- 1Process and Systems Engineering Center (PROSYS), Department of Chemical and Biochemical Engineering, Technical University of Denmark, Lyngby, Denmark
- 2Faculty of Education and Social Work, The University of Auckland, Auckland, New Zealand
- 3Department of Chemical and Materials Engineering, Faculty of Engineering, The University of Auckland, Auckland, New Zealand
The integration of online platforms in (bio)chemical engineering education has increasingly become a matter of fact at all educational levels. Examples such as virtual laboratories in tertiary education have shown their benefits, such as the decreased cost and resources needed as well as providing a safer environment for practical experimentation. However, students or stakeholders are not usually involved in developing the virtual laboratory, even if, when given the opportunity, they can provide valuable feedback for improvement and acquire ownership over the platform. Hence, when proposing a novel educational process virtual laboratory that targets teaching bioprocess modeling to undergraduate students, the best approach is to involve the students in the development as its future users. To this end, in this work, four co-participatory design experiences are presented that show the journey from a paper prototype to an online educational virtual laboratory (www.biovl.com). Qualitative and quantitative data have been collected in two different universities through surveys, semi-structured interviews, and informal conversations among the students and the developer. The students’ perspectives were found to provide valuable feedback about the platform’s content, usability, and functionality. For example, interest in adding bioprocess-related coding activities, or suggesting to change the platform name, were opinions collected and carefully considered. These suggestions can be easily integrated since the software is at the early stages of the virtual laboratory prototype. Although the usability and functionality features of the platform are under continuous advancement, this work’s findings show that the students are interested in contributing to the virtual laboratory’s development. Therefore, it opens the door to modifications and improvements, which are strongly based on the users’/students’ perceptions and perspectives as the virtual laboratory’s co-designers. Although the primary target of the BioVL prototype is to teach bioprocessing modelling, several advanced tools such as virtual and augmented reality (VR and AR) are being considered to be included at a future stage. Furthermore, the educational strategy proposed in this work can be replicated by those who want to develop other virtual laboratories.
1 Introduction
1.1 Virtual laboratories in engineering education
Virtual laboratories (VLs) have increasingly become a common educational tool integrated into the curriculum in this new era marked by technological advances and flexible education (Heradio et al., 2016; Potkonjak et al., 2016). In addition, although VLs have been considered a better option for early explorations of phenomena by undergraduate students (Abdulwahed and Nagy, 2009; Balamuralithara and Woods, 2009), new computation methods (i.e virtual reality, augmented reality, better computer graphics) can improve the VL’s experience and VLs can become a more attractive option for advanced students/users. Furthermore, over 12,400 publications can be found under the search of educational virtual laboratories only considering engineering education (Dimensions.ai, 2021), which proves an interest in this type of educational tool.
Virtual laboratories are interactive environments that allow students to explore a topic by testing and comparing different scenarios; a playground for experimentation (Penner, 2001). Multitude VLs have been created to provide online education at different levels and fields/topics. For example, ABCmouse provides a learning-simulated environment for children aged from 2 to 8 (ABCmouse, 2021), LABSTER has successfully been used by students in high school (Bonde et al., 2014) as well as in early university levels (Dyrberg et al., 2017), ASPEN HYSYS (AsperTech, 2017), SuperPro (I. Inc., 2017) or SimaPro (SimaPro, 2021) are other examples of software used by university students as well as professionals in the (bio)chemical engineering field. In engineering education, VLs are of great importance and widely used since “learning by doing” plays an essential part (Feisel and Rosa, 2005). Although this has been traditionally provided by “in-person” laboratories, physical experimentation presents several economic and logistic constraints (Kirschner and Meester, 1988; Baroutian et al., 2016) (e.g., difficult to deliver high quality when faced with an increasing number of students or students unable to attend due to the recent COVID pandemic). Therefore, some of the motivating arguments for developing and implementing VLs in traditional education are: 1) the need to teach practical aspects of complicated processes; 2) the need for a more flexible and accessible learning system; and, 3) to proactively prepare students to take part in the current transition to Industry 4.0 (de las Heras et al., 2021a). Industry 4.0, or the fourth industrial revolution, is the rapid change of technology, industry, and social patterns and process due to the increasing interconnectivity and smart automation (Bai et al., 2020). The embrace of “digital” has brought a set of its own challenges that education must also meet. However, even though VLs bring learning benefits and opportunities, they also have intrinsic operational and content-related challenges.
1.2 Challenges in VL
Designing an educational VL first requires clear definitions as well as being able to provide user-friendly interactions and an interface. Previous studies assessing the students’ perceptions (before and after using a VL), show that the students are (Balamuralithara and Woods, 2009; Dyrberg et al., 2017; Cano de las Heras et al., 2021): 1) discontent with the lack of collaborative learning; 2) frustrated over terms and calculations that were new and difficult to understand; and, 3) wanting a more interactive simulator with open-ended scenarios. Overlooking these perceptions can negatively affect the students’ learning experience; these design characteristics are ultimately connected with the fact that the students are not involved in the VL’s design. Furthermore, the learning experience can also be negatively influenced if there are usability and functionality issues. For example, two independent studies performed in 2017 (Dyrberg et al., 2017) and 2019 (Cano de las Heras et al., 2021) investigate the students’ experience with a commercial virtual laboratory named Labster (Labster, 2018). These studies reported technical problems (e.g., black screens or operating errors) and software “bugs,” which negatively affected the students’ engagement and overall user experience. Solving issues and implementing features concerning usability and functionality can be more easily done at an earlier stage of design. This allows issues to be avoided and features to be implemented before the final software is made available. These challenges can be tackled through an active engagement of future users as co-designers (Matthews et al., 2019) to not only improve human-computer interaction but also to better align and define the learning strategy (Figure 1).
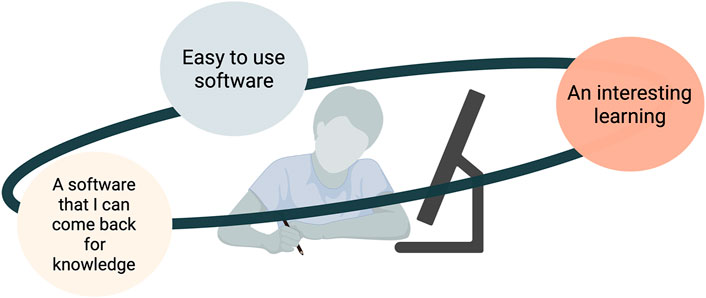
FIGURE 1. The student as the focus of the VL and inquiring about the learning goals’ suitability, the software’s features and user-friendliness in the VL.
Furthermore, previous studies have shown the importance of students’ revision and finding explanations (Berland et al., 2016; Zhu et al., 2020) as well as their involvement while shaping their learning (Galloway and Bretz, 2015). This also includes VLs and computer-aided tools (Gerard et al., 2022). As well, the student’s perception of the value of an activity is strongly linked to their performance and success in the learning activity (Wigfield and Eccles, 2000). Therefore, involving the students in defining what is important and what they would like to learn has already been proved to have a positive impact in the outcome of their learning experience (Matthews et al., 2019). Thus, in this work, we focus on investigating and presenting a strategy to involve the learner as partner in developing a VL for engineering education through the use of (co-)participatory design.
1.3 Participatory design in learning environments
Participatory design aims to include the users in the design process so that their opinions and preferences are reflected in the design of a technology they use (Bodker et al., 2009; Yamauchi, 2012; Greenbaum and Kyng, 2020). Druin (2002) (Druin, 2002) proposed roles for the students as: user, tester, informant, and designer partner while designing technology. Although, the students’ perspective in the final evaluation of the VLs have been amply studied (i.e. (Ebner and Holzinger, 2003; Bonde et al., 2014; Al-Khalifa, 2017; Dyrberg et al., 2017)), the early stages of the development of VLs have not been sufficiently analyzed. Furthermore, traditionally, students have not participated actively as designer partners in the design process of an educational tool (including educational VLs) (Rudduck and McIntyre, 2007). This, as previously mentioned, might have a potential negative impact on their learning and learning experience as “students should help shape rather than simply be shaped by educational policies and practices” (Cook-Sather, 2003). However, as mentioned on the previous section, there is a switch of this tendency, involving more the students.
However, it is noteworthy that considering the users’ perspectives while developing software is not novel per se; for example, this has been used before in agile software development (e.g., scrum-based systems) [? ]. In this study, it is proposed to involve the students as design partners during the development of an educational VL through (co)-participatory design. The use of (co-)participatory design since the beginning ensures that the final platform meets the students’ needs and standards as well as decreases the issues in current VL platforms. By combining the expertise of different agents - teachers, students, and designers – this can improve the quality of the instructional design process and the resulting learning environment (Könings et al., 2014). Furthermore, (co-)participatory studies indicate that the users are more likely engaged and take pride in the final tool (Falcão et al., 2018).
Therefore, a co-participatory design strategy, supported by workshops and design sessions, is recommended, aiming to embolden and support the future users to 1) provide feedback; 2) be creative; and, 3) come forward with their own ideas. Several co-participatory design methods (Yamauchi, 2012) are listed in Table 1.
The selection of methods depends on the areas of knowledge and the designers’ preferences, the maturity of the design, and/or the objective of the experience. Hence, several co-participatory design trials should be performed before a “final” product version is available (e.g., the VL tool).
In summary, in this work, we add to and expand the research on the co-participatory design of an educational VL for undergraduate (bio)chemical engineering students. This is addressed by investigating and presenting a strategy to involve the learner as partners in developing a VL for engineering education through the use of (co-)participatory design.
The remainder of this article is organized as follows. In Section 2, we introduce the theoretical framework on which the VL is built upon. Section 3 presents the different methods and the data collected in four different co-participatory design experiences. The subsequent analysis and discussion of results are presented in Section 4. Finally, conclusions are drawn in section 5.
2 Theoretical framework
The design of an educational VL requires a theoretical framework that strongly supports the learning process.
2.1 Blended learning
Blended learning is defined as the integration of online with face-to-face modes of communication (Dziuban et al., 2018; de Brito Lima et al., 2021). Its learning practices include a wide range of models and methods which typically implies: 1) having part of its content accessed through an online platform; 2) flexibility in terms of the sequence of tasks that students should perform; and 3) the role of the teacher becomes a mediator rather than an instructor (Horn and Staker, 2017; Dziuban et al., 2018; de Brito Lima et al., 2021). Blended or “hybrid” course offers were estimated to be used by 79% of higher education public institutions in the U.S. in 2007 (Allen et al., 2007), and it has only increased as 87.6% of students worldwide were out of their educational institutions due to the Covid-19 pandemic by March 2020 (The, 2020). Furthermore, blended learning can support the SDGs agenda (Sustainable Development Goal), especially Goal 4 - quality education for all (Caird and Roy, 2019). Blended learning has already proven benefits; it brings: 1) increased interactions between teachers and students (Jusoff and Khodabandelou, 2009), 2) more flexibility, 3) pedagogical richness, and 4) a better cost-effectiveness ratio (Graham, 2006). However, the combination of online and face-to-face modes of communication does not ensure better learning outcomes in itself (Driscoll et al., 2012; Arrosagaray et al., 2019). In fact, previous studies have demonstrated that the learning outcomes highly depend on the teaching practice and the creation of structured learning with well predefined learning goals (Rasheed et al., 2020). In addition, teachers have stated that there is a lack of suitable didactic technology (Rasheed et al., 2020). In summary, blended learning is a well-established learning strategy requiring online educational technologies appropriate for all involved stakeholders. Hence, to maximize the benefits of blended learning, all active parts - teachers, designers, and students - should be included in designing the online educational platform/technology.
2.2 P2Si - A gamification strategy
In this work, a previously developed framework by the authors, named P2Si, is used (for detail see (de las Heras et al., 2021b)). P2Si integrates the aspects of 1) the use, reuse, and explanation of process models; 2) a tailored learning design; 3) game elements as motivation strategy; and, 4) the use of students as co-designers through participatory design to improve the human-computer interaction. In this earlier study, the P2Si framework was applied with the aim of teaching undergraduate students in the biochemical engineering program about bioprocesses and their mathematical model formulation. The learning is supported by Kolb’s experiential cycle (Abdulwahed and Nagy, 2009): 1) a new experience or situation is confronted (experience stage); 2) the experience is observed and reflected upon (review stage); 3) abstract concepts and generalizations are made in order to assimilate the learning content (concluding stage); and, 4) derived hypotheses are applied to future situations leading to new experiences (action stage) (Cano de las Heras et al., 2021). For this study, the Kolb’s experiential cycle is also applied towards “hand-on” open-ended exercises for programming of specific bioprocesses models and for overall bioprocess modeling (i.e. optimization of a bioprocess based on a set of feed constraints). This is additionally supported by a collaborative e-learning strategy. The collaborative learning is implemented through the use of two bots (de Las Heras et al., 2020). Menten (bot 1) provides the students with the knowledge and help on the topic; whereas Michaelis (bot 2) behaves like the “evil twin” providing faulty/wrong information which the students must (knowingly) analyze critically. Furthermore, additional theoretical information and related quizzes are inbuilt into the platform. To conclude, since this work aims to give the students the opportunity to be designers of their learning tools, the different elements here explained can be modified, added, or eliminated based on the students’ feedback.
3 Methods and data collection
Four co-participatory experiences were built and are presented in this section. Each of the experiences was performed at different VL design stages; from need identification on paper to a learning verification through an online software. It is worth noting that the questionnaires and co-participatory experiences were performed with unbiased groups of students who independently volunteered for these experiments without prior knowledge of the content of the experiment. Figure 2 illustrates the different VL prototypes used in each of the experiences.
3.1 Mock-up: paper prototype
In 2018, a first co-participatory experience was done using a paper prototype (Figure 3).
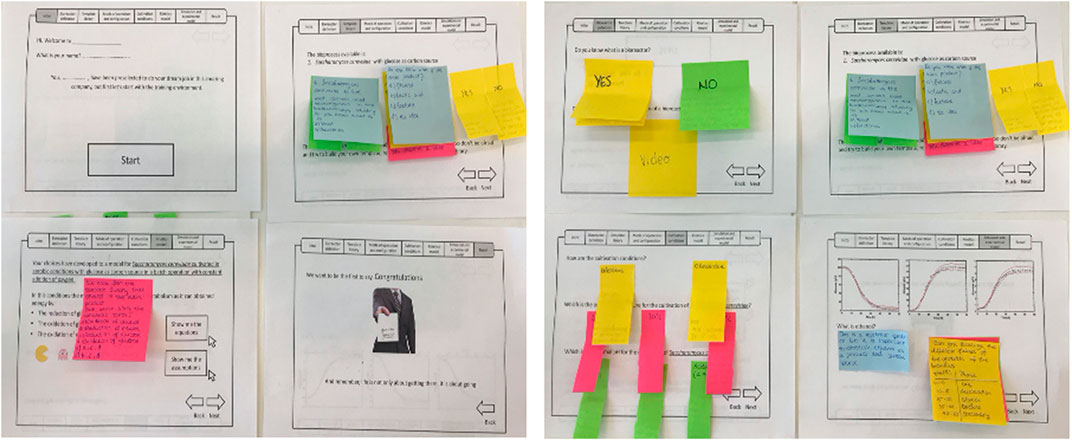
FIGURE 3. Paper prototype for participatory design (de las Heras, 2018).
Paper prototypes are a well-established strategy to design software and software interfaces in an interactive setting with students and developers. The use of paper allows quick changes and can provide useful feedback that helps to design user-friendly products. The first experience was performed with the help of 10 students from the second year of the bachelor’s degree in Sustainable Biotechnology, Aalborg University Copenhagen. In the session, quantitative and qualitative data were collected through a pre- and post-survey. More information about this experience and the data collected can be found in Cano (2021) (de las Heras et al., 2021a).
3.2 Workshop: University of Auckland
During the 2019 academic year, seven students from the course of engineering biotechnology at the University of Auckland (New Zealand) participated in a co-participatory design experience using an in-line software prototype (Figure 4).
This prototype (see Figure 4) was not, at this stage, deployed online yet - it was only rendering at a local computer (inline). In the session, information was collected by using a mixed-methods methodology, which included: 1) a post-laboratory survey about the learning content (six multiple-choice questions, quantitative and qualitative); 2) a post-laboratory survey about usability and functionality (seven multiple-choice questions, quantitative and qualitative); 3) an informal conversation with the software designer; and, 4) a semi-structured focus group interview by an independent educational researcher (not part of the teaching team). The surveys can be found in A. Each questionnaire had five options: strongly agree, agree, neutral, disagree, or strongly disagree, and a comment section was also provided. In addition, six prompt questions were used during the semi-structured focus group interview. These prompt questions were: 1) Do you use the internet to get more information or activities/exercises about bioprocesses? 2) What do you think/feel about virtual learning? 3) What do you think about the prototype? 4) Do you think it is engaging? 5) What can be improved? and, 6) In the next iteration, what do you think will make the software better? and/or what do you want to see on it? The interview was audio-taped and transcribed. Ethics approval was obtained from the University of Auckland Human Participants Ethics Committee.
3.3 Workshop: Technical University of Denmark
During the 2020 academic year, under the COVID-19 health crisis, students from the Bioprocess Technology course at the Biochemical Engineering bachelor program at the Technical University of Denmark participated in an online co-participatory design experience (Figure 5). Only three people actively participated in this experience due to the health crisis and social distance requirements.
In the session, information was again collected through a mixed-methods methodology, although a different set of questionnaires were used (see B, C, and D). Data was then gathered through: 1) a post-laboratory survey about the learning content (five multiple-choice questions, quantitative); 2) a post-laboratory survey about usability and functionality (eight multiple-choice questions, quantitative) (Chin et al., 1988; Tullis and Albert, 2013); 3) an informal conversation with the software designer. As only three students answered the surveys, the quantitative data collected cannot support conclusions. However, the informal conversation was a valuable source of information about potential modifications to the software.
Finally, in 2021, the last co-participatory design experience was performed with students of the biochemical and chemical engineering master’s education using an updated online version of the software (Figure 6, available at www.biovl.com).
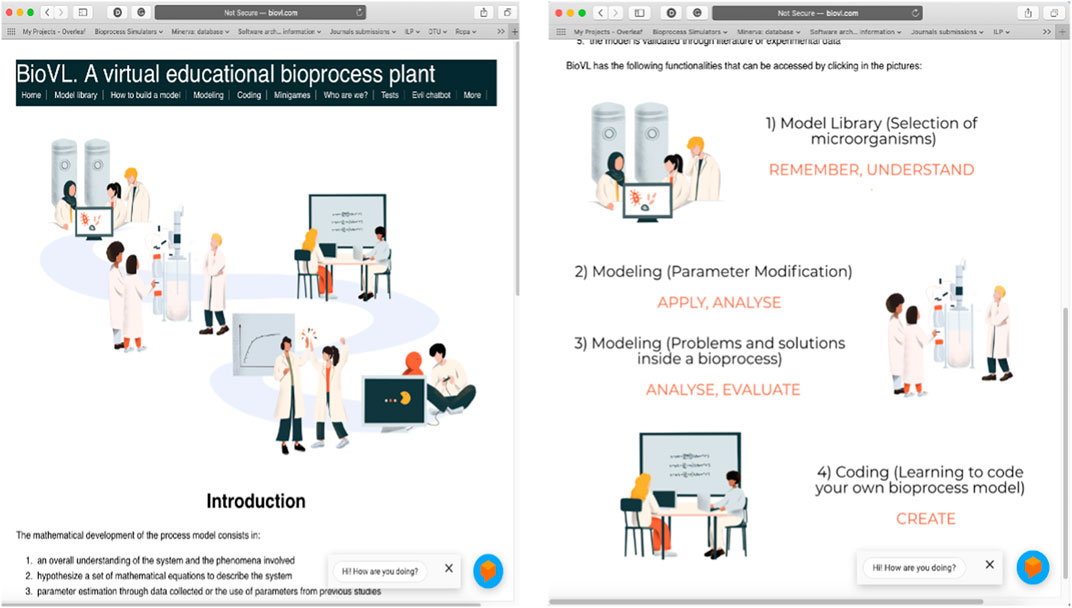
FIGURE 6. Online prototype of BioVL. More at www.biovl.com.
In this session, with 13 participants, the questionnaires (see B, C, and D) were again used to collect information related to learning verification, usability and functionality. Additionally, in order to collect qualitative data, an informal conversation with the software designer and a semi-structured group interview by an independent educational researcher were performed. The prompt questions of the focus group semi-structured interview were the same as those used during the University of Auckland experience (see Section 3.2). The interview was audio-taped and transcribed.
4 Results and discussion
(Co-)participatory design enabled us to engage the students in designing software they will actively use in their education. It also allows creating a tool that is more in tune with the students’ needs and preferences. Consequently, the tool evolves each time a new participatory experience is performed and new co-participatory data is collected. In this section, the feedback, subsequent software evolution, and students’ feedback are presented.
4.1 Participatory experience at Aalborg University
The data collected in this experience has been previously reported and discussed in (de las Heras et al., 2021b). This experience focused mostly on inquiring with a small group of bachelor-level students, aiming at finding out whether they thought the software was a good idea and also posing questions to the students about its usability as an educational tool (or similar tools). The most important conclusion from this experience was that the students’ perceived the software as a potentially valuable addition to their education, especially as a supporting tool. However, they also highlighted the need for explicit rules and learning objectives and paid further interest in it being a possible way for collaborative online learning.
As previously described in Section 3.2, after validating the software idea through the paper prototype, a software prototype was designed and implemented as a desktop platform. Some screenshots are shown in Figure E.14 in Appendix E as well as in https://youtu.be/BSCEiVMTreE. Although the desktop platform, named FermProc (Fermentation Processes) at the time, showed promising results based on the interest demonstrated by students, professors, and industry, the in-line nature of the platform presented several issues. For example, the need to install it on local computers (private or corporate) presented a significant disadvantage. As well, students have also indicated that it would be beneficial to have certain features (e.g., multiplayer environment for collaborative learning) that are particularly easier to have and implement in an online software. In response to the students’ feedback and especially the participatory experience at the University of Auckland, FermProc was re-built as a web-based software (and later re-named to BioVL).
4.2 Participatory experience at the University of Auckland
Seven students joined in the participatory experience at the University of Auckland. They were asked about their perceptions regarding the learning content and the usability/functionality of the tool. The data collected are presented in Figures 7, 8, respectively.
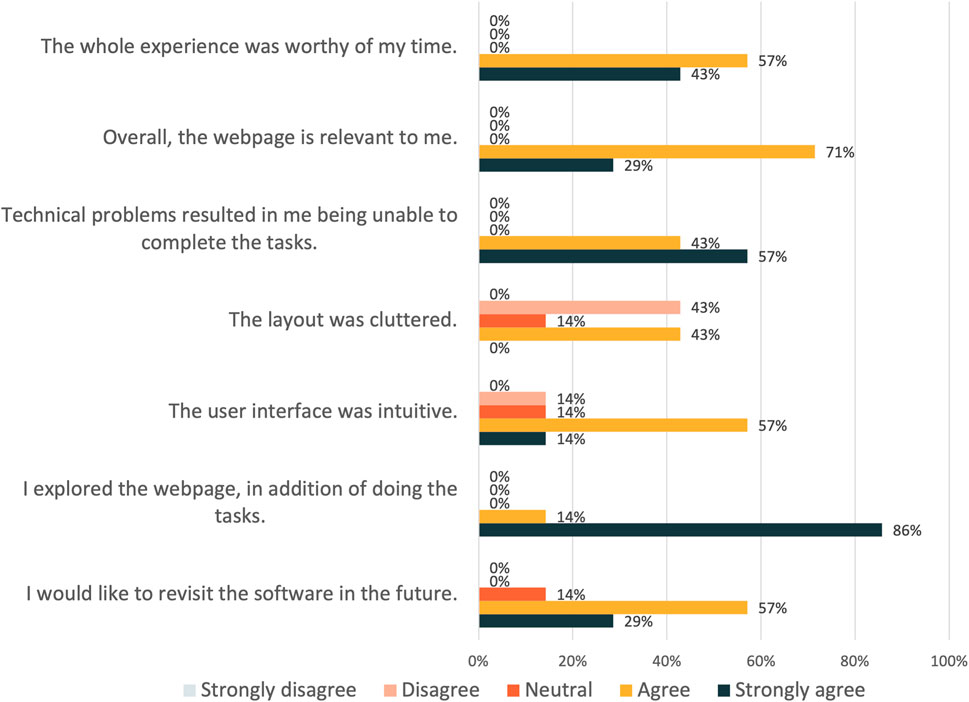
FIGURE 8. Student’s perspective about the usability and functionality provided by FermProc(later BioVL).
Aiming to verify the learning, a task was specified for the students to perform: learn as much as possible about the aerobic growth of Saccharomyces cerevisiae. Figure 7 shows that the students were dissatisfied with the learning task and the information provided on the platform. As the learning objectives were not fulfilled, i.e., the students did not perceive that they had learned as much as possible; this reflects that the information provided by the platform might have been insufficient and/or the teaching method was also not satisfactory. Therefore, the platform and the learning design need to be improved and reformulated.
Moreover, software bugs (“some links did not work so I could not participate in the simulations”) further led to the students’ discontent regarding the online platform. This was due to the fact that human-computer iteration highly influences the learning experience. The identified bugs (usability issues) were fixed on the next iteration. Concerning qualitative data, students indicated their preference for a video instead of reading material; they also pointed out that there was too much information, thus requiring a more interactive environment. Figure 7 also shows that the students were interested in using FermProc because it has: 1) relevant content, and 2) easy to find and understand information. This was highlighted in the comments section with statements such as “the information regarding the yeast was well written and provided useful information about the process.” Figure 8 also indicates that even when the content was less than expected, the experience was still worthy and relevant to the students. Furthermore, the majority of the students were interested in seeing the next version of the software. This reinforced our hypothesis that such type of educational tools have great potential and could bring considerable benefits if integrated into the (bio)chemical engineering curriculum. In the semi-structured interview and conversation with the designer, some students expressed their interest in 1) having the learning content divided into “smaller bits,” and 2) learning and acquiring more coding experience. They foresee the need for coding/programming in their future careers due to increased digitalisation and the Industry 4.0 paradigm shift. Thus, acquiring advanced programming skills in their curriculum is perceived as essential. Therefore, introducing more coding/programming exercises in the online software was further investigated and tested with a different set of students at the Technical University of Denmark (de Las Heras et al., 2021a). This study aimed to gather the students’ interests about programming: the if, what and how. Some of the key findings in (de Las Heras et al., 2021b) were that: 1) only 15.7% of the students believe that they have enough programming in their curriculum; 2) the students preferred to learn Python (79%) over other programming languages; and, 3) they considered that hands-on activities about modelling and optimization are the best strategy to naturally develop coding skills. Consequently, these insights were used by including coding exercises in Python into the platform. This would then be evaluated in the next co-participatory experience.
Lastly, the students were asked about the use of gamification elements as a motivational approach. Although most of the students requested a more interactive environment, some students believed that a more gamified environment would not improve their experience but rather decrease the flexibility of the software. Their argument was based on a previous experience with an educational game-based software from a different course in their educational program. In this experience, the students’ perceived the gamified software as confusing, lacking clear learning objectives and a clear learning path. Moreover, they found the gamification too “childish,” which negatively affected their interest and learning. These disadvantages related to gamified learning platforms have been previously documented by [? ]. [? ] has stated that game-based learning can, in fact, decrease the students’ motivation and engagement if the learning strategy is not perceived as valuable.
To conclude, the external/independent teachers involved in the co-participatory experience commented that FermProc was a difficult name, and they did not like it. Therefore, the authors came up with a new name for the software: BioVL (Bioprocess Virtual Laboratory).
4.3 Participatory experience at the Technical University of Denmark
There were two experiences performed at the Technical University of Denmark. The first experience was performed with a group of only three participants in 2019. The participatory design experience and interview settings had to be adjusted due to the COVID-19 health crisis. As only three students participated, the quantitative data related to learning, functionality, and usability cannot be considered meaningful. However, the feedback given by the students during an informal conversation with the designer was valuable and taken into account when developing the next version of the software. A more developed prototype was used, where the students focused on adding/modifying some of the content. Of note is that coding exercises were still not available due to technical issues. Ideas that the students wanted to see in the next iteration were given in the form of comments, for example, “maybe you can enable the users to modify several (like three) parameters in the simulation instead of only one.” These were also used to make further modifications to the software (e.g. Figure 9).
The last co-participatory user experience took place in 2021, where 13 students participated using a new version of BioVL. They were also asked about the software’s pedagogical usefulness, usability, and functionality.
As previously described in Section B (learning verification), to assess the learning satisfaction, applicability, and value as well as quantifying the students’ motivation, a specific learning task was set up. The task prepared for the students is presented in Figure 10.
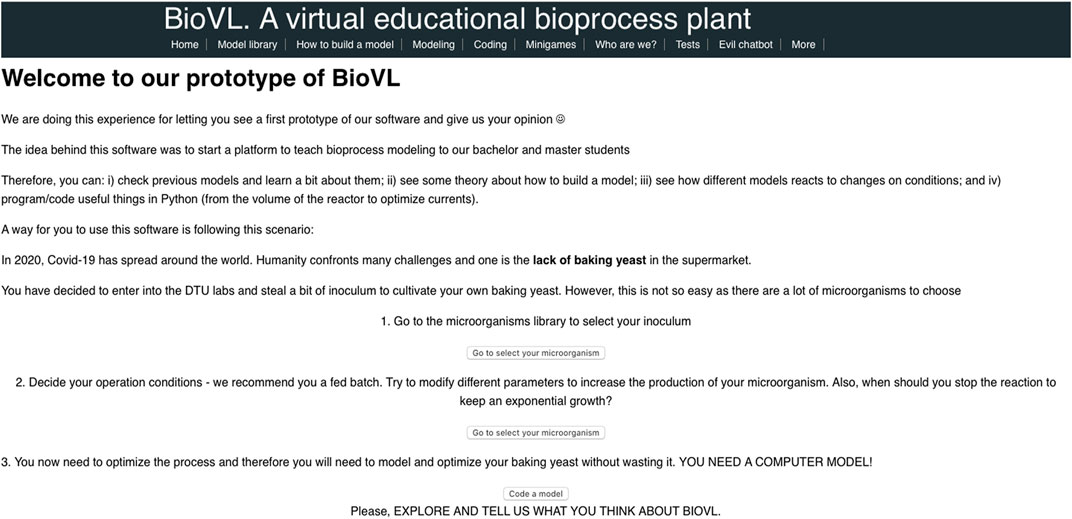
FIGURE 10. A semi-guided task for learning verification of the BioVL prototype. More scenarios will be continuously added. More at http://biovl.com/intro_popup.
Figure 11 shows the students’ perspectives concerning the encoding and usefulness of the pedagogical domain of BioVL.
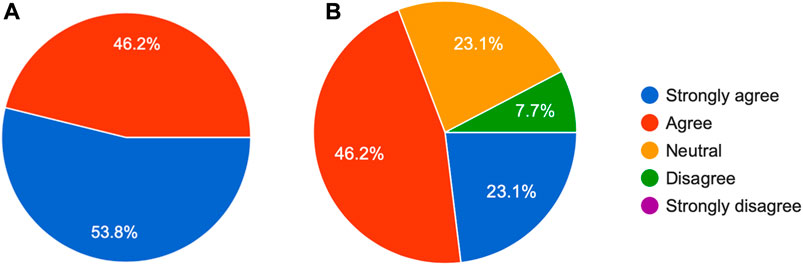
FIGURE 11. Student’s perspectives: (A) are they interested in the topic of this learning material?, and (B) will the skills and knowledge taught by this software be useful in the future?
As observed in Figure 11, the participants considered the topics introduced in BioVL (bioprocesses and modeling) as meaningful for them, and 69.3% considered it useful for their future careers. The decision to use a co-participatory design was based on the interest of involving the students in creating an educational tool that they would find interesting. These results indicated that the central requirements (meaningful and long-term learning) for the students’ learning satisfaction and motivation are being fulfilled. It also provided the designer with qualitative information. This was given in the form of comments reflecting their interest in more in-depth learning, for example: “an introduction to programming and more examples,” “a introduction to data types and structures in Python,” and “examples/exercises related to mass balances, mixing types, options to calculate kla, different bioreactors,” etc.
Furthermore, Figure 12 presents the remaining pedagogical domains inquired in the survey.
Figure 12 also shows that 1) 69.2% of the students agree over the size of the “portions” of new material that are presented; and 2) 53.8% agree that the learning material provides learning challenges without a pre-defined solution model. A high agreement is desirable as the lack of open-ended problems has been an issue with previous VLs. However, a lower agreement among the students over problem-based implementation in BioVL has been recorded. 23.1% of the participants agree that BioVL needs to provide the solutions without the instructors’ help. This point was tackled by implementing chatbots (de Las Heras et al., 2020). However, this feature is still not fully implemented (usability and functionality), which is demonstrated by the students’ opinions, such as “the evil chat bot is an amazing feature; please make it work well.” Furthermore, better explanatory videos, more clues/hints, as well as more, and even personalized, feedback will be implemented in the next prototype version to tackle the pedagogical domain. Important to highlight is that these results are not final as the platform is under continuous development, and thus the prototype’s learning verification is premature. Therefore, additional pedagogical verification is expected as BioVL matures.
The students also evaluated the software in terms of usability and functionality, i.e., the weaknesses and strengths of the human-machine interactions available in BioVL, which play a prominent role in the learning and the motivation to continue using the tool. Figure 13 depicts these results; it quantifies how the students perceive these interactions on a scale 0–10. As demonstrated in Figure 13, a significant percentage of the students voted 6, which qualitatively means that the human-computer interaction is above average, but there is still a significant room for improvement; this is very important and is currently being implemented.
Overall, the participants’ opinions varied and indicated that BioVL has certain areas and domains worth improving in functionality and usability. Of note is that the students perceived the software as more rigid than flexible and rather frustrating, which can be a result of the software being in its prototype stage. The qualitative data from the usability test provides valuable information about how to modify and improve the software. Some of the comments (qualitative data) provided by the students are: 1) “it is a great tool but needs UI simplification and consistency and clear statement of purpose in intro page” and “generally, I think the idea is good, especially as an introduction for new people as it can give an easy overview of what bioprocessing is. However, currently, there seems to be a lot of problems, which is a bit frustrating. I often encountered “Internal Server Problems,” and it seemed a lot of functions were not finished/available.”
To conclude, as demonstrated, BioVL has increasingly become a useful educational tool. However, being an online software, it is under continuous development to keep it improving it. Thus, the authors encourage the readers to try the software and welcome any comments and suggestions (http://biovl.com/more).
4.4 Limitations
Several limitations have constrained this study and affected its outcomes. One of the limitations has been preventable technical issues that has negatively affected the students’ experience. Another limitation is that the focus group participants were not chosen randomly and instead self-selected to participate. Different groups of students from different universities may have different learning objectives or design preferences. Although seen as a strength this can also bring contradicting opinions between the different groups. Additional research focused on the adaptation of the software, increased interactivity, and overall human-computer interaction would be beneficial for the future stages. Furthermore, it should be noted and carefully considered that these new teaching methods can be perceived differently depending on the education line, curriculum, syllabus, and even cultural aspects of the students/design partners. However, such aspects are not within the scope of the current paper.
4.5 Future perspectives
`The students provided valuable feedback on how to further develop BioVL. Thus, many of the suggestions that arose from the surveys will be explored in future releases of the software. Future work will mainly focus on performing additional co-participatory experiments, such as integrating the teachers’ perspective and feedback to the platform, and the fit of a video-based learning environment, with particular emphasis on the multi-modal integration of video and auditory content.
5 Conclusion
Educational VLs are being increasingly developed and used as teaching aid. Therefore, new strategies are needed to create tools that better understand, support, and implement user-friendly human-computer iteration. Current VLs bring several challenges, which are especially valid for graduate and undergraduate engineering education. The highlighted concerns can be eased or even overcome by actively involving all stakeholders in designing the learning software platform. In this work, a four steps co-participatory design strategy has been presented, starting with a paper prototype and ending in a full online platform (BioVL). The co-participatory user experiences have been performed with students from different educational programs, universities, and countries. The first co-participatory experience assessed the interests of the focus group (undergraduates at biochemical engineering) on such a platform, while the second co-participatory experience identified the learning content which the students were most interested in. Finally, the third and fourth co-participatory experiences provided information that could improve and polish the educational software; these experiences were applied to a more significant test group of students. During the experiences, important information (qualitative and quantitative data) and overall feedback were collected, highlighting areas/features to be improved in the subsequent versions of the software (e.g., add coding exercises, decrease written theoretical explanations).
In summary, in the last co-participatory design experience, the students pointed out that some usability and functionality issues somewhat jeopardized its use in the early stage prototype. However, the students also described the tool as “great” and as “a good idea.” Thus, if developed further and by continuously using the users as co-designers, BioVL has a great potential in becoming a worthwhile tool to integrate into the curriculum as an educational tool and active teaching aid.
Data availability statement
The original contributions presented in the study are included in the article/Supplementary Material, further inquiries can be directed to the corresponding author.
Ethics statement
Ethics approval was obtained from the University of Auckland Human Participants Ethics Committee.
Author contributions
All authors listed have made a substantial, direct, and intellectual contribution to the work and approved it for publication.
Conflict of interest
The authors declare that the research was conducted in the absence of any commercial or financial relationships that could be construed as a potential conflict of interest.
Publisher’s note
All claims expressed in this article are solely those of the authors and do not necessarily represent those of their affiliated organizations, or those of the publisher, the editors and the reviewers. Any product that may be evaluated in this article, or claim that may be made by its manufacturer, is not guaranteed or endorsed by the publisher.
Supplementary material
The Supplementary Material for this article can be found online at: https://www.frontiersin.org/articles/10.3389/fceng.2022.959188/full#supplementary-material
References
Abcmouse (2021). I. Age of learning, ABCmouse: Early learning academy (2017-2021). Available at: https://www.abcmouse.com/abt/homepage.
Abdulwahed, M., and Nagy, Z. K. (2009). Applying Kolb’s experiential learning cycle for laboratory education. J. Eng. Educ. 98 (3), 283–294. doi:10.1002/j.2168-9830.2009.tb01025.x
Al-Khalifa, H. S. (2017). Chemotion: A gesture based chemistry virtual laboratory with leap motion. Comput. Appl. Eng. Educ. 25 (6), 961–976. doi:10.1002/cae.21848
Allen, I. E., Seaman, J., and Garrett, R. (2007). Blending in: The extent and promise of blended education in the United States. Columbus, Ohio: ERIC.
Arrosagaray, M., González-Peiteado, M., Pino-Juste, M., and Rodríguez-López, B. (2019). A comparative study of Spanish adult students’ attitudes to ict in classroom, blended and distance language learning modes. Comput. Educ. 134, 31–40. doi:10.1016/j.compedu.2019.01.016
Bai, C., Dallasega, P., Orzes, G., and Sarkis, J. (2020). Industry 4.0 technologies assessment: A sustainability perspective. Int. J. Prod. Econ. 229, 107776. doi:10.1016/j.ijpe.2020.107776
Balamuralithara, B., and Woods, P. C. (2009). Virtual laboratories in engineering education: The simulation lab and remote lab. Comput. Appl. Eng. Educ. 17, 20186. doi:10.1002/cae.20186
Baroutian, S., Kensington-Miller, B., Wicaksana, F., and Young, B. R. (2016). Bridging theory with real world research experience: Co-Teaching engineering biotechnology with R&D professionals. Educ. Chem. Eng. 16, 9–16. doi:10.1016/j.ece.2016.05.002
Berland, L. K., Schwarz, C. V., Krist, C., Kenyon, L., Lo, A. S., and Reiser, B. J. (2016). Epistemologies in practice: Making scientific practices meaningful for students. J. Res. Sci. Teach. 53 (7), 1082–1112. doi:10.1002/tea.21257
Bodker, K., Kensing, F., and Simonsen, J. (2009). Participatory IT design: Designing for business and workplace realities. Cambridge, MA, USA: MIT press.
Bonde, M. T., Makransky, G., Wandall, J., Larsen, M. V., Morsing, M., Jarmer, H., et al. (2014). Improving biotech education through gamified laboratory simulations. Nat. Biotechnol. 32 (7), 694–697. doi:10.1038/nbt.2955
Caird, S., and Roy, R. (2019). Blended learning and sustainable development. Encycl. Sustain. High. Educ. 2019, 107–116. doi:10.1007/978-3-030-11352-0_197
Cano de las Heras, S., Kensington-Miller, B., Young, B., Gonzalez, V., Krühne, U., Mansouri, S. S., et al. (2021). Benefits and challenges of a virtual laboratory in chemical and biochemical engineering: Students’ experiences in fermentation. J. Chem. Educ. 98, 866–875. doi:10.1021/acs.jchemed.0c01227
Chin, J. P., Diehl, V. A., and Norman, K. L. (1988). “Development of an instrument measuring user satisfaction of the human-computer interface,” in CHI ’88: Proceedings of the SIGCHI Conference on Human Factors in Computing Systems, 213–218. doi:10.1145/57167.57203
Cook-Sather, A. (2003). Listening to students about learning differences. Teach. Except. Child. 35 (4), 22–26. doi:10.1177/004005990303500404
de Brito Lima, F., Lautert, S. L., and Gomes, A. S. (2021). Contrasting levels of student engagement in blended and non-blended learning scenarios. Comput. Educ. 2021, 104241. doi:10.1016/j.compedu.2021.104241
de las Heras, S. (2018). A systematic computer-aided framework for development of pedagogical process simulators using gamification elements A fermentation case study. Ph.D. thesis (Copenhagen, Denmark: Aalborg Universy Copenhagen).
de Las Heras, S. C., Gargalo, C. L., Gernaey, K. V., and Krühne, U. (2021a). “Programming skills across the (bio) engineering curriculum–a students’ perspective,” in Computer aided chemical engineering (Amsterdam, Netherlands: Elsevier), Vol. 50, 2039–2044.
de las Heras, S. C., Gargalo, C. L., Weitze, C. L., Mansouri, S. S., Gernaey, K. V., and Krühne, U. (2021b). A framework for the development of Pedagogical Process Simulators (P2Si) using explanatory models and gamification. Comput. Chem. Eng. 151, 107350. doi:10.1016/j.compchemeng.2021.107350
de Las Heras, S. C., Jones, M. N., Gernaey, K. V., Kruhne, U., and Mansouri, S. S. (2020). “An E-learning bot for bioprocess systems engineering,” in Computer aided chemical engineering (Amsterdam, Netherlands: Elsevier), Vol. 48, 2023–2028.
Dimensions.ai (2021). Dimensions.ai: Educational virtual laboratory (engineering). Available at: https://app.dimensions.ai/discover/publication?search_mode=content&search_text=educational%20virtual%20laboratory&search_type=kws&search_field=full_search&and_facet_for=2209.
Driscoll, A., Jicha, K., Hunt, A. N., Tichavsky, L., and Thompson, G. (2012). Can online courses deliver in-class results? A comparison of student performance and satisfaction in an online versus a face-to-face introductory sociology course. Teach. Sociol. 40 (4), 312–331. doi:10.1177/0092055x12446624
Druin, A. (2002). The role of children in the design of new technology. Behav. Inf. Technol. 21 (1), 1–25.
Dyrberg, N. R., Treusch, A. H., and Wiegand, C. (2017). Virtual laboratories in science education: Students’ motivation and experiences in two tertiary biology courses. J. Biol. Educ. 51 (4), 358–374. doi:10.1080/00219266.2016.1257498
Dziuban, C., Graham, C. R., Moskal, P. D., Norberg, A., and Sicilia, N. (2018). Blended learning: The new normal and emerging technologies. Int. J. Educ. Technol. High. Educ. 15 (1), 3–16. doi:10.1186/s41239-017-0087-5
Ebner, M., and Holzinger, A. (2003). Instructional use of engineering visualization: Interaction-design in e-learning for civil engineering. Proc. HCI Int. 2, 926–930.
Falcão, T. P., e Peres, F. M. d. A., de Morais, D. C. S., and da Silva Oliveira, G. (2018). Participatory methodologies to promote student engagement in the development of educational digital games. Comput. Educ. 116, 161–175. doi:10.1016/j.compedu.2017.09.006
Feisel, L. D., and Rosa, A. J. (2005). The role of the laboratory in undergraduate engineering education. J. Eng. Educ. 94 (1), 121–130. doi:10.1002/j.2168-9830.2005.tb00833.x
Galloway, K. R., and Bretz, S. L. (2015). Measuring meaningful learning in the undergraduate chemistry laboratory: A national, cross-sectional study. J. Chem. Educ. 92 (12), 2006–2018. doi:10.1021/acs.jchemed.5b00538
Gerard, L., Linn, M. C., and Berkeley, U. (2022). Computer-based guidance to support students’ revision of their science explanations. Comput. Educ. 176, 104351. doi:10.1016/j.compedu.2021.104351
Graham, R. (2006). Definition, current trends, and future directions, the handbook of blended learning: Global perspectives, local designs, 3–21.
Greenbaum, J., and Kyng, M. (2020). Design at work: Cooperative design of computer systems. Boca Raton, FL, USA: CRC Press.
Harper, B. D., and Norman, K. L. (1993). “Improving user satisfaction: The questionnaire for user interaction satisfaction version 5.5,” in Proceedings of the 1st Annual Mid-Atlantic Human Factors Conference, 224–228.
Heradio, R., De La Torre, L., Galan, D., Cabrerizo, F. J., Herrera-Viedma, E., and Dormido, S. (2016). Virtual and remote labs in education: A bibliometric analysis. Comput. Educ. 98, 14–38. doi:10.1016/j.compedu.2016.03.010
Horn, M. B., and Staker, H. (2017). Blended: Using disruptive innovation to improve schools. Hoboken, NJ, USA: John Wiley & Sons.
I. Inc., (2017). SuperPro designer. Available at: http://home.aspentech.com/products/engineering/aspen-hysys.
Jusoff, K., and Khodabandelou, R. (2009). Preliminary study on the role of social presence in blended learning environment in higher education. Int. Educ. Stud. 2 (4), 79–83. doi:10.5539/ies.v2n4p79
Kirschner, P. A., and Meester, M. A. M. (1988). The laboratory in higher science education: Problems, premises and objectives. High. Educ. (Dordr). 17 (1), 81–98. doi:10.1007/bf00130901
Könings, K. D., Seidel, T., and van Merriënboer, J. J. (2014). Participatory design of learning environments: Integrating perspectives of students, teachers, and designers. Instr. Sci. 42 (1), 1–9. doi:10.1007/s11251-013-9305-2
Koretsky, M., Kelly, C., and Gummer, E. (2011). Student perceptions of learning in the laboratory: Comparison of industrially situated virtual laboratories to capstone physical laboratories. J. Eng. Educ. 100 (3), 540–573. doi:10.1002/j.2168-9830.2011.tb00026.x
Labster, (2018). L. ApS., LABSTER. Available at: https://www.labster.com/about/.
Matthews, K. E., Cook-Sather, A., Acai, A., Dvorakova, S. L., Felten, P., Marquis, E., et al. (2019). Toward theories of partnership praxis: An analysis of interpretive framing in literature on students as partners in teaching and learning. High. Educ. Res. Dev. 38 (2), 280–293. doi:10.1080/07294360.2018.1530199
Nokelainen, P. (2006). An empirical assessment of pedagogical usability criteria for digital learning material with elementary school students. J. Educ. Technol. Soc. 9 (2), 178–197. Available at: http://search.ebscohost.com/login.aspx?direct=true&db=afh&AN=85866426&site=ehost-live.
Penner, J. (2001). The virtual laboratory environment. Available at: http://pages.cpsc.ucalgary.ca/pwp/bmv/vlab-for-linux/html-docs/environment.html.
Potkonjak, V., Gardner, M., Callaghan, V., Mattila, P., Guetl, C., Petrović, V. M., et al. (2016). Virtual laboratories for education in science, technology, and engineering: A review. Comput. Educ. 95, 309–327. doi:10.1016/j.compedu.2016.02.002
Rasheed, R. A., Kamsin, A., and Abdullah, N. A. (2020). Challenges in the online component of blended learning: A systematic review. Comput. Educ. 144, 103701. doi:10.1016/j.compedu.2019.103701
Rudduck, J., and McIntyre, D. (2007). Improving learning through consulting pupils. London, UK: Routledge.
SimaPro (2021). P. S. B.V., SimaPro: Life cycle assessment (LCA) software. Available at: https://simapro.com.
The, S. (2020). United nations educational, C. Organization, COVID-19 impact on education (UNESCO). Paris, France: The United Nations Educational, Scientific and Cultural Organization.
Tullis, T., and Albert, B. (2013). Self-reported metrics. Meas. User Exp. 2013, 121–161. doi:10.1016/b978-0-12-415781-1.00006-6
Wigfield, A., and Eccles, J. S. (2000). Expectancy–value theory of achievement motivation. Contemp. Educ. Psychol. 25 (1), 68–81. doi:10.1006/ceps.1999.1015
Yamauchi, Y. (2012). “Participatory design,” in Field informatics (Berlin, Heidelberg: Springer), 123–138. doi:10.1007/978-3-642-29006-0_8
Keywords: co-participation design, blended learning, engineering, computers, virtual laboratories
Citation: Caño de las Heras S, Gargalo CL, Caccavale F, Kensington-Miller B, Gernaey KV, Baroutian S and Krühne U (2022) From Paper to web: Students as partners for virtual laboratories in (Bio)chemical engineering education. Front. Chem. Eng. 4:959188. doi: 10.3389/fceng.2022.959188
Received: 01 June 2022; Accepted: 09 September 2022;
Published: 14 October 2022.
Edited by:
Jose Porro, Cobalt Water Global, Inc., United StatesReviewed by:
Ville Alopaeus, Aalto University, FinlandStefan Radl, Graz University of Technology, Austria
Copyright © 2022 Caño de las Heras, Gargalo, Caccavale, Kensington-Miller, Gernaey, Baroutian and Krühne. This is an open-access article distributed under the terms of the Creative Commons Attribution License (CC BY). The use, distribution or reproduction in other forums is permitted, provided the original author(s) and the copyright owner(s) are credited and that the original publication in this journal is cited, in accordance with accepted academic practice. No use, distribution or reproduction is permitted which does not comply with these terms.
*Correspondence: Ulrich Krühne, dWxrckBrdC5kdHUuZGs=