- 1Research Centre for Sustainable Technologies, Faculty of Engineering, Computing and Science, Swinburne University of Technology, Kuching, Malaysia
- 2College of Safety Engineering, Chongqing University of Science and Technology, Chongqing, China
The reactive-extractive distillation (RED) has the advantages of combining the beneficial features of both reaction and separation processes. Relative to the conventional reactive or extractive distillation-based processes, the RED is also less energy intensive as it relies on the reaction heat produced for the subsequent separation process. Therefore, the application of RED for ternary azeotropic separation has become the subject of popular interest, as evident by the increasing number of publications since 2020. This review paper is the first that puts together, in a chronological manner, all the existing studies on the RED to date, starting from the initial three column reactive-extractive distillation (TCRED) to the most recent dividing wall reactive-extractive distillation (DW-RED), for ternary azeotropic separation, so that the rapid progress in this area is summarised and any existing gaps in these studies become easier to identify for future work. The last part of this review provides three potential areas for future work, i.e. analysis of the sustainability of RED, combination of different process intensification strategies to improve the energy efficiency and separation performance, and further exploration on the control studies for the RED.
Introduction
The reactive distillation (RD) was first conceptually developed in the early 1920s, with the first industrial application recorded in the early 1980 (Kiss et al., 2018), that features both physical separation and chemical reaction in one−unit operation, instead of carrying out the reaction−separation individually in series as is the case of the traditional reaction−distillation process (Gao et al., 2018). It also has the advantage of overcoming the equilibrium limitations of many reactions such as transesterification or dehydration, which translates to a higher reactant conversion and an increase in the product selectivity and product yield (Kiss et al., 2018). Other than that, another important feature of the RD is its ability to provide the necessary reaction heat for the simultaneous separation process. Today, RD has been widely applied for various industrial applications such as for the production of methyl tert butyl ether (Almeida-Rivera et al., 2004), tert amyl methyl ether (Gao et al., 2014), and dimethyl ether (Kiss and Suszwalak, 2012). Despite its numerous applications, however, it is important to note that RD is an energy-intensive process and hence, much efforts have been devoted to reduce the energy consumption such as applying the process intensification (PI) techniques to the existing RD process.
The thermally coupled (Contreras-Zarazúa et al., 2017) and dividing wall (Kaur and Sangal, 2017; Santaella et al., 2017), side-stream (Thotla and Mahajani, 2009; Yu et al., 2017), and vapour recompression heat-pump (VRHP) (Yang et al., 2019) are some of the traditional PI techniques that have been applied to the RD to reduce the energy consumption. Other than that, another feasible PI technique, which has rapidly emerged in the last 2 years is the hybrid reactive-extractive distillation (RED), as evident by the increasing number of studies. The mechanism of the RED is analogous to the RD, except for the introduction of an additional solvent to the same column to alter relative volatility for enhancing the azeotropic mixtures separation.
Our literature search using SCOPUS database with phrases containing: Reactive-extractive AND distillation AND separation AND ternary AND azeotropic AND mixture, in the title, abstract, and keywords, gave back 5 journal publications that worked on the design and 1 journal publication that worked on the control of RED for separation of ternary azeotropic mixture. Here, note that there may be publication on RED for the separation of ternary azeotropic mixture but without those words used in the above search, such as the work of Wang et al. (2021). Therefore, those papers that were not included above, were added manually into this review, to the best of authors knowledge. During our literature search, we realised that although there are already a handful number of publications that worked on the RED for the separation of ternary azeotropic mixture, there is no review paper available that presents the essential knowledge and insights in these studies. Note that most of these studies were investigated concurrently by different research groups at different locations, which resulted in the lack of integration, synchronisation, and interaction between these different RED studies. Therefore, this review paper aims to put together all these studies so that the rapid progress in the RED is summarised and the existing gaps in these studies can be identified for future work.
The process flow diagram for reactive-extractive distillation
In this section, we briefly describe the process flow diagram for the RED to provide our readers with a better understanding of the process, while a more detailed description is made available in our previous review paper for interested readers (Kong et al., 2022). In general, the application of RED for separation of ternary azeotropic mixture can conceptually be categorised into two different configurations. The first configuration is a three-column reactive-extractive distillation (TCRED) where the reactive and extractive distillation processes take place consecutively in different columns (Figure 1A). The first column is usually the reactive distillation column (RDC) where the hydration reaction takes place between the reactant (e.g. ethylene oxide (EO)) and water to form a product (e.g. ethylene glycol (EG)), according to Eq. 1, where xi refers to the mole fraction of the respective component. The remainder mixture, which is usually a binary azeotropic mixture (A + B), is obtained from the distillate of the RDC and subsequently channelled to the extractive distillation (ED) section for azeotropic separation. In the ED section, a foreign solvent (S) is introduced to the extractive distillation column (EDC) (i.e. second column) to facilitate the azeotropic separation and high purity product A can be obtained from the distillate of EDC. The remaining mixture that contains product B and solvent (S) is obtained from the bottom of EDC and is directed to the solvent recovery column (SRC) for solvent regeneration. The high purity product B is obtained from the distillate while the regenerated solvent (S) obtained from the bottom of the SRC is cooled and recycled back to the EDC (i.e. 2nd column). Here, the solvent (S) can either be a foreign solvent obtained through external injection or it can alternatively be obtained internally from the product formed (i.e. EG) from the earlier RD section (i.e. 1st column).
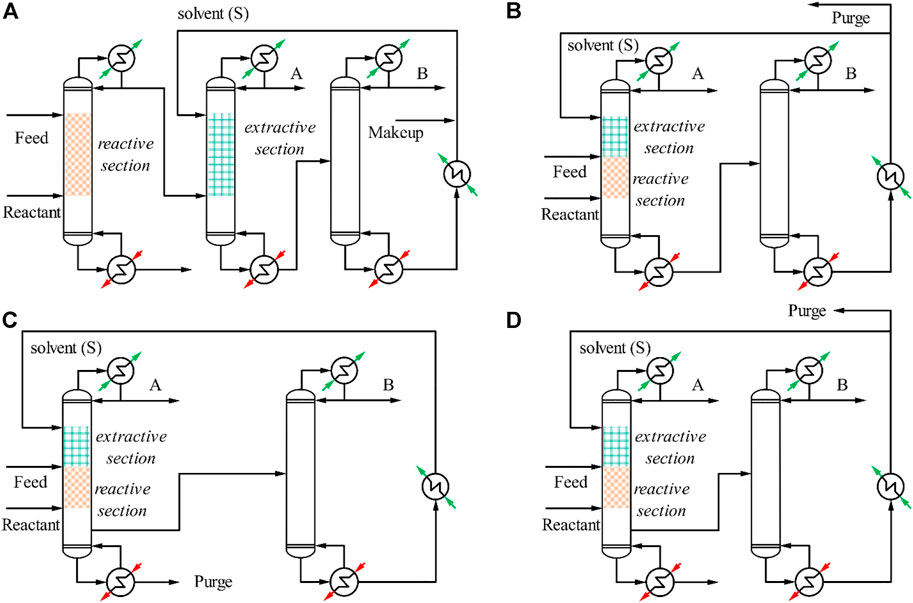
FIGURE 1. Process flow diagram for (A) TCRED, (B) DCRED, (C) SS-DCRED without purge, and (D) SS-DCRED with purge.
The second configuration is the double-column reactive-extractive distillation (DCRED) where both reactive and extractive distillation processes are integrated into a single column (Figure 1B). In the DCRED, the fresh feed, reactant, and solvent enter the reactive-extractive distillation column (REDC) where water reacts with EO to form EG according to Eq. 1, which is subsequently used as solvent to facilitate the separation between the azeotropic mixture (A and B) within the same column. The high purity product A is removed as a distillate from the REDC while the remainder mixture containing mainly EG solvent and product B is channelled to the SRC, where high purity product B is obtained as distillate. The recovered EG obtained from the bottom of SRC is cooled before recycling back to the REDC. Here, excessive amount of solvent is purged out of the system.
Current status
Today, our literature search has revealed that the RED has been extensively used for the separation of ternary azeotropic mixture containing water such as ethanol/ethyl acetate/water, tetrahydrofuran (THF)/ethanol/water, tert-butanol (TBA)/ethanol/water, and acetonitrile/isopropanol/water. Figure 2 graphically summarised all the existing studies done for the separation and recovery of ternary azeotropic mixture using RED.
Among the different studies illustrated in Figure 2, the application of RED for ternary azeotropic separation was first initiated by the Shen’s research group in 2020 where they employed the TCRED (Figure 1A) for the separation of THF/ethanol/water (Su et al., 2020; Yang et al., 2020). In comparison to the pressure-swing distillation, the RED reduces the total annual cost (TAC) and CO2 emission by 63 and 84%, respectively. At the end of their study, they recommended exploring the application of RED for separation of some other ternary azeotropic mixture such as THF/methanol/water, acetonitrile/methanol/water, and ethyl acetate/ethanol/water. Among the mixtures recommended, Wang et al. (2021) worked on the separation of ethyl acetate/ethanol/water using TCRED with two different solvents (Figure 1A), i.e. dimethyl sulfoxide (DMSO) and EG, while no studies have yet worked on THF/methanol/water and acetonitrile/methanol/water. Among the two different TCRED configurations, Wang et al. (2021) reported that using DMSO provides the most significant reduction in TAC and CO2 emission by approximately 32 and 50%, respectively, in comparison to the TCED. Other than that, Wang et al. (2021) proposed a DCRED (Figure 1B) using EG as solvent, which is obtained within the separation process itself instead of relying on a foreign solvent (e.g. DMSO) as in the previous TCRED (Figure 1A). Wang et al. (2021) demonstrated that using DCRED provides lower TAC and CO2 emission with respect to the earlier TCRED using DMSO by about 35 and 20%, respectively. Likewise, the TAC and CO2 emission are lower than the conventional TCED by about 56 and 100%, respectively. Then. Zhang et al. (2021) extended the application of DCRED for the separation of THF/ethanol/water, TBA/ethanol/water, and acetonitrile/isopropanol/water. The performance of the DCRED are compared against the TCRED and in all three cases, it was concluded that the DCRED provides better economic performance by about 32–64%.
Other than extending the application of RED to other ternary azeotropic mixture, another recommendation given by Shen’s group (Su et al., 2020; Yang et al., 2020) was to explore on the integration of PI techniques such as thermally coupled, dividing wall, side-stream, and vapour-recompression to the RED for further energy saving. Following this, the same group took the initiative to analyse the benefits of integrating the dividing wall to the DCRED to become a dividing wall reactive-extractive distillation (DW-RED) for the separation of TBA/ethanol/water (Yang et al., 2022b). In comparison to the DCRED and TCED, the DW-RED provides a lower total capital cost by about 45 and 6%, respectively, owing to the fact that the DW-RED only required one distillation column. Nonetheless, the total operational cost for the DW-RED was the highest and this was attributed to the higher steam grade requirement by the system. Altogether, the DW-RED is inferior to the DCRED owing to the higher TAC, although both the DCRED-based processes provide significant improvement in TAC and CO2 emission relative to the TCED. The integrated configuration (i.e. DW-RED) was later employed by Sun’s group for the separation of benzene/isopropanol/water and ethyl acetate/ethanol/water (Liu et al., 2022; Yan et al., 2022). A similar conclusion was drawn by Yan et al. (2022) for the separation of benzene/isopropanol/water where the DCRED provides the lowest TAC and CO2 emission relative to the DW-RED and TCED. Contrary to the findings from the work of Yang et al. (2022b) and Yan et al. (2022), Liu et al. (2022) showed that the DW-RED with pre-heated feed provides 5% improvement for both TAC and CO2 emission relative to the DCRED for the separation of ethyl acetate/ethanol/water. In addition, they also attempted to integrate the Organic Rankine Cycle (ORC) into the DW-RED, as part of the energy conservation strategy to improve the separation process performance, and it was demonstrated that the application of ORC provides better economic and environmental performance relative to DCRED (Liu et al., 2022).
Future work
Several recommendations stemming from the existing studies presented above are as follows:
Sustainability of RED
From Section 3, it was observed that most of the existing studies on RED tend to prioritise the economic factor with only two studies accounted for some other factors such as environmental and thermodynamic efficiency (Liu et al., 2022; Yan et al., 2022). Therefore, there is no overview of the role played by the other sustainability indicators such as the inherent safety. For instance, all the RED presented in Section 3 employed the same reaction scheme (Eq. 1) that consumed EO as reactant. It is worth noting that EO is a highly flammable substance, which possesses certain risk to the process plant. This can be regarded as one of the limitations of the RED and therefore, future work should further evaluate the sustainability of RED, especially for industrial application.
Potential process intensification
Upon meticulous analysis on the trend for existing studies, it becomes apparent that all the existing studies today are moving towards sustainable separation by means of reducing the energy, environmental, and economic impacts through combination of different PI techniques such the DCRED (i.e. combination between reaction and separation in one column) (Su et al., 2020; Wang et al., 2021; Zhang et al., 2021), DW-RED (i.e. combination of DCRED with dividing wall) (Yang et al., 2022b; Liu et al., 2022; Yan et al., 2022), and integrating side-stream extractive distillation with VRHP (Yang et al., 2022a). Most of the recent studies today always demonstrate lower energy consumption, TAC, and CO2 emission as compared to the earlier processes (i.e. the work back in 2017 or 2018). Based on such trend, there are still a handful number of other possible combinations, and our opinion is that future studies can continue to explore these different combinations for further energy saving. One example is the application of side-stream to the DCRED to become a side-stream double column reactive-extractive distillation (SS-DCRED) (Figures 1C,D). The side-stream configuration is a promising intensification technique used for reducing the remixing effect in the conventional ED, which substantially reduces the energy consumption (Shi et al., 2020; Lyu et al., 2021). Instead of having an interconnected vapour and liquid stream as in the case of thermally coupled or dividing wall configuration, a liquid side-stream is fed directly from one column to another in the case of side-stream configuration. Additionally, the side-stream configuration does not lose any control degree of freedom that may potentially deteriorate the control performance as in the case of thermally coupled or dividing wall configuration. Other than that, future studies can also consider integrating the VRHP to the DW-RED or the SS-DCRED for further maximising the energy recovery.
Control performance of reactive-extractive distillation
Although the steady state design for the RED first appeared in 2020, it was not until recently (i.e. 2022) that Chien’s group analysed the control performance of the RED by using only the temperature controller to handle various feed composition and throughput disturbances (Wu and Chien, 2022). Here, it is worth noting that the obstacle for developing a robust control structure for the RED lies in the complicated interaction between the reaction and the vapour-liquid equilibrium in the REDC. Further, the simultaneous control of the EO flowrate to avoid this highly flammable substance from escaping to the environment while maintaining the water impurity in the products stream is a challenging task. In the work of Wu and Chien (2022), several different control strategies were investigated, i.e. dual temperature control, triple-point temperature control, temperature-invariant control, and temperature-difference control. Overall, it was demonstrated that the usage of temperature-difference controller enables the process to stabilise at a new steady state values without sacrificing the product purities significantly under feed composition disturbance. The same control strategy can also handle the throughput disturbances, simply by manipulating the setpoints of the quality control loops based on the throughput change. Nonetheless, such approach (i.e. changing the setpoint) is only efficient when the system is subjected to known throughput disturbance and further test is recommended on the control performance under random throughput disturbance. For future work, we recommend investigating the control performance of the different energy intensified RED systems such as the DW-RED and SS-DCRED. We also recommend future studies to conduct a thorough comparison between the steady state and dynamic performances of the TCED and DCRED.
Conclusion
In a nutshell, we have chronologically summarised all the existing studies using RED for the separation of ternary azeotropic mixtures. The performance of the RED was compared against the conventional distillation-based processes and in all cases, the RED was proven to provide significant improvement in energy consumption, TAC, and CO2 emission, owing to the fact that the RED relies on the reaction heat produced from the reaction for the subsequent separation process. Despite using less energy, the RED consumed EO (i.e. a highly flammable substance) as reactant and thus, may requires a stringent safety measure for the entire process. Following this, we recommend future studies to further evaluate the sustainability aspects of using RED for ternary azeotropic separation. Moreover, future studies can also explore the possibility of combining different PI strategies such as SS-DCRED for maximising the energy recovery. Lastly, future work should also work on the dynamic simulation for the RED, especially for the energy-intensified RED, to ensure that there is no trade-off between the steady state and dynamic performances.
Author contributions
AY: Conceptualization, Investigation, Methodology, Roles/Writing - original draft, Project administration; ZK: Writing—review editing; JS: Writing—review editing.
Acknowledgments
ZK gratefully acknowledged the support from Swinburne University of Technology Sarawak Campus. This work is also funded by the Research Foundation of Chongqing University of Science and Technology (No. ckrc2021081).
Conflict of interest
The authors declare that the research was conducted in the absence of any commercial or financial relationships that could be construed as a potential conflict of interest.
Publisher’s note
All claims expressed in this article are solely those of the authors and do not necessarily represent those of their affiliated organizations, or those of the publisher, the editors and the reviewers. Any product that may be evaluated in this article, or claim that may be made by its manufacturer, is not guaranteed or endorsed by the publisher.
References
Almeida-Rivera, C. P., Swinkels, P. L. J., and Grievink, J. (2004). Designing reactive distillation processes: present and future. Comput. Chem. Eng. 28, 1997–2020. doi:10.1016/j.compchemeng.2004.03.014
Contreras-Zarazúa, G., Vázquez-Castillo, J. A., Ramírez-Márquez, C., Pontis, G. A., Segovia-Hernández, J. G., and Alcántara-Ávila, J. R. (2017). Comparison of intensified reactive distillation configurations for the synthesis of diphenyl carbonate. Energy 135, 637–649. doi:10.1016/j.energy.2017.06.156
Gao, X., Wang, F., Li, H., and Li, X. (2014). Heat-integrated reactive distillation process for TAME synthesis. Sep. Purif. Technol. 132, 468–478. doi:10.1016/j.seppur.2014.06.003
Gao, X., Zhao, Y., Li, H., and Li, X. (2018). Review of basic and application investigation of reactive distillation technology for process intensification. CIESC J. 69, 218–238.
Kaur, J., and Sangal, V. K. (2017). Reducing energy requirements for ETBE synthesis using reactive dividing wall distillation column. Energy 126, 671–676. doi:10.1016/j.energy.2017.03.072
Kiss, A. A., Jobson, M., and Gao, X. (2018). Reactive distillation: Stepping up to the next level of process intensification. Ind. Eng. Chem. Res. 58, 5909–5918. doi:10.1021/acs.iecr.8b05450
Kiss, A. A., and Suszwalak, D. J. P. C. (2012). Innovative dimethyl ether synthesis in a reactive dividing-wall column. Comput. Chem. Eng. 38, 74–81. doi:10.1016/j.compchemeng.2011.11.012
Kong, Z.-Y., Lee, H.-Y., and Sunarso, J. (2022). The evolution of process design and control for ternary azeotropic separation: recent advances in distillation and future directions. Sep. Purif. Technol. 284, 120292. doi:10.1016/j.seppur.2021.120292
Liu, J., Yan, J., Liu, W., Kong, J., Wu, Y., Li, X., et al. (2022). Design and multi-objective optimization of reactive-extractive dividing wall column with organic rankine cycles considering safety. Sep. Purif. Technol. 287, 120512. doi:10.1016/j.seppur.2022.120512
Lyu, H., Li, S., Cui, C., Yu, X., and Sun, J. (2021). Superstructure modeling and stochastic optimization of side-stream extractive distillation processes for the industrial separation of benzene/cyclohexane/cyclohexene. Sep. Purif. Technol. 257, 117907. doi:10.1016/j.seppur.2020.117907
Santaella, M. A., Jiménez, L. E., Orjuela, A., and Segovia-Hernández, J. G. (2017). Design of thermally coupled reactive distillation schemes for triethyl citrate production using economic and controllability criteria. Chem. Eng. J. 328, 368–381. doi:10.1016/j.cej.2017.07.015
Shi, T., Chun, W., Yang, A., Su, Y., Jin, S., Ren, J., et al. (2020). Optimization and control of energy saving side-stream extractive distillation with heat integration for separating ethyl acetate-ethanol azeotrope. Chem. Eng. Sci. 215, 115373. doi:10.1016/j.ces.2019.115373
Su, Y., Yang, A., Jin, S., Shen, W., Cui, P., and Ren, J. (2020). Investigation on ternary system tetrahydrofuran/ethanol/water with three azeotropes separation via the combination of reactive and extractive distillation. J. Clean. Prod. 273, 123145. doi:10.1016/j.jclepro.2020.123145
Thotla, S., and Mahajani, S. (2009). Reactive distillation with side draw. Chem. Eng. Process. Process Intensif. 48, 927–937. doi:10.1016/j.cep.2008.12.007
Wang, C., Zhuang, Y., Liu, L., Zhang, L., and Du, J. (2021). Design and comparison of energy-saving double column and triple column reactive-extractive hybrid distillation processes for ternary multi-azeotrope dehydration. Sep. Purif. Technol. 259, 118211. doi:10.1016/j.seppur.2020.118211
Wu, T.-W., and Chien, I. L. (2022). Novel control strategy of intensified hybrid reactive-extractive distillation process for the separation of water-containing ternary mixtures. Sep. Purif. Technol. 294, 121159. doi:10.1016/j.seppur.2022.121159
Yan, J., Liu, J., Ren, J., Wu, Y., Li, X., Sun, T., et al. (2022). Design and multi-objective optimization of hybrid reactive-extractive distillation process for separating wastewater containing benzene and isopropanol. Sep. Purif. Technol. 290, 120915. doi:10.1016/j.seppur.2022.120915
Yang, A., Kong, Z.-Y., Sunarso, J., Su, Y., Wang, Q., and Zhu, S. (2022a). Insights on sustainable separation of ternary azeotropic mixture tetrahydrofuran/ethyl acetate/water using hybrid vapor recompression assisted side-stream extractive distillation. Sep. Purif. Technol. 290, 120884. doi:10.1016/j.seppur.2022.120884
Yang, A., Su, Y., Sun, S. R., Shen, W. F., Bai, M. N., and Ren, J. Z. (2022b). Towards sustainable separation of the ternary azeotropic mixture based on the intensified reactive-extractive distillation configurations and multi-objective particle swarm optimization. J. Clean. Prod. 332, 130116. doi:10.1016/j.jclepro.2021.130116
Yang, A., Su, Y., Teng, L., Jin, S., Zhou, T., and Shen, W. (2020). Investigation of energy-efficient and sustainable reactive/pressure-swing distillation processes to recover tetrahydrofuran and ethanol from the industrial effluent. Sep. Purif. Technol. 250, 117210. doi:10.1016/j.seppur.2020.117210
Yang, A., Sun, S., Eslamimanesh, A., Wei, S. a., and Shen, W. (2019). Energy-saving investigation for diethyl carbonate synthesis through the reactive dividing wall column combining the vapor recompression heat pump or different pressure thermally coupled technique. Energy 172, 320–332. doi:10.1016/j.energy.2019.01.126
Yu, N., Li, L., Chen, M., Wang, J., Liu, D., and Sun, L. (2017). Novel reactive distillation process with two side streams for dimethyl adipate production. Chem. Eng. Process. Process Intensif. 118, 9–18. doi:10.1016/j.cep.2017.04.010
Keywords: reactive-extractive distillation, sustainable separation, process intensification, ternary azeotropic mixture, resource recovery
Citation: Kong ZY, Sunarso J and Yang A (2022) Recent progress on hybrid reactive-extractive distillation for azeotropic separation: A short review. Front. Chem. Eng. 4:986411. doi: 10.3389/fceng.2022.986411
Received: 05 July 2022; Accepted: 02 August 2022;
Published: 23 August 2022.
Edited by:
Juan Gabriel Segovia Hernandez, University of Guanajuato, MexicoReviewed by:
Jun Gao, Shandong University of Science and Technology, ChinaCopyright © 2022 Kong, Sunarso and Yang. This is an open-access article distributed under the terms of the Creative Commons Attribution License (CC BY). The use, distribution or reproduction in other forums is permitted, provided the original author(s) and the copyright owner(s) are credited and that the original publication in this journal is cited, in accordance with accepted academic practice. No use, distribution or reproduction is permitted which does not comply with these terms.
*Correspondence: Ao Yang, YW95YW5nMjAyMUBjcXVzdC5lZHUuY24=