Corrigendum: Mechanism of LncRNA Gm2044 in germ cell development
- 1School of Life Science, Bengbu Medical University, Bengbu, China
- 2First Affiliated Hospital, Bengbu Medical University, Bengbu, China
Germ cell development in mammals is a complex physiological process that involves the proliferation of primordial germ cells, meiosis, and the formation of male and female gametes. Long non-coding RNA (lncRNA) is a type of RNA with more than 200 nucleotides that does not code for proteins. A small number of lncRNAs have been shown to participate in spermatogenesis in the testes and in follicular development in the ovaries, but the role of the vast majority of lncRNAs and their molecular mechanisms still need further study. LncRNA Gm2044 was identified as a differentially expressed lncRNA in mouse spermatogenesis by microarray technology. In mouse testis, lncRNA Gm2044 can act as competing endogenous RNA to regulate SYCP1 expression in GC-2 cells derived from mouse spermatocyte cells, and it can also act as a host gene for miR-202 to regulate RBFOX2 protein expression. In female mouse ovaries, lncRNA Gm2044 regulates 17β-estradiol synthesis through the miRNA-138-5p-Nr5a1 pathway or by interacting with EEF2. In addition, studies suggest that lncRNA Gm2044 is also involved in the progression of reproductive system diseases such as male nonobstructive azoospermia. Here, we summarize the roles and molecular mechanisms of lncRNA Gm2044 in male and female gametogenesis and its potential role in some infertility disorders.
1 Introduction
The development of mammalian germ cells is a complex and delicate physiological process, which mainly includes spermatogenesis in the male testis and oocyte generation in the female ovary. Mammalian spermatogenesis needs to go through three stages: Self-renewal and mitosis of spermatogonial stem cells (SSC), meiosis of spermatocytes, and differentiation of haploid spermatids, and eventually the production of mature sperm (Kubota and Brinster, 2018). The development of oocytes begins in the fetal ovary and matures in adulthood through meiosis. Such a long period of meiotic arrest makes it easy for the oocytes to be affected by the surrounding microenvironment. The surrounding somatic cells of oocytes can regulate the growth and development of oocytes by regulating hormones, growth factors, and metabolites (Petro et al., 2012). However, the role of the ovary is not only to produce functional oocytes, but also to synthesize and secrete some of the steroid hormones necessary for fertilization and germ cell development, such as estrogen, progesterone, and androgen (Palermo, 2007; Alexander et al., 2023). These steroid hormones are all produced by cholesterol in mitochondria through complex biosynthetic pathways (Miller, 2013; Esmaeilian et al., 2023). Estradiol is an endogenous hormone secreted by mature ovarian follicles (Yang et al., 2023), and changes in its level not only play a key role in the regulation of female reproductive development, but also in the development of mammalian bones, brain function and skin health (Baddela et al., 2023).
It is well known that lncRNA is a class of non-coding RNA molecule with a length of more than 200 nucleotides. In the early years, there was a lack of research and understanding of lncRNA. Three-quarters of the human genome can be transcribed into RNA, but about 99% of these mRNAs cannot encode proteins (Wu et al., 2020). As a result, many people believe that lncRNAs are just “junk” or “transcriptional noise” generated by gene transcription, and the importance of lncRNA has not been apparent until recent years (Palazzo and Lee, 2015). Research has shown that lncRNA can be expressed through differences involved in the pathogenesis of diseases such as inflammation, metabolic diseases, and cancer. For example, in inflammation, lncRNA TNF can participate in the progression of non-alcoholic steatohepatitis (NASH) (Atanasovska et al., 2021), and lncRNA Helf promotes hepatic inflammation and fibrosis (Han et al., 2023). In metabolic diseases, lncRNA TUG1 can improve diabetic nephropathy (Meng et al., 2022), and LncRNA H19 is involved in osteoblast differentiation (Liang et al., 2016). There are also many lncRNAs involved in cancer, and lncRNA HOTTP is the most classic. LncRNA HOTTIP can play an important role in the pathogenesis of various cancers. Moreover, the knockdown of HOTTIP can inhibit the proliferation of gastric cancer cells (Xiao et al., 2020), and patients with high HOTTIP expression have higher metastasis potential of rectal cancer cells (Rui et al., 2019). HOTTIP is also highly expressed in ovarian cancer cells and can promote the development of ovarian cancer and metastasis of ovarian cancer cells by regulating the transcription factor HIF-1α or pyroptosis (Tan et al., 2021; Zhang et al., 2022). Increased lncRNA HOTTIP can regulate γ-H2AX and p53 signaling in UV-induced spermatogonia G2/M arrest and early apoptosis (Liang and Hu, 2019). In addition, our previous study revealed that hundreds of significantly differentially expressed lncRNAs were identified in testicular heat exposure mouse by high-throughput sequencing, and these lncRNAs may act synergistically with associated differentially expressed cirRNAs, miRNAs, and mRNAs (Hu et al., 2021). More and more studies have confirmed that lncRNA can participate in various biological processes.
In recent years, the effects of lncRNA on reproductive cells has been reported (He et al., 2021). Premature ovarian insufficiency (POI) is a disease of the female reproductive system. LncRNA HCP5 is involved in the progression of POI by regulating the expression of two genes, MSH5 and YB1 (Wang et al., 2020). The high expression of lncRNA PCAT6 can inhibit the expression level of PTEN, thereby promoting the development of ovarian cancer (Kong et al., 2019). Overexpression and knockdown of lncRNA5251 can decrease and improve sperm quality in mice, respectively, and lncRNA5251 can regulate spermatogenesis through interaction with cell link-related genes (Zhang et al., 2023). An increasing number of non-protein-coding RNAs have been confirmed to be involved in the regulation of mammalian germ cell genesis. Among these, lncRNA Gm2044 is specifically expressed in the ovaries and testis of mice and has regulatory effects on the processes of estrogen synthesis and spermatogenesis through related signaling pathways. This paper describes the important roles and molecular mechanisms of lncRNA Gm2044 in the testis and ovaries.
2 LncRNA Gm2044
2.1 Classification of lncRNA
The complex molecular mechanism of lncRNA production has been widely reported. The most common classification divides lncRNAs into three categories according to their position in the genome: 1) Intronic lncRNAs, which are transcribed from the intron region of coding genes, e.g., Mrhl lncRNA, which is transcribed from the 15th intron of mouse Phkb gene on chromosome 18, was found to regulate the meiosis of B-type spermatogonia in mouse testis by regulating related signaling pathways or mediating Sox8 (Kataruka et al., 2017; Kayyar et al., 2023). 2) Sense and antisense lncRNAs, which are transcribed from either the sense or antisense strand of the coding gene, e.g., lncRNA NNT-AS1 is an antisense lncRNA that can promote the progression of esophageal squamous cell carcinoma by acting as a sponge for miR-382-5p (Pan et al., 2022). 3) Intergenic lncRNA (lincRNA) is transcribed in the middle region of two coding genes (Ma et al., 2013) e.g., the recently discovered two lincRNAs, which have a significant function in mouse macrophage inflammatory regulation (lincRNA-Cox2) (Salih et al., 2023) and play an important role in cancer cells (lincRNA p21) (Huang et al., 2022).
2.2 Discovery of lncRNA Gm2044
We know that sperm originate from primordial germ cells (PGCs), and PGC is produced by embryonic cells that have been partially converted into somatic cells (Ohinata et al., 2009; Stukenborg et al., 2014). PGC produces SSCs through a complex process (Phillips et al., 2010). SSC can both self-renew and differentiate into spermatogonia (SPG), which can be divided into type A spermatogonia (A), Intermediate (In), and type B spermatogonia (B) (Subash and Kumar, 2021). Type B spermatogonia undergo mitosis for proliferation or meiosis to transform into primary spermatocytes, and the primary spermatocytes undergo meiosis to differentiate into the secondary spermatocytes, resulting in a haploid round sperm (RS) that becomes a mature elongated sperm (Stukenborg et al., 2014; Cannarella et al., 2020). In addition, Sertoli cells are required to provide nutrition and support, and Leydig cells secrete androgens to regulate spermatogenesis precisely (Zhou et al., 2019). But the function of lncRNA in mammalian reproductive cells remains largely unexplored. So some groups have analyzed the expression profiling of lncRNAs in germ cells at different stages of the testis by microarray (Bao et al., 2013; Sun and Wu, 2015). Liang et al. analyzed the expression of lncRNA and mRNAs in four specific types of germ cells in the testis by microarray analysis (Liang et al., 2014). The four types of germ cells were spermatogonial stem cells, type A spermatogonia, pachytene spermatocytes (PS), and round spermatocytes. The expression of lncRNA was the highest in type A spermatogonia and the lowest in pachytene spermatocytes, while the expression trend of mRNA was similar to that of lncRNA, indicating that lncRNA and mRNA may play a synergistic role in spermatogenesis (Liang et al., 2014). Further analysis identified four lncRNAs that were differentially expressed in male germ cells. The UCSC genome Browser database (https://genome.ucsc.edu/) showed that lncRNA Gm2044 was 912 nt in length, had two exons, and was located on chromosome 7qF4 (Figure 1). In addition, four ORFs were predicted in ORF Finder (https://www.ncbi.nlm.nih.gov/orffinder/): ORF4 could not translate proteins on the lncRNA antisense chain, and the other three ORFs were verified by experiments and could not translate proteins, so lncRNA Gm2044 was proved to be non-coding RNA (Hu et al., 2022).
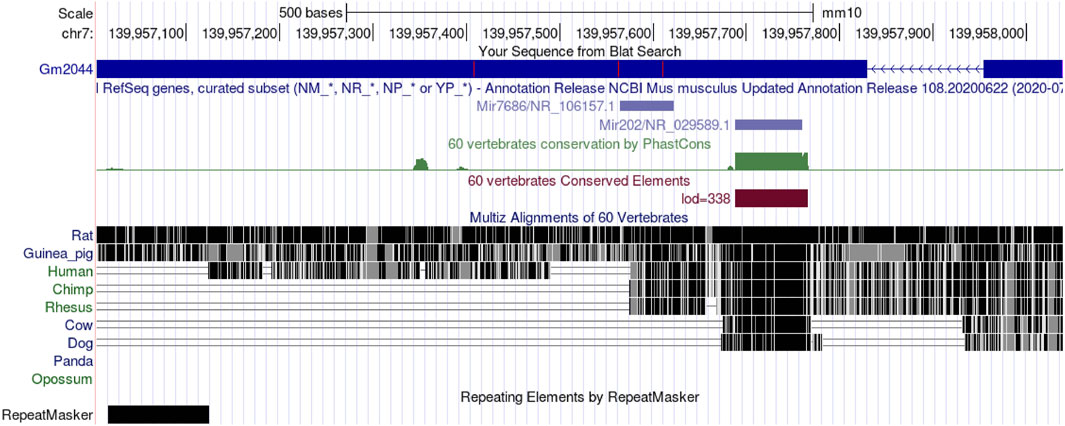
Figure 1. LncRNA Gm2044 is located on mouse chromosome seven and is the host gene of miR-202. Moreover, lncRNA Gm2044 is highly conserved in rodents.
3 Regulatory mechanism of lncRNA Gm2044 in male reproduction
3.1 LncRNA Gm2044 interacts with mRNA
To understand the role of lncRNA Gm2044 in germ cells, the potential effect of lncRNA Gm2044 on spermatogenesis in the testis was first investigated. In our previous study, we found that lncRNA Gm2044 was highly expressed in pachytene spermatocytes and meiosis of the mouse testis by microarray analysis (Liang et al., 2014). And its expression level was also downregulated when the number of spermatocytes was relatively low due to the differentiation of spermatocytes into spermatides (Hu et al., 2018). This shows that lncRNA Gm2044 is important in the meiosis of spermatogenesis. UTF1, or undifferentiated embryonic cell transcription factor 1, is expressed in early embryonic cells and primordial germ cells of mammals and has the function of regulating gene expression and cell proliferation and differentiation (Okuda et al., 1998; Raina et al., 2021). In addition, UTF1 has an effect on male germ cells. Inactivation of UTF1 leads to a decrease in the number of germ cells at birth, while conditional inactivation of UTF1 leads to impaired spermatogenesis in adult mice, indicating that UTF1 plays an important role in spermatogenesis (Kasowitz et al., 2017). LncRNA Gm2044 is located on the minus strand of DNA, while Utf1 is located on its adjacent plus strand. Blast analysis showed that Utf1 mRNA and lncRNA Gm2044 had five complementary sequences (Hu et al., 2018). Furthermore, overexpression of lncRNA Gm2044 in mouse spermatogonia and knockdown of lncRNA Gm2044 in mouse spermatocytes resulted in the inhibition and increase in UTF1 translation level, respectively, but did not affect Utf1 mRNA. This suggests that lncRNA Gm2044 can regulate the expression of UTF1 protein by interacting with mRNA, which is important for germ cell meiosis and development (Hu et al., 2018). In addition, overexpression of lncRNA Gm2044 also reduced Rbfox2 mRNA levels and inhibited the translation of RBFOX2 (RNA binding fox-1 homolog 2), as detailed below (Chen et al., 2017; Liang et al., 2019).
3.2 lncRNA Gm2044 as miRNA sponge/host gene
MiRNA is a class of endogenous non-coding RNA with regulatory functions and a length of about 20–25 nucleotides. Previous studies have demonstrated the importance of miRNAs in germ cell development (Loke et al., 2019). There are many mechanisms of interaction between miRNA and lncRNA, the most common one being that lncRNA competitively binds miRNA, that is, lncRNA acts as a miRNA sponge to regulate downstream signaling pathways and biological processes (Ma et al., 2023). MiR-202 is a regulator of meiosis initiation, and the knockdown of miR-202 can lead to premature differentiation and maturation of mouse spermatogonia (Chen et al., 2017; Chen et al., 2021). In our previous study, the expression of lncRNA Gm2044 and miR-202 was found to be significantly upregulated in non-obstructive azoospermia (NOA) with spermatogonia arrest (Liang et al., 2019). Moreover, lncRNA Gm2044 inhibited the expression of Rbfox2 (a known direct target gene of miR-202) by acting as the host gene of miR-202 and participated in the miR-202-Rbfox2 signaling pathway to inhibit the proliferation of human testicular embryonic carcinoma cells NCCIT (Liang et al., 2019).
In addition, lncRNA Gm2044 regulates the proliferation of GC-2 cells (cells differentiated from mouse spermatocytes) through its interaction with miRNA335-3P (Liang et al., 2020). A-MYB is a transcription factor encoded by the Mybl1 gene that appears in the early stage of spermatogenesis. It can bind to the −819 bp binding site in the distal promoter region of lncRNA Gm2044 and increase the transcription level of lncRNA Gm 2044 (Tang and Goldberg, 2012; Alexander et al., 2023). LncRNA Gm2044 is a competing endogenous RNA of miRNA335-3P, which directly targets Sycp1 (Meiotic transverse filament proteins). Overexpression of miRNA335-3P promoted SYCP1 protein synthesis (Figure 2). In summary, in the presence of A-MYB, lncRNA Gm2044 acted as a miRNA335-3P sponge to regulate the downstream signaling pathway of SYCP1 protein expression and the proliferation of GC-2 cells (Liang et al., 2020; Soheila et al., 2022). Similarly, lncRNA AK015322, which is highly expressed in spermatogonial stem cells of the testis, can act as a miR-19b-3p sponge to promote the proliferation of spermatogonial stem cells (Hu et al., 2017).
4 Regulation mechanism of lncRNA Gm2044 in female reproduction
4.1 LncRNA Gm2044 interacts with miR-138-5p to regulate estradiol synthesis
It has been reported that lncRNA plays an important role in ovarian follicle development. Through the gene annotation database BioGPS, we observed that lncRNA Gm2044 was highly expressed in pachytene spermatocytes in the testis, and it was also highly expressed in ovarian follicles, suggesting that lncRNA Gm2044 may have a potential effect on ovarian follicle development (Hu et al., 2018). RT-qRCR showed that lncRNA Gm2044 was significantly expressed in pre-antral follicular granulosa cells to preovulatory follicles in the four stages of follicular cells (Hu et al., 2019). The expression level of lncRNA Gm2044 was significantly higher in mouse pre-antral follicular granulosa cells (mpGCs) isolated from mouse follicles than in other mouse cell lines (Hu et al., 2019). LncRNA Gm2044 was predicted to be a competing endogenous RNA of miR-138-5p, and miR-138-5p has potential effects on downstream steroid hormones. Nr5a1 was confirmed to be a direct target of miR-138-5p, and miR-138-5p inhibited the translation of Nr5a1 mRNA and reduced the expression of NR5A1 protein (Hu et al., 2019). NR5A1, an important transcription factor for Cyp19a1, a key aromatase for 17β-estradiol synthesis, is a 461-amino acid nuclear receptor steroidogenic factor, and loss of Nr5a1 causes adrenal and gonadal dysgenesis in mice. Moreover, the absence of this transcription factor in ovarian granulosa cells can lead to damage to follicle formation in the ovary, leading to infertility (Pelusi et al., 2008; Hu et al., 2019). By targeting the miR-138-5p-Nr5a1 pathway, the inhibitory effect of knockdown of lncRNA Gm2044 on 17β-estradiol was relieved when Nr5a1 was overexpressed in mpGCs, and Nr5a1 upregulation promoted estradiol synthesis. This suggests that lncRNA Gm2044 can promote the synthesis of 17β-estradiol in mpGCs isolated from preantral follicles by acting as a miR-138-5p sponge (Hu et al., 2019). In addition, Sirt1 was found to be a direct target of miR-138-5p, and lncRNA Gm2044 also promoted the synthesis of 17β-estradiol by targeting Sirt1 with miR-138-5p (Zhu et al., 2017).
4.2 LncRNA Gm2044 interacts with EEF2 to regulate estradiol synthesis
Recent studies have found that EEF2 is also involved in the regulation of 17β-estradiol by lncRNA Gm 2044. There have been many studies on EEF2, for example, miR-642a-5p can directly target EEF2 to mediate the high permeability and apoptosis of pulmonary microvascular endothelial cells induced by lipopolysaccharide (Fei et al., 2020). EEF2 plays a dual role in the apoptosis of cardiomyocytes during myocardial ischemia-reperfusion (Zhang et al., 2017). ChIRP-MS analysis of adolescent mouse ovaries showed that 21 proteins could specifically bind to lncRNA Gm 2044. Bioinformatics analysis showed that EEF2 may affect the synthesis of 17β-estradiol by interacting with lncRNA Gm 2044. To understand the mechanism of lncRNA Gm2044 and EEF2 in the female ovary, lncRNA Gm2044 knockout mice were generated. The results showed that the fertility of lncRNA Gm2044 knockout mice was similar to that of normal mice, but the concentration of estradiol was significantly reduced. Meanwhile, overexpression of lncRNA Gm2044 increased the NR5A1 protein level and estradiol concentration, while Eef2 knockdown attenuated the effects of lncRNA Gm2044 on NR5A1 and estradiol concentration because EEF2 can bind to Nr5a1 mRNA and promote NR5A1 protein synthesis. Finally, it promotes an increase in estradiol concentration. These results suggest that Gm2044 interacts with EEF2 to promote estradiol synthesis (Figure 3). In addition, some differentially expressed genes (Tph1, Sost, etc.) were found to be involved in estradiol synthesis in lncRNA Gm2044 KO mice, and the new regulatory network composed of these differentially expressed genes and lncRNA Gm2044 will provide a new scheme for studying estradiol in the ovary (Hu et al., 2023).
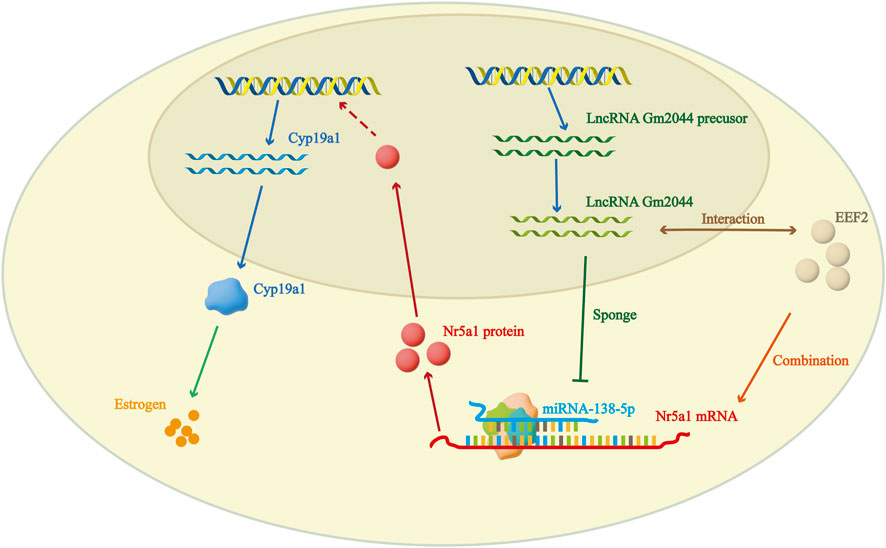
Figure 3. LncRNA Gm2044 can regulate 17β-estradiol synthesis by interacting with EEF2 or by acting as a miRNA sponge.
5 Reproductive function diseases potentially related to lncRNA Gm2044
5.1 Non-obstructive azoospermia
There are also many types of male infertility, and about 15% of infertile men each year suffer from azoospermia, such as NOA caused by spermatogenesis disorders and obstructive azoospermia caused by obstruction of the vas deferens, in which no sperm are found in the semen. The common causes of NOA include congenital chromosomal abnormalities, gene mutations, and varicocele, but the specific pathogenesis of NOA remains unknown (Peña et al., 2020). A previous study found that lncRNA participates in the progression of NOA (Zhou et al., 2022). LINC00467 upregulated the expression levels of genes related to spermatogenesis (TDRD6 and LRGUK) to promote the progression of NOA (Bo et al., 2020). LncRNA Gm2044 was found to be highly expressed in the spermatocyte cell line GC-2, abnormally expressed in NOA with spermatogonia arrest, and able to inhibit the proliferation of mouse spermatogonia GC-1 and mouse spermatogonia GC-2 (Liang et al., 2019), suggesting that lncRNA Gm2044 is involved in the progression of NOA, which is an interesting finding. However, the specific role of lncRNA Gm2044 in the pathogenesis of NOA has not been elucidated and needs further study.
5.2 Polycystic ovary syndrome
It has been reported that approximately 10% of couples of reproductive age worldwide are affected by infertility, a disorder of reproductive dysfunction (Agarwal et al., 2021). There are many causes of infertility, but for women, infertility caused by ovulation disorder due to polycystic ovary syndrome (PCOS) is the most common. However, the etiology of PCOS remains unclear; it may be caused by a combination of environmental, genetic, and other diseases, and the estrogen in PCOS patients is often unbalanced. Some lncRNA are related to PCOS. For example, silencing of lncRNA UCA1 can mediate the AKT signaling pathway to inhibit the progression of PCOS (Yang et al., 2021). LncRNA BANCR is involved in PCOS by up-regulating the pro-apoptotic protein Bax and p53 to promote apoptosis (Yang et al., 2019). CYP19A1 is an aromatase that can convert steroid hormones to estrogen, and together with CYP11A1, CYP17A1 may play a key role in the progression of PCOS (Parween et al., 2020; Heidarzadehpilehrood et al., 2022). Nr5a1 is an important transcription factor of CYP19A1 and a target gene of miR-138-5p, suggesting that lncRNA Gm2044 may regulate its downstream signaling pathway by acting as a sponge for miR-138-5p-Nr5a1. It may eventually participate in the pathogenesis of PCOS (Hu et al., 2019), which provides new ideas for the treatment of female infertility in the future.
6 Future perspectives
In recent years, more and more attention has been paid to lncRNA, and the main functional mechanisms of lncRNAs have gradually become clear. LncRNAs can be divided into those involved in transcriptional regulation or post-transcriptional regulation, according to their functional mechanisms. Among these mechanisms, splicing regulation and translation control are the two most important regulatory mechanisms of lncRNA in post-transcriptional regulation. In addition, lncRNA can also act as ceRNA to interact with miRNA to regulate downstream signaling pathways. LncRNA Gm2044 was used as a ceRNA to participate in spermatogenesis and estrogen regulation.
Infertility is a problem that has troubled people for a long time, and there is an urgent need to solve all kinds of infertility. LncRNA plays an important role in germ cell development, but research on lncRNA as a drug therapy is still in its infancy. There are a wide variety of lncRNAs that are expected to become potential targets for gene-drug therapy. Targeting one or more lncRNAs alone may be the most promising method for the treatment of infertility. At the same time, the lncRNA-miRNA-mRNA regulatory network also provides a new perspective for the treatment of various types of infertility.
In this review, we summarize recent findings on lncRNA Gm 2044, from the discovery of lncRNA Gm2044 to the biological functions it plays in the testis and ovary. LncRNA Gm2044 is highly expressed in specific stages of meiosis of female and male gametes, and can interact with a variety of miRNAs and transcription factors to participate in spermatogenesis or estrogen synthesis in follicles. LncRNA Gm2044 is also involved in the progression of NOA and PCOS, which provides a new target for the treatment of infertility. Whether lncRNA Gm2044 plays a role in other infertility or molecular signaling pathways remains to be investigated.
The differentially expressed genes Tph1, Sost, and Zp3 found in lncRNA Gm2044 knockout mice are also related to follicle development (Hu et al., 2023). It is believed that with the elucidation of the functional mechanism of these genes in germ cells, a new regulatory network composed of lncRNA may be discovered, providing new ideas for the treatment of diseases of the reproductive system.
Author contributions
QZ: Writing–original draft, Writing–review and editing, Conceptualization, Investigation, Visualization. JS: Supervision, Methodology, Validation, Formal-analysis, Writing–review and editing. CA: Writing–review and editing, Conceptualization, Formal Analysis, Validation, Visualization. XL: Writing–review and editing, Conceptualization, Data curation, Formal Analysis, Validation, Visualization. SX: Writing–review and editing, Conceptualization, Formal Analysis, Validation, Visualization. YH: Methodology, Project administration, Supervision, Writing–review and editing. XZ: Writing–review and editing, Methodology, Project administration, Supervision. LL: Funding acquisition, Validation, Writing–review and editing, Formal Analysis, Resources. KH: Writing–review and editing, Formal Analysis, Funding acquisition, Resources, Validation. ML: Writing–review and editing, Formal Analysis, Funding acquisition, Resources, Validation.
Funding
The author(s) declare that financial support was received for the research, authorship, and/or publication of this article. This research was supported by Anhui Provincial Key Research and Development Project of China (202104j07020016) and 512 Talent Cultivation Plan of Bengbu Medical College of China (by51201207).
Conflict of interest
The authors declare that the research was conducted in the absence of any commercial or financial relationships that could be construed as a potential conflict of interest.
Publisher’s note
All claims expressed in this article are solely those of the authors and do not necessarily represent those of their affiliated organizations, or those of the publisher, the editors and the reviewers. Any product that may be evaluated in this article, or claim that may be made by its manufacturer, is not guaranteed or endorsed by the publisher.
References
Agarwal, A., Baskaran, S., Parekh, N., Cho, C. L., Henkel, R., Vij, S., et al. (2021). Male infertility. Lancet 397 (10271), 319–333. doi:10.1016/s0140-6736(20)32667-2
Alexander, A. K., Rice, E. J., Lujic, J., Simon, L. E., Tanis, S., Barshad, G., et al. (2023). A-MYB and BRDT-dependent RNA Polymerase II pause release orchestrates transcriptional regulation in mammalian meiosis. Nat. Commun. 14 (1), 1753. doi:10.1038/s41467-023-37408-w
Atanasovska, B., Rensen, S. S., Marsman, G., Shiri-Sverdlov, R., Withoff, S., Kuipers, F., et al. (2021). Long non-coding RNAs involved in progression of non-alcoholic fatty liver disease to steatohepatitis. Cells 10 (8), 1883. doi:10.3390/cells10081883
Baddela, V. S., Michaelis, M., Tao, X., Koczan, D., and Vanselow, J. (2023). ERK1/2-SOX9/FOXL2 axis regulates ovarian steroidogenesis and favors the follicular-luteal transition. Life Sci. Alliance 6 (10), e202302100. doi:10.26508/lsa.202302100
Bao, J., Wu, J., Schuster, A. S., Hennig, G. W., and Yan, W. (2013). Expression profiling reveals developmentally regulated lncRNA repertoire in the mouse male germline. Biol. Reprod. 89 (5), 107. doi:10.1095/biolreprod.113.113308
Bo, H., Liu, Z., Zhu, F., Zhou, D., Tan, Y., Zhu, W., et al. (2020). Long noncoding RNAs expression profile and long noncoding RNA-mediated competing endogenous RNA network in nonobstructive azoospermia patients. Epigenomics 12 (8), 673–684. doi:10.2217/epi-2020-0008
Cannarella, R., Condorelli, R. A., Mongioì, L. M., La Vignera, S., and Calogero, A. E. (2020). Molecular biology of spermatogenesis: novel targets of apparently idiopathic male infertility. Int. J. Mol. Sci. 21 (5), 1728. doi:10.3390/ijms21051728
Chen, J., Cai, T., Zheng, C., Lin, X., Wang, G., Liao, S., et al. (2017). MicroRNA-202 maintains spermatogonial stem cells by inhibiting cell cycle regulators and RNA binding proteins. Nucleic Acids Res. 45 (7), 4142–4157. doi:10.1093/nar/gkw1287
Chen, J., Gao, C., Lin, X., Ning, Y., He, W., Zheng, C., et al. (2021). The microRNA miR-202 prevents precocious spermatogonial differentiation and meiotic initiation during mouse spermatogenesis. Development 148 (24), dev199799. doi:10.1242/dev.199799
Esmaeilian, Y., Hela, F., Bildik, G., İltumur, E., Yusufoglu, S., Yildiz, C. S., et al. (2023). Autophagy regulates sex steroid hormone synthesis through lysosomal degradation of lipid droplets in human ovary and testis. Cell Death Dis. 14 (5), 342. doi:10.1038/s41419-023-05864-3
Fei, L., Sun, G., and You, Q. (2020). miR-642a-5p partially mediates the effects of lipopolysaccharide on human pulmonary microvascular endothelial cells via eEF2. FEBS Open Bio 10 (11), 2294–2304. doi:10.1002/2211-5463.12969
Han, X., Guo, B., Zhao, S., Li, Y., Zhu, J., He, Y., et al. (2023). lncRNA Helf promotes hepatic inflammation and fibrosis by interacting with PTBP1 to facilitate PIK3R5 mRNA stabilization. Cell Mol. Biol. Lett. 28 (1), 77. doi:10.1186/s11658-023-00492-3
He, C., Wang, K., Gao, Y., Wang, C., Li, L., Liao, Y., et al. (2021). Roles of noncoding RNA in reproduction. Front. Genet. 12, 777510. doi:10.3389/fgene.2021.777510
Heidarzadehpilehrood, R., Pirhoushiaran, M., Abdollahzadeh, R., Binti Osman, M., Sakinah, M., Nordin, N., et al. (2022). A review on CYP11A1, CYP17A1, and CYP19A1 polymorphism studies: candidate susceptibility genes for polycystic ovary syndrome (PCOS) and infertility. Genes (Basel) 13 (2), 302. doi:10.3390/genes13020302
Hu, K., Gao, Y., Xu, Y., He, C., Wang, K., Li, L., et al. (2022). Overexpression of lncRNA-Gm2044 in spermatogonia impairs spermatogenesis in partial seminiferous tubules. Poult. Sci. 101 (7), 101930. doi:10.1016/j.psj.2022.101930
Hu, K., He, C., Sun, X., Li, L., Xu, Y., Zhang, K., et al. (2021). Integrated study of circRNA, lncRNA, miRNA, and mRNA networks in mediating the effects of testicular heat exposure. Cell Tissue Res. 386 (1), 127–143. doi:10.1007/s00441-021-03474-z
Hu, K., Zhang, J., and Liang, M. (2017). LncRNA AK015322 promotes proliferation of spermatogonial stem cell C18-4 by acting as a decoy for microRNA-19b-3p. Vitro Cell Dev. Biol. Anim. 53 (3), 277–284. doi:10.1007/s11626-016-0102-5
Hu, K., He, C., Ren, H., Wang, H., Liu, K., Li, L., et al. (2019). LncRNA Gm2044 promotes 17β-estradiol synthesis in mpGCs by acting as miR-138-5p sponge. Mol. reproduction Dev. 86 (8), 1023–1032. doi:10.1002/mrd.23179
Hu, K., Wang, C., Xu, Y., Li, F., Han, X., Song, C., et al. (2023). Interaction of lncRNA Gm2044 and EEF2 promotes estradiol synthesis in ovarian follicular granulosa cells. J Ovarian. Res. 16 (1), 171. doi:10.1186/s13048-023-01232-z
Hu, K., Li, L., Liao, Y., and Liang, M. (2018). LncRNA Gm2044 highly expresses in spermatocyte and inhibits Utf1 translation by interacting with Utf1 mRNA. Genes and genomics 40 (7), 781–787. doi:10.1007/s13258-018-0690-4
Huang, Y., Yi, Q., Feng, J., Xie, W., Sun, W., and Sun, W. (2022). The role of lincRNA-p21 in regulating the biology of cancer cells. Hum. Cell 35 (6), 1640–1649. doi:10.1007/s13577-022-00768-4
Kataruka, S., Akhade, V. S., Kayyar, B., and Rao, M. R. S. (2017). Mrhl long noncoding RNA mediates meiotic commitment of mouse spermatogonial cells by regulating Sox8 expression. Mol. Cell Biol. 37 (14), e00632. doi:10.1128/MCB.00632-16
Kasowitz, S. D., Luo, M. C., Ma, J., Leu, N. A., and Wang, P. J. (2017). Embryonic lethality and defective male germ cell development in mice lacking UTF1. Sci. Rep. 7 (1), 17259. doi:10.1038/s41598-017-17482-z
Kayyar, B., Kataruka, S., Suresh Akhade, V., and Rao, M. R. S. (2023). Molecular functions of Mrhl lncRNA in mouse spermatogenesis. Reproduction 166 (3), R39–r50. doi:10.1530/REP-23-0065
Kong, F. R., Lv, Y. H., Yao, H. M., Zhang, H. Y., Zhou, Y., and Liu, S. E. (2019). LncRNA PCAT6 promotes occurrence and development of ovarian cancer by inhibiting PTEN. Eur. Rev. Med. Pharmacol. Sci. 23 (19), 8230–8238. doi:10.26355/eurrev_201910_19132
Kubota, H., and Brinster, R. L. (2018). Spermatogonial stem cells. Biol. Reprod. 99 (1), 52–74. doi:10.1093/biolre/ioy077
Liang, M., and Hu, K. (2019). Involvement of lncRNA-HOTTIP in the repair of ultraviolet light-induced DNA damage in spermatogenic cells. Mol. Cells 42 (11), 794–803. doi:10.14348/molcells.2019.0121
Liang, M., Li, W., Tian, H., Hu, T., Wang, L., Lin, Y., et al. (2014). Sequential expression of long noncoding RNA as mRNA gene expression in specific stages of mouse spermatogenesis. Sci. Rep. 4, 5966. doi:10.1038/srep05966
Liang, M., Wang, H., He, C., Zhang, K., and Hu, K. (2020). LncRNA-Gm2044 is transcriptionally activated by A-MYB and regulates Sycp1 expression as a miR-335-3p sponge in mouse spermatocyte-derived GC-2spd(ts) cells. Differentiation 114, 49–57. (prepublish). doi:10.1016/j.diff.2020.05.004
Liang, W. C., Fu, W. M., Wang, Y. B., Sun, Y. X., Xu, L. L., Wong, C. W., et al. (2016). H19 activates Wnt signaling and promotes osteoblast differentiation by functioning as a competing endogenous RNA. Sci. Rep. 6, 20121. doi:10.1038/srep20121
Liang, M., Hu, K., He, C., Zhou, J., and Liao, Y. (2019). Upregulated lncRNA Gm2044 inhibits male germ cell development by acting as miR-202 host gene. Animal cells Syst. 23 (2), 128–134. doi:10.1080/19768354.2019.1591506
Loke, H., Rainczuk, K., and Dimitriadis, E. (2019). MicroRNA biogenesis machinery is dysregulated in the endometrium of infertile women suggesting a role in receptivity and infertility. J. Histochem Cytochem 67 (8), 589–599. doi:10.1369/0022155419854064
Ma, B., Wang, S., Wu, W., Shan, P., Chen, Y., Meng, J., et al. (2023). Mechanisms of circRNA/lncRNA-miRNA interactions and applications in disease and drug research. Biomed. Pharmacother. 162, 114672. doi:10.1016/j.biopha.2023.114672
Ma, L., Bajic, V. B., and Zhang, Z. (2013). On the classification of long non-coding RNAs. RNA Biol. 10 (6), 925–933. doi:10.4161/rna.24604
Meng, D., Wu, L., Li, Z., Ma, X., Zhao, S., Zhao, D., et al. (2022). LncRNA TUG1 ameliorates diabetic nephropathy via inhibition of PU.1/RTN1 signaling pathway. J. Leukoc. Biol. 111 (3), 553–562. doi:10.1002/jlb.6a1020-699rrr
Miller, W. L. (2013). Steroid hormone synthesis in mitochondria. Mol. Cell. Endocrinol. 379 (1-2), 62–73. doi:10.1016/j.mce.2013.04.014
Ohinata, Y., Ohta, H., Shigeta, M., Yamanaka, K., Wakayama, T., and Saitou, M. (2009). A signaling principle for the specification of the germ cell lineage in mice. Cell 137 (3), 571–584. doi:10.1016/j.cell.2009.03.014
Okuda, A., Fukushima, A., Nishimoto, M., Orimo, A., Yamagishi, T., Nabeshima, Y., et al. (1998). UTF1, a novel transcriptional coactivator expressed in pluripotent embryonic stem cells and extra-embryonic cells. Embo J. 17 (7), 2019–2032. doi:10.1093/emboj/17.7.2019
Palazzo, A. F., and Lee, E. S. (2015). Non-coding RNA: what is functional and what is junk? Front. Genet. 6, 2. doi:10.3389/fgene.2015.00002
Palermo, R. (2007). Differential actions of FSH and LH during folliculogenesis. Reprod. Biomed. Online 15 (3), 326–337. doi:10.1016/s1472-6483(10)60347-1
Pan, X., Wang, Q., Yu, Y., Wu, W., Chen, L., Wang, W., et al. (2022). Antisense lncRNA NNT-AS1 promoted esophageal squamous cell carcinoma progression by regulating its sense gene NNT expression. Cell Death Discov. 8 (1), 424. doi:10.1038/s41420-022-01216-w
Parween, S., DiNardo, G., Baj, F., Zhang, C., Gilardi, G., and Pandey, A. V. (2020). Differential effects of variations in human P450 oxidoreductase on the aromatase activity of CYP19A1 polymorphisms R264C and R264H. J. Steroid Biochem. Mol. Biol. 196, 105507. doi:10.1016/j.jsbmb.2019.105507
Pelusi, C., Ikeda, Y., Zubair, M., and Parker, K. L. (2008). Impaired follicle development and infertility in female mice lacking steroidogenic factor 1 in ovarian granulosa cells. Biol. Reprod. 79 (6), 1074–1083. doi:10.1095/biolreprod.108.069435
Peña, V. N., Kohn, T. P., and Herati, A. S. (2020). Genetic mutations contributing to non-obstructive azoospermia. Best. Pract. Res. Clin. Endocrinol. Metab. 34 (6), 101479. doi:10.1016/j.beem.2020.101479
Petro, E. M., Leroy, J. L., Covaci, A., Fransen, E., De Neubourg, D., Dirtu, A. C., et al. (2012). Endocrine-disrupting chemicals in human follicular fluid impair in vitro oocyte developmental competence. Hum. Reprod. 27 (4), 1025–1033. doi:10.1093/humrep/der448
Phillips, B. T., Gassei, K., and Orwig, K. E. (2010). Spermatogonial stem cell regulation and spermatogenesis. Philos. Trans. R. Soc. Lond B Biol. Sci. 365 (1546), 1663–1678. doi:10.1098/rstb.2010.0026
Raina, K., Dey, C., Thool, M., Sudhagar, S., and Thummer, R. P. (2021). An insight into the role of UTF1 in development, stem cells, and cancer. Stem Cell Rev. Rep. 17 (4), 1280–1293. doi:10.1007/s12015-021-10127-9
Rui, Y., Hu, M., Wang, P., Zhang, C., Xu, H., Li, Y., et al. (2019). LncRNA HOTTIP mediated DKK1 downregulation confers metastasis and invasion in colorectal cancer cells. Histol. Histopathol. 34 (6), 619–630. doi:10.14670/HH-18-043
Salih, M. M., Robinson, E. K., Malekos, E., Perez, E., Capili, A., Kim, K., et al. (2023). LincRNA-Cox2 regulates smoke-induced inflammation in murine macrophages. Am. J. Respir. Cell Mol. Biol. 68 (5), 511–522. doi:10.1165/rcmb.2022-0413OC
Soheila, N., Masomeh, A., Maryam, R., Najmeh, S., Navid, A., Yaser, T., et al. (2022). A rare frameshift mutation in SYCP1 is associated with human male infertility. Mol. Hum. Reprod. 28, gaac009. doi:10.1093/molehr/gaac009
Stukenborg, J. B., Kjartansdóttir, K. R., Reda, A., Colon, E., Albersmeier, J. P., and Söder, O. (2014). Male germ cell development in humans. Horm. Res. Paediatr. 81 (1), 2–12. doi:10.1159/000355599
Subash, S. K., and Kumar, P. G. (2021). Spermatogonial stem cells: a story of self-renewal and differentiation. Front. Biosci. Landmark Ed. 26 (1), 163–205. doi:10.2741/4891
Sun, J., and Wu, J. (2015). Expression profiling of long noncoding RNAs in neonatal and adult mouse testis. Data Brief. 4, 322–327. doi:10.1016/j.dib.2015.06.004
Tan, C., Liu, W., Zheng, Z. H., and Wan, X. G. (2021). LncRNA HOTTIP inhibits cell pyroptosis by targeting miR-148a-3p/AKT2 axis in ovarian cancer. Cell Biol. Int. 45 (7), 1487–1497. doi:10.1002/cbin.11588
Tang, H., and Goldberg, E. (2012). A-MYB (MYBL1) stimulates murine testis-specific Ldhc expression via the cAMP-responsive element (CRE) site. Biol. Reprod. 86 (2), 30. doi:10.1095/biolreprod.111.095661
Wang, X., Zhang, X., Dang, Y., Li, D., Lu, G., Chan, W. Y., et al. (2020). Long noncoding RNA HCP5 participates in premature ovarian insufficiency by transcriptionally regulating MSH5 and DNA damage repair via YB1. Nucleic Acids Res. 48 (8), 4480–4491. doi:10.1093/nar/gkaa127
Wu, L., Liu, S., Qi, H., Cai, H., and Xu, M. (2020). Research progress on plant long non-coding RNA. Plants (Basel) 9 (4), 408. doi:10.3390/plants9040408
Xiao, Z. S., Long, H., Zhao, L., Li, H. X., and Zhang, X. N. (2020). LncRNA HOTTIP promotes proliferation and inhibits apoptosis of gastric carcinoma cells via adsorbing miR-615-3p. Eur. Rev. Med. Pharmacol. Sci. 24 (12), 6692–6698. doi:10.26355/eurrev_202006_21656
Yang, B., An, Y., Yang, Y., Zhao, Y., Yu, K., Weng, Y., et al. (2023). The ERβ-cAMP signaling pathway regulates estradiol-induced ovine oocyte meiotic arrest. Theriogenology 214, 81–88. doi:10.1016/j.theriogenology.2023.10.009
Yang, D., Wang, Y., Zheng, Y., Dai, F., Liu, S., Yuan, M., et al. (2021). Silencing of lncRNA UCA1 inhibited the pathological progression in PCOS mice through the regulation of PI3K/AKT signaling pathway. J. Ovarian Res. 14 (1), 48. doi:10.1186/s13048-021-00792-2
Yang, R., Chen, J., Wang, L., and Deng, A. (2019). LncRNA BANCR participates in polycystic ovary syndrome by promoting cell apoptosis. Mol. Med. Rep. 19 (3), 1581–1586. doi:10.3892/mmr.2018.9793
Zhang, C., Liu, X., Zhang, C., Li, J., Guo, W., Yan, D., et al. (2017). Phosphorylated eEF2 is SUMOylated and induces cardiomyocyte apoptosis during myocardial ischemia reperfusion. J. Cardiol. 69 (4), 689–698. doi:10.1016/j.jjcc.2016.05.020
Zhang, C., Lu, D., Niu, T., Sun, Z., Wang, Y., Han, X., et al. (2023). LncRNA5251 inhibits spermatogenesis via modification of cell-cell junctions. Biol. Direct 18 (1), 31. doi:10.1186/s13062-023-00381-x
Zhang, S., Ma, Q., Wu, X., and Chen, P. (2022). LncRNA HOTTIP promotes ovarian cancer cell invasion and metastasis by stabilizing HIF-1α in the anoxic cellular microenvironment. Acta Endocrinol. (Buchar) 18 (3), 263–270. doi:10.4183/aeb.2022.263
Zhou, G., Zhang, M., Zhang, J., Feng, Y., Xie, Z., Liu, S., et al. (2022). The gene regulatory role of non-coding RNAs in non-obstructive azoospermia. Front. Endocrinol. (Lausanne) 13, 959487. doi:10.3389/fendo.2022.959487
Zhou, R., Wu, J., Liu, B., Jiang, Y., Chen, W., Li, J., et al. (2019). The roles and mechanisms of Leydig cells and myoid cells in regulating spermatogenesis. Cell Mol. Life Sci. 76 (14), 2681–2695. doi:10.1007/s00018-019-03101-9
Keywords: lncRNA, spermatogenesis, estrogen, ovaries, miRNA
Citation: Zhu Q, Sun J, An C, Li X, Xu S, He Y, Zhang X, Liu L, Hu K and Liang M (2024) Mechanism of LncRNA Gm2044 in germ cell development. Front. Cell Dev. Biol. 12:1410914. doi: 10.3389/fcell.2024.1410914
Received: 24 April 2024; Accepted: 03 June 2024;
Published: 04 July 2024.
Edited by:
Massimo Venditti, University of Campania Luigi Vanvitelli, ItalyReviewed by:
Rosaria Meccariello, University of Naples Parthenope, ItalyGeng Tian, Shanghai Jiao Tong University, China
Copyright © 2024 Zhu, Sun, An, Li, Xu, He, Zhang, Liu, Hu and Liang. This is an open-access article distributed under the terms of the Creative Commons Attribution License (CC BY). The use, distribution or reproduction in other forums is permitted, provided the original author(s) and the copyright owner(s) are credited and that the original publication in this journal is cited, in accordance with accepted academic practice. No use, distribution or reproduction is permitted which does not comply with these terms.
*Correspondence: Lei Liu, bGl1bGVpQGJibWMuZWR1LmNu; Ke Hu, aHVrZTg1MTFAMTYzLmNvbQ==; Meng Liang, bG1oa0BtYWlsLnVzdGMuZWR1LmNu