- Department of Chemical Engineering, National Institute of Technology, Rourkela, India
The present study aimed to investigate the treatment of butachlor and other commonly used herbicides by the synthetically formulated microbial consortium SMC1 immobilized on the ceramic raschig rings in a packed-bed bioreactor (PBBR). The PBBR was operated in continuous mode at various flow rates over a period of 70 days to determine the effect of hydraulic retention time (HRT) and initial butachlor concentration on the removal efficiency and elimination capability of the bioreactor. It was observed that the overall operation of the bioreactor changes from being controlled by the mass transfer limitations to the controlled bio-reaction , thus proposing the range of 270–325 mg/L/d to be the optimum operating range for the efficient removal of butachlor by the PBBR. The bioreactor can reduce up to 90% of the initial chemical oxygen demand (COD) value while treating the mixture of herbicides. The operating parameters were optimized using response surface methodology where the feed flow rate of 2.9 ml/min, initial herbicide concentration of 454.63 mg/L, and concentration of an additional nitrogen source at 1.41 g/L was found to yield maximal COD reduction. To date, a continuous study in the field of butachlor biodegradation is yet to be reported. Hence, the study could be used as a model to design a better herbicide biotreatment technology.
Introduction
Glyphosates and chloroacetanilide herbicides are the two most widely applied herbicides used extensively in agricultural practices to protect various crops (Choudhury et al., 2016; Atwood and Paisley-Jones 2017; Shuman-Goodier et al., 2021). Butachlor, a synthetic pre-emergent or early post-emergent herbicide belonging to the class of chloroacetanilide, has been widely used for the control of various types of annual grasses and broad-leaved weeds and the submerged freshwater macrophytes (Mohanty et al., 2004; Chattopadhyay et al., 2006; Liu et al., 2008; University of Hertfordshire 2013). Similarly, glyphosate is a broad-spectrum, post-emergent, and non-selective systemic herbicide used in various crops such as soybean, cotton, canola, maize, etc. (Gianessi 2005; Annett et al., 2014). Based on its worldwide usage, the U.S. Environmental Protection Agency has ranked glyphosate as the principal herbicide (Atwood and Paisley-Jones 2017). Asia alone consumes approximately 4.5 × 107 kg of butachlor per year (Kaur and Goyal 2020; Shuman-Goodier et al., 2021). Recent reports on herbicides in Indian agriculture state that butachlor in rice, alachlor in groundnut, and glyphosate in cotton and tea are the highest consumed herbicides in India, with an annual consumption of 6,750 metric tons and 6,003 metric tons, (Choudhury et al., 2016). Evaluating their potential toxicity toward the non-target organisms, studies revealed that the abov mentioned herbicides pose a major concern to aquatic ecosystems especially when applied in the areas near water bodies (Polard et al., 2011; Mohanty and Jena 2019a). Butachlor is reported to be clastogenic, cytotoxic, and carcinogenic in nature and along with alachlor has been enlisted as a B2 group carcinogen by the USEPA and relegated it to be highly toxic to humans (Orme and Kegley 2004; Lauga et al., 2013; Kaur and Goyal 2020). As butachlor is a recalcitrant and highly persistent herbicide having a negative impact on the environment; a number of studies on the removal of buatchlor have been reported previously (Abigail et al., 2015). Several physico-chemical techniques such as adsorption, ozonation, and catalytic degradation have been applied previously. However, these techniques have several shortcomings such as higher opreating cost, generation of secondary toxic wastes, etc (Mohanty and Jena 2019a). Hence, a biological approach for the removal of butachlor has gained focus with time. One such technique is bioremediation where the microbial species can be used for the disintegration of harmful pollutants in to harmless products. However, the major limitation of this alternative is the slower degradation rate which prohibits its application for the treatment of industrial wastewater. Till date, the study of biodegradation of butachlor has been limited to the batch biodegradation of butachlor of low concentrations and the degradation pathways related to it. Hence, these studies are unable to address the limitations such as uneven cell density, mediocre butachlor biodegradation efficiency due to slow microbial growth rate which is due to low substrate utilization, inhibition due to high butachlor concentration, or mass transfer limitations. Thus, to overcome the aforementioned limitations, the potential microbial strains immobilized on the solid support may be used in bioreactors in continuous mode (Yadav et al., 2014). The available body of work on the biodegradation of butachlor supports the scope of in-depth investigation in this topic with a focus on enhancing the butachlor biodegradation efficiency. Biofilm reactors have been designed to maintain a high biomass density to achieve a higher removal rate even under the conditions of slow microbial growth by protecting the microbial cells involved from stressful conditions due to substrate inhibition (Acuna-Askar et al., 2000). Moreover, since the packed-bed biofilm reactors operates at high active biomass concentrations, even reactors with small reactor volume perform exceedingly well at lower substrate concentrations without the necessity for the separation of active biomass from the treated effluent, thus enhancing the stability and productivity of the reactor and making it easier as well as cheaper to operate (Ercan and Demirci 2015). Due to the aforementioned advantages, packed-bed biofilm reactors have been extensively used in the field of bioremediation technology for the treatment of various environmental pollutants (Alfonso-Gordillo et al., 2016a).
Considering the aforementioned specifics, the aim of the present study was to evaluate the performance of a lab-scale packed-bed biofilm reactor packed with immobilized butachlor degrading the microbial consortium at high loading rates. To the very best of our understanding, this is the first-of-its-kind work to report on the biodegradation of butachlor in a continuous packed-bed bioreactor.
Materials and Methods
Chemicals and Medium
HPLC-grade methanol, acetonitrile, water, and analytical grade butachlor were procured from Sigma–Aldrich (India). Commercial grade butachlor, alachlor, and glyphosate were obtained from Insecticides India, Ltd., Sinochem India Pvt. Ltd., and Excel Cropcare Ltd., respectively. Other chemicals and reagents used in this study were of analytical grade and were procured from Merck (India) and Himedia (India). The minimal salt medium (MSM) constituted (in g/L) of (NH4)2SO4 (1.0), NaCl (1.0), K2HPO4 (1.5), KH2PO4 (0.5), and MgSO4·7H2O (0.2) (pH 7.0) (Liu et al., 2012). The medium was sterilized by autoclaving, and the herbicides were added after filter sterilization.
Microorganisms
The synthetic microbial consortium, SMC1, used in this study was formulated from the bacterial strains Serratia ureilytica AS1, Enterobacter cloacae FP2, and Pseudomonas putida G3 isolated previously from the soil samples contaminated with butachlor and other herbicides through the selective enrichment technique (Mohanty and Jena 2018, 2019b, c). The individual strains were grown overnight in a nutrient broth at 32.5°C at 120 RPM in an orbital shaker incubator. The biomass was harvested by centrifugation and resuspended in the nutrient broth prior to the experiment. Equal amount of the cell biomass (approximately 1 g/L of dry cell biomass) was inoculated in the mineral salt medium, and the setup was incubated overnight at 32.5°C, 120 RPM. The mixed bacterial culture obtained by mixing an equal volume of the cell biomass was used for further experiments.
Packed-Bed Bioreactor
The biodegradation of synthetic wastewater containing butachlor and other herbicides in continuous mode was studied in an up-flow packed-bed batch bioreactor of 100 cm length and 8.5 cm internal diameter with a 55-cm-high reactor bed (Supplementary Figure S1, Supplementary Figure S2). The feed is supplied in to the bioreactor from a feed tank through a peristaltic pump, and the effluent is discharged out of the bioreactor in a collection tank. Sterilized air is supplied in to the system using an air pump having filters at its nozzle through an air sparger at the center of the reactor base with a pore size of 1 mm, and the air feed is controlled by the gas rotameter having a range of 0–30 LPM attached to it. While the outlet for the discharge of exhaust gas was designed at the top of the reactor covered with the lid, the outlet for the effluent was placed above 70 cm from the bottom in the reactor column. To maintain the sterile conditions, the sampling ports were plugged with sterilized silicon tubing and stopper cork. The airflow within the system was regulated by the help of a pressure-regulating valve. The air flow rate was maintained at 2 LPM to maintain the required oxygen level without creating turbulence within the feedflow. All the experiments were carried out at ambient room temperature. The active reactor bed volume was packed with ceramic raschig rings of 6 mm diameter and 6 mm height as the packing material with a stainless steel wire-mesh at both the ends to prevent their movement. Synthetic wastewater comprising butachlor and other herbicides were used as the feed for the reactor and were fed continuously with the help of peristaltic pump in an up-flow mode of operation.
Surface Immobilization of Bacterial Consortium
The bacterial consortium prepared for this study was inoculated to sterile MSM containing butachlor and incubated for 24–36 h at 32.5°C and 120 RPM. The bacterial biomass was harvested by centrifugation at 5,000 rpm for 10 min and resuspended in 4 L of mineral salt medium containing butachlor (200 mg/L), making the final biomass concentration of the inoculant in the inflow solution to 108 CFU/ml (approximate). The cell suspension was transferred into the column packed with previously autoclaved ceramic raschig rings using a peristaltic pump. The raschig rings were washed properly, first with the regular laboratory-grade detergent, followed by 70% ethanol, and finally with sterile double-distilled water repeatedly five times. The raschig rings were vacuum-dried at 45°C for 24 h prior to the immobilization. The biomass washed out of the reactor was recycled back. The reactor was continuously fed with the aforementioned medium containing butachlor and was operated for 15 days until a steady-state performance was achieved. To ensure proper biomass growth; the raschig rings were acclimated for 15 days at the ambient room temperature of 30°C before the commencement of the biodegradation studies. To confirm the formation of bacterial biomass on the support material, a few raschig rings were sampled for ESEM analysis.
ESEM Observation
Formation of the biofilm on the ceramic raschig rings was confirmed by the environmental scanning electron microscope (ESEM). For ESEM sample preparation, the raschig rings were washed with phosphate buffer (pH 7.5) twice and fixed with 2% (w/v) glutaraldehyde for 1 h at 4°C followed by 0.1% tannic acid for 30 min. The fixed samples were washed with PBS and later dehydrated with increasing concentration of ethanol solutions. The samples were washed thrice with 100% ethanol and kept at 37°C for drying. The dried particles were then coated with a gold layer and attached on to the microscope for ESEM analysis.
Continuous Study
The packed-bed bioreactor was operated in continuous mode for 70 days by varying the feed flow rate and inlet butachlor concentration to determine the effect of HRT and inlet loading on the butachlor biodegradation efficiency. The effect of hydraulic retention time on the butachlor removal efficiency of the reactor was determined by keeping the initial butachlor concentration constant at 300 mg/L and gradually increasing the retention time for the operation. Next, the effect of the inlet substrate loading rate was evaluated by keeping the HRT constant and varying the initial butachlor concentration from 500 mg/L to 2000 mg/L. The required nutrient solutions were added separately, and the DO was maintained at the required level. The performance of the PBBR was evaluated in terms of removal efficiency and elimination capacity.
Combined Removal of the Mixture of Herbicides
Approximately 1 g/L each of butachlor, alachlor, and glyphosate was added in a container to prepare the homogenous mixture of the multisubstrate stock solution for the study. The removal efficiency of the bioreactor was evaluated by varying the concentration of the herbicide-mixture (500–2000 mg/L) in the feed keeping all other operating conditions same at a constant HRT of 18 h. The sSamples were collected in the regular interval of 12 h from the outlet and analyzed for COD removal.
Parameter Optimization Using RSM
To evaluate the relationship between the operating parameters on the COD removal efficiency of the packed-bed bioreactor, a statistical optimization technique such as response surface methodology (RSM) was used. Three operational parameters, such as initial substrate concentration, feed flow rate, and additional nitrogen source were considered for the study. To optimize the factors, a 23 full factorial central composite design (CCD) for the three parameters was used. A total of 20 experiments were performed, the particulars of which have been enlisted in Table 1.
Analytical Methods
The concentration of butachlor in the medium was evaluated as per the protocol discussed in the available literature (Mohanty and Jena 2019b). First, the sample was saturated with sodium chloride and then an equal volume of hexane/ethyl acetate (1:1) was added to it. The organic phase was separated and air-dried at room temperature and resuspended in HPLC-grade methanol. The butachlor concentration in the extracted samples was analyzed by HPLC using a 5 μm, C–18 column of Agilent Technologies (United States) with methanol: water as the mobile phase in a ratio of 70:30. The flow rate was maintained at 1 ml/min, and the peak absorbance was obtained at 225 nm.
The COD value of the synthetic wastewater was determined by the protocol as discussed in the literature (Sung Wang et al., 2017). First, the sample was placed in a container and the digestion solution containing K2Cr2O7 was added to it. Then, sulfuric acid was added to the mixture in excess so that an acid layer is created under the mixture. The container was closed tightly and inverted several times for complete mixing of the contents. The containers were placed in a block digester preheated to 150°C and refluxed for 2 h behind a protective shield. Then, the container was cooled to room temperature and one–two drops of Ferroin indicator was added to it. Then, it was stirred rapidly on a magnetic stirrer while titrating with 0.1 M FAS till the color changed from blue-green to reddish brown. The blank containing only reagents and distilled water equal to the volume of the sample was refluxed and titrated in the same manner, and the COD value was determined using the following formula:
where A is the volume of FAS used for the blank in mL, B is the volume of FAS used for the sample, and M is the molarity of FAS, that is, 0.1.
Statistical Analysis
The performance evaluation of the bioreactor in case of the single-substrate treatment study has been expressed in terms of elimination capacity, removal efficiency, and inlet loading rate calculated by the following formulae (Sonwani et al., 2020):
where Bin and Bout are the butachlor concentrations at the inlet and outlet of the bioreactor, respectively;
where CODin and CODout are the COD concentrations at the inlet and outlet of the bioreactor, respectively. All the experiments were conducted in triplicate, and the reported data presented in the graphical form are the mean and standard deviation of these replicates shown as the error bars. Statistical analysis of data was performed using one-way ANOVA at the significance level of 0.05 and Tukey’s post-test using the Graph Pad Prism® software version 6.0c.
In case of process parameter optimization to achieve maximal COD removal efficiency, the relationship between the parameters was expressed in terms of a second-order polynomial equation shown below:
where R being the predicted response is the COD removal percentage; V is the influencing variables/parameters to be optimized; and P0, Pi, Pi,i and Pij are the intercept coefficient, linear coefficient, quadratic coefficient, and interaction coefficient, respectively. The three-dimensional plots obtained depict the interaction among the associated variables. Analysis of variance (ANOVA) furnishes the significance of the correlative coefficient value (R2) and the regression coefficients of the model which in turn defines the quality fit of the model. For designing the experiment and analysis, statistical software Minitab (Version 17.1) has been used.
Result and Discussion
Biofilm Formation on Ceramic Beads
In the present study, ESEM analysis of the immobilized beads in various time frames has been carried out to evaluate the successful biofilm formation of the microbial consortium SMC1 on the hollow ceramic raschig rings. Microbial cells have reportedly been immobilized on various support materials such as calcium alginate beads, cellulose acetates, ceramics, volcanic rocks etc., (Dzionek et al., 2016). Hollow ceramic raschig rings have been opted for the current study as the support material due to a variety of reasons such as it is non-biodegradable, inert, and has high mechanical strength (Foroughi et al., 2019). After the initial period where the cells were allowed to get acclimatized to the new environment and get adsorbed to the support material, the microbial strains grew rapidly to form a thick layer of biofilm around the beads. Figure 1A presents the raschig rings on Day 0 as the control without biomass before the inoculation of the bacterial consortium. The micrograph reveals that the raschig rings have a very rough and porous surface texture with micro-pores scattered randomly throughout the surface, thus providing ample surface area for the immobilization of microbial cells and passage of the medium through the structure (Muter et al., 2017). Figure 1B presents the raschig ring on Day 15, which depicts the porous surface of the ceramic raschig rings being colonized with the biofilm and a dense population of rod-shaped bacterial strains. The figure exhibits the biofilm as a complex structure where the abundant extracellular polymeric matrix fortifies the bacterial cells imitating a microbial mat. The micrograph in Figure 1C corresponds to the 70th day of operation and is quite similar to the micrograph taken during the commencement of the continuous study, thus indicating the fact that the microbial consortium is capable of withstanding high and variable loading rates of butachlor.
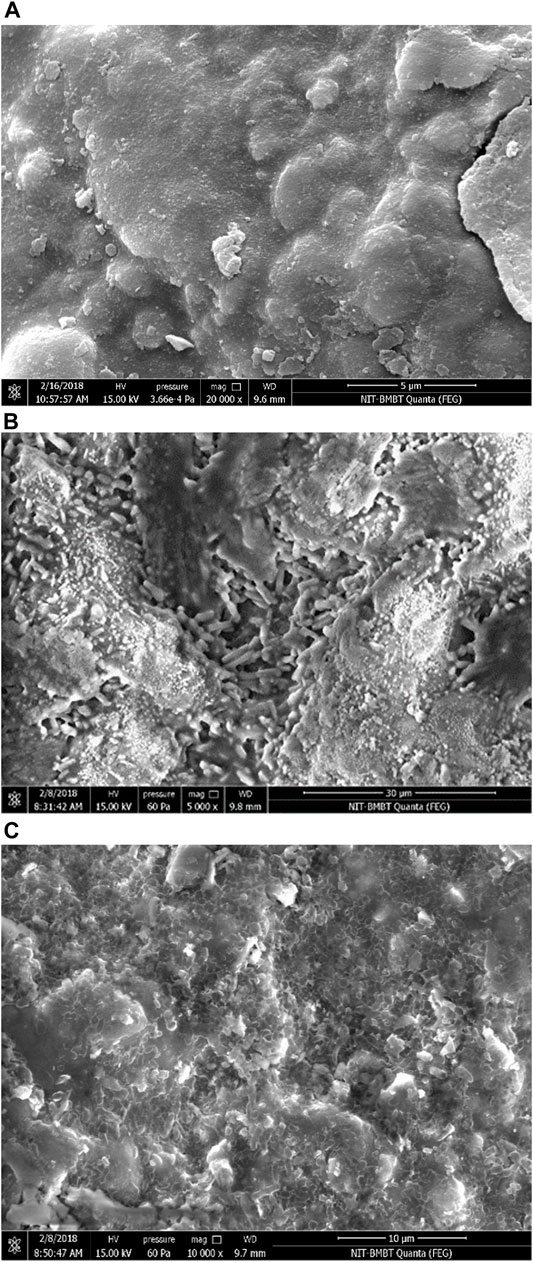
FIGURE 1. ESEM micrographs of ceramic raschig rings (A) without biofilm; (B) with biofilm Day 15; (C) with biofilm Day 70.
The microporous structure of the ceramic raschig ring posseses high surface area which helps in retaining higher biomass on its surface, thus resulting in a high degradation rate. The hollow raschig ring reduces the channeling of the inlet feed, resulting in even distribution of butachlor within the bioreactor. The micrograph confirms the presence of cells both on the surface and within the hollow structure of the ring. From the batch study, it was evident that the degradation efficiency of the bacterial consortium has enhanced significantly on being immobilized as compared to its freely suspended form. This may be attributed to the increased metabolic activity and tolerance of the microbial cells toward the adverse environment on being immobilized (Shukla et al., 2014). Similar results have previously been reported where immobilizing the bacterial strains on any support materials such as low-density polyethylene enhances the removal efficiency from 78 to 91% of the organic contaminant such as 4-chlorophenol from the wastewater sample (Swain et al., 2021).
Continuous Study
The PBBR was operated in continuous mode for 55 days during which the effect of HRT on biodegradation of butachlor by the microbial consortium SMC1 was studied. The study was carried out by varying the feed flow rates ranging from 1.28 ml/min to 5.5 ml/min at ambient room temperature. The performance of the PBBR was evaluated in terms of removal efficiency (RE) and elimination capacity (EC). Figure 2 and Figure 3 represent the variation of the removal efficiency and elimination capacity of the bioreactor system with respect to hydraulic retention time, respectively. After the initial acclimatization period, the PBBR was operated at a low feed flow rate of 1.28 ml/min, which corresponds to the HRT of 36 h and inlet loading rate of 133.2 mg/L/d. The steady-state condition was established within 15 days which was evident from the constant RE of 97%. The elimination capacity of the system was found to be 194 mg/L/d of butachlor. On day 16, the feed flow rate was increased to 2.22 ml/min which corresponds to the HRT of 30 h and the inlet loading rate of 159.84 mg/L/d. A sharp dip in both the RE and EC is observed on day 16 with increase in the retention time. However, both RE and EC recovered by day 24 and remained constant at 91% and 217 mg/L/d, respectively, with a very little change. A similar trend was observed with each change in HRT of 24, 18, and 12 h. The effect of retention time on the overall performance of the packed-bed bioreactor has previously been reported for various other organic pollutants where higher contaminant removal efficiency has been obtained on increasing the retention time of the bioreactor system. The contaminant removal efficiency of the packed-bed bioreactor increased from 80 to more than 95% on increasing the HRT from 0.25 to 4 days, respectively (Chen et al., 2016; Khalid and Hashmi 2016). The decrease in the removal efficiency (RE) at low retention time may be attributed to the insufficient contact time between the contaminant present in the feed and the immobilized microbial strains, which leads to incomplete or low degradation efficiency of the substrates (Alfonso-Gordillo et al., 2016b).
Since the effective functioning of a bioreactor is dependent upon the inlet loading rate, its effect on the butachlor removal efficiency and elimination capacity has been investigated for the PBBR, and the relationship has been presented in Figure 4. From the graph, it is clearly evident that while the removal efficiency of the bioreactor remains almost stable, the elimination capacity increases linearly up to the inlet loading rate of 271.44 mg/L/d beyond which the removal efficiency starts decreasing noticeably and the elimination capacity of the bioreactor tends on increasing. This phenomenon has been discussed in various bioreactor studies carried out previously (Yadav et al., 2014; Briceño et al., 2020). In the present study, the inlet loading rate of 271.44 mg/L/d is the controlling step of the bioreactor that changes operation from mass transfer to bio-reaction controlling. Below the critical rate, diffusion of butachlor into the biomass is low, resulting in mass transfer limitations. Therein, the innermost layer of the biofilm may be depleted of the substrate, thus not utilizing the full potential of the microbial system. However, at higher loading rates, the system overcomes the mass transfer limitations since the diffusional flux is high and under this condition, the butachlor removal efficiency depends on the biodegradation efficiency of the biomass. At higher loading rate, lower removal efficiency may be the result of substrate inhibition or bacterial plugging and channeling due to excess biomass (Patel and Kumar 2016; Kureel et al., 2018). The present study proposes the range of 270–325 mg/L/d to be the optimum operating range for the removal of butachlor by the PBBR.
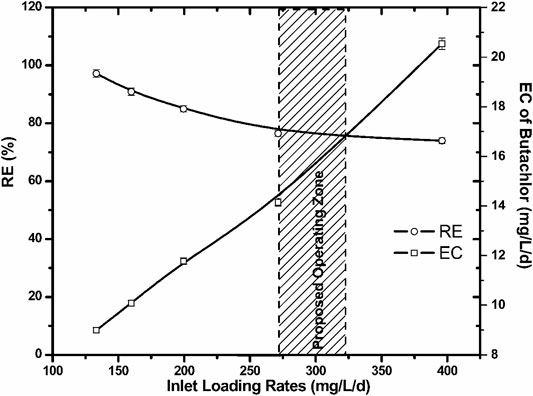
FIGURE 4. Influence of inlet butachlor loading on the removal efficiency and elimination capacity of the PBBR.
Effect of Initial Substrate Concentration on RE of the PBBR
In the present study, continuous biodegradation of butachlor fed at a constant flowrate of 2.77 ml/min was assessed for 25 days with the butachlor concentration varying from 500 to 2000 mg/L. Initial substrate concentration plays an important role in determining the performance evaluation of the continuous removal of the contaminant in a bioreactor. Wastewater generated from the industrial applications is prone to have shock loading on the biomass activity due to the inhibitory effect of the contaminants at higher concentrations. Hence, from the practical standpoint, evaluation of the shock loading operation of the PBBR for the treatment of butachlor is highly essential. Figure 5 demonstrates the effect of initial butachlor concentration on the removal efficiency for different initial butachlor concentrations. For an initial concentration of 500 mg/L of butachlor, the removal efficiency increased from 51 to 92% within 4 days and remained constant. On day 6, increasing the butachlor concentration to 750 mg/L, the removal efficiency sharply decreased to 50% and recovered to 87% by day 9, indicating the effect of shock loading on the bioreactor to be temporary. A similar trend of sharp dip followed by gradual increase in the RE till a steady point was observed when the butachlor concentration was increased to 1,000 mg/L, 1,500 mg/L, and 2000 mg/L. The result obtained in the present investigation indicates that the PBBR can adapt to shock loading pertaining to higher butachlor concentrations. This may be due to the formation of the biofilm on the support material. Formation of biofilm by the bacterial cells has been reported as one of the major adaptation strategies which has been reported for the purpose of bioremediation of various organic contaminants (Sahoo and Panigrahy 2018; Dash and Osborne 2020).
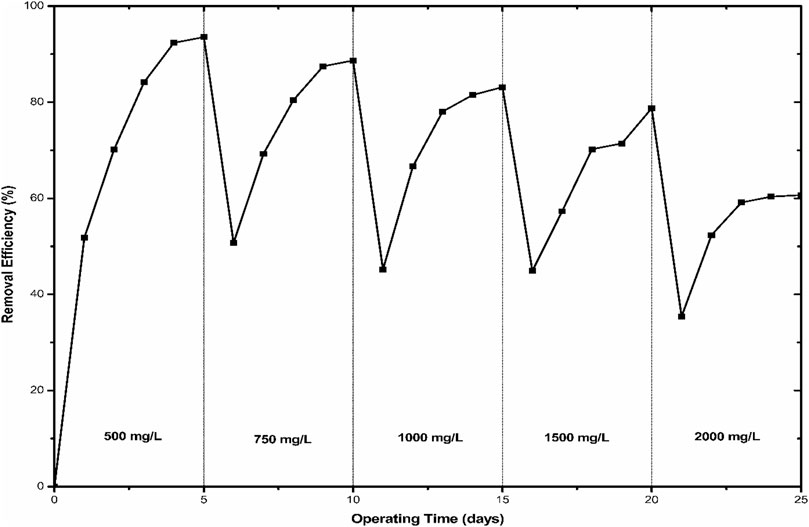
FIGURE 5. Effect of initial butachlor concentration on the removal efficiencies and elimination capacity of the PBBR.
Combined Removal of the Mixture of Herbicides
The previous study refers to the evaluation of the performance of the PBBR using a single-target contaminant, butachlor. However, in most of the cases, more than one contaminant occurs as a mixture in the environment, which may affect the removal efficiency of the bioreactor. Since, COD is a widely used parameter that is measured to assess the magnitude of pollution in any wastewater, in the present study, performance evaluation of the PBBR for the treatment of synthetic wastewater comprising the mixture of herbicides was investigated with respect to its COD removal efficiency (Figure 6). The removal efficiency of the PBBR was evaluated for various initial COD concentrations in a continuous mode to evaluate the simultaneous removal of the herbicides. In the present study, percentage of COD reduction was monitored as a function of time. Based on the results of the single-substrate system, the flow rate of the PBBR was maintained at 2.77 ml/min corresponding to the HRT of 24 h for the simultaneous removal of the mixture of pesticides from the wastewater. The figure indicates that at lower concentration, degradation efficiencies of the PBBR is considerably high, which decreases gradually with increasing concentrations. Though complete removal cannot be achieved, more than 90% removal efficiency is achieved at stage 1, where the COD level of the influent was maintained at 500 mg/L. The COD removal efficiency was further reduced to less than 80% on increasing the COD level of the influent to 1,000 mg/L. A similar trend of gradual decrease in the COD removal efficiency with increasing concentration of the pollutant in the synthetic wastewater was observed previously in various studies (Alfonso-Gordillo et al., 2016b; Arikan et al., 2019). The relative underperformance of the PBBR for the herbicide mixture as compared to the single-substrate system may be attributed to the increased toxicity due to the presence of multiple herbicides in the influent that inhibits the normal metabolic activities of the microbial strain (Arikan et al., 2019). However, the PBBR demonstrates excellent COD removal efficiency and projects itself as a potential alternative to treat wastewater generating from the herbicide industries.
Parameter Optimization Using RSM
The available literature survey evidenced that three independent variables, that is, inlet feed flow rate, initial substrate concentration, and additional biogenic substrate concentration have a significant effect on the COD removal efficiency of the bioreactor (Patel and Kumar 2016; Nguyen et al., 2017). Hence, in the present study, the aforementioned parameters were optimized by RSM to yield maximum COD removal by the bioreactor. The design matrix of the subjected variables and the experimental results are being presented in Table 1. The significance of each variable and the model was determined by their probability value and the correlation value, respectively (Supplementary Table ST1). The results revealed the statistically significant (p < 0.05) effects of influent feed flow rate and initial substrate concentration on COD removal efficiency of the PBBR. Regardless of their significance, all the terms (both linear and quadratic) have been included in the following second-order polynomial equation:
where RE represents COD removal efficiency; FFR represents feed flow rate; ISC represents initial substrate concentration; and ANS represents additional biogenic substrate concentration.
A regression model is usually considered statistically accurate, highly correlated, and reproducible when the coefficient of correlation (R2) value is higher than 0.9 (Bakkiyaraj et al., 2016). The R2 value of 0.9018 indicates a good agreement between the experimental and the predicted values, which can be verified from the normal probability plot of the residual (Supplementary Figure S3). The adjusted and predicted R2 values were found to be 0.9616 and 0.9846, respectively. The “Lack of Fit” of 0.0687 suggests that the Lack of Fit is nonsignificant compared to the pure error. The model is found to be competent for prediction within the range of variables used. The mutual interaction among the variables and their effect on the COD removal efficiency of the PBBR were determined by mapping three-dimensional response surface plots where the function of two variables was observed while retaining the other variable constant at the center point (Figure 7). The shape of the plot evidently indicates the prominence of the involved parameters and the significance of the mutual interaction among them. From the figures and from the ANOVA table, it is highly evident that feed flow rate and substrate concentration play a major role in determining the COD removal efficiency of the PBBR. Previous studies pertaining to optimization of the process parameters have reported the significance of substrate concentration and feed flow rate on the COD removal efficiency of the packed-bed bioreactors (Dev et al., 2017; Pandey and Sarkar 2019). It is an established concept that the feed flow rate of the influent has the higest impact in determining the efficiency of the PBBR (Mohanty et al., 2021). The feed flow rate determines the retention time of the pollutant within the bioreactor. The more the retention time, the more opportunities obtained by the to metabolize the pollutant efficiently. However, an exceedingly low feed flow rate may also lead to mass transfer limitations, which may result in lower removal percentage (Yadav et al., 2014). Similarly, up to a certain initial concentration, the substrate may act as the nutrient for the microbial biomass; however, increase in the substrate concentration may confer the toxic effect of the herbicides on the metabolic activity of the microbial biomass, thus resulting in a low removal competency. The desirability function thus predicted that maximum COD removal can be achieved at the optimum range of a feed flow rate of 2.9 ml/min, substrate concentration of 454.63 mg/L, and concentration of additional nitrogen source at 1.41 g/L. The proposed model was validated experimentally, and it was found that the difference between the predicted and actual COD removal rate was less that 3%, thus indicating the adequacy of the model.
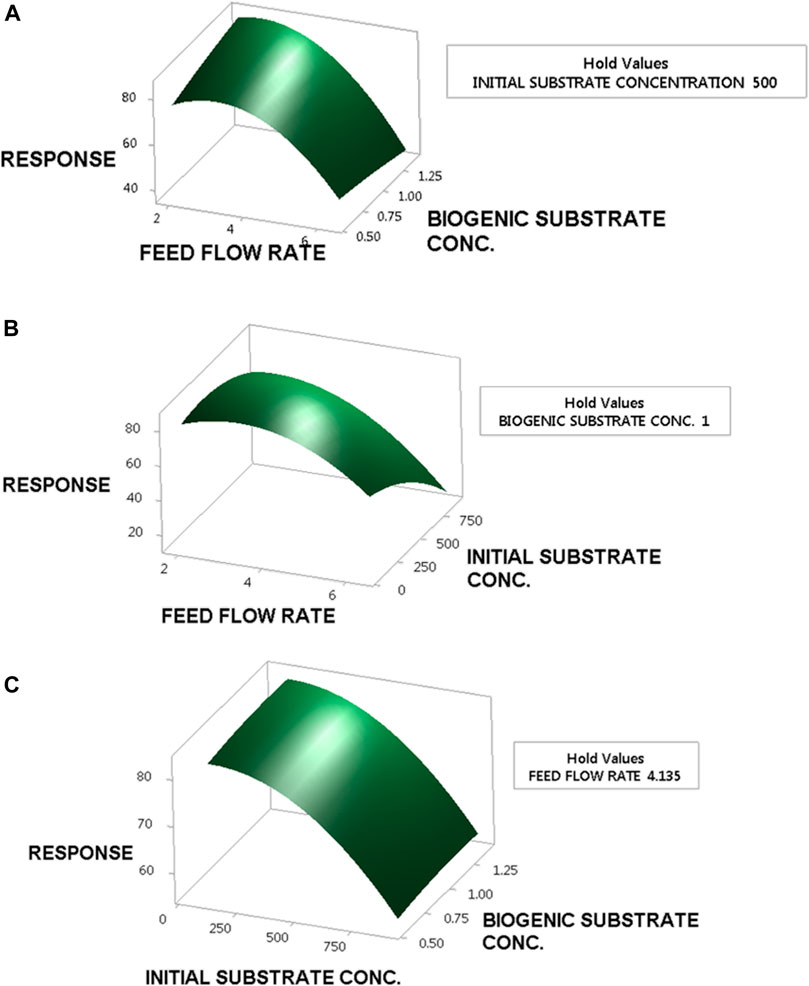
FIGURE 7. Three-dimensional plot of COD removal efficiency as a function of (A) feed flow rate and additional biogenic substrate concentration; (B) feed flow rate and initial substrate concentration; (C) initial substrate concentration and additional biogenic substrate concentration.
Conclusion
The present study aims to achieve high butachlor removal efficiency both as a single-substrate system and in a mixture of herbicides from wastewater using microbial consortium SMC1 immobilized on ceramic raschig rings in a continuous vertical flow packed-bed biofilm reactor system. It was observed that both the removal efficiency and the elimination capacity of the system are affected by the retention time, inlet loading rates and the intial substrate concentration of the wastewater. The bioreactor system is also capable of treating the mixture of herbicides efficiently and reduces the overall COD concentration of the wastewater. Optimization of the operating parameters such as feed flow rate, substrate concentrations, and additional biogenic substances that have a major effect on the performance of the bioreactor was successfully carried out using RSM. The removal efficiency obtained in the PBBR system was found to be superior to the previously studied bio-systems. Bioremediation of chloroacetanalide class of herbicides in a continuous-system bioreactor has been one of the least explored fields of study. Hence, the present study demonstrates the feasibility of developing a large-scale biofilm-mediated bioreactor for the effective treatment of wastewater from herbicide industries.
Data Availability Statement
The raw data supporting the conclusion of this article will be made available by the authors, without undue reservation.
Author Contributions
SM conceived the presented idea, carried out the experiment, performed the analyses, and wrote the manuscript. HJ supervised the investigation and verified the findings of this work. All authors discussed the results and contributed to the final version of the manuscript.
Conflict of Interest
The authors declare that the research was conducted in the absence of any commercial or financial relationships that could be construed as a potential conflict of interest.
Publisher’s Note
All claims expressed in this article are solely those of the authors and do not necessarily represent those of their affiliated organizations, or those of the publisher, the editors, and the reviewers. Any product that may be evaluated in this article, or claim that may be made by its manufacturer, is not guaranteed or endorsed by the publisher.
Supplementary Material
The Supplementary Material for this article can be found online at: https://www.frontiersin.org/articles/10.3389/fceng.2022.721923/full#supplementary-material
References
Abigail, M. E. A., Samuel, S. M., and Ramalingam, C. (2015). Addressing the Environmental Impacts of Butachlor and the Available Remediation Strategies: a Systematic Review. Int. J. Environ. Sci. Technol. 12, 4025–4036. doi:10.1007/s13762-015-0866-2
Acuna-Askar, K., Englande, A. J., Hu, C., and Jin, G. (2000). Methyl Tertiary-Butyl Ether (MTBE) Biodegradation in Batch and Continuous Upflow Fixed-Biofilm Reactors. Water Sci. Technol. 42, 153–161. doi:10.2166/wst.2000.0509
Alfonso-Gordillo, G., Cristiani-Urbina, E., Flores-Ortiz, C. M., Peralta, H., Cancino-Díaz, J. C., Cruz-Maya, J. A., et al. (2016a). Stenotrophomonas Maltophilia Isolated from Gasoline-Contaminated Soil Is Capable of Degrading Methyl Tert-Butyl Ether. Electron. J. Biotechnol. 23, 12–20. doi:10.1016/j.ejbt.2016.06.006
Alfonso-Gordillo, G., Flores-Ortiz, C. M., Morales-Barrera, L., and Cristiani-Urbina, E. (2016b). Biodegradation of Methyl Tertiary Butyl Ether (MTBE) by a Microbial Consortium in a Continuous Up-Flow Packed-Bed Biofilm Reactor: Kinetic Study, Metabolite Identification and Toxicity Bioassays. PLoS One 11, e0167494. doi:10.1371/journal.pone.0167494
Annett, R., Habibi, H. R., and Hontela, A. (2014). Impact of Glyphosate and Glyphosate-Based Herbicides on the Freshwater Environment. J. Appl. Toxicol. 34, 458–479. doi:10.1002/jat.2997
Arikan, E. B., Isik, Z., Bouras, H. D., and Dizge, N. (2019). Investigation of Immobilized Filamentous Fungi for Treatment of Real Textile Industry Wastewater Using up Flow Packed Bed Bioreactor. Bioresour. Tech. Rep. 7, 100197. doi:10.1016/J.BITEB.2019.100197
Atwood, D., and Paisley-Jones, C. (2017). Pesticides Industry Sales and Usage https://www.epa.gov/sites/production/files/2017-01/documents/pesticides-industry-sales-usage-2016_0.pdf.
Bakkiyaraj, S., Syed, M. B., Devanesan, M. G., and Thangavelu, V. (2016). Production and Optimization of Biodiesel Using Mixed Immobilized Biocatalysts in Packed Bed Reactor. Environ. Sci. Pollut. Res. 23, 9276–9283. doi:10.1007/s11356-015-4583-7
Briceño, G., Levio, M., González, M. E., Saez, J. M., Palma, G., Schalchli, H., et al. (2020). Performance of a Continuous Stirred Tank Bioreactor Employing an Immobilized Actinobacteria Mixed Culture for the Removal of Organophosphorus Pesticides. 3 Biotech. 10, 252. doi:10.1007/s13205-020-02239-9
Chattopadhyay, A., Adhikari, S., Adhikary, S. P., and Ayyappan, S. (2006). Evaluation of Butachlor for Control of Submerged Macrophytes along with its Impact on Biotic Components of Freshwater System. Iran J. Environ. Heal Sci. Eng. 3, 103–108.
Chen, C.-Y., Wang, G.-H., Tseng, I.-H., and Chung, Y.-C. (2016). Analysis of Bacterial Diversity and Efficiency of Continuous Removal of Victoria Blue R from Wastewater by Using Packed-Bed Bioreactor. Chemosphere 145, 17–24. doi:10.1016/j.chemosphere.2015.11.061
Choudhury, P. P., Singh, R., Ghosh, D., and Sharma, A. R. (2016). Herbicide Use in Indian Agriculture. Technical report. doi:10.13140/RG.2.2.20822.75848
Dash, D. M., and Osborne, W. J. (2020). Rapid Biodegradation and Biofilm-Mediated Bioremoval of Organophosphorus Pesticides Using an Indigenous Kosakonia Oryzae Strain -VITPSCQ3 in a Vertical-Flow Packed Bed Biofilm Bioreactor. Ecotoxicology Environ. Saf. 192, 110290. doi:10.1016/J.ECOENV.2020.110290
Dev, S., Roy, S., and Bhattacharya, J. (2017). Optimization of the Operation of Packed Bed Bioreactor to Improve the Sulfate and Metal Removal from Acid Mine Drainage. J. Environ. Manage. 200, 135–144. doi:10.1016/J.JENVMAN.2017.04.102
Dzionek, A., Wojcieszyńska, D., and Guzik, U. (2016). Natural Carriers in Bioremediation: A Review. Electron. J. Biotechnol. 23, 28–36. doi:10.1016/j.ejbt.2016.07.003
Ercan, D., and Demirci, A. (2015). Current and Future Trends for Biofilm Reactors for Fermentation Processes. Crit. Rev. Biotechnol. 35, 1–14. doi:10.3109/07388551.2013.793170
Foroughi, M., Sarabi Jamab, M., Keramat, J., and Najaf Najafi, M. (2019). The Use ofSaccharomyces Cerevisiaeimmobilized on Activated Alumina, and Alumina Silicate Beads for the Reduction of Aflatoxin M1In Vitro. J. Food Process. Preserv 43, e13876. doi:10.1111/JFPP.13876
Gianessi, L. P. (2005). Economic and Herbicide Use Impacts of Glyphosate-Resistant Crops. Pest Manag. Sci. 61, 241–245. doi:10.1002/ps.1013
Kaur, R., and Goyal, D. (2020). Biodegradation of Butachlor by Bacillus Altitudinis and Identification of Metabolites. Curr. Microbiol. 77, 2602–2612. doi:10.1007/s00284-020-02031-1
Khalid, S., and Hashmi, I. (2016). Biotreatment of Chlorpyrifos in a Bench Scale Bioreactor Using Psychrobacter Alimentarius T14. Environ. Tech. 37, 316–325. doi:10.1080/09593330.2015.1069406
Kureel, M. K., Geed, S. R., Rai, B. N., and Singh, R. S. (2018). Novel Investigation of the Performance of Continuous Packed Bed Bioreactor (CPBBR) by Isolated Bacillus Sp. M4 and Proteomic Study. Bioresour. Tech. 266, 335–342. doi:10.1016/j.biortech.2018.06.064
Lauga, B., Girardin, N., Karama, S., Le Ménach, K., Budzinski, H., and Duran, R. (2013). Removal of Alachlor in Anoxic Soil Slurries and Related Alteration of the Active Communities. Environ. Sci. Pollut. Res. 20, 1089–1105. doi:10.1007/s11356-012-0999-5
Liu, H., Cao, L., Lu, P., Ni, H., Li, X. Y., Yan, X., et al. (2012). Biodegradation of Butachlor by Rhodococcus Sp. Strain B1 and Puri Fi Cation of its Hydrolase (ChlH) Responsible for N - Dealkylation of Chloroacetamide Herbicides. J. Agric. Food Chem. 60, 12238–12244. doi:10.1021/jf303936j
Liu, Z., He, Y., Xu, J., Huang, P., and Jilani, G. (2008). The Ratio of clay Content to Total Organic Carbon Content Is a Useful Parameter to Predict Adsorption of the Herbicide Butachlor in Soils. Environ. Pollut. 152, 163–171. doi:10.1016/j.envpol.2007.05.006
Mohanty, S. R., Nayak, D. R., Babu, Y. J., and Adhya, T. K. (2004). Butachlor Inhibits Production and Oxidation of Methane in Tropical rice Soils under Flooded Condition. Microbiol. Res. 159, 193–201. doi:10.1016/j.micres.2004.03.004
Mohanty, S. S., and Jena, H. M. (2019a). A Systemic Assessment of the Environmental Impacts and Remediation Strategies for Chloroacetanilide Herbicides. J. Water Process Eng. 31, 100860. doi:10.1016/j.jwpe.2019.100860
Mohanty, S. S., and Jena, H. M. (2019c). Degradation Kinetics and Mechanistic Study on Herbicide Bioremediation Using Hyper Butachlor-Tolerant Pseudomonas Putida G3. Process Saf. Environ. Prot. 125, 172–181. doi:10.1016/j.psep.2019.03.014
Mohanty, S. S., and Jena, H. M. (2019b). Evaluation of Butachlor Biodegradation Efficacy of Serratia Ureilytica Strain AS1: a Statistical Optimization Approach. Int. J. Environ. Sci. Technol. 16, 5807–5816. doi:10.1007/s13762-018-1958-6
Mohanty, S. S., and Jena, H. M. (2018). Process Optimization of Butachlor Bioremediation by Enterobacter cloacae Using Plackett Burman Design and Response Surface Methodology. Process Saf. Environ. Prot. 119, 198–206. doi:10.1016/j.psep.2018.08.009
Mohanty, S. S., Reddy, D. K., and Jena, H. M. (2021). Mass Transfer Study of Butachlor Biodegradation Using Immobilized Microbial Consortium SMC1 in a Packed Bed Bioreactor. Can. J. Chem. Eng. 100, 1–9. doi:10.1002/cjce.24118
Muter, O., Perkons, I., Svinka, V., Svinka, R., and Bartkevics, V. (2017). Distinguishing the Roles of Carrier and Biofilm in Filtering media for the Removal of Pharmaceutical Compounds from Wastewater. Process Saf. Environ. Prot. 111, 462–474. doi:10.1016/j.psep.2017.08.010
Nguyen, P. Y., Carvalho, G., Reis, A. C., Nunes, O. C., Reis, M. A. M., and Oehmen, A. (2017). Impact of Biogenic Substrates on Sulfamethoxazole Biodegradation Kinetics by Achromobacter Denitrificans Strain PR1. Biodegradation 28, 205–217. doi:10.1007/s10532-017-9789-6
Orme, S., and Kegley, S. (2004). Alachlor - Toxicity, Ecological Toxicity and Regulatory Information PAN Pestic Database - Chem. AvaliableAt: http://www.pesticideinfo.org/Detail_Chemical.jsp?Rec_Id=PC35160#Toxicity.
Pandey, S., and Sarkar, S. (2019). Performance Evaluation and Substrate Removal Kinetics of an Anaerobic Packed-Bed Biofilm Reactor. Int. J. Environ. Res. 13, 223–233. doi:10.1007/S41742-019-00168-X
Patel, B. P., and Kumar, A. (2016). Biodegradation of 2,4-dichlorophenol in Packed-Bed Biofilm Reactor: Effect of Hydraulic Retention Time, Biogenic Substrate, and Loading Rate. Water Environ. Res. 88, 2191–2197. doi:10.2175/106143016X14733681695726
Polard, T., Jean, S., Gauthier, L., Laplanche, C., Merlina, G., Sánchez-Pérez, J. M., et al. (2011). Mutagenic Impact on Fish of Runoff Events in Agricultural Areas in South-West France. Aquat. Toxicol. 101, 126–134. doi:10.1016/j.aquatox.2010.09.014
Sahoo, N. K., and Panigrahy, N. (2018). Biodegradation and Kinetic Study of 4-Chlorophenol in Bioreactor Packed with Stabilized Bacteria Entrapped in Calcium Alginate Beads System. Environ. Process. 5, 287–302. doi:10.1007/S40710-018-0294-7/FIGURES/5
Shukla, S. K., Mangwani, N., Rao, T. S., and Das, S. (2014). “Biofilm-Mediated Bioremediation of Polycyclic Aromatic Hydrocarbons,” in Microbial Biodegradation and Bioremediation. Editor S. Das (New Delhi, Elsevier: Elsevier BV), 203–232. doi:10.1016/b978-0-12-800021-2.00008-x
Shuman-Goodier, M. E., Singleton, G. R., Forsman, A. M., Hines, S., Christodoulides, N., Daniels, K. D., et al. (2021). Developmental Assays Using Invasive Cane Toads, Rhinella marina, Reveal Safety Concerns of a Common Formulation of the rice Herbicide, Butachlor. Environ. Pollut. 272, 115955. doi:10.1016/J.ENVPOL.2020.115955
Sonwani, R. K., Giri, B. S., Jaiswal, R. P., Singh, R. S., and Rai, B. N. (2020). Performance Evaluation of a Continuous Packed Bed Bioreactor: Bio-Kinetics and External Mass Transfer Study. Ecotoxicology Environ. Saf. 201, 110860–110868. doi:10.1016/j.ecoenv.2020.110860
Sung Wang, M.-H., Wang, L. K., and De Michele, E. (2017). “Principles, Procedures, and Heavy Metal Management of Dichromate Reflux Method for COD Determination in Laboratories,” in Handbook of Advanced Industrial and Hazardous Wastes Management. L. K. Wang, M. H. Wang, and Y. T. Hung. (Washington, Taylor & Francis: CRC Press), 809–818. doi:10.1201/9781315117423-24
Swain, G., Sonwani, R. K., Singh, R. S., Jaiswal, R. P., and Rai, B. N. (2021). Removal of 4-Chlorophenol by Bacillus Flexus as Free and Immobilized System: Effect of Process Variables and Kinetic Study. Environ. Tech. Innovation 21, 101356. doi:10.1016/J.ETI.2021.101356
University of Hertfordshire (2013). “PPDB: Pesticide Properties DataBase,” in Pestic. Prop. Database Website Database. Hatfield, Hertfordshire: University of Hertfordshire.
Keywords: biofilm, bioreactor, butachlor, chemical oxygen demand (COD), response surface methodology, bioremediation
Citation: Mohanty SS and Jena HM (2022) Biodegradation of Herbicide by the Immobilized Microbial Consortium SMC1 in Continuous Packed-Bed Biofilm Reactor. Front. Chem. Eng. 4:721923. doi: 10.3389/fceng.2022.721923
Received: 07 June 2021; Accepted: 08 April 2022;
Published: 01 June 2022.
Edited by:
Kun-Yi Andrew Lin, National Chung Hsing University, TaiwanReviewed by:
Jianlong Wang, Tsinghua University, ChinaWillian Garcia Birolli, Barretos Cancer Hospital, Brazil
Copyright © 2022 Mohanty and Jena. This is an open-access article distributed under the terms of the Creative Commons Attribution License (CC BY). The use, distribution or reproduction in other forums is permitted, provided the original author(s) and the copyright owner(s) are credited and that the original publication in this journal is cited, in accordance with accepted academic practice. No use, distribution or reproduction is permitted which does not comply with these terms.
*Correspondence: Hara Mohan Jena, aG1qZW5hQG5pdHJrbC5hYy5pbg==, aGFyYS5qZW5hQGdtYWlsLmNvbS5jb20=