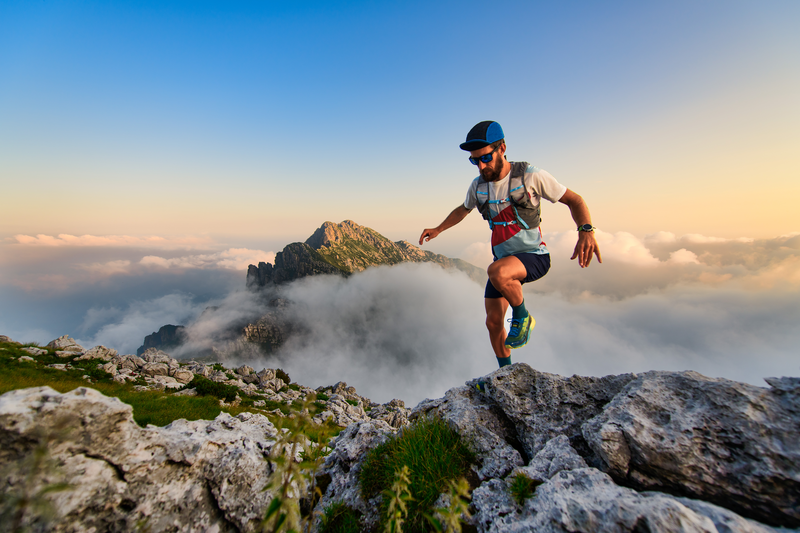
95% of researchers rate our articles as excellent or good
Learn more about the work of our research integrity team to safeguard the quality of each article we publish.
Find out more
MINI REVIEW article
Front. Cell Dev. Biol. , 21 August 2024
Sec. Stem Cell Research
Volume 12 - 2024 | https://doi.org/10.3389/fcell.2024.1446050
A correction has been applied to this article in:
Corrigendum: Advances in application of hypoxia-preconditioned mesenchymal stem cell-derived exosomes Advances in application of hypoxia-preconditioned mesenchymal stem cell-derived exosomes
Mesenchymal stem cells (MSCs) primarily secrete physiologically functional exosomes via paracrine effects that act on various adjacent and distant cells, thus exerting their therapeutic effects. In recent years, hypoxic preconditioning, as a novel MSC culture mode, has emerged as a research hotspot. Many previous studies have shown the role and underlying regulatory mechanisms of hypoxic preconditioning in various diseases, which has provided sufficient reference materials for the MSC research field. Therefore, this review summarizes the progress in application of hypoxia-preconditioned MSC-derived exosomes that substantially increases and improves the biological activity of specific molecules, such as microRNA.
Mesenchymal stem cells (MSCs) are pluripotent cells with self-renewal and differentiation properties that are widely distributed in many parts of the human body (Bianco and Gehron Robey, 2000). These include bone marrow, adipose tissue, dental pulp, umbilical cord and placenta, among others (Bianco and Gehron Robey, 2000). The efficacy of stem cell therapy has been well documented in numerous preclinical studies (Uccelli et al., 2008). However, the therapy’s limitations, including low implantation rates (Fouillard et al., 2007) and implantation side effects (Fennema et al., 2018; Jeong et al., 2011; Kusuma et al., 2015; Wang et al., 2015) have prompted researchers to seek improvements.
Exosomes are currently one of the most popular areas of research in the field of stem cells. Exosomes are biological nanovesicles with a diameter of 30–150 nm, secreted by different types of cells, containing messenger molecules, including coding and non-coding RNAs, which can regulate the functions of proximal and distal cells (Lai et al., 2015). The term “proximal” refers to functions inside the cell, such as metabolism and signalling, while “distal” refers to functions outside the cell, such as intercellular communication and tissue repair. It can be posited that the role of mesenchymal stem cell-derived exosomes (MSC-Exos) is to regulate and influence intra- and extracellular functions in order to maintain tissue homeostasis and promote tissue repair. Furthermore, it can be hypothesised that MSC-Exos possess the ability to interact with a wide range of cell types in neighbouring and remote regions, thereby triggering appropriate cellular responses. Although exosomes are characterised by low toxicity, high stability, low immunogenicity and efficient transport of donor cells (Zhu J. et al., 2018), the therapeutic use of exosomes is limited by the limited number of cells from which they are derived and the different components they secrete. Consequently, the current scientific challenge in the field of MSC-Exos applications is to identify effective methods for optimising the therapeutic effects of MSC-Exos. Recent studies have indicated that hypoxic pretreatment of MSCs represents an effective strategy for modulating their therapeutic potential, resulting in the release of exosomes with enhanced biological functions through paracrine effects. The low oxygen concentration required for MSC culture was preset according to the tissue source, and the exosomes obtained from the hypoxic pretreated MSCs were extracted within a specified time period, designated as hypoxic MSC-Exos (HypMSC-Exos). This paper reviews the progress of the application of HypMSC-Exos.
Exosomes are lipid bilayer vesicles with a diameter of 30–150 nm, which carry a variety of substances, including lipids, metabolites, proteins, microRNAs (miRNAs), mRNAs, long noncoding RNAs (lncRNAs) and DNA (Pegtel and Gould, 2019). Previous studies have demonstrated that HypMSC-Exos enhance mRNA expression and secretion of several crucial cytokines, including vascular endothelial growth factor (VEGF) and basic fibroblast growth factor. Notably, this hypoxic preconditioning did not result in any adverse effects on the release of other cytokines (Kinnaird et al., 2004). It was subsequently demonstrated that hypoxic pre-acclimatisation augmented the protective factors of MSC-Exos against future hypoxia-injured tissues, including hypoxia-inducible factor-1α (HIF-1α), angiogenic factors (VEGF, angiopoietin 1 and erythropoietin), survival proteins (P65, P50, and P105) and anti-apoptotic proteins (Bcl-xL and Bcl-2) expression (Hu et al., 2008). A considerable number of studies have demonstrated that hypoxic preconditioning of mesenchymal stem cells (MSCs) modulates the expression of specific molecules and enhances the therapeutic effect of MSC-derived exosomes (MSC-Exos). Among these, microRNAs (miRNAs) have received particular attention due to their pivotal role in regulating gene expression. One study demonstrated that 215 miRNAs were upregulated and 369 miRNAs were downregulated in HypMSC-Exos in comparison to normoxic exosomes (Casado-Díaz et al., 2020; Zhang et al., 2017). Among the observed effects, HypMSC-Exos demonstrated a notable increase in the expression of miRNAs with specific functions, indicating a potential joint role for miRNAs in the observed processes (Liang et al., 2022). Consequently, these studies indicate that miRNAs in exosomes play a pivotal role in intracellular signalling, transport and cellular therapy in vitro (Ong et al., 2014; Gray et al., 2015).
A substantial body of evidence indicates that the characteristics of mesenchymal stem cells (MSCs) are influenced by alterations in environmental conditions. It has been demonstrated that changes in atmospheric oxygen concentrations may result in DNA damage to cellular components and may consequently lead to genetic instability (Estrada et al., 2012), which may in turn result in differences in the therapeutic efficacy of MSCs. In vitro culture of MSCs typically occurs under normoxic conditions (21% O₂). However, it is known that MSCs are in a hypoxic ecological niche in vivo, and that the majority of MSCs exist in environments with 2%–8% (or even lower) oxygen content in vivo (Xue et al., 2018). Consequently, culturing MSCs in a low-oxygen environment would be more closely aligned with their physiological environment. There is accumulating evidence that maintaining MSCs under low partial pressure of oxygen may assist in preserving their characteristics and enhancing their therapeutic potential. For instance, reduced oxygen supply is a common occurrence in damaged tissues. Once MSCs migrate to the hypoxic zone, their paracrine effect produces a substantial quantity of therapeutic paracrine factors to repair damaged tissues (Han et al., 2019). Furthermore, it has been demonstrated that hypoxic preconditioning or the activation of HIF-1α expression in vitro reduces the apoptosis of bone marrow mesenchymal stem cells (BMMSCs) cultured in vitro, thus playing an important role in the survival of BMMSCs after transplantation (Luo et al., 2019). Consequently, hypoxic preconditioning can be employed as a therapeutic strategy to enhance the growth environment of MSCs and stimulate the secretion of exosomes with physiological functions (Figure 1).
Figure 1. Flow chart of hypoxic pretreatment of mesenchymal stem cells (MSCs) to produce hypoxic MSC-derived exosomes (HypMSC-Exos).
A number of studies have demonstrated the importance of HypMSC-Exos in the field of angiogenesis and repair. The mechanism by which HypMSC-Exos exerts its effects may be to promote angiogenesis and repair by altering the miRNA and proteomic profiles and modulating multiple signalling pathways. An early study demonstrated that hypoxic preconditioning could enhance the promotion of neovascularisation in transplanted adipose tissue by hypoxic adipose mesenchymal stem cell-derived exosomes (HypADSC-Exos) (Han et al., 2018). The results of this experiment indicated that the composition of HypMSC-Exos was altered by the hypoxic environment, which enhanced the angiogenic potential of MSC-Exos. This was evidenced by the alteration of their proteomic profile (Li et al., 2022).
A significant number of studies have demonstrated that the HIF-1α or VEGF signalling pathway is a downstream target of HypMSC-Exos, which enhances angiogenesis. The key signalling molecules and upstream signalling pathways are currently being investigated. For instance, the up-regulation of high mobility group box 1 (HMGB1) in HypMSC-Exos mediates the expression of the c-Jun N-terminal kinase (JNK) pathway activation, which induces the expression of HIF-1α/VEGF, thereby promoting angiogenesis (Gao et al., 2021; Chen and Zhang, 2021). Furthermore, extracellular vesicles derived from hypoxic mucosal MSCs have been shown to upregulate miR-612 by inhibiting tumour protein 53 (Tp53), thereby inducing the expression of the HIF-1α/VEGF pathway, which promotes the generation of microvascular endothelial cells in the human brain (Ge et al., 2021). Furthermore, studies have demonstrated that HypADSC-Exo may facilitate angiogenesis by activating the protein kinase A signalling pathway and promoting the expression of the VEGF pathway (Xue et al., 2018). HIF-1α is a crucial transcription factor for MSCs in hypoxic environments. Studies have demonstrated that hypoxic conditions may markedly enhance the pro-angiogenic effects of MSC-Exos by mediating the activation of miR-210 and neutral sphingomyelinase 2 through the action of HIF-1α (Zhu J. et al., 2018). Nevertheless, a few articles have also reported the existence of other signalling pathways regulated by HypMSC-Exos. It has been demonstrated that the expression of let-7f-5p and miR-210-3p is elevated in exosomes derived from hypoxia-pretreated human deciduous tooth stem cells. This upregulation has been shown to promote angiogenesis via two distinct pathways: the let-7f-5p/AGO1 (argonaute RISC component 1)/VEGF pathway and the miR-210-3p/ephrinA3 pathway (Liu P. et al., 2022). This suggests that they may become a novel signalling pathway for pro-angiogenic therapy with HypMSC-Exos. Hypoxic preconditioning represents an effective method for enriching pro-angiogenic factors in MSC-Exos. HypMSC-Exos have been demonstrated to promote angiogenesis by upregulating the expression of LOXL2 (lysyl oxidase-like 2), CXCR4 (C-X-C chemokine receptor 4) and SDF-1 (stromal cell-derived factor-1) (Li et al., 2022), although the specific molecular mechanisms remain to be elucidated. The aforementioned findings indicate that HIF-1α may play a pivotal role in the regulation of the signalling pathway by HypMSC-Exos. However, the precise mechanism by which hypoxia influences the release of MSC-Exos remains elusive. In conclusion, HypMSC-Exos may enhance the effect of angiogenesis. Therefore, future research should focus on studying the upstream and downstream signalling pathway regulation mechanisms of molecules such as miRNAs and proteins.
The burden of heart failure arising after myocardial infarction remains a significant problem in clinical practice. A growing number of studies have demonstrated that HypMSC-Exos significantly improves ischemic cardiomyocyte apoptosis, promotes their remodelling, and protects cardiac function. A study demonstrated that hypoxic umbilical cord mesenchymal stem cell-derived exosomes (HypUCMSC-Exos) significantly promoted the repair of injured myocardium after myocardial infarction in SD rats (Zhang P. et al., 2019). The changes of miRNAs and lncRNAs in HypUCMSC-Exos are important key regulatory signals in the study of myocardial injury repair mechanisms. Hypoxic BMMSC-derived exosomes (HypBMMSC-Exos) have been demonstrated to inhibit apoptosis of rat cardiomyocytes following acute infarction by upregulating miR-24 (Zhang C. S. et al., 2019). Furthermore, miR-125b-5p in HypMSCExos inhibits cardiomyocyte apoptosis by suppressing the expression of P53 and BAK1 (BCL2 antagonist/killer 1), thereby promoting ischaemic heart repair (Zhu L. P. et al., 2018). Additionally, miR-98-5p within HypBMMSC-Exos mediates the inhibition of myocardial ischaemia-reperfusion injury by targeting TLR4 through the activation of the PI3K/Akt signalling pathway (Zhang L. et al., 2021). It has been demonstrated that HypMSC-Exos upregulate the expression of lncRNA-UCA1, which in turn activates XIAP (X-linked inhibitor of apoptosis protein) to exert cardioprotective effects through the inhibition of miR-873-5p (Sun et al., 2020), suggesting that exosomal lncRNA-UCA1 may be a promising new biomarker for cardioprotection. In a further study, Liu et al. (2019) demonstrated that HypUCMSC-Exo could regulate autophagy through the PI3K/Akt/mTOR pathway, thereby inhibiting apoptosis in myocardial H9C2 cells induced by hypoxia and serum deprivation. A limited number of articles have also reported the study of HypMSC-Exos against adriamycin-induced myocardial injury. HypMSC-Exos has been demonstrated to counteract adriamycin-induced myocardial injury through two distinct pathways: the lncRNA-MALAT1/miR-92a-3p/ATG4a (autophagy-related 4a cysteine peptidase) axis and the Trx1 (thioredoxin 1)/mTORC1 signalling pathway. These pathways have been shown to counteract adriamycin-induced cardiomyocyte damage and senescence, thereby facilitating cardiac recovery (Xia et al., 2020; Yu et al., 2022). This further demonstrates the therapeutic potential of HypMSC-Exos for acute cardiotoxicity. In summary, HypMSC-Exos represents a promising therapeutic approach for the treatment of tissue ischaemia and cardiotoxic injury. However, the specific targeting of exosomes to the ischaemic heart remains a challenge.
HypMSC-Exos has been demonstrated to have significant therapeutic effects on a number of conditions affecting bone, including hormonal osteonecrosis of the femoral head (Yuan et al., 2021), bioactive fibrous ring repair (DiStefano et al., 2022), tendon-bone tunnel healing (Zhang T. et al., 2022), and other bony injuries. However, the exact molecular mechanism of the therapeutic effect remains to be investigated. Liu et al. (2020a) demonstrated that the hypoxic environment may significantly enhance fracture healing through the action of HIF-1α, which mediates the up-regulation of miR-126. This significantly improves fracture healing. In the field of cartilage regeneration engineering, the incorporation of hydrogel-embedded HypMSC-Exos has been demonstrated to promote cartilage regeneration through the up-regulation of miR-205-5p expression and the activation of the PTEN/AKT pathway (Shen et al., 2022), thereby suggesting that the composite of HypMSC-Exos and silk fibroin hydrogel has a broad application prospect in cartilage regeneration. In the meantime, bioinformatics analysis indicated that hypoxia pretreatment of extracellular vesicles derived from mesenchymal stem cells (MSCs) may stimulate the proliferation and migration of chondrocytes and inhibit the apoptosis of chondrocytes via the miR-18-3p/JAK/STAT or miR-181c-5p/MAPK signalling pathways, thus promoting cartilage repair (Zhang B. et al., 2022). Recent studies have demonstrated that exosomes secreted by HypADSC-Exos and tendon cells protect the tendon matrix from damage by releasing regenerative mediators into the extracellular matrix, thereby triggering an autocrine/paracrine response (Thankam et al., 2020). This study also demonstrated that certain mRNA and protein levels in these two exosomes are in a state of dynamic equilibrium, and that they are involved in a multitude of signalling pathways that pertain to the extracellular matrix. Furthermore, the study demonstrated that certain mRNAs and proteins in the two exocrine species exhibited dynamic equilibrium, and were implicated in a multitude of extracellular matrix-related signaling pathways. In conclusion, hypoxic preconditioning represents an effective and promising method to optimise the therapeutic effects of MSC-Exos on bone repair and regeneration.
Nerve injuries can result in severe motor and sensory dysfunction, with high rates of disability and mortality. An initial study observed that HypMSC-Exos facilitated cerebral remodelling and neurological recovery in mice following focal cerebral ischaemia (Gregorius et al., 2021). Nevertheless, the optimal therapeutic strategy for neurological injury is the polarization transition of microglia and the suppression of deleterious, excessive neuroinflammation. It has now been demonstrated that HypMSC-Exos plays a more efficacious therapeutic role in the polarisation transition of microglia and represents a promising therapeutic target in the field of neurological injury. For instance, (1) HypBMMSC-Exos enriched with miR-216a-5p facilitates the transition of microglia from the M1 to the M2 phenotype by inhibiting the TLR4/NF-κB pathway and activating the PI3K/Akt signalling pathway (Liu et al., 2020b). (2) The up-regulation of lncRNA-Gm37494 expression in HypADSC-Exos has been demonstrated to promote microglia M1/M2 polarisation by inhibiting miR-130b-3p and promoting PPARγ expression (Shao et al., 2020). (3) HypADSC-Exos reduces miR-7703p expression, thereby altering microglia M1/M2 polarisation and improving cognition in Alzheimer’s disease mice. This is achieved by delivering circ-Epc1-mediated TREM2 expression (Liu H. et al., 2022). (4) HypADSC-Exos inhibited miR-124-3p by delivering circ-Rps5 to promote SIRT7 expression, thereby attenuating acute ischemic stroke-induced brain injury and promoting microglia M1/M2 polarisation (Yang H. et al., 2022). In addition, HypBMMSC-Exos demonstrated robust protective effects against apoptosis in neuronal injury. For instance, HypBMMSC-Exos has been demonstrated to repair oxygen and sugar deprivation-induced neuronal injury by inhibiting NLRP3 inflammatory vesicle-mediated caspase death (Kang et al., 2021). (2) MiR-499-5p within HypADSC-Exos plays a pivotal role in regulating neuronal apoptosis by negatively regulating the JNK3/MAPK10/c-Jun signalling pathway, thereby reducing neuronal apoptosis in rat neuronal cells (Liang et al., 2022). (3) circOXNAD1 within HypUCMSC-Exos activates FOXO3a expression, increases neuronal viability, and inhibits cell death and inflammation by parceling out miR-29a-3p (Wang et al., 2023). In materials engineering, the combined use of HypMSCExos and 3D-CS scaffolds has been demonstrated to promote nerve regeneration after traumatic brain injury, while inhibiting chronic neuroinflammation and neural apoptosis (Liu X. et al., 2022). This indicates that the combination of HypMSC-Exos therapy with carrier materials represents a novel approach to the treatment of traumatic brain injury.
Poor healing of skin wounds can be life-threatening and is a significant public health concern globally. In a previous study, Qiu et al. (2020) demonstrated that MSC-Exos exhibited a more pronounced therapeutic effect on skin wound healing, suggesting a promising avenue for cell-free treatment of skin injury. Among the various types of skin injury, diabetic wounds are particularly challenging to heal due to persistent inflammation and delayed healing caused by hypoxia. HypADSC-Exos has been shown to promote fibroblast proliferation and migration through the activation of the PI3K/Akt pathway, which can accelerate the rate of diabetic wound healing and improve the quality of wound healing. Additionally, HypADSC-Exos has been demonstrated to inhibit inflammatory responses (Wang et al., 2021). Zhang X. F. et al. (2021) demonstrated that miR-125b upregulation within HypUCMSC-Exos enhances endothelial cell survival and migration during wound healing by targeting and inhibiting TP53INP1 (tumor protein p53 inducible nuclear protein 1). Furthermore, HypADSC-Exos facilitates wound healing in diabetic mice by delivering circ-Snhg11 and activating the miR-144-3p/HIF-1α pathway (Shi et al., 2022). In conclusion, HypMSC-Exos represents a promising area for further investigation in the context of diabetic wound healing. Consequently, future research should prioritise the exploration of additional signalling pathway mechanisms associated with HypMSC-Exos.
A number of studies have demonstrated that HypMSC-Exos has been shown to have more positive therapeutic effects in modulating inflammation compared to normoxic exosomes in basic research. Yang T. et al. (2022) demonstrated that HypBMMSC-Exos up-regulated the expression of HIF-1α and activated the antioxidant signalling of superoxide dismutase to attenuate the ototoxicity of cisplatin and mitigate excessive oxidative stress. This study suggests that HypMSC-Exos may have a potential preventive effect on severe oxidative stress. The up-regulation of miR-182-5p within HypBMSC-Exo has been demonstrated to promote macrophage polarisation through modulation of the FOXO1/TLR4 signalling pathway. This evidence supports the therapeutic potential of HypMSC-Exos in liver regeneration, as outlined in reference (Xu et al., 2022). Recent studies have shown that the up-regulation of miR-216a-5p in HypADSC-Exos has the dual effect of promoting macrophage M2-type polarisation through the regulation of the HMGB1/TLR4/NF-κB axis to attenuate excessive inflammation in colitis (Qian et al., 2023) and of regulating the accumulation of reactive oxygen species in intestinal epithelial cells, DNA damage, and immune homeostasis by HIF-1α, thereby attenuating mucosal damage in ulcerative colitis (Zhu et al., 2022). Mucosal damage is a well-documented consequence of ulcerative colitis. This indicates that HypMSC-Exos represents a promising avenue for optimising colitis treatment. Furthermore, Cao et al. (2022) observed and confirmed that HypADSC-Exos, which highly express mmu_circ_0001295, improved the outcome and inhibited sepsis-induced kidney injury in septic mice. This study demonstrates the feasibility of MSC-Exos as a novel target for the treatment of sepsis-induced kidney injury.
This paper presents a comprehensive overview of the progress made in the application of hypoxic pretreatment on the therapeutic role of MSC-Exo. A significant body of evidence from numerous studies indicates that HypMSC-Exos exhibit remarkable therapeutic potential in promoting angiogenesis and repair, myocardial repair, and other areas (Figure 2). This suggests that exploring the optimal conditions of hypoxic pretreatment of MSCs is an effective strategy for enhancing the biological functions of MSC-Exos, which in turn contributes to the effectiveness of exosome therapy. Nevertheless, the utilisation of hypoxic preconditioning in MSC-Exos is still in its nascent stages and is subject to numerous unresolved issues. For instance, it is necessary to determine the optimal concentration of hypoxia for MSCs of different tissue origins. (2) What are the differences in exosomes secreted by MSCs from different tissue sources under the same hypoxic preconditioning conditions? Does the administration of MSCs from different tissue sources result in different outcomes? (3) Currently, the majority of experiments investigating HypMSC-Exos are conducted at the cellular level. Therefore, further research is required to investigate the administration and dosage of HypMSC-Exos in animal experiments. (4) The current research on HypMSC-Exos is focused on the internal miRNAs, while the biological potential of the secreted proteins, lipids and other non-coding RNAs still requires further elucidation. (5) Following systemic administration, it remains challenging to elucidate the specific targeting mechanism of HypMSC-Exos to target organs, tissues and cells. Furthermore, the potential of HypMSC-Exos as a nanocarrier or active factor in tissue engineering and regenerative medicine requires further investigation. In conclusion, a comprehensive examination of the aforementioned pertinent issues will facilitate the enhanced efficacy of HypMSC-Exos in clinical exosome-based therapies for various diseases.
Figure 2. Molecular mechanisms of HypMSC-Exos regulation of related disease progression. (Abbreviations: HMGB1, high mobility group box 1; JNK, c-Jun N-terminal kinase; HIF-1α, hypoxia-inducible factor-1α; VEGF, vascular endothelial growth factor; Tp53, tumor protein 53; PKA, protein kinase A; nSMase2, neutral sphingomyelinase 2; AGO1, argonaute RISC component 1; LOXL2, lysyl oxidase-like 2; CXCR4, C-X-C chemokine receptor 4; SDF-1, stromal cell-derived factor-1; PI3K, phosphatidylinositol 3-kinase; TLR4, Toll-like receptor 4; ATG4a, autophagy related 4a cysteine peptidase; BAK1, BCL2 antagonist/killer 1; Trx1, thioredoxin 1; mTORC1, mammalian target of rapamycin complex 1; MARK, mitogen-activated protein kinase; JAK, janus kinase; STAT, signal transducer and activator of transcription; PTEN, phosphatase and tensin homolog; NLRP3, NOD-like receptor family, pyrin domain containing 3; Epc1, enhancer of polycomb homolog 1; TREM2, triggering receptor expressed on myeloid cells 2; SIRT7, sirtuin 7; FOXO3a, forkhead box O3; PPARγ, peroxisome proliferator-activated receptor γ; TP53INP1, tumor protein p53 inducible nuclear protein 1).
HZ: Conceptualization, Writing–original draft, Writing–review and editing. YC: Data curation, Writing–original draft. GZ: Funding acquisition, Writing–original draft, Writing–review and editing.
The author(s) declare that financial support was received for the research, authorship, and/or publication of this article. This study was supported by the Scientific Research Project of Guangdong Provincial Bureau of Traditional Chinese Medicine (No. 20231411), Project of Jilin Provincial Science and Technology Department (No. 20210101216JC), Project of Qingyuan Science and Technology Programme (No. 2022KJJH026), Project of Guangdong Provincial College Students’ Innovative Entrepreneurship Training Programme (S202310570053), Guangzhou Medical University 2023-2024 Science and Technology Innovation Project for University Students (No. 2022A144).
We wish to acknowledge all the researchers in our study.
The authors declare that the research was conducted in the absence of any commercial or financial relationships that could be construed as a potential conflict of interest.
All claims expressed in this article are solely those of the authors and do not necessarily represent those of their affiliated organizations, or those of the publisher, the editors and the reviewers. Any product that may be evaluated in this article, or claim that may be made by its manufacturer, is not guaranteed or endorsed by the publisher.
Bianco, P., and Gehron Robey, P. (2000). Marrow stromal stem cells. J. Clin. investigation 105 (12), 1663–1668. doi:10.1172/JCI10413
Cao, S., Huang, Y., Dai, Z., Liao, Y., Zhang, J., Wang, L., et al. (2022). Circular RNA mmu_circ_0001295 from hypoxia pretreated adipose-derived mesenchymal stem cells (ADSCs) exosomes improves outcomes and inhibits sepsis-induced renal injury in a mouse model of sepsis. Bioengineered 13 (3), 6323–6331. doi:10.1080/21655979.2022.2044720
Casado-Díaz, A., Quesada-Gómez, J. M., and Dorado, G. (2020). Extracellular vesicles derived from mesenchymal stem cells (MSC) in regenerative medicine: applications in skin wound healing. Front. Bioeng. Biotechnol. 8, 146. doi:10.3389/fbioe.2020.00146
Chen, H., and Zhang, Q. (2021). Mechanism of activation of HIF-1α/VEGF/JNK pathway by BMSCs-derived exosomes to promote vascular regeneration under hypoxic conditions. Shenzhen J. Integr. Med. 31 (14), 33–35+205. doi:10.16458/j.cnki.1007-0893.2021.14.013
DiStefano, T. J., Vaso, K., Panebianco, C. J., Danias, G., Chionuma, H. N., Kunnath, K., et al. (2022). Hydrogel-embedded poly (lactic-co-glycolic acid) microspheres for the delivery of hMSC-derived exosomes to promote bioactive annulus fibrosus repair. Cartilage 13 (3), 19476035221113959. doi:10.1177/19476035221113959
Estrada, J. C., Albo, C., Benguría, A., Dopazo, A., López-Romero, P., Carrera-Quintanar, L., et al. (2012). Culture of human mesenchymal stem cells at low oxygen tension improves growth and genetic stability by activating glycolysis. Cell death Differ. 19 (5), 743–755. doi:10.1038/cdd.2011.172
Fennema, E. M., Tchang, L. A. H., Yuan, H., van Blitterswijk, C. A., Martin, I., Scherberich, A., et al. (2018). Ectopic bone formation by aggregated mesenchymal stem cells from bone marrow and adipose tissue: a comparative study. J. tissue Eng. Regen. Med. 12 (1), e150–e158. doi:10.1002/term.2453
Fouillard, L., Chapel, A., Bories, D., Bouchet, S., Costa, J. M., Rouard, H., et al. (2007). Infusion of allogeneic-related HLA mismatched mesenchymal stem cells for the treatment of incomplete engraftment following autologous haematopoietic stem cell transplantation. Leukemia 21 (3), 568–570. doi:10.1038/sj.leu.2404550
Gao, W., He, R., Ren, J., Zhang, W., Wang, K., Zhu, L., et al. (2021). Exosomal HMGB1 derived from hypoxia-conditioned bone marrow mesenchymal stem cells increases angiogenesis via the JNK/HIF-1α pathway. FEBS open bio 11 (5), 1364–1373. doi:10.1002/2211-5463.13142
Ge, L., Xun, C., Li, W., Jin, S., Liu, Z., Zhuo, Y., et al. (2021). Extracellular vesicles derived from hypoxia-preconditioned olfactory mucosa mesenchymal stem cells enhance angiogenesis via miR-612. J. nanobiotechnology 19 (1), 380. doi:10.1186/s12951-021-01126-6
Gray, W. D., French, K. M., Ghosh-Choudhary, S., Maxwell, J. T., Brown, M. E., Platt, M. O., et al. (2015). Identification of therapeutic covariant microRNA clusters in hypoxia-treated cardiac progenitor cell exosomes using systems biology. Circulation Res. 116 (2), 255–263. doi:10.1161/CIRCRESAHA.116.304360
Gregorius, J., Wang, C., Stambouli, O., Hussner, T., Qi, Y., Tertel, T., et al. (2021). Small extracellular vesicles obtained from hypoxic mesenchymal stromal cells have unique characteristics that promote cerebral angiogenesis, brain remodeling and neurological recovery after focal cerebral ischemia in mice. Basic Res. Cardiol. 116 (1), 40. doi:10.1007/s00395-021-00881-9
Han, Y., Ren, J., Bai, Y., and Pei, X. (2019). Exosomes from hypoxia-treated human adipose-derived mesenchymal stem cells enhance angiogenesis through VEGF/VEGF-R. Int. J. Biochem. and cell Biol. 109, 59–68. doi:10.1016/j.biocel.2019.01.017
Han, Y. D., Bai, Y., Yan, X. L., Ren, J., Zeng, Q., Li, X. D., et al. (2018). Co-transplantation of exosomes derived from hypoxia-preconditioned adipose mesenchymal stem cells promotes neovascularization and graft survival in fat grafting. Biochem. biophysical Res. Commun. 497 (1), 305–312. doi:10.1016/j.bbrc.2018.02.076
Hu, X., Yu, S. P., Fraser, J. L., Lu, Z., Ogle, M. E., Wang, J. A., et al. (2008). Transplantation of hypoxia-preconditioned mesenchymal stem cells improves infarcted heart function via enhanced survival of implanted cells and angiogenesis. J. Thorac. Cardiovasc. Surg. 135 (4), 799–808. doi:10.1016/j.jtcvs.2007.07.071
Jeong, J. O., Han, J. W., Kim, J. M., Cho, H. J., Park, C., Lee, N., et al. (2011). Malignant tumor formation after transplantation of short-term cultured bone marrow mesenchymal stem cells in experimental myocardial infarction and diabetic neuropathy. Circulation Res. 108 (11), 1340–1347. doi:10.1161/CIRCRESAHA.110.239848
Kang, X., Jiang, L., Chen, X., Wang, X., Gu, S., Wang, J., et al. (2021). Exosomes derived from hypoxic bone marrow mesenchymal stem cells rescue OGD-induced injury in neural cells by suppressing NLRP3 inflammasome-mediated pyroptosis. Exp. cell Res. 405 (1), 112635. doi:10.1016/j.yexcr.2021.112635
Kinnaird, T., Stabile, E., Burnett, M. S., Lee, C. W., Barr, S., Fuchs, S., et al. (2004). Marrow-derived stromal cells express genes encoding a broad spectrum of arteriogenic cytokines and promote in vitro and in vivo arteriogenesis through paracrine mechanisms. Circulation Res. 94 (5), 678–685. doi:10.1161/01.RES.0000118601.37875.AC
Kusuma, G. D., Menicanin, D., Gronthos, S., Manuelpillai, U., Abumaree, M. H., Pertile, M. D., et al. (2015). Ectopic bone formation by mesenchymal stem cells derived from human term placenta and the decidua. PloS one 10 (10), e0141246. doi:10.1371/journal.pone.0141246
Lai, R. C., Yeo, R. W., and Lim, S. K. (2015). Mesenchymal stem cell exosomes. Seminars cell and Dev. Biol. 40, 82–88. doi:10.1016/j.semcdb.2015.03.001
Li, B., Xian, X., Lin, X., Huang, L., Liang, A., Jiang, H., et al. (2022). Hypoxia alters the proteome profile and enhances the angiogenic potential of dental pulp stem cell-derived exosomes. Biomolecules 12 (4), 575. doi:10.3390/biom12040575
Liang, Y., Wu, J. H., Zhu, J. H., and Yang, H. (2022). Exosomes secreted by hypoxia-pre-conditioned adipose-derived mesenchymal stem cells reduce neuronal apoptosis in rats with spinal cord injury. J. neurotrauma 39 (9–10), 701–714. doi:10.1089/neu.2021.0290
Liu, H., Jin, M., Ji, M., Zhang, W., Liu, A., and Wang, T. (2022). Hypoxic pretreatment of adipose-derived stem cell exosomes improved cognition by delivery of circ-Epc1 and shifting microglial M1/M2 polarization in an Alzheimer’s disease mice model. Aging 14 (7), 3070–3083. doi:10.18632/aging.203989
Liu, H., Sun, X., Gong, X., and Wang, G. (2019). Human umbilical cord mesenchymal stem cells derived exosomes exert antiapoptosis effect via activating PI3K/Akt/mTOR pathway on H9C2 cells. J. Cell. Biochem. 120 (9), 14455–14464. doi:10.1002/jcb.28705
Liu, P., Qin, L., Liu, C., Mi, J., Zhang, Q., Wang, S., et al. (2022). Exosomes derived from hypoxia-conditioned stem cells of human deciduous exfoliated teeth enhance angiogenesis via the transfer of let-7f-5p and miR-210-3p. Front. cell Dev. Biol. 10, 879877. doi:10.3389/fcell.2022.879877
Liu, W., Li, L., Rong, Y., Qian, D., Chen, J., Zhou, Z., et al. (2020a). Hypoxic mesenchymal stem cell-derived exosomes promote bone fracture healing by the transfer of miR-126. Acta biomater. 103, 196–212. doi:10.1016/j.actbio.2019.12.020
Liu, W., Rong, Y., Wang, J., Zhou, Z., Ge, X., Ji, C., et al. (2020b). Exosome-shuttled miR-216a-5p from hypoxic preconditioned mesenchymal stem cells repair traumatic spinal cord injury by shifting microglial M1/M2 polarization. J. neuroinflammation 17 (1), 47. doi:10.1186/s12974-020-1726-7
Liu, X., Wang, J., Wang, P., Zhong, L., Wang, S., Feng, Q., et al. (2022). Hypoxia-pretreated mesenchymal stem cell-derived exosomes-loaded low-temperature extrusion 3D-printed implants for neural regeneration after traumatic brain injury in canines. Front. Bioeng. Biotechnol. 10, 1025138. doi:10.3389/fbioe.2022.1025138
Luo, Z., Wu, F., Xue, E., Huang, L., Yan, P., Pan, X., et al. (2019). Hypoxia preconditioning promotes bone marrow mesenchymal stem cells survival by inducing HIF-1α in injured neuronal cells derived exosomes culture system. Cell death and Dis. 10 (2), 134. doi:10.1038/s41419-019-1410-y
Ong, S. G., Lee, W. H., Huang, M., Dey, D., Kodo, K., Sanchez-Freire, V., et al. (2014). Cross talk of combined gene and cell therapy in ischemic heart disease: role of exosomal microRNA transfer. Circulation 130 (Suppl. 1), S60–S69. doi:10.1161/CIRCULATIONAHA.113.007917
Pegtel, D. M., and Gould, S. J. (2019). Exosomes. Annu. Rev. Biochem. 88, 487–514. doi:10.1146/annurev-biochem-013118-111902
Qian, W., Huang, L., Xu, Y., Lu, W., Wen, W., Guo, Z., et al. (2023). Hypoxic ASCs-derived exosomes attenuate colitis by regulating macrophage polarization via miR-216a-5p/HMGB1 Axis. Inflamm. bowel Dis. 29 (4), 602–619. doi:10.1093/ibd/izac225
Qiu, X., Liu, J., Zheng, C., Su, Y., Bao, L., Zhu, B., et al. (2020). Exosomes released from educated mesenchymal stem cells accelerate cutaneous wound healing via promoting angiogenesis. Cell Prolif. 53 (8), e12830. doi:10.1111/cpr.12830
Shao, M., Jin, M., Xu, S., Zheng, C., Zhu, W., Ma, X., et al. (2020). Exosomes from long noncoding RNA-gm37494-ADSCs repair spinal cord injury via shifting microglial M1/M2 polarization. Inflammation 43 (4), 1536–1547. doi:10.1007/s10753-020-01230-z
Shen, K., Duan, A., Cheng, J., Yuan, T., Zhou, J., Song, H., et al. (2022). Exosomes derived from hypoxia preconditioned mesenchymal stem cells laden in a silk hydrogel promote cartilage regeneration via the miR-205-5p/PTEN/AKT pathway. Acta biomater. 143, 173–188. doi:10.1016/j.actbio.2022.02.026
Shi, R., Jin, Y., Zhao, S., Yuan, H., Shi, J., and Zhao, H. (2022). Hypoxic ADSC-derived exosomes enhance wound healing in diabetic mice via delivery of circ-Snhg11 and induction of M2-like macrophage polarization. Biomed. Pharmacother. 153, 113463. doi:10.1016/j.biopha.2022.113463
Sun, L., Zhu, W., Zhao, P., Wang, Q., Fan, B., Zhu, Y., et al. (2020). Long noncoding RNA UCA1 from hypoxia-conditioned hMSC-derived exosomes: a novel molecular target for cardioprotection through miR-873-5p/XIAP axis. Cell death Dis. 11 (8), 696. doi:10.1038/s41419-020-02783-5
Thankam, F. G., Chandra, I., Diaz, C., Dilisio, M. F., Fleegel, J., Gross, R. M., et al. (2020). Matrix regeneration proteins in the hypoxia-triggered exosomes of shoulder tenocytes and adipose-derived mesenchymal stem cells. Mol. Cell. Biochem. 465 (1-2), 75–87. doi:10.1007/s11010-019-03669-7
Uccelli, A., Moretta, L., and Pistoia, V. (2008). Mesenchymal stem cells in health and disease. Nat. Rev. Immunol. 8 (9), 726–736. doi:10.1038/nri2395
Wang, J., Wu, H., Peng, Y., Zhao, Y., Qin, Y., Zhang, Y., et al. (2021). Hypoxia adipose stem cell-derived exosomes promote high-quality healing of diabetic wound involves activation of PI3K/Akt pathways. J. nanobiotechnology 19 (1), 202. doi:10.1186/s12951-021-00942-0
Wang, S., Guo, L., Ge, J., Yu, L., Cai, T., Tian, R., et al. (2015). Excess integrins cause lung entrapment of mesenchymal stem cells. Stem cells (Dayton, Ohio) 33 (11), 3315–3326. doi:10.1002/stem.2087
Wang, X., Li, W., Hao, M., Yang, Y., and Xu, Y. (2023). Hypoxia-treated umbilical mesenchymal stem cell alleviates spinal cord ischemia-reperfusion injury in SCI by circular RNA circOXNAD1/miR-29a-3p/FOXO3a axis. Biochem. biophysics Rep. 34, 101458. doi:10.1016/j.bbrep.2023.101458
Xia, W., Chen, H., Xie, C., and Hou, M. (2020). Long-noncoding RNA MALAT1 sponges microRNA-92a-3p to inhibit doxorubicin-induced cardiac senescence by targeting ATG4a. Aging 12 (9), 8241–8260. doi:10.18632/aging.103136
Xu, J., Chen, P., Yu, C., Shi, Q., Wei, S., Li, Y., et al. (2022). Hypoxic bone marrow mesenchymal stromal cells-derived exosomal miR-182-5p promotes liver regeneration via FOXO1-mediated macrophage polarization. FASEB J. 36 (10), e22553. doi:10.1096/fj.202101868RRR
Xue, C., Shen, Y., Li, X., Li, B., Zhao, S., Gu, J., et al. (2018). Exosomes derived from hypoxia-treated human adipose mesenchymal stem cells enhance angiogenesis through the PKA signaling pathway. Stem cells Dev. 27 (7), 456–465. doi:10.1089/scd.2017.0296
Yang, H., Tu, Z., Yang, D., Hu, M., Zhou, L., Li, Q., et al. (2022). Exosomes from hypoxic pre-treated ADSCs attenuate acute ischemic stroke-induced brain injury via delivery of circ-Rps5 and promote M2 microglia/macrophage polarization. Neurosci. Lett. 769, 136389. doi:10.1016/j.neulet.2021.136389
Yang, T., Li, W., Peng, A., Liu, J., and Wang, Q. (2022). Exosomes derived from bone marrow-mesenchymal stem cells attenuates cisplatin-induced ototoxicity in a mouse model. J. Clin. Med. 11 (16), 4743. doi:10.3390/jcm11164743
Yu, Y., Wu, T., Lu, Y., Zhao, W., Zhang, J., Chen, Q., et al. (2022). Exosomal thioredoxin-1 from hypoxic human umbilical cord mesenchymal stem cells inhibits ferroptosis in doxorubicin-induced cardiotoxicity via mTORC1 signaling. Free Radic. Biol. Med. 193 (Pt 1), 108–121. doi:10.1016/j.freeradbiomed.2022.10.268
Yuan, N., Ge, Z., Ji, W., and Li, J. (2021). Exosomes secreted from hypoxia-preconditioned mesenchymal stem cells prevent steroid-induced osteonecrosis of the femoral head by promoting angiogenesis in rats. BioMed Res. Int. 2021, 6655225. doi:10.1155/2021/6655225
Zhang, B., Tian, X., Qu, Z., Hao, J., and Zhang, W. (2022). Hypoxia-preconditioned extracellular vesicles from mesenchymal stem cells improve cartilage repair in osteoarthritis. Membranes 12 (2), 225. doi:10.3390/membranes12020225
Zhang, C. S., Shao, K., Liu, C. W., and Li, C. J. (2019). Hypoxic preconditioning BMSCs-exosomes inhibit cardiomyocyte apoptosis after acute myocardial infarction by upregulating microRNA-24. Eur. Rev. Med. Pharmacol. Sci. 23 (15), 6691–6699. doi:10.26355/eurrev_201908_18560
Zhang, D., Xuan, J., Zheng, B. B., Zhou, Y. L., Lin, Y., Wu, Y. S., et al. (2017). Metformin improves functional recovery after spinal cord injury via autophagy flux stimulation. Mol. Neurobiol. 54 (5), 3327–3341. doi:10.1007/s12035-016-9895-1
Zhang, L., Wei, Q., Liu, X., Zhang, T., Wang, S., Zhou, L., et al. (2021). Exosomal microRNA-98-5p from hypoxic bone marrow mesenchymal stem cells inhibits myocardial ischemia-reperfusion injury by reducing TLR4 and activating the PI3K/Akt signaling pathway. Int. Immunopharmacol. 101 (Pt B), 107592. doi:10.1016/j.intimp.2021.107592
Zhang, P., Guo, Y., Gao, Y., Wang, Z., Li, B., Zhang, X., et al. (2019). Exosomes derived from human umbilical cord mesenchymal stem cells promote myocardial repair after myocardial infarction under hypoxia. CSTPCD 23 (17), 2630–2636. doi:10.3969/j.issn.2095-4344.1716
Zhang, T., Yan, S., Song, Y., Chen, C., Xu, D., Lu, B., et al. (2022). Exosomes secreted by hypoxia-stimulated bone-marrow mesenchymal stem cells promote grafted tendon-bone tunnel healing in rat anterior cruciate ligament reconstruction model. J. Orthop. Transl. 36, 152–163. doi:10.1016/j.jot.2022.08.001
Zhang, X. F., Wang, T., Wang, Z. X., Huang, K. P., Zhang, Y. W., et al. (2021). Hypoxic ucMSC-secreted exosomal miR-125b promotes endothelial cell survival and migration during wound healing by targeting TP53INP1. Mol. Ther. Nucleic acids 26, 347–359. doi:10.1016/j.omtn.2021.07.014
Zhu, F., Wei, C., Wu, H., Shuai, B., Yu, T., Gao, F., et al. (2022). Hypoxic mesenchymal stem cell-derived exosomes alleviate ulcerative colitis injury by limiting intestinal epithelial cells reactive oxygen species accumulation and DNA damage through HIF-1α. Int. Immunopharmacol. 113 (Pt A), 109426. doi:10.1016/j.intimp.2022.109426
Zhu, J., Lu, K., Zhang, N., Zhao, Y., Ma, Q., Shen, J., et al. (2018). Myocardial reparative functions of exosomes from mesenchymal stem cells are enhanced by hypoxia treatment of the cells via transferring microRNA-210 in an nSMase2-dependent way. Artif. cells, nanomedicine, Biotechnol. 46 (8), 1659–1670. doi:10.1080/21691401.2017.1388249
Keywords: hypoxic preconditioning, mesenchymal stem cells, exosomes, microRNA, molecular mechanism
Citation: Zhuo H, Chen Y and Zhao G (2024) Advances in application of hypoxia-preconditioned mesenchymal stem cell-derived exosomes. Front. Cell Dev. Biol. 12:1446050. doi: 10.3389/fcell.2024.1446050
Received: 12 June 2024; Accepted: 12 August 2024;
Published: 21 August 2024.
Edited by:
Stefania Bruno, University of Turin, ItalyReviewed by:
Naseem Ahamad, The University of Texas Health Science Center at San Antonio, United StatesCopyright © 2024 Zhuo, Chen and Zhao. This is an open-access article distributed under the terms of the Creative Commons Attribution License (CC BY). The use, distribution or reproduction in other forums is permitted, provided the original author(s) and the copyright owner(s) are credited and that the original publication in this journal is cited, in accordance with accepted academic practice. No use, distribution or reproduction is permitted which does not comply with these terms.
*Correspondence: Guifang Zhao, emhhb2dmMTFAbWFpbHMuamx1LmVkdS5jbg==
Disclaimer: All claims expressed in this article are solely those of the authors and do not necessarily represent those of their affiliated organizations, or those of the publisher, the editors and the reviewers. Any product that may be evaluated in this article or claim that may be made by its manufacturer is not guaranteed or endorsed by the publisher.
Research integrity at Frontiers
Learn more about the work of our research integrity team to safeguard the quality of each article we publish.