastewater to meet the essential physical, biological and chemical standards before disposing it into the environment (Bora et al., 2020). Utilising sewage sludge and wastewater as a source of nutrients and value-added goods is essential for the world’s transformation from a linear to a circular economy when resource availability is constrained to lessen the mounting stress on our water resources (Puyol et al., 2017). High-end wastewater treatment systems and advanced waste management technologies offer multiple ways for nutrient recovery and transformation to value-added products. Establishing a circular resource flow can help the water sector alleviate water scarcity and generate additional revenue (Singh et al., 2022) (Figure 1).
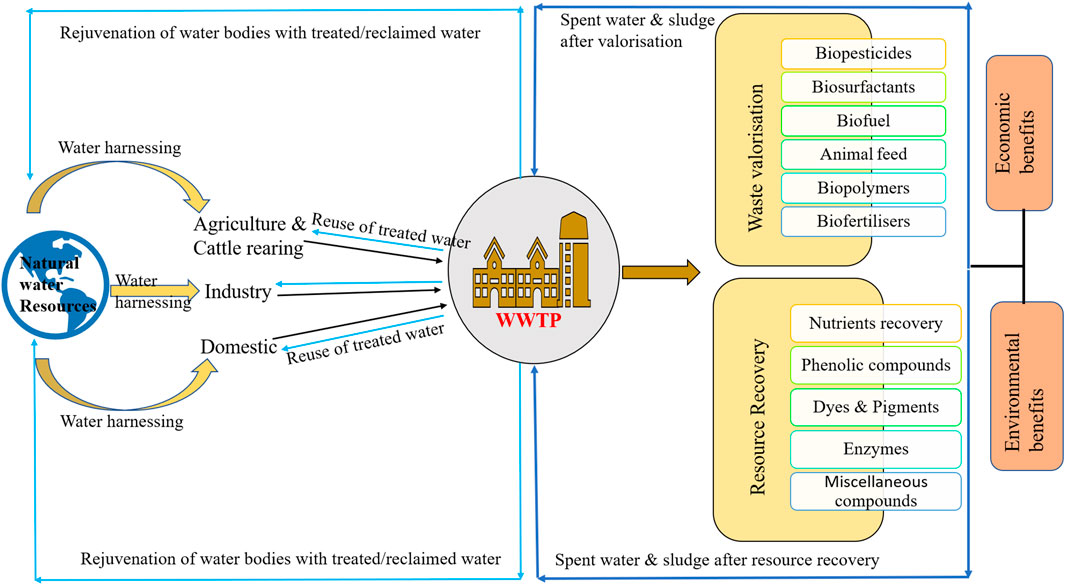
FIGURE 1. The circular economy concept of waste valorisation and resource recovery from wastewater and sludge. Adapted from Singh et al., 2022, with permission from Elsevier.
Wastewater and sludge, which are now recognised as valuable sources of nutrients like phosphorus and ammonium, contain massive volumes of both organic and inorganic compounds (Meena et al., 2022). The world’s population is increasing, which means that more food needs to be produced. To do this, farmers use fertilisers which contain phosphorous. To make these fertilisers, two substances called ammonium and phosphate are needed. Studies have shown that the demand for these two substances is increasing as more fertilisers are needed. (Tilman et al., 2002; Gong et al., 2022).
Partially or untreated wastewater containing excess phosphorous and ammonium released in surface waters may lead to eutrophication and imbalance in the aquatic ecosystem; therefore, removing these nutrients is essential to maintain healthy aquatic life (Diaz et al., 2022; el-Maghrabi et al., 2022). Moreover, the depletion of natural phosphorous resources from agroecosystems has become a global issue (Sattari et al., 2012; Alewell et al., 2020). Therefore, wastewater and sludge valorisation have multiple benefits of nutrient recovery, generation of value-added products, sustainable wastewater treatment, alleviating water scarcity, additional revenue generation, and reduction in environmental contamination (Singh et al., 2022).
Conventional wastewater treatment plants and their integrated system are primarily designed to treat wastewater to reduce organic and suspended solid load and meet the basic discharge requirements (Mahmoud and Kathi, 2022). Traditional physical techniques mainly use various screens, settling tanks and adsorbents with enhanced adsorption efficiency and offer a modest process for wastewater treatment (Kaviya, 2022). Emerging high-end technologies and advanced membrane systems empowered by hydraulic and osmotic pressure, thermal and electrical systems are promising technologies for enhanced treatment and valorisation, leading to resource recovery and generation of value-added products (Xu et al., 2017; Ray et al., 2020). For the oxidative elimination of contaminants, chemical methods utilising radical chemistry and electrochemical technologies based on the catalytic electrode have also received considerable attention recently (Logan and Regan, 2006; Tarpeh et al., 2018). The latter is predominantly operative for highly saline wastewaters, especially for brine and tannery wastewaters. Biological techniques such as bioelectrochemical systems, enzymatic catalysis, and microalgae cultivation have been employed to remediate wastewater of a wide range along with the generation of various value-added compounds (Singh et al., 2022; Yadav et al., 2022). In contrast, biological approaches are more technologically sound than Physico-chemical approaches available for wastewater treatment and valorisation due to their sturdiness, goal-specific, eco-friendly and resilient characteristics. However, the capital and operational costs used for wastewater treatment and valorisation are important to consider while deciding which method to use, as they can have a big impact on the overall cost of the project.
Therefore, the present article highlights the most novel approaches for wastewater valorisation and the generation of value-added products (such as biofuels, biofertilisers, and biopesticides) and recovery of biopolymers, vitamins, enzymes, dyes, pigments, and phenolic compounds. In addition, we have addressed the life cycle assessment and a critical overview of the barriers to the large-scale application of resource recovery strategies and economic, environmental, and social concerns associated with using waste-derived products.
2 Novel approaches for wastewater treatment
2.1 Membrane technology
Membrane separation is one of the cutting-edge technologies for wastewater treatment. Here, a permeable membrane is used to let wastewater pass through it. Any solute larger than the membrane’s pore size will be restricted, while the remaining solution passes through the membrane. The nutrients/solutes trapped in the filter cake are continuously removed throughout this filtration process to ensure the consistence of filtration process. Based on the size of the pores present in the membranes, we may categorise the membrane separation processes (Mahmoud et al., 2022a) into four categories, (i) RO/FO (Reverse osmosis/Forward osmosis), (ii) NF (Nanofiltration), (iii) UF (Ultrafiltration), and (iv) MF (Microfiltration) (Figure 2).
Pore size, structure, and operational pressure are the fundamental properties of pressure-driven membranes. MF uses a precise sieving mechanism along with cutting-edge, environmentally friendly technology. It has extensive industrial applications in food and dairy industry, textile industry, biotechnology, and pharmaceutical industry (Foorginezhad and Zerafat, 2017; Khan and Boddu, 2021). The feasibility of MF was reported by Marchesi et al. (2019) for the recycling and reuse of chicken pre-chiller wastewater owing to its high rejection efficiency (up to 92.5% of COD, 89.1% of TOC, and 100% of microbes). However, UF membranes work on the principle of size exclusion, which have pores with a size range of 0.005–0.1 μm. However, additional elements, including molecular structure, charge, and hydrodynamic circumstances, can also significantly impact the rejection efficiency (al Aani et al., 2020). The use of UF for separating proteins from food industry wastewater has been reported frequently. NF membranes’ pore sizes range from 0.1 to 10 mm. It can display intense permeate flux and significant macromolecule rejection. While NF has been used to recover comparatively smaller molecules such as lactose and phenols (Lee and Stuckey, 2022). According to Pronk et al. (2006), the NF membrane have potential to discard micropollutants such as phosphate, diclofenac, propanol, carbamazepine, and ibuprofen while allowing nitrogen to pass through for its recovery.
NF and RO are high-pressure membrane processes, therefore, frequently employed to recover ammonium from greywater as they significantly reject the salts and ions (Adam et al., 2018; Ray et al., 2020). In the RO process, feed water is propelled through a semipermeable membrane from a dilute to a concentrated solution by the difference in osmotic pressure (Ahuchaogu et al., 2018). Ray et al. (2020), reported successful recovery (64%–90%) of unionised ammonia from hydrolysed urine by using RO and NF procedures. However, the high expense of the process of nutrient recovery from wastewater is a drawback for NF and RO (Xu et al., 2017). Other factors can further increase operational costs, including membrane fouling, a decrease in membrane shelf life, and reduced rejection efficiency (Shi et al., 2014). As a result, effective treatment and valorisation methods are needed for treatment and resource recovery, such as FO technology, which is highly operative in the case of specific target molecules.
In order to recover nutrients from the wastewater and produce clean water, the nitrogen and phosphorous in primary treated municipal wastewater can be concentrated by the FO membrane method (Jafarinejad, 2021). Due to the size-sieving action of FO membranes, high rejection efficiency for the majority of nutrients, including the high-in-demand phosphate, has been well documented in the literature (Linares et al., 2013; Liu et al., 2019). If the technological and operational shortcomings can be successfully resolved, membrane technology is anticipated to attract more interest and profitability in this market segment in the upcoming years (Table 1).
2.2 Enzymatic catalysis
Enzymes, which are fundamental to all biological activities, are highly effective biocatalysts that speed up chemical reactions under physiological conditions by breaking down complex compounds into simpler ones (Bell et al., 2021). Many wastewaters contain heavy grease content, dyes, lignocellulosic mass, agrochemicals, blood, tissues, pathogens, and other emerging contaminants from food processing industries, restaurants, dairy, oil refineries, meat processing, tannery, slaughterhouse, cosmetics, and pharmaceuticals industries (Mahmoud et al., 2016; Mahmoud et al., 2018; Mahmoud et al., 2021b; Khan et al., 2022; Mahmoud, 2022). If left untreated, such wastewater can cause severe environmental pollution, potentially contributing to the spread of these pollutants into the various environmental matrices and eventually threatening human health (Singh et al., 2022).
Treatment and valorisation methods based on enzymatic catalysis offer numerous advantages over conventional methods, such as low energy input, low toxicity, the ability to function under mild conditions, abridged sludge, and byproduct generation (Rout et al., 2022) (Table 2). Enzymes can be used in both free form and immobilised forms however, the enzymes’ storage stability and operation ability upsurge upon immobilisation. The development in enzyme reutilisation and recoverability, that elevates its productivity and lowers the entire process’s cost, is the most crucial benefit of the immobilised enzyme (Chen et al., 2019; Lassouane et al., 2019). Predominantly, enzymes viz. laccases, tyrosinases, lipases, cellulases, lignin, and phenol peroxidases hold a promising role in mitigating any kind of contamination from an extensive array of wastewater types such as greasy wastewater, sugarcane industry wastewaters, dairy wastewaters, pharmaceutical industry, tannery textile, paper and pulp, mining, etc. in a precise, manageable, and controlled manner (Brugnari et al., 2018; Chen et al., 2019).
Lipase is a class of hydrolase enzymes with multiple benefits and high catalytic rates (Nimkande and Bafana, 2022). lipases have both high catalytic efficiency and substrate specificity, enabling it to achieve excellent wastewater treatment efficiencies (Ariaeenejad et al., 2023). For instance, lipase consortium from Pseudomonas aeruginosa was utilised for the remediation of restaurant wastewater by Sutar et al. (2023). Another class of enzyme, laccase, was found to be effective in degradation of phenolic compounds such as the very unfamous Bisphenol A, an endocrine disruptor, commonly used in plastic production, and barely degraded in conventional wastewater treatment systems. The laccase from Pleurotus ostreatus immobilised onto mono aminoethyl-N-aminoethyl agarose achieved 90% removal efficiency of BPA in 15 catalytic cycles (Brugnari et al., 2018). Additionally, Lassouane et al. (2019) employed immobilised laccase from Trametes pubescens to remove BPA. at pH 5 and temperature at 30°C, this biocatalytic system aided the degradation of BPA with 100% contaminant removal efficiency within 2 hours. In a different study, Chen et al. (2019) degraded nine pesticides, owing to immobilised laccase enzyme, using wheat straw and peanut shells as supports for the reaction system, and syringaldehyde to enhance the catalytic characteristics of the laccase. 54.5% decomposition rate was achieved using laccase immobilised on peanut shells. However, the reaction efficiency rose to 65.9% when wheat straw was utilised.
Although enzyme technology in wastewater treatment has a promising role, it has its limitations that need to be addressed. An efficient immobilisation system can circumvent these challenges by enhancing enzyme stability, thereby increasing the shelf life of the enzymes in the reaction system.
2.3 Microalgae cultivation
Urban, agricultural, and industrial activities produce massive amounts of nutrients rich wastewater, which would inevitably result in eutrophication in the aquatic environment as well as the threat of releasing harmful emerging contaminants that can enter the food chain and pose a risk to human health (Iqbal et al., 2022; Minhas et al., 2022).
Central and local governments should follow strict laws and regulations to limit the pollutants released into the environment. They should also work to reclaim and reuse resources, such as water, in order to improve the current state of the environment and promote the use of a circular economy that focuses on reducing waste and reusing resources (Bolger and Doyon, 2019; Singh et al., 2022). However, the majority of currently used methods for treating sewage and wastewater, such as the aerobic activated sludge-based treatment process, nitrification-denitrification, chemical phosphorus removal, and coagulating sedimentation, face difficulties due to their high energy requirements, unstable treatment effects, lengthy processes, carbon emissions, excess sludge discharge, and waste of recyclable resources (Sathya et al., 2022). These challenges are also clear impediments to integrating wastewater treatment with low-carbon emissions, low-energy consumption, and resource recycling (Gu et al., 2017).
One of the most forefront technologies for wastewater treatment is the microalgae-based treatment process for advanced treatment, valorisation and nutrient recovery (Figure 3). Microalgae includes photosynthetic microorganisms that can thrive easily in industrial and municipal wastewaters, remediate it and produce biomass rich in nutrients, bioactive compounds, biomolecules, and other value-added products (Daneshvar et al., 2018; Mishra et al., 2022). Moreover, these form the raw materials for generating value-added products, energy, and economy along with the bioremediation process simultaneously (Michelon et al., 2021). Microalgae’s strong nutrient removal efficiency in the advanced treatment of urban, agricultural, and industrial wastewater have made it possible to use it as a supplement for tertiary wastewater treatment (Rout et al., 2022). Cultivation of microalgae from wastewater utilises the nutrients present in the wastewater to support its growth and allows the reduction of production costs and greenhouse gas emissions (Mishra et al., 2022; Dias et al., 2023). To date, a wide range of microalgae species (Scenedesmus, Botryococcus, Chlorella, Dunaliella, Parachlorella, etc.) have been reported to be used in valorisation and treatment of wide range of wastewaters (Amini et al., 2020) as mentioned in Table 3.
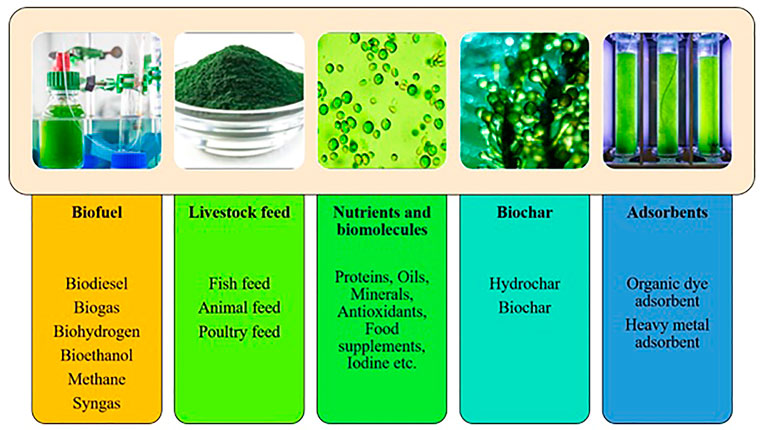
FIGURE 3. Wastewater valorisation and production of value-added products using microalgae cultivation.
Microalgae can tolerate a wide range of water salinity, pH, and temperature. They can also grow in high concentrations of oxides of sulfur, carbon and nitrogen, making them suitable for wastewater cultivation (Ebhodaghe et al., 2022). Scenedesmus quadricauda and Tetraselmis suecica, two freshwater and marine water strains, have recently been studied by Daneshvar et al. (2018) for the treatment of dairy wastewater. While T. suecica strain removed 86.21% of TN, 44.92% of p, and 40.16% of total organic carbon, this strain had an efficiency of removal of 86.21% TN, 64.47% phosphate, and 42.18% TOC. Additionally, 295.34 and 56.25 mg g-1 of tetracycline could be removed from water by each species, respectively (Daneshvar et al., 2018).
3 Resource recovery from wastewater and sludge
Wastewater may be beheld as a reservoir of resources as it contains a wide array of nutrients and components that can be valorised into value-added products such as fertilisers, biofuels, biopesticides and many more (Singh et al., 2022). Wastewater sources, including wastewater and sludge, are rich in nutrients and are now recognised as important sources of nutrients. Nutrient recovery such as phosphorus and nitrogen, from wastewater can make wastewater treatment more sustainable, also reduce the costs associated with removing these nutrients from the wastewater, and provide additional fertilisers that can be used to help grow food (Zin and Kim, 2021; Hui et al., 2022). For the treatment of wastewater and concurrent resource recovery and production of value-added products (nutrients, organic acids, bioplastics, bio-pesticides, bio-surfactants, bio-flocculants etc.) as well as bioenergy, biofuels, and bioelectricity, more advanced and novel technologies are currently being identified and fundamentally considered. Numerous technologies, including conventional ones like chemical precipitation and adsorption and more cutting-edge ones like bio electrochemical systems and osmotic membrane bioreactors, have been successfully examined for their efficacy in nutrient recovery (Kurup et al., 2019; Nazir et al., 2019; Canadas et al., 2022).
3.1 Recovery of nutrients (phosphate, nitrates, ammonia, proteins, lipids, vitamins)
A crucial step toward sustainable development is the recovery of nutrients from wastewaters and sludge. Urban and industrial wastewater are potential raw materials to recover significant biologically active components. Sewage sludge from wastewater treatment facilities provide rich source of biomolecules that include, nutrients such as NPK, antioxidants, lipids, proteins, enzymes, carbohydrates, vitamins, nitrogen, phosphates, minerals, ammonium compounds, and many more (Zhu et al., 2017; Chen et al., 2020; Sichler et al., 2022).
Due to the commercialization of agriculture and the need to feed an expanding population, the demand for fertiliser has increased over the past few decades. By 2022, it has already been predicted that most regions of the world will experience a shortage of at least one NPK fertiliser, Moreover, In 2014, the European Commission added phosphate rock to the list of critical raw materials for the first time (EC, 2014). Scientists are looking at alternatives to provide these crucial agricultural nutrients due to the restricted availability and the high cost of production. Compared to nitrogen and phosphorus, potassium has not yet had a significant economic impact on agriculture because, at the pace it is being consumed, there are more than 330 years of reserves. In contrast, there is no substitute for phosphorus in agricultural output and its supply is expected to run out in 100 years, making it a limited resource and a critical commodity (Cordell et al., 2009).
Municipal wastewater and sludge, offer a promising secondary phosphorus source, comprising around 40% of the phosphorus in human excreta (Cordell and White, 2014; Klinglmair et al., 2015). Several techniques, such as biological release, pH adjustment, ozonation, mechanical treatment, and heat treatment, have been used to recover phosphorus from municipal wastewater and sewage sludge (Xue and Huang, 2007; Quist-Jensen et al., 2018; Wang et al., 2021). Notably, the total amount of phosphorus found in human waste (urine and excreta) may meet around 22% of the world’s phosphorus needs (Cordell and White, 2014). However, due to worries about possible infections, persistent organic pollutants, heavy metals, and odor, direct wastewater and sludge application to agriculture is regarded as unsafe in many places (Cieslik et al., 2015). Therefore, nutrient recovery is considered the best way to confiscate phosphorous, nitrogen, ammonium, and other nutrients to support sustainability by putting less stress on natural gas and other renewable energy sources spent on producing these nutrients (Theregowda et al., 2019; Ersahin et al., 2023).
Agents like magnesium, calcium, and other alkaline metals are introduced in the precipitation process to change pH and create the precipitate (Owodunni et al., 2023). Nutrient recovery from sludge centrates is largely accomplished commercially through chemical precipitation and crystallization (Melia et al., 2017). Sludge centrate is low in volume but high in phosphate and ammonium concentration when compared to wastewater discharge. The dry mass of wastewater sludge can contain up to 15% phosphorus, which is typically coupled to metal ions like calcium, iron, and aluminium or contained in biomass (Yu et al., 2021). The possibility of recovering phosphorus from wastewater sludge will be greatly increased by leaching phosphorus into the aqueous phase. Due to the dissolution of inorganic phosphorus, acidification is one of the most successful methods (Quist-Jensen et al., 2020).
In a study, phosphate and ammonium nitrate were recovered from sewage sludge ash and food industry wastewater using Mg-Biochar derived from ground coffee bean and palm tree trunk waste. Palm tree trunk Mg-biochar could recover 92.2% of phosphate and 54.8% of ammonium nitrate, while ground coffee bean biochar recovered 79.5% of phosphate and 38.6% ammonium (Zin and Kim, 2021). Besides, wastewaters also are a rich source of nutrients such as proteins, lipids, vitamins, etc. Several industries generate copious volumes of protein-laden wastewater. Starch processing water from the potato manufacturing industry is known to have a high content of protein, where foam separation and membrane technology by ultrafiltration are used for its recovery (Dabestani et al., 2017).
Similarly, multiple industrial, domestic wastewater, and sewage sludge are rich source of proteins. Bulk proteins have countless industrial applications, in manufacturing of food and beverages, personal care products, medicines, cosmetics and animal feed. The most common use of bulk proteins derived from waste is in animal feed (Ritala et al., 2017). Hwang et al. (2008) recovered protein from sludge to be used as animal feed by the method of sludge disintegration. Precipitation and drying methods were used to separate the soluble proteins from the sludge. This concept is feasible because sludge typically contains beneficial organic substances such as nucleic acids, enzymes, proteins, and polysaccharides (Jung et al., 2002). Protein has been estimated to account for approximately half of the dry weight of bacterial cells (Shier and Purwono, 1994). Protein is one of the most vital elements in animal feed, providing energy and nitrogen (Adebayo et al., 2004). Single-cell protein (SCP) is a type of protein that is made from microbial biomass and is commonly used as an additive in animal feed. It is less expensive to produce SCP using microbial communities, which are groups of different types of microorganisms, than it is to produce it using a single type of organism (Vethathirri et al., 2021). Furthermore, protein recovery also facilitates wastewater discharge by significantly reducing the Biological Oxygen Demand (BOD) and COD (Buchanan et al., 2023). Like proteins, Lipids have a variety of industrial applications in a variety of products, including manufacturing and production of biodiesel, agrochemicals, animal feed, personal care items, and cosmetics (De Luca et al., 2021) to name a few.
Physical methods of extracting and recovering proteins and lipids from wastewater and sewage sludge involve using adsorption, microwaves, ultrasonics, heat, centrifuges, and membrane filtration. Chemical processes involve using thermal alkali, thermal acidic, pH regulation, and ethylenediamine tetraacetic acid (EDTA). Hui et al. (2022), conducted a study to isolate proteins from waste-activated sludge (WAS). Maximum protein concentration (549.86 mg/L) was recovered with high extraction rate (60.72%) under alkaline conditions at 70°C. The relative molecular weight of the sludge proteins was between 6.5 kDa and 16 kDa. Kurup et al. (2019) demonstrated the use of low-cost Na-lignosulphonate to recover lipids and proteins from dairy industry effluents. He reported that change in Na-lignosulphonate concentration (0.002%–0.032%, w/v), medium’s pH (1.0–3.5) and the temperature (22°C and 40°C) enhanced the rate of precipitation in the wastewater samples. The procedure was highly effective (96% of lipids and proteins recovery and 73% BOD removal at 22°C) over methanol and chloroform-based solvent extraction of lipids which is neither cost-effective nor environmentally beneficial. In another study on the extraction of lipids from synthetic primary sludge by Villalobos-Delgados et al. (2021), the lipid component was successfully recovered using ethyl butyrate (13%–23% of the total solids).
3.2 Recovery of phenolic compounds
Phenol is a significant environmental contaminant that may result in water pollution. Natural occurrences or anthropogenic activities are the two critical sources. In nature phenol is produced when dead plants and animals decompose in water. Humans utilise phenol in their daily activities in chemical production or petrochemical industries. Alkylphenols, cresols, and resin are also synthesized using it as a precursor (Careghini et al., 2015). The building business in the wood sector mainly provided phenolic resin and appliances to suit varied needs (Takeichi and Furukawa, 2012). Additionally, phenol is a key component of explosives, textile industries, and dyes (Stamatelatou et al., 2011; Patel and Vashi, 2015). Epoxy resins and polycarbonate plastics are commonly made using phenolic replacements like bisphenol A (Sirasanagandla et al., 2022).
Some industries including the food industry, paper and pulp manufacturing, and wood distillation produce phenol, specifically chloro-phenol, as part of their processes (Olaniran and Igbinosa, 2011). These industrial activities directly or indirectly release phenol-containing wastewater into water bodies, resulting in water contamination. Phenol contamination of the water sources would result from the runoff of the agrochemicals such as pesticides and herbicides (Badanthadka and Mehendale, 2014). Domestic and municipal sources of phenol discharge into water sources were also a factor in addition to the industrial sector (Villegas et al., 2016; Mu’azu et al., 2017).
Olive Mill Wastewaters (OMWW) are a great source of phenolic chemicals. These phenolic substances pose a serious threat to aquatic life, microbes, and plants since they are both extremely phytotoxic and resistant (Chedeville et al., 2009; Kathi, 2022). Wastewater toxicity may suppress the microbial population, therefore, biological treatment facilities are insufficient. Hence, it is essential that phenolic wastewater be properly treated before its discharge (Sun et al., 2015). Despite the fact that these techniques have already been created and proposed, they have not produced satisfying outcomes (Ochando-Pulido et al., 2013). An integrated approach is necessary for the practical management of OMWW.
The combination of membrane processes, such as MF, UF, NF, and RO, is recommended as a good alternative in this regard by a number of studies (Zagklis et al., 2015; Bazzarelli et al., 2016; Nazir et al., 2019) (Table 4). Solvent extraction is popularly used on OMWW to recover phenolic compounds (Goula and Gerasopoulos, 2017). In order to get all of the phenolic compounds out of the OMWW, different solvents are needed that make the process more complicated, take longer time, and cost more currency (Tsakona et al., 2012; Galanakis. 2013). Adsorption is considered the most appropriate method for recovering OMWW phenolic compounds because it is cost-efficient and commonly used (Mahmoud et al., 2020; Mahmoud et al., 2022b).
3.3 Recovery of dyes and pigments
In the production of pharmaceuticals, textiles, food, paper and pulp, and cosmetics, synthetic dyes are utilised. Water is used extensively throughout the fixing, dying, and washing stages of the textile industry’s dyeing process. The dyes are organic soluble substances that can be categorised as direct, reactive, acidic, or basic. Due to their great solubility in water, dyes are more difficult to remove using standard methods. The main carcinogenic and hazardous pollutants are industrial dyes and their byproducts.
A number of studies demonstrate the harmful effects of azo dyes on plant growth and germination. The production and growth of crops may be impacted if such contaminated effluent is used for irrigation (Rahman et al., 2018). For the treatment of wastewater from the dye industry, advanced oxidation processes, membrane filtration, microbial technologies, bio-electrochemical degradation, and photocatalytic degradation have been documented (Gao et al., 2023; Iqbal et al., 2023; Verma et al., 2023). In addition to assisting in the treatment of wastewater, innovative techniques including microalgae cultivation, biobased adsorbents, and membrane technologies also aid in the recovery of dyes and pigments that may be reused and recycled sustainably (Eskikaya et al., 2023; Xiong et al., 2023). Wastewater phytoremediation represents a promising circular economy-based approach for wastewater reclamation, resource recovery, and generation of value-added products. With unique health benefits, natural pigments are now in high demand in the market, as natural colouring agents in food industry sector (Christaki et al., 2015; Villaro et al., 2021). Phycobiliproteins are photosynthetic pigments found in the microalgae, and are widely used in the food, cosmetic, and pharmaceutical industries (Sun et al., 2023). While microalgae are seen to be a promising contender for the production of natural pigments and the demand for natural dyes is rising, the high costs associated with large-scale systems necessitate the use of enormous amounts of water and nutrients. It also makes it more difficult to produce and market these bioproducts. Natural pigments’ production from microalgal culture grown over wastewater as growth medium has been recently foreseen as a potential solution to both environmental pollution and high cost production of pigments (Blanco-Vieites et al., 2023). This approach will remediate the wastewater and produce natural dyes and pigments (Xiong et al., 2023). Further, the algal biomass may be utilized to produce biogas, biofertilisers, and other byproducts. Arashiro et al. (2020) cultivated microalgae consortium on industrial wastewater for the recovery of phycobiliproteins aiming to bioremediation of wastewater and sustainable production of value-added compounds. The high recovery rates were observed for Phycocyanin (103 mg/g dry wt), allophycocyanin (57 mg/g dry wt), and phycoerythrin (30 mg/g dry wt), from algal biomass respectively. Furthermore, microalgae culture successfully removed the COD, inorganic nitrogen, and phosphate up to 98%, 94%, and 100%. Stepnowski et al. (2004) used fish scale waste as a natural adsorbent for the recovery of astaxanthin, a carotenoid pigment from seafood industry wastewater. Astaxanthin is widely utilised as a coloration agent for farmed salmonids, including the natural or synthetic pigment in the fish diet (Hatlen et al., 1995). The pigment is also considered in medical and biomedical studies and applications due to its biological function as a vitamin A precursor and its high antioxidative effects. The results show that astaxanthin bound onto the scales-based adsorbent was 88%–95% of the total sorbed pigment in its esterified form. The maximum loading capacity of astaxanthin onto the scales was 362 mg/kg dry wt.
3.4 Recovery of enzymes
Most commercial enzymes are derived from bacterial biomass. Additionally, they are extracted of other fungi, including yeast made in a commercial fermentation method. Specific culture mediums are used in the synthesis of industrial enzymes. These culture media are capable of offering all the nutrients required for microbial growth, consolidation, and purification. By disrupting the biomass, enzymes are extracted, then combined with stabilisers to create various solid/liquid products that can be sold. Particularly synthetic culture mediums are among the most expensive components of commercial enzyme synthesis, accounting for approximately to 30%–40% of the overall cost of production. Therefore, cost-effective approaches to producing hydrolytic microbial biomass, avoiding the need for synthetic growth media, might offer significant commercial advantages for industrial enzyme production.
Cheng et al. (2015) studied how to extract an enzyme called polyphenol oxidase (PPO) from potato starch wastewater. PPO has many uses but is expensive to produce commercially, so it is not used as much as it could be. The researchers adjusted the pH of the wastewater to 3.5 to cause a chemical reaction that would cause a protein called PPO to separate from the wastewater. They then added 50% acetone to the wastewater to cause a different chemical reaction that would cause a different protein called beta-amylase to separate from the wastewater. Finally, they added 80% acetone to the wastewater to cause a reaction that would cause a type of carbohydrate called sporamins to separate from the wastewater. After the acetone was recovered, the researchers were able to obtain 4.3 × 105 units of PPO, 4.0 × 106 units of beta-amylase, 8.70 g of sporamins, and 20.2 g of SMNs from 1 kg of sweet potato wastewater. Libardi et al. (2017) studied the possibility of using wastewater from toilets containing toilet paper as a source of cellulose for producing cellulases. Using wastewater as a source of cellulose makes it possible to produce an added-value product while also reducing the pollution charge of the wastewater by consuming it as a source of carbon and nutrients. In another study Libardi et al. (2019) conducted a study to produce cellulase enzymes from domestic wastewater. They used Trichoderma harzianum as the culture medium and tested three bioreactor systems; a bubble column bioreactor (BCB), column-packed bed reactor (CPBR), and stirred tank reactors (STR). The BCB system was the most successful, producing the highest cellulase activity and productivity of 31 UFP/mL and 645 UFP/mL.h without needing immobilisation. The fermented broth was then microfiltered and ultrafiltered, resulting in a cellulase recovery of 73.5% using a 30 kDa membrane, which increased the activity concentration by 4.23 times. Additionally, the study found that the COD and nitrogen concentration in the wastewater was reduced by 81.37% and 52.9%, respectively. The production of cellulase enzymes from domestic wastewater and the subsequent development of an added-value process with minimal environmental impact are both highly anticipated opportunities. Moreover, accounting about 30% of the global enzyme market, amylase is a vital industrial enzyme. (Gomez-Villegas et al., 2021). It has significant role in multiple industries, such as pharmaceuticals, textiles, chemicals, paper, and food. Yusree et al. (2022) used a Liquid biphasic system (LBS) to extract and purify a type of amylase called beta-amylase from sweet potato slurry. This LBS is a green extraction technology that combines polyethylene glycol and citrate to separate the beta-amylase from the sweet potato slurry. The results demonstrate an upward trend in recovery yield (78.24%), purification factor (3.5087), and selectivity when salt and polymer concentrations upsurged.
4 Valorisation of wastewater and sludge
4.1 Production of biofuels
Wastewater and sewage sludge are no longer considered a waste because it may be used to recover, recycle and produce valuable products (Singh et al., 2022). Biofuels like biogas, biodiesel, biohydrogen, bioethanol, etc., can be produced using wastewater and sludge. Anaerobic digestion methods are already being used on an industrial scale to effectively valorise the sludge as these provide various social and environmental benefits in addition to green energy and manure (Pasciucco et al., 2023). Biogas production from organic waste helps reduce the negative impacts associated with organic wastes in landfills causing groundwater and soil pollution, the emission of air pollutants such as dioxins and furans, methane, and more substantial greenhouse gases. However, the amount of methane gas produced from sludge by anaerobic digestion is lower than other types of organic waste. Thus, pretreatment and co-digestion contribute to improving the degradability of organic matter and methane production potential of sludge, respectively (Kumar et al., 2017; Lewis et al., 2017; Elalami et al., 2019; Yadav et al., 2023).
Municipal wastewater sludge, pulp, and paper wastewater containing cellulose combined with lignin could be used as raw materials for bioethanol production (Bon et al., 2022). Using cellulases enzymes from Trichoderma reesei, cellulose components are hydrolyzed into sugar (saccharification), and the resulting sugar is used as a raw ingredient for the production of bioethanol by Saccharomyces cerevisiae (el-Zawawy et al., 2011). Further, in a study led by Dufreche et al. (2007), Lipids were used as a cheap source of raw material for the production of biodiesel after being extracted from municipal wastewater sludge using solvents such n-hexane, methanol, acetone, and supercritical CO2. Additionally, dried sludge was reported to be used to produce 6.23% biodiesel using in situ transesterification process (Dufreche et al., 2007). Owing to its clean product and high energy production (122 kJ/g), hydrogen is an attractive energy source. Water electrolysis, steam reforming of hydrocarbons, and auto-thermal processes are well-known ways of producing hydrogen gas, but these are expensive due to high energy needs. Environment-friendly anaerobic biohydrogen fermentation from organic wastes is gaining popularity because of its minimum waste and bioenergy produced. A low hydrogen-yielding rate is essential in biohydrogen synthesis from the garbage. Several aspects must be addressed to maximise hydrogen generation throughout the fermentation process, such as minimising hydrogen loss to hydrogen-consuming anaerobes like methanogens. Other elements, such as inoculum, substrate, temperature, nitrogen sparging, and early startup, have all been investigated by researchers to increase hydrogen generation (Li et al., 2008; Tang et al., 2008). However, biofuel production from wastewater and sludge is still in its infancy. Therefore, further large-scale studies are required to realise the benefits of this new technology at an industrial scale.
4.2 Production of biopolymers
To build a sustainable wastewater treatment facility, resource recovery from wastewater sludge is emphasised. Wastewater sludge is thought of as a viable source for recovering high-value products like biopolymers and biofertilizers. (Sharma et al., 2022). Activated sludge is a matrix of microbial cells entrenched in an extracellular polymeric substance (EPS) released by microbes. About 10%–40% dry weight of total activated sludge accounts for the EPS. EPS is considered as rich source of polysaccharides, nucleic acid, lipids, proteins, and humic acids (Basuvaraj et al., 2015; Boltz et al., 2017). In a recent study, EPS mined from wastewater sludge has been characterised to measure physical and chemical properties (Lin et al., 2013; 2018; Pronk et al., 2015). The matrix’s biopolymers provide a distinctive structure that can be salvaged to create potentially useful biomaterials. (Sheng et al., 2010; More et al., 2014). For instance, alginate-like extracellular polymers (ALE) can potentially be extracted from aerobic granular sludge (AGS) (Rehm, 2010; Lin et al., 2015; Lin et al., 2018). Due of their widespread use in the food, paper, medicinal, construction, and textile industries, the recovery of these ALE polymers from sludge is receiving a lot more attention lately (Kim et al., 2020; Li et al., 2021).
By using solution casting, EPS recovered from surplus granular sludge was successfully integrated into polyvinyl alcohol (PVA) (Kim et al., 2022). Polyvinyl alcohol (PVA) is a type of material that has many uses. It is known for its strong resistance to chemicals and its physical qualities, making it useful for various applications. These applications include textile production, paper sizing, and the manufacture of filaments (Mohareb et al., 2011). In the building industry, it is common to use a certain substance as a binding and thickening agent for latex paints and seals. This substance helps to hold the paint and seal together, making it thicker and more durable (Marten, 2002) and as a modifier to improve the strength and durability of the material in fibre reinforcement and cement-based composite materials (Thong et al., 2016).
4.3 Production of biofertilisers
The valorisation of waste into byproducts by applying a circularity principle is a central and essential issue to foster sustainable economic development. In this context, municipal wastewater has a high potential for nutrient valorisation and sustainable production chain development. An example is sewage sludge as a source of nutrients for crops (Table 5). Dewatered sludge containing nutritional and organic content is utilised as agricultural fertiliser. Sludge’s organic matter, nitrogen, and phosphorus content promotes plant development and improves agricultural soil quality. Majority of farmers from West African and South Asian countries view untreated sewage as a useful fertiliser, despite the fact that using untreated sludge as a fertiliser is unacceptable (Cofie et al., 2005; Verhagen et al., 2012). Farmers use untreated dried sewage as free fertiliser, including nitrogen (322 kg N/ha/year) and phosphorus (64 kg P/ha/year). The chemical composition and characteristics of untreated and digested sewage sludge are shown in Table 1. The demand for fertilisers for agricultural production is increasing due to the world population (Mahmoud et al., 2022c). At current demand for nitrogen is more than 130 million tonnes, and the demand for phosphorus is about 16 million tonnes. Recovery of nutrients from wastewater sludge could meet the local requirement of fertilisers for agricultural production (FAO, 2008; FAO, 2012; Balasubramanian and Tyagi, 2017).
Recent advancement in sewage sludge treatment technology allows the recovery of nutrients such as nitrogen and phosphorus. These recovered nutrients can be utilised as bio-fertilisers by replacing synthetic fertilisers (Herzel et al., 2016). Rebah et al. (2002) and Singh et al. (2013) conducted experiments to explore the utilisation of sludge as a medium to grow Rhizobium bacteria which has been applied as a bio-fertiliser directly onto the soil. Paliya et al. (2019) also experimented on sludge ash. They concluded that sludge ash could be utilised as a sustainable raw material to produce bio-fertilisers. This conclusion was made possible by incorporating it in a definite amount with the inoculum of different microbial species. Industrial and municipal wastewater sludge (primary and secondary sludge from wastewater treatment plants) was used as a raw material to grow various rhizobial strains such as Sinorhizobium meliloti, and Rhizobium leguminosarum bv. viciae, Bradyrhizobium japonicum, and Bradyrhizobium elkanii. Among all these strains S. meliloti strain grew well compared to other strains in the sludge. Sludge characteristics also influenced bacterial growth, cell yield, and fermentation time. Suspended solid particles increased in sludge significantly reduced the oxygen transfer and the rhizobial growth (Rebah et al., 2001). Romero-Garcia et al. (2022) used wastewater sludge as a raw material to form a microalgal bio fertiliser high-pressure homogenisation process. This process converts the microalgal biomass produced in the wastewater treatment plant into a bio-fertiliser used in agriculture to improve crops and reduce traditional fertilisers. Subsequently, progress toward a sustainable food system for the twenty-first century under the United Nations’ 2030 Agendas of Sustainable Development Goals. Microalgae grown in wastewater can be utilised as a bio-fertiliser for soil amendment. Microalgal biomass can enhance soil nitrogen and phosphorus levels and numerous other plant-required trace elements (Ca, K, Fe, and Mn). When combined with soil-based phosphorus-solubilizing organisms, microalgal fertilisers have boosted phosphorus release in the soil. The landfill leachate biomass possesses great potential to generate biofertilizers (Tighiri and Erkurt, 2019; Solovchenko et al., 2021). A study led by Umamaheswari and Shanthakumar (2021), reported 30% increase in the dry weight and biochemical content of wheat crop upon input of filamentous microalgae strains as bio-fertilizers. Microalgae and bacteria symbiotically transform organic and inorganic compounds into smaller biomolecules that plants can absorb, making them efficient biofertilizers (Ferreira et al., 2019).
4.4 Production of biopesticides
Biological agents might be used to manage and target insects in a specific and selective manner, reducing the need for hazardous chemical pesticides while simultaneously improving environmental quality and human health (Sabbahi et al., 2022). To attain a high yield of biopesticide manufacturing, wastewater sludge has been proven an economically viable solution (Butu et al., 2022). Wastewater sludge is considered a global problem, and its sustainable management via value addition is an efficient alternative to other disposal methods, such as landfilling and incineration (Sachdeva et al., 2000). Tyagi et al. (2002) achieved the production of Bacillus thuringiensis, a potential biopesticide by using both industrial and municipal wastewater sludge as raw materials. Both municipal and industrial wastewater sludge has been proven viable for biopesticide synthesis; however, the entomotoxicity potential of the biopesticide produced was found to rely on the nutrient composition of the sludge (Tyagi et al., 2002; Yezza et al., 2005). Utilisation of sludge waste for Bt biopesticide has potential to can considerably cut the production costs while contributing significantly to sustainable sludge usage and management. Brar et al. (2006) used submerged fermentation in the bioconversion of wastewater sludge into Bt-based biopesticides. However, extraction of microorganism was found to be challenging due to the low concentration of products in submerged fermentation, resulting in complex downstream processing (Brar et al., 2006) and necessitating pricy and high end equipments such as a high-speed cooling centrifuge and spray dryer (el-Bendary, 2006). In contrast to traditional submerged fermentation, solid-state fermentation technology has several biotechnological advantages, including low to medium cost and energy consumption, little effluent output, and decent product stability (Pandey, 2003; Holker and Lenz, 2005; Singhania et al., 2009).
Therefore, producing Bt-based biopesticides from sludge or wastewater could be a novel and ingenious way to clean up the environment and an addition to the path for commercialising affordable biopesticides (Tiwari and Awasthi, 2022). Biopesticide production from the starch industry wastewater and sludge has been thoroughly studied including pretreatment, medium amelioration agents, optimising process parameters, scaleup, and formulation and development studies (Tyagi et al., 2002; Brar et al., 2004). Wastewater sludge and starch industry wastewater proved to be very effective media for the cultivation of Bt (Ndao et al., 2021). The shift from linear to circular economies, in which the value of resources, materials, and products is retained throughout the value chain, is an essential step towards establishing a more sustainable, waste free, and competitive economy. Bacillus thuringiensis (Bt) is the most frequently employed microbial biopesticide, producing crystal proteins with biopesticide activity against insects from the orders Coleoptera, Lepidoptera, Diptera, Hymenoptera, Hemiptera, and Orthoptera, as well as phytopathogenic nematodes and terrestrial gastropods (Malovichko et al., 2019). The sporulation process is linked to the synthesis of this protein (Cry protein) (Chandler et al., 2011). Nowadays, 90% of commercialised biopesticides are produced from this entomopathogenic bacteria. These biopesticides are more effective to target pests even in a small dose, and are fast decomposable without leaving hazardous residues as compare to chemical conventional pesticides (Kumar and Singh, 2015; Damalas and Koutroubas, 2018; Yadav et al., 2022). Biopesticides, bioherbicides, biofertilizers, bioplastics, and enzymes derived from wastewater sludge are low-cost biological alternatives that can compete with chemicals or other cost-intensive biological goods in present market. The bioconversion of wastewater sludge into biopesticides or other bio-control agents, has been contemplated, with promising results. Trichoderma, Rhizobium, and Bacillus sp. are the more capable of growing in wastewater and wastewater sludge and producing microbial derivatives utilised in biocontrol and bio-bleaching processes. (Verma et al., 2007a; b; Subramanian et al., 2008). Unfortunately, Trichoderma sp. has not been widely used due to lack of economically feasible procedures, as most existing techniques uses expensive raw ingredients and have lower spore production, which is critical for their performance as a bio-control agents. Alam and Fakhrul-Razi (2003) discussed the use of Trichoderma sp. for wastewater and wastewater sludge treatment to decompose organic matter and hazardous organic compounds, but they did not use the waste as a raw material for the manufacturing of value-added products. According to Verma et al. (2007a), Verma et al. (2007b), Trichoderma viride is a promising bacterium that forms laccases in wastewater sludge and has the ability to breakdown or detoxify organic contaminants such as BPA. Cells of Rhizobium sp. and Sinorhizobium meliloti are utilised as microbial inoculants in leguminous cultures and as biological control agent in agricultural crops. Laccases generated by S. meliloti have the capacity to breakdown or detoxify organic chemicals, particularly phenolic groups contained in wastewater sludge, while also bio-converting wastewater sludge into value-added products (Rosconi et al., 2005). Bacillus sp. can generate enzymes that breakdown organic contaminants in wastewater and wastewater sludge, such as oxidases, dehydrogenases, and peroxidases. Bacillus thuringiensis generates tyrosinases, which may breakdown phenolic substances (Donova et al., 2005; Quan et al., 2005). Biopesticides based on B. thuringiensis have been demonstrated to breakdown an Endocrine disrupting chemical, Dimethyle patholate, concurrently with biopesticide synthesis (Brar et al., 2009). Consequently, value-added product-generating microorganisms like Rhizobium sp., Bacillus sp., and Trichoderma sp. have an enzyme system that can efficiently break down organic chemicals (Mohapatra et al., 2010). Several researchers have utilised SSF technology to create Bt-derived biopesticides from soy waste, sugar beet pulp, sesame meal, wastewater sludge and wasted mushrooms. Zhang et al. (2013) achieved a successful fermentation procedure with 35 kg of fermentation media that was mostly made of kitchen garbage. When the spore count was increased from 4 to 8 kg, the ultimate spore counts increased. When the procedure was expanded up to 35 kg, spore output reduced marginally. When 40 kg of substrate was used, this value significantly reduced (from 9.6108 CFU/g to 6.4106 CFU/g), owing to insufficient ventilation and heat removal as the primary hypothetic factors. The feasibility of utilising digestate as a fermentation substrate for B. thuringiensis var kurstaki (Btk) culture and spore generation was investigated by Rodriguez et al. (2019). This proof of concept demonstrated that the procedure was achievable at lab and bench-scale (10-L), with scanning electron microscopy pictures confirming the existence of protein crystals. Nevertheless, scaling up to 22 and 100-L proved that Bt was unable to develop, revealing only its capacity to sporulate. As a result, the procedure for producing biopesticide must be improved to improve Bt’s capacity to colonise solid material at these sizes. A successful operational strategy for B. thuringiensis spore production was achieved using a digestate-biowaste mixture (62.5%–37.5%, wet weight basis) and a novel aeration strategy divided into two stages: a micro aeration phase during the first hours of fermentation, followed by a high-rate aerated period after 22 h. Under these circumstances, Bt spore production ranged between 5 × 107 and 1.5 × 108 spores/g dry matter, with a maximum production yield of 5–7.3 spores/CFU. Biodegradability has emerged as a critical issue in the feasibility of both Bt growth and two-stage aeration strategies. This research established the operational framework for carrying out the developed method on a demonstration scale (Mejias et al., 2020). Therefore, using sludge for biopesticide production will minimise the environmental problem of sludge disposal and the problems encountered with chemical pesticides.
5 Life cycle assessment and techno-economic analysis
The term “life cycle assessment” (LCA) refers to the process of measuring a product’s environmental impact throughout the course of its whole life cycle, from conception to disposal. LCA is a methodical process that uses ISO 14040 (2006) standards to translate a system’s inputs and outputs into corresponding environmental consequences. LCA is divided into four stages—goal and scope definition, data acquisition and life cycle inventory, life cycle impact assessment, and outcome interpretation (Cortes et al., 2020).
Sangma and Chalie (2023) performed LCA and techno-economic analysis (TEA) to demonstrate that wastewater treatment with microalgae can significantly lessen adverse environmental effects in comparison to conventional methods. The system also provided the benefit of low operating costs, scope for nutrient recovery, waste valorisation into value added products, and the ability to reduce emissions by absorbing CO2 from flue gases. A more comprehensive environmental assessment was also recommended in the review by Lee and Jepson (2021) which compared the LCA studies of RO against emerging technologies, such as forward osmosis and capacitive deionization. This review highlighted the trade-off between carbon emissions and chemical treatment and concluded emerging technologies that aim to reduce energy consumption have the potential to increase other environmental burdens such as chemical usage; Hand and Cusick (2021) performed LCA for electrochemical oxidation (EO) technology and reported that EO is a promising technique for wastewater treatment and purification, but it may pose unintended negative consequences on the environment due to the consumption of resources and energy during the electrodes manufacturing and operational stages. Sun et al. (2023) quantitatively assessed the LCA of the EO method’s environmental effects from the lab to the industrial scale. As per their findings EO was found to be efficient in destroying organic pollutants in wastewater, however, the benefits were outweighed by the environmental burdens related to the manufacturing of anode materials, use of electrolytes, and energy consumption during various operational stages. This obligated the attention to the LCA by taking reactor design, anode materials, electrolyte, and flow pattern into consideration, as well as demanded decentralized location with a significant share of renewable power source and stringent contamination control policies for WWTPs.
Juneja and Murthy (2017) applied an engineering course model on a renewable diesel (RD) production facility with a processing capacity of 60 Mgal wastewater/day to conduct a TEA of RD production on a commercial scale. Algal RD yields were estimated as 10.18 MML/year, with a production cost per RD of $1.75. According to the LCA, the manufacturing of RD resulted in GHG emissions that were 6.2 times lower than those of regular diesel. Sensitivity study suggested that using high lipid algae or expanding the production facility could lower the cost of producing ethanol. The long-term viability of RD in an ecologically friendly path was found to be helped by the integrated TEA and LCA evaluations. Gholkar et al. (2021) used microalgal biomass grown on steel mill wastewater to perform TEA and LCA on hydrogen and methane generation, and they found life cycle climate change impact was 7.56 kg CO2 eq./kg hydrogen generated, which was 36.47% less than steam reforming of methane. It was not economically possible to produce methane. Yet, with a life cycle climate change impact of 1.18 kg CO2 eq./kg methane generated, it was more environmentally friendly. Sensitivity analysis was done to find areas where costs and life cycle impacts could be further reduced.
Mu et al. (2014), evaluated the environmental performance of wastewater based algal biofuels and reported that environmental performance of wastewater based algal biofuels was much better than freshwater based algal biofuels however, the characteristics of wastewater and conversion technologies played key role in performance and LCA. Arashiro et al. (2022) conducted LCA to compare microalgae systems for wastewater treatment and bioproducts for treating industrial wastewater (food sector) and urban wastewater, with the goal of recovering bioproducts and bioenergy. Findings showed that microalgal based WWT systems assisted bioproduct recovery can minimise the environmental impacts up to five times in comparison to conventional systems owing to the lower chemical consumption for microalgae cultivation. Because of its higher quality compared to urban wastewater, which also permits the growing of a single species of microalgae, food industry effluent emerged as the most efficient set-up for the recovery of bioproducts from microalgae treated wastewater. In conclusion, the LCA advocated the microalgae wastewater treatment systems a promising alternative for wastewater treatment and nutrient recovery. Castro et al. (2020) used LCA to compare the environmental effects of producing microalgae biomass-based phosphate biofertilizer to triple superphosphate. Phosphorus recovery was made from the wastewater of the meat processing sector using microalgae. Climate change impact was found to be 3.17 kg CO2eq. Microalgae biofertilizer had higher environmental effect than conventional fertilizer in all impact categories. To get around this, a seamless set-up was established that used photovoltaic panels as the power source and a drying bed for biomass drying instead of thermal drying; Styles et al. (2018) used LCA to evaluate the production and usage of digestate biofertilizer (DBF) derived from liquid digestate (LD), accounting for SFS efficacy, with the conventional management of LD from food waste. More judicious use of nutrients in the DBF product could result in benefits in terms of environment and economy by preventing methane, nitrogen oxides, and ammonia gas emissions from LD and SFS. They came to the conclusion that DBF extraction was economically advantageous and sustainable after averaging these results against larger environmental loadings.
Henceforth, the use of LCA is extremely helpful in determining the energy products and co-products which will demonstrate higher economic viability and environmental performance (Collet et al., 2015). Since there is a large variability in assessments done by multiple studies, Quinn and Davis (2014) emphasized the need for integrated Resource assessment (RA), Techno economic analysis (TEA), and LCA constructed on a foundation of experimentally validated technological system models.
6 Challenges in resource recovery and wastewater valorisation
Wastewater valorisation is a potential method to reuse and recycle water and nutrients for economic generation and environmental protection. However, the current status and technological bottlenecks still inhibit the effective use of this concept. Different countries have different obstacles that they need to overcome. The difficulty of these obstacles can vary depending on the situation.
1. The main challenge with Membrane separation technology is figuring out which membrane is best suited for the desired outcome. It is important to choose the right membrane suitable for the process to be successful. To compete with the existing conventional technologies, membrane clogging and fouling are still considered the primary stumbling blocks for membrane-based separation.
2. Enzymatic catalysis and recovery of products depend upon the physicochemical properties of wastewater, such as pH; since enzymes are heat and pH labile and are easily denatured in highly basic or acidic solutions, this may limit their functioning, increasing the cost of treatment and valorisation.
3. Microalgae-based WWT is one of the most optimistic technologies for waste’s advanced treatment and nutrient recovery. Most studies have been conducted at pilot or bench scale and under controlled conditions. Thus, cultivation under outdoor conditions and scaling up of reactors are still needed to evaluate the direct interferences on processes involved in the valorisation of wastewater. However, large-scale cultivation under outdoor conditions is still a challenge.
4. Economic, technical, political, institutional, and social challenges are frequently encountered in implementing small and large-scale valorisation operations.
5. Another big challenge is the lack of trust and awareness of recycled/recovered and valorised products. Many consumers may be skeptical about the quality of valorised and recycled products. The consumer may perceive the products based on the Circular economy as high-risk and uncertain.
6. Additionally, if the price of non-circular products is higher than circular/valorised products, then taxation on virgin resources (resources that have not been used before) could be used to make the circular/valorised products more attractive to consumers. Thus, taxation on virgin resources might provide an advantage.
7. A key barrier often presents unavailable and irregular infrastructure. in developing countries, these systems and services are often not up to the same standards as in more developed countries.
7 Conclusion and recommendations
Adopting the circular economy concept is crucial to meet global challenges. These global challenges include increasing demand for water supply, water scarcity, and declining non-renewable resources. Sludge and wastewater are no longer considered to be trash, but rather a source of valuable products that can benefit environmentally and social-economically. In this review, authors have explained the wastewater and sludge valorisation for multiple aspects such as water resource management, environmental protection, resource recovery, and generation of value-added products. With the aid of the cutting-edge technologies, wastewater can be efficiently treated, resulting in effluents of a calibre that meets the standards of industry and agriculture. Water reuse is not the only way to value wastewater. Recognizing that wastewater contains a variety of valuable resources that can be recovered is vital. In order to reduce pollution, protect the environment, wastewater treatment processes must recover nutrients. Traditional approaches by themselves are insufficient to extract nutrients in high quantities and of desired quality until they are united with membrane technology. The use of the unique hybrid systems in nutrient recovery is highlighted. To increase the technological viability and lower operational expenses, further work must still be done to improve the recovery system’s usability. Finally, apart from this, governments should put into place relevant legislation, policies, and regulations to support and encourage nutrient recovery strategies.
Nutrients such as phosphorous, nitrogen, phenolic compounds, antioxidants, vitamins, proteins, enzymes, and other value-added products can be potentially recovered from wastewater from various industrial sectors. High-end and novel separation and purification technologies, such as membrane technology, enzyme-mediated separation, chromatography, microalgae cultivation, and novel adsorbents, enable efficient recovery of nutrients and treatment of wastewater. Various strategies can be applied to valorise this waste related to excess sludge produced during wastewater treatment. Value-added substances made from anaerobic sludge digestion, such as bio-fertilizers, bioplastics, biofuels, and biocompost, are already used extensively in large-scale processes. Yet, incorporating the water sector into the circular economy has increased collaboration between the public sector, academia, and industry. Furthermore, comprehensive cost-benefit analyses (CBA) and LCA may support the implementation of the suggested technologies as workable substitutes. Moreover, valorisation procedures are more dependable as compared to conventional disposal methods because they generate value and minimise pollution, even when the market value of the recovered materials may not be high enough to justify their application.
Author contributions
SK compiled and edited the manuscript. SS and RY framed the concept and worked on the figures and tables. AS supervised the various concepts distributed across the institutions. AM completed the final editing. All authors contributed to the article and approved the submitted version.
Funding
The authors gratefully acknowledge the reviewers for their insightful comments and suggestions. SS acknowledges financial assistance in the form of a research project under Women Scientist Scheme-B, WISE-KIRAN DIVISION, Department of Science and Technology, Ministry of Science and Technology, Government of India (Project Grant No. DST/WOS-B/2018/1589).
Conflict of interest
The authors declare that the research was conducted in the absence of any commercial or financial relationships that could be construed as a potential conflict of interest.
Publisher’s note
All claims expressed in this article are solely those of the authors and do not necessarily represent those of their affiliated organizations, or those of the publisher, the editors and the reviewers. Any product that may be evaluated in this article, or claim that may be made by its manufacturer, is not guaranteed or endorsed by the publisher.
References
Adam, G., Mottet, A., Lemaigre, S., Tsachidou, B., Trouv´e, E., and Delfosse, P. (2018). Fractionation of anaerobic digestates by dynamic nanofiltration and reverse osmosis: An industrial pilot case evaluation for nutrient recovery. J. Environ. Chem. Eng. 6 (5), 6723–6732. doi:10.1016/j.jece.2018.10.033
Adebayo, O. T., Fagbenro, O. A., and Jegede, T. (2004). Evaluation of Cassia fistula meal as a replacement for soybean meal in practical diets of Oreochromis niloticus fingerlings. Aquac. Nutr. 10 (2), 99–104. doi:10.1111/j.1365-2095.2003.00286.x
Ahuchaogu, A. A., Chukwu, O. J., Obike, A. I., Igara, C. E., Nnorom, I. C., Bull, J., et al. (2018). Reverse osmosis technology, its applications and nano-enabled membrane. Int. J. Adv. Res. Chem. Sci. 5, 20–26. doi:10.20431/2349-0403.0502005
al Aani, S., Mustafa, T. N., and Hilal, N. (2020). Ultrafiltration membranes for wastewater and water process engineering: A comprehensive statistical review over the past decade. J. Water Process Eng. 35. doi:10.1016/j.jwpe.2020.101241
Alam, M. Z., and Fakhrul-Razi, A. (2003). Enhanced settleability and dewaterability of fungal treated domestic wastewater sludge by liquid state bioconversion process. Water Res. 37 (5), 1118–1124. doi:10.1016/S0043-1354(02)00452-9
Alewell, C., Ringeval, B., Ballabio, C., Robinson, D. A., Panagos, P., and Borrelli, P. (2020). Global phosphorus shortage will be aggravated by soil erosion. Nat. Commun. 11, 4546. doi:10.1038/s41467-020-18326-7
Amini, E., Babaei, A., Mehrnia, M. R., Shayegan, J., and Safdari, M. S. (2020). Municipal wastewater treatment by semi-continuous and membrane algal-bacterial photo-bioreactors. J. Water Process Eng. 36, 101274. doi:10.1016/j.jwpe.2020.101274
Arashiro, L. T., Boto-Ordóñez, M., Hulle, S. W. H. V., Ferrer, I., Garfí, M., and Rousseau, D. P. L. (2020). Natural pigments from microalgae grown in industrial wastewater. Bioresour. Technol. 303, 122894. doi:10.1016/j.biortech.2020.122894
Arashiro, L. T., Josa, I., Ferrer, I., Van Hulle, S. W. H., Rousseau, D. P. L., and Garfi, M. (2022). Life cycle assessment of microalgae systems for wastewater treatment and bioproducts recovery: Natural pigments, biofertilizer and biogas. Sci. Total Environ. 847, 157615. doi:10.1016/j.scitotenv.2022.157615
Ariaeenejad, S., Kavousi, K., Han, J. L., Ding, X. Z., and Salekdeh, G. H. (2023). Efficiency of an alkaline, thermostable, detergent compatible, and organic solvent tolerant lipase with hydrolytic potential in biotreatment of wastewater. Sci. Total Environ. 866, 161066. doi:10.1016/j.scitotenv.2022.161066
Badanthadka, M., and Mehendale, H. M. (2014). Encyclopedia of toxicology. 3rd ed. Cambridge, Massachusetts, United States: Academic Press.
Balasubramanian, S., and Tyagi, R. D. (2017). Value-added bio-products from sewage sludge” in Current developments in Biotechnology and Bioengineering. Amsterdam, Netherlands: Elsevier, 27–42. doi:10.1016/B978-0-444-63664-5.00002-2
Bashir, I., Lone, F. A., Bhat, R. A., Mir, S. A., Dar, Z. A., and Dar, S. A. (2020). in Concerns and threats of contamination on aquatic ecosystems” in bioremediation and biotechnology Editors K. Hakeem, R. Bhat, and H. Qadri (Cham: Springer), 1–26. doi:10.1007/978-3-030-35691-0_1
Basuvaraj, M., Fein, J., and Liss, S. N. (2015). Protein and polysaccharide content of tightly and loosely bound extracellular polymeric substances and the development of a granular activated sludge floc. Water Res. 82, 104–117. doi:10.1016/j.watres.2015.05.014
Bazzarelli, F., Piacentini, E., Poerio, T., Mazzei, R., Cassano, A., and Giorno, L. (2016). Advances in membrane operations for water purification and biophenols recovery/valorization from OMWWs. J. Membr. Sci. 497, 402–409. doi:10.1016/j.memsci.2015.09.049
Bell, E. L., Finnigan, W., France, S. P., Hayes, M. A., Hepworth, L. J., Flitsch, S. L., et al. (2021). Biocatalysis. Nat. Rev. Methods Prim. 1, 46. doi:10.1038/s43586-021-00044-z
Bendary, M. A. (2006). Bacillus thuringiensis and Bacillus sphaericus biopesticides production. J. Basic Microbiol. 46, 158. doi:10.1002/jobm.200510585
Blanco-Vieites, M., Casado, V., Battez, A. H., and Rodríguez, E. (2023). Culturing Arthrospira maxima in mining wastewater: Pilot-scale culturing and biomass valorisation into C-phycocyanin and crude lipid extract. Environ. Technol. Innov. 29, 102978. doi:10.1016/j.eti.2022.102978
Bolger, K., and Doyon, A. (2019). Circular cities: Exploring local government strategies to facilitate a circular economy. Eur. Plan. Stud. 27 (11), 2184–2205. doi:10.1080/09654313.2019.1642854
Boltz, J. P., Smets, B. F., Rittmann, B. E., Van Loosdrecht, M. C., Morgenroth, E., and Daigger, G. T. (2017). From biofilm ecology to reactors: A focused review. Water Sci. Technol. 75 (8), 1753–1760. doi:10.2166/wst.2017.061
Bon, D. C., Okada, D. Y., and Coneglian, C. M. R. (2022). Benefits of biological additive inoculation in the treatment of effluent from the paper recycling industry. J. Water Process Eng. 50, 103269. doi:10.1016/j.jwpe.2022.103269
Bora, R. R., Richardson, R. E., and You, F. (2020). Resource recovery and waste-to-energy from wastewater sludge via thermochemical conversion technologies in support of circular economy: A comprehensive review. BMC Chem. Eng. 2 (1), 8–16. doi:10.1186/s42480-020-00031-3
Brar, S. K., Verma, M., Tyagi, R. D., and Valéro, J. R. (2006). Recent advances in downstream processing and formulations of Bacillus thuringiensis based biopesticides. Process Biochem. 41 (2), 323–342. doi:10.1016/j.procbio.2005.07.015
Brar, S. K., Verma, M., Tyagi, R. D., Valéro, J. R., Surampalli, R. Y., and Banerji, S. K. (2004). Development of sludge based stable aqueous Bacillus thuringiensis formulations. Water Sci. Technol. 50 (9), 229–236. doi:10.2166/wst.2004.0578
Brar, S. K., Verma, M., Tyagi, R. D., Valéro, J. R., and Surampalli, R. Y. (2009). Entomotoxicity, protease and chitinase activity of Bacillus thuringiensis fermented wastewater sludge with a high solids content. Bioresour. Technol. 100 (19), 4317–4325. doi:10.1016/j.biortech.2007.09.093
Brugnari, T., Pereira, M. G., Bubna, G. A., de Freitas, E. N., Contato, A. G., Corrêa, R. C. G., et al. (2018). A highly reusable MANAE-agarose-immobilized Pleurotus ostreatus laccase for degradation of bisphenol A. Sci. Total Environ. 634, 1346–1351. doi:10.1016/j.scitotenv.2018.04.051
Buchanan, D., Martindale, W., Romeih, E., and Hebishy, E. (2023). Recent advances in whey processing and valorisation: Technological and Environmental perspectives. Int. J. Dairy Technol. 2023. doi:10.1111/1471-0307.12935
Butu, M., Rodino, S., and Butu, A. (2022). in Biopesticide formulations - current challenges and future perspectives” in advances in bio-inoculant science, biopesticides Editors A. Rakshit, V. S. Meena, P. C. Abhilash, B. K. Sarma, H. B. Singh, and L. Fracto (Sawston, Cambridge: Woodhead Publishing), 19–29. doi:10.1016/B978-0-12-823355-9.00010-9
Canadas, R., Díaz, I., Rodríguez, M., Gonzalez, E. J., and Gonzalez-Miquel, M. (2022). An integrated approach for sustainable valorization of winery wastewater using bio-based solvents for recovery of natural antioxidants. J. Clean. Prod. 334, 130181. doi:10.1016/j.jclepro.2021.130181
Careghini, A., Mastorgio, A. F., Saponaro, S., and Sezenna, E. (2015). Bisphenol A, nonylphenols, benzophenones, and benzotriazoles in soils, groundwater, surface water, sediments, and food: A review. Environ. Sci. Pollut. Res. 22, 5711–5741. doi:10.1007/s11356-014-3974-5
Cassano, A., Conidi, C., and Ruby Figueroa, R. (2014). Recovery of flavonoids from orange press liquor by an integrated membrane process. Membranes 4, 509–524. doi:10.3390/membranes4030509
Castro, J. S., Calijuri, M. L., Ferreira, J., Assemany, P. P., and Ribeiro, V. J. (2020). Microalgae based biofertilizer: A life cycle approach. Sci. Total Environ. 1, 138138. doi:10.1016/j.scitotenv.2020.138138
Chandler, D., Bailey, A. S., Tatchell, G. M., Davidson, G., Greaves, J., and Grant, W. P. (2011). The development, regulation and use of biopesticides for integrated pest management. Philos. Trans. R. Soc. Lond., B, Biol. Sci. 366 (1573), 1987–1998. doi:10.1098/rstb.2010.0390
Chedeville, O., Debacq, M., and Porte, C. (2009). Removal of phenolic compounds present in olive mill wastewaters by ozonation. Desalination 249, 865–869. doi:10.1016/j.desal.2009.04.014
Chen, J., Li, J., Zhang, X., and Wu, Z. (2020). Pretreatments for enhancing sewage sludge reduction and reuse in lipid production. Biotechnol. Biofuels. 13, 204. doi:10.1186/s13068-020-01844-3
Chen, X., Zhou, Q., Liu, F., Peng, Q., and Teng, P. (2019). Removal of nine pesticide residues from water and soil by biosorption coupled with degradation on biosorbent immobilized laccase. Chemosphere 233, 49–56. doi:10.1016/j.chemosphere.2019.05.144
Cheng, S., Zhang, Y. F., Zeng, Z. Q., Lin, J., and Ni, H. (2015). Screening, separating, and completely recovering polyphenol oxidases and other biochemicals from sweet potato wastewater in starch production. Appl. Microbiol. Biotechnol. 99, 1745–1753. doi:10.1007/s00253-014-6034-7
Christaki, E., Bonos, E., and Florou-Paneri, P. (2015). in Innovative microalgae pigments as functional ingredients in nutrition” in Handbook of marine microalgae Editor S. K. Kim (Amsterdam: Elsevier), 233–243. doi:10.1016/B978-0-12-800776-1.00014-5
Cieslik, B. M., Namieśnik, J., and Konieczka, P. (2015). Review of sewage sludge management: Standards, regulations and analytical methods. J. Clean. Prod. 90, 1–15. doi:10.1016/j.jclepro.2014.11.031
Cofie, O. O., Kranjac-Berisavljevic, G., and Drechsel, P. (2005). The use of human waste for peri-urban agriculture in Northern Ghana. Renew. Agric. Food Syst. 20 (2), 73–80. doi:10.1079/RAF200491
Collet, P., Hélias, A., Lardon, L., Steyer, J. P., and Bernard, O. (2015). Recommendations for life cycle assessment of algal fuels. Appl. Energy. 154, 1089–1102. doi:10.1016/j.apenergy.2015.03.056
Conidi, C., Rodriguez-Lopez, A. D., Garcia-Castello, E. M., and Cassano, A. (2015). Purification of artichoke polyphenols by using membrane filtration and polymeric resins. Sep. Purif. Technol. 144, 153–161. doi:10.1016/j.seppur.2015.02.025
Cordell, D., Drangert, J. O., and White, S. (2009). The story of phosphorus: Global food security and food for thought. Glob. Environ. Chang. 19, 292–305. doi:10.1016/j.gloenvcha.2008.10.009
Cordell, D., and White, S. (2014). Tracking phosphorus security: Indicators of phosphorus vulnerability in the global food system. Food secur. 7, 337–350. doi:10.1007/s12571-015-0442-0
Cortes, A., Oliviera, L. F. S., Ferrari, V., Taffarel, S. R., Feijoo, and G., and Moreira, M. T. (2020). Environmental assessment of viticulture waste valorisation through composting as a biofertilisation strategy for cereal and fruit crops. Environ. Pollut. 264, 114794. doi:10.1016/j.envpol.2020.114794
Dabestani, S., Arcot, J., and Chen, V. (2017). Protein recovery from potato processing water: Pre-treatment and membrane fouling minimization. J. Food Engin. 195, 85–96. doi:10.1016/j.jfoodeng.2016.09.013
Damalas, C. A., and Koutroubas, S. D. (2018). Current status and recent developments in biopesticide use. Agriculture 8 (1), 13. doi:10.3390/agriculture8010013
Daneshvar, E., Zarrinmehr, M. J., Hashtjin, A. M., Farhadian, O., and Bhatnagar, A. (2018). Versatile applications of freshwater and marine water microalgae in dairy wastewater treatment, lipid extraction and tetracycline biosorption. Bioresour. Technol. 268, 523–530. doi:10.1016/j.biortech.2018.08.032
De Luca, M., Pappalardo, I., Limongi, A. R., Viviano, E., Radice, R. P., Todisco, S., et al. (2021). Lipids from microalgae for cosmetic applications. Cosmetics 8 (2), 52. doi:10.3390/cosmetics8020052
Dias, C., Santos, J. A., Reis, A., and da Silva, L. T. (2023). The use of oleaginous yeasts and microalgae grown in brewery wastewater for lipid production and nutrient removal: A review. Waste Biomass Valor 2023, 1–24. doi:10.1007/s12649-023-02032-8
Diaz, R., Mackey, B., Chadalavada, S., Kainthola, J., Heck, P., and Goel, R. (2022). Enhanced Bio-P removal: Past, present, and future – a comprehensive review. Chemosphere 309 (2), 136518. doi:10.1016/j.chemosphere.2022.136518
Donova, M. V., Egorova, O. V., and Nikolayeva, V. M. (2005). Steroid 17β-reduction by microorganisms—A review. Process Biochem. 40 (7), 2253–2262. doi:10.1016/j.procbio.2004.09.025
Dufreche, S., Hernandez, R., French, T., Sparks, D., Zappi, M., and Alley, E. (2007). Extraction of lipids from municipal wastewater plant microorganisms for production of biodiesel. J. Am. Oil Chem. Soc. 84 (2), 181–187. doi:10.1007/s11746-006-1022-4
Ebhodaghe, S. O., Imanah, O. E., and Ndibe, H. (2022). Biofuels from microalgae biomass: A review of conversion processes and procedures. Arab. J. Chem. 15 (2), 103591. doi:10.1016/j.arabjc.2021.103591
el-Maghrabi, N., Fawzy, M., and Mahmoud, A. E. (2022). Efficient removal of phosphate from wastewater by a novel phyto-graphene composite derived from palm byproducts. ACS Omega 7 (49), 45386–45402. doi:10.1021/acsomega.2c05985
el-Zawawy, W. K., Ibrahim, M. M., Abdel-Fattah, Y. R., Soliman, N. A., and Mahmoud, M. M. (2011). Acid and enzyme hydrolysis to convert pretreated lignocellulosic materials into glucose for ethanol production. Carbohydr. Polym. 84 (3), 865–871. doi:10.1016/j.carbpol.2010.12.022
Elalami, D., Carrere, H., Monlau, F., Abdelouahdi, K., Oukarroum, A., and Barakat, A. (2019). Pretreatment and co-digestion of wastewater sludge for biogas production: Recent research advances and trends. Renew. Sustain. Energy Rev. 114, 109287. doi:10.1016/j.rser.2019.109287
Ersahin, M. E., Cicekalan, B., Cengiz, A. I., Zhang, X., and Ozgun, H. (2023). Nutrient recovery from municipal solid waste leachate in the scope of circular economy: Recent developments and future perspectives. J. Environ. Manag. 335, 117518. doi:10.1016/j.jenvman.2023.117518
Eskikaya, O., Isik, Z., Arslantas, C., Yabalak, E., Balakrishnan, D., Dizge, N., et al. (2023). Preparation of hydrochar bio-based catalyst for fenton process in dye-containing wastewater treatment. Environ. Res. 216, 114357. doi:10.1016/j.envres.2022.114357
FAO (2012). Crop yield response to water, irrigation and drainage paper 66. Rome: Food and Agriculture Organization of the United Nations.
FAO (2008). Current world fertilizer trends and outlook to 2011/12. Rome: Food and Agriculture Organization of the United Nations.
Ferreira, A., Ribeiro, B., Ferreira, A. F., Tavares, M. L., Vladic, J., Vidović, S., et al. (2019). Scenedesmus obliquus microalga-based biorefinery–from brewery effluent to bioactive compounds, biofuels and biofertilizers–aiming at a circular bioeconomy. Biofuel. Bioprod. Bioref. 13 (5), 1169–1186. doi:10.1002/bbb.2032
Foorginezhad, S., and Zerafat, M. M. (2017). Microfiltration of cationic dyes using nano-clay membranes. Ceram. Int. 43, 15146–15159. doi:10.1016/j.ceramint.2017.08.045
Galanakis, C. M. (2013). Emerging technologies for the production of nutraceuticals from agricultural by-products: A viewpoint of opportunities and challenges. Food Bioprod. process. 91, 575–579. doi:10.1016/j.fbp.2013.01.004
Gao, Q., Bouwen, D., Yuan, S., Gui, X., Xing, Y., Zheng, J., et al. (2023). Robust loose nanofiltration membrane with fast solute transfer for dye/salt separation. J. Memb. Sci. 121518, 121518. doi:10.1016/j.memsci.2023.121518
Gholkar, P., Shastri, Y., and Tanksale, A. (2021). Renewable hydrogen and methane production from microalgae: A techno-economic and life cycle assessment study. J. Clean. Prod. 279, 123726. doi:10.1016/j.jclepro.2020.123726
Gomez-Villegas, P., Vigara, J., Romero, L., Gotor, C., Raposo, S., Gonçalves, B., et al. (2021). Biochemical characterization of the amylase activity from the new Haloarchaeal strain Haloarcula sp. Hs isolated in the Odiel Marshlands. Biology 10 (4), 337. doi:10.3390/biology10040337
Gong, H., Meng, F., Wang, G., Hartmann, T. E., Feng, G., Wu, J., et al. (2022). Toward the sustainable use of mineral phosphorus fertilizers for crop production in China: From primary resource demand to final agricultural use. Sci. Total Environ. 804, 150183. doi:10.1016/j.scitotenv.2021.150183
Goula, A. M., and Gerasopoulos, D. (2017). in Integrated olive mill waste (OMW) processing toward complete by-product recovery of functional components” in Olives and olive oil as functional foods: Bioactivity, Chemistry and Processing Editors F. Shahidi, and A. Kiritsakis (New York: Wiley), 177–204.
Gu, Y., Li, Y., Li, X., Luo, P., Wang, H., Robinson, Z. P., et al. (2017). The feasibility and challenges of energy self-sufficient wastewater treatment plants. Appl. Ener. 204, 1463–1475. doi:10.1016/j.apenergy.2017.02.069
Hafiz, M. A., Hawari, A. H., and Altaee, A. (2019). A hybrid forward osmosis/reverse osmosis process for the supply of fertilizing solution from treated wastewater. J. Water Process Eng. 32, 100975. doi:10.1016/j.jwpe.2019.100975
Hand, S., and Cusick, R. D. (2021). Electrochemical disinfection in water and wastewater treatment: Identifying impacts of water quality and operating conditions on performance. Environ. Sci. Technol. 55 (6), 3470–3482. doi:10.1021/acs.est.0c06254
Hatlen, B., Aas, G. H., Jørgensen, E. H., Storebakken, T., and Goswami, U. C. (1995). Pigmentation of 1,2 and 3year old Arctic charr (Salvelinus alpinus) fed two different dietary astaxanthin concentrations. Aquaculture 138 (1–4), 303–312. doi:10.1016/0044-8486(95)01072-6
Herzel, H., Krüger, O., Hermann, L., and Adam, C. (2016). Sewage sludge ash-A promising secondary phosphorus source for fertilizer production. Sci. Total Environ. 542, 1136–1143. doi:10.1016/j.scitotenv.2015.08.059
Holker, U., and Lenz, J. (2005). Solid-state fermentation—Are there any biotechnological advantages? Curr. Opin. Microbiol. 8 (3), 301–306. doi:10.1016/j.mib.2005.04.006
Hui, W., Zhou, J., and Jin, R. (2022). Proteins recovery from waste activated sludge by thermal alkaline treatment. J. Environ. Chem. Eng. 10, 107311. doi:10.1016/j.jece.2022.107311
Hwang, J., Zhang, L., Seo, S., Lee, Y. W., and Jahng, D. (2008). Protein recovery from excess sludge for its use as animal feed. Bioresour. Tech. 99 (18), 8949–8954. doi:10.1016/j.biortech.2008.05.001
Iqbal, A., Yusaf, A., Usman, M., Hussain Bokhari, T., and Mansha, A. (2023). Insight into the degradation of different classes of dyes by advanced oxidation processes; a detailed review. Int. J. Environ. Anal. Chem. 1-35, 1–35. doi:10.1080/03067319.2022.2125312
Iqbal, Z., Abbas, F., Ibrahim, M., Mahmood, A., Gul, M., and Qureshi, T. I. (2022). Ecological risk assessment of soils under different wastewater irrigation farming system in Punjab, Pakistan. Int. J. Environ. Sci. Technol. 19, 1925–1936. doi:10.1007/s13762-021-03237-x
ISO 14040 (2006). Environmental management–life cycle assessment–principles and framework, ISO 14000 international standards compendium. Gene`ve, Switzerland: International Organization for Standardization.
Jadon, N., Sharma, H. K., Guruaribam, N., and Chauhan, A. K. S. (2022). Recent scenario of agricultural contaminants on water resources” in current directions in water scarcity research Editors A. K. Tiwari, A. Kumar, A. K. Singh, T. N. Singh, E. Suozzi, G. Mattaet al. (Amsterdam, Netherlands: Elsevier), 5, 225–246. doi:10.1016/B978-0-323-85378-1.00012-X
Jafarinejad, S. (2021). Forward osmosis membrane technology for nutrient removal/recovery from wastewater: Recent advances, proposed designs, and future directions. Chemosphere 263, 128116. doi:10.1016/j.chemosphere.2020.128116
Jankowska, K., Zdarta, J., Grzywaczyk, A., Degórska, O., Kijeńska-Gawrońska, E., Pinelo, M., et al. (2021). Horseradish peroxidase immobilised onto electrospun fibres and its application in decolourisation of dyes from model sea water. Process Biochem. 102, 10–21. doi:10.1016/j.procbio.2020.11.015
Jiang, W., Lin, L., Gedara, S. M. H., Schaub, T. M., Jarvis, J. M., Wang, X., et al. (2020). Potable-quality water recovery from primary effluent through a coupled algal-osmosis membrane system. Chemosphere 240, 124883. doi:10.1016/j.chemosphere.2019.124883
Juneja, A., and Murthy, G. S. (2017). Evaluating the potential of renewable diesel production from algae cultured on wastewater: Techno-economic analysis and life cycle assessment. AIMS Energy 5 (2), 239–257. doi:10.3934/energy.2017.2.239
Jung, J., Xing, X. H., and Matsumoto, K. (2002). Recoverability of protease released from disrupted excess sludge and its potential application to enhanced hydrolysis of proteins in wastewater. Biochem. Eng. J. 10 (1), 67–72. doi:10.1016/s1369-703x(01)00163-2
Kathi, S. (2022). “Phytoremediation of heavy metals and petroleum hydrocarbons using Cynodon dactylon (L.) Pers,” in Cost effective technologies for solid waste and wastewater treatment (Amsterdam, Netherlands: Elsevier), 135–145.
Kaviya, S. (2022). in Recent advances in water treatment facilities for wastewater reuse in the urban water supply” in Current Directions in Water Scarcity Research Editors A. L. Srivastav, S. Madhav, A. K. Bhardwaj, and E. Valsami-Jones (Amsterdam, Netherlands: Elsevier), 6, 361–379. doi:10.1016/B978-0-323-91838-1.00027-0
Khan, A. A., and Boddu, S. (2021). in Hybrid membrane process: An emerging and promising technique toward industrial wastewater treatment” in membrane-based hybrid processes for wastewater treatment Editors M. P. Shah, and S. Rodriguez-Couto (Amsterdam, Netherlands: Elsevier), 257–277. doi:10.1016/B978-0-12-823804-2.00002-1
Khan, A. U., Khan, A. N., Abdul, W., Muhammad, I., and Doaa, Z. (2022). Phytoremediation of pollutants from wastewater: A concise review. Open Life Sci. 17 (1), 488–496. doi:10.1515/biol-2022-0056
Kim, N. K., Lin, R., Bhattacharyya, D., van Loosdrecht, M. C., and Lin, Y. (2022). Insight on how biopolymers recovered from aerobic granular wastewater sludge can reduce the flammability of synthetic polymers. Sci. Total Environ. 805, 150434. doi:10.1016/j.scitotenv.2021.150434
Kim, N. K., Mao, N., Lin, R., Bhattacharyya, D., van Loosdrecht, M. C., and Lin, Y. (2020). Flame retardant property of flax fabrics coated by extracellular polymeric substances recovered from both activated sludge and aerobic granular sludge. Water Res. 170, 115344. doi:10.1016/j.watres.2019.115344
Klinglmair, M., Lemming, C., Jensen, L. S., Rechberger, H., Astrup, T. F., and Scheutz, C. (2015). Phosphorus in Denmark: National and regional anthropogenic flows. Resour. Conserv. Recyc. 105, 311–324. doi:10.1016/j.resconrec.2015.09.019
Korenak, J., Helix-Nielsen, C., Bukˇsek, H., and Petrini, C. I. (2019). Efficiency and economic feasibility of forward osmosis in textile wastewater treatment. J. Clean. Prod. 210, 1483–1495. doi:10.1016/j.jclepro.2018.11.130
Kumar, A. K., Sharma, S., Patel, A., Dixit, G., and Shah, E. (2019). Comprehensive evaluation of microalgal based dairy effluent treatment process for clean water generation and other value-added products. Int. J. Phytorem. 21 (6), 519–530. doi:10.1080/15226514.2018.1537248
Kumar, S., and Singh, A. (2015). Biopesticides: Present status and the future prospects. J. Fertil. Pestic. 6 (2), 100–129. doi:10.4172/2471-2728.1000e129
Kumar, S., Smith, S. R., Fowler, G., Velis, C., Kumar, S. J., Arya, S., et al. (2017). Challenges and opportunities associated with waste management in India. R. Soc. Open Sci. 4 (3), 160764–160811. doi:10.1098/rsos.160764
Kurup, G. G., Adhikari, B., and Zisu, B. (2019). Recovery of proteins and lipids from dairy wastewater using food grade sodium lignosulphonate. Water Resour. indust. 22, 100114. doi:10.1016/j.wri.2019.100114
Kusmayadi, A., Lu, P. H., Huang, C. Y., Leong, Y. K., Yen, H. W., and Chang, J. S. (2022). Integrating anaerobic digestion and microalgae cultivation for dairy wastewater treatment and potential biochemicals production from the harvested microalgal biomass. Chemosphere 291, 133057. doi:10.1016/j.chemosphere.2021.133057
Lassouane, F., Ait-Amar, H., Amrani, S., and Rodriguez-Couto, S. (2019). A promising laccase immobilization approach for Bisphenol A removal from aqueous solutions. Bioresour. Technol. 271, 360–367. doi:10.1016/j.biortech.2018.09.129
Lee, K., and Jepson, W. (2021). Environmental impact of desalination: A systematic review of life cycle assessment. Desalination 509, 115066. doi:10.1016/j.desal.2021.115066
Lee, S. Y., and Stuckey, D. C. (2022). Separation and biosynthesis of value-added compounds from food-processing wastewater: Towards sustainable wastewater resource recovery. J. Clean. Prod. 357, 131975. doi:10.1016/j.jclepro.2022.131975
Lewis, J. J., Hollingsworth, J. W., Chartier, R. T., Cooper, E. M., Foster, W. M., Gomes, G. L., et al. (2017). Biogas stoves reduce firewood use, household air pollution, and hospital visits in Odisha, India. Environ. Sci. Technol. 51 (1), 560–569. doi:10.1021/acs.est.6b02466
Li, J., Hao, X., Gan, W., van Loosdrecht, M. C., and Wu, Y. (2021). Recovery of extracellular biopolymers from conventional activated sludge: Potential, characteristics and limitation. Water Res. 205, 117706. doi:10.1016/j.watres.2021.117706
Li, M., Zhao, Y., Guo, Q., Qian, X., and Niu, D. (2008). Bio-hydrogen production from food waste and sewage sludge in the presence of aged refuse excavated from refuse landfill. Renew. Energy. 33 (12), 2573–2579. doi:10.1016/j.renene.2008.02.018
Libardi, N., Soccol, C. R., de Carvalho, J. C., and de Souza Vandenberghe, L. P. (2019). Simultaneous cellulase production using domestic wastewater and bioprocess effluent treatment–A biorefinery approach. Bioresour. Technol. 276, 42–50. doi:10.1016/j.biortech.2018.12.088
Libardi, N., Soccol, C. R., Góes-Neto, A., de Oliveira, J., and de Souza Vandenberghe, L. P. (2017). Domestic wastewater as substrate for cellulase production by Trichoderma harzianum. Process Biochem. 57, 190–199. doi:10.1016/j.procbio.2017.03.006
Lin, Y. M., Nierop, K. G. J., Girbal-neuhauser, E., Adriaanse, M., and van Loosdrecht, M. C. M. (2015). Sustainable polysaccharide-based biomaterial recovered from waste aerobic granular sludge as a surface coating material. Sustain. Mat. Technol. 4, 24–29. doi:10.1016/j.susmat.2015.06.002
Lin, Y. M., Sharma, P. K., and van Loosdrecht, M. C. M. (2013). The chemical and mechanical differences between alginate-like exopolysaccharides isolated from aerobic flocculent sludge and aerobic granular sludge. Water Res. 47 (1), 57–65. doi:10.1016/j.watres.2012.09.017
Lin, Y., Reino, C., Carrera, J., Pérez, J., and van Loosdrecht, M. C. (2018). Glycosylated amyloid-like proteins in the structural extracellular polymers of aerobic granular sludge enriched with ammonium-oxidizing bacteria. Microbiol. Open. 7 (6), e00616. doi:10.1002/mbo3.616
Linares, R. V., Li, Z., Abu-Ghdaib, M., Wei, C. H., Amy, G., and Vrouwenvelder, J. S. (2013). Water harvesting from municipal wastewater via osmotic gradient: An evaluation of process performance. J. Membr. Sci. 447, 50–56. doi:10.1016/j.memsci.2013.07.018
Liu, X., Wu, J., Hou, L. A., and Wang, J. (2019). Removal of co, sr and cs ions from simulated radioactive wastewater by forward osmosis. Chemosphere 232, 87–95. doi:10.1016/j.chemosphere.2019.05.210
Logan, B. E., and Regan, J. M. (2006). Microbial fuel cells—Challenges and applications. Environ. Sci. Technol. 40, 5172–5180. doi:10.1021/es0627592
Lv, J., Liu, Y., Feng, J., Liu, Q., Nan, F., and Xie, S. (2018). Nutrients removal from undiluted cattle farm wastewater by the two-stage process of microalgae-based wastewater treatment. Bioresour. Technol. 264, 311–318. doi:10.1016/j.biortech.2018.05.085
Ma, C., Wen, H., Xing, D., Pei, X., Zhu, J., Ren, N., et al. (2017). Molasses wastewater treatment and lipid production at low temperature conditions by a microalgal mutant Scenedesmus sp. Z-4. Biotechnol. Biofuel. 10, 1–13.
Mahmoud, A. E. D. (2022). in Recent advances of TiO2 nanocomposites for photocatalytic degradation of water contaminants and rechargeable sodium ion batteries” in advances in nanocomposite materials for environmental and energy harvesting applications Editors A. E. Shalan, A. S. Hamdy Makhlouf, and S. Lanceros-Méndez (Cham: Springer International Publishing), 757–770.
Mahmoud, A. E. D., Al-Qahtani, K. M., Alflaij, S. O., Al-Qahtani, S. F., and Alsamhan, F. A. (2021b). Green copper oxide nanoparticles for lead, nickel, and cadmium removal from contaminated water. Sci. Rep. 11 (1), 12547. doi:10.1038/s41598-021-91093-7
Mahmoud, A. E. D., Fawzy, M., and Abdel-Fatah, M. M. A. (2022a). in Technical aspects of nanofiltration for dyes wastewater treatment” in membrane based methods for dye containing wastewater: Recent advances Editors S. S. Muthu, and A. Khadir (Singapore: Springer), 23–35.
Mahmoud, A. E. D., Fawzy, M., and Hosny, M. (2022c). in Feasibility and challenges of biopesticides application” in new and future developments in microbial biotechnology and bioengineering Editors H. B. Singh, and A. Vaishnav (Amsterdam, Netherlands: Elsevier). 83-97.
Mahmoud, A. E. D., Fawzy, M., and Radwan, A. (2016). Optimization of Cadmium (CD2+) removal from aqueous solutions by novel biosorbent. Int. J. Phytorem. 18 (6), 619–625. doi:10.1080/15226514.2015.1086305
Mahmoud, A. E. D., Franke, M., and Braeutigam, P. (2022b). Experimental and modeling of fixed-bed column study for phenolic compounds removal by graphite oxide. J. Water Proc. Eng. 49, 103085. doi:10.1016/j.jwpe.2022.103085
Mahmoud, A. E. D., Franke, M., Stelter, M., and Braeutigam, P. (2020). Mechanochemical versus chemical routes for graphitic precursors and their performance in micropollutants removal in water. Powder Tech. 366, 629–640. doi:10.1016/j.powtec.2020.02.073
Mahmoud, A. E. D., and Kathi, S. (2022). in Assessment of biochar application in decontamination of water and wastewater” in cost effective technologies for solid waste and wastewater treatment Editors S. Kathi, S. Devipriya, and K. Thamaraiselvi (Amsterdam, Netherlands: Elsevier), 69–74.
Mahmoud, A. E. D., Stolle, A., and Stelter, M. (2018). Sustainable synthesis of high-surface-area graphite oxide via dry ball milling. ACS Sust. Chem. Eng. 6 (5), 6358–6369. doi:10.1021/acssuschemeng.8b00147
Mahmoud, A. E. D., Umachandran, K., Sawicka, B., and Mtewa, T. K. (2021a). in Water resources security and management for sustainable communities” in Phytochemistry, the Military and Health Editors A. G. Mtewa, and C. Egbuna (Amsterdam, Netherlands: Elsevier), 509–522.
Malovichko, Y. V., Nizhnikov, A. A., and Antonets, K. S. (2019). Repertoire of the Bacillus thuringiensis virulence factors unrelated to major classes of protein toxins and its role in specificity of host-pathogen interactions. Toxins 11 (6), 347. doi:10.3390/toxins11060347
Marchesi, C. M., Paliga, M., Oro, C. E. D., Dallago, R. M., Zin, G., Di Luccio, M., et al. (2019). Use of membranes for the treatment and reuse of water from the pre-cooling system of chicken carcasses. Environ. Technol. 42, 126–133. doi:10.1080/09593330.2019.1624834
Markandeya, M. D., and Shukla, S. P. (2022). Hazardous consequences of textile mill effluents on soil and their remediation approaches. Clean. Eng. Technol. 7, 100434. doi:10.1016/j.clet.2022.100434
Meena, S. K., Dwivedi, B. S., Meena, M. C., Datta, S. P., Singh, V. K., Mishra, R. P., et al. (2022). Long-term nutrient supply options: Strategies to improve soil phosphorus availability in the rice-wheat system. Sustainability 14, 8629. doi:10.3390/su14148629
Mejias, L., Estrada, M., Barrena, R., and Gea, T. (2020). A novel two-stage aeration strategy for Bacillus thuringiensis biopesticide production from biowaste digestate through solid-state fermentation. Biochem. Eng. J. 161, 107644. doi:10.1016/j.bej.2020.107644
Melia, P. M., Cundy, A. B., Sohi, S. P., Hooda, P. S., and Busquets, R. (2017). Trends in the recovery of phosphorus in bioavailable forms from wastewater. Chemosphere 186, 381–395. doi:10.1016/j.chemosphere.2017.07.089
Michelon, W., da Silva, M. L. B., Matthiensen, A., de Andrade, C. J., de Andrade, L. M., and Soares, H. M. (2021). Amino acids, fatty acids, and peptides in microalgae biomass harvested from phycoremediation of swine wastewaters. Biomass conver. biorefin. 12, 869–880. doi:10.1007/s13399-020-01263-2
Minhas, P. S., Saha, J. K., Dotaniya, M. L., Sarkar, A., and Saha, M. (2022). Wastewater irrigation in India: Current status, impacts and response options. Sci. Total Environ. 808, 152001. doi:10.1016/j.scitotenv.2021.152001
Mishra, B., Tiwari, A., and Mahmoud, A. E. D. (2022). Microalgal potential for sustainable aquaculture applications: Bioremediation, biocontrol, aquafeed. Clean. Tech. Env. Policy 2022. doi:10.1007/s10098-021-02254-1
Mohapatra, D. P., Brar, S. K., Tyagi, R. D., and Surampalli, R. Y. (2010). Physico-chemical pre-treatment and biotransformation of wastewater and wastewater Sludge–Fate of bisphenol A. Chemosphere 78 (8), 923–941. doi:10.1016/j.chemosphere.2009.12.053
Mohareb, A., Thévenon, M. F., Wozniak, E., and Gérardin, P. (2011). Effects of polyvinyl alcohol on leachability and efficacy of boron wood preservatives against fungal decay and termite attack. Wood Sci. Technol. 45 (3), 407–417. doi:10.1007/s00226-010-0344-4
More, T. T., Yadav, J. S. S., Yan, S., Tyagi, R. D., and Surampalli, R. Y. (2014). Extracellular polymeric substances of bacteria and their potential environmental applications. J. Environ. Manage. 144, 1–25. doi:10.1016/j.jenvman.2014.05.010
Motamedi, E., Kavousi, K., Motahar, S. F. S., Ghaffari, M. R., Mamaghani, A. S. A., Salekdeh, G. H., et al. (2021). Efficient removal of various textile dyes from wastewater by novel thermo-halotolerant laccase. Bioresour. Technol. 337, 125468. doi:10.1016/j.biortech.2021.125468
Mu, D., Min, M., Krohn, B., Mullins, Ka., Ruan, R., and Hill, J. (2014). Life cycle environmental impacts of wastewater-based algal biofuels. Environ. Sci. Technol. 48 (19), 11696–11704. doi:10.1021/es5027689
Mu'azu, N. D., Jarrah, N., Zubair, M., and Alagha, O. (2017). Removal of phenolic compounds from water using sewage sludge-based activated carbon adsorption: A review. Int. J. Environ. Res. Pub. Health. 14 (10), 1094. doi:10.3390/ijerph14101094
Nazir, H., Batool, M., Osorio, F. J. B., Isaza-Ruiz, M., Xu, X., Vignarooban, K., et al. (2019). Recent developments in phase change materials for energy storage applications: A review. Int. J. Heat. Mass Transf. 129, 491–523. doi:10.1016/j.ijheatmasstransfer.2018.09.126
Ndao, A., Sellamuthu, B., Kumar, L. R., Tyagi, R. D., and Valero, J. R. (2021). Biopesticide production using Bacillus thuringiensis kurstaki by valorization of starch industry wastewater and effluent from aerobic, anaerobic digestion. Syst. Microbiol. Biomanuf. 1, 494–504. doi:10.1007/s43393-021-00043-x
Nguyen, N. C., Chen, S. S., Yang, H. Y., and Hau, N. T. (2013). Application of forward osmosis on dewatering of high nutrient sludge. Bioresour. Technol. 132, 224–229. doi:10.1016/j.biortech.2013.01.028
Nimkande, V. D., and Bafana, A. (2022). A review on the utility of microbial lipases in wastewater treatment. J. Water Process Eng. 46, 102591. doi:10.1016/j.jwpe.2022.102591
Ochando-Pulido, J. M., Hodaifa, G., Victor-Ortega, M. D., Rodriguez-Vives, S., and Martinez-Ferez, A. (2013). Effective treatment of olive mill effluents from two-phase and three-phase extraction processes by batch membranes in series operation upon threshold conditions. J. Hazard. Mat. 263, 168–176. doi:10.1016/j.jhazmat.2013.03.041
Olaniran, A. O., and Igbinosa, E. O. (2011). Chlorophenols and other related derivatives of environmental concern: Properties, distribution and microbial degradation processes. Chemosphere 83 (10), 1297–1306. doi:10.1016/j.chemosphere.2011.04.009
Owodunni, A. A., Ismail, S., Kurniawan, S. B., Ahmad, A., Imron, M. F., and Abdullah, S. R. S. (2023). A review on revolutionary technique for phosphate removal in wastewater using green coagulant. J. Water Process. Eng. 52, 103573. doi:10.1016/j.jwpe.2023.103573
Paliya, S., Mandpe, A., Kumar, S., and Kumar, M. S. (2019). Enhanced nodulation and higher germination using sludge ash as a carrier for biofertilizer production. J. Environ. Manag. 250, 109523. doi:10.1016/j.jenvman.2019.109523
Pandey, A. (2003). Solid-state fermentation. Biochem. Eng. J. 13 (2-3), 81–84. doi:10.1016/S1369-703X(02)00121-3
Pasciucco, F., Francini, G., Pecorini, I., Baccioli, A., Lombardi, L., and Ferrari, L. (2023). Valorization of biogas from the anaerobic co-treatment of sewage sludge and organic waste: Life cycle assessment and life cycle costing of different recovery strategies. J. Clean. Prod. 136762, 136762. doi:10.1016/j.jclepro.2023.136762
Patel, H., and Vashi, R. T. (2015). Characterization and column adsorptive treatment for COD and color removal using activated neem leaf powder from textile wastewater. J. Urban Environ. Eng. 9 (1), 45–53. doi:10.4090/juee.2015.v9n1.045053
Pena, A. C., Agustini, C. B., Trierweiler, L. F., and Gutterres, M. (2020). Influence of period light on cultivation of microalgae consortium for the treatment of tannery wastewaters from leather finishing stage. J. Clean. Prod. 263, 121618. doi:10.1016/j.jclepro.2020.121618
Pronk, M., De Kreuk, M. K., De Bruin, B., Kamminga, P., Kleerebezem, R. V., and Van Loosdrecht, M. C. M. (2015). Full scale performance of the aerobic granular sludge process for sewage treatment. Water Res. 84, 207–217. doi:10.1016/j.watres.2015.07.011
Pronk, W., Palmquist, H., Biebow, M., and Boller, M. (2006). Nanofiltration for the separation of pharmaceuticals from nutrients in source-separated urine. Water Res. 40 (7), 1405–1412. doi:10.1016/j.watres.2006.01.038
Puyol, D., Batstone, D. J., Hülsen, T., Astals, S., Peces, M., and Krömer, J. O. (2017). Resource recovery from wastewater by biological technologies: Opportunities, challenges, and prospects. Front. Microbiol. 7, 2106. doi:10.3389/fmicb.2016.02106
Quan, C. S., Liu, Q., Tian, W. J., Kikuchi, J., and Fan, S. D. (2005). Biodegradation of an endocrine-disrupting chemical, di-2-ethylhexyl phthalate, by Bacillus subtilis No. 66. Appl. Microbiol. Biotechnol. 66, 702–710. doi:10.1007/s00253-004-1683-6
Quinn, J. C., and Davis, R. (2014). The potentials and challenges of algae based biofuels: A review of the techno-economic, life cycle, and resource assessment modeling. Bioresourc. Technol. 184, 444–452. doi:10.1016/j.biortech.2014.10.075
Quist-Jensen, C. A., Wybrandt, L., Løkkegaard, H., Antonsen, S. B., and Christensen, M. L. (2020). Pilot-scale study for phosphorus recovery by sludge acidification and dewatering. Environ. Technol. 41 (22), 2928–2934. doi:10.1080/09593330.2019.1588385
Quist-Jensen, C. A., Wybrandt, L., Løkkegaard, H., Antonsen, S. B., Jensen, H. C., Nielsen, A. H., et al. (2018). Acidification and recovery of phosphorus from digested and non-digested sludge. Water Res. 146, 307–317. doi:10.1016/j.watres.2018.09.035
Rahman, M. A., Rayhan, M. Y. H., Chowdhury, M. A. H., Mohiuddin, K. M., and Chowdhury, M. A. K. (2018). Phytotoxic effect of synthetic dye effluents on seed germination and early growth of red amaranth. Fundam. Appl. Agric. 3 (2), 480–490. doi:10.5455/faa.299239
Ray, H., Perreault, F., and Boyer, T. H. (2020). Rejection of nitrogen species in real fresh and hydrolyzed human urine by reverse osmosis and nanofiltration. J. Environ. Chem. Eng. 8 (4), 103993. doi:10.1016/j.jece.2020.103993
Rebah, F. B., Prevost, D., and Tyagi, R. D. (2002). Growth of alfalfa in sludge-amended soils and inoculated with rhizobia produced in sludge. J. Environ. Qual. 31 (4), 1339–1348. doi:10.2134/jeq2002.1339
Rebah, F. B., Tyagi, R. D., and Prévost, D. (2001). Acid and alkaline treatments for enhancing the growth of rhizobia in sludge. Canad. J. Microbiol. 47 (6), 467–474. doi:10.1139/w01-033
Rehm, B. H. (2010). Bacterial polymers: Biosynthesis, modifications and applications. Nat. Rev. Microbiol. 8 (8), 578–592. doi:10.1038/nrmicro2354
Ritala, A., Häkkinen, S. T., Toivari, M., and Wiebe, M. G. (2017). Single cell protein—State-of-the-art, industrial landscape and patents 2001–2016. Front. Microbiol. 8, 2009. doi:10.3389/fmicb.2017.02009
Rodriguez, P., Cerda, A., Font, X., Sánchez, A., and Artola, A. (2019). Valorisation of biowaste digestate through solid state fermentation to produce biopesticides from Bacillus thuringiensis. Waste Manag. 93, 63–71. doi:10.1016/j.wasman.2019.05.026
Romero-García, J. M., González-López, C. V., Brindley, C., Fernández-Sevilla, J. M., and Acién-Fernández, F. G. (2022). Simulation and techno-economical evaluation of a microalgal biofertilizer production process. Biology 11 (9), 1359. doi:10.3390/biology11091359
Rosconi, F., Fraguas, L. F., Martínez-Drets, G., and Castro-Sowinski, S. (2005). Purification and characterization of a periplasmic laccase produced by Sinorhizobium meliloti. Enz. Microb. Technol. 36 (5-6), 800–807. doi:10.1016/j.enzmictec.2005.01.003
Rout, P. R., Mohanty, A., Sharma, A., Miglani, M., Liu, D., and Varjani, S. (2022). Micro-and nanoplastics removal mechanisms in wastewater treatment plants: A review. J. Hazard. Mat. Adv. 6, 100070. doi:10.1016/j.hazadv.2022.100070
Sabbahi, R., Hock, V., Azzaoui, K., Saoiabi, S., and Hammouti, B. (2022). A global perspective of entomopathogens as microbial biocontrol agents of insect pests. J. Agric. Food Res. 10, 100376. doi:10.1016/j.jafr.2022.100376
Sachdeva, V., Tyagi, R. D., and Valero, J. R. (2000). Production of biopesticides as a novel method of wastewater sludge utilization/disposal. Water Sci. Technol. 42 (9), 211–216. doi:10.2166/wst.2000.0209
Sangma, C. B. K., and Chalie, R. U. (2023). in Life cycle assessment of wastewater treatment by microalgae” in valorization of microalgal biomass and wastewater treatment Editors S. A. Bandh, and F. A. Malla (Amsterdam, Netherlands: Elsevier), 137–178. doi:10.1016/B978-0-323-91869-5.00008-9
Sathya, K., Nagarajan, K., Carlin Geor Malar, G., Rajalakshmi, S., and Raja Lakshmi, P. (2022). A comprehensive review on comparison among effluent treatment methods and modern methods of treatment of industrial wastewater effluent from different sources. Appl. Water Sci. 12, 70. doi:10.1007/s13201-022-01594-7
Sattari, S. Z., Bouwman, A. F., Giller, K. E., and van Ittersum, M. K. (2012). Residual soil phosphorus as the missing piece in the global phosphorus crisis puzzle. Proc. Natl. Acad. Sci. U. S. A. 109, 6348–6353. doi:10.1073/pnas.1113675109
Scanlon, B. R., Fakhreddine, S., Rateb, A., de Graaf, I., Famiglietti, J., Gleeson, T., et al. (2023). Global water resources and the role of groundwater in a resilient water future. Nat. Rev. Earth Environ. 4, 87–101. doi:10.1038/s43017-022-00378-6
Sharma, R., Mishra, A., Pant, D., and Malaviya, P. (2022). Recent advances in microalgae-based remediation of industrial and non-industrial wastewaters with simultaneous recovery of value-added products. Bioresour. Technol. 344, 126129. Part B. doi:10.1016/j.biortech.2021.126129
Sheng, G. P., Yu, H. Q., and Li, X. Y. (2010). Extracellular polymeric substances (EPS) of microbial aggregates in biological wastewater treatment systems: A review. Biotechnol. Adv. 28, 882–894. doi:10.1016/j.biotechadv.2010.08.001
Shi, X., Tal, G., Hankins, N. P., and Gitis, V. (2014). Fouling and cleaning of ultrafiltration membranes: A review. J. Water Process Engin. 1, 121–138. doi:10.1016/j.jwpe.2014.04.003
Shier, W. T., and Purwono, S. K. (1994). Extraction of single-cell protein from activated sewage sludge: Thermal solubilization of protein. Bioresour. Technol. 49 (2), 157–162. doi:10.1016/0960-8524(94)90079-5
Sichler, T. C., Adam, C., Montag, D., and Barjenbruch, M. (2022). Future nutrient recovery from sewage sludge regarding three different scenarios-German case study. J. Clean. Prod. 333, 130130. doi:10.1016/j.jclepro.2021.130130
Singh, A. K., Singh, G., Gautam, D., and Bedi, M. K. (2013). Optimization of dairy sludge for growth of Rhizobium cells. Biomed. Res. Int. 1-5, 1–5. doi:10.1155/2013/845264
Singh, N., Petrinic, I., H´elix-Nielsen, C., Basu, S., and Balakrishnan, M. (2018). Concentrating molasses distillery wastewater using biomimetic forward osmosis (FO) membranes. Water Res. 130, 271–280. doi:10.1016/j.watres.2017.12.006
Singh, S., Yadav, R., and Singh, A. N. (2022). Applications of waste-to-economy practices in the urban wastewater sector: Implications for ecosystem, human health and environment” in waste management and resource recycling in the developing world Editors P. Singh, P. Verma, R. Singh, and A. Ahamad (Batalhão: ACS), 625–646. doi:10.1016/B978-0-323-90463-6.00010-5
Singhania, R. R., Patel, A. K., Soccol, C. R., and Pandey, A. (2009). Recent advances in solid-state fermentation. Biochem. Eng. J. 44 (1), 13–18. doi:10.1016/j.bej.2008.10.019
Sirasanagandla, S. R., Al-Huseini, I., Sakr, H., Moqadass, M., Das, S., Juliana, N., et al. (2022). Natural products in mitigation of bisphenol A toxicity: Future therapeutic use. Molecules 27, 5384. doi:10.3390/molecules27175384
Solovchenko, A., Zaitsev, P., and Zotov, V. (2021). “Phosphorus biofertilizer from microalgae,” in Biofertilizers (Sawston, Cambridge: Woodhead Publishing), 57–68. doi:10.1016/B978-0-12-821667-5.00022-1
Stamatelatou, K., Antonopoulou, G., Tremouli, A., and Lyberatos, G. (2011). Production of gaseous biofuels and electricity from cheese whey. Industrial Eng. Chem. Res. 50 (2), 639–644. doi:10.1021/ie1002262
Stepnowski, P., Olafsson, G., Helgason, H., and Jastorff, B. (2004). Recovery of astaxanthin from seafood wastewater utilizing fish scales waste. Chemosphere 54 (3), 413–417. doi:10.1016/S0045-6535(03)00718-5
Styles, D., Adams, P., Thelin, G., Vaneeckhaute, C., Chadwick, D., and Withers, P. J. A. (2018). Life cycle assessment of biofertilizer production and use compared with conventional liquid digestate management. Environ. Sci. Technol. 52 (13), 7468–7476. doi:10.1021/acs.est.8b01619
Subramanian, S. B., Yan, S., Tyagi, R. D., and Surampalli, R. Y. (2008). A new, pellet-forming fungal strain: Its isolation, molecular identification, and performance for simultaneous sludge-solids reduction, flocculation, and dewatering. Water Environ. Res. 80 (9), 840–852. doi:10.2175/106143008X304703
Sun, H., Wang, Y., He, Y., Liu, B., Mou, H., Chen, F., et al. (2023). Microalgae-derived pigments for the food industry. Mar. Drugs 21 (2), 82. doi:10.3390/md21020082
Sun, X., Wang, C., Li, Y., Wang, W., and Wei, J. (2015). Treatment of phenolic wastewater by combined UF and NF/RO processes. Desalination 355, 68–74. doi:10.1016/j.desal.2014.10.018
Sun, Y., Bai, S., Wang, X., Ren, N., and You, S. (2023). Prospective life cycle assessment for the electrochemical oxidation wastewater treatment process: From laboratory to industrial scale. Environ. Sci. Technol. 57 (3), 1456–2146. doi:10.1021/acs.est.2c04185
Sutar, V. P., Mali, G. V., Upadhye, V., Singh, V. K., and Sinha, R. P. (2023). Purification of lipase from Pseudomonas aeruginosa VSJK R-9 and its application in combination with the lipolytic consortium for bioremediation of restaurant wastewater. Appl. Biochem. Biotechnol. 195, 1888–1903. doi:10.1007/s12010-022-04230-x
Takeichi, T., and Furukawa, N. (2012). “5.25-Epoxy resins and phenol-formaldehyde resins,” in Polymer science: A comprehensive reference (Amsterdam, Netherlands: Elsevier), 723–751.
Tang, G. L., Huang, J., Sun, Z. J., Tang, Q. Q., Yan, C. H., and Liu, G. Q. (2008). Biohydrogen production from cattle wastewater by enriched anaerobic mixed consortia: Influence of fermentation temperature and pH. J. Biosci. Bioeng. 106 (1), 80–87. doi:10.1263/jbb.106.80
Tarpeh, W. A., Barazesh, J. M., Cath, T. Y., and Nelson, K. L. (2018). Electrochemical stripping to recover nitrogen from source-separated urine. Environ. Sci. Technol. 52, 1453–1460. doi:10.1021/acs.est.7b05488
Theregowda, R. B., González-Mejía, A. M., Ma, X., and Garland, J. (2019). Nutrient recovery from municipal wastewater for sustainable food production systems: An alternative to traditional fertilizers. Environ. Eng. Sci. 36 (7), 833–842. doi:10.1089/ees.2019.0053
Thirugnanasambandham, K., and Sivakumar, V. (2015). Enzymatic catalysis treatment method of meat industry wastewater using lacasse. J. Environ. Health Sci. Engin. 13, 86–88. doi:10.1186/s40201-015-0239-2
Thong, C. C., Teo, D. C. L., and Ng, C. K. (2016). Application of polyvinyl alcohol (PVA) in cement-based composite materials: A review of its engineering properties and microstructure behavior. Constr. Build. Mat. 107, 172–180. doi:10.1016/j.conbuildmat.2015.12.188
Tighiri, H. O., and Erkurt, E. A. (2019). Biotreatment of landfill leachate by microalgae-bacteria consortium in sequencing batch mode and product utilization. Bioresour. Technol. 286, 121396. doi:10.1016/j.biortech.2019.121396
Tilman, D., Cassman, K. G., Matson, P. A., Naylor, R., and Polasky, S. (2002). Agricultural sustainability and intensive production practices. Nature 418, 671–677. doi:10.1038/nature01014
Tiwari, N., and Awasthi, S. (2022). in Sewage treatment and reuse by aerobic and anaerobic digestion and physicochemical post-treatment” in Development in Waste Water Treatment Research and Processes Editors M. P. Shah, S. Rodriguez-Couto, N. Shah, and R. Banerjee (Amsterdam, Netherlands: Elsevier), 409–431. doi:10.1016/B978-0-323-85584-6.00023-6
Tsakona, S., Galanakis, C. M., and Gekas, V. (2012). Hydro-ethanolic mixtures for the recovery of phenols from Mediterranean plant materials. Food Bioprocess Technol. 5, 1384–1393. doi:10.1007/s11947-010-0419-0
Tyagi, R. D., Sikati Foko, V., Barnabe, S., Vidyarthi, A. S., Valero, J. R., and Surampalli, R. Y. (2002). Simultaneous production of biopesticide and alkaline proteases by Bacillus thuringiensis using sewage sludge as a raw material. Water Sci. Technol. 46 (10), 247–254. doi:10.2166/wst.2002.0344
Umamaheswari, J., and Shanthakumar, S. (2021). Paddy-soaked rice mill wastewater treatment by phycoremediation and feasibility study on use of algal biomass as biofertilizer. J. Chem. Technol. Biotechnol. 96 (2), 394–403. doi:10.1002/jctb.6551
Verhagen, J., Kvarnström, E., Nilsson, M., Srikantaiah, V., Ramachandran, S., and Singh, K. (2012). “The business of the Honey-Suckers in Bengaluru (India),” in The potentials and limitations of commercial faecal sludge recycling–an explorative case study (Dhaka, Bangladesh: Asia Regional Sanitation and Hygiene Practitioners Workshop).
Verma, M., Brar, S. K., Tyagi, R. D., Surampalli, R. Y., and Valero, J. R. (2007b). Bench-scale fermentation of Trichoderma viride on wastewater sludge: Rheology, lytic enzymes and biocontrol activity. Enzyme Microb. Technol. 41 (6–7), 764–771. doi:10.1016/j.enzmictec.2007.06.013
Verma, M., Brar, S. K., Tyagi, R. D., Surampalli, R. Y., and Valero, J. R. (2007a). Industrial wastewaters and dewatered sludge: Rich nutrient source for production and formulation of biocontrol agent, Trichoderma viride. World J. Microbiol. Biotechnol. 23, 1695–1703. doi:10.1007/s11274-007-9417-4
Verma, N., Chundawat, T. S., Chandra, H., and Vaya, D. (2023). An efficient time reductive photocatalytic degradation of carcinogenic dyes by TiO2-GO nanocomposite. Mat. Res. Bullet. 158, 112043. doi:10.1016/j.materresbull.2022.112043
Ververi, M., and Goula, A. M. (2019). Pomegranate peel and orange juice by-product as new biosorbents of phenolic compounds from olive mill wastewaters. Chem. Engin. Process Process Intensif. 138, 86–96. doi:10.1016/j.cep.2019.03.010
Vethathirri, R. S., Santillan, E., and Wuertz, S. (2021). Microbial community-based protein production from wastewater for animal feed applications. Bioresour. Technol. 341, 125723. doi:10.1016/j.biortech.2021.125723
Villalobos-Delgado, F. J., di Bitonto, L., Reynel-Avila, H. E., Mendoza-Castillo, D. I., Bonilla-Petriciolet, A., and Pastore, C. (2021). Efficient and sustainable recovery of lipids from sewage sludge using ethyl esters of volatile fatty acids as sustainable extracting solvent. Fuel 295, 120630. doi:10.1016/j.fuel.2021.120630
Villaró, S., Ciardi, M., Morillas-España, A., Sánchez-Zurano, A., Acién-Fernández, G., and Lafarga, T. (2021). Microalgae derived astaxanthin: Research and consumer trends and industrial use as food. Foods 10 (10), 2303. doi:10.3390/foods10102303
Villegas, L. G. C., Mashhadi, N., Chen, M., Mukherjee, D., Taylor, K. E., and Biswas, N. (2016). A short review of techniques for phenol removal from wastewater. Curr. Pollut. Rep. 2, 157–167. doi:10.1007/s40726-016-0035-3
Wang, P., Tyndall, S., Rahman, T., Roy, P., Jahromi, H., Adhikari, S., et al. (2022). Sorption and recovery of phenolic compounds from aqueous phase of sewage sludge hydrothermal liquefaction using bio-char. Chemosphere 287, 131934. doi:10.1016/j.chemosphere.2021.131934
Wang, Y., Mou, J., Liu, X., and Chang, J. (2021). Phosphorus recovery from wastewater by struvite in response to initial nutrients concentration and nitrogen/phosphorus molar ratio. Sci. Total Environ. 789, 147970. doi:10.1016/j.scitotenv.2021.147970
Wei, L., Wang, S., Zhang, F., Fan, Y., Liao, Y., Liao, B., et al. (2022). Efficient degradation of molasses wastewater from sugar mill by lipase via addition reaction. LWT- Food Sci. Technol. 161, 113366. doi:10.1016/j.lwt.2022.113366
Wu, Z., Zou, S., Zhang, B., Wang, L., and He, Z. (2018). Forward osmosis promoted in-situ formation of struvite with simultaneous water recovery from digested swine wastewater, Chem. Eng. J. 342 274–280. doi:10.1016/j.cej.2018.02.082
Xiong, J. Q., Kabra, A. N., and Salama, E. S. (2023). in Microalgae-based biotechnologies for treatment of textile wastewater” in current developments in bioengineering and biotechnology Editors S. P. Govindwar, M. B. Kurade, B. H. Jeon, and A. Pandey (Amsterdam, Netherlands: Elsevier), 457–471.
Xu, W., Chen, Q., and Ge, Q. (2017). Recent advances in forward osmosis (FO) membrane: Chemical modifications on membranes for FO processes. Desalination 419, 101–116. doi:10.1016/j.desal.2017.06.007
Xue, T., and Huang, X. (2007). Releasing characteristics of phosphorus and other substances during thermal treatment of excess sludge. J. Environ. Sci. 19 (10), 1153–1158. doi:10.1016/s1001-0742(07)60188-0
Yadav, D., Ranjan, B., Mchunu, N., Le Roes-Hill, M., and Kudanga, T. (2021). Enzymatic treatment of phenolic pollutants by a small laccase immobilized on APTES-functionalised magnetic nanoparticles. Biotech 11, 302–312. doi:10.1007/s13205-021-02854-0
Yadav, R., Singh, S., Kaur, A., Tokas, D., Kathi, S., and Singh, A. N. (2023). “Harnessing energy from animal waste: A win–win approach for India,” in Manure technology and sustainable development (Singapore: Springer Nature Singapore), 283–304.
Yadav, R., Singh, S., and Singh, A. N. (2022). Biopesticides: Current status and future prospects. Proc. Int. Acad. Ecol. Environ. Sci. 12, 211–233.
Yao, T., Li, H., Ren, Y., Feng, M., Hu, Y., Yan, H., et al. (2022). Extraction and recovery of phenolic compounds from aqueous solution by thermo-separating magnetic ionic liquid aqueous two-phase system. Sep. Purif. Technol. 282, 120034. doi:10.1016/j.seppur.2021.120034
Yezza, A., Tyagi, R. D., Valero, J. R., and Surampalli, R. Y. (2005). Wastewater sludge pre-treatment for enhancing entomotoxicity produced by Bacillus thuringiensis var. kurstaki. World J. Microbiol. Biotechnol. 21 (6), 1165–1174. doi:10.1007/s11274-005-0700-y
You, S., Lu, J., Wang, C. Y., and Tang, X. (2017). Rejection of heavy metals in acidic wastewater by a novel thin-film inorganic forward osmosis membrane. Chem. Eng. J. 320, 532–538. doi:10.1016/j.cej.2017.03.064
Yu, B., Luo, J., Xie, H., Yang, H., Chen, S., Liu, J., et al. (2021). Species, fractions, and characterization of phosphorus in sewage sludge: A critical review from the perspective of recovery. Sci. Total Environ. 786, 147437. doi:10.1016/j.scitotenv.2021.147437
Yusree, F. I. F. M., Peter, A. P., Zulkifli, N. A. B., Zuhair, M., Show, P. L., Mokhtar, M. N., et al. (2022). Towards green recovery of β-amylase from slurry of sweet potato (Ipomoea batatas) of Vit A to variety via liquid biphasic system. Sustain. Chem. Pharm. 25, 100579. doi:10.1016/j.scp.2021.100579
Zagklis, D. P., Vavouraki, A. I., Kornaros, M. E., and Paraskeva, C. A. (2015). Purification of olive mill wastewater phenols through membrane filtration and resin adsorption/desorption. J. Hazard. Mat. 285, 69–76. doi:10.1016/j.jhazmat.2014.11.038
Zhang, W., Qiu, L., Gong, A., Cao, Y., and Wang, B. (2013). Solid-state fermentation of kitchen waste for production of Bacillus thuringiensis-based bio-pesticide. Bio Resour. 8 (1), 1124–1135. doi:10.15376/biores.8.1.1124-1135
Zheng, H., Liu, M., Lu, Q., Wu, X., Ma, Y., Cheng, Y., et al. (2018). Balancing carbon/nitrogen ratio to improve nutrients removal and algal biomass production in piggery and brewery wastewaters. Bioresour. Technol. 249, 479–486. doi:10.1016/j.biortech.2017.10.057
Zhu, F., Wu, X., Zhao, L., Liu, X., Qi, J., Wang, X., et al. (2017). Lipid profiling in sewage sludge. Water Res. 116, 149–158. doi:10.1016/j.watres.2017.03.032
Keywords: resource recovery, SDG 6, value-added products, sustainable development goal, circular economy, waste valorization
Citation: Kathi S, Singh S, Yadav R, Singh AN and Mahmoud AED (2023) Wastewater and sludge valorisation: a novel approach for treatment and resource recovery to achieve circular economy concept. Front. Chem. Eng. 5:1129783. doi: 10.3389/fceng.2023.1129783
Received: 22 December 2022; Accepted: 05 April 2023;
Published: 27 April 2023.
Edited by:
Surindra Suthar, Doon University, IndiaReviewed by:
Daniel Pinto Fernandes, Federal University of Alagoas, BrazilVinay Kumar Tyagi, National Institute of Hydrology, India
Copyright © 2023 Kathi, Singh, Yadav, Singh and Mahmoud. This is an open-access article distributed under the terms of the Creative Commons Attribution License (CC BY). The use, distribution or reproduction in other forums is permitted, provided the original author(s) and the copyright owner(s) are credited and that the original publication in this journal is cited, in accordance with accepted academic practice. No use, distribution or reproduction is permitted which does not comply with these terms.
*Correspondence: Anand Narain Singh, dranand1212@gmail.com