- 1Key Laboratory of Artificial Organs and Computational Medicine in Zhejiang Province, Shulan International Medical College, Zhejiang Shuren University, Hangzhou, China
- 2State Key Laboratory for Diagnosis and Treatment of Infectious Diseases, National Clinical Research Center for Infectious Diseases, National Medical Center for Infectious Diseases, Collaborative Innovation Center for Diagnosis and Treatment of Infectious Diseases, The First Affiliated Hospital, Zhejiang University School of Medicine, Hangzhou, China
- 3Hangzhou Tongchuang Medical Laboratory, Department of pathology, Hangzhou, China
- 4Key Laboratory of Artificial Organs and Computational Medicine in Zhejiang Province, Shulan (Hangzhou) Hospital Affiliated to Shulan International Medical College, Zhejiang Shuren University, Hangzhou, China
- 5Department of Breast Surgery, Renji Hospital, School of Medicine, Shanghai Jiao Tong University, Shanghai, China
Hepatocellular Carcinoma (HCC) is a malignant tumor with high morbidity and mortality worldwide, which represents a serious threat to human life, health and quality of life. Blood-based detection is essential for HCC screening, early diagnosis, prognosis evaluation, and surveillance. Current non-invasive detection strategy including serum alpha-fetoprotein (AFP), ultrasound, computerized tomography, and magnetic resonance imaging. The limited specificity of an AFP and the dependence on operator experience and diagnostic personnel for ultrasound have constrained their utility in early HCC diagnosis. In recent years, with the development of various detection technologies, there has been an increasing focus on exploring blood-based detection markers for HCC. The types of markers include protein markers, DNA mutation, DNA epigenetic modification, mRNA, miRNA, and so on. However, numerous methodological and biological factors limit the clinical sensitivity and generalization performance of these new biomarkers. In this review, we describe the state-of-the-art technologies for cfDNA analysis, and discuss outstanding biological and technical challenges that, if addressed, would substantially improve HCC diagnostics and patient care.
1 Introduction
Liver cancer is one of the most common cancers, the sixth most common cancer in the world, with >860,000 new cases and >750,000 deaths in 2022 (Bray et al., 2024), and the fifth most common cancer in China (Zhang et al., 2021). Primary liver cancers include hepatocellular carcinoma (HCC) (75%–85% of cases) and intrahepatic cholangiocarcinoma (10%–15% of cases), as well as other rare types. The onset of liver cancer is occult, lacking typical clinical symptoms in the early stage, and many patients are already in the advanced stage when they are found, losing the opportunity for surgery. However, systemic chemotherapy or radiotherapy is not ideal for primary liver cancer, immune-checkpoint inhibitor (ICI)-based therapies only ∼30% of advanced-stage HCC patients have an objective response, and liver cancer has become the third highest mortality cancer. In most countries, liver cancer survival rates have barely improved from 1995 to 2022, and the 5-year survival rate remains far below 20% (Villanueva, 2019). Even in the United States, where medical conditions are better, liver cancer death rates have been rising while mortality rates for other types of cancer have declined (Bray et al., 2018). More than half of the world’s liver cancer cases and deaths occur in China. In recent years, data show that the incidence and mortality of liver cancer are on the rise globally, and the incidence of men is twice that of women (Rumgay et al., 2022).
2 Risk factors for HCC
The main risk factors for liver cancer are chronic infection with hepatitis B virus (HBV) or hepatitis C virus (HCV), aflatoxin-contaminated food, heavy alcohol consumption, obesity, smoking and type 2 diabetes. Major risk factors vary by region. In most high-risk HCC regions (China, East Africa), the key risks are chronic HBV infection and aflatoxin exposure, while in other countries (Japan, Egypt, etc.), HCV infection may be the main cause. In Mongolia, HBV and HCV and co-infection of HBV carriers with HCV or hepatitis E virus (HEV), as well as alcohol abuse, contribute to the high incidence of liver cancer. The increase in obesity is also responsible for the increased incidence of low-risk liver cancer. Studies have found that diabetes can increase the risk of cancer in hepatitis B patients by 2 to 3 folds. Patients with a glycated hemoglobin level of more than 9% and chronic liver disease (alcoholic liver injury, cirrhosis, HBV or HCV infection, and other chronic liver disease) have a higher risk of liver cancer. In addition, diabetes can significantly reduce the survival rate and increase the recurrence rate of liver cancer (Alexander et al., 2019; Pang et al., 2018). In addition to control of HBV infection, HBV vaccination might avert the development of liver cancer and reduce the risk of death due to liver cancer. Therefore, HBV vaccination was recommended as primary prevention of liver cancer since 1982. WHO recommends its incorporation into routine infant immunization programme and by the end of 2016, 186 countries had introduced HBV vaccine into heir national immunization schedules. The vaccine has dramatically reduced the prevalence of HBV infection and the incidence of HCC in young people in high-risk countries where mass vaccination is being introduced. However, there is currently no vaccine to prevent HCV infection. Although HCV transmission has declined substantially in resource-rich countries, the continued use of contaminated needles and unsafe blood transfusions in some low-income countries are also important contributors to the spread of infection. Recent advances in anti-HBV and HCV therapy suggest that, although currently expensive, HCC risk can be significantly reduced (Kanwal et al., 2017; Yang et al., 2022).
Metabolic fatty liver disease (MFLD) not only increases the risk of HCC but also HCC-related mortality, but it does not increase the risk of HCC recurrence or all-cause mortality, suggesting the need for interventions in the MFLD population and for HCC surveillance in patients with metabolic steatohepatitis and fibrosis (Cholankeril and Kanwal, 2021; Norero and Dufour, 2023; Orci et al., 2022; Thomas et al., 2024).
Understanding these risk factors is helpful for risk stratification of the population to improve the precision of screening and save medical resources.
3 Implications of blood-based biomarker
In recent years, tremendous progress has been made in the treatment of HCC. However, all treatment options are only feasible if diagnosed early. According to the 2019 Cancer Report of the United States of America, the key to reducing cancer mortality is early detection and treatment of cancer, which has almost become common knowledge in the field of cancer (Siegel et al., 2019). HCC surveillance is of high value in high-risk individuals, for example, hepatitis virus carriers, patients with cirrhosis (Singal et al., 2022). However, the insidious onset and atypical symptoms of liver cancer make it difficult to detect early liver cancer. Finding reliable biomarkers for early diagnosis of liver cancer has become a key way for early detection of liver cancer (Parikh et al., 2020). Early HCC screening/diagnostic biomarkers should have the following characteristics (Kimhofer et al., 2015):
1) The target marker should be detectable in noninvasively obtained samples such as blood or urine;
2) The marker should have good diagnostic and/or prognostic performance (e.g., high sensitivity and specificity);
3) The markers should be able to be tested with reliable and stable equipment, and the test results should be interpreted in the field according to a simple format without additional equipment; This standard essentially excludes Next-generation sequencing (NGS) technology;
4) Testing should be inexpensive so that it is available to all who need it;
5) Biomarkers should be validated in a wide range of populations.
Additionally, we believe that prospective studies should be conducted in populations with hepatitis virus infection to verify the performance of markers for early screening of HCC before conducting applicability studies in all populations (Sachar et al., 2021).
4 Current guidelines and consensus
Early diagnosis of liver cancer is of great significance, and domestic and foreign guidelines emphasize early screening and detection of liver cancer. Serum alpha-fetoprotein (AFP) and liver ultrasound are the two most commonly used methods for liver cancer screening. The main serological biomarker of liver cancer is serum AFP, which is the most commonly used diagnostic method at present. The positive diagnostic cut-off value of serum AFP is >400 ng/mL. It is worth noting that the main application of AFP detection is in diagnosis, rather than screening and surveillance. The test typically has a sensitivity of only about 40%–60%, a rate of false-negative of 30%–40%, a specificity of 80%–90% (Huang et al., 2022; 2023; Yang et al., 2019). AFP can also be elevated in chronic hepatitis, liver cirrhosis and other diseases, which can easily lead to false positive results when used for tumor screening. This is also an important reason why the American Association for the Study of Liver Diseases (AASLD) guidelines and the European Association for the Study of the Liver and the European Organization for Research and Treatment of Cancer no longer use AFP as a screening index (Bruix et al., 2011; Galle et al., 2018; Singal et al., 2023). However, AFP serological test is still widely used as a screening index for HCC in the Asia-Pacific region. The Asian-Pacific Association for the Study of the Liver (APASL) recommends surveillance for HBV-infected men older than 40 and women older than 50: Liver ultrasound and AFP detection should be performed every 6 months, and areas where conditions are available can consider increasing the screening frequency or other tumor markers and imaging examinations, such as DCP, AFP-L3, CT/MRI, etc. (Omata et al., 2010). In addition, Tong et al. (2010) suggested that surveillance for liver cancer can improve the survival of patients with hepatitis B and should be included in the standard medical regimen for patients.
The European Association for the Study of the Liver and the European Organization for Research and Treatment of Cancer jointly recommend the detection of heat shock protein (HSP70), glypican (GPC3) and glutamine synthetase (GS) to improve the early diagnosis rate of HCC (Galle et al., 2018). The International Consensus Group of Hepatocellular Neoplasia and the World Health Organization (WHO) has also included these three tests in their recommendations (Nagtegaal et al., 2020).
In summary, the main problems of the currently widely used liver cancer screening programs are insufficient sensitivity, low accuracy, and poor compliance due to invasiveness (Zeng et al., 2023). There is an urgent need to develop more accurate and less invasive detection methods that can detect smaller tumor size and be suitable for general population screening. In theory, detection of circulating tumor cells (CTC), circulating cell-free DNA (ccfDNA), circulating RNA (mRNA, miRNA, lncRNA), circulating proteins or exosomes are potential target markers (Parikh et al., 2023).
5 Blood-based biomarkers of liver cancer
In addition to traditional biomarkers, AFP, newer blood-based biomarkers such as ctDNA, microRNAs, circulating tumor cells, and exosomes are being investigated for their utility in HCC diagnosis and prognosis. These biomarkers offer the potential for more accurate and sensitive detection of HCC, as well as monitoring of treatment response and disease progression (Figure 1).
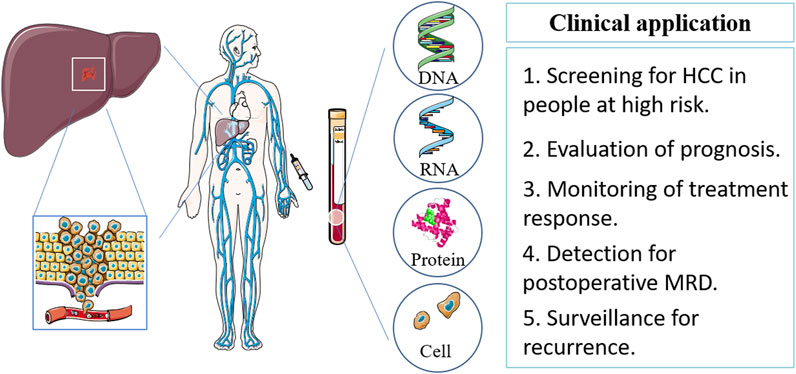
Figure 1. Blood-based biomarker sources, types and clinical implications in HCC. HCC, hepatocellular carcinoma; MRD, minimal residual disease.
5.1 Protein markers
5.1.1 Serum alpha-fetoprotein (AFP)
Serum protein markers is most current clinical practice of blood markers for HCC, and new protein markers are emerging due to the development of proteomics technology (Table 1). The most widely used HCC biomarker is AFP that sensitivity for HCC range from 40% to 65%. Semiannual AFP screening of HBV carriers can lead to earlier diagnosis of associated HCC, but has no effect on five-year survival rate (Chen et al., 2003). Randomized controlled trials have shown that screening HBV carriers and patients with chronic hepatitis with AFP and ultrasound twice a year can reduce HCC-related mortality. Compared with the control group, the detection rate of HCC in the early and operable stages was significantly improved in the screening group (Zhang et al., 2004). Three kinds of AFP with different glycosyl modifications have been identified, among which AFP-L3 was more specific than AFP. Although the sensitivity of AFP-L3 detection is controversial, Oda et al. (2011) confirmed the clinical application value of AFP-L3 detection using a highly sensitive automatic immune system (Kagebayashi et al., 2009).
5.1.2 Serum prothrombin induced by vitamin K absence-Ⅱ (PIVKA-Ⅱ)
Several studies have found another useful protein biomarker is prothrombin induced by vitamin K absence-Ⅱ (PIVKA-Ⅱ), also known as Des-γ-carboxyprothrombin (DCP). It can promote the proliferation of HCC cells. The sensitivity of DCP for detecting HCC ranges from 48% to 62%, depending on the study and the stage of the cancer. Combined detection of other markers can further improve the sensitivity and specificity of early HCC diagnosis (Bae et al., 2011; Kamel et al., 2014; Poté et al., 2015; Xing et al., 2018).
5.1.3 Serum glypican-3 (GPC3)
Studies have reported that the level of serum Glypican-3 (GPC3) in HCC patients is increased, which can accurately distinguish early HCC from cirrhosis, which secsitivity around 50%–72% (Hippo et al., 2004). Tangkijvanich et al. (2010) found that the serum GPC3 level was increased in HCC patients, which was not related to AFP. Combined detection of the two proteins can improve the sensitivity of early HCC detection. It has also been reported that combined detection of GPC3 and Heat shock protein 70 or heat shock protein 70 (HSP70) glutamine synthetase can more sensitively and specifically distinguish early or grade 1 HCC from cirrhosis (Silvia et al., 2012). These serum protein markers are generally cost-effective compared to other diagnostic tests. However, the conventional detection technology of protein sensitivity is not high, and protein biomarker lack of specificity.
5.1.4 Others
New protein markers are still emerging. Some studies have also found that the Alpha-l-fucosidase (AFU) was elevated in serum of patients with cancer, included HCC (El-Houseini et al., 2005; Wang and Cao, 2004). That implicate its low specificity. DKK1 (dickkopf-1) protein was reported first time as a biomarker for HCC in 2012 (Shen et al., 2012). Its sensitivity of DKK1 protein for early HCC and small HCC was 70.9% and 58.5%, and the specificity was 90.5% and 84.7% (Shen et al., 2012). Liu Jie’s team from Fudan University obtained DKK1 aptamers with different dissociation rates in serum samples, and expected to replace DKK1 capture antibody of enzyme-linked immunoassay in early HCC detection (Zhou et al., 2019). Laminin (Ln)-332 contains three strands, α3, β3, and γ2. Recently, Japanese scientists have found that Ln-γ2/Ln-322 plays an important role in the occurrence and development of HCC, and Ln-γ2 monomer can be used as a potential biomarker to diagnose early HCC (Kiyokawa et al., 2017). This team evaluated the diagnostic value of Ln-γ2, AFP, and PIVKA-II in differentiating patients with chronic liver diseases (CLD) from HCC. The results showed that Ln-γ2 was more effective than AFP in differentiating CLD from HCC (Yasuda et al., 2019). In addition, such as Cytokeratin 19 (CK19), Golgi protein 73 (GP73), Osteopontin (OPN), heparin binding cytokines (Midkine, MDK) and Annexin A2 have also been shown to have the potential as early HCC diagnostic markers.
5.2 5-methylation-cyanine (5 mc) DNA biomarkers
Some new cfDNA markers we summarized in Table 2. Among them, aberrant 5 mc DNA methylation alterations, including hypermethylation of CpG islands (CGIs) and extensive hypomethylation, are a hallmark of HCC. DNA methylation is one of the most well-studied epigenetic modification mechanisms. It widely exists in the mammalian genome, and the level and pattern of genome methylation are specific in different tissues. DNA methylation detection technology has experienced comprehensive development in the era of high-throughput gene sequencing (Table 2).
5.2.1 Genomic level identification of cfDNA methylation markers
In the study of clinical application of liquid biopsy (Zeng et al., 2019), cfDNA methylation has been studied most extensively (Feng et al., 2019; Guo et al., 2017; Kang et al., 2017; Leygo et al., 2017; Xu et al., 2017; Zeng et al., 2018). In HCC, the 5-mc biomarkers of ctDNA has shown better diagnostic (such as serum AFP) and prognostic value (such as TNM staging) than current clinical indicators (Xu et al., 2017). In addition, repetitive sequences, such as long interspersed nuclear elements (LINE-1) and Alu repeats, are proxies for genomic methylation (Barchitta et al., 2014). In diffuse large B-cell lymphoma, DNA methylation of free LINE-1 shows a strong correlation with clinical outcomes, indicating its potential application as a prognostic marker (Wedge et al., 2017). Another approach to finding 5 mc markers is to identify tumor-specific methylated haplotypes as cell-free DNA markers to assess tumor burden and tumor origin sites (Guo et al., 2017). Multiple CpG haplotypes are more effective in tumor typing than single CpG markers (Guo et al., 2017). Recently, other types of epigenetic signatures, such as 5-hydroxymethylation (5-hmc) of cfDNA and nucleosome positioning and proportion, have also been used to assess tumor location and tumor (Chun-Xiao et al., 2017; Ivanov et al., 2015; Li et al., 2017; Snyder et al., 2016). Although the genome wide distribution of nucleosomes in cfDNA provides valuable information to identify the organizational origin of chaos in cfDNA, its clinical utility has been understudied.
Shen et al. (2018) from the University Health Network in Toronto, Canada, reported a study using MeDIP-Seq technology combined with machine learning to analyze the tumor methylation pattern of cfDNA. The investigators compared 300 patient tumor samples from seven disease sites (lung, pancreatic, colorectal, breast, leukemia, bladder, and kidney) with samples from healthy donors and analyzed methylation patterns in circulating cfDNA in plasma to track cancer origin and type. Although this study did not include liver cancer, it shows the potential of cfMeDIP-Seq for early cancer detection and localization. Nassiri et al. (2020) used cfMeDIP-seq technology to identify cfDNA methylation markers of intracranial tumors. Using cfMeDIP-seq, differentially hemi-methylated regions could serve as independent diagnostic biomarkers for HCC (Hua et al., 2024).
5.2.2 Specific methylated genes or loci
Wong et al. (2000) and Wong et al. (1999) used Methylation specific PCR (MSP) to show that abnormal methylation of CDKN2A and CDKN2B genes, which encode p16 and p15 proteins, could be easily detected in the blood of HCC patients. The hypermethylation analysis of RASSF1A (ras association domain family 1A) in HCC patients showed that the sensitivity was 92.5% (37/40) in tissue and 42.5% (17/40) in plasma. RASSF1A hypermethylation was not detected in tissue samples, nor in plasma DNA samples (Yeo et al., 2005). Feng et al. (2010) used fluorescence quantitative PCR method (Methylight) to detect the methylation levels of 10 genes in liver tissues with 65 different viral infection states and found that the methylation levels of 5 genes such as ACP, CDKN2A, HOXA9, RASSF1 and RUNX were significantly increased in malignant liver tissues. The methylation levels of HOXA9, RASSF1 and SFRP1 were higher in HBV-positive HCC samples. In contrast, CDKN2A was more methylated in HCV-positive HCC samples. The researchers used MSP to analyze 24 tumor suppressor genes and 3 cancer methylation sites in HCC tissues and adjacent tissues as well as chronic hepatitis tissues, and found that 8 tumor suppressor genes, including HIC1, GSTP1, SOCS1, RASSF1, CDKN2A, APC, RUNX3 and PRDM2, were differentially expressed. Methylation levels are significantly elevated in early-stage HCC tissues and are associated with HCC progression in chronic hepatitis C. Therefore, it is speculated that the methylation changes of these genes play an important role in the early stage of cancer and are potential biomarkers for assessing the risk of chronic hepatitis C carcinogenesis (Nishida et al., 2012). Researchers also found that the methylation levels of different TSGS changed differently with the progression of HCC. The methylation levels of some tumor suppressor genes, including RASSF2, MINT1, MINT2, RPRAM, SFRP2, CDH1 and DCC, changed more in the early stage of HCC than in the advanced stage. However, CASP8, MINT31, PTGS2 and CACNA1G are the genes with methylation changes mainly in the high stage of liver cancer (Nishida et al., 2012). Zhou et al. (2018) conducted a meta-analysis of CDKN2A gene promoter region methylation and HCC risk. The results showed that the CDKN2A gene promoter region was hypermethylated in HCC, and its methylation level was significantly positively correlated with the risk of HCC (OR, 7.07; 95% CI, 5.67–8.80). In addition, this methylation is also positively correlated with HBV and HCV infection, the degree of cirrhosis, and age, which has the possibility of being HCC biomarker (Zhou et al., 2018). A study using bisulfite sequencing technology to analyze HCV-related liver tissues found that the promoter regions of Wnt suppressor genes SFRP2 and DKK1 were hypermethylated in the precancerous stages of chronic HCV infection, leading to Wnt silencing and eventual HCC development. The promoter region of SFRP2 has the highest methylation level in HCC tissues, followed by cirrhosis tissues and chronic hepatitis tissues, and the lowest methylation level in normal liver tissues (Umer et al., 2014). Using methylation chip and MSP technology, researchers found 24 abnormally methylated CpG promoter regions, among which 4 hypermethylated genes (TNFRSF10C, HOXA9, NPY and IRF5) were downregulated in tumor tissues (Shin et al., 2010). Hernandez-Vargas et al. (2010) also used the same technical method to identify the unique methylation characteristics of HCC, which can distinguish HCC tumor tissues from paracancerous tissues and other types of tumor tissues. Furthermore, these specific methylation signatures are independent risk factors for cancer progression. Using Illumina Human Methylation 27K chip, Ammerpohl et al. (2012) detected and analyzed HCC (N = 12), cirrhosis (N = 15) and normal liver tissues (N = 12), and found that compared with normal liver tissues, A total of 167 hypomethylation sites and 100 hypermethylation sites associated with liver cirrhosis maintained a similar methylation pattern in HCC. Further analysis of the distribution of methylation levels in functional regions (promoter structures, PRC2-binding domains and CpG islands) across the genome revealed that the methylation patterns of cirrhosis and HCC were significantly different. A 450K methylation chip analysis was performed on 800 HCC patients, and 90% of HCC tissues showed hypomethylation or CpG island methylation phenotype at the genome-wide level. Similarly, another study found that most of the significantly hypermethylated CpG sites (60.1%) in liver cancer tissues were located on CpG islands, while the significantly hypomethylated CpG sites located on CpG islands accounted for only 8.2% of all hypomethylated CpG sites (SHI et al., 2016). Guo et al. (2017) performed genomic methylation analysis on ∼800 HCC samples, and selected 10 sites from 80 hypermethylated promoter sites as biomarkers of tumor and non-tumor tissues, with sensitivity and specificity close to 100%.
The scientists investigated the application of aberrant methylation of cfDNA in peripheral blood for the diagnosis of liver cancer. Zhang et al. (2007) used MSP technology to detect the methylation of P15, P16 and RASSF1A in serum samples collected repeatedly before the diagnosis of liver cancer patients and normal people. The results showed that RASSF1A hypermethylation was detected at least once in 35 (70%) of 50 HCC patients, while p15 and p16 hypermethylation were detected in 22 (44%) and 12 (22%) of 50 HCC patients, respectively. The sensitivity and specificity of serum DNA methylation of these three genes as early predictive markers of HCC were 84% and 94%, respectively. SHI et al. (2016) analyzed the methylation differences between HCC patients’ cancer and adjacent tissues by methylation chip, and screened 2,324 differentially methylated CpG sites, including 684 hypermethylated sites and 1,640 hypomethylated sites in cancer tissues. Bisulfite Genomic Sequence (BSP) was used to detect the methylation levels of five genes (CDKL2, STEAP4, HIST1H3G, CDKN2A and ZNF154) in plasma samples from 38 patients. The feasibility of detecting plasma DNA methylation levels was confirmed. Wen et al. (2015) used MCTA-Seq to detect the cfDNA of 151 liver cancer patients and normal human controls, and found dozens of loci that could identify patients with early liver cancer. A classification model for plasma DNA identification of HCC patients achieved 94% sensitivity and 89% specificity. Wu et al. (2017) also used BSP to detect the methylation level of six genes (CDKN2A, RASSF1A, STEAP4, TBX2, VIM and ZNF154) in plasma DNA. The results showed that TBX2 hypermethylation in plasma DNA was associated with an increased risk of HCC with an average odds ratios (ORs) of 3.2 (95% CI). Zhao et al. (2024) identified two novel methylation biomarkers, GNB4 and Riplet through a genome-wide discovery, and showed sensitivity of 84.39% for any-stage HCC detection in plasma samples. Ruihua Xu from Sun Yat-sen University and Kang Zhang from University of California San Diego used Bisulfite padlock probes (BSPP) to detect cfDNA methylation. Ten early diagnosis and therapeutic related sites and eight prognostic related sites were identified from more than 400,000 candidate sites. The methylation levels of these ten early diagnosis sites showed a diagnostic sensitivity of 84.8% and a specificity of 93.1% in a total of 1098 HCC patients and 835 healthy controls. It can also accurately predict tumor staging, efficacy and recurrence (Xu et al., 2017). Methylation of RNF135 and LDHB in cfDNA could be detected by Methylation Sensitive High-Resolution Analysis (MS-HRM) and could be used for HCC liquid biopsy (Kim D. Y. et al., 2023).
5.3 5-hydroxymethylcytosine (5 hmC) biomarkers
As one of the most important DNA modifications discovered in recent years, 5-hydroxymethylcytosine (5 hmC) is currently considered to be closely related to gene regulation and tumor pathogenesis, and can be used as a marker for early diagnosis of tumors. Unlike 5-methylcytosine (5 mC), which inhibits gene expression, 5 hmC is widely regarded as a marker of activated gene expression.
Li et al. (2016) used oxBS-Seq (oxidative bisulfite sequencing) to obtain methylation and hydroxymethylation profiles with single base resolution in the genome, and found that the enrichment characteristics of 5 hmc modification were tumor and tissue specific.
Starting with chemical marker detection technology, Professor He developed 5 hmc-seal technology with high sensitivity and stability, which can stably reproduce the sample detection under the requirements of trace DNA. Li et al. (2017) studied the molecular characteristics of 5 hmc in 90 healthy people, 260 cancer patients (including 80 colorectal cancer, 71 gastric cancer, 25 liver cancer, 34 pancreatic cancer, 46 thyroid cancer) and 71 benign gastrointestinal diseases. 5 hmC sequences in tissue and peripheral blood cfDNA were captured by 5 hmc-SEAL technology, and 5 hmC modification levels were analyzed by high-throughput sequencing technology. After normalization of 5 hmc modification levels, bioinformatics tools were used to find 5 hmC gene loci with significant differences in modification levels between the patient group and the normal group. Subsequently, the regression model was used to further screen and optimize the difference sites obtained as above, so as to obtain a set of 5 hmC biomarkers with high sensitivity and specificity. The obtained biomarkers were further validated in independent samples. This study reveals the potential of 5 hmc as a molecular marker for clinical application, especially in early cancer screening and cancer localization and classification.
At the same time, it was also explored that the value of cfDNA 5 hmC for tumor diagnosis (Chun-Xiao et al., 2017). A total of 49 patients with primary tumors were included in the study, including 15 lung cancer, 10 HCC, 7 pancreatic cancer, 4 glioma, 5 gastric cancer, 4 colorectal cancer and 4 breast cancer samples. It was found that tSNE dimensionality reduction analysis of the enrichment degree of all genes 5 hmC could distinguish HBV infection, HCC and healthy human samples. HCC-specific hypermethylated and hypomethylated genes can be used to distinguish HCC, some HBV samples and healthy samples. The high hydroxymethylated genes characteristic of HCC are also highly expressed in liver tissue, which is also consistent with the permissive effect of 5 hmC on gene expression. Subsequently, Stephen R Quake and Dan Xie’s team also collected blood samples from 4 HCC patients after surgery, as well as samples from 3 recurrent HCC patients, and found that the postoperative samples returned to the level of healthy samples, while the changes of markers in recurrent HCC samples were similar to those in HCC samples. Similarly, the change trend of 5 hmC enriched gene AHSG and lacking gene TET2 can also be used to observe the change of 5 hmC in the short term after surgery until recurrence. These results suggest that cfDNA 5 hmC sequencing technology has the potential to be used in the detection, diagnosis, treatment and recurrence monitoring of liver cancer.
Jia Fan from Zhongshan Hospital, Fudan University and Hongyang Wang from National Liver Cancer Science Center/Eastern Hepatobiliary Surgery Hospital, together with Chuan He from the University of Chicago and Wei Zhang from Northwestern University, further studied the application value of peripheral blood 5 hmC technology in the early diagnosis and screening of liver cancer. Genome-wide mapping of 5 hmc in cell-free DNA samples was performed in 2,544 subjects (1,204 with HCC, 392 with chronic hepatitis B or cirrhosis, 958 with healthy or benign liver damage). A case-control analysis using elastic network regularization for feature selection developed a model using 32 genes for early HCC diagnosis. The results showed that the 5 hmC model not only accurately distinguished patients with early-stage liver cancer from healthy controls, but also could distinguish patients with early-stage liver cancer from patients with chronic hepatitis B or cirrhosis, and its prediction performance was significantly better than that of AFP. However, combining 5 hmC markers with AFP can further improve the performance of AUC (Cai et al., 2019). Liu et al. (2019) found that in the early stage of HCC, 5 hmc and 5 fmc modification levels were significantly reduced, mainly due to the reduction of 5 mc modification levels, and were related to HBV infection, reduced TET enzyme activity, and abnormal expression of DNA methylation-related enzymes.
Hlady et al. (2019) integrated and analyzed the genomic epigenetic modifications of liver cancer, cirrhosis and normal liver tissues, including the histone modifications of 5 mc, 5 hmc and the promoter/enhancer regions of four genes (H3K4me1, H3K27ac, H3K3me3 and H3K27me3). The results demonstrated that these modifications jointly regulated gene expression and subsequently affected the proliferation of HCC cells. By integrated analysis of epigenetic modifications, two epigenetic driver sites of HCC, COMT and FMO3, were also identified.
5.4 Markers of somatic mutation
The initiation and development of tumors are essentially caused by the activation of proto-oncogenes and the inactivation of tumor suppressor genes. For example, the Ser249 site of tumor suppressor gene TP53 is the most reported hotspot mutation in HCC (Szymańska et al., 2004). Mutations at this site cause the tp53 protein to lose its ability to bind specifically to DNA. It has been reported that TP53 Ser249 mutation in plasma DNA is associated with cirrhosis and HCC (Hosny et al., 2008; Kirk et al., 2005). This mutation was also detected in paracancerous tissues of HCC patients, suggesting that it may be involved in the early development of HCC and accumulated during the progression of HCC (Aguilar et al., 1994). Like many other point mutations, the Ser249 variant in TP53 has been found in samples from other cancer types. In recent years, cancer genome studies have found that most HBV-related HCC patients have at least one mutation in TP53, CTNNB1, AXIN1 and TERT promoter, and most of these mutations appear in the early stage of liver cancer, showing their close relationship with liver cancer development (Totoki et al., 2014; Zhang et al., 2017). Other somatic mutations that may be involved in the occurrence of liver cancer include ARID1A, ARID2, NFE2L2, KEAP1, JAK1 and RPS6KA3 (Guichard et al., 2012; Kan et al., 2013; Nault et al., 2014; Schulze et al., 2015; Totoki et al., 2014).
5.5 Other molecular features of cfDNA serve as markers
Professor Lo of the Chinese University of Hong Kong has led a team of researchers to discover the DNA in the plasma of patients with HCC by massively parallel sequencing technology. The results confirmed the existence of DNA fragments with abnormal lengths in the plasma of HCC patients. The shorter DNA molecules are more likely to carry some tumor-associated copy number abnormalities. It has also been found that the amount of mitochondrial DNA in the plasma of HCC patients is increased. These mtDNA molecules are shorter than plasma nuclear derived DNA (Jiang et al., 2015). In another study, the same team found that ccfDNA with breakpoints at specific locations in the genome was most likely derived from liver cancer cells. This finding reveals the possibility that plasma DNA breakpoint signatures can be used as biomarkers for liquid biopsy that are easier to detect than somatic mutation markers (Jiang et al., 2018). Wen et al. (2015) found that the concentration of cfDNA in liver cancer patients varies greatly and is independent of tumor size. Yan et al. (2018) found that the total amount of cfDNA in HCC patients was significantly higher than that in non-patients. With the constructed HCC diagnostic model including age, cfDNA content, and AFP, 87% sensitivity and 100% specificity could be achieved. In recently, fragmentomics patterns of cfDNA were investigated and provides new insights into the biological properties and potential clinical applications of noninvasive detection of HCC (An et al., 2023; Che et al., 2024; Deng et al., 2023; Gonçalves et al., 2022; Liu et al., 2023; Xu et al., 2024).
5.6 Small RNA (miRNA) markers
MiRNAs are a class of small single-stranded RNAs of about 22 nt that are processed from transcripts of hairpin structures produced endogenously in cells. Chen et al. (2008) from Nanjing University found that a large number of miRNAs stably exist in serum and plasma, which constitute the main part of circulating nucleic acids in serum. These miRNAs have good tolerance to different temperatures, pH values, storage time, repeated freezing and thawing, etc., which may be related to the fact that miRNA is mostly bound to proteins and encapsulated in exosomes and protected (Cheng et al., 2014).
In recent years, numbers of studies have confirmed that the detection of miRNA expression levels in tissues and serum can be used for the diagnosis, prediction of pathological classification and prognosis of liver cancer, and has certain reference value in the screening and follow-up of liver cancer. Zhou et al. (2011) used chip technology to screen 723 miRNAs in 934 HBV-positive liver cancer patients, and designed a diagnostic panel containing 7 miRNAs, with a sensitivity and specificity of 68.6% and 90.1%, respectively. The accuracy of this method in distinguishing HCC from normal subjects, hepatitis from cirrhosis was 94.1%, 84.2% and 88.4%, respectively. An in vitro diagnostic kit for this method has been developed and approved by National Medical Products Administration (NMPA). Studies on exosomal miRNA expression levels found that miR-18a, miR-221, miR-222 and miR-224 were significantly increased in the serum of HBV-infected HCC patients (Hayes and Chayama, 2016; Sohn et al., 2015). Jin et al. (2019) also found 12 plasma miRNA markers highly expressed in HCC patients from more than 700 miRNAs.
5.7 Combined detection of multiple types of markers
Cohen et al. (2018) combined the results of somatic mutation detection based on single-molecule tags and protein markers (CA125, CEA, cancer antigen 19–9, prolactin, hepatocyte growth factor, etc.) in 8, and constructed the CancerSEEK model by comparing cancer patients and healthy controls. The results showed that 43 of 44 (98%) HCC patients were detectable. HCCscreen, which detects four point mutations and one HBV integration site in cfDNA in combination with two protein markers, was developed by several scientists from National Cancer Center/Cancer Hospital, Chinese Academy of Medical Sciences. It was named HCCscreen and its application in the early screening of liver cancer in a prospective cohort of HBV carriers. In this study, 331 asymptomatic HBV carriers with normal AFP and B ultrasound were screened and followed up for 6–8 months. The results showed that among the 24 positive samples, 4 cases were subsequently diagnosed as liver cancer. Due to the early detection, 4 patients were expected to get a good prognosis. None of the 307 participants who tested negative developed liver cancer. In this study, the HCCscreen combined detection scheme achieved 100% sensitivity, 94% specificity and 17% positive predictive value, which is expected to be applied to early screening of liver cancer (Qu et al., 2019). By DNA sequencer-assisted fluorophore-assisted carbohydrate electrophoresis, the blood glucose spectrum of patients with HCC were evaluated, and the log ratio of peak 9 to peak 7 was named the GlycoHCCTest (G-test). The G-test is a promising noninvasive method to screening HCC in patients with chronic hepatitis B and cirrhosis (de Oliveira et al., 2018; Wan et al., 2021), and obtained the NMPA Class III medical device registration certificate for non-invasive detection of HCC (Cong et al., 2020; DelaCourt et al., 2021). Integrating cfDNA methylation, copy number, and fragmentation using 950 plasma and 240 tissue samples, which could to facilitates multi-cancer (including HCC) early detection (Kim S. Y. et al., 2023). CAMP-B score (cirrhosis, age ≥50 years, male sex and platelet count <150,000/mm3/L) can be easily implemented in real-world clinical practice and helps stratify HCC risk in patients with CHB following HBsAg seroclearance (Lee et al., 2024). Its application in HCC screening needs further investigate.
6 Summary
Noninvasive detection of liver cancer is very important for improving the prognosis and quality of life of patients. There are still many shortcomings in the current screening methods in clinical application, and there is an urgent need for new assays that are more reliable, convenient and less expensive. Future research prospects focus on two aspects: a. Optimization and combined application of traditional markers; b. Development of novel blood markers.
DNA methylation has advantages as a biomarker and has been clinically applied in a variety of cancers. The advantages of DNA methylation over other markers such as proteins and RNA include: a. Higher Specificity: DNA methylation patterns can provide more specific information related to disease states compared to protein markers, which may have broader implications across various conditions. Stability: DNA methylation patterns are relatively stable in clinical samples, making them robust biomarkers for long-term storage and analysis compared to RNA, which can degrade more easily. Early Detection Potential: Changes in DNA methylation can occur early in disease progression, offering potential for early detection of conditions like hepatocellular carcinoma (HCC) before clinical symptoms manifest. Quantifiability: DNA methylation levels can be quantitatively measured with high precision, allowing for accurate monitoring of disease progression and treatment efficacy. Technological Accessibility: Advances in technology have made DNA methylation analysis increasingly accessible and cost-effective, facilitating its integration into clinical diagnostics. These advantages collectively highlight DNA methylation as a promising biomarker for improving diagnostic accuracy and clinical outcomes in diseases like HCC. There are many studies on the application of DNA methylation in the early screening, diagnosis, and postoperative monitoring of liver cancer, but there is still a lack of methylation markers that can be applied to clinical promotion. Finding sensitive and specific DNA methylation markers of liver cancer at the genomic level may be a feasible research scheme to realize its clinical application in early screening and early diagnosis.
Author contributions
JsZ: Writing–original draft. ZH: Data curation, Writing–original draft. XZ: Validation, Writing–original draft. YL: Visualization, Writing–original draft. XL: Data curation, Writing–original draft. JjZ: Data curation, Writing–original draft. JP: Writing–review and editing. HG: Supervision, Writing–review and editing.
Funding
The author(s) declare that financial support was received for the research, authorship, and/or publication of this article. This work was supported by the Startup fund for Advanced Talents from Zhejiang Shuren University (grant no. 2023R038) and the Key Laboratory of Artificial Organs and Computational Medicine in Zhejiang Province.
Acknowledgments
The authors apologize to the investigators and research laboratories whose original studies were not cited because of space limitations.
Conflict of interest
The authors declare that the research was conducted in the absence of any commercial or financial relationships that could be construed as a potential conflict of interest.
Publisher’s note
All claims expressed in this article are solely those of the authors and do not necessarily represent those of their affiliated organizations, or those of the publisher, the editors and the reviewers. Any product that may be evaluated in this article, or claim that may be made by its manufacturer, is not guaranteed or endorsed by the publisher.
Abbreviations
HCC, hepatocellular carcinoma; HBV, hepatitis B virus; HCV, hepatitis C virus; HEV, hepatitis E virus; MFLD, Metabolic fatty liver disease; AFP, alpha-fetoprotein; cfDNA, cell-free DNA; DMR, differentially methylated regions; NGS, Next-generation sequencing; qMSP, quantitative methylation-specific polymerase chain reaction; PBMC, peripheral blood mononuclear cell; PCR, polymerase chain reaction.
References
Abass, S. A., Abdel-Hamid, N. M., Elshazly, A. M., Abdo, W., and Zakaria, S. (2023). Oma1 and yme1l as a diagnostic panel in hepatocellular carcinoma. Yale J. Biol. Med. 96 (4), 443–454. doi:10.59249/BWBY8971
Aguilar, F., Harris, C. C., Sun, T., Hollstein, M., and Cerutti, P. (1994). Geographic variation of p53 mutational profile in nonmalignant human liver. Science 264 (5163), 1317–1319. doi:10.1126/science.8191284
Alexander, M., Loomis, A. K., van der Lei, J., Duarte-Salles, T., Prieto-Alhambra, D., Ansell, D., et al. (2019). Risks and clinical predictors of cirrhosis and hepatocellular carcinoma diagnoses in adults with diagnosed nafld: real-world study of 18 million patients in four european cohorts. BMC Med. 17 (1), 95. doi:10.1186/s12916-019-1321-x
Ammerpohl, O., Pratschke, J., Schafmayer, C., Haake, A., Faber, W., von Kampen, O., et al. (2012). Distinct dna methylation patterns in cirrhotic liver and hepatocellular carcinoma. Int. J. Cancer. 130 (6), 1319–1328. doi:10.1002/ijc.26136
An, Y., Zhao, X., Zhang, Z., Xia, Z., Yang, M., Ma, L., et al. (2023). Dna methylation analysis explores the molecular basis of plasma cell-free dna fragmentation. Nat. Commun. 14 (1), 287. doi:10.1038/s41467-023-35959-6
Angeli-Pahim, I., Chambers, A., Duarte, S., Soma, D., Beduschi, T., Sahin, I., et al. (2024). Methylated ctdna quantification: noninvasive approach to monitoring hepatocellular carcinoma burden. J. Am. Coll. Surg. 238 (4), 770–778. doi:10.1097/XCS.0000000000000939
Bae, H., Lee, J., Yoon, J., Kim, Y. J., Heo, D. S., and Lee, H. (2011). Protein induced by vitamin k absence or antagonist-ii production is a strong predictive marker for extrahepatic metastases in early hepatocellular carcinoma: a prospective evaluation. BMC Cancer 11 (1), 435. doi:10.1186/1471-2407-11-435
Barchitta, M., Quattrocchi, A., Maugeri, A., Vinciguerra, M., and Agodi, A. (2014). Line-1 hypomethylation in blood and tissue samples as an epigenetic marker for cancer risk: a systematic review and meta-analysis. PLoS One 9 (10), e109478. doi:10.1371/journal.pone.0109478
Bie, F., Wang, Z., Li, Y., Guo, W., Hong, Y., Han, T., et al. (2023). Multimodal analysis of cell-free dna whole-methylome sequencing for cancer detection and localization. Nat. Commun. 14 (1), 6042. doi:10.1038/s41467-023-41774-w
Bray, F., Ferlay, J., Soerjomataram, I., Siegel, R. L., Torre, L. A., and Jemal, A. (2018). Global cancer statistics 2018: globocan estimates of incidence and mortality worldwide for 36 cancers in 185 countries. CA A Cancer J. Clin. 68 (6), 394–424. doi:10.3322/caac.21492
Bray, F., Laversanne, M., Sung, H., Ferlay, J., Siegel, R. L., Soerjomataram, I., et al. (2024). Global cancer statistics 2022: globocan estimates of incidence and mortality worldwide for 36 cancers in 185 countries. CA-Cancer J. Clin. 74 (3), 229–263. doi:10.3322/caac.21834
Bruix, J., Sherman, M., and American, A. F. T. S. (2011). Management of hepatocellular carcinoma: an update. Hepatol. Baltim. Md. 53 (3), 1020–1022. doi:10.1002/hep.24199
Cai, J., Chen, L., Zhang, Z., Zhang, X., Lu, X., Liu, W., et al. (2019). Genome-wide mapping of 5-hydroxymethylcytosines in circulating cell-free dna as a non-invasive approach for early detection of hepatocellular carcinoma. Gut 68 (12), 2195–2205. doi:10.1136/gutjnl-2019-318882
Chalasani, N. P., Porter, K., Bhattacharya, A., Book, A. J., Neis, B. M., Xiong, K. M., et al. (2022). Validation of a novel multitarget blood test shows high sensitivity to detect early stage hepatocellular carcinoma. Clin. Gastroenterol. Hepatol. 20 (1), 173–182.e7. doi:10.1016/j.cgh.2021.08.010
Che, H., Jiang, P., Choy, L., Cheng, S. H., Peng, W., Chan, R., et al. (2024). Genomic origin, fragmentomics, and transcriptional properties of long cell-free dna molecules in human plasma. Genome Res. 34 (2), 189–200. doi:10.1101/gr.278556.123
Cheishvili, D., Wong, C., Karim, M. M., Kibria, M. G., Jahan, N., Das, P. C., et al. (2023). A high-throughput test enables specific detection of hepatocellular carcinoma. Nat. Commun. 14 (1), 3306. doi:10.1038/s41467-023-39055-7
Chen, G., Peng, F., Dong, X., Cai, Z., Li, Z., He, L., et al. (2023). Identification of tumor mutations in plasma based on mutation variant frequency change (mvfc). Mol. Oncol. 17 (9), 1871–1883. doi:10.1002/1878-0261.13498
Chen, J., Parkin, D. M., Chen, Q., Lu, J., Shen, Q., Zhang, B., et al. (2003). Screening for liver cancer: results of a randomised controlled trial in qidong, China. J. Med. Screen 10 (4), 204–209. doi:10.1258/096914103771773320
Chen, L., Wu, T., Fan, R., Qian, Y. S., Liu, J. F., Bai, J., et al. (2024). Cell-free dna testing for early hepatocellular carcinoma surveillance. EBioMedicine 100, 104962. doi:10.1016/j.ebiom.2023.104962
Chen, X., Ba, Y., Ma, L., Cai, X., Yin, Y., Wang, K., et al. (2008). Characterization of micrornas in serum: a novel class of biomarkers for diagnosis of cancer and other diseases. Cell Res. 18 (10), 997–1006. doi:10.1038/cr.2008.282
Cheng, L., Sharples, R., Scicluna, B., and Hill, A. (2014). Exosomes provide a protective and enriched source of mirna for biomarker profiling compared to intracellular and cell-free blood. J. Extracell. Vesicles 3. doi:10.3402/jev.v3.23743
Cholankeril, G., and Kanwal, F. (2021). Nafld and hcc: time to bridge the gap. Hepatology 74 (5), 2336–2338. doi:10.1002/hep.32025
Chun-Xiao, S., Senlin, Y., Li, M., Amanda, W., Yu, C., Yan, Z., et al. (2017). 5-hydroxymethylcytosine signatures in cell-free dna provide information about tumor types and stages. bioRxiv, 162081. doi:10.1101/162081
Cohen, J. D., Li, L., Wang, Y., Thoburn, C., Afsari, B., Danilova, L., et al. (2018). Detection and localization of surgically resectable cancers with a multi-analyte blood test. Sci. (New York, N.Y.) 359 (6378), 926–930. doi:10.1126/science.aar3247
Cong, M., Ou, X., Huang, J., Long, J., Li, T., Liu, X., et al. (2020). A predictive model using n-glycan biosignatures for clinical diagnosis of early hepatocellular carcinoma related to hepatitis b virus. OMICS 24 (7), 415–423. doi:10.1089/omi.2020.0055
Cowzer, D., White, J. B., Chou, J. F., Chen, P. J., Kim, T. H., Khalil, D. N., et al. (2023). Targeted molecular profiling of circulating cell-free dna in patients with advanced hepatocellular carcinoma. JCO Precis. Oncol. 7, e2300272. doi:10.1200/PO.23.00272
DelaCourt, A., Black, A., Angel, P., Drake, R., Hoshida, Y., Singal, A., et al. (2021). N-glycosylation patterns correlate with hepatocellular carcinoma genetic subtypes. Mol. Cancer Res. 19 (11), 1868–1877. doi:10.1158/1541-7786.MCR-21-0348
Deng, Z., Ji, Y., Han, B., Tan, Z., Ren, Y., Gao, J., et al. (2023). Early detection of hepatocellular carcinoma via no end-repair enzymatic methylation sequencing of cell-free dna and pre-trained neural network. Genome Med. 15 (1), 93. doi:10.1186/s13073-023-01238-8
de Oliveira, R. M., Ornelas Ricart, C. A., and Araujo Martins, A. M. (2018). Use of mass spectrometry to screen glycan early markers in hepatocellular carcinoma. Front. Oncol. 7, 328. doi:10.3389/fonc.2017.00328
Du, L., Wang, M., Li, H., Li, N., and Wang, F. (2022). Identification of ccl20 and lcn2 as efficient serological tools for detection of hepatocellular carcinoma. Dis. Markers 2022 (1), 7758735. doi:10.1155/2022/7758735
Du, Z., Liu, X., Wei, X., Luo, H., Li, P., Shi, M., et al. (2020). Quantitative proteomics identifies a plasma multi-protein model for detection of hepatocellular carcinoma. Sci. Rep. 10 (1), 15552. doi:10.1038/s41598-020-72510-9
El-Houseini, M. E., Mohammed, M. S., Elshemey, W. M., Hussein, T. D., Desouky, O. S., and Elsayed, A. A. (2005). Enhanced detection of hepatocellular carcinoma. Cancer control 12 (4), 248–253. doi:10.1177/107327480501200407
Feng, H., Jin, P., and Wu, H. (2019). Disease prediction by cell-free dna methylation. Brief. Bioinform. 20 (2), 585–597. doi:10.1093/bib/bby029
Feng, Q., Stern, J. E., Hawes, S. E., Lu, H., Jiang, M., and Kiviat, N. B. (2010). Dna methylation changes in normal liver tissues and hepatocellular carcinoma with different viral infection. Exp. Mol. Pathol. 88 (2), 287–292. doi:10.1016/j.yexmp.2010.01.002
Galle, P. R., Forner, A., Llovet, J. M., Mazzaferro, V., Piscaglia, F., Raoul, J., et al. (2018). Easl clinical practice guidelines: management of hepatocellular carcinoma. J. Hepatol. 69 (1), 182–236. doi:10.1016/j.jhep.2018.03.019
Gonçalves, E., Gonçalves-Reis, M., Pereira-Leal, J. B., and Cardoso, J. (2022). Dna methylation fingerprint of hepatocellular carcinoma from tissue and liquid biopsies. Sci. Rep. 12 (1), 11512. doi:10.1038/s41598-022-15058-0
Guichard, C., Amaddeo, G., Imbeaud, S., Ladeiro, Y., Pelletier, L., Maad, I. B., et al. (2012). Integrated analysis of somatic mutations and focal copy-number changes identifies key genes and pathways in hepatocellular carcinoma. Nat. Genet. 44 (6), 694–698. doi:10.1038/ng.2256
Guo, D. Z., Huang, A., Wang, Y. C., Zhou, S., Wang, H., Xing, X. L., et al. (2024). Early detection and prognosis evaluation for hepatocellular carcinoma by circulating tumour dna methylation: a multicentre cohort study. Clin. Transl. Med. 14 (5), e1652. doi:10.1002/ctm2.1652
Guo, S., Diep, D., Plongthongkum, N., Fung, H., Zhang, K., and Zhang, K. (2017). Identification of methylation haplotype blocks aids in deconvolution of heterogeneous tissue samples and tumor tissue-of-origin mapping from plasma dna. Nat. Genet. 49 (4), 635–642. doi:10.1038/ng.3805
Hayes, C. N., and Chayama, K. (2016). Micrornas as biomarkers for liver disease and hepatocellular carcinoma. Int. J. Mol. Sci. 17, 280. doi:10.3390/ijms17030280
Hernandez-Vargas, H., Lambert, M., Le Calvez-Kelm, F., Gouysse, G., McKay-Chopin, S., Tavtigian, S. V., et al. (2010). Hepatocellular carcinoma displays distinct dna methylation signatures with potential as clinical predictors. PLoS One 5 (3), e9749. doi:10.1371/journal.pone.0009749
Hippo, Y., Watanabe, K., Watanabe, A., Midorikawa, Y., Yamamoto, S., Ihara, S., et al. (2004). Identification of soluble nh2-terminal fragment of glypican-3 as a serological marker for early-stage hepatocellular carcinoma. Cancer Res. 64 (7), 2418–2423. doi:10.1158/0008-5472.CAN-03-2191
Hlady, R. A., Sathyanarayan, A., Thompson, J. J., Zhou, D., Wu, Q., Pham, K., et al. (2019). Integrating the epigenome to identify drivers of hepatocellular carcinoma. Hepatol. Baltim. Md. 69 (2), 639–652. doi:10.1002/hep.30211
Hosny, G., Farahat, N., Tayel, H., and Hainaut, P. (2008). Ser-249 tp53 and ctnnb1 mutations in circulating free dna of egyptian patients with hepatocellular carcinoma versus chronic liver diseases. Cancer Lett. 264 (2), 201–208. doi:10.1016/j.canlet.2008.01.031
Hua, X., Zhou, H., Wu, H. C., Furnari, J., Kotidis, C. P., Rabadan, R., et al. (2024). Tumor detection by analysis of both symmetric- and hemi-methylation of plasma cell-free dna. Nat. Commun. 15 (1), 6113. doi:10.1038/s41467-024-50471-1
Huang, C., Fang, M., Xiao, X., Wang, H., Gao, Z., Ji, J., et al. (2022). Validation of the galad model for early diagnosis and monitoring of hepatocellular carcinoma in Chinese multicenter study. Liver Int. 42 (1), 210–223. doi:10.1111/liv.15082
Huang, C., Xiao, X., Zhou, L., Chen, F., Wang, J., Hu, X., et al. (2023). Chinese expert consensus statement on the clinical application of afp/afp-l3%/dcp using galad and galad-like algorithm in hcc. J. Clin. Lab. Anal. 37 (23-24), e24990. doi:10.1002/jcla.24990
Ivanov, M., Baranova, A., Butler, T., Spellman, P., and Mileyko, V. (2015). Non-random fragmentation patterns in circulating cell-free dna reflect epigenetic regulation. BMC Genomics 16 (13), S1. doi:10.1186/1471-2164-16-S13-S1
Jiang, P., Chan, C. W. M., Chan, K. C. A., Cheng, S. H., Wong, J., Wong, V. W., et al. (2015). Lengthening and shortening of plasma dna in hepatocellular carcinoma patients. Proc. Natl. Acad. Sci. 112 (11), E1317–E1325. doi:10.1073/pnas.1500076112
Jiang, P., Sun, K., Tong, Y. K., Cheng, S. H., Cheng, T. H. T., Heung, M. M. S., et al. (2018). Preferred end coordinates and somatic variants as signatures of circulating tumor dna associated with hepatocellular carcinoma. Proc. Natl. Acad. Sci. 115 (46), E10925–E10933. doi:10.1073/pnas.1814616115
Jin, Y., Wong, Y. S., Goh, B. K. P., Chan, C. Y., Cheow, P. C., Chow, P. K. H., et al. (2019). Circulating micrornas as potential diagnostic and prognostic biomarkers in hepatocellular carcinoma. Sci. Rep. 9 (1), 10464. doi:10.1038/s41598-019-46872-8
Kagebayashi, C., Yamaguchi, I., Akinaga, A., Kitano, H., Yokoyama, K., Satomura, M., et al. (2009). Automated immunoassay system for afp–l3% using on-chip electrokinetic reaction and separation by affinity electrophoresis. Anal. Biochem. 388 (2), 306–311. doi:10.1016/j.ab.2009.02.030
Kamel, M. M., Saad, M. F., Mahmoud, A. A., Edries, A. A., and Abdel-Moneim, A. S. (2014). Evaluation of serum pivka-ii and mif as diagnostic markers for hcv/hbv induced hepatocellular carcinoma. Microb. Pathog. 77, 31–35. doi:10.1016/j.micpath.2014.10.009
Kan, Z., Zheng, H., Liu, X., Li, S., Barber, T. D., Gong, Z., et al. (2013). Whole-genome sequencing identifies recurrent mutations in hepatocellular carcinoma. Genome Res. 23 (9), 1422–1433. doi:10.1101/gr.154492.113
Kang, S., Li, Q., Chen, Q., Zhou, Y., Park, S., Lee, G., et al. (2017). Cancerlocator: non-invasive cancer diagnosis and tissue-of-origin prediction using methylation profiles of cell-free dna. Genome Biol. 18 (1), 53. doi:10.1186/s13059-017-1191-5
Kanwal, F., Kramer, J., Asch, S. M., Chayanupatkul, M., Cao, Y., and El-Serag, H. B. (2017). Risk of hepatocellular cancer in hcv patients treated with direct-acting antiviral agents. Gastroenterology 153 (4), 996–1005. doi:10.1053/j.gastro.2017.06.012
Ke, H., Yuan, R., Liu, H., Luo, M., Hu, H., Zhang, E., et al. (2023). Serum protein biomarkers for hcc risk prediction in hiv/hbv co-infected people: a clinical proteomic study using mass spectrometry. Front. Immunol. 14, 1282469. doi:10.3389/fimmu.2023.1282469
Kim, D. Y., Cho, E. H., Kim, J. S., Chie, E. K., and Kang, H. C. (2023). Plasma circulating cell-free dna in advanced hepatocellular carcinoma patients treated with radiation therapy. Vivo 37 (5), 2306–2313. doi:10.21873/invivo.13333
Kim, S., Kim, D., Cho, E. J., Lee, J., Kim, J., Kwon, C., et al. (2023). A circulating cell-free dna methylation signature for the detection of hepatocellular carcinoma. Mol. Cancer. 22 (1), 164. doi:10.1186/s12943-023-01872-1
Kim, S. Y., Jeong, S., Lee, W., Jeon, Y., Kim, Y., Park, S., et al. (2023). Cancer signature ensemble integrating cfdna methylation, copy number, and fragmentation facilitates multi-cancer early detection. Exp. and Mol. Med. 55 (11), 2445–2460. doi:10.1038/s12276-023-01119-5
Kimhofer, T., Fye, H., Taylor-Robinson, S., Thursz, M., and Holmes, E. (2015). Proteomic and metabonomic biomarkers for hepatocellular carcinoma: a comprehensive review. Br. J. Cancer. 112 (7), 1141–1156. doi:10.1038/bjc.2015.38
Kirk, G. D., Lesi, O. A., Mendy, M., Szymañska, K., Whittle, H., Goedert, J. J., et al. (2005). 249ser tp53 mutation in plasma dna, hepatitis b viral infection, and risk of hepatocellular carcinoma. Oncogene 24 (38), 5858–5867. doi:10.1038/sj.onc.1208732
Kiyokawa, H., Yasuda, H., Oikawa, R., Okuse, C., Matsumoto, N., Ikeda, H., et al. (2017). Serum monomeric laminin-γ2 as a novel biomarker for hepatocellular carcinoma. Cancer Sci. 108 (7), 1432–1439. doi:10.1111/cas.13261
Kuzuya, T., Kawabe, N., Hashimoto, S., Miyahara, R., Sawaki, A., Nakano, T., et al. (2022). Early changes in alpha-fetoprotein are a useful predictor of efficacy of atezolizumab plus bevacizumab treatment in patients with advanced hepatocellular carcinoma. Oncology 100 (1), 12–21. doi:10.1159/000519448
Lee, H. W., Yip, T. C., Wong, V. W., Lim, Y. S., Chan, H. L., Ahn, S. H., et al. (2024). Camp-b score predicts the risk of hepatocellular carcinoma in patients with chronic hepatitis b after hbsag seroclearance. Aliment. Pharmacol. Ther. 59 (10), 1223–1235. doi:10.1111/apt.17933
Leygo, C., Williams, M., Jin, H. C., Chan, M. W. Y., Chu, W. K., Grusch, M., et al. (2017). Dna methylation as a noninvasive epigenetic biomarker for the detection of cancer. Dis. Markers. 2017, 3726595. doi:10.1155/2017/3726595
Li, N., Huang, J., He, S., Zheng, Q., Ye, F., Qin, Z., et al. (2024). The development of a novel zeolite-based assay for efficient and deep plasma proteomic profiling. J. Nanobiotechnol. 22 (1), 164. doi:10.1186/s12951-024-02404-9
Li, W., Zhang, X., Lu, X., You, L., Song, Y., Luo, Z., et al. (2017). 5-hydroxymethylcytosine signatures in circulating cell-free dna as diagnostic biomarkers for human cancers. Cell Res. 27 (10), 1243–1257. doi:10.1038/cr.2017.121
Li, X., Liu, Y., Salz, T., Hansen, K., and Feinberg, A. (2016). Whole genome analysis of the methylome and hydroxymethylome in normal and malignant lung and liver. Genome Res. 26, 1730–1741. doi:10.1101/gr.211854.116
Liu, J., Jiang, J., Mo, J., Liu, D., Cao, D., Wang, H., et al. (2019). Global dna 5-hydroxymethylcytosine and 5-formylcytosine contents are decreased in the early stage of hepatocellular carcinoma. Hepatol. Baltim. Md. 69 (1), 196–208. doi:10.1002/hep.30146
Liu, R., Guo, D., Huang, A., Ma, C., Xu, M., Liu, Y., et al. (2023). Development and validation of a non-invasive cfdna targeted sequencing assay for early-stage hepatocellular carcinoma detection using cfdna methylation and fragmentomics. J. Clin. Oncol. 41 (16_Suppl. l), 4128. doi:10.1200/JCO.2023.41.16_suppl.4128
Nagtegaal, I. D., Odze, R. D., Klimstra, D., Paradis, V., Rugge, M., Schirmacher, P., et al. (2020). The 2019 who classification of tumours of the digestive system. Histopathology 76 (2), 182–188. doi:10.1111/his.13975
Nassiri, F., Chakravarthy, A., Feng, S., Shen, S. Y., Nejad, R., Zuccato, J. A., et al. (2020). Detection and discrimination of intracranial tumors using plasma cell-free dna methylomes. Nat. Med. 26 (7), 1044–1047. doi:10.1038/s41591-020-0932-2
Nault, J. C., Calderaro, J., Di Tommaso, L., Balabaud, C., Zafrani, E. S., Bioulac-Sage, P., et al. (2014). Telomerase reverse transcriptase promoter mutation is an early somatic genetic alteration in the transformation of premalignant nodules in hepatocellular carcinoma on cirrhosis. Hepatology 60 (6), 1983–1992. doi:10.1002/hep.27372
Nishida, N., Kudo, M., Nagasaka, T., Ikai, I., and Goel, A. (2012). Characteristic patterns of altered dna methylation predict emergence of human hepatocellular carcinoma. Hepatol. Baltim. Md. 56 (3), 994–1003. doi:10.1002/hep.25706
Norero, B., and Dufour, J. F. (2023). Should we undertake surveillance for hcc in patients with mafld? Ther. Adv. Endocrinol. Metab. 14, 20420188231160389. doi:10.1177/20420188231160389
Oda, K., Ido, A., Tamai, T., Matsushita, M., Kumagai, K., Mawatari, S., et al. (2011). Highly sensitive lens culinaris agglutinin-reactive α-fetoprotein is useful for early detection of hepatocellular carcinoma in patients with chronic liver disease. Oncol. Rep. 26 (5), 1227–1233. doi:10.3892/or.2011.1425
Okanoue, T., Yamaguchi, K., Shima, T., Mitsumoto, Y., Mizuno, M., Katayama, T., et al. (2022). Serum levels of immunoglobulin m-free inhibitors of macrophage/cd5l as a predictive and early diagnostic marker for nonalcoholic steatohepatitis-associated hepatocellular carcinoma. Hepatol. Res. 52 (12), 998–1008. doi:10.1111/hepr.13826
Omata, M., Lesmana, L. A., Tateishi, R., Chen, P., Lin, S., Yoshida, H., et al. (2010). Asian pacific association for the study of the liver consensus recommendations on hepatocellular carcinoma. Hepatol. Int. 4 (2), 439–474. doi:10.1007/s12072-010-9165-7
Orci, L. A., Sanduzzi-Zamparelli, M., Caballol, B., Sapena, V., Colucci, N., Torres, F., et al. (2022). Incidence of hepatocellular carcinoma in patients with nonalcoholic fatty liver disease: a systematic review, meta-analysis, and meta-regression. Clin. Gastroenterol. Hepatol. 20 (2), 283–292.e10. doi:10.1016/j.cgh.2021.05.002
Pang, Y., Kartsonaki, C., Turnbull, I., Guo, Y., Clarke, R., Chen, Y., et al. (2018). Diabetes, plasma glucose, and incidence of fatty liver, cirrhosis, and liver cancer: a prospective study of 0.5 million people. Hepatology 68 (4), 1308–1318. doi:10.1002/hep.30083
Parikh, N. D., Mehta, A. S., Singal, A. G., Block, T., Marrero, J. A., and Lok, A. S. (2020). Biomarkers for the early detection of hepatocellular carcinoma. Cancer Epidemiol. Biomarkers and Prev. 29 (12), 2495–2503. doi:10.1158/1055-9965.EPI-20-0005
Parikh, N. D., Tayob, N., and Singal, A. G. (2023). Blood-based biomarkers for hepatocellular carcinoma screening: approaching the end of the ultrasound era? J. Hepatol. 78 (1), 207–216. doi:10.1016/j.jhep.2022.08.036
Poté, N., Cauchy, F., Albuquerque, M., Voitot, H., Belghiti, J., Castera, L., et al. (2015). Performance of pivka-ii for early hepatocellular carcinoma diagnosis and prediction of microvascular invasion. J. Hepatol. 62 (4), 848–854. doi:10.1016/j.jhep.2014.11.005
Qu, C., Wang, Y., Wang, P., Chen, K., Wang, M., Zeng, H., et al. (2019). Detection of early-stage hepatocellular carcinoma in asymptomatic hbsag-seropositive individuals by liquid biopsy. Proc. Natl. Acad. Sci. 116 (13), 6308–6312. doi:10.1073/pnas.1819799116
Rumgay, H., Arnold, M., Ferlay, J., Lesi, O., Cabasag, C. J., Vignat, J., et al. (2022). Global burden of primary liver cancer in 2020 and predictions to 2040. J. Hepatol. 77 (6), 1598–1606. doi:10.1016/j.jhep.2022.08.021
Sachar, Y., Brahmania, M., Dhanasekaran, R., and Congly, S. E. (2021). Screening for hepatocellular carcinoma in patients with hepatitis B. Viruses 13, 1318. doi:10.3390/v13071318
Schulze, K., Imbeaud, S., Letouzé, E., Alexandrov, L. B., Calderaro, J., Rebouissou, S., et al. (2015). Exome sequencing of hepatocellular carcinomas identifies new mutational signatures and potential therapeutic targets. Nat. Genet. 47 (5), 505–511. doi:10.1038/ng.3252
Shen, Q., Fan, J., Yang, X., Tan, Y., Zhao, W., Xu, Y., et al. (2012). Serum dkk1 as a protein biomarker for the diagnosis of hepatocellular carcinoma: a large-scale, multicentre study. Lancet Oncol. 13 (8), 817–826. doi:10.1016/S1470-2045(12)70233-4
Shen, S. Y., Singhania, R., Fehringer, G., Chakravarthy, A., Roehrl, M. H. A., Chadwick, D., et al. (2018). Sensitive tumour detection and classification using plasma cell-free dna methylomes. Nature 563 (7732), 579–583. doi:10.1038/s41586-018-0703-0
Shi, X. W., Shi, B. H., Lyu, A. L., Zhang, F., Zhou, T. T., and Guo, X. (2016). Exploring genome-wide dna methylation profiles altered in kashin-beck disease using infinium human methylation 450 bead chips. Biomed. Environ. Sci. 29 (7), 539–543. doi:10.3967/bes2016.072
Shimizu, T., Sawada, T., Asai, T., Kanetsuki, Y., Hirota, J., Moriguchi, M., et al. (2022). Hepatocellular carcinoma diagnosis using a novel electrochemiluminescence immunoassay targeting serum igm-free aim. Clin. J. Gastroenterol. 15 (1), 41–51. doi:10.1007/s12328-021-01567-4
Shin, S., Kim, B., Suh, K., Jang, J., and Kang, G. (2010). Identification of novel methylation markers in hepatocellular carcinoma using a methylation array. FASEB J. 24 (S1), 712–749. doi:10.1096/fasebj.24.1_supplement.749.12
Siegel, R. L., Miller, K. D., and Jemal, A. (2019). Cancer statistics, 2019. CA-Cancer J. Clin. 69 (1), 7–34. doi:10.3322/caac.21551
Silvia, T., Alejandro, F., Loreto, B., Ramon, V., Luis, B., Maria, R., et al. (2012). Prospective validation of an immunohistochemical panel (glypican 3, heat shock protein 70 and glutamine synthetase) in liver biopsies for diagnosis of very early hepatocellular carcinoma. Gut 61 (10), 1481–1487. doi:10.1136/gutjnl-2011-301862
Singal, A. G., Haaland, B., Parikh, N. D., Ozbay, A. B., Kirshner, C., Chakankar, S., et al. (2022). Comparison of a multitarget blood test to ultrasound and alpha-fetoprotein for hepatocellular carcinoma surveillance: results of a network meta-analysis. Hepatol. Commun. 6 (10), 2925–2936. doi:10.1002/hep4.2045
Singal, A. G., Llovet, J. M., Yarchoan, M., Mehta, N., Heimbach, J. K., Dawson, L. A., et al. (2023). Aasld practice guidance on prevention, diagnosis, and treatment of hepatocellular carcinoma. Hepatology 78 (6), 1922–1965. doi:10.1097/HEP.0000000000000466
Singal, A. G., Zhang, E., Narasimman, M., Rich, N. E., Waljee, A. K., Hoshida, Y., et al. (2022). Hcc surveillance improves early detection, curative treatment receipt, and survival in patients with cirrhosis: a meta-analysis. J. Hepatol. 77 (1), 128–139. doi:10.1016/j.jhep.2022.01.023
Snyder, M. W., Kircher, M., Hill, A. J., Daza, R. M., and Shendure, J. (2016). Cell-free dna comprises an in vivo nucleosome footprint that informs its tissues-of-origin. Cell 164 (1), 57–68. doi:10.1016/j.cell.2015.11.050
Sogbe, M., Bilbao, I., Marchese, F. P., Zazpe, J., De Vito, A., Pozuelo, M., et al. (2024). Prognostic value of ultra-low-pass whole-genome sequencing of circulating tumor dna in hepatocellular carcinoma under systemic treatment. Clin. Mol. Hepatol. 30 (2), 177–190. doi:10.3350/cmh.2023.0426
Sohn, W., Kim, J., Kang, S. H., Yang, S. R., Cho, J., Cho, H. C., et al. (2015). Serum exosomal micrornas as novel biomarkers for hepatocellular carcinoma. Exp. and Mol. Med. 47 (9), e184. doi:10.1038/emm.2015.68
Sultan, M. Q., Charfeddine, B., and Hussain, A. A. (2023). Evaluation of the diagnostic performance of alpha-1-antitrypsin in early detection of hepatocellular carcinoma. Cell. Mol. Biol. 69 (14), 177–185. doi:10.14715/cmb/2023.69.14.29
Syriha, A., Pantzios, S., Mandilara, D., Galanis, P., Stathopoulou, I., Barla, G., et al. (2024). Diagnostic accuracy of serum protein induced by vitamin k absence (pivka-ii), serum a-fetoprotein and their combination for hepatocellular carcinoma among caucasian cirrhotic patients with diagnostic or non-diagnostic serum a-fetoprotein levels. Cancer Med. 13 (3), e6825. doi:10.1002/cam4.6825
Szymańska, K., Lesi, O. A., Kirk, G. D., Sam, O., Taniere, P., Scoazec, J., et al. (2004). Ser-249tp53 mutation in tumour and plasma dna of hepatocellular carcinoma patients from a high incidence area in the Gambia, west africa. Int. J. Cancer. 110 (3), 374–379. doi:10.1002/ijc.20103
Tangkijvanich, P., Chanmee, T., Komtong, S., Mahachai, V., Wisedopas, N., Pothacharoen, P., et al. (2010). Diagnostic role of serum glypican-3 in differentiating hepatocellular carcinoma from non-malignant chronic liver disease and other liver cancers. J. Gastroenterol. Hepatol. 25 (1), 129–137. doi:10.1111/j.1440-1746.2009.05988.x
Thomas, J. A., Kendall, B. J., El-Serag, H. B., Thrift, A. P., and Macdonald, G. A. (2024). Hepatocellular and extrahepatic cancer risk in people with non-alcoholic fatty liver disease. Lancet Gastroenterol. Hepatol. 9 (2), 159–169. doi:10.1016/S2468-1253(23)00275-3
Tong, M. J., Sun, H., Hsien, C., and Lu, D. S. K. (2010). Surveillance for hepatocellular carcinoma improves survival in asian-american patients with hepatitis b: results from a community-based clinic. Dig. Dis. Sci. 55 (3), 826–835. doi:10.1007/s10620-009-1059-y
Totoki, Y., Tatsuno, K., Covington, K. R., Ueda, H., Creighton, C. J., Kato, M., et al. (2014). Trans-ancestry mutational landscape of hepatocellular carcinoma genomes. Nat. Genet. 46 (12), 1267–1273. doi:10.1038/ng.3126
Umer, M., Qureshi, S. A., Hashmi, Z. Y., Raza, A., Ahmad, J., Rahman, M., et al. (2014). Promoter hypermethylation of wnt pathway inhibitors in hepatitis c virus - induced multistep hepatocarcinogenesis. Virol. J. 11 (1), 117. doi:10.1186/1743-422X-11-117
Villanueva, A. (2019). Hepatocellular carcinoma. N. Engl. J. Med. 380 (15), 1450–1462. doi:10.1056/NEJMra1713263
Wan, L., Guo, L., Hu, Y., Huang, H., Zhang, M., Xu, K., et al. (2021). Comparing the diagnostic value of serum oligosaccharide chain (g-test) and alpha-fetoprotein for hepatitis b virus-related liver cancer. Clin. Biochem. 89, 44–50. doi:10.1016/j.clinbiochem.2020.12.005
Wang, J., and Cao, E. (2004). Rapid kinetic rate assay of the serum alpha-L-fucosidase in patients with hepatocellular carcinoma by using a novel substrate. Clin. Chim. Acta. 347 (1), 103–109. doi:10.1016/j.cccn.2004.04.007
Wang, S., Chen, S., Jin, M., Hu, M., Huang, W., Jiang, Z., et al. (2022). Diagnostic and prognostic value of serum chitinase 3-like protein 1 in hepatocellular carcinoma. J. Clin. Lab. Anal. 36 (2), e24234. doi:10.1002/jcla.24234
Wedge, E., Hansen, J. W., Garde, C., Asmar, F., Tholstrup, D., Kristensen, S. S., et al. (2017). Global hypomethylation is an independent prognostic factor in diffuse large b cell lymphoma. Am. J. Hematol. 92 (7), 689–694. doi:10.1002/ajh.24751
Wen, L., Li, J., Guo, H., Liu, X., Zheng, S., Zhang, D., et al. (2015). Genome-scale detection of hypermethylated cpg islands in circulating cell-free dna of hepatocellular carcinoma patients. Cell Res. 25 (11), 1250–1264. doi:10.1038/cr.2015.126
Wong, I. H., Lo, Y. M., Yeo, W., Lau, W. Y., and Johnson, P. J. (2000). Frequent p15 promoter methylation in tumor and peripheral blood from hepatocellular carcinoma patients. Clin. cancer Res. official J. Am. Assoc. Cancer Res. 6 (9), 3516–3521.
Wong, I. H., Lo, Y. M., Zhang, J., Liew, C. T., Ng, M. H., Wong, N., et al. (1999). Detection of aberrant p16 methylation in the plasma and serum of liver cancer patients. Cancer Res. 59 (1), 71–73.
Wu, H., Yang, H., Wang, Q., Chen, C., and Santella, R. (2017). Plasma dna methylation marker and hepatocellular carcinoma risk prediction model for the general population. Carcinogenesis 38, 1021–1028. doi:10.1093/carcin/bgx078
Xing, H., Zheng, Y., Han, J., Zhang, H., Li, Z., Lau, W., et al. (2018). Protein induced by vitamin k absence or antagonist-ii versus alpha-fetoprotein in the diagnosis of hepatocellular carcinoma: a systematic review with meta-analysis. Hepatob. Pancreat. Dis. Int. 17 (6), 487–495. doi:10.1016/j.hbpd.2018.09.009
Xing, X., Cai, L., Ouyang, J., Wang, F., Li, Z., Liu, M., et al. (2023). Proteomics-driven noninvasive screening of circulating serum protein panels for the early diagnosis of hepatocellular carcinoma. Nat. Commun. 14 (1), 8392. doi:10.1038/s41467-023-44255-2
Xu, J., Chen, H., Fan, W., Qiu, M., and Feng, J. (2024). Plasma cell-free dna as a sensitive biomarker for multi-cancer detection and immunotherapy outcomes prediction. J. Cancer Res. Clin. Oncol. 150 (1), 7. doi:10.1007/s00432-023-05521-4
Xu, R., Wei, W., Krawczyk, M., Wang, W., Luo, H., Flagg, K., et al. (2017). Circulating tumour dna methylation markers for diagnosis and prognosis of hepatocellular carcinoma. Nat. Mater. 16 (11), 1155–1161. doi:10.1038/nmat4997
Yan, L., Chen, Y., Zhou, J., Zhao, H., Zhang, H., and Wang, G. (2018). Diagnostic value of circulating cell-free dna levels for hepatocellular carcinoma. Int. J. Infect. Dis. IJID official Publ. Int. Soc. Infect. Dis. 67, 92–97. doi:10.1016/j.ijid.2017.12.002
Yang, J., Pan, G., Guan, L., Liu, Z., Wu, Y., Liu, Z., et al. (2022). The burden of primary liver cancer caused by specific etiologies from 1990 to 2019 at the global, regional, and national levels. Cancer Med. 11 (5), 1357–1370. doi:10.1002/cam4.4530
Yang, J. D., Hainaut, P., Gores, G. J., Amadou, A., Plymoth, A., and Roberts, L. R. (2019a). A global view of hepatocellular carcinoma: trends, risk, prevention and management. Nat. Rev. Gastroenterol. Hepatol. 16 (10), 589–604. doi:10.1038/s41575-019-0186-y
Yang, T., Wang, N., Wang, F., Liu, H., Shen, F., and Lv, G. (2023). Refinement and validation of a comprehensive clinical diagnostic model (gamad) based on gender, age, multitarget circulating tumour dna methylation signature and commonly used serological biomarkers for early detection of hepatocellular carcinoma: a multicentre, prospective observational study protocol. BMJ Open 13 (9), e076467. doi:10.1136/bmjopen-2023-076467
Yang, T., Xing, H., Wang, G., Wang, N., Liu, M., Yan, C., et al. (2019b). A novel online calculator based on serum biomarkers to detect hepatocellular carcinoma among patients with hepatitis b. Clin. Chem. 65 (12), 1543–1553. doi:10.1373/clinchem.2019.308965
Yasuda, H., Nakagawa, M., Kiyokawa, H., Yoshida, E., Yoshimura, T., Koshikawa, N., et al. (2019). Unique biological activity and potential role of monomeric laminin-γ2 as a novel biomarker for hepatocellular carcinoma: a review. Int. J. Mol. Sci. 20, 226. doi:10.3390/ijms20010226
Yeo, W., Wong, W., Wong, N., Law, B. K., Tse, G. M., and Zhong, S. (2005). High frequency of promoter hypermethylation of rassf1a in tumorous and non-tumourous tissue of breast cancer. Pathology 37 (2), 125–130. doi:10.1080/00313020500058623
Zeng, C., Stroup, E. K., Zhang, Z., Chiu, B. C. H., and Zhang, W. (2019). Towards precision medicine: advances in 5-hydroxymethylcytosine cancer biomarker discovery in liquid biopsy. Cancer Commun. 39 (1), 12. doi:10.1186/s40880-019-0356-x
Zeng, H., Cao, M., Xia, C., Wang, D., Chen, K., Zhu, Z., et al. (2023). Performance and effectiveness of hepatocellular carcinoma screening in individuals with hbsag seropositivity in China: a multicenter prospective study. Nat. Cancer. 4 (9), 1382–1394. doi:10.1038/s43018-023-00618-8
Zeng, H., He, B., Yi, C., and Peng, J. (2018). Liquid biopsies: dna methylation analyses in circulating cell-free dna. J. Genet. Genomics. 45 (4), 185–192. doi:10.1016/j.jgg.2018.02.007
Zhang, B., Yang, B., and Tang, Z. (2004). Randomized controlled trial of screening for hepatocellular carcinoma. J. Cancer Res. Clin. Oncol. 130 (7), 417–422. doi:10.1007/s00432-004-0552-0
Zhang, S., Sun, K., Zheng, R., Zeng, H., Wang, S., Chen, R., et al. (2021). Cancer incidence and mortality in China, 2015. J. Natl. Cancer Cent. 1 (1), 2–11. doi:10.1016/j.jncc.2020.12.001
Zhang, W., He, H., Zang, M., Wu, Q., Zhao, H., Lu, L., et al. (2017). Genetic features of aflatoxin-associated hepatocellular carcinoma. Gastroenterology 153 (1), 249–262. doi:10.1053/j.gastro.2017.03.024
Zhang, Y., Wu, H., Shen, J., Ahsan, H., Tsai, W. Y., Yang, H., et al. (2007). Predicting hepatocellular carcinoma by detection of aberrant promoter methylation in serum dna. Clin. Cancer Res. 13 (8), 2378–2384. doi:10.1158/1078-0432.CCR-06-1900
Zhao, Y., Zhao, L., Jin, H., Xie, Y., Chen, L., Zhang, W., et al. (2024). Plasma methylated gnb4 and riplet as a novel dual-marker panel for the detection of hepatocellular carcinoma. Epigenetics 19 (1), 2299044. doi:10.1080/15592294.2023.2299044
Zheng, K., Dai, L., Zhao, Y., Li, L., Li, W., Zhang, X., et al. (2023). Methylated sept9 combined with afp and pivka-ii is effective for the detection of hcc in high-risk population. BMC Gastroenterol. 23 (1), 260. doi:10.1186/s12876-023-02900-6
Zhou, J., and Yang, D. (2023). Diagnostic value of opni in hepatocellular carcinoma. Oncology 101 (8), 481–490. doi:10.1159/000530319
Zhou, J., Yu, L., Gao, X., Hu, J., Wang, J., Dai, Z., et al. (2011). Plasma microrna panel to diagnose hepatitis b virus–related hepatocellular carcinoma. J. Clin. Oncol. 29 (36), 4781–4788. doi:10.1200/JCO.2011.38.2697
Zhou, Y., Li, W., Tseng, Y., Zhang, J., and Liu, J. (2019). Developing slow-off dickkopf-1 aptamers for early-diagnosis of hepatocellular carcinoma. Talanta 194, 422–429. doi:10.1016/j.talanta.2018.10.014
Zhou, Y., Wang, X., Qiu, X., Shuai, Z., Wang, C., and Zheng, F. (2018). Cdkn2a promoter methylation and hepatocellular carcinoma risk: a meta-analysis. Clin. Res. Hepatol. Gastroenterol. 42 (6), 529–541. doi:10.1016/j.clinre.2017.07.003
Keywords: hepatocellular carcinoma, early diagnosis, blood biomarkers, biomarker detection technology, prognosis
Citation: Zhao J, Hu Z, Zheng X, Lin Y, Liu X, Zhang J, Peng J and Gao H (2024) Blood biomarkers of hepatocellular carcinoma: a critical review. Front. Cell Dev. Biol. 12:1489836. doi: 10.3389/fcell.2024.1489836
Received: 02 September 2024; Accepted: 13 November 2024;
Published: 22 November 2024.
Edited by:
Meng Zhang, First Affiliated Hospital of Anhui Medical University, ChinaReviewed by:
Zhao Yang, Beijing University of Chemical Technology, ChinaJyothi Padiadpu, National Institutes of Health (NIH), United States
Yuqing Li, Shenzhen University, China
Copyright © 2024 Zhao, Hu, Zheng, Lin, Liu, Zhang, Peng and Gao. This is an open-access article distributed under the terms of the Creative Commons Attribution License (CC BY). The use, distribution or reproduction in other forums is permitted, provided the original author(s) and the copyright owner(s) are credited and that the original publication in this journal is cited, in accordance with accepted academic practice. No use, distribution or reproduction is permitted which does not comply with these terms.
*Correspondence: Hainv Gao, Z2FvaGFpbnZAMTYzLmNvbQ==; Jing Peng, cGVubnlwZW5nMTk4N0AxNjMuY29t