- 1Institute for Tissue Engineering and Regenerative Medicine, The Chinese University of Hong Kong, Shatin, Hong Kong SAR, China
- 2School of Biomedical Sciences, Faculty of Medicine, The Chinese University of Hong Kong, Shatin, Hong Kong SAR, China
- 3Center for Neuromusculoskeletal Restorative Medicine (CNRM), Hong Kong Science Park, Shatin, Hong Kong SAR, China
- 4Department of Orthopaedics and Traumatology, Faculty of Medicine, The Chinese University of Hong Kong, Shatin, Hong Kong SAR, China
Insulin-like Growth Factor-Binding Protein 7 (IGFBP7) is an extracellular matrix (ECM) glycoprotein, highly enriched in activated vasculature during development, physiological and pathological tissue remodeling. Despite decades of research, its role in tissue (re-)vascularization is highly ambiguous, exhibiting pro- and anti-angiogenic properties in different tissue remodeling states. IGFBP7 has multiple binding partners, including structural ECM components, cytokines, chemokines, as well as several receptors. Based on current evidence, it is suggested that IGFBP7’s bioactivity is strongly dependent on the microenvironment it is embedded in. Current studies indicate that during physiological angiogenesis, IGFBP7 promotes endothelial cell attachment, luminogenesis, vessel stabilization and maturation. Its effects on other stages of angiogenesis and vessel function remain to be determined. IGFBP7 also modulates the pro-angiogenic properties of other signaling factors, such as VEGF-A and IGF, and potentially acts as a growth factor reservoir, while its actual effects on the factors’ signaling may depend on the environment IGFBP7 is embedded in. Besides (re-)vascularization, IGFBP7 clearly promotes progenitor and stem cell commitment and may exhibit anti-inflammatory and anti-fibrotic properties. Nonetheless, its role in inflammation, immunomodulation, fibrosis and cellular senescence is again likely to be context-dependent. Future studies are required to shed more light on the intricate functioning of IGFBP7.
Introduction
The gene for insulin-like growth factor-binding protein 7 (IGFBP7) was initially identified and characterized as a gene suppressed in meningiomas. Today, IGFBP7 is also known under various aliases, including insulin-like growth factor binding protein-related protein 1 (IGFBP-rp1), MAC25, angiomodulin (AGM), tumor-derived adhesion factor (TAF), or prostacyclin-stimulating factor (PSF) (Murphy et al., 1993).
This 30 kDa secretory glycoprotein was classified as a member of the insulin-like growth factor-binding protein (IGFBP) superfamily, due to its ability to bind insulin growth factor (IGF). Noteworthily, it only shares about 20%–25% sequence homology with other proteins of the IGFBP family, while its ability to bind IGF was reported to be 100 times weaker than that of other IGFBPs (Oh et al., 1996).
IGFBP7 is expressed in various tissues and by various cell types such as peripheral nerves, smooth muscle cells, and epithelial cells, while lymphocytes, plasma cells, and adipocytes did not express IGFBP7. It was also noted that the intensity of IGFBP7 expression within the same cell type, such as kidney epithelial cells, varied spatially within the organ, suggesting that IGFBP7 may have specific functions in these organs (Akiel et al., 2014).
IGFBP7 is upregulated in the angiogenic vasculature during physiological (Li Y. et al., 2023) and some pathological processes, is expressed by endothelial cells, perivascular and stromal cells, and is predominantly localized in the extracellular matrix (ECM) (Akaogi et al., 1996a; Usui et al., 2002). There it binds to various ECM components such as collagen IV (Col IV) (Akaogi et al., 1996a) and heparan sulfate (Sato et al., 1999) and is well embedded within the vascular basement membrane (Akiel et al., 2014). It was also found to be stored in the Weibel Palade bodies of endothelial cells (Van Breevoort et al., 2012). IGFBP7 can bind to insulin (Oh et al., 1996), activin (Kato, 2000), various chemokines such as C-C motif chemokine 5 (CCL5), C-C motif chemokine 21 (CCL21), and C-X-C motif chemokine 10 (CXCL10) (Nagakubo et al., 2003), as well as growth factors such as vascular endothelial growth factor A (VEGF-A) (Usui et al., 2002) and IGF (Oh et al., 1996). Known receptors of IGFBP7 are IGF-I receptor (Evdokimova et al., 2012), integrin αvβ3 (Komiya et al., 2014), and CD93 (Sun et al., 2021), a C-type lectin transmembrane receptor.
IGFBP7 is mainly studied in tumorigenesis (Kato, 2000; Jiang et al., 2008; Chen R. Y. et al., 2010; Chen et al., 2015; Tomimaru et al., 2012; Rupp et al., 2015; Li et al., 2019; Li et al., 2023a; Zhang et al., 2019; Hong et al., 2023), acute kidney failure (Titeca-Beauport et al., 2019; Esmeijer et al., 2021; Yu et al., 2022), as well as in liver (Yan et al., 2019) and cardiovascular diseases (Zhang et al., 2022). Despite all efforts invested into researching IGFBP7, the biological function of this protein is not well understood. Within the tumorigenesis context, accumulating studies clarified that IGFBP7 is upregulated in some types of cancers, while downregulated in others (Liu Y. et al., 2023). These entirely different manifestations suggest that IGFBP7 may function as a “double-edged sword” in cancer cell growth and progression, displaying an ambiguous action in distinct types of cancers (Jin et al., 2020). For example, IGFBP7 was previously reported to act as an oncogene in some cancers (Jiang et al., 2008; Rupp et al., 2015; Li D. et al., 2023; Hong et al., 2023), but also exhibited tumor-suppressing properties in others (Kato, 2000; Chen R. Y. et al., 2010; Chen et al., 2015; Tomimaru et al., 2012; Li et al., 2019; Zhang et al., 2019). Likewise, the role of IGFBP7 in neovascularization has been the subject of significant research, and conflicting biological functions of IGFBP7 during angiogenesis have been described.
This review provides a new and critical perspective on the complex role of IGFBP7 in angiogenesis and vascular remodeling in embryonic development, as well as re-vascularization during physiological and pathological conditions. It also highlights new insights into the role of IGFBP7 in other tissue remodeling scenarios such as progenitor cell commitment, inflammation, immunomodulation, fibrosis and cellular senescence. Based on the critical analysis of current research studies, we conclude that IGFBP7 acts in unison with its ECM microenvironment, resulting in its biological activity being context-dependent.
IGFBP7 in physiological vasculature formation
Upregulated IGFBP7 expression has been observed in activated vasculature during various biological processes including tissue injury and repair, wound healing, and during embryonic development. Here the angiogenic role of IGFBP7 will be dissected based on various remodeling vasculature scenarios, experimental approaches and key findings.
IGFBP7 in vascular development
In humans, mutation of IGFBP7 was linked to familial retinal arterial macro aneurysms (Abu-Safieh et al., 2011). In an IGFBP7lacZ/+ mouse model IGFBP7 was expressed in the vasculature by E10.5 and increased in intensity throughout embryogenesis, suggesting that it may play a role in embryonic angiogenesis (Hooper et al., 2009). In a zebrafish model, whole mount in situ hybridization was utilized to examine the expression of IGFBP7 during zebrafish embryogenesis. IGFBP7 transcripts were detected in the vasculature of the head, eye, and trunk and more specifically in the vascular endothelium, although it was not exclusive to developing vasculature. Knockdown of IGFBP7 using morpholinos resulted in a multifactorial phenotype consistent with angiogenic defects, including an obvious reduction in blood flow through intersomitic vessels, which usually form via sprouting angiogenesis from the dorsal aorta. These defects in circulation were accompanied by pericardial oedema, suggestive of vascular sprouting and patterning defects (Hooper et al., 2009). Intriguingly, the IGFBP7 loss-of-function phenotype was strikingly similar to the phenotype of VEGF-A knockdown and their double knockdown resulted in synergistic effects, including strongly disrupted endothelial sprouting. When VEGF-A was overexpressed, the compensatory induction of the VEGF receptor 2 (VEGFR-2) was blocked by the simultaneous IGFBP7 knockdown, suggesting an interaction between IGFBP7 and VEGF-A, where IGFBP7 potentially modulates vascular remodeling in part by temporizing the pro-angiogenic effects of VEGF-A (Hooper et al., 2009).
A comparative transcriptome study that investigated the role of IGFBP7 in central nervous system (CNS) and blood-brain-barrier (BBB) development in mice at E13.5 revealed that IGFBP7 expression was significantly increased in cortical endothelial cells as compared to lung endothelial cells (Bar et al., 2020). Moreover, transcript levels of IGFBP7 in cortex endothelial cells were comparable to levels of the most commonly used BBB markers, while pan-endothelial markers exhibited similar levels in both endothelial cell types. Histological evaluation of protein levels confirmed that IGFBP7 was found in cortical vasculature, where it co-localized with endothelial cells and pericytes. Interestingly, IGFBP7 could still be detected in adult CNS vasculature, as well as CNS parenchyma. Indeed, researchers found that from embryonic stages E14.5 onwards IGFBP7 was expressed in all CNS vasculature, including choroid plexus vasculature, and therefore should not be considered as a BBB-specific marker or inducer at these stages.
The consistent expression of IGFBP7 in developing vasculature across organs and species (Hooper et al., 2009; Bar et al., 2020), suggests that IGFBP7 may have a crucial role in related angiogenic processes. Especially since its knockdown resulted in reduced vascularization, oedema, and patterning defects (Hooper et al., 2009), it may be crucial for the formation of functional vasculature during development (Figure 1; Table 1).
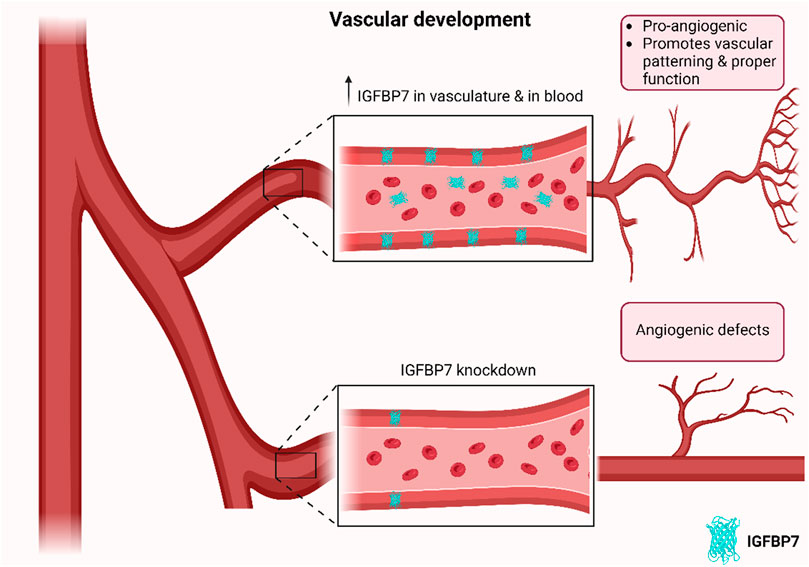
Figure 1. Schematic of effects of IGFBP7 on developing vasculature. IGFBP7 is upregulated in developing vasculature across organs and species, while its depletion results in angiogenic defects, suggesting that IGFBP7 may have a crucial role in related angiogenic processes in developing vasculature (Schematic was generated using BioRender.com with an academic license).
IGFBP7 in activated physiological post-natal vasculature
In IGFBP7lacZ/+ mice, IGFBP7 was upregulated in the granulation tissue of regenerating skin post-wounding and in neoangiogenic tufts in an oxygen-induced retinopathy model. In a model of hemangiogenesis, where myelosuppression causes an angiogenic response in bone marrow sinusoids, IGFBP7 was upregulated in sinusoidal endothelial cells (Hooper et al., 2009). Immunofluorescent staining also confirmed that IGFBP7 was upregulated in the vasculature of patients with post-traumatic brain injury (Wang et al., 2021) and stroke (Buga et al., 2014). These findings suggest that IGFBP7 was upregulated during physiological angiogenesis and may be involved in vascular repair and remodeling.
Co-injection of VEGF-A and soluble IGFBP7 sub-dermally (Miles assay) confirmed that VEGF-A-induced hyperpermeability of blood vessels could be abolished by IGFBP7, suggesting it may modulate VEGF-A mediated processes (Bar et al., 2020). Indeed, some studies exist that even reported anti-angiogenic properties of IGFBP7. In vivo, intraocular injection of soluble IGFBP7 in young post-natal mice resulted in a marked inhibition of retinal angiogenesis and severe patterning defects in retinal vessels, while early injection of soluble IGFBP7 into a calvarial bone defect in adult rats resulted in impaired angiogenesis and reduced bone mineral density (Sun et al., 2022).
In vitro, recombinant IGFBP7 inhibited VEGF-A- and luteinizing hormone (LH)-induced proliferation, migration, and tube formation of rat luteal microvascular endothelial cells (LECs), while IGFBP7 had no effects on any of the biological processes by itself (Tamura et al., 2009; Tamura et al., 2014). Another study demonstrated that treatment of human umbilical vein endothelial cells (HUVECs) with exogenous IGFBP7 could reduce their tube formation ability (Liu F. et al., 2023), spheroid sprouting, and downregulate CD34, VEGFR-2, C-X-C chemokine receptor type 4 (CXCR-4), and Ephrin B2 (EFNB2) expression (Sun et al., 2022). IGFBP7 was also reported to attenuate phosphorylation of mitogen-activated protein kinase (MAPK), extracellular signal-regulated kinase 1/2 (ERK1/2), VEGF-A-enhanced cyclooxygenase-2 (COX-2) mRNA expression and prostaglandin E2 (PGE2) secretion in HUVECs (Tamura et al., 2009) and rat LECs (Tamura et al., 2014; Tamura et al., 2014; Tamura et al., 2014; Tamura et al., 2014). Moreover, the knockdown of endogenous IGFBP7 in HUVECs enhanced COX-2 and VEGF mRNA expression (Tamura et al., 2009). Congruently, another study showed that the knockdown of IGFBP7 in rhesus monkey RF/6A choroidal endothelial cells promoted cell viability, cell migration and tube formation in vitro. This coincided with increased levels of VEGF and phosphorylated RAF/MAPK/ERK and the observed effect could be attenuated with the addition of exogenous IGFBP7 (Zhu et al., 2020).
Nonetheless, it is important to note that a range of studies exists that reported contrasting and pro-angiogenic effects of IGFBP7. As such, VEGF-A was reported to be able to induce IGFBP7 expression in HUVECs (Komiya et al., 2014). The knockdown of IGFBP7 gene expression inhibited tube formation in HUVECs, while the addition of exogenous IGFBP7 protein increased their tube formation and migration (Sun et al., 2021). Upon knockdown of IGFBP7 receptor CD93 in HUVECs, IGFBP7 protein lost this promoting effect (Sun et al., 2021). Similarly, neutralizing antibodies against IGFBP7 inhibited bovine capillary endothelial cell sprouting in vitro (Van Beijnum et al., 2006), while exogenous IGFBP7 addition promoted tube formation of human brain endothelial cells on Matrigel (Pen et al., 2008). This is in stark contrast to the above-mentioned studies (Tamura et al., 2009; Tamura et al., 2014).
Besides endothelial cells, various stromal cells including fibroblasts (Newman et al., 2011; Velázquez-Enríquez et al., 2021; Moita et al., 2022) and mesenchymal stem cells (MSCs) (Lam et al., 2022) are known to secrete IGFBP7. When HUVECs were co-cultured with adipose-derived stem cells (ASCs), optionally overexpressing IGFBP7, tube formation was notably reduced for wild-type and genetically modified ASCs. This effect could be abrogated, when IGFBP7 was knocked down in ASCs prior to co-culture (Liu F. et al., 2023). Comparably, conditioned medium derived from osteogenically induced MSCs inhibited HUVEC tube formation, sprouting and migration (Sun et al., 2022), while silencing of IGFBP7 expression in these MSCs was able to counter the inhibition. Proteomic analysis indicated that proteins functionally related to cell movement and cellular cytoskeleton organization exhibited significant changes in HUVECs exposed to soluble IGFBP7. Indeed, these HUVECs exhibited less cell polarization, slower migration and impaired tip-cell specification (Sun et al., 2022).
On the other hand, it is essential to note that stromal cells such as MSCs and fibroblasts, as well as their paracrine factors, are generally believed to promote angiogenic processes, while the extent of this bioactivity is influenced by stromal cell heterogenicity (Chang et al., 2002; Jiang et al., 2020), cell culture duration and conditions (Newman et al., 2011). In fact, a high throughput bioassay based on a vasculogenesis model in microfluidic devices demonstrated that MSCs derived from multiple donors at different passages exhibited significant heterogeneity in their ability to support HUVEC lumen formation and maintenance in co-cultures. MSCs’ vasculogenic bioactivity was also positively correlated with the baseline expression of several genes including IGFBP7 (Lam et al., 2022). The validity of these results was further supported by an independent study investigating the influence of immortalized fibroblasts from different passages on lumenogenesis of immortalized HUVECs (Wan et al., 2021). HUVECs could only form functional perfusable microvascular networks with immortalized fibroblasts from earlier passages but not later passages, which correlated with a decrease of Thy1 expression in fibroblasts from later passages. Compared to Thy1− fibroblasts, Thy1+ fibroblasts expressed higher IGFBP2, IGFBP7, and secreted protein acidic and rich in cysteine (SPARC). Although the addition of IGFBP7 and SPARC together partially rescued morphological defects in microvascular networks formed with Thy1- fibroblasts, supplementing the combination of all three factors fully rescued those defects. These findings were further confirmed by knocking out IGFBP7 and SPARC individually or together in fibroblasts (Wan et al., 2021).
Consistently, when normal human lung fibroblasts were co-cultured with HUVECs in a 3-dimensional fibrin gel bead assay, robust sprouting and lumenogenesis were observed (Newman et al., 2011). Substituting fibroblasts with fibroblast-derived conditioned medium also promoted endothelial sprouting and lumen formation, although to a lesser extent. In the absence of fibroblasts, a combination of angiopoietin-1 (ANG-1), angiogenin, heypatocyte growth factor (HGF), transforming growth factor-α (TGF-α), and tumor necrosis factor (TNF) drove robust endothelial cell sprouting without the formation of lumina. Subsequent addition of fibroblast-conditioned medium restored lumenogenesis. The study also claimed that combinational knockdown of IGFBP7, collagen I (Col I), procollagen C endopeptidase enhancer 1 (PCOLCE), SPARC and TGF-β–induced protein ig-h3 (βig-h3) in fibroblasts impaired lumen formation significantly, without affecting the sprouting process, whereas individual knockdown of any of the aforementioned proteins did not influence lumenogenesis. The addition of IGFBP7, together with Col I and SPARC, to fibrin gels resulted in increased stiffness of these hydrogels and could rescue the impaired lumen formation in knockdown experiments (Newman et al., 2011). The biomechanical properties of the microenvironment are known to affect angiogenesis. Substrates that are too stiff (Saunders and Hammer, 2010) or too soft (Califano and Reinhart-King, 2008; Guo et al., 2022) can impair angiogenic processes in vitro and in vivo. Hence, the current studies suggest that IGFBP7 may partially modulate angiogenic processes by modulating matrix stiffness.
Recently, it was even reported that IGFBP7 was embedded in the insoluble ECM of cultured MSCs and that matrices with enhanced pro-angiogenic properties were strongly enriched in IGFBP7, besides other pro-angiogenic components (Spater et al., 2022). Indeed, these MSC-derived ECM-based biologics most significantly facilitated the expression of pro-angiogenic cytokines in macrophages, promoted HUVEC proliferation and sprouting in vitro, and enhanced revascularization in mouse full-thickness skin wounds in vivo (Spater et al., 2022).
Although the reported in vitro results are inconsistent, at the current stage, there is a strong notion of IGFBP7 being crucial in lumen formation and, thus, in later stages of angiogenesis (Figure 2; Table 2). The role of IGFBP7 in earlier angiogenic stages, such as endothelial cell proliferation, migration, and sprouting remains to be determined (Figure 2; Table 2), especially since the physiological relevance of the performed in vitro studies needs to be taken into consideration. Experiments conducted in microphysiological systems, such as vasculogenesis-based assays in microfluidic devices, are more physiologically relevant than tube formation assays on Matrigel (Beyer et al., 2021; Lam et al., 2022; Wan et al., 2023). Here, microvascular network-on-a-chip experiments have shown that IGFBP7 is not acting alone, but in synergy with other secreted ECM proteins (Wan et al., 2021; Lam et al., 2022). Furthermore, current reports on IGFBP7 modulating matrix stiffness in these microphysiological systems point to IGFBP7 being embedded within the microenvironment, thus acting as part of a multifactorial insoluble milieu (Newman et al., 2011). In fact, insoluble MSC-derived ECM enriched in IGFBP7 exhibited strong angiogenic effects in vitro and in vivo (Spater et al., 2022). This is in stark contrast to studies that utilized exogenous soluble IGFBP7 alone or as part of a soluble secretome in the supernatant of 2-dimensional cultures (Tamura et al., 2009; Tamura et al., 2014; Sun et al., 2022; Liu F. et al., 2023), where IGFBP7 would potentially act outside its inherent context, which may explain the conflicting results. Furthermore, this is also in line with in vivo experiments, where the introduction of soluble IGFBP7 resulted in anti-angiogenic properties (Sun et al., 2022), while knockdown experiments in the developing zebrafish (Hooper et al., 2009) and high expression of IGFBP7 in angiogenic developing (Hooper et al., 2009; Bar et al., 2020) and adult vasculature (Hooper et al., 2009; Buga et al., 2014; Wang et al., 2021) pointed to pro-angiogenic properties of IGFBP7. Indeed, soluble IGFBP7 may even compete with ECM-bound IGFBP7 for receptor binding, resulting in opposite or inhibitory effects. Overall, bioactivities of IGFBP7, while embedded in its integral microenvironment, are believed to be more physiologically pertinent.
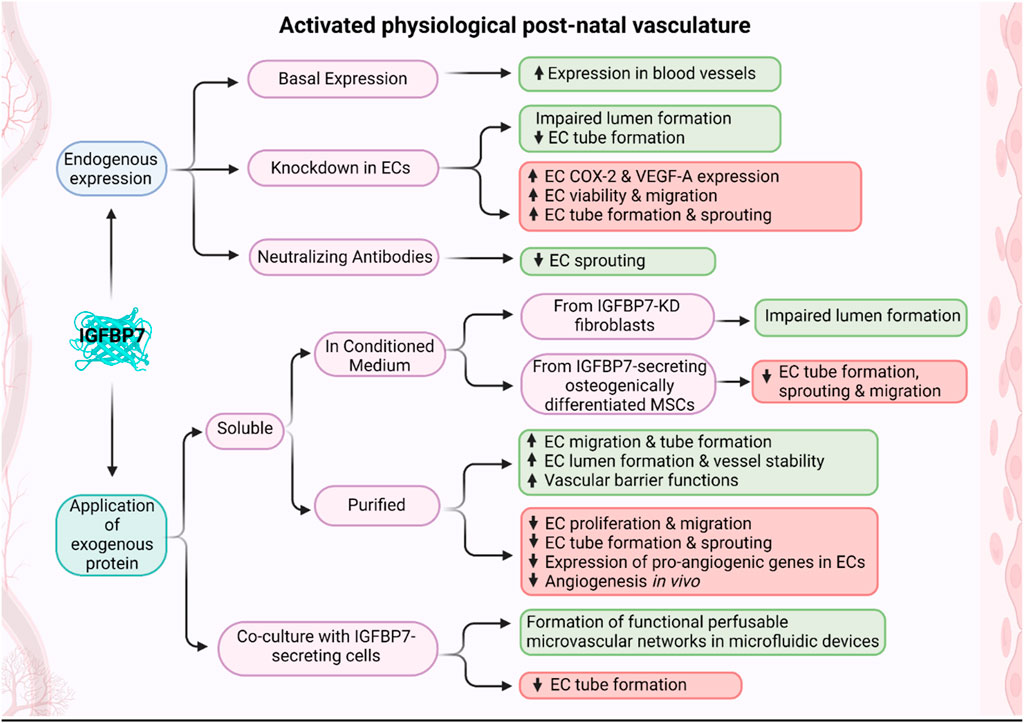
Figure 2. Schematic of effects of IGFBP7 on activated post-natal vasculature. Depending on the experimental design, when IGFBP7 was either added exogenously or its endogenous expression/activity modified, pro-angiogenic (green boxes) or anti-angiogenic (red boxes) properties of IGFBP7 were reported (Schematic was generated using BioRender.com with an academic license).
IGFBP7 in tumor vasculature formation
The aggressive growth of cancer cells and the associated overexpression of pro-angiogenic factors often lead to the development of disorganized blood vessel networks that are immature, tortuous, hyperpermeable and fundamentally different from normal vasculature (Siemann, 2011). The role of IGFBP7 in pathological tumor vasculature is thus reviewed separately.
Within the tumorigenesis context, IGFBP7 was suggested to function as a “double-edged sword” in cancer progression, acting as an oncogene in some and as a tumor-suppressor in other cancer types (Jin et al., 2020). Likewise, an ambiguous role of IGFBP7 was reported in tumor angiogenesis. Vascularization is a critical process in tumor growth and is therefore investigated as a promising therapeutic target (Li Y. et al., 2023). The IGFBP7 receptor CD93 has been identified as one of the top 20 genes in tumor angiogenesis. Studies have shown that tumor development in CD93 knockout mice or loss of CD93 in endothelial cells were associated with disruption of endothelial junctions and increased vascular permeability. In contrast, it was also demonstrated that the IGFBP7-CD93 axis was related to disordered tumor vasculature and that CD93 neutralizing antibody could normalize tumor vasculature. The authors illustrated that neutralizing antibodies against IGFBP7 administered to tumor-bearing mice revealed no differences in blood vessel density, but improved pericyte coverage, indicative of vessel maturation (Sun et al., 2021). Blockade of the CD93/IGFBP7 interaction by monoclonal antibodies thus promoted vascular maturation and reduced leakage, leading to reduced tumor hypoxia and increased tumor perfusion (Figure 3; Table 3). This promoted drug delivery, resulting in an improved antitumor response to chemo- and immuno-therapy (Sun et al., 2021).
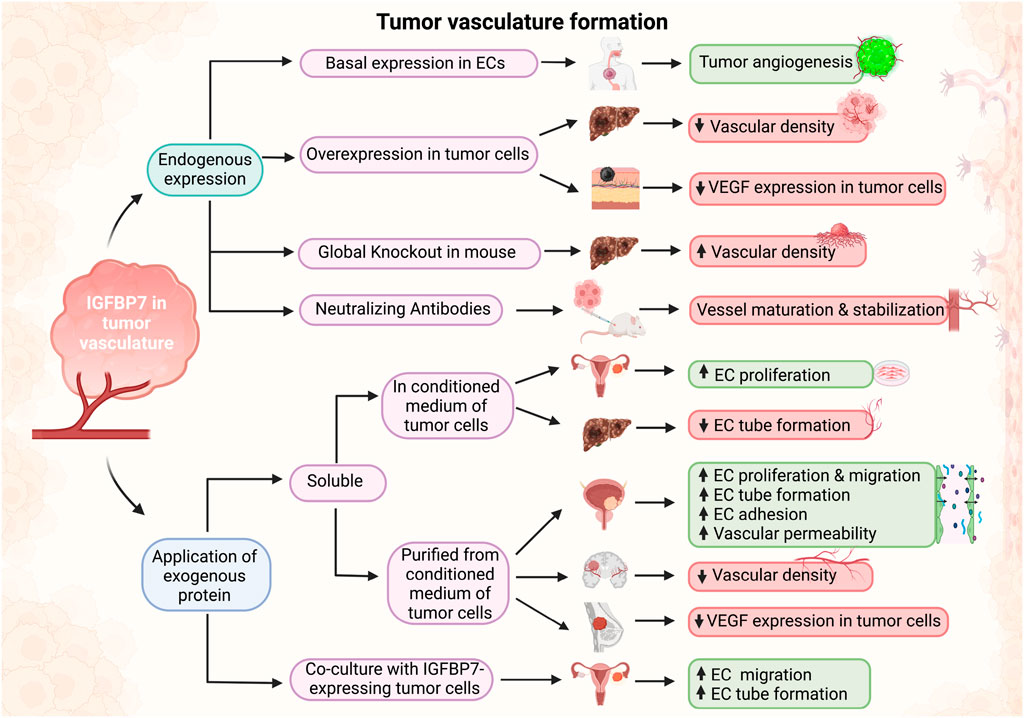
Figure 3. Schematic of effects of IGFBP7 in tumor vasculature formation. Depending on the experimental design, when IGFBP7 was either added exogenously or its endogenous expression/activity modified, pro-angiogenic (green boxes) or anti-angiogenic (red boxes) properties of IGFBP7 were reported (Schematic was generated using BioRender.com with an academic license).
Integrin αvβ3 was identified as another receptor of IGFBP7 in HUVECs. When isolated from conditioned medium of human bladder carcinoma cells and coated on cell culture plates, IGFBP7 did not stimulate the growth or migration of HUVECs, but enhanced their efficient adhesion (Komiya et al., 2014). This result was consistent with a previous study showing that IGFBP7 facilitated ECV-304 HUVEC attachment to Col IV (Akaogi et al., 1996a). IGFBP7 was also implicated in the formation of tube-like structures of endothelial cells in collagen I hydrogels, while these effects could be abolished when exogenous heparin was added to compete with the binding of IGFBP7 to cell surface heparan sulfate (Akaogi et al., 1996a). IGFBP7 also promoted the retraction of endothelial cells by inducing actin stress fibers and loosening their VE-cadherin-mediated intercellular junction. Consequently, IGFBP7 increased vascular permeability both in vitro and in vivo (Komiya et al., 2014) (Figure 3; Table 3).
IGFBP7 expression was significantly downregulated in human hepatocellular carcinoma (HCC) tissue samples and cell lines as compared with normal liver and hepatocytes, respectively. Forced overexpression of IGFBP7 in HCC cells induced senescence, and profoundly suppressed in vivo tumor growth. When HCC cells overexpressing IGFBP7 were implanted in a chicken chorioallantoic membrane (CAM) assay, angiogenesis was significantly reduced in the formed tumor masses. Similarly, HUVECs exposed to a conditioned medium from these cells exhibited reduced tube formation on Matrigel (Chen et al., 2011). Hence, reduced tumor growth may at least be partially attributed to reduced revascularization due to IGFBP7 overexpression. Increased CD31 staining in hepatocarcinoma tissues was also noted in IGFBP7−/− mice, which were reported to be more susceptible to developing tumors (Akiel et al., 2017). Congruently, xenografts of human HCC HepG3 cells overexpressing IGFBP7 showed downregulation in vessel density (Chen et al., 2011) (Figure 3; Table 3).
Exogenously applied IGFBP7 also reduced proliferation and induced senescence in glioblastoma (GBM) cell lines. When implanted in a CAM assay, GBM cells formed solid and highly vascularized tumors that were reduced when treated with soluble IGFBP7. Vessels in IGFBP7-treated tumors were clustered, unevenly distributed, and associated with a higher number of perivascular cells (Pen et al., 2011). Systematically administered IGFBP7 into mice with breast cancer cell line xenografts also resulted in reduced vessel density in xenografts sections (Benatar et al., 2012) (Figure 3; Table 3).
In other tumors, hypoxia, VEGF-A and TGF-β1 were associated with the expression of IGFBP7 in vascular endothelial cells, and indeed, IGFBP7 was described to be expressed at much higher levels in some tumors as compared to non-carcinogenic tissues (Pen et al., 2007; 2008; Hooper et al., 2009). In patients with metastatic carcinomas, IGFBP7 was reported to be highly expressed in circulating endothelial cells (CECs) (Smirnov et al., 2006), where the number of CECs correlated with the degree of tumor angiogenesis (Shaked et al., 2005). The proliferation of human ovarian microvascular endothelial cells (HOMECs) was noted to be enhanced when the cells were treated with a conditioned medium derived from epithelial ovarian cancer (EOC). Secretomics identified IGFBP7 as one of the enriched factors within the conditioned medium. When co-cultured with EOC cells, HOMECs exhibited enhanced migration in a transwell assay, as well as tube formation. Likewise, the addition of exogenous IGFBP7 promoted the proliferation, migration, and tube formation of HOMECs (Winiarski et al., 2013).
The tumor microenvironment (TME) plays a decisive role in tumor progression and angiogenesis. Hence, IGFBP7 may also affect these processes by modulating the TME (Xiuqing et al., 2022). Indeed, IGFBP7 was reported to be highly expressed in the stroma of some tumors and co-localized with activated cancer-associated fibroblasts (CAFs). In vitro analysis indicated that TGF-β1 can act as an important inducer of IGFBP7 expression in fibroblasts. IGFBP7, purified from the conditioned medium from bladder carcinoma cells, stimulated fibronectin and α-smooth muscle actin (α-SMA) synthesis in normal fibroblasts, as well as promoted fibroblast proliferation and migration. A transforming growth factor-β (TGF-β) signal inhibitor attenuated IGFBP7-induced expression of fibronectin and α-SMA, but did not affect IGFBP7’s stimulation of cell growth and migration, suggesting TGF-β dependent and independent mechanisms for IGFBP7-driven CAF activation, which is known to contribute to tumor progression (Komiya et al., 2012). Similar results were recently confirmed in gastric cancer, where IGFBP7 was mainly expressed by myofibroblastic CAFs (Hong et al., 2023). TGF-β signaling promoted the expression of IGFBP7 in CAFs, which in turn enhanced fibroblast growth factor-2 (FGF-2) expression in gastric cancer and resulted in increased macrophage infiltration and poor prognosis. Treatment of macrophages and gastric cancer cells with recombinant IGFBP7 further showed that IGFBP7 promoted tissue-associated macrophages (TAM)/M2 polarization via FGF-2/Fibroblast Growth Factor Receptor 1 (FGFR-1)/Phosphoinositide 3-kinases (PI3K)/Protein kinase B (Akt) axis (Li D. et al., 2023). Activation of CAFs and abundance of TAMs with a M2 phenotype are associated with enhanced angiogenesis and tumor progression (Wang et al., 2019).
Vascular permeability is an indicator of activated angiogenic vasculature (Dvorak H F et al., 1995) and this process is often dysregulated in tumors (Tomita et al., 2021). In contrast, increased vascular barrier functions indicate quiescent and mature vasculature (Vandoorne et al., 2010; Wan et al., 2023). IGFBP7 has been observed to be overexpressed in some tumors (Pen et al., 2007; Hooper et al., 2009; Liu Y. et al., 2023; Xu et al., 2023), while repressed in others (Murphy et al., 1993; Liu Y. et al., 2023; Xu et al., 2023). Similarly, IGFBP7 has been suggested to increase vascular permeability in some tumors, while decreasing it in others. It is noteworthy that the knockdown of IGFBP7 increased permeability, while its partial inhibition by neutralizing antibodies decreased permeability (Sun et al., 2021). The observed differences may be related to the degree of IGFBP7-based signaling being silenced in tumors, where its ablation led to vascular dysfunction, while partial neutralization may have downregulated its overactivation and thus normalized vasculature. For the inconsistent findings on vascular permeability upon application of soluble IGFBP7 (Komiya et al., 2014; Sun et al., 2022), it has to be taken into consideration that IGFBP7 acted outside its inherent microenvironment, potentially resulting in different bioactivity. This is further supported by the notion that integrin αvβ3 acts as a receptor for IGFBP7 and that the interaction of cells with immobilized IGFBP7 resulted in cytoskeletal reorganization (Komiya et al., 2014), suggesting that IGFBP7 may function via mechanotransduction, thus requiring IGFBP7 to be immobilized in the ECM for relevant signal transduction.
IGFBP7 expression is not restricted to the tumor vasculature but can be expressed by tumor cells, as well as stromal cells in the TME. Since it has been implicated by studies of physiological angiogenesis that IGFBP7 acts in synergy with other ECM components (Newman et al., 2011; Wan et al., 2021; Lam et al., 2022) and that IGFBP7’s bioactivity is dependent on the microenvironment, conflicting results observed in tumor angiogenesis and progression may be explained by differences in the composition of the TME in which IGFBP7 is embedded in. Furthermore, IGFBP7 may exert different functions in various cell types, with IGFBP7 signaling in CAFs or tumor vasculature overriding signaling in tumor cells and vice versa (Rupp et al., 2015; Li Y. et al., 2023). Hence, the indirect effects of IGFBP7 on tumor vasculature must also be considered, where IGFBP7 signaling on tumor cells may enhance or reduce their expression of pro-angiogenic factors (Chen R.-Y. et al., 2010; Benatar et al., 2012; Akiel et al., 2017; Zhu et al., 2020). Indeed, overexpression of IGFBP7 in B16-F10 melanoma cells downregulated the expression of VEGF (Chen R.-Y. et al., 2010). Congruently, increased VEGF levels were recorded in lung tumors of IGFBP7−/− mice (Akiel et al., 2017) and administration of exogenous IGFBP7 reduced VEGF expression in breast cancer cell lines 1833 xenografts implanted in mice (Benatar et al., 2012).
Ligand binding and regulation of down-stream signaling
To further understand the function of IGFBP7, its interaction with known ligands and downstream effects on signaling activation need to be examined.
Soluble IGFBP7 was shown to bind IGF-I in an immunoblotting assay (Oh et al., 1996). Treatment of mouse fibroblasts with soluble IGFBP7 promoted their proliferation, while the pro-proliferative effect of IGFBP7 was enhanced when IGF-I was added to cultures (Akaogi et al., 1996b). In acute lymphoblastic leukaemia (ALL) cells, soluble IGFBP7 was observed to synergistically activate the IGF-I receptor with IGF-I (Chen R.-Y. et al., 2010). These studies suggested that IGFBP7 could enhance IGF-I binding to its receptor and thus promote IGF signaling.
Nonetheless, IGFBP7, which contains an IGF-I receptor binding motif, was also reported to block IGF binding to its receptor and thus prevent the activation of downstream IGF-I receptor/Akt/Serine/threonine-protein kinase mTOR (mTOR)/Eukaryotic translation initiation factor 4E-binding protein 1 (4E-BP1) pathway in breast cancer cells. This suppressed expression of genes associated with cancer cell proliferation (Evdokimova et al., 2012). Another study in IGFBP7−/− mice partially supported these findings, as constitutive activation along the IGF-I receptor/Akt/Glycogen synthase kinase-3 beta (GSK-3 beta) axis was observed in hepatocytes and embryonic fibroblasts (Akiel et al., 2017).
IGFBP7 was also shown to bind insulin (Oh et al., 1996), and soluble IGFBP7 and insulin synergistically enhanced the proliferation of mouse fibroblasts (Akaogi et al., 1996b). In another study, it was reported that soluble IGFBP7 and insulin synergistically activated insulin receptor/Akt and/ERK1/2 pathways to promote the proliferation of ALL cells. IGFBP7 binding to the IGF-I receptor was also reported to protect the receptor from internalization, preventing it from lysosomal degradation and thus prolonging insulin-induced responses in stimulating the proliferation of ALL cells. Interestingly, IGFBP7 and insulin synergistically prolonged the activation of the IGF-I receptor, but not the insulin receptor (Artico et al., 2021). On the other hand, it was also suggested that binding of soluble IGFBP7 to insulin blocked insulin-stimulated insulin receptor activation in NIH-3T3 fibroblasts (Yamanaka et al., 1997). Other reports indicated that endogenously expressed IGFBP7 had no significant effects on insulin signaling in the IGFBP7-expressing human bladder carcinoma cell line EJ-1 and the human colon cancer cell line DLD-1 transfected with the IGFBP7 gene (Sato et al., 2007).
In contrast to other members of the IGFBP family, IGFBP7 exhibits a strong homology to follistatin (Kato, 2000). Similar to follistatin, IGFBP7 was found to bind activin A (Kato, 2000) and when supplemented to cultures, neutralized the activity of activin to inhibit osteogenesis in human bone marrow MSCs (Bolomsky et al., 2015), and promote steroidogenesis in rat ovarial granulosa cells (Tamura et al., 2007).
IGFBP7 also contains a heparin-binding motif (Sato et al., 1999; Evdokimova et al., 2012) and heparan sulfate is involved in the interaction of cells with IGFBP7. Indeed, when ECV-304 HUVECs and BALB/c3T3 mouse fibroblasts were treated with heparinase their ability to adhere to IGFBP7-coated plates was abolished (Sato et al., 1999). Furthermore, heparan sulfate was implicated in modulating IGFBP7’s activity. In fact, a truncated IGFBP7 protein, lacking the heparan sulfate binding motif abolished the binding of IGFBP7 to the IGF-I receptor (Evdokimova et al., 2012).
Other ligands of IGFBP7 are chemokines such as CCL5, CCL21 and CXCL10. It was suggested that IGFBP7 can bind those factors, while embedded in the basal lamina of blood vessels and present them to trafficking lymphocytes (Nagakubo et al., 2003). Congruently, IGFBP7 was shown to bind VEGF-A, when immobilized on a surface (Usui et al., 2002), while unable to bind VEGF, when added in its soluble form into cell culture supernatant (Tamura et al., 2009), suggesting again that IGFBP7 may exert distinct functions when properly embedded in its microenvironment. This is in line with studies on IGFBP7 modulating VEGF signaling, which reported conflicting results (Hooper et al., 2009; Tamura et al., 2009; Tamura et al., 2014; Sun et al., 2022).
Current results clearly indicate that IGFBP7 can act in an IGF-dependent and -independent manner. It can also modulate the signaling of other ligands, including VEGF (Usui et al., 2002), insulin (Oh et al., 1996) and activin (Kato, 2000), although the reports on resulting effects are conflicting, suggesting again that IGFBP7’s bioactivity may be context-dependent. IGFBP7’s ability to directly bind other cytokines and chemokines also opens the avenue of IGFBP7 acting as a signaling factor reservoir, where it can bind sequester, and/or present them to cells. Notably, IGFBP7 ligands such as VEGF (Leung et al., 1989), IGFs (Nakao-Hayashi et al., 1992; Lee et al., 2000), insulin (Nakao-Hayashi et al., 1992) and activin (Kaneda et al., 2011; de Oliveira et al., 2020) were implicated in angiogenic processes. The here-described signaling pathways may thus also be relevant in the modulation of angiogenic processes by IGFBP7.
IGFBP7 in stem cell commitment
Besides its crucial role in vasculature development, IGFBP7’s involvement in stem and progenitor cell commitment has been observed in adult and embryonic cells. During the differentiation of bovine pre-adipocytes into adipocytes, IGFBP7 was significantly upregulated. IGFBP7 gene overexpression and RNA interference promoted lipid accumulation and inhibited triglyceride production in mature adipocytes, respectively, suggesting that IGFBP7 may play a regulatory role in adipocyte commitment (Hu et al., 2021).
During the differentiation of human MSCs along the osteogenic lineage (Wan et al., 2022), overexpression of IGFBP7 enhanced the expression of osteo-specific genes and proteins, while IGFBP7 knockdown decreased osteogenesis-specific markers. Researchers demonstrated that IGFBP7 promoted osteogenic differentiation via the Wnt/β-catenin signaling pathway. Using a rat tibial osteotomy model, a sheet of IGFBP7–overexpressing MSCs improved bone healing over MSC-sheets with unmodified IGFBP7 expression levels (Zhang et al., 2018).
Intermittent administration of parathyroid hormone (PTH), an anabolic agent approved for the treatment of osteoporosis, was found to increase the expression of IGFBP7 in mouse MSCs and pre-osteoblasts. Anabolic effects of PTH were interrupted upon knockdown of IGFBP7 and supplementation of IGFBP7 could enhance the bone-forming efficacy of PTH, suggesting that IGFBP7 was involved in the bone-forming effects of PTH. Researchers also suggested that IGFBP7 may inhibit the mTOR pathway and enhance TGF-β/bone morphogenetic protein (BMP) and Wnt-β-catenin signaling cascades during osteogenic differentiation (Xia et al., 2022). Indeed, this was supported by other studies, where treatment with IGFBP7 was shown to upregulate the expression of BMP2 in MSCs (Xia et al., 2022) and fibroblasts (Lu et al., 2020). In vivo, bone healing was accelerated by the intravenous administration of IGFBP7 along with PTH in a mouse tibia fracture model (Xia et al., 2022).
Another study showed that IGFBP7 expression and secretion gradually increased during osteogenic differentiation of bone marrow-derived-MSCs, while during cranial bone defect healing IGFBP7 peaked at 2 weeks. Injection of soluble IGFBP7 into the defect side at day 4 of healing, potentially shifting this peak to an earlier time point, inhibited neo-vasculature in the defect region and reduced bone formation (Sun et al., 2022). Although the experiments were again performed using soluble IGFBP7, thus not within its inherent microenvironment, current results suggest that while IGFBP7 is important, coordination of temporal dosing is crucial for proper bone healing.
IGFBP7 was also found to be necessary for cardiac commitment of mouse embryonic stem cells (ESCs) and its regulation depended on the transcription factor TAp63, a p63 isoform. TAp63 directly activated both IGFBP7 and Activin-A during ESC cardiogenesis while these secreted factors modulated TAp63 gene expression by a feedback loop mechanism (Wolchinsky et al., 2014).
Collectively, current results suggest that IGFBP7 is involved in progenitor and stem cell commitment. Nonetheless, the amount of evidence is still relatively limited and future studies are needed to understand the role of IGFBP7 in cell differentiation processes.
IGFBP7 in tissue remodeling
IGFBP7 is also implicated in physiological and pathological tissue remodeling, including muscle hypertrophy, wound healing, as well as physiological and pathological fibrosis.
Besides being upregulated in the activated vasculature (Van Beijnum et al., 2006; Pen et al., 2008; Hooper et al., 2009; Tamura et al., 2009; Tamura et al., 2014; Abu-Safieh et al., 2011; Buga et al., 2014; Komiya et al., 2014; Bar et al., 2020; Zhu et al., 2020; Sun et al., 2021; Sun et al., 2022; Wan et al., 2021; Wang et al., 2021; Liu F. et al., 2023), IGFBP7 was reported also to modulate other processes and affect other cell types during wound healing. In vitro, IGFBP7-containing conditioned medium from MSCs, as well as recombinant IGFBP7 enhanced the migration of human small airway epithelial cells, suggesting that IGFBP7 may be involved in the MSC-mediated lung injury repair (Akram et al., 2013). IGFBP7 was also expressed in keratinocytes and the knockdown of IGFBP7 in these cells resulted in decreased susceptibility to TNFα-induced apoptosis but did not affect senescence. IGFBP7 silencing also downregulated genes associated with calcium-induced differentiation of keratinocytes (Severino et al., 2013), suggesting that IGFBP7 may also be involved in the re-epithelialization of skin wounds.
During dysregulated skin wound healing, such as keloid formation, IGFBP7 has been described to have inhibitory effects. Indeed, IGFBP7 expression was decreased in keloid tissues. Treatment of keloid fibroblasts with recombinant IGFBP7 in vitro decreased their proliferation and induced apoptosis, while their migration was unaffected. Analysis of the keloid fibroblast transcriptome and protein levels upon IGFBP7 treatment revealed decreased levels of TGF-β1, Col I, VEGF, and pro-inflammatory markers interleukin-6 (IL-6) and interleukin-8 (IL-8), while levels of TGF-β3 were increased. Similarly, co-culture of ASCs, known to express IGFBP7, inhibited keloid fibroblast proliferation, while their migration or apoptosis was unaffected. Knockdown of IGFBP7 in ASCs was able to mitigate the observed anti-proliferative effect. Congruently, ASCs were able to inhibit collagen I production in keloid fibroblasts, while there was no change when co-cultured with IGFBP7-silenced ASCs. Hence, current data suggest that IGFBP7 may have anti-inflammatory and anti-fibrotic properties during dysregulated wound healing. Moreover, the authors concluded that IGFBP-7 secreting ASCs inhibited keloid formation via the BRAF/MAPK/ERK signaling pathway (Liu F. et al., 2023).
In contrast, increased expression of IGFBP7 has been observed in patients with non-alcoholic fatty liver disease (NAFLD), which could be reproduced in a zebrafish model. IGFBP7 knockout significantly decreased lipid accumulation, inflammation, and liver fibrosis, whereas liver-specific IGFBP7 overexpression dramatically exacerbated liver fibrosis in the zebrafish NAFLD model, suggesting that IGFBP7 may act as an important regulator in NAFLD progression (Wang et al., 2023).
IGFBP7 was also found to be secreted by liver macrophages and regulate liver metabolism thereby contributing to insulin resistance. It was reported that obesity did not induce pro-inflammatory polarization of liver macrophages from mice and humans and obesity-induced insulin resistance occurred independently of liver macrophage activation. Nonetheless, liver macrophages from mice fed with a high-fat diet exhibited major changes in their transcriptome with IGFBP7 being one of the most upregulated genes. IGFBP7 knockdown in liver macrophages resulted in an upregulation of pro-inflammatory markers in these cells, while there was no major impact on inflammatory gene expression and immune cell content in the overall liver. Hence, IGFBP7 was suggested to exhibit anti-inflammatory or even immunomodulatory properties in liver macrophages. Moreover, IGFBP7 silencing in insulin-resistant animals improved glucose homeostasis, suggesting a detrimental role for IGFBP7 in metabolically impaired animals. Mechanistically, it was shown that IGFBP7 bound to the insulin receptor, enhanced insulin activation of Akt and induced lipogenesis and gluconeogenesis via activation of ERK signaling, confirming its direct involvement in insulin metabolism (Morgantini et al., 2019).
The immunomodulatory properties of IGFBP7 were also demonstrated in an experimental colitis model (Liao et al., 2016). IGFBP7 knockdown in MSCs significantly decreased their immunomodulatory properties, decreasing the antiproliferative functions of MSCs against T-cells, while also affecting the pro-inflammatory cytokine production of the T-cells in vitro. In a mouse experimental colitis model, intraperitoneally injected MSCs ameliorated the clinical and histopathological severity of induced colonic inflammation and restored the injured gastrointestinal mucosal tissues, while MSCs that were knocked down for IGFBP7 had minimal effects (Liao et al., 2016).
In addition to the anti-inflammatory effects, IGFBP7 was implicated in the protective effects of exercise on proliferating muscle satellite cells. Exercise is known to be beneficial for skeletal muscle homeostasis and regeneration, which is crucial as exhaustion of muscle satellite cells is correlated with muscle diseases. IGFBP7 was upregulated in satellite cells of exercising mice, leading to the inhibition of Akt phosphorylation, mTOR activity, and reduced mitochondrial metabolism, suggesting that exercise protected proliferative satellite cells against exhaustion via the IGFBP7/Akt/mTOR axis (Chen et al., 2020).
IGFBP7 has been described to be secreted by failing cardiomyocytes in advanced heart failure (Ko et al., 2022). In a pressure overload mouse heart failure model, IGFBP7 deficiency attenuated cardiac dysfunction by reducing cardiac inflammatory injury, tissue fibrosis, and cellular senescence. Antibody-mediated IGFBP7 neutralization reversed IGFBP7-induced suppression of Forkhead box O3 (FOXO3a), thereby restoring DNA repair and reactive oxygen species detoxification signals and attenuated heart failure in mice (Zhang et al., 2022). IGFBP7 also exhibited increased transcription levels in senescent human mammary epithelial cells (Chen et al., 2020) and MSCs (Severino et al., 2013), while being an integral part of the secretome of senescent MSCs that could induce senescence in young MSCs (Severino et al., 2013). Nonetheless, another study reported that IGFBP7 was able to protect MSCs from senescence and identified the underlying mechanism as the reduction of p21 transcription (Li et al., 2022).
Although conflicting results are reported, IGFBP7 appears to exhibit cell-protective, anti-inflammatory, immunomodulatory, and anti-fibrotic properties. Nonetheless, IGFBP7’s function may be affected by its microenvironment, as elaborated above, and it may exhibit opposite biological functions in strongly dysregulated environments, such as those found in NAFLD and heart failure.
Conclusion
Decades of research investigating the role of IGFBP7 in tissue (re-)vascularization confirmed that IGFBP7 indeed plays a substantial role in physiological and pathological angiogenesis, however its exact function is difficult to predict. IGFBP7 is highly expressed in activated developing, healing and remodeling vasculature, while its role in these processes is highly ambiguous. It is important to consider that the observed effects on angiogenesis are starkly different, when IGFBP7 is studied in its inherent context, namely, embedded within the native ECM, as compared to studies that supplemented soluble IGFBP7. Indeed, IGFBP7 is an ECM component, while the ECM is composed of a plethora of macromolecules that are assembled into a complex and precise network (Assunção et al., 2020b; Assunção et al., 2021), enabling signaling via biochemical and biophysical (spatial organization and mechanical properties) cues (Assunção et al., 2020a). The complex interplay of these properties synergistically enables intricate signaling and the modulation of biological processes (Chiang et al., 2021). It is therefore not surprising that IGFBP7 was shown to act in collaboration with other ECM components and signaling factors, suggesting that IGFBP7’s functional role in angiogenic processes is dependent on the ECM microenvironment and present signaling partners. This notion is supported by the fact that IGFBP7 has multiple binding partners within the ECM, including Col IV (Akaogi et al., 1996a) and heparan sulfate (Sato et al., 1999), is able to interact with cell surface heparan sulfate and various cell receptors. Integrin αvβ3 is especially noteworthy, as it suggests that IGFBP7 may act via mechanotransduction (Komiya et al., 2014; Driscoll et al., 2021), rendering it crucial for IGFBP7 to be properly embedded within the ECM. This understanding gives a possible explanation for the highly conflicting results obtained from research utilizing soluble IGFBP7 and suggests that they may not be as physiologically relevant.
Having established that IGFBP7’s bioactivity is microenvironment context-dependent, its contradictory effect on the activity of its various binding partners, such as IGF (Akaogi et al., 1996b; Evdokimova et al., 2012; Akiel et al., 2017; Artico et al., 2021) and VEGF (Hooper et al., 2009; Tamura et al., 2009) could also be explained. Indeed, the presence of synergistically promoting components or competitive ligands (e.g., presence of IGFBPs with higher affinity for IGF), relative abundance of relevant receptors on the cell surface, and cellular identity may be decisive in the role of IGFBP7 in angiogenesis. Moreover, IGFBP7’s ability to directly bind other cytokines and chemokines (Nagakubo et al., 2003) also opens the avenue of IGFBP7 acting as a signaling factor reservoir, where it can bind, sequester and/or present them to cells.
Consequently, IGFBP7 role in angiogenesis will be dependent on the composition of the local microenvironment, which is starkly different during development, physiological tissue healing and remodeling, as compared to the pathological context of the TME, dysregulated wound healing, NAFLD, and failing myocardium. Moreover, its effect may be strongly influenced by temporal, spatial, and concentration-dependent dosing. Nonetheless, current studies suggest that IGFBP7 promotes endothelial cell attachment, luminogenesis, and potentially vessel stabilization and maturation during physiological angiogenesis. Its effects on earlier stages of angiogenesis including endothelial cell proliferation, migration, and initial stages of sprouting, as well as vascular barrier functions, remain to be determined. It also modulates the pro-angiogenic properties of other signaling factors, such as VEGF and IGF, and their downstream signaling, introducing an additional level of complexity.
Besides vascularization, IGFBP7 is evidently involved in other biological processes in tissue development and remodeling. It has a promoting role in progenitor and stem cell commitment and is likely anti-inflammatory and anti-fibrotic. Nonetheless, its role in inflammation, immunomodulation, fibrosis, and cellular senescence is again likely to be context-dependent.
Future studies should thus explore the function of IGFBP7 in (re-)vascularization and tissue remodeling within its inherent microenvironment and compare it to functions of its soluble counterpart. This could involve in vivo studies with temporal tissue- or cell-specific depletion or over-expression of IGFBP7, as well as in vitro studies involving other ECM components, decellularized tissue-derived or cell culture-derived ECM. Especially in vitro studies would allow to introduce changes into the ECM microenvironment and investigate how these changes affect IGFBP7’s bioactivity, as well as explore involved molecular pathways. Studies in microphysiological systems and more complex physiologically relevant co-cultures will further allow to investigate how different cell sources of IGFBP7 and bioactive co-factors affect its biological functions. Advanced studies are thus required to shed more light on the intricate functioning of IGFBP7.
Author contributions
KL: Data curation, Investigation, Resources, Validation, Writing–original draft. ZZ: Data curation, Investigation, Visualization, Writing–review and editing. AB: Conceptualization, Formal Analysis, Funding acquisition, Investigation, Project administration, Supervision, Writing–original draft.
Funding
The author(s) declare that financial support was received for the research, authorship, and/or publication of this article. This work was supported by the general research fund [GRF, 14115723, (AB)] from the University Grants Committee, Hong Kong SAR and by the research fund to the Center for Neuromusculoskeletal Restorative Medicine from Health@InnoHK program launched by Innovation and Technology Commission, the Government of the Hong Kong Special Administrative Region of the People’s Republic of China (AB).
Acknowledgments
KKL and ZZ would like to acknowledge the Institute for Tissue Engineering and Regenerative Medicine and the School of Biomedical Sciences (SBS), CUHK, for their postgraduate scholarship.
Conflict of interest
The authors declare that the research was conducted in the absence of any commercial or financial relationships that could be construed as a potential conflict of interest.
Publisher’s note
All claims expressed in this article are solely those of the authors and do not necessarily represent those of their affiliated organizations, or those of the publisher, the editors and the reviewers. Any product that may be evaluated in this article, or claim that may be made by its manufacturer, is not guaranteed or endorsed by the publisher.
References
Abu-Safieh, L., Abboud, E. B., Alkuraya, H., Shamseldin, H., Al-Enzi, S., Al-Abdi, L., et al. (2011). Mutation of IGFBP7 causes upregulation of BRAF/MEK/ERK pathway and familial retinal arterial macroaneurysms. Am. J. Hum. Genet. 89, 313–319. doi:10.1016/j.ajhg.2011.07.010
Akaogi, K., Okabe, Y., Sato, J., Nagashimat, Y., Yasumitsu, H., Sugahara, K., et al. (1996a). Specific accumulation of tumor-derived adhesion factor in tumor blood vessels and in capillary tube-like structures of cultured vascular endothelial cells. Proc. Natl. Acad. Sci. U. S. A. 93, 8384–8389. doi:10.1073/pnas.93.16.8384
Akaogi, K., Sato, J., Okabe, Y., Sakamoto, Y., Yasumitsu, H., and Miyazaki, K. (1996b). Synergistic growth stimulation of mouse fibroblasts by tumor-derived adhesion factor with insulin-like growth factors and insulin. Cell Growth Differ. 7, 1671–1677.
Akiel, M., Guo, C., Li, X., Rajasekaran, D., Mendoza, R. G., Robertson, C. L., et al. (2017). IGFBP7 deletion promotes hepatocellular carcinoma. Cancer Res. 77, 4014–4025. doi:10.1158/0008-5472.CAN-16-2885
Akiel, M., Rajasekaran, D., Gredler, R., Siddiq, A., Srivastava, J., Robertson, C., et al. (2014). Emerging role of insulin-like growth factor-binding protein 7 in hepatocellular carcinoma. J. Hepatocell. Carcinoma 1, 9–19. doi:10.2147/JHC.S44460
Akram, K. M., Samad, S., Spiteri, M. A., and Forsyth, N. R. (2013). Mesenchymal stem cells promote alveolar epithelial cell wound repair in vitro through distinct migratory and paracrine mechanisms. Respir. Res. 14, 9. doi:10.1186/1465-9921-14-9
Artico, L. L., Laranjeira, A. B. A., Campos, L. W., Corrêa, J. R., Zenatti, P. P., Carvalheira, J. B. C., et al. (2021). Physiologic IGFBP7 levels prolong IGF1R activation in acute lymphoblastic leukemia. Blood Adv. 5, 3633–3646. doi:10.1182/bloodadvances.2020003627
Assunção, M., Dehghan-Baniani, D., Yiu, C. H. K., Später, T., Beyer, S., and Blocki, A. (2020a). Cell-derived extracellular matrix for tissue engineering and regenerative medicine. Front. Bioeng. Biotechnol. 8, 602009–602010. doi:10.3389/fbioe.2020.602009
Assunção, M., Wong, C. W., Richardson, J. J., Tsang, R., Beyer, S., Raghunath, M., et al. (2020b). Macromolecular dextran sulfate facilitates extracellular matrix deposition by electrostatic interaction independent from a macromolecular crowding effect. Mater. Sci. Eng. C 106, 110280. doi:10.1016/j.msec.2019.110280
Assunção, M., Yiu, C. H. K., Wan, H. Y., Wang, D., Ker, D. F. E., Tuan, R. S., et al. (2021). Hyaluronic acid drives mesenchymal stromal cell-derived extracellular matrix assembly by promoting fibronectin fibrillogenesis. J. Mater Chem. B 9, 7205–7215. doi:10.1039/d1tb00268f
Bar, O., Gelb, S., Atamny, K., Anzi, S., and Ben-Zvi, A. (2020). Angiomodulin (IGFBP7) is a cerebral specific angiocrine factor, but is probably not a blood-brain barrier inducer. Fluids Barriers CNS 17, 27–12. doi:10.1186/s12987-020-00188-2
Benatar, T., Yang, W., Amemiya, Y., Evdokimova, V., Kahn, H., Holloway, C., et al. (2012). IGFBP7 reduces breast tumor growth by induction of senescence and apoptosis pathways. Breast Cancer Res. Treat. 133, 563–573. doi:10.1007/s10549-011-1816-4
Beyer, S., Blocki, A., Cheung, M. C. Y., Wan, Z. H. Y., Mehrjou, B., and Kamm, R. D. (2021). Lectin staining of microvascular glycocalyx in microfluidic cancer cell extravasation assays. Life 11, 179–212. doi:10.3390/life11030179
Bolomsky, A., Hose, D., Schreder, M., Seckinger, A., Lipp, S., Klein, B., et al. (2015). Insulin like growth factor binding protein 7 (IGFBP7) expression is linked to poor prognosis but may protect from bone disease in multiple myeloma. J. Hematol. Oncol. 8, 10. doi:10.1186/s13045-014-0105-1
Buga, A. M., Margaritescu, C., Scholz, C. J., Radu, E., Zelenak, C., and Popa-Wagner, A. (2014). Transcriptomics of post-stroke angiogenesis in the aged brain. Front. Aging Neurosci. 6, 44–20. doi:10.3389/fnagi.2014.00044
Califano, J. P., and Reinhart-King, C. A. (2008). A balance of substrate mechanics and matrix chemistry regulates endothelial cell network assembly. Cell Mol. Bioeng. 1, 122–132. doi:10.1007/s12195-008-0022-x
Chang, H. Y., Chi, J.-T., Dudoit, S., Bondre, C., Van De Rijn, M., Botstein, D., et al. (2002). Diversity, topographic differentiation, and positional memory in human fibroblasts. Proc. Natl. Acad. Sci. U. S. A. 99, 12877–12882. doi:10.1073/pnas.162488599
Chen, D., Yoo, B. K., Santhekadur, P. K., Gredler, R., Bhutia, S. K., Das, S. K., et al. (2011). Insulin-like growth factor-binding protein-7 functions as a potential tumor suppressor in hepatocellular carcinoma. Clin. Cancer Res. 17, 6693–6701. doi:10.1158/1078-0432.CCR-10-2774
Chen, L. H., Liu, D. W., Chang, J. L., Chen, P. R., Hsu, L. P., Lin, H. Y., et al. (2015). Methylation status of insulin-like growth factor-binding protein 7 concurs with the malignance of oral tongue cancer. J. Exp. Clin. Cancer Res. 34, 20–12. doi:10.1186/s13046-015-0138-5
Chen, R.-Y., Chen, H.-X., Jian, P., Xu, L., Li, J., Fan, Y.-M., et al. (2010b). Intratumoral injection of pEGFC1-IGFBP7 inhibits malignant melanoma growth in C57BL/6J mice by inducing apoptosis and down-regulating VEGF expression. Oncol. Rep. 23, 981–988. doi:10.3892/or_00000723
Chen, R. Y., Chen, H. X., Lin, J. X., She, W. B., Jiang, P., Xu, L., et al. (2010a). In-vivo transfection of pcDNA3.1-IGFBP7 inhibits melanoma growth in mice through apoptosis induction and VEGF downexpression. J. Exp. Clin. Cancer Res. 29, 13–18. doi:10.1186/1756-9966-29-13
Chen, Z., Li, L., Wu, W., Liu, Z., Huang, Y., Yang, L., et al. (2020). Exercise protects proliferative muscle satellite cells against exhaustion via the Igfbp7-Akt-mTOR axis. Theranostics 10, 6448–6466. doi:10.7150/thno.43577
Chiang, C. E., Fang, Y. Q., Ho, C. T., Assunção, M., Lin, S. J., Wang, Y. C., et al. (2021). Bioactive decellularized extracellular matrix derived from 3D stem cell spheroids under macromolecular crowding serves as a scaffold for tissue engineering. Adv. Healthc. Mater 10, 21000244–e2100113. doi:10.1002/adhm.202100024
de Oliveira, C. E., Rocha Dourado, M., Sawazaki-Calone, Í., de Medeiros, M. C., Rossa Júnior, C., de Karla Cervigne, N., et al. (2020). Activin A triggers angiogenesis via regulation of VEGFA and its overexpression is associated with poor prognosis of oral squamous cell carcinoma. Int. J. Oncol. 57, 364–376. doi:10.3892/ijo.2020.5058
Driscoll, T. P., Bidone, T. C., Ahn, S. J., Yu, A., Groisman, A., Voth, G. A., et al. (2021). Integrin-based mechanosensing through conformational deformation. Biophys. J. 120, 4349–4359. doi:10.1016/j.bpj.2021.09.010
Dvorak, H. F., Brown, L. F., Detmar, M., and Dvorak, A. M. (1995). Vascular permeability factor/vascular endothelial growth factor, microvascular hyperpermeability, and angiogenesis. Am. J. ofPathology 146, 1029–1039. doi:10.1007/978-3-642-59953-8_6
Esmeijer, K., Schoe, A., Ruhaak, L. R., Hoogeveen, E. K., Soonawala, D., Romijn, F. P. H. T. M., et al. (2021). The predictive value of TIMP-2 and IGFBP7 for kidney failure and 30-day mortality after elective cardiac surgery. Sci. Rep. 11, 1071–1078. doi:10.1038/s41598-020-80196-2
Evdokimova, V., Tognon, C. E., Benatar, T., Yang, W., Krutikov, K., Pollak, M., et al. (2012). IGFBP7 binds to the IGF-1 receptor and blocks its activation by insulin-like growth factors. Sci. Signal 5, ra92. doi:10.1126/scisignal.2003184
Guo, Y., Mei, F., Huang, Y., Ma, S., Wei, Y., Zhang, X., et al. (2022). Matrix stiffness modulates tip cell formation through the p-PXN-Rac1-YAP signaling axis. Bioact. Mater 7, 364–376. doi:10.1016/j.bioactmat.2021.05.033
Hong, Z., Xie, W., Zhuo, H., Wei, X., Wang, K., Cheng, J., et al. (2023). Crosstalk between cancer cells and cancer-associated fibroblasts mediated by TGF-β1–IGFBP7 signaling promotes the progression of infiltrative gastric cancer. Cancers (Basel) 15, 3965. doi:10.3390/cancers15153965
Hooper, A. T., Shmelkov, S. V., Gupta, S., Milde, T., Bambino, K., Gillen, K., et al. (2009). Angiomodulin is a specific marker of vasculature and regulates vascular endothelial growth factor-A-dependent neoangiogenesis. Circ. Res. 105, 201–208. doi:10.1161/CIRCRESAHA.109.196790
Hu, Z., Wu, J., Qin, L., Jin, H., Cao, Y., and Zhao, Y. (2021). IGFBP7 downregulation or overexpression effect on bovine preadipocyte differentiation. Anim. Biotechnol. 32, 21–30. doi:10.1080/10495398.2019.1642906
Jiang, W., Xiang, C., Cazacu, S., Brodie, C., and Mikkelsen, T. (2008). Insulin-like growth factor binding protein 7 mediates glioma cell growth and migration. Neoplasia 10, 1335–1342. doi:10.1593/neo.08694
Jiang, Y., Wang, D., Blocki, A., and Tuan, R. S. (2020). Mesenchymal stem cells in musculoskeletal tissue engineering. Cambridge, MA: INC. doi:10.1016/B978-0-12-818422-6.00051-4
Jin, L., Shen, F., Weinfeld, M., and Sergi, C. (2020). Insulin growth factor binding protein 7 (IGFBP7)-Related cancer and IGFBP3 and IGFBP7 crosstalk. Front. Oncol. 10, 727–814. doi:10.3389/fonc.2020.00727
Kaneda, H., Arao, T., Matsumoto, K., De Velasco, M. A., Tamura, D., Aomatsu, K., et al. (2011). Activin A inhibits vascular endothelial cell growth and suppresses tumour angiogenesis in gastric cancer. Br. J. Cancer 105, 1210–1217. doi:10.1038/bjc.2011.348
Kato, M. V. (2000). A secreted tumor-suppressor, mac25, with activin-binding activity. Mol. Med. 6, 126–135. doi:10.1007/s0089400060126
Ko, T., Nomura, S., Yamada, S., Fujita, K., Fujita, T., Satoh, M., et al. (2022). Cardiac fibroblasts regulate the development of heart failure via Htra3-TGF-β-IGFBP7 axis. Nat. Commun. 13, 3275–3317. doi:10.1038/s41467-022-30630-y
Komiya, E., Furuya, M., Watanabe, N., Miyagi, Y., Higashi, S., and Miyazaki, K. (2012). Elevated expression of angiomodulin (AGM/IGFBP-rP1) in tumor stroma and its roles in fibroblast activation. Cancer Sci. 103, 691–699. doi:10.1111/j.1349-7006.2012.02203.x
Komiya, E., Sato, H., Watanabe, N., Ise, M., Higashi, S., Miyagi, Y., et al. (2014). Angiomodulin, a marker of cancer vasculature, is upregulated by vascular endothelial growth factor and increases vascular permeability as a ligand of integrin αvβ3. Cancer Med. 3, 537–549. doi:10.1002/cam4.216
Lam, J., Lee, B., Yu, J., Kwee, B. J., Kim, Y., Kim, J., et al. (2022). A microphysiological system-based potency bioassay for the functional quality assessment of mesenchymal stromal cells targeting vasculogenesis. Biomaterials 290, 121826. doi:10.1016/j.biomaterials.2022.121826
Lee, O. H., Bae, S. K., Bae, M. H., Lee, Y. M., Moon, E. J., Cha, H. J., et al. (2000). Identification of angiogenic properties of insulin like growth factor II in in vitro angiogenesis models. Br. J. Cancer 82, 385–391. doi:10.1054/bjoc.1999.0931
Leung, D. W., Cachianes, G., Kuang, W. J., Goeddel, D. V., and Ferrara, N. (1989). Vascular endothelial growth factor is a secreted angiogenic mitogen. Science 246, 1306–1309. doi:10.1126/science.2479986
Li, D., Xia, L., Huang, P., Wang, Z., Guo, Q., Huang, C., et al. (2023a). Cancer-associated fibroblast-secreted IGFBP7 promotes gastric cancer by enhancing tumor associated macrophage infiltration via FGF2/FGFR1/PI3K/AKT axis. Cell Death Discov. 9, 17. doi:10.1038/s41420-023-01336-x
Li, X., Feng, L., Zhang, C., Wang, J., Wang, S., and Hu, L. (2022). Insulin-like growth factor binding proteins 7 prevents dental pulp-derived mesenchymal stem cell senescence via metabolic downregulation of p21. Sci. China Life Sci. 65, 2218–2232. doi:10.1007/s11427-021-2096-0
Li, Y., Fu, L., Wu, B., Guo, X., Shi, Y., Lv, C., et al. (2023b). Angiogenesis modulated by CD93 and its natural ligands IGFBP7 and MMRN2: a new target to facilitate solid tumor therapy by vasculature normalization. Cancer Cell Int. 23, 189–214. doi:10.1186/s12935-023-03044-z
Li, Y., Xi, Y., Zhu, G., Jia, J., Huang, H., Liu, Y., et al. (2019). Downregulated IGFBP7 facilitates liver metastasis by modulating epithelial-mesenchymal transition in colon cancer. Oncol. Rep. 42, 1935–1945. doi:10.3892/or.2019.7303
Liao, Y., Lei, J., Liu, M., Lin, W., Hong, D., Tuo, Y., et al. (2016). Mesenchymal stromal cells mitigate experimental colitis via insulin-like growth factor binding protein 7-mediated immunosuppression. Mol. Ther. 24, 1860–1872. doi:10.1038/mt.2016.140
Liu, F., Yu, T., Liu, J., Yang, Q., Wu, J., Ren, J., et al. (2023a). IGFBP-7 secreted by adipose-derived stem cells inhibits keloid formation via the BRAF/MEK/ERK signaling pathway. J. Dermatol Sci. 111, 10–19. doi:10.1016/j.jdermsci.2023.05.004
Liu, Y., Shen, S., Yan, Z., Yan, L., Ding, H., Wang, A., et al. (2023b). Expression characteristics and their functional role of IGFBP gene family in pan-cancer. BMC Cancer 23, 371. doi:10.1186/s12885-023-10832-3
Lu, Z. F., Chiu, J., Lee, L. R., Schindeler, A., Jackson, M., Ramaswamy, Y., et al. (2020). Reprogramming of human fibroblasts into osteoblasts by insulin-like growth factor-binding protein 7. Stem Cells Transl. Med. 9, 403–415. doi:10.1002/sctm.19-0281
Moita, M. R., Silva, M. M., Diniz, C., Serra, M., Hoet, R. M., Barbas, A., et al. (2022). Transcriptome and proteome profiling of activated cardiac fibroblasts supports target prioritization in cardiac fibrosis. Front. Cardiovasc Med. 9, 1015473. doi:10.3389/fcvm.2022.1015473
Morgantini, C., Jager, J., Li, X., Levi, L., Azzimato, V., Sulen, A., et al. (2019). Liver macrophages regulate systemic metabolism through non-inflammatory factors. Nat. Metab. 1, 445–459. doi:10.1038/s42255-019-0044-9
Murphy, M., Pykett, M. J., Harnish, P., Zang, K. D., and George, D. L. (1993). Identification and characterization of genes differentially expressed in meningiomas. Cell Growth Differ. 4, 715–722.
Nagakubo, D., Murai, T., Tanaka, T., Usui, T., Matsumoto, M., Sekiguchi, K., et al. (2003). A high endothelial venule secretory protein, mac25/angiomodulin, interacts with multiple high endothelial venule-associated molecules including chemokines. J. Immunol. 171, 553–561. doi:10.4049/jimmunol.171.2.553
Nakao-Hayashi, J., Ito, H., Kanayasu, T., Morita, I., and Murota, S. I. (1992). Stimulatory effects of insulin and insulin-like growth factor I on migration and tube formation by vascular endothelial cells. Atherosclerosis 92, 141–149. doi:10.1016/0021-9150(92)90273-J
Newman, A. C., Nakatsu, M. N., Chou, W., Gershon, P. D., and Hughes, C. C. W. (2011). The requirement for fibroblasts in angiogenesis: fibroblast-derived matrix proteins are essential for endothelial cell lumen formation. Mol. Biol. Cell 22, 3791–3800. doi:10.1091/mbc.E11-05-0393
Oh, Y., Nagalla, S. R., Yamanaka, Y., Kim, H.-S., Wilson, E., and Rosenfeld, R. G. (1996). Synthesis and characterization of insulin-like growth factor-binding protein (IGFBP)-7. Recombinant human mac25 protein specifically binds IGF-I and -II. J. Biol. Chem. 271, 30322–30325. doi:10.1074/jbc.271.48.30322
Pen, A., Durocher, Y., Slinn, J., Rukhlova, M., Charlebois, C., Stanimirovic, D. B., et al. (2011). Insulin-like growth factor binding protein 7 exhibits tumor suppressive and vessel stabilization properties in U87MG and T98G glioblastoma cell lines. Cancer Biol. Ther. 12, 634–646. doi:10.4161/cbt.12.7.17171
Pen, A., Moreno, M. J., Durocher, Y., Deb-Rinker, P., and Stanimirovic, D. B. (2008). Glioblastoma-secreted factors induce IGFBP7 and angiogenesis by modulating Smad-2-dependent TGF-beta signaling. Oncogene 27, 6834–6844. doi:10.1038/onc.2008.287
Pen, A., Moreno, M. J., Martin, J., and Stanimirovic, D. B. (2007). Molecular markers of extracellular matrix remodeling in glioblastoma vessels: microarray study of laser-captured glioblastoma vessels. Glia 55, 559–572. doi:10.1002/glia.20481
Rupp, C., Scherzer, M., Rudisch, A., Unger, C., Haslinger, C., Schweifer, N., et al. (2015). IGFBP7, a novel tumor stroma marker, with growth-promoting effects in colon cancer through a paracrine tumor-stroma interaction. Oncogene 34, 815–825. doi:10.1038/onc.2014.18
Sato, J., Hasegawa, S., Akaogi, K., Yasumitsu, H., Yamada, S., Sugahara, K., et al. (1999). Identification of cell-binding site of angiomodulin (AGM/TAF/Mac25) that interacts with heparan sulfates on cell surface. J. Cell Biochem. 75, 187–195. doi:10.1002/(sici)1097-4644(19991101)75:2<187::aid-jcb1>3.3.co;2-i
Sato, Y., Chen, Z., and Miyazaki, K. (2007). Strong suppression of tumor growth by insulin-like growth factor-binding protein-related protein 1/tumor-derived cell adhesion factor/mac25. Cancer Sci. 98, 1055–1063. doi:10.1111/j.1349-7006.2007.00502.x
Saunders, R. L., and Hammer, D. A. (2010). Assembly of human umbilical vein endothelial cells on compliant hydrogels. Cell Mol. Bioeng. 3, 60–67. doi:10.1007/s12195-010-0112-4
Severino, V., Alessio, N., Farina, A., Sandomenico, A., Cipollaro, M., Peluso, G., et al. (2013). Insulin-like growth factor binding proteins 4 and 7 released by senescent cells promote premature senescence in mesenchymal stem cells. Cell Death Dis. 4, e911–11. doi:10.1038/cddis.2013.445
Shaked, Y., Bertolini, F., Man, S., Rogers, M. S., Cervi, D., Foutz, T., et al. (2005). Genetic heterogeneity of the vasculogenic phenotype parallels angiogenesis: implications for cellular surrogate marker analysis of antiangiogenesis. Cancer Cell 7, 101–111. doi:10.1016/j.ccr.2004.11.023
Siemann, D. W. (2011). The unique characteristics of tumor vasculature and preclinical evidence for its selective disruption by Tumor-Vascular Disrupting Agents. Cancer Treat. Rev. 37, 63–74. doi:10.1016/j.ctrv.2010.05.001
Smirnov, D. A., Foulk, B. W., Doyle, G. V., Connelly, M. C., Terstappen, L. W. M. M., and O’Hara, S. M. (2006). Global gene expression profiling of circulating endothelial cells in patients with metastatic carcinomas. Cancer Res. 66, 2918–2922. doi:10.1158/0008-5472.CAN-05-4003
Spater, T., Assunçao, M., Lit, K. K., Gong, G., Wang, X., Chen, Y.-Y., et al. (2022). Engineering microparticles based on solidified stem cell secretome with an augmented pro-angiogenic factor portfolio for therapeutic angiogenesis. Bioact. Mater 17, 526–541. doi:10.1016/j.bioactmat.2022.03.015
Sun, S., Cao, C., Guo, Y., Hou, Y., Meng, Y., Li, Y., et al. (2022). Osteogenic differentiated human bone marrow stem cells contribute to sprouting angiogenesis deceleration via paracrine excreted IGFBP7. Adv. Mater Interfaces 9. doi:10.1002/admi.202201719
Sun, Y., Chen, W., Torphy, R. J., Yao, S., Zhu, G., Lin, R., et al. (2021). Blockade of the CD93 pathway normalizes tumor vasculature to facilitate drug delivery and immunotherapy. Sci. Transl. Med. 13, 8922. doi:10.1126/scitranslmed.abc8922
Tamura, K., Hashimoto, K., Suzuki, K., Yoshie, M., Kutsukake, M., and Sakurai, T. (2009). Insulin-like growth factor binding protein-7 (IGFBP7) blocks vascular endothelial cell growth factor (VEGF)-induced angiogenesis in human vascular endothelial cells. Eur. J. Pharmacol. 610, 61–67. doi:10.1016/j.ejphar.2009.01.045
Tamura, K., Matsushita, M., Endo, A., Kutsukake, M., and Kogo, H. (2007). Effect of insulin-like growth factor-binding protein 7 on steroidogenesis in granulosa cells derived from equine chorionic gonadotropin-primed immature rat ovaries. Biol. Reprod. 77, 485–491. doi:10.1095/biolreprod.106.058867
Tamura, K., Yoshie, M., Hashimoto, K., and Tachikawa, E. (2014). Inhibitory effect of insulin-like growth factor-binding protein-7 (IGFBP7) on in vitro angiogenesis of vascular endothelial cells in the rat corpus luteum. J. Reproduction Dev. 60, 447–453. doi:10.1262/jrd.2014-069
Titeca-Beauport, D., Daubin, D., Chelly, J., Zerbib, Y., Brault, C., Diouf, M., et al. (2019). The urine biomarkers TIMP2 and IGFBP7 can identify patients who will experience severe acute kidney injury following a cardiac arrest: a prospective multicentre study. Resuscitation 141, 104–110. doi:10.1016/j.resuscitation.2019.06.008
Tomimaru, Y., Eguchi, H., Wada, H., Kobayashi, S., Marubashi, S., Tanemura, M., et al. (2012). IGFBP7 downregulation is associated with tumor progression and clinical outcome in hepatocellular carcinoma. Int. J. Cancer 130, 319–327. doi:10.1002/ijc.25994
Tomita, T., Kato, M., and Hiratsuka, S. (2021). Regulation of vascular permeability in cancer metastasis. Cancer Sci. 112, 2966–2974. doi:10.1111/cas.14942
Usui, T., Murai, T., Tanaka, T., Yamaguchi, K., Nagakubo, D., Lee, C. M., et al. (2002). Characterization of mac25/angiomodulin expression by high endothelial venule cells in lymphoid tissues and its identification as an inducible marker for activated endothelial cells. Int. Immunol. 14, 1273–1282. doi:10.1093/intimm/dxf102
Van Beijnum, J. R., Dings, R. P., Van Der Linden, E., Zwaans, B. M. M., Ramaekers, F. C. S., Mayo, K. H., et al. (2006). Gene expression of tumor angiogenesis dissected: specific targeting of colon cancer angiogenic vasculature. Blood 108, 2339–2348. doi:10.1182/blood-2006-02-004291
Van Breevoort, D., Van Agtmaal, E. L., Dragt, B. S., Gebbinck, J. K., Dienava-Verdoold, I., Kragt, A., et al. (2012). Proteomic screen identifies IGFBP7 as a novel component of endothelial cell-specific weibel-palade bodies. J. Proteome Res. 11, 2925–2936. doi:10.1021/pr300010r
Vandoorne, K., Addadi, Y., and Neeman, M. (2010). Visualizing vascular permeability and lymphatic drainage using labeled serum albumin. Angiogenesis 13, 75–85. doi:10.1007/s10456-010-9170-4
Velázquez-Enríquez, J. M., Santos-álvarez, J. C., Ramírez-Hernández, A. A., Reyes-Jiménez, E., López-Martínez, A., Pina-Canseco, S., et al. (2021). Proteomic analysis reveals key proteins in extracellular vesicles cargo associated with idiopathic pulmonary fibrosis in vitro. Biomedicines 9, 1058. doi:10.3390/biomedicines9081058
Wan, H. Y., Chen, J. C. H., Xiao, Q., Wong, C. W., Yang, B., Cao, B., et al. (2023). Stabilization and improved functionality of three-dimensional perfusable microvascular networks in microfluidic devices under macromolecular crowding. Biomater. Res. 27, 32–16. doi:10.1186/s40824-023-00375-w
Wan, H. Y., Shin, R. L. Y., Chen, J. C. H., Assunção, M., Wang, D., Nilsson, S. K., et al. (2022). Dextran sulfate-amplified extracellular matrix deposition promotes osteogenic differentiation of mesenchymal stem cells. Acta Biomater. 140, 163–177. doi:10.1016/j.actbio.2021.11.049
Wan, Z., Zhang, S., Zhong, A. X., Shelton, S. E., Campisi, M., Sundararaman, S. K., et al. (2021). A robust vasculogenic microfluidic model using human immortalized endothelial cells and Thy1 positive fibroblasts. Biomaterials 276, 121032–121125. doi:10.1016/j.biomaterials.2021.121032
Wang, F. T., Sun, W. E. I., Zhang, J. T., and Fan, Y. Z. (2019). Cancer-associated fibroblast regulation of tumor neo-angiogenesis as a therapeutic target in cancer. Oncol. Lett. 17, 3055–3065. doi:10.3892/ol.2019.9973
Wang, J., Deng, X., Xie, Y., Tang, J., Zhou, Z., Yang, F., et al. (2021). An integrated transcriptome analysis reveals IGFBP7 upregulation in vasculature in traumatic brain injury. Front. Genet. 11, 599834. doi:10.3389/fgene.2020.599834
Wang, Y., Bo, J., Zhao, Z., Han, Y., Zhang, Q., and Liu, L. (2023). Depletion of Igfbp7 alleviates zebrafish NAFLD progression through inhibiting hepatic ferroptosis. Life Sci. 332, 122086. doi:10.1016/j.lfs.2023.122086
Winiarski, B. K., Wolanska, K. I., Rai, S., Ahmed, T., Acheson, N., Gutowski, N. J., et al. (2013). Epithelial ovarian cancer-induced angiogenic phenotype of human omental microvascular endothelial cells may occur independently of VEGF signaling. Transl. Oncol. 6, 703–714. doi:10.1593/tlo.13529
Wolchinsky, Z., Shivtiel, S., Kouwenhoven, E. N., Putin, D., Sprecher, E., Zhou, H., et al. (2014). Angiomodulin is required for cardiogenesis of embryonic stem cells and is maintained by a feedback loop network of p63 and Activin-A. Stem Cell Res. 12, 49–59. doi:10.1016/j.scr.2013.09.015
Xia, H., Tian, Y., Lin, Y., Huang, Q., and Xue, Y. (2022). Evaluating osteogenic differentiation of osteoblastic precursors upon intermittent administration of PTH/IGFBP7. Front. Pharmacol. 13, 839035. doi:10.3389/fphar.2022.839035
Xiuqing, L. I., Zhang, J., Youshan, W. U., Chuntao, M. A., Dongying, W. E. I., Lijuan, P. A. N., et al. (2022). IGFBP7 remodels the tumor microenvironment of esophageal squamous cell carcinoma by activating the TGFβ1/SMAD signaling pathway. Oncol. Lett. 24, 1–7. doi:10.3892/ol.2022.13371
Xu, H. W., Wang, M. Q., and Zhu, S. L. (2023). Analysis of IGFBP7 expression characteristics in pan-cancer and its clinical relevance to stomach adenocarcinoma. Transl. Cancer Res. 12, 2596–2612. doi:10.21037/tcr-23-1055
Yamanaka, Y., Wilson, E. M., Rosenfeld, R. G., and Oh, Y. (1997). Inhibition of insulin receptor activation by insulin-like growth factor binding proteins. J. Biol. Chem. 272, 30729–30734. doi:10.1074/jbc.272.49.30729
Yan, H., Li, T., Wang, Y., Li, H., Xu, J., and Lu, X. (2019). Insulin-like growth factor binding protein 7 accelerates hepatic steatosis and insulin resistance in non-alcoholic fatty liver disease. Clin. Exp. Pharmacol. Physiol. 46, 1101–1110. doi:10.1111/1440-1681.13159
Yu, J. T., Hu, X. W., Yang, Q., Shan, R. R., Zhang, Y., Dong, Z. H., et al. (2022). Insulin-like growth factor binding protein 7 promotes acute kidney injury by alleviating poly ADP ribose polymerase 1 degradation. Kidney Int. 102, 828–844. doi:10.1016/j.kint.2022.05.026
Zhang, L., Lian, R., Zhao, J., Feng, X., Ye, R., Pan, L., et al. (2019). IGFBP7 inhibits cell proliferation by suppressing AKT activity and cell cycle progression in thyroid carcinoma. Cell Biosci. 9, 44. doi:10.1186/s13578-019-0310-2
Zhang, L., Smyth, D., Al-Khalaf, M., Blet, A., Du, Q., Bernick, J., et al. (2022). Insulin-like growth factor-binding protein-7 (IGFBP7) links senescence to heart failure. Nat. Cardiovasc. Res. 1, 1195–1214. doi:10.1038/s44161-022-00181-y
Zhang, W., Chen, E., Chen, M., Ye, C., Qi, Y., Ding, Q., et al. (2018). IGFBP7 regulates the osteogenic differentiation of bone marrow-derived mesenchymal stem cells via Wnt/β-catenin signaling pathway. FASEB J. 32, 2280–2291. doi:10.1096/fj.201700998RR
Zhu, S., Wang, H., Zhang, Z., Ma, M., Zheng, Z., Xu, X., et al. (2020). IGFBP-rP1-silencing promotes hypoxia-induced angiogenic potential of choroidal endothelial cells via the RAF/MEK/ERK signaling pathway. Mol. Med. Rep. 22, 4837–4847. doi:10.3892/mmr.2020.11578
Glossary
Keywords: insulin-like growth factor-binding protein 7 (IGFBP7), luminogenesis, microenvironment, tissue remodeling, angiogenesis, vascularization
Citation: Lit KK, Zhirenova Z and Blocki A (2024) Insulin-like growth factor-binding protein 7 (IGFBP7): A microenvironment-dependent regulator of angiogenesis and vascular remodeling. Front. Cell Dev. Biol. 12:1421438. doi: 10.3389/fcell.2024.1421438
Received: 22 April 2024; Accepted: 10 June 2024;
Published: 09 July 2024.
Edited by:
Lei Hu, Capital Medical University, ChinaReviewed by:
Yuyao Tian, Massachusetts General Hospital, Harvard Medical School, United StatesUgo Cavallaro, European Institute of Oncology, Italy
Md Shaifur Rahman, Atomic Energy Research Establishment, Bangladesh
Copyright © 2024 Lit, Zhirenova and Blocki. This is an open-access article distributed under the terms of the Creative Commons Attribution License (CC BY). The use, distribution or reproduction in other forums is permitted, provided the original author(s) and the copyright owner(s) are credited and that the original publication in this journal is cited, in accordance with accepted academic practice. No use, distribution or reproduction is permitted which does not comply with these terms.
*Correspondence: Anna Blocki, anna.blocki@cuhk.edu.hk