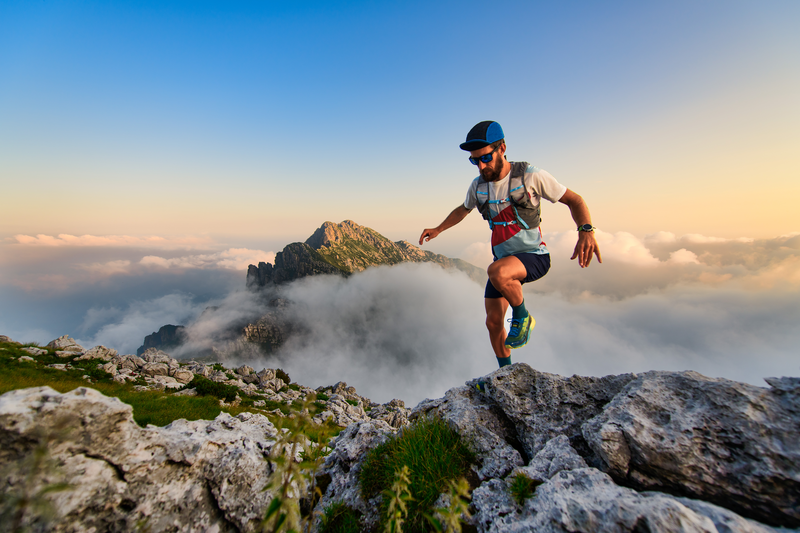
94% of researchers rate our articles as excellent or good
Learn more about the work of our research integrity team to safeguard the quality of each article we publish.
Find out more
REVIEW article
Front. Cell Dev. Biol. , 27 May 2024
Sec. Epigenomics and Epigenetics
Volume 12 - 2024 | https://doi.org/10.3389/fcell.2024.1397788
Lung cancer is the second most common form of cancer worldwide Research points to the pivotal role of non-coding RNAs (ncRNAs) in controlling and managing the pathology by controlling essential pathways. ncRNAs have all been identified as being either up- or downregulated among individuals suffering from lung cancer thus hinting that they may play a role in either promoting or suppressing the spread of the disease. Several ncRNAs could be effective non-invasive biomarkers to diagnose or even serve as effective treatment options for those with lung cancer, and several molecules have emerged as potential targets of interest. Given that ncRNAs are contained in exosomes and are implicated in the development and progression of the malady. Herein, we have summarized the role of ncRNAs in lung cancer. Moreover, we highlight the role of exosomal ncRNAs in lung cancer.
Lung cancer is one of the deadliest cancers and has a low 5-year survival rate, with only 22% of people affected surviving beyond that period. It is the most common form of cancer in both males and females (Siegel et al., 2018). The two varieties of lung cancer can be distinguished by the size of the cells that form them. The more prevalent type, non-small cell lung cancer, is seen in the majority of cases, around 85 percent. Small-cell lung cancer, though less common, still affects 15 percent of individuals (Inamura, 2017).
Lung cancer is a complicated disease, and it is brought about by a combination of genetic and epigenetic changes. This alteration permits pro-oncogenic and tumor suppressor genes, in addition to growth hormones, transcription factors, and other controlling molecules, to all factor into the rise of the cancer (Braicu et al., 2019). Recent studies on lung cancer have revealed the influential role of non-coding RNAs (ncRNAs). ncRNAs are pieces of RNA that do not form proteins but rather affect gene expression at both the transcriptional and post-transcriptional levels. These ncRNAs are separated into two classes: ones shorter than 200 base-pairs (bp) known as small non-coding RNAs and those longer than 200 bp referred to as long non-coding RNAs (Brosnan and Voinnet, 2009).
Exosomes are small extracellular sacs, measuring 40–160 nm across, which are created by invaginating the plasma membrane twice into numerous vesicles encased in a single intracellular container. The exosomes are eventually discharged by releasing these internal vesicles (ILVs) (Kalluri and LeBleu, 2020). Exosomes have been demonstrated to be produced and secreted from numerous types of cells, such as carcinoma cells (Wolfers et al., 2001), stromal cells (Fang et al., 2018), immune cells (Pitt et al., 2016), stem cells (Riazifar et al., 2019), and neurons (Fauré et al., 2006), etc. Research data aggregates to suggest that exosomes can act as messengers, ferrying intracellular material such as proteins, metabolites, amino acids, and DNA and RNA between cells (Kalluri and LeBleu, 2020). Previous investigations proposed that mRNA and miRNA were put into exosomes specifically to influence gene expression and oversee the activities of cells that received them (Valadi et al., 2007). Herein, we have summarized the role of ncRNAs in lung cancer. Moreover, we highlight the role of exosomal ncRNAs in lung cancer.
Cancer is an immensely complex condition across multiple levels, and its development and behavior require thorough investigation. In 2000, Hanahan et al. identified six significant traits of cancer such as sustaining a proliferation signal, evading growth inhibitors, and activating invasion and metastasis. Ten years down the line, Hanahan and colleagues added two more components to the list: avoiding immune destruction and disrupting cell energy. They have also suggested the aspects of promoting inflammation and genetic instability augment the emergence of tumors (Hanahan and Weinberg, 2011). For understanding cancer biology, both genetic and epigenetic factors must be considered. In this paper, we will discuss microRNAs (miRNAs) and their connected pathways that are involved in each pillar of cancer biology. Figure 1 presents an overview of miRNAs and their relevant pathways.
Figure 1. (A) schema of microRNAs and their pathways network in cancer. Epidermal growth factor (EGF) and its receptors, EGFR, work together to stimulate cell growth in lung cancer through the downstream RAS/ERK and PI3K/Akt/mTOR pathways. Similarly, EML4-ALK and ROS1 also trigger cancer cell growth through these pathways. Relevant miRNAs are depicted in the diagram. (B) Disruptions in tumor suppressors, RB and p53, enable cancer cells to avoid inhibition of growth. (C) The human telomerase reverse transcriptase (hTERT) is linked to the immortality of cancer cells. MiR-299, miR-491, miR-512, and miR-1182 have been found to target hTERT, but these findings have been studied in various cancer cells other than lung cancer. Additionally, the miR-29 family can control telomere length by targeting DNA methyltransferases (DNMT). (D) Snail, Slug, and Wnt are key factors in epithelial to mesenchymal transition (EMT), which is associated with cancer metastasis and invasion. The diagram shows the related miRNAs. (E) Vascular endothelial growth factors (VEGF) play a crucial role in promoting tumor angiogenesis. MiR-126, miR-128, and miR0-200 have been shown to target VEGF. (F) Cancer cells use aerobic glycolysis as their main metabolic pathway. For example, lung cancer cells with decreased levels of miR-144 exhibit increased expression of glucose transporter (GLUT1) and higher glucose uptake. (G) The interaction between programmed death-ligand 1 (PD-L1) and its receptor (PD-1) allows cancer cells to evade immune destruction. MiR-34, miR-138, miR-200, and miR-513 have been found to target PD-L1 and inhibit its expression. (H) Fas receptors (intrinsic pathway) and BH3-only proteins (extrinsic pathway) play important roles in preventing cell apoptosis. MiR-301b targets BH3-only proteins and miR-16 targets Bcl, both of which are involved in regulating cell apoptosis. Note: This diagram simplifies the major pathways involved in cancer, but there may also be cross-talk and interactions between different pathways that are not shown. For example, the EGFR pathway can not only promote cell proliferation but also enhance invasion, metastasis, angiogenesis, and resistance to apoptosis. This figure adapted from (Wu et al., 2019).
miRNAs play a role in regulating various genes and signal pathways. A considerable amount of evidence indicates that miRNAs are involved in lung cancer progression, either functioning as tumor suppressors or oncomirs. They exert their effects by modulating the expression of target mRNAs, which in turn impacts cancer biology and facilitates tumor growth, invasion, angiogenesis, and evasion of the immune system. Numerous studies have demonstrated the significance of miRNAs in regulating processes such as cell development, proliferation, invasion, migration, apoptosis, and metastasis in both SCLC and NSCLC (Frydrychowicz et al., 2023). When miRNA expression is deregulated, it leads to changes in their functionality. When miRNAs are overexpressed or underexpressed, they can act as either oncogenes, promoting the development of cancer, or tumor suppressor genes, inhibiting cancer growth. Interestingly, the same miRNA that acts as an oncogene in one type of cancer may have the opposite effect of inducing tumor suppression in another type of cancer. This is because miRNAs have multiple targets and can carry out various biological functions (Iqbal et al., 2019).
miR-21 exerts oncogenic activities by inhibiting the activity of various vital tumor suppressor genes (Far et al., 2022). A study of miRNA expression in various human tumor tissue samples uncovered that miR-21 was the sole miRNA that demonstrated an increment in each of the tumors surveyed (Far et al., 2022). NSCLC patients who show a substantial amount of miR-21 in their systems tend to be at an advanced stage of progression and frequently experience metastasis. Additionally, miR-21 has been demonstrated to accelerate the growth of cells and further a tumor’s invasion by impeding PTEN, a suppressor of tumor cells (Zhang J.-g. et al., 2010; Xue et al., 2016). The expression levels of miR-21 are heightened and linked to worse survival outcomes in NSCLC. miR-21 likely has oncogenic effects in NSCLC, as it has been proven in experiments that it hampers the action of Ras/MEK/ERK signaling mechanism inhibitors (Hatley et al., 2010). This indicates that miR-21 may present potential opportunities for cancer treatment.
Reinhart made the first identification of a 21-nucleotide-long non-coding RNA, namely, let 7, in the context of his research on developmental timing in the nematode Caenorhabditis elegans (Reinhart et al., 2000). Research has indicated that the Let-7 gene family consists of 11 members, six of which are situated in areas of the DNA that are sensitive to changes, making them likely to be affected by genetic modifications (Calin et al., 2004). It is possible that the lowering of let-7 levels may be caused by an alteration to its genetic material through epigenetic or genetic pathways, or because of agents that stop its transcribing process (Yamada et al., 2008). Let-7 plays a major part in reducing the activity of proliferation, inflammation, and anti-apoptotic pathways by lessening the activity of their consequent effectors, which include KRAS, c-MYC, CDK6, HOXA9, TGFBR1, BCL-XL, and MAP4K3, and thus producing an anti-cancerous atmosphere inside the cell (Wang X. et al., 2012). Various members of the Let-7 family of genes are transcribed from parts of the genome that are often absent in people with lung cancer, including let-7a, let-7c, and let-7g (Boyerinas et al., 2010). MiR-let-7 has been linked to tumor suppression, as demonstrated by its ability to decrease the expression of KRAS (Slack and Weidhaas, 2006). Recent studies involving the injection of MiR-let-7 mimic into a laboratory model of NSCLC led to a substantial decrease in the tumor area, size, and spread when compared to the control group (Trang et al., 2011). This has led to the suggestion that MiR-let-7 could be a useful treatment technique for lung cancer, having a positive correlation with patient prognosis in lung cancer (Xia et al., 2014).
It is believed that cancer’s core features involve prolonged cell division and unregulated cell growth. This process involves a variety of different genes and proteins, primarily kinases and their associated receptors (Van Roosbroeck and Calin, 2017). One of the most well-known signaling pathways in lung cancer is the Epidermal Growth Factor Receptor (EGFR) pathway. When certain molecules such as EGF or TGF-α bind to EGFR, it is in turn activated and transphosphorylated. This generates additional activation of two significant signaling pathways - Ras/Raf/MEK/ERK and PI3K/Akt/mTOR - leading to an increase in cell growth and an enhanced development of the cell cycle (Brambilla and Gazdar, 2009). It has been established that EGFR has an active association with miR-34, miR-27a-3p, miR-7, miR-30, miR-133, miR-146, miR-145, miR-218, miR-149, miR-128, miR-134, and miR-542-5p (Chan et al., 2012; Qin et al., 2016; Zhu et al., 2016; Van Roosbroeck and Calin, 2017).
The discovery of EML4-ALK fusion proteins as potential therapeutic targets for NSCLC is based on their capacity to activate the Ras/Raf/MEK/ERK and PI3K/Akt/mTOR signaling pathways (Karachaliou and Rosell, 2014). Vishwamitra et al. conducted research on a cell model that revealed that miR-96 acts as a post-transcriptional inhibitor of ALK (Vishwamitra et al., 2012).
The enzymes of glycolysis control the amount of glucose moving into cells, particularly the hexokinases (HKs), which act as gatekeepers by converting glucose to glucose-6-phosphate (G6P) in the initial reaction of glucose entering the metabolic process (Robey and Hay, 2006). HK1 and HK2 are identified as enzymes with a strong affinity, although the amount of these enzymes present can vary throughout different tissues. An unusual amount of HK2 is seen as a sign of malignant tumor formation (Mathupala et al., 2001). Studies using cancer cell lines and mice models as test subjects have shown that higher levels of HK2 are only found in cases of lung cancer (Patra et al., 2013). miR-124 is a microRNA that can impede tumor growth and affect glycolysis, lactate manufacturing, and ATP creation negatively. In lung cancer cells, when the expression of miR-124 is diminished, there is a considerable increment of glucose consumption and ATP production brought about by the bigger expression of GLUT1 and HK2 enzymes, two elements that are critical for controlling the glycolysis pathway (Zhao et al., 2017). The fact that changing the expression of Akt can reverse the effects of inhibiting miR-124 further emphasizes the role of miR-124 in modulating the activity of the AKt1 and AKt2 subunits, as well as regulating glycolysis.
The miR-17/92 family, located at human chromosome 13q31.3 in the intron three region of gene C13orf 25, contains six microRNAs: miR-17, miR-18a, miR-19a, miR-20a, miR-19b-1, and miR-92a-1. This chromosome location is commonly found to be increased in various kinds of tumors (Ota et al., 2004). Hayashita et al. were the first to discover that the miR-17/92 cluster is expressed at higher levels in lung cancer cells and helps to stimulate the expansion of these cells (Hayashita et al., 2005). The inhibition of the miR-17/92 family has a detrimental effect on non-small cell lung cancer cells that lack p53, because of its role in the reduction of CYP24A1 expression (Borkowski et al., 2015). The miR-19 family has been shown to upregulate Wnt signaling through targeting of p38α in non-small cell lung cancer (NSCLC), thus leading to an increased malignant potential in the cell type (Guinot et al., 2016). It has been revealed that the miR-19 family influences the malignant potential of NSCLC cells by increasing Wnt signaling through the blocking of p38α. Additionally, the suppression of miR-19b has been found to lower the phosphorylation of ERK, AKT, and effector proteins in EGFR mutant NSCLC cells. This means that targeting miR-19b could be a viable option for the treatment of EGFR mutant NSCLC (Baumgartner et al., 2018).
The KRAS gene is often linked to receptor tyrosine kinases such as EGFR, ALK, and ROS. Kirsten rat sarcoma 2 viral oncogene homolog is the specific name for this gene (Friday and Adjei, 2005). Approximately one-sixth of lung cancer specimens contain at least one KRAS mutation, which can prompt the Ras/Raf/MEK/ERK pathway to become active (Kopp et al., 2014). In laboratory and mouse testing, the let-7 family showed its ability to control KRAS expression and suppress the growth of lung cancer cells (He et al., 2010; Wang Y. Y. et al., 2013). Seviour and their team’s investigation uncovered that miR-193a-3p has a direct influence on KRAS, thereby inhibiting the growth of KRAS-mutated lung tumors in a living organism (Seviour et al., 2017). Given that miR-181a-5p is known to inhibit the activity of KRAS, it appears that this molecule may play a role in the progression and movement of A549 cells (Ma et al., 2015). Research has shown that miR-148a-3p can limit the development of non-small cell lung cancer cells in the lab through the regulation of SOS2, a chemical found in the Ras signaling pathway’s chain of events (Xie et al., 2019). Jiang et al. also found that miR-1258 could influenc GRB2 resulting in lower Grb2 levels, which was a fundamental protein needed to carry on Ras activation, consequently lowering the MEK/ERK pathway in mouse models (Jiang et al., 2018). The research conducted in a lab indicated that the JmiR-520a-3p participated in a downstream PI3K/Akt/mTOR signaling pathway (Lv et al., 2018). Table 1, lists various miRNAs that are involved in lung cancer.
LncRNAs, which make up 81.8% of all ncRNAs, are the most abundant type of ncRNA. They demonstrate distinct patterns in terms of their origins, timing, and location within specific tissues and cells, although they are not as abundant, stable, or conserved as mRNA. These crucial molecules play a role in regulating nearly every aspect of gene expression (Liu et al., 2021). The primary method in which they operate is to block the expression of target genes by attaching to miRNA and implementing an additional level of post-transcriptional regulation. In addition, lncRNAs have the ability to divert transcription factors (TFs) away from chromatin as a means of acting as molecular reservoirs, effectively changing the expression of genes. Furthermore, evidence suggests that they act as building blocks to form scaffolding complexes with regulators, ultimately leading to modifications in gene expression. Additionally, lncRNAs can direct the coordination of ribonucleoprotein to the promoters of downstream target genes, ultimately altering the transcriptional activity of genes. In addition, lncRNAs have the capacity to modify gene expression by influencing the processing, maturation, and stability of mRNAs (Lv et al., 2023).
Many research projects have used microarray profiling and deep sequencing data to demonstrate that an alteration in lncRNA expression is a primary component of both the initiation and progression of lung cancer (Gencel-Augusto et al., 2023; Rajakumar et al., 2023; Tang et al., 2023). There are specific mechanisms that can affect their expression in pathological conditions, which include the influence of chemicals and microenvironments in tumors. Furthermore, gene expression can be modified without any changes to the content of the DNA through epigenetic modification. The binding of transcription factors on the promoters of lncRNAs can trigger either their activation or their suppression; these various regulation models of lncRNAs in lung cancer have been summarized in Figure 2 and Table 2.
Figure 2. (A) diagram illustrating how regulation of dysregulated long non-coding RNAs (lncRNA) in lung cancer is influenced by various factors is shown. (A) Chemical compounds and reduction in oxygen levels regulate lncRNA expression which subsequently results in the advancement of cancer. (B) Modifications to the epigenetic makeup of lncRNAs can impact the growth of lung tumors. (C) Several transcription factors connect to lncRNAs and either incite or discourage their transcription, leading to changes in cancer development.
Research has demonstrated that PM2.5 could make people more susceptible to developing lung cancer. It appears lncRNA is involved in this process, as there is an increase in Reactive Oxygen Species (ROS) resulting from large exposure and an increase in lncRNA expression in NSCLC cells. This could also be affected by the autophagy of cancer cells, boosting the infiltration and migration of tumors. Additionally, employment-related exposure greatly affects the rate of occurrence of NSCLC, such as a decrease in the activity of the lncRNA MEG3 – which has anti-growth characteristics – when exposed to nickel (Zhang X. et al., 2010; Wang P. et al., 2012; Lu et al., 2013; Mondal et al., 2015; Zhou et al., 2017). Nickel exposure can lead to hypermethylation and inhibition of the MEG3 promoter, which can eventually cause carcinogenic effects on human bronchial epithelial cells. This research shows just how influential long non-coding RNAs are in the incidence of Non-Small Cell Lung Cancer. Understanding these factors and pathways should help ascertain the biological basis of NSCLC and pave the way to its prevention and treatment (Figure 3). CDKs and CKIs are essential for keeping the cell cycle in balance, an occurrence that is common in cancer. For this, lncRNA lnc00152 and lnc00511, both observed to be increased in LUAD cases, are capable of promoting tumor growth by preventing the activity of IL24 and p57, which are components of the tumor suppressor mechanism respectively (Sun et al., 2016; Chen Q. N. et al., 2017). According to recent research, LUADT1, a long noncoding RNA that has been observed to be significantly expressed in lung adenocarcinoma tumors, is associated with histological T stage. When LUADT1 joins with SUZ12, the trimethylation of H3K27 is cause in the promoter area of the antitumor P27. As a result, this hinders the expression of P27, thus affecting its scope of action epigenetically (Qiu et al., 2015).
Figure 3. The role of lncRNAs in lung cancer. The lncRNA XLOC_008466 exhibits a high level of expression in patients with NSCLC. When XLOC_008466 expression is suppressed, there is a decrease in cell proliferation and invasion, along with a promotion of apoptosis. This lncRNA acts in a manner similar to a ceRNA, directly binding to and downregulating miR-874. As a result, the expression of miR-874 downstream targets, MMP2 and XIAP, is increased. Additionally, there is an axis involving lncRNA Gm15290, miR-615-5p, and targeted genes in lung cancer. AS5 expression is downregulated in lung cancer tissues and cells, while miR-135b expression is upregulated. The high expression of GAS5 and low expression of miR-135b have been shown to significantly reduce the survival rate of lung cancer cells under irradiation and improve radiotherapy sensitivity. Furthermore, this can also effectively inhibit tumor occurrence by suppressing the proliferation and invasion of tumor cells.
It is believed that Cancer stem cells (CSCs) represent a group of tumor cells which display characteristics such as the capacity to renew themselves or to transform into non-stem cancerous progeny. Therefore, these cells tend to play a major role in various functions such as resistance to therapy, development of tumors, change from epithelial to mesenchymal cells (EMT) and metastatic spread of tumors (Clarke and Fuller, 2006; Zhao, 2016). The expression of certain cell surface biomarkers such as CD44, CD133, OCT-4, Bmi-1, ALDH1, ABCG2 and KLF4 is highly characteristic of cancer stem cells (CSCs), and is thought to be linked to the decreased susceptibility of CSCs to cancer treatments. For instance, previous studies have investigated the characteristics of CSCs resistance to cancer treatment, including slower-than-normal cell division rates and dormant states (Vidal et al., 2014; Ajani et al., 2015). The cancer microenvironment, along with multiple regulatory factors, are integral to both the maintenance of cancer stem cells (CSCs) and the growth and advancement of tumors. Recently, lncRNAs that are involved in both CSCs and tumor evolution have been the focus of many studies (Schwerdtfeger et al., 2021).
LnRNA HOTAIR has been observed to activate tumor growth through its influence on CSCs (cancer stem cells) and EMT processes, and its activity is triggered directly by STAT3 (signal transducer and activator of transcription 3) when a person is exposed to cigarette smoke (Liu et al., 2015). Previous research has evaluated the connection between being a stem cell and EMT pathways (Wilson et al., 2020). The influence of DUXAP10 increased in Cd-induced lung cells, while eliminating DUXAP10 caused a decrease in stemness markers like KLF4, KLF5 and Nanog. Moreover, this led to a reduction in the number of stem cells labeled by CD133, which was due to the inhibition of the Hedgehog signaling pathway which played a part in Cd-induced cancer of the lung (Lin et al., 2021). Liu et al. demonstrated that HOTAIR, a long non-coding RNA, could generate resistance to cancer treatments such as cisplatin by prompting two stem cell markers - β-catenin and KLF4, with an emphasis on specifically influencing KLF4 to enhance stemness (Liu M. Y. et al., 2016).
Xue et al. found that GAS5 levels were lower and miR-135b levels higher in NSCLC tissues and cells (Xue et al., 2017). The analysis discovered that non-small cell lung cancer cells were more sensitive to the effects of radiation when there was an elevated presence of GAS5 and a depleted presence of miR-135b, making radiation treatments more efficient. This was seen via decreased survival rates when the cells were exposed to radiation, and decreased spread and increased deterrence of tumor growth due to more restricted tumor cell proliferation and invasion (illustrated in Figure 4).
The lncRNA MALAT1 has a length of 8.7 kb, and is commonly found in human tissue types. Overall, it exhibits conservation across mammalian species (Zhang et al., 2017). The dysregulation of MALAT1 has been observed in different types of cancers such as lung, liver, prostate, colon, uterus, ovarian, breast, neuroblastoma, and blood-based cancers. Its role appears to involve the post-transcriptional control of the gene expression and splicing of mRNA (Bhan et al., 2017; Huang et al., 2017; Li Z. X. et al., 2018; He et al., 2019; Sun and Ma, 2019).
Malat1 is an oncogenic lncRNA that can increase cancer cell proliferation, migration, invasion, and EMT. Thus, Malat1 may be responsible for cancer cell survival and growth. Malat1 may also play a role in cancer chemoresistance (Schmidt et al., 2011; Li Z. X. et al., 2018; Sun and Ma, 2019). It seems that MALAT1 is more highly expressed in NSCLC tissues than in normal tissues and that MALAT1 expression is correlated with the overall survival of NSCLC. If this is true, then MALAT1 may be a useful biomarker for identifying patients who are likely to benefit from treatment with chemotherapy (Chen et al., 2018). Some research suggests that MALAT1 might play a role in regulating the myeloid-derived suppressor cells (MDSCs) in lung cancer patients. This could potentially lead to problems with the immune system’s ability to fight cancer (Zhou et al., 2018). Silencing of MALAT1 has been shown in cultured NSCLC cells to decrease proliferation and colony formation (Gutschner et al., 2013).
MALAT1 may also act as a regulator of several important genes by way of a non-coding RNA-mediated process. For example, MALAT1 can increase the expression of zinc finger E-box binding homeobox 1 (ZEB1) in A549 cells by rapidly soaking up miR-200a, thus stimulating cell proliferation (Feng et al., 2019). The miR-200b protein plays a role in linking up with the E2F transcription factor 3 (E2F3) and ZEB1 mRNAs which are found in the cytoplasm of the cells. When miR-200b interacts with these mRNAs, it can lead to the levels of E2F3 and ZEB1 proteins being raised in DTX (docetaxel) - resistant lung adenocarcinoma cells. This then allows these cells to become more tolerant of chemotherapy. Therefore, miR-200a could be proposed as a potential prognostic marker to identify DTX–resistant patients (Chen J. et al., 2019). The effects of MALAT1 on the regulation of different processes are outlined in Figure 4.
Figure 4. Biology of exosomal miRNAs. In animals, RNA polymerase II transcribes microRNA (miRNA) genes, producing primary miRNAs (pri-miRNAs), which are then modified by the Drosha complex into precursor miRNAs (pre-miRNAs). These pre-miRNAs are then transported to the cytoplasm by the exportin5 complex. After being digested by the Dicer complex, they become double-stranded miRNAs and are converted into single-stranded mature miRNAs with the help of a helicase. These mature miRNAs are then sorted into multivesicular bodies (MVBs), which are transported along microtubules to the plasma membrane and released as exosomes. These exosomes, containing specific miRNAs from the parent cell, can interact with recipient cells through various mechanisms including fusion through clathrin-dependent or independent endocytosis, caveolae-mediated endocytosis, or lipid raft-dependent endocytosis. Once inside the recipient cell, the exosomal miRNAs may repress their target genes.
CircRNAs are non-coding RNA molecules that originate from the back-splicing of pre-mRNA and have a closed-loop structure. This configuration allows them to resist degradation by exonucleases, enabling their presence in different subcellular compartments. Due to their involvement in critical biological activities such as gene regulation, protein production, immune response, and cancer development, circRNAs play a significant role in numerous cellular functions and contribute to drug resistance (Wang et al., 2020a). The main focus of research on circRNAs is their ability to diminish the impact on target mRNAs by acting as miRNA decoys, ultimately leading to changes in correlated gene expression. These circRNAs typically contain numerous miRNA binding sites. Additionally, circRNAs play a role in various biological processes by modulating the functions of proteins (Zhang et al., 2021b). Moreover, studies have revealed that EIciRNAs play a role in promoting the transcription of their source genes by interacting with U1 small nuclear ribonucleoproteins and facilitating RNA polymerase II activity. Additionally, it has been shown that CircURI1 plays a crucial role in regulating alternative splicing of numerous genes associated with cell migration by directly binding with hnRNPM, ultimately suppressing the metastatic progression of gastric cancer. Furthermore, some endogenous circRNAs containing open reading frames have been observed to undergo translation, resulting in the production of peptides or proteins (Shoda et al., 2022). However, their potential functions are still unclear.
Much interest has arisen with regard to circRNAs potentially having a role in tumor formation and tumor evolution. Research conducted on certain forms of lung cancer has reaffirmed the crucial role circRNAs play in the disease (Gao L. et al., 2020; Pandey et al., 2020). The main ideas with regard to tumorigenic mechanisms revolve around the disruption of the normal cell cycle and the emergence of greater proliferative power. The dynamic of CDKs and cyclins during this process has been determined to be a critical factor in the regulation of the cell cycle (Arcinas et al., 2019). As of late, it has been identified that certain circRNAs may support the biological activities of cyclin-dependent kinases (CDKs) or cyclins in lung cancer (You et al., 2015). As an illustration, circPVT1 interferes with the expression of miR-30d and miR-30e to quicken the transcription of cyclin F mRNA, causing the increased production of cyclin F that encourages the advancement of LUSC cells (Stoll et al., 2020). In contrast, circRNA cESRP1 impacts the cell cycle by attaching to miR-93–5p; this in turn promotes the activity of p21, thereby blocking the activity of CDKs (Zhao Q. et al., 2020). Additionally, an earlier investigation found that circRACGAP1 preserved the growth of NSCLC cells through its capacity to absorb miR-144–5p; this also enabled circRNA to directly bind linear mRNA, resulting in the modulation of CDKL1 expression (Hanan et al., 2017).
An array of data suggests that aberrant expression of circRNAs may participate in the development and spread of lung cancer. Evidence points to circPTK2 which appears to interact with miR-429/miR-200b-3p to boost EMT through TIF1γ, posing a higher risk of NSCLC cells (Zhang H. et al., 2018).
The expression of Circ-ABCB10 is augmented in non-small cell lung cancer (NSCLC) cell lines, and knocking down the circ-ABCB10 increases microRNA miR-1252 while reducing levels of Forkhead box 2 (FOXR2), resulting in decreased migration of the NSCLC cells (Huang W. et al., 2020). The Circ-MAN2B2 gene has been shown to increase lung cancer cell invasion through its activation of the miR-1275/FOXK1 pathway (Qu et al., 2018). Circ-0067934 has been reported to have elevated expression concerning the capacity of cancer cells to migrate and infiltrate, together with increased expression of several proteins related to Epithelial-Mesenchymal Transition (EMT) processes, such as N-cadherin (Xue et al., 2019). Circular RNA cESRP1 acts as a sponge for miR-93-5p, leading to reduced levels of Smad 7/p21(CDKN1A), thus preventing TGF-β-driven EMT from progressing in lung cancer (Wei et al., 2019). Circ-BANP amplifies the mobility, incursion, and augmented manifestation of LARP1 in lung cancer cells using the enhancement of miR-93-5p and the decrease of miR-503 (Liu G. et al., 2019). The level of the circulatory gene CircAGFG1 is higher in Non-Small Cell Lung Cancer cells and it increases the process of invasion, migration, and switching between epithelial and mesenchymal forms through the combined action of circ-AGFG1, miR-203, and ZNF281 (Tan et al., 2020). It appears that Circ-PVT1 has a marked presence in LUAC cell lines and tissues, indicating its correlation with CDDP and MTA. It is speculated that Circ PVT1 is responsible for transporting resistance to CDDP and MTA through the miR-145-5p/ABCC1 axis and that its deficiency may magnify tumor cells’ susceptibility to these chemotherapeutics (Mao and Xu, 2020). Mao and his colleagues showed that CDR1-AS is also increased in LUAC tissues and cells, promoting patient immunity to PTX and CDDP using the EGFR/PI3K path (Kong, 2020). The expression of cESRP1 is considerably lower in chemoresistant cells, which increases the cells’ sensitivity to drugs by interacting with miR-93-5p in small cell lung cancer, thereby raising the levels of CDKN1A and averting the transformations caused by transforming growth factor-β using miR-93-5p (Wei et al., 2019).
MAPK pathways are vital for regulating diverse cellular activities, including the stimulation of cell proliferation, differentiation, and cell death (Fang and Richardson, 2005; Guo Y. J. et al., 2020). These pathways involve the MAPK/ERK family, BMK-1, JNK, and p38 pathways as part of the classical route (Sun et al., 2015). Chen et al. identified that hsa_circ_0007580 was an increased circRNA in lung cancer and affected the MAPK signaling pathways. This complex mechanism instigated miR-545-3P to produce a sponge effect on PKCA, thus stimulating NSCLC cell growth and invasion by igniting the p38/MAPK signaling pathway (Chen et al., 2020). In a xenograft tumor model, hsa_circ_0007580 which had a lower expression level impeded the growth of NSCLC tumors by switching off the p38/MAPK pathway. Additionally, circ_0074027 augmented the malignant character of NSCLC cells by absorbing miR-185-3p and amplifying the amount of bromodomain-containing protein 4 (BRD4) and MAPK-activating death domain-containing protein (MADD) (Gao P. et al., 2020). A study conducted by Zhang and their colleagues determined that hsa_circRNA_101237 enhanced MAPK1 expression through its association with miRNA-490-3p, which then had a direct influence on the proliferation, migration, and invasion of non-small cell lung cancer cells. This is indicative of hsa_circRNA_101237s role as a significant one-circRNA (Zhang Z. Y. et al., 2020). Wang et al. observed that circ-ZKSCAN1 can absorb the cancer-causing miR-330-5p, leading to an increase in Family with Sequence Similarity 83 (FAM83)-member A, hindering the MAPK signal transduction pathway and stimulating non-small cell lung cancer (Wang Y. et al., 2019). There was a great reduction in the amount of JNK, p38, and ERK when there was an excessive amount of circZKSCAN1 present in NSCLC cells (Wang Y. et al., 2019). The results suggested that, in addition to circ-ZKSCAN1, which inhibited MAPK signal transduction and had a carcinogenic effect, other circRNAs could possibly promote lung cancer growth through the activation of MAPK signaling.
The generation of circular protein kinase C iota (CircPRKCI) from exons 15 and 16 of the PRKCI gene (chr3:170013698-170015181) at the 3q26.2 amplicon has been linked to tumor progression in lung adenocarcinoma (LAD). Studies have produced evidence of a positive correlation between the presence of CircPRKCI and high T stage and TNM stage in LAD patients (Qiu et al., 2018). The efficacy of circPRKCI in therapy was tested using the PDTX model in nude mice. This was done by introducing cholesterol-conjugated si-circPRKCI via an intratumoral injection and observing the consequences. There was a decrease in tumor size and mass in the si-circPRKCI group compared to the control group. This finding brings into focus the potential of circPRKCI for the treatment of tumors (Qiu et al., 2018). In the study, researchers looked at how effective different combinations of EGFR-TKIs and knockdown of circPRKCI (a protein that regulates EGFR) were in treating NSCLC patients. They found that the combination of EGFR-TKIs and knockdown of circPRKCI had a more notable inhibitory effect on cancer progression than gefitinib or knockdown of circPRKCI alone. This suggests that a combination of EGFR-TKIs and attenuation of circPRKCI may have a synergistic effect on reducing cancer progression (Qiu et al., 2018). Table 3, lists various circular RNAs that are involved in lung cancer.
Various research has emphasized the significance of exosomes in both the wellbeing and ailment of humans. Furthermore, more recent investigations have showcased the ability of exosomes to perform their functions through impacting immune response, oxidative stress, autophagy, gut microbiota, and cell cycle (Li C. et al., 2021). Recent research has shown that exosomal ncRNAs are closely linked to the advancement of various human cancers, such as lung cancer. These particular ncRNAs present in exosomes contribute to crucial processes in cancer development, such as EMT, cell growth, formation of new blood vessels (angiogenesis), spread of cancer to other parts of the body (metastasis), resistance to treatment, and immune-inflammatory responses (Hu et al., 2020).
In the field of cell biology, three categories of extracellular vesicles are distinguished by their size and the process involved in their liberation: apoptotic bodies (larger than 1,000 nm), microvesicles (measuring between 100 nm and 1,000 nm), and exosomes (whose length varies between 30 nm and 150 nm) (Shen et al., 2023). Exosomes are small vesicles that resemble a saucer in shape, having two lipid layers. They play a vital role in communication and maintaining balance between cells by transporting their contents from 1 cell to another (Ruzycka-Ayoush et al., 2023). Cell surfaces are specifically targeted by adhesion molecules and tetraspanin complexes (Rana et al., 2012). The SNAREs complex and ESCRT machinery promote the merger of cellular components, using four diverse proteins (sequentially called ESCRT type 0-III) to produce exosomes in a crucial capacity (Henne et al., 2013).
Exosomes can be identified in multiple types of bodily fluids such as blood, urine, breast milk, and bronchoalveolar lavage fluid (Ruzycka-Ayoush et al., 2023). The results of the cell vesicular trafficking process, typically beginning with the fusion of two membranous objects in late endosomes, are products of this machinery (Hessvik and Llorente, 2018). The biogenesis of exosomes progresses through three stages: initially the plasma membrane inward folds creating an early endosome; subsequently, the endosome membrane bulges out forming multiple vesicular bodies (MVBs); and finally, the late endosome fuses with the plasma membrane and expelling MVBs (Batista et al., 2011).
The production of exosomes is a carefully controlled activity which is impacted by the cell type, the environment, and other cells. For instance, mesenchymal stem cells will produce substantially more exosomes than immature dendritic cells. Furthermore, when surrounded by a stressful environment like hypoxia, the release of exosomes increases. It is also known that cells which have contact inhibition and those that are resting will have much lesser amounts of exosomes released (Hayes et al., 2005; Gurunathan et al., 2019). It has been demonstrated that cells with higher division rates, such as cancerous cells, produce and release more exosomes (Liu S.-L. et al., 2019).
Exosomes, which are enclosed in membranes, are found in a variety of biological liquids. These vesicles serve as key communicators between cells and controllers of biological processes. Their loads are complex, consisting of proteins, lipids, DNA, mRNA, and miRNA (Jabalee et al., 2018). One of the most interesting and widely examined molecules are miRNAs, which are short, internal, non-coding RNAs. Their role in regulating gene expression after transcription and translation is remarkable and has attracted considerable attention (Zhang J. et al., 2015; Jabalee et al., 2018) (Figure 4). Evidence suggests that miRNA exchange among tumor and stromal cells within the tumor microenvironment, especially with regards to lung cancer, could be responsible for the initiation and development of the cancer (Adi Harel et al., 2015; Liu Y. et al., 2016; Sun et al., 2018; Xu et al., 2018; Zhang et al., 2019a).
Angiogenesis, which is essential for the expansion and spread of tumors, is regulated by exosomes that are released by different cell types and act as messengers between cells (Zhang L. et al., 2015). A recent study suggested that exosomal miR-9 may encourage blood vessel growth through the activation of the JAK/STAT signaling pathway (Zhuang et al., 2012). The tissue inhibitor of metalloproteinases-1 (TIMP-1) increased exosomal miR-210 originating from lung adenocarcinoma (LUAD) samples, leading to the promotion of angiogenesis in stromal cells (Cui et al., 2015). Liu et al. also found that miR-21, which is located within exosomes, activated signal transducer and activator of transcription (STAT) 3, which then led to enhanced expression of vascular endothelial growth factor (VEGF) and the transformation of normal human bronchial epithelial cells (HBECs) into malignant cells (Liu Y. et al., 2016). At the end, it was shown that exosomal miR-23a obtained from lung cancer cells had the capability of boosting tumour vascularization in environments with either normal or low oxygen, suggesting that lung cancer cells are capable of passing on genetic material to remote endothelial cells (Hsu et al., 2017).
Immune checkpoint proteins are extremely important in controlling the immune system in order to keep the body in balance and keep it from attacking itself (Smolle et al., 2019). Treating advanced NSCLC patients with medications which focus on the immune checkpoint molecules PD-1 and PD-L1 has been linked to greater lifespans (Arbour and Riely, 2019). Research has revealed that miRNAs can act as a network to regulate processes associated with immune checkpoint pathways. One example is miR-34, which is managed by p53 and directly binds to the PD-L1 3′UTR, ultimately suppressing its presence in non-small cell lung cancer models (Cortez et al., 2016). The function of MiR-200 was additionally found to regulate the expression of PD-L1 (Chen et al., 2014).
More and more research suggests that exosomes play a role in cancer growth by transferring molecules and substances that weaken the body’s defences (Greening et al., 2015). MiRNAs that are packaged into exosomes are pivotal in modulating the activities of various immune cells, particularly dendritic cells and T-lymphocytes, in the context of cancer (Sun et al., 2018). For instance, as mentioned above, exosomes from lung cancer cells have been seen to transfer miR-21/29a to set off TLR7 and TLR8 on immune cells, which could be linked to cancer proliferation and metastasis (Fabbri et al., 2012). Yang et al. found that miR-214 was transferred from human cancer cells, including those related to lung cancer, to recipient CD4+ T cells, mediated by exosomes. The result of this was a decrease in PTEN expression and an increased rate of Treg expansion, as well as an increased tumour growth (Yin et al., 2014).
PTEN has an anti-tumor effect on multiple types of cancer, achieved through its ability to inhibit the PI3K/Akt signaling pathway (Tang et al., 2020; Li et al., 2021b). Conversely, EZH2 is capable of attaching to the promoter region of genes like PTEN to alter their expression levels (Pappas et al., 2021). It has been observed that the exosomal long non-coding ribonucleic acid UFC1 presents a hindrance to apoptosis and the termination of the cell cycle in lung cancer cells, and its presence is correlated with a marked rise in cellular proliferation and metastasis. This effect is thought to take place through UFC1’s interference with enhancer of zeste homolog 2 (EZH2), which consequently causes PTEN expression to drastically diminish, thus prompting the forming of conditions necessary for the manifestation of non-small-cell lung cancer (Zang et al., 2020). In addition, lncRNAs that are carried in exosomes can cause lung cancer to become resistant to drugs. Specifically, the lncRNA RP11–838N2.4 can be found in exosomes, thus reducing the effectiveness of erlotinib chemotherapy. Interestingly, the FOXO1 protein has been observed to attach to the promoter of RP11–838N2.4, thereby recruiting histone deacetylases that lessen the expression of RP11–838N2.4 and consequently raise the sensitivity of the cancer to erlotinib chemotherapy (Zhang W. et al., 2018). Comparatively, the exosomal lncRNA GAS5 has been established to act as a tumor-suppressor, but its amounts are substantially lower in individuals with NSCLC. Regrettably, the overexpression of GAS5 can cause lymph node metastasis in advanced stages, resulting in a grim prognosis (Li et al., 2019). In conclusion, DLX6-AS1 and SNHG15, two exosomal lncRNAs with tumor-promoting properties, are found to be expressed at higher levels in lung cancer patients, resulting in a worse prognosis (Table 4) (Zhang et al., 2019b; Stamm et al., 2021).
Exosomal circRNAs are pivotal in multiple biological functions which can either stimulate or restrict cancer development (Huang X. Y. et al., 2020; Yang et al., 2021). Evidence is mounting that exo-circRNAs have an essential part to play in a range of malignancies, including lung cancer, through various pathways. It has been proposed that exosomal circRNAs function similarly in cancerous cells, where they act like miRNA sponges (Wang et al., 2020b). When epithelial cells become able to move, the process known as EMT occurs and they transform to a mesenchymal state while still having the capability to be invasive (Kalluri and Weinberg, 2009). Many biological occurrences, like the development of an embryo, the forming of scar tissue, the spread of cancer, and metastasis, have been studied by using this approach (Prieto-García et al., 2017). The EMT process can cause LC, a type of malignant tumor, to spread and invade surrounding tissue just as other malignant tumors do (Bakir et al., 2020). A substantial concentration of circRNAs is evident in lung cancer, and certain of them demonstrate cancer-causing roles by spurring EMT activities in vitro (Figure 5). Take for instance, the suppression of microRNA-137 by circ-LDLRAD3 augmented the proliferation and EMT through the elevation of glutamine transporter, a portion of the SLC1A5 family, in lung cancer cells (Xue et al., 2020). It was discovered that deactivating SLC1A5, an essential element in the formation and maintenance of LC cells, significantly reduced their survivability (Hassanein et al., 2013). The circulatory function of circ 0012673 may be responsible for the spread and entry of LUADs (Qin et al., 2020). Decreasing the amounts of circ 0012673 interfered with cell development, motility, and EMT by boosting LIM domain kinase 1 in lung adenocarcinoma cell lines, which also caused apoptosis mediated by miR-320a in targeting (Qin et al., 2020). Li et al. discovered that elevating the amount of hsa circ 0079530 can stimulate cancer cells to permeate and proliferate through controlling EMT activities (Li J. et al., 2018).
Figure 5. Exosomal circRNA molecules play an important role in the EMT process in lung cancer cells. Circular RNA molecules found in exosomes, which are elevated in levels and contribute to cancer-causing actions, facilitate the process of EMT by stimulating or hindering various pathways in lung cancer. This figure adapted from (Hussen et al., 2022).
Piwi-interacting RNAs (piRNAs) are a newly identified type of small non-coding RNAs (sncRNAs), ranging from 24 to 31 nucleotides (nt) in length, that have a crucial role in cancer diagnosis by affecting gene expression. These molecules bind to the PIWI protein family and act as gene regulators, controlling transcriptional activity, preserving the function of germline and stem cells, and regulating translation and mRNA stability (Li et al., 2021d).
Li and colleagues indicated the abnormally manifested piRNAs in lung adenocarcinoma (LUAD), and suggested that the serum exosomal piR-hsa-26925 and piR-hsa-5444 may have promising utility as diagnostic biomarkers for LUAD (Li et al., 2021d). In their analysis, it was determined that 76 piRNAs displayed elevated expression while 9 piRNAs showed reduced expression in LUAD tissues compared to non-tumor tissues. The 10 most noteworthy overexpressed piRNAs were selected and upon conducting qRT-PCR, it was confirmed that 4 of them (piR-hsa-26925, piR-hsa-5444, piR-hsa-30636, and piR-hsa-8757) were significantly upregulated in LUAD tissues. Furthermore, the levels of piR-hsa-26925 and piR-hsa-5444 were significantly higher in the serum exosome samples of LUAD patients compared to those of healthy controls. To conclude, they developed a 2-piRNA panel composed of piR-hsa-26925 and piR-hsa-5444, which displayed superior diagnostic accuracy for LUAD with an AUC value of 0.833 (Li et al., 2021d).
In order to successfully detect NSCLC at an early stage, Li and colleagues conducted a thorough investigation of serum-based extracellular vesicle piRNA in order to identify potential diagnostic indicators for the disease (Li Y. et al., 2022). Researchers used a high-throughput sequencing method to identify potential piRNA biomarkers in cancerous and noncancerous tissues of patients with NSCLC. These piRNAs were then tested in a sample group of 115 patients (including 95 in stage I) and 47 healthy individuals through a quantitative real-time PCR. They found that piR-hsa-164586 was significantly increased compared to noncancerous tissues and extracellular vesicles from healthy individuals’ serum samples. Its diagnostic value was also validated, with an area under the curve (AUC) of 0.623 and 0.624 for distinguishing all stages of NSCLC and stage I specifically from healthy individuals. Compared to a commonly used biomarker, CYFRA21-1, piR-hsa-164586 showed better diagnostic performance. The piR-hsa-164586 was also correlated with clinical characteristics of NSCLC patients, such as age and TNM stage, making it a promising biomarker for early detection of NSCLC (Li Y. et al., 2022).
SnoRNAs, which are RNA molecules that do not code for proteins, are found in the nucleolus where they play a role in altering and cutting ribosomal RNAs. They were first discovered in the 1960s and extensive research has been conducted to understand their creation and functions. Although their involvement in cancer has only recently been uncovered, studies have revealed that snoRNAs play a significant role in lung cancer. By analyzing the expression of snoRNAs, researchers have been able to define snoRNA-related patterns not only in tissues but also in bodily fluids, suggesting their potential as non-invasive biomarkers. Furthermore, snoRNAs have been found to be crucial in the initiation and spread of lung cancer, influencing a variety of cellular processes such as cell growth and death, and promoting cancer cell adaptability. They possess both cancer-causing and tumor-suppressing qualities that are essential in the development and advancement of lung cancer (Mourksi et al., 2020).
Researching snoRNAs in relation to lung cancer has the potential to lead to novel methods for clinical use, including their use as both biomarkers and targets for treatment. The unique biochemical characteristics of snoRNAs make them an excellent candidate for non-invasive biomarkers in the context of lung cancer. These molecules can be found in exosomes or released from dying cells into bodily fluids such as sputum, making them easily accessible for diagnostic purposes (Su et al., 2016) or plasma (Liao et al., 2010). SnoRNAs can be easily and consistently identified without the need for invasive methods, allowing for their detection in a stable manner. If snoRNA patterns are precisely identified throughout the progression of the illness, this can serve as a significant indicator of both the subtype and the severity of the illness, as well as potentially predicting future relapses (Gong et al., 2017). The identification of new markers will largely depend on the use of RNA-sequencing methods, however, challenges related to the classification of various snoRNA annotations and determining snoRNA identity based on a single 10-20 nt sequence remain to be resolved.
Gao and colleagues (Gao et al., 2015) proposed using RNA-seq to profile small nucleolar RNAs (snoRNAs) in lung cancer. This technology allowed them to analyze 458 mature snoRNAs in 12 pairs of normal and tumor lung tissue from stage I NSCLC patients. They found that 29 snoRNAs, including SNORA71A, were significantly overexpressed (at least 3-fold higher) in tumor tissue compared to normal biopsies. In a separate study, Wang and colleagues investigated the effectiveness of plasma small nucleolar RNAs in diagnosing NSCLC at an early stage. Based on databases, they selected SNORD83A for further analysis and confirmed its expression in both formalin-fixed, paraffin-embedded tissues from 48 paired NSCLC patients, as well as in plasma from 150 NSCLC patients and 150 healthy individuals. Receiver operating characteristic analysis revealed that SNORD83A, alone or in combination with carcinoembryonic antigen, had a high diagnostic efficiency for NSCLC. The levels of SNORD83A were significantly elevated not only in tissues, but also in plasma from NSCLC patients compared to healthy individuals. This indicates that plasma SNORD83A could serve as a diagnostic biomarker for NSCLC. When combined with carcinoembryonic antigen, the diagnostic efficiency for early-stage NSCLC was significantly improved (Wang K. et al., 2022).
To sum up, it has been revealed that lung cancer is an intricate disorder with many unknown regulators. ncRNAs can influence gene expression which in turn can increase or decrease the risk of lung cancer. Examples of ncRNAs that affect these pathways are microRNAs (miRNAs), circRNAs, piRNAs, and lncRNAs. In certain instances, these molecules, such as lnc-JPX, can exercise their influence on multiple pathways. ncRNAs can either be induced or inhibited, which can be utilized to diagnose the early onset of lung cancer, establish the occurrence of metastasis, or foresee recurrent cancer after surgery. Furthermore, certain ncRNAs can either resist or restore sensitivity to medication. For example, miR-21 is known to promote the formation of tumors and occurs in many targets. Nevertheless, the exact mechanisms of action for many of the molecules remain unclear. Some potential future directions for research on ncRNAs in cancer include: 1) Identification and validation of ncRNA biomarkers: Researchers are actively searching for specific ncRNA biomarkers that could help with early detection, diagnosis, and prognostication of cancer. For example, miRNAs have shown promise as potential biomarkers for breast, colorectal, and lung cancer, and other ncRNAs like lncRNAs and circRNAs are also being studied as potential biomarkers. Scientists are currently working on large-scale studies to validate these biomarkers and determine their clinical usefulness. 2) Understanding the role of ncRNAs in cancer progression: Many ncRNAs have been found to play critical roles in cancer progression by promoting tumor growth, invasion, and metastasis. Therefore, further research is needed to fully understand the mechanisms of how these ncRNAs contribute to cancer development and progression, which could lead to the identification of new therapeutic targets. 3) Development of therapeutic strategies targeting ncRNAs: ncRNAs are potential targets for cancer therapy, and there are various methods being explored. One such approach involves using antisense oligonucleotides (ASOs) or small interfering RNAs (siRNAs) to target and degrade specific ncRNAs. Other methods include using small molecules or natural compounds to inhibit the function of specific ncRNAs. Additionally, researchers are exploring the use of CRISPR-Cas9 gene editing to modify ncRNA expression in cancer cells. 4) Combination therapy with ncRNA-targeting agents: Some researchers are investigating the potential of combining ncRNA-targeting agents with other cancer treatments, such as chemotherapy, radiation therapy, or immunotherapy. This combination therapy could potentially overcome resistance to existing cancer treatments and improve patient outcomes.
Recent findings suggest that EVs, specifically exosomal ncRNAs, have been a significant focus of research in lung cancer development in recent years. These EVs contain numerous RNAs that can play a role in oncogenic transfer, angiogenesis, immune modulation, and premetastatic niche formation. Among these EV components, microRNAs and lncRNAs have been shown to dysregulate the expression of molecules involved in epithelial-mesenchymal transition (EMT) and angiogenesis in recipient cells by interacting with signaling pathways. Furthermore, these “harmful” RNAs can also disrupt the microenvironment in secondary organs, leading to increased tumorigenic heterogeneity and the formation of premetastatic niches. Conversely, immune cell-derived exosomal RNAs have a modest impact on the composition and activation of T cells, B cells, and other immune cells, ultimately promoting therapeutic resistance in tumor cells. These EVs also play a crucial role in the maintenance of non-cancer stem cells (CSCs) and CSCs, and further research is needed to fully understand their function in tumors. They have potential as both diagnostic markers and therapeutic targets, allowing for personalized medicine to be developed based on their specific characteristics. However, the challenge of therapeutic resistance during cancer treatment remains a hurdle. Additionally, more research is needed to fully understand the effects and regulation of EV-derived RNAs, as well as ensure the safety and quality of new methods for their isolation and use. As we continue to gain a better understanding of EVs, more emphasis will likely be placed on in vivo models and clinical applications to further address these questions.
XL: Data curation, Investigation, Methodology, Project administration, Resources, Visualization, Writing–original draft. LY: Data curation, Investigation, Methodology, Project administration, Resources, Writing–original draft. YX: Data curation, Formal Analysis, Investigation, Methodology, Project administration, Validation, Visualization, Supervision, Writing–original draft. MM: Conceptualization, Data curation, Supervision, Funding acquisition, Investigation, Methodology, Resources, Supervision, Writing–original draft, Writing–review and editing.
The author(s) declare that no financial support was received for the research, authorship, and/or publication of this article.
The authors declare that the research was conducted in the absence of any commercial or financial relationships that could be construed as a potential conflict of interest.
All claims expressed in this article are solely those of the authors and do not necessarily represent those of their affiliated organizations, or those of the publisher, the editors and the reviewers. Any product that may be evaluated in this article, or claim that may be made by its manufacturer, is not guaranteed or endorsed by the publisher.
Adi Harel, S., Bossel Ben-Moshe, N., Aylon, Y., Bublik, D. R., Moskovits, N., Toperoff, G., et al. (2015). Reactivation of epigenetically silenced miR-512 and miR-373 sensitizes lung cancer cells to cisplatin and restricts tumor growth. Cell Death Differ. 22 (8), 1328–1340. doi:10.1038/cdd.2014.221
Ajani, J. A., Song, S., Hochster, H. S., and Steinberg, I. B. (2015). Cancer stem cells: the promise and the potential. Semin. Oncol. 42 (Suppl. 1), S3–S17. doi:10.1053/j.seminoncol.2015.01.001
Arbour, K. C., and Riely, G. J. (2019). Systemic therapy for locally advanced and metastatic non-small cell lung cancer: a review. Jama 322 (8), 764–774. doi:10.1001/jama.2019.11058
Arcinas, C., Tan, W., Fang, W., Desai, T. P., Teh, D. C. S., Degirmenci, U., et al. (2019). Adipose circular RNAs exhibit dynamic regulation in obesity and functional role in adipogenesis. Nat. Metab. 1 (7), 688–703. doi:10.1038/s42255-019-0078-z
Bakir, B., Chiarella, A. M., Pitarresi, J. R., and Rustgi, A. K. (2020). EMT, MET, plasticity, and tumor metastasis. Trends Cell Biol. 30 (10), 764–776. doi:10.1016/j.tcb.2020.07.003
Batista, B. S., Eng, W. S., Pilobello, K. T., Hendricks-Muñoz, K. D., and Mahal, L. K. (2011). Identification of a conserved glycan signature for microvesicles. J. Proteome Res. 10 (10), 4624–4633. doi:10.1021/pr200434y
Baumgartner, U., Berger, F., Hashemi Gheinani, A., Burgener, S. S., Monastyrskaya, K., and Vassella, E. (2018). miR-19b enhances proliferation and apoptosis resistance via the EGFR signaling pathway by targeting PP2A and BIM in non-small cell lung cancer. Mol. Cancer 17 (1), 44. doi:10.1186/s12943-018-0781-5
Bhan, A., Soleimani, M., and Mandal, S. S. (2017). Long noncoding RNA and cancer: a new paradigm. Cancer Res. 77 (15), 3965–3981. doi:10.1158/0008-5472.CAN-16-2634
Borkowski, R., Du, L., Zhao, Z., McMillan, E., Kosti, A., Yang, C. R., et al. (2015). Genetic mutation of p53 and suppression of the miR-17∼92 cluster are synthetic lethal in non-small cell lung cancer due to upregulation of vitamin D Signaling. Cancer Res. 75 (4), 666–675. doi:10.1158/0008-5472.CAN-14-1329
Boyerinas, B., Park, S. M., Hau, A., Murmann, A. E., and Peter, M. E. (2010). The role of let-7 in cell differentiation and cancer. Endocrine-Related Cancer 17 (1), F19–F36. doi:10.1677/ERC-09-0184
Braicu, C., Zimta, A. A., Harangus, A., Iurca, I., Irimie, A., Coza, O., et al. (2019). The function of non-coding RNAs in lung cancer tumorigenesis. Cancers 11 (5), 605. doi:10.3390/cancers11050605
Brambilla, E., and Gazdar, A. (2009). Pathogenesis of lung cancer signalling pathways: roadmap for therapies. Eur. Respir. J. 33 (6), 1485–1497. doi:10.1183/09031936.00014009
Brosnan, C. A., and Voinnet, O. (2009). The long and the short of noncoding RNAs. Curr. Opin. Cell Biol. 21 (3), 416–425. doi:10.1016/j.ceb.2009.04.001
Cai, J., Zheng, Y., Dong, L., Zhang, X., and Huang, Y. (2022b). Circular RNA hsa_circ_0018189 drives non-small cell lung cancer growth by sequestering miR-656-3p and enhancing xCT expression. J. Clin. Lab. Anal. 36 (11), e24714. doi:10.1002/jcla.24714
Cai, Y., Dong, Z., and Wang, J. (2022a). Circ_0000808 promotes the development of non-small cell lung cancer by regulating glutamine metabolism via the miR-1827/SLC1A5 axis. World J. Surg. Oncol. 20 (1), 329. doi:10.1186/s12957-022-02777-x
Calin, G. A., Sevignani, C., Dumitru, C. D., Hyslop, T., Noch, E., Yendamuri, S., et al. (2004). Human microRNA genes are frequently located at fragile sites and genomic regions involved in cancers. Proc. Natl. Acad. Sci. U. S. A. 101 (9), 2999–3004. doi:10.1073/pnas.0307323101
Cao, G., Fan, P., Ma, R., Wang, Q., Niu, H., He, L., et al. (2023). MiR-210 regulates lung adenocarcinoma by targeting HIF-1α. Heliyon 9 (5), e16079. doi:10.1016/j.heliyon.2023.e16079
Cao, X., Zhong, W., Guo, S., Zhang, Z., and Xie, C. (2022). Low expression of miR-27b in serum exosomes of non-small cell lung cancer facilitates its progression by affecting EGFR. Open Med. (Wars) 17 (1), 816–825. doi:10.1515/med-2022-0472
Chan, L. W., Wang, F. F., and Cho, W. C. (2012). Genomic sequence analysis of EGFR regulation by microRNAs in lung cancer. Curr. Top. Med. Chem. 12 (8), 920–926. doi:10.2174/156802612800166747
Chang, R. M., Fu, Y., Zeng, J., Zhu, X. Y., and Gao, Y. (2022). Cancer-derived exosomal miR-197-3p confers angiogenesis via targeting TIMP2/3 in lung adenocarcinoma metastasis. Cell Death Dis. 13 (12), 1032. doi:10.1038/s41419-022-05420-5
Chen, H., Wang, L., Liu, J., Wan, Z., Zhou, L., Liao, H., et al. (2023b). LncRNA ITGB2-AS1 promotes cisplatin resistance of non-small cell lung cancer by inhibiting ferroptosis via activating the FOSL2/NAMPT axis. Cancer Biol. Ther. 24 (1), 2223377. doi:10.1080/15384047.2023.2223377
Chen, J., Liu, X., Xu, Y., Zhang, K., Huang, J., Pan, B., et al. (2019b). TFAP2C-Activated MALAT1 modulates the chemoresistance of docetaxel-resistant lung adenocarcinoma cells. Mol. Ther. Nucleic Acids 14, 567–582. doi:10.1016/j.omtn.2019.01.005
Chen, J., Zhou, D., Liao, H., and Li, Y. (2023a). miR-183-5p regulates ECM and EMT to promote non-small cell lung cancer progression by targeting LOXL4. J. Thorac. Dis. 15 (4), 1734–1748. doi:10.21037/jtd-23-329
Chen, L., Gibbons, D. L., Goswami, S., Cortez, M. A., Ahn, Y. H., Byers, L. A., et al. (2014). Metastasis is regulated via microRNA-200/ZEB1 axis control of tumour cell PD-L1 expression and intratumoral immunosuppression. Nat. Commun. 5, 5241. doi:10.1038/ncomms6241
Chen, P., Zhao, Y., and Li, Y. (2017a). MiR-218 inhibits migration and invasion of lung cancer cell by regulating Robo1 expression. Zhongguo Fei Ai Za Zhi 20 (7), 452–458. doi:10.3779/j.issn.1009-3419.2017.07.03
Chen, Q. N., Chen, X., Chen, Z. Y., Nie, F. Q., Wei, C. C., Ma, H. W., et al. (2017b). Long intergenic non-coding RNA 00152 promotes lung adenocarcinoma proliferation via interacting with EZH2 and repressing IL24 expression. Mol. Cancer 16 (1), 17. doi:10.1186/s12943-017-0581-3
Chen, Q. W., Cai, Q. Q., Yang, Y., Dong, S., Liu, Y. Y., Chen, Z. Y., et al. (2023c). LncRNA BC promotes lung adenocarcinoma progression by modulating IMPAD1 alternative splicing. Clin. Transl. Med. 13 (1), e1129. doi:10.1002/ctm2.1129
Chen, S., Lu, S., Yao, Y., Chen, J., Yang, G., Tu, L., et al. (2020). Downregulation of hsa_circ_0007580 inhibits non-small cell lung cancer tumorigenesis by reducing miR-545-3p sponging. Aging (Albany NY) 12 (14), 14329–14340. doi:10.18632/aging.103472
Chen, S. W., Zhu, S. Q., Pei, X., Qiu, B. Q., Xiong, D., Long, X., et al. (2021b). Cancer cell-derived exosomal circUSP7 induces CD8(+) T cell dysfunction and anti-PD1 resistance by regulating the miR-934/SHP2 axis in NSCLC. Mol. Cancer 20 (1), 144. doi:10.1186/s12943-021-01448-x
Chen, T., Feng, G., Xing, Z., and Gao, X. (2022a). Circ-EIF3I facilitates proliferation, migration, and invasion of lung cancer via regulating the activity of Wnt/β-catenin pathway through the miR-1253/NOVA2 axis. Thorac. Cancer 13 (22), 3133–3144. doi:10.1111/1759-7714.14665
Chen, T., Liu, Y., Chen, J., Zheng, H., Chen, Q., and Zhao, J. (2021a). Exosomal miR-3180-3p inhibits proliferation and metastasis of non-small cell lung cancer by downregulating FOXP4. Thorac. Cancer 12 (3), 372–381. doi:10.1111/1759-7714.13759
Chen, T., Xiao, Q., Wang, X., Wang, Z., Hu, J., Zhang, Z., et al. (2019a). miR-16 regulates proliferation and invasion of lung cancer cells via the ERK/MAPK signaling pathway by targeted inhibition of MAPK kinase 1 (MEK1). J. Int. Med. Res. 47 (10), 5194–5204. doi:10.1177/0300060519856505
Chen, W., Tang, D., Lin, J., Huang, X., Lin, S., Shen, G., et al. (2022b). Exosomal circSHKBP1 participates in non-small cell lung cancer progression through PKM2-mediated glycolysis. Mol. Ther. Oncolytics 24, 470–485. doi:10.1016/j.omto.2022.01.012
Chen, W., Zhao, W., Chen, S., Zhang, L., Guo, Z., Wang, L., et al. (2018). Expression and correlation of MALAT1 and SOX9 in non-small cell lung cancer. Clin. Respir. J. 12 (7), 2284–2291. doi:10.1111/crj.12906
Chen, Y. F., and Xu, A. P. (2023). Circular RNA circDLG1 (has_circ_0068706) functions as an oncogene in nonsmall cell lung cancer through regulating AKT/mTOR signaling and direct binding to miR-144. Kaohsiung J. Med. Sci. 39 (5), 446–457. doi:10.1002/kjm2.12662
Chen, Z., Bian, C., Huang, J., Li, X., Chen, L., Xie, X., et al. (2022c). Tumor-derived exosomal HOTAIRM1 regulates SPON2 in CAFs to promote progression of lung adenocarcinoma. Discov. Oncol. 13 (1), 92. doi:10.1007/s12672-022-00553-7
Clarke, M. F., and Fuller, M. (2006). Stem cells and cancer: two faces of eve. Cell 124 (6), 1111–1115. doi:10.1016/j.cell.2006.03.011
Cortez, M. A., Ivan, C., Valdecanas, D., Wang, X., Peltier, H. J., Ye, Y., et al. (2016). PDL1 Regulation by p53 via miR-34. J. Natl. Cancer Inst. 108 (1), djv303. doi:10.1093/jnci/djv303
Cui, H., Seubert, B., Stahl, E., Dietz, H., Reuning, U., Moreno-Leon, L., et al. (2015). Tissue inhibitor of metalloproteinases-1 induces a pro-tumourigenic increase of miR-210 in lung adenocarcinoma cells and their exosomes. Oncogene 34 (28), 3640–3650. doi:10.1038/onc.2014.300
Dai, J., Qu, T., Yin, D., Cui, Y., Zhang, C., Zhang, E., et al. (2023). LncRNA LINC00969 promotes acquired gefitinib resistance by epigenetically suppressing of NLRP3 at transcriptional and posttranscriptional levels to inhibit pyroptosis in lung cancer. Cell Death Dis. 14 (5), 312. doi:10.1038/s41419-023-05840-x
Ding, C., Xi, G., Wang, G., Cui, D., Zhang, B., Wang, H., et al. (2020). Exosomal circ-MEMO1 promotes the progression and aerobic glycolysis of non-small cell lung cancer through targeting MiR-101-3p/KRAS Axis. Front. Genet. 11, 962. doi:10.3389/fgene.2020.00962
Ding, L., Feng, Y., and Li, L. (2022). Circ_0001955 promotes the progression of non-small cell lung cancer via miR-769-5p/EGFR axis. Cell Cycle 21 (22), 2433–2443. doi:10.1080/15384101.2022.2100681
Dodangeh, F., Sadeghi, Z., Maleki, P., and Raheb, J. (2023). Long non-coding RNA SOX2-OT enhances cancer biological traits via sponging to tumor suppressor miR-122-3p and miR-194-5p in non-small cell lung carcinoma. Sci. Rep. 13 (1), 12371. doi:10.1038/s41598-023-39000-0
Fabbri, M., Paone, A., Calore, F., Galli, R., Gaudio, E., Santhanam, R., et al. (2012). MicroRNAs bind to Toll-like receptors to induce prometastatic inflammatory response. Proc. Natl. Acad. Sci. U. S. A. 109 (31), E2110–E2116. doi:10.1073/pnas.1209414109
Fang, J. Y., and Richardson, B. C. (2005). The MAPK signalling pathways and colorectal cancer. Lancet Oncol. 6 (5), 322–327. doi:10.1016/S1470-2045(05)70168-6
Fang, T., Lv, H., Lv, G., Li, T., Wang, C., Han, Q., et al. (2018). Tumor-derived exosomal miR-1247-3p induces cancer-associated fibroblast activation to foster lung metastasis of liver cancer. Nat. Commun. 9 (1), 191. doi:10.1038/s41467-017-02583-0
Far, B. F., Vakili, K., Fathi, M., Yaghoobpoor, S., Bhia, M., Naimi-Jamal, M. R., et al. (2022). The role of microRNA-21 (miR-21) in pathogenesis, diagnosis, and prognosis of gastrointestinal cancers: a review. Life Sci. 316, 121340. doi:10.1016/j.lfs.2022.121340
Fauré, J., Lachenal, G., Court, M., Hirrlinger, J., Chatellard-Causse, C., Blot, B., et al. (2006). Exosomes are released by cultured cortical neurones. Mol. Cell. Neurosci. 31 (4), 642–648. doi:10.1016/j.mcn.2005.12.003
Feng, C., Zhao, Y., Li, Y., Zhang, T., Ma, Y., and Liu, Y. (2019). LncRNA MALAT1 promotes lung cancer proliferation and gefitinib resistance by acting as a miR-200a sponge. Arch. Bronconeumol Engl Ed. 55 (12), 627–633. doi:10.1016/j.arbres.2019.03.026
Feng, Y., Wu, F., Wu, Y., Guo, Z., and Ji, X. (2023). LncRNA DGUOK-AS1 facilitates non-small cell lung cancer growth and metastasis through increasing TRPM7 stability via m6A modification. Transl. Oncol. 32, 101661. doi:10.1016/j.tranon.2023.101661
Friday, B. B., and Adjei, A. A. (2005). K-ras as a target for cancer therapy. Biochim. Biophys. Acta 1756 (2), 127–144. doi:10.1016/j.bbcan.2005.08.001
Frydrychowicz, M., Kuszel, Ł., Dworacki, G., and Budna-Tukan, J. (2023). MicroRNA in lung cancer—a novel potential way for early diagnosis and therapy. J. Appl. Genet. 64 (3), 459–477. doi:10.1007/s13353-023-00750-2
Gan, J., Zhang, Y., Liu, S., Mu, G., Zhao, J., Jiang, W., et al. (2023). MicroRNA-375 restrains the progression of lung squamous cell carcinoma by modulating the ERK pathway via UBE3A-mediated DUSP1 degradation. Cell Death Discov. 9 (1), 199. doi:10.1038/s41420-023-01499-7
Gao, L., Chang, S., Xia, W., Wang, X., Zhang, C., Cheng, L., et al. (2020a). Circular RNAs from BOULE play conserved roles in protection against stress-induced fertility decline. Sci. Adv. 6 (46), eabb7426. doi:10.1126/sciadv.abb7426
Gao, L., Ma, J., Mannoor, K., Guarnera, M. A., Shetty, A., Zhan, M., et al. (2015). Genome-wide small nucleolar RNA expression analysis of lung cancer by next-generation deep sequencing. Int. J. cancer 136 (6), E623–E629. doi:10.1002/ijc.29169
Gao, P., Wang, Z., Hu, Z., Jiao, X., and Yao, Y. (2020b). Circular RNA circ_0074027 indicates a poor prognosis for NSCLC patients and modulates cell proliferation, apoptosis, and invasion via miR-185-3p mediated BRD4/MADD activation. J. Cell Biochem. 121 (3), 2632–2642. doi:10.1002/jcb.29484
Gao, W., Yang, N., Yin, C., Zeng, Y., and Zhu, X. (2022). Engineered exosomes loaded with miR-563 inhibit lung cancer growth. J. Oncol. 2022, 6141857. doi:10.1155/2022/6141857
Gao, X., Yang, X., He, F., Liu, X., Liu, D., and Yuan, X. (2023). Downregulation of microRNA-494 inhibits cell proliferation in lung squamous cell carcinoma via the induction of PUMA-α-mediated apoptosis. Exp. Ther. Med. 25 (6), 242. doi:10.3892/etm.2023.11941
Gencel-Augusto, J., Wu, W., and Bivona, T. G. (2023). Long non-coding RNAs as emerging targets in lung cancer. Cancers 15 (12), 3135. doi:10.3390/cancers15123135
Gong, J., Li, Y., Liu, C. J., Xiang, Y., Ye, Y., Zhang, Z., et al. (2017). A pan-cancer analysis of the expression and clinical relevance of small nucleolar RNAs in human cancer. Cell Rep. 21 (7), 1968–1981. doi:10.1016/j.celrep.2017.10.070
Greening, D. W., Gopal, S. K., Xu, R., Simpson, R. J., and Chen, W. (2015). Exosomes and their roles in immune regulation and cancer. Semin. Cell Dev. Biol. 40, 72–81. doi:10.1016/j.semcdb.2015.02.009
Guinot, A., Oeztuerk-Winder, F., and Ventura, J. J. (2016). miR-17-92/p38α dysregulation enhances Wnt signaling and selects Lgr6+ cancer stem-like cells during lung adenocarcinoma progression. Cancer Res. 76 (13), 4012–4022. doi:10.1158/0008-5472.CAN-15-3302
Guo, H., Li, T., Peng, C., Mao, Q., Shen, B., Shi, M., et al. (2022). Overexpression of lncRNA A2M-AS1 inhibits cell growth and aggressiveness via regulating the miR-587/bone morphogenetic protein 3 axis in lung adenocarcinoma. Hum. Exp. Toxicol. 41, 9603271221138971. doi:10.1177/09603271221138971
Guo, S., Zhang, J., Zhao, Y. Y., Zhou, L. Y., Xie, Y., Wu, X. Y., et al. (2020a). The expressions of miR-151a-5p and miR-23b in lung cancer tissues and their effects on the biological functions of lung cancer A549 cells. Eur. Rev. Med. Pharmacol. Sci. 24 (12), 6779–6785. doi:10.26355/eurrev_202006_21666
Guo, Y. J., Pan, W. W., Liu, S. B., Shen, Z. F., Xu, Y., and Hu, L. L. (2020b). ERK/MAPK signalling pathway and tumorigenesis. Exp. Ther. Med. 19 (3), 1997–2007. doi:10.3892/etm.2020.8454
Gurunathan, S., Kang, M. H., Jeyaraj, M., Qasim, M., and Kim, J. H. (2019). Review of the isolation, characterization, biological function, and multifarious therapeutic approaches of exosomes. Cells 8 (4), 307. doi:10.3390/cells8040307
Gutschner, T., Hämmerle, M., Eissmann, M., Hsu, J., Kim, Y., Hung, G., et al. (2013). The noncoding RNA MALAT1 is a critical regulator of the metastasis phenotype of lung cancer cells. Cancer Res. 73 (3), 1180–1189. doi:10.1158/0008-5472.CAN-12-2850
Han, L., Yang, H., Wei, W., Hu, F., and Yuan, L. (2022). Hsa_circ_0000520 promotes non-small cell lung cancer progression through the miR-1258/AKT3 Axis. J. Oncol. 2022, 3676685. doi:10.1155/2022/3676685
Hanahan, D., and Weinberg, R. A. (2011). Hallmarks of cancer: the next generation. Cell 144 (5), 646–674. doi:10.1016/j.cell.2011.02.013
Hanan, M., Soreq, H., and Kadener, S. (2017). CircRNAs in the brain. RNA Biol. 14 (8), 1028–1034. doi:10.1080/15476286.2016.1255398
Hao, D., Li, Y., Shi, J., and Jiang, J. (2023). Circ_0110498 facilitates the cisplatin resistance of non-small cell lung cancer by mediating the miR-1287-5p/RBBP4 axis. Thorac. Cancer 14 (7), 662–672. doi:10.1111/1759-7714.14787
Hassanein, M., Hoeksema, M. D., Shiota, M., Qian, J., Harris, B. K., Chen, H., et al. (2013). SLC1A5 mediates glutamine transport required for lung cancer cell growth and survival. Clin. Cancer Res. 19 (3), 560–570. doi:10.1158/1078-0432.CCR-12-2334
Hatley, M. E., Patrick, D. M., Garcia, M. R., Richardson, J. A., Bassel-Duby, R., van Rooij, E., et al. (2010). Modulation of K-Ras-dependent lung tumorigenesis by MicroRNA-21. Cancer Cell 18 (3), 282–293. doi:10.1016/j.ccr.2010.08.013
Hayashita, Y., Osada, H., Tatematsu, Y., Yamada, H., Yanagisawa, K., Tomida, S., et al. (2005). A polycistronic microRNA cluster, miR-17-92, is overexpressed in human lung cancers and enhances cell proliferation. Cancer Res. 65 (21), 9628–9632. doi:10.1158/0008-5472.CAN-05-2352
Hayes, O., Ramos, B., Rodríguez, L. L., Aguilar, A., Badía, T., and Castro, F. O. (2005). Cell confluency is as efficient as serum starvation for inducing arrest in the G0/G1 phase of the cell cycle in granulosa and fibroblast cells of cattle. Anim. Reprod. Sci. 87 (3-4), 181–192. doi:10.1016/j.anireprosci.2004.11.011
He, R. Z., Luo, D. X., and Mo, Y. Y. (2019). Emerging roles of lncRNAs in the post-transcriptional regulation in cancer. Genes Dis. 6 (1), 6–15. doi:10.1016/j.gendis.2019.01.003
He, X. Y., Chen, J. X., Zhang, Z., Peng, Q. L., and Peng, H. M. (2010). The let-7a microRNA protects from growth of lung carcinoma by suppression of k-Ras and c-Myc in nude mice. J. Cancer Res. Clin. Oncol. 136 (7), 1023–1028. doi:10.1007/s00432-009-0747-5
Henne, W. M., Stenmark, H., and Emr, S. D. (2013). Molecular mechanisms of the membrane sculpting ESCRT pathway. Cold Spring Harb. Perspect. Biol. 5 (9), a016766. doi:10.1101/cshperspect.a016766
Hessvik, N. P., and Llorente, A. (2018). Current knowledge on exosome biogenesis and release. Cell Mol. Life Sci. 75 (2), 193–208. doi:10.1007/s00018-017-2595-9
Hsu, Y. L., Hung, J. Y., Chang, W. A., Lin, Y. S., Pan, Y. C., Tsai, P. H., et al. (2017). Hypoxic lung cancer-secreted exosomal miR-23a increased angiogenesis and vascular permeability by targeting prolyl hydroxylase and tight junction protein ZO-1. Oncogene 36 (34), 4929–4942. doi:10.1038/onc.2017.105
Hu, C., Meiners, S., Lukas, C., Stathopoulos, G. T., and Chen, J. (2020). Role of exosomal microRNAs in lung cancer biology and clinical applications. Cell Prolif. 53 (6), e12828. doi:10.1111/cpr.12828
Hu, R., Xu, B., Ma, J., Zhang, L., Wang, L., Zhu, J., et al. (2023b). LINC00963 promotes the malignancy and metastasis of lung adenocarcinoma by stabilizing Zeb1 and exosomes-induced M2 macrophage polarization. Mol. Med. 29 (1), 1. doi:10.1186/s10020-022-00598-y
Hu, S., Zhang, Q., Sun, J., Xue, J., and Wang, C. (2023a). Circular RNA circ_0000376 promotes paclitaxel resistance and tumorigenesis of non-small cell lung cancer via positively modulating KPNA4 by sponging miR-1298-5p. Thorac. Cancer 14 (22), 2116–2126. doi:10.1111/1759-7714.14994
Huang, J. L., Liu, W., Tian, L. H., Chai, T. T., Liu, Y., Zhang, F., et al. (2017). Upregulation of long non-coding RNA MALAT-1 confers poor prognosis and influences cell proliferation and apoptosis in acute monocytic leukemia. Oncol. Rep. 38 (3), 1353–1362. doi:10.3892/or.2017.5802
Huang, W., Yang, Y., Wu, J., Niu, Y., Yao, Y., Zhang, J., et al. (2020a). Circular RNA cESRP1 sensitises small cell lung cancer cells to chemotherapy by sponging miR-93-5p to inhibit TGF-β signalling. Cell Death Differ. 27 (5), 1709–1727. doi:10.1038/s41418-019-0455-x
Huang, X. Y., Huang, Z. L., Huang, J., Xu, B., Xu, Y. H., Huang, X., et al. (2020b). Exosomal circRNA-100338 promotes hepatocellular carcinoma metastasis via enhancing invasiveness and angiogenesis. J. Exp. Clin. Cancer Res. 39 (1), 20. doi:10.1186/s13046-020-1529-9
Hussen, B. M., Abdullah, S. R., Hama Faraj, G. S., Rasul, M. F., Salihi, A., Ghafouri-Fard, S., et al. (2022). Exosomal circular RNA: a signature for lung cancer progression. Cancer Cell Int. 22 (1), 378. doi:10.1186/s12935-022-02793-7
Inamura, K. (2017). Lung cancer: understanding its molecular pathology and the 2015 WHO classification. Front. Oncol. 7, 193. doi:10.3389/fonc.2017.00193
Iqbal, M. A., Arora, S., Prakasam, G., Calin, G. A., and Syed, M. A. (2019). MicroRNA in lung cancer: role, mechanisms, pathways and therapeutic relevance. Mol. Aspects Med. 70, 3–20. doi:10.1016/j.mam.2018.07.003
Jabalee, J., Towle, R., and Garnis, C. (2018). The role of extracellular vesicles in cancer: cargo, function, and therapeutic implications. Cells 7 (8), 93. doi:10.3390/cells7080093
Jiang, W., Wei, K., Pan, C., Li, H., Cao, J., Han, X., et al. (2018). MicroRNA-1258 suppresses tumour progression via GRB2/Ras/Erk pathway in non-small-cell lung cancer. Cell Prolif. 51 (6), e12502. doi:10.1111/cpr.12502
Jin, J., and Yu, G. (2022). Hypoxic lung cancer cell-derived exosomal miR-21 mediates macrophage M2 polarization and promotes cancer cell proliferation through targeting IRF1. World J. Surg. Oncol. 20 (1), 241. doi:10.1186/s12957-022-02706-y
Jin, M., Liu, X., Wu, Y., Lou, Y., Li, X., and Huang, G. (2022). Circular RNA EPB41 expression predicts unfavorable prognoses in NSCLC by regulating miR-486-3p/eIF5A axis-mediated stemness. Cancer Cell Int. 22 (1), 219. doi:10.1186/s12935-022-02618-7
Jin, M., Wang, Y., Zhou, D., Liu, W., Han, R., and Chi, Y. (2023). Downregulation of circ-YES1 suppresses NSCLC migration and proliferation through the miR-142-3p-HMGB1 axis. Respir. Res. 24 (1), 100. doi:10.1186/s12931-023-02378-6
Kalluri, R., and LeBleu, V. S. (2020). The biology, function, and biomedical applications of exosomes. Science 367 (6478), eaau6977. doi:10.1126/science.aau6977
Kalluri, R., and Weinberg, R. A. (2009). The basics of epithelial-mesenchymal transition. J. Clin. Invest. 119 (6), 1420–1428. doi:10.1172/JCI39104
Kan, L., Yang, M., and Zhang, H. (2023). Long noncoding RNA PSMA3-AS1 functions as a competing endogenous RNA to promote gastric cancer progression by regulating the miR-329-3p/ALDOA axis. Biol. Direct 18 (1), 36. doi:10.1186/s13062-023-00392-8
Karachaliou, N., and Rosell, R. (2014). Systemic treatment in EGFR-ALK NSCLC patients: second line therapy and beyond. Cancer Biol. Med. 11 (3), 173–181. doi:10.7497/j.issn.2095-3941.2014.03.003
Kazempour Dizaji, M., Farzanegan, B., Bahrami, N., Khoshnam, Z., Fathi, M., Dargahi, H., et al. (2022). Expression of miRNA1, miRNA133, miRNA191, and miRNA24, as good biomarkers, in non-small cell lung cancer using real-time PCR method. Asian Pac J. Cancer Prev. 23 (5), 1565–1570. doi:10.31557/APJCP.2022.23.5.1565
Kong, R. (2020). Circular RNA hsa_circ_0085131 is involved in cisplatin-resistance of non-small-cell lung cancer cells by regulating autophagy. Cell Biol. Int. 44 (9), 1945–1956. doi:10.1002/cbin.11401
Kong, X. M., Zhang, G. H., Huo, Y. K., Zhao, X. H., Cao, D. W., Guo, S. F., et al. (2015). MicroRNA-140-3p inhibits proliferation, migration and invasion of lung cancer cells by targeting ATP6AP2. Int. J. Clin. Exp. Pathol. 8 (10), 12845–12852.
Kong, Y. J., Tan, X. X., Zhang, Y., He, Q. J., Zhao, L., and Meng, Q. (2019). MiR-141 promotes cell proliferation and invasion in non-small cell lung cancer by targeting KLF9. Eur. Rev. Med. Pharmacol. Sci. 23 (23), 10370–10378. doi:10.26355/eurrev_201912_19676
Kopp, F., Wagner, E., and Roidl, A. (2014). The proto-oncogene KRAS is targeted by miR-200c. Oncotarget 5 (1), 185–195. doi:10.18632/oncotarget.1427
Li, C., Lv, Y., Shao, C., Chen, C., Zhang, T., Wei, Y., et al. (2019). Tumor-derived exosomal lncRNA GAS5 as a biomarker for early-stage non-small-cell lung cancer diagnosis. J. Cell Physiol. 234 (11), 20721–20727. doi:10.1002/jcp.28678
Li, C., Ni, Y. Q., Xu, H., Xiang, Q. Y., Zhao, Y., Zhan, J. K., et al. (2021a). Roles and mechanisms of exosomal non-coding RNAs in human health and diseases. Signal Transduct. Target. Ther. 6 (1), 383. doi:10.1038/s41392-021-00779-x
Li, J., Gao, L., Wang, A., Qian, H., Zhu, J., Ji, S., et al. (2023a). Forkhead box L2 is a target of miR-133b and plays an important role in the pathogenesis of non-small cell lung cancer. Cancer Med. 12 (8), 9826–9842. doi:10.1002/cam4.5746
Li, J., Wang, J., Chen, Z., Chen, Y., and Jin, M. (2018b). Hsa_circ_0079530 promotes cell proliferation and invasion in non-small cell lung cancer. Gene 665, 1–5. doi:10.1016/j.gene.2018.04.059
Li, J., Wang, N., Zhang, F., Jin, S., Dong, Y., Dong, X., et al. (2021d). PIWI-interacting RNAs are aberrantly expressed and may serve as novel biomarkers for diagnosis of lung adenocarcinoma. Thorac. cancer 12 (18), 2468–2477. doi:10.1111/1759-7714.14094
Li, J., Yu, T., Cao, J., Liu, L., Liu, Y., Kong, H. W., et al. (2015). MicroRNA-148a suppresses invasion and metastasis of human non-small-cell lung cancer. Cell Physiol. Biochem. 37 (5), 1847–1856. doi:10.1159/000438546
Li, J., Zhou, S., Li, H., Xu, Y., Zhou, N., and Liu, R. (2021b). PTEN/AKT upregulation of TMSB10 contributes to lung cancer cell growth and predicts poor survival of the patients. Biosci. Biotechnol. Biochem. 85 (4), 805–813. doi:10.1093/bbb/zbaa113
Li, J., Zhu, T., Weng, Y., Cheng, F., Sun, Q., Yang, K., et al. (2022a). Exosomal circDNER enhances paclitaxel resistance and tumorigenicity of lung cancer via targeting miR-139-5p/ITGB8. Thorac. Cancer 13 (9), 1381–1390. doi:10.1111/1759-7714.14402
Li, X., Chen, Z., Ni, Y., Bian, C., Huang, J., Chen, L., et al. (2021c). Tumor-associated macrophages secret exosomal miR-155 and miR-196a-5p to promote metastasis of non-small-cell lung cancer. Transl. Lung Cancer Res. 10 (3), 1338–1354. doi:10.21037/tlcr-20-1255
Li, X., Zhu, G., Li, Y., Huang, H., Chen, C., Wu, D., et al. (2023b). LINC01798/miR-17-5p axis regulates ITGA8 and causes changes in tumor microenvironment and stemness in lung adenocarcinoma. Front. Immunol. 14, 1096818. doi:10.3389/fimmu.2023.1096818
Li, Y., Dong, Y., Zhao, S., Gao, J., Hao, X., Wang, Z., et al. (2022b). Serum-derived piR-hsa-164586 of extracellular vesicles as a novel biomarker for early diagnosis of non-small cell lung cancer. Front. Oncol. 12, 850363. doi:10.3389/fonc.2022.850363
Li, Z. X., Zhu, Q. N., Zhang, H. B., Hu, Y., Wang, G., and Zhu, Y. S. (2018a). MALAT1: a potential biomarker in cancer. Cancer Manag. Res. 10, 6757–6768. doi:10.2147/CMAR.S169406
Liang, Z. Y., Zhang, Z. M., Sun, G. R., Zhao, B. S., Xin, G. H., and Zhang, L. (2023). lncRNA ASBEL and lncRNA Erbb4-IR reduce chemoresistance against gemcitabine and cisplatin in stage IV lung squamous cell carcinoma via the microRNA-21/LZTFL1 axis. Am. J. Cancer Res. 13 (6), 2732–2750.
Liao, J., Chen, Z., Luo, X., Su, Y., Huang, T., Xu, H., et al. (2022). Hsa_circ_0006692 promotes lung cancer progression via miR-205-5p/CDK19 Axis. Genes (Basel) 13 (5), 846. doi:10.3390/genes13050846
Liao, J., Yu, L., Mei, Y., Guarnera, M., Shen, J., Li, R., et al. (2010). Small nucleolar RNA signatures as biomarkers for non-small-cell lung cancer. Mol. cancer 9, 198. doi:10.1186/1476-4598-9-198
Lin, H. P., Wang, Z., and Yang, C. (2021). LncRNA DUXAP10 upregulation and the Hedgehog pathway activation are critically involved in chronic cadmium exposure-induced cancer stem cell-like property. Toxicol. Sci. 184 (1), 33–45. doi:10.1093/toxsci/kfab099
Liu, B., Li, H., Zhou, J., Wang, L., Fang, J., Pu, Z., et al. (2023b). LINC00943 regulates miR-1252-5p/YWHAH axis to promote tumor proliferation and metastasis in lung adenocarcinoma. Heliyon 9 (6), e16736. doi:10.1016/j.heliyon.2023.e16736
Liu, G., Shi, H., Deng, L., Zheng, H., Kong, W., Wen, X., et al. (2019a). Circular RNA circ-FOXM1 facilitates cell progression as ceRNA to target PPDPF and MACC1 by sponging miR-1304-5p in non-small cell lung cancer. Biochem. Biophys. Res. Commun. 513 (1), 207–212. doi:10.1016/j.bbrc.2019.03.213
Liu, H., Lu, C., Jia, H., Wang, Y., Cheng, J., Cheng, R., et al. (2023a). Long non-coding RNA DSCAS regulates cisplatin sensitivity in lung squamous cell carcinoma by competitively binding to miR-646-3p. Heliyon 9 (6), e16865. doi:10.1016/j.heliyon.2023.e16865
Liu, J., Han, Y., Liu, X., and Wei, S. (2020b). Serum miR-185 is a diagnostic and prognostic biomarker for non-small cell lung cancer. Technol. Cancer Res. Treat. 19, 1533033820973276. doi:10.1177/1533033820973276
Liu, J., Jiang, M., Guan, J., Wang, Y., Yu, W., Hu, Y., et al. (2022b). LncRNA KCNQ1OT1 enhances the radioresistance of lung squamous cell carcinoma by targeting the miR-491-5p/TPX2-RNF2 axis. J. Thorac. Dis. 14 (10), 4081–4095. doi:10.21037/jtd-22-1261
Liu, M. Y., Li, X. Q., Gao, T. H., Cui, Y., Ma, N., Zhou, Y., et al. (2016a). Elevated HOTAIR expression associated with cisplatin resistance in non-small cell lung cancer patients. J. Thorac. Dis. 8 (11), 3314–3322. doi:10.21037/jtd.2016.11.75
Liu, P. J., Chen, Y. H., Tsai, K. W., Yeah, H. Y., Yeh, C. Y., Tu, Y. T., et al. (2020a). Involvement of MicroRNA-1-fam83a Axis dysfunction in the growth and motility of lung cancer cells. Int. J. Mol. Sci. 21 (22), 8833. doi:10.3390/ijms21228833
Liu, S., Wang, W., Ning, Y., Zheng, H., Zhan, Y., Wang, H., et al. (2022c). Exosome-mediated miR-7-5p delivery enhances the anticancer effect of Everolimus via blocking MNK/eIF4E axis in non-small cell lung cancer. Cell Death Dis. 13 (2), 129. doi:10.1038/s41419-022-04565-7
Liu, S.-L., Sun, P., Li, Y., and Lu, Y. (2019b). Exosomes as critical mediators of cell-to-cell communication in cancer pathogenesis and their potential clinical application. Transl. Cancer Res. 8 (1), 298–311. doi:10.21037/tcr.2019.01.03
Liu, T., Ma, J., Hou, D., Wang, W., and Cao, H. (2023d). Haplotype-GGGT in long non-coding RNA MALAT1 inhibits brain metastatic lung cancer and lymph nodes of lung cancer via the MALAT1/miR-328/KATNB1. Aging (Albany NY) 15 (6), 1918–1930. doi:10.18632/aging.204563
Liu, W., Wan, Q., Zhou, E., He, P., and Tang, L. (2023e). LncRNA LINC01833 is a prognostic biomarker and correlates with immune infiltrates in patients with lung adenocarcinoma by integrated bioinformatics analysis. J. Oncol. 2023, 3965198. doi:10.1155/2023/3965198
Liu, X., Lv, X., Yang, Q., Jin, H., Zhou, W., and Fan, Q. (2018). MicroRNA-29a functions as a tumor suppressor and increases cisplatin sensitivity by targeting NRAS in lung cancer. Technol. Cancer Res. Treat. 17, 1533033818758905. doi:10.1177/1533033818758905
Liu, Y., Ding, W., Yu, W., Zhang, Y., Ao, X., and Wang, J. (2021). Long non-coding RNAs: biogenesis, functions, and clinical significance in gastric cancer. Mol. Therapy-Oncolytics 23, 458–476. doi:10.1016/j.omto.2021.11.005
Liu, Y., Luo, F., Wang, B., Xu, Y., Liu, X., Shi, L., et al. (2016b). STAT3-regulated exosomal miR-21 promotes angiogenesis and is involved in neoplastic processes of transformed human bronchial epithelial cells. Cancer Lett. 370 (1), 125–135. doi:10.1016/j.canlet.2015.10.011
Liu, Y., Luo, F., Xu, Y., Wang, B., Zhao, Y., Xu, W., et al. (2015). Epithelial-mesenchymal transition and cancer stem cells, mediated by a long non-coding RNA, HOTAIR, are involved in cell malignant transformation induced by cigarette smoke extract. Toxicol. Appl. Pharmacol. 282 (1), 9–19. doi:10.1016/j.taap.2014.10.022
Liu, Y., Zhang, Y., Li, Q., Xu, R., and Huang, N. (2022a). MiR-200c-3p and miR-485-5p overexpression elevates cisplatin sensitivity and suppresses the malignant phenotypes of non-small cell lung cancer cells through targeting RRM2. Thorac. Cancer 13 (13), 1974–1985. doi:10.1111/1759-7714.14475
Liu, Z., Wang, Q., Bi, Y., Chubarov, A. S., Li, Y., Liu, L., et al. (2023c). Long non-coding RNA DINO promotes cisplatin sensitivity in lung adenocarcinoma via the p53-Bax axis. J. Thorac. Dis. 15 (4), 2198–2212. doi:10.21037/jtd-23-465
Liu, Z. Y., Zhang, G. L., Wang, M. M., Xiong, Y. N., and Cui, H. Q. (2011). MicroRNA-663 targets TGFB1 and regulates lung cancer proliferation. Asian Pac J. Cancer Prev. 12 (11), 2819–2823.
Lu, G., and Zhang, Y. (2019). MicroRNA-340-5p suppresses non-small cell lung cancer cell growth and metastasis by targeting ZNF503. Cell Mol. Biol. Lett. 24, 34. doi:10.1186/s11658-019-0161-1
Lu, J., Shi, Y., Zhang, F., Zhang, Y., Zhao, X., Zheng, H., et al. (2023). Mechanism of lnRNA-ICL involved in lung cancer development in COPD patients through modulating microRNA-19-3p/NKRF/NF-κB axis. Cancer Cell Int. 23 (1), 58. doi:10.1186/s12935-023-02900-2
Lu, K. H., Li, W., Liu, X. h., Sun, M., Zhang, M. l., Wu, W. q., et al. (2013). Long non-coding RNA MEG3 inhibits NSCLC cells proliferation and induces apoptosis by affecting p53 expression. BMC Cancer 13, 461. doi:10.1186/1471-2407-13-461
Lv, H., Shi, Z., Sui, A., Zhang, Y., Peng, L., Wang, M., et al. (2022). hsa_circ_0000518 facilitates non-small-cell lung cancer progression via moderating miR-330-3p and positively regulating SLC1A5. J. Immunol. Res. 2022, 4996980. doi:10.1155/2022/4996980
Lv, J., Li, Q., Ma, R., Wang, Z., Yu, Y., Liu, H., et al. (2021). Long noncoding RNA FGD5-AS1 knockdown decrease viability, migration, and invasion of non-small cell lung cancer (NSCLC) cells by regulating the MicroRNA-944/MACC1 Axis. Technol. Cancer Res. Treat. 20, 1533033821990090. doi:10.1177/1533033821990090
Lv, N., Shen, S., Chen, Q., and Tong, J. (2023). Long noncoding RNAs: glycolysis regulators in gynaecologic cancers. Cancer Cell Int. 23 (1), 4. doi:10.1186/s12935-023-02849-2
Lv, X., Li, C. Y., Han, P., and Xu, X. Y. (2018). MicroRNA-520a-3p inhibits cell growth and metastasis of non-small cell lung cancer through PI3K/AKT/mTOR signaling pathway. Eur. Rev. Med. Pharmacol. Sci. 22 (8), 2321–2327. doi:10.26355/eurrev_201804_14822
Ma, J., Bai, Y., Chen, F., Zhou, F., Zhang, L., Xue, P., et al. (2023). MicroRNA-185-5p targets tyrosine 3-monooxygenase/tryptophan 5-monooxygenase activation protein zeta to regulate non-small cell lung cancer progression. J. Cardiothorac. Surg. 18 (1), 241. doi:10.1186/s13019-023-02342-x
Ma, P., Han, W., Meng, C., Tan, X., Liu, P., and Dong, L. (2022). LINC02389/miR-7-5p regulated cisplatin resistance of non-small-cell lung cancer via promoting oxidative stress. Anal. Cell Pathol. (Amst) 2022, 6100176. doi:10.1155/2022/6100176
Ma, Z., Qiu, X., Wang, D., Li, Y., Zhang, B., Yuan, T., et al. (2015). MiR-181a-5p inhibits cell proliferation and migration by targeting Kras in non-small cell lung cancer A549 cells. Acta Biochim. Biophys. Sin. (Shanghai) 47 (8), 630–638. doi:10.1093/abbs/gmv054
Ma, Z., Wei, K., Yang, F., Guo, Z., Pan, C., He, Y., et al. (2021). Tumor-derived exosomal miR-3157-3p promotes angiogenesis, vascular permeability and metastasis by targeting TIMP/KLF2 in non-small cell lung cancer. Cell Death Dis. 12 (9), 840. doi:10.1038/s41419-021-04037-4
Mao, G., Mu, Z., and Wu, D. A. (2021b). Exosomal lncRNA FOXD3-AS1 upregulates ELAVL1 expression and activates PI3K/Akt pathway to enhance lung cancer cell proliferation, invasion, and 5-fluorouracil resistance. Acta Biochim. Biophys. Sin. (Shanghai) 53 (11), 1484–1494. doi:10.1093/abbs/gmab129
Mao, S., Zheng, S., Lu, Z., Wang, X., Wang, Y., Zhang, G., et al. (2021a). Exosomal miR-375-3p breaks vascular barrier and promotes small cell lung cancer metastasis by targeting claudin-1. Transl. Lung Cancer Res. 10 (7), 3155–3172. doi:10.21037/tlcr-21-356
Mao, Y., and Xu, R. (2020). Circular RNA CDR1-AS contributes to pemetrexed and cisplatin chemoresistance through EGFR/PI3K signaling pathway in lung adenocarcinoma. Biomed. Pharmacother. 123, 109771. doi:10.1016/j.biopha.2019.109771
Mathupala, S. P., Rempel, A., and Pedersen, P. L. (2001). Glucose catabolism in cancer cells: identification and characterization of a marked activation response of the type II hexokinase gene to hypoxic conditions. J. Biol. Chem. 276 (46), 43407–43412. doi:10.1074/jbc.M108181200
Meng, Q., Li, Y., Sun, Z., and Yang, X. (2022). CircRNA hsa_circ_0070659 predicts poor prognosis and promotes non-small cell lung cancer (NSCLC) progression via microRNA-377 (miR-377)/Ras-Associated Binding Protein 3C (RAB3C) pathway. Bioengineered 13 (6), 14578–14594. doi:10.1080/21655979.2022.2091572
Meng, Q., Liu, M., and Cheng, R. (2020). LINC00461/miR-4478/E2F1 feedback loop promotes non-small cell lung cancer cell proliferation and migration. Biosci. Rep. 40 (2). doi:10.1042/BSR20191345
Min, L., Zhu, T., Lv, B., An, T., Zhang, Q., Shang, Y., et al. (2022). Exosomal LncRNA RP5-977B1 as a novel minimally invasive biomarker for diagnosis and prognosis in non-small cell lung cancer. Int. J. Clin. Oncol. 27 (6), 1013–1024. doi:10.1007/s10147-022-02129-5
Ming, F., Li, B., Yi, S., and Pi, G. (2023). Circ_0087378 intensifies the malignant behavior of non-small cell lung cancer cells in vitro by facilitating DDR1 via sponging miR-199a-5p. Transl. Lung Cancer Res. 12 (4), 770–785. doi:10.21037/tlcr-23-88
Mondal, T., Subhash, S., Vaid, R., Enroth, S., Uday, S., Reinius, B., et al. (2015). MEG3 long noncoding RNA regulates the TGF-β pathway genes through formation of RNA-DNA triplex structures. Nat. Commun. 6, 7743. doi:10.1038/ncomms8743
Mourksi, N.-E.-H., Morin, C., Fenouil, T., Diaz, J. J., and Marcel, V. (2020). snoRNAs offer novel insight and promising perspectives for lung cancer understanding and management. Cells 9 (3), 541. doi:10.3390/cells9030541
Nie, J., Yang, R., Zhou, R., Deng, Y., Li, D., Gou, D., et al. (2022). Circular RNA circFARSA promotes the tumorigenesis of non-small cell lung cancer by elevating B7H3 via sponging miR-15a-5p. Cell Cycle 21 (24), 2575–2589. doi:10.1080/15384101.2022.2105087
Ota, A., Tagawa, H., Karnan, S., Tsuzuki, S., Karpas, A., Kira, S., et al. (2004). Identification and characterization of a novel gene, C13orf25, as a target for 13q31-q32 amplification in malignant lymphoma. Cancer Res. 64 (9), 3087–3095. doi:10.1158/0008-5472.can-03-3773
Ou, H., Qian, Y., and Ma, L. (2023). MCF2L-AS1 promotes the biological behaviors of hepatocellular carcinoma cells by regulating the miR-33a-5p/FGF2 axis. Aging (Albany NY) 15 (13), 6100–6116. doi:10.18632/aging.204795
Pan, H., Peng, J., Qiao, X., and Gao, H. (2023). Upregulation of lncRNA LANCL1-AS1 inhibits the progression of non-small-cell lung cancer via the miR-3680-3p/GMFG axis. Open Med. (Wars) 18 (1), 20230666. doi:10.1515/med-2023-0666
Pandey, P. R., Yang, J. H., Tsitsipatis, D., Panda, A. C., Noh, J. H., Kim, K. M., et al. (2020). circSamd4 represses myogenic transcriptional activity of PUR proteins. Nucleic Acids Res. 48 (7), 3789–3805. doi:10.1093/nar/gkaa035
Pappas, K., Martin, T. C., Wolfe, A. L., Nguyen, C. B., Su, T., Jin, J., et al. (2021). NOTCH and EZH2 collaborate to repress PTEN expression in breast cancer. Commun. Biol. 4 (1), 312. doi:10.1038/s42003-021-01825-8
Park, C. R., Lee, M., Lee, S. Y., Kang, D., Park, S. J., Lee, D. C., et al. (2023). Regulating POLR3G by MicroRNA-26a-5p as a promising therapeutic target of lung cancer stemness and chemosensitivity. Noncoding RNA Res. 8 (3), 273–281. doi:10.1016/j.ncrna.2023.03.001
Patnaik, S. K., Kannisto, E., Mallick, R., and Yendamuri, S. (2011). Overexpression of the lung cancer-prognostic miR-146b microRNAs has a minimal and negative effect on the malignant phenotype of A549 lung cancer cells. PLoS One 6 (7), e22379. doi:10.1371/journal.pone.0022379
Patra, K. C., Wang, Q., Bhaskar, P. T., Miller, L., Wang, Z., Wheaton, W., et al. (2013). Hexokinase 2 is required for tumor initiation and maintenance and its systemic deletion is therapeutic in mouse models of cancer. Cancer Cell 24 (2), 213–228. doi:10.1016/j.ccr.2013.06.014
Peng, X., Zhao, L., Yao, L., Dong, J., Wu, W., and Luo, T. (2023). Exosomal ERBB2IP contributes to tumor growth via elevating PSAT1 expression in non-small cell lung carcinoma. Thorac. Cancer 14 (19), 1812–1823. doi:10.1111/1759-7714.14926
Pitt, J. M., André, F., Amigorena, S., Soria, J. C., Eggermont, A., Kroemer, G., et al. (2016). Dendritic cell-derived exosomes for cancer therapy. J. Clin. Invest. 126 (4), 1224–1232. doi:10.1172/JCI81137
Prieto-García, E., Díaz-García, C. V., García-Ruiz, I., and Agulló-Ortuño, M. T. (2017). Epithelial-to-mesenchymal transition in tumor progression. Med. Oncol. 34 (7), 122. doi:10.1007/s12032-017-0980-8
Pulliero, A., Mastracci, L., Tarantini, L., Khalid, Z., Bollati, V., and Izzotti, A. (2023). Let-7a downregulation accompanied by KRAS mutation is predictive of lung cancer onset in cigarette smoke-exposed mice. Int. J. Mol. Sci. 24 (14), 11778. doi:10.3390/ijms241411778
Qi, Y., Zha, W., and Zhang, W. (2019). Exosomal miR-660-5p promotes tumor growth and metastasis in non-small cell lung cancer. J. buon 24 (2), 599–607.
Qin, H., Liu, J., Du, Z. H., Hu, R., Yu, Y. K., and Wang, Q. A. (2020). Circular RNA hsa_circ_0012673 facilitates lung cancer cell proliferation and invasion via miR-320a/LIMK18521 axis. Eur. Rev. Med. Pharmacol. Sci. 24 (4), 1841–1852. doi:10.26355/eurrev_202002_20362
Qin, Q., Wei, F., Zhang, J., Wang, X., and Li, B. (2016). miR-134 inhibits non-small cell lung cancer growth by targeting the epidermal growth factor receptor. J. Cell Mol. Med. 20 (10), 1974–1983. doi:10.1111/jcmm.12889
Qiu, M., Xia, W., Chen, R., Wang, S., Xu, Y., Ma, Z., et al. (2018). The circular RNA circPRKCI promotes tumor growth in lung adenocarcinoma. Cancer Res. 78 (11), 2839–2851. doi:10.1158/0008-5472.CAN-17-2808
Qiu, M., Xu, Y., Wang, J., Zhang, E., Sun, M., Zheng, Y., et al. (2015). A novel lncRNA, LUADT1, promotes lung adenocarcinoma proliferation via the epigenetic suppression of p27. Cell Death Dis. 6 (8), e1858. doi:10.1038/cddis.2015.203
Qu, D., Yan, B., Xin, R., and Ma, T. (2018). A novel circular RNA hsa_circ_0020123 exerts oncogenic properties through suppression of miR-144 in non-small cell lung cancer. Am. J. Cancer Res. 8 (8), 1387–1402.
Rajakumar, S., Jamespaulraj, S., Shah, Y., Kejamurthy, P., Jaganathan, M. K., Mahalingam, G., et al. (2023). Long non-coding RNAs: an overview on miRNA sponging and its co-regulation in lung cancer. Mol. Biol. Rep. 50 (2), 1727–1741. doi:10.1007/s11033-022-07995-w
Rana, S., Yue, S., Stadel, D., and Zöller, M. (2012). Toward tailored exosomes: the exosomal tetraspanin web contributes to target cell selection. Int. J. Biochem. Cell Biol. 44 (9), 1574–1584. doi:10.1016/j.biocel.2012.06.018
Rao, L., Luo, L., Luo, L., Chen, S., and Liu, X. (2019). Identification of plasma exosomes long non-coding RNA HAGLR and circulating tumor cells as potential prognosis biomarkers in non-small cell lung cancer. Transl. Cancer Res. 8 (6), 2264–2273. doi:10.21037/tcr.2019.09.43
Reinhart, B. J., Slack, F. J., Basson, M., Pasquinelli, A. E., Bettinger, J. C., Rougvie, A. E., et al. (2000). The 21-nucleotide let-7 RNA regulates developmental timing in Caenorhabditis elegans. Nature 403 (6772), 901–906. doi:10.1038/35002607
Riazifar, M., Mohammadi, M. R., Pone, E. J., Yeri, A., Lässer, C., Segaliny, A. I., et al. (2019). Stem cell-derived exosomes as nanotherapeutics for autoimmune and neurodegenerative disorders. ACS Nano 13 (6), 6670–6688. doi:10.1021/acsnano.9b01004
Robey, R. B., and Hay, N. (2006). Mitochondrial hexokinases, novel mediators of the antiapoptotic effects of growth factors and Akt. Oncogene 25 (34), 4683–4696. doi:10.1038/sj.onc.1209595
Ruzycka-Ayoush, M., Nowicka, A. M., Kowalczyk, A., Gluchowska, A., Targonska, A., Mosieniak, G., et al. (2023). Exosomes derived from lung cancer cells: isolation, characterization, and stability studies. Eur. J. Pharm. Sci. 181, 106369. doi:10.1016/j.ejps.2022.106369
Schmidt, L. H., Spieker, T., Koschmieder, S., Schäffers, S., Humberg, J., Jungen, D., et al. (2011). The long noncoding MALAT-1 RNA indicates a poor prognosis in non-small cell lung cancer and induces migration and tumor growth. J. Thorac. Oncol. 6 (12), 1984–1992. doi:10.1097/JTO.0b013e3182307eac
Schwerdtfeger, M., Desiderio, V., Kobold, S., Regad, T., Zappavigna, S., and Caraglia, M. (2021). Long non-coding RNAs in cancer stem cells. Transl. Oncol. 14 (8), 101134. doi:10.1016/j.tranon.2021.101134
Seviour, E. G., Sehgal, V., Mishra, D., Rupaimoole, R., Rodriguez-Aguayo, C., Lopez-Berestein, G., et al. (2017). Targeting KRas-dependent tumour growth, circulating tumour cells and metastasis in vivo by clinically significant miR-193a-3p. Oncogene 36 (10), 1339–1350. doi:10.1038/onc.2016.308
She, K., He, S., Lu, X., Yu, S., Li, M., Xiong, W., et al. (2023). LncRNA SNHG7 promotes non-small cell lung cancer progression and cisplatin resistance by inducing autophagic activity. J. Thorac. Dis. 15 (1), 155–167. doi:10.21037/jtd-22-1826
She, K., Yu, S., Wang, W., and Chen, B. (2022). CircRNA 0009043 suppresses non-small-cell lung cancer development via targeting the miR-148a-3p/DNAJB4 axis. Biomark. Res. 10 (1), 61. doi:10.1186/s40364-022-00407-y
Shen, H., Cao, D., and Zhang, X. (2023). Advances in exosome research in the management of lung cancer. Curr. Top. Med. Chem. 23 (10), 921–930. doi:10.2174/1568026623666230504101208
Shen, W., Pu, J., Gu, S., Sun, J., Wang, L., Tan, B., et al. (2022). LINC01635, a long non-coding RNA with a cancer/testis expression pattern, promotes lung cancer progression by sponging miR-455-5p. Oncol. Lett. 24 (6), 438. doi:10.3892/ol.2022.13558
Shi, L., Huang, Y., Chen, S., Wang, S., Chen, Y., Chen, X., et al. (2023). Circ_0003028 enhances the proliferation and glycolytic capacity and suppresses apoptosis in non-small cell lung cancer cells via the miR-1305/miR-1322-SLC5A1 axis. Ann. Transl. Med. 11 (5), 215. doi:10.21037/atm-23-178
Shi, L., Zhu, W., Huang, Y., Zhuo, L., Wang, S., Chen, S., et al. (2022). Cancer-associated fibroblast-derived exosomal microRNA-20a suppresses the PTEN/PI3K-AKT pathway to promote the progression and chemoresistance of non-small cell lung cancer. Clin. Transl. Med. 12 (7), e989. doi:10.1002/ctm2.989
Shoda, K., Kuwano, Y., Ichikawa, D., and Masuda, K. (2022). circRNA: a new biomarker and therapeutic target for esophageal cancer. Biomedicines 10 (7), 1643. doi:10.3390/biomedicines10071643
Siegel, R. L., Miller, K. D., and Jemal, A. (2018). Cancer statistics, 2018. CA a cancer J. Clin. 68 (1), 7–30. doi:10.3322/caac.21442
Slack, F. J., and Weidhaas, J. B. (2006). MicroRNAs as a potential magic bullet in cancer. Future Oncol. 2 (1), 73–82. doi:10.2217/14796694.2.1.73
Smolle, M. A., Prinz, F., Calin, G. A., and Pichler, M. (2019). Current concepts of non-coding RNA regulation of immune checkpoints in cancer. Mol. Asp. Med. 70, 117–126. doi:10.1016/j.mam.2019.09.007
Stamm, P., Kirmes, I., Palmer, A., Molitor, M., Kvandova, M., Kalinovic, S., et al. (2021). Doxorubicin induces wide-spread transcriptional changes in the myocardium of hearts distinguishing between mice with preserved and impaired cardiac function. Life Sci. 284, 119879. doi:10.1016/j.lfs.2021.119879
Stoll, L., Rodríguez-Trejo, A., Guay, C., Brozzi, F., Bayazit, M. B., Gattesco, S., et al. (2020). A circular RNA generated from an intron of the insulin gene controls insulin secretion. Nat. Commun. 11 (1), 5611. doi:10.1038/s41467-020-19381-w
Su, J., Liao, J., Gao, L., Shen, J., Guarnera, M. A., Zhan, M., et al. (2016). Analysis of small nucleolar RNAs in sputum for lung cancer diagnosis. Oncotarget 7 (5), 5131–5142. doi:10.18632/oncotarget.4219
Sun, C., Guan, H., Li, J., and Gu, Y. (2023b). circ_0000376 knockdown suppresses non-small cell lung cancer cell tumor properties by the miR-545-3p/PDPK1 pathway. Open Med. (Wars) 18 (1), 20230641. doi:10.1515/med-2023-0641
Sun, C. C., Li, S. J., Li, G., Hua, R. X., Zhou, X. H., and Li, D. J. (2016). Long intergenic noncoding RNA 00511 acts as an oncogene in non-small-cell lung cancer by binding to EZH2 and suppressing p57. Mol. Ther. Nucleic Acids 5 (11), e385. doi:10.1038/mtna.2016.94
Sun, J. C., Wang, L., Zhu, X. H., and Shen, M. L. (2022). Hsa_circ_0006427 suppresses multiplication, migration and invasion of non-small cell lung cancer cells through miR-346/VGLL4 pathway. Cell J. 24 (5), 245–254. doi:10.22074/cellj.2022.7795
Sun, K., Hu, C., Li, Z., Zhu, J., Zhang, L., Shao, X., et al. (2023a). LINC00115 regulates lung adenocarcinoma progression via sponging miR-154-3p to modulate Sp3 expression. Mol. Cell Probes 68, 101909. doi:10.1016/j.mcp.2023.101909
Sun, L., Zhang, Y., Yao, Y., Du, H., Zhang, Y., and Fang, A. (2021). Long noncoding RNA LINC01124 activates hepatocellular carcinoma cell proliferation, migration, and invasion by absorbing microRNA-1247-5p and overexpressing FOXO3. Oncol. Res. 29 (3), 175–187. doi:10.32604/or.2022.03550
Sun, Y., Liu, W. Z., Liu, T., Feng, X., Yang, N., and Zhou, H. F. (2015). Signaling pathway of MAPK/ERK in cell proliferation, differentiation, migration, senescence and apoptosis. J. Recept Signal Transduct. Res. 35 (6), 600–604. doi:10.3109/10799893.2015.1030412
Sun, Y., and Ma, L. (2019). New insights into long non-coding RNA MALAT1 in cancer and metastasis. Cancers (Basel) 11 (2), 216. doi:10.3390/cancers11020216
Sun, Z., Shi, K., Yang, S., Liu, J., Zhou, Q., Wang, G., et al. (2018). Effect of exosomal miRNA on cancer biology and clinical applications. Mol. Cancer 17 (1), 147. doi:10.1186/s12943-018-0897-7
Tan, Z., Sun, Y., Liu, M., Xia, L., Cao, F., Qi, Y., et al. (2020). Retracted: naringenin inhibits cell migration, invasion, and tumor growth by regulating circFOXM1/miR-3619-5p/SPAG5 Axis in lung cancer. Cancer Biother Radiopharm. 35 (10), e826–e838. doi:10.1089/cbr.2019.3520
Tang, J. Y., Li, D. Y., He, L., Qiu, X. S., Wang, E. H., and Wu, G. P. (2020). HPV 16 E6/E7 promote the glucose uptake of GLUT1 in lung cancer through downregulation of TXNIP due to inhibition of PTEN phosphorylation. Front. Oncol. 10, 559543. doi:10.3389/fonc.2020.559543
Tang, P., Sun, D., Xu, W., Li, H., and Chen, L. (2023). Long non-coding RNAs as potential therapeutic targets in non-small cell lung cancer. Int. J. Mol. Med. 52 (2), 1–13. doi:10.3892/ijmm.2023.5271
Tao, X., Li, Y., Fan, S., Wu, L., Xin, J., Su, Y., et al. (2023). Downregulation of Linc00173 increases BCL2 mRNA stability via the miR-1275/PROCA1/ZFP36L2 axis and induces acquired cisplatin resistance of lung adenocarcinoma. J. Exp. Clin. Cancer Res. 42 (1), 12. doi:10.1186/s13046-022-02560-6
Tian, W., Yang, X., Yang, H., Lv, M., Sun, X., and Zhou, B. (2021). Exosomal miR-338-3p suppresses non-small-cell lung cancer cells metastasis by inhibiting CHL1 through the MAPK signaling pathway. Cell Death Dis. 12 (11), 1030. doi:10.1038/s41419-021-04314-2
Tong, Z., Wang, Z., Jiang, J., Tong, C., and Wu, L. (2023). A novel molecular mechanism mediated by circCCDC134 regulates non-small cell lung cancer progression. Thorac. Cancer 14 (20), 1958–1968. doi:10.1111/1759-7714.14942
Trang, P., Wiggins, J. F., Daige, C. L., Cho, C., Omotola, M., Brown, D., et al. (2011). Systemic delivery of tumor suppressor microRNA mimics using a neutral lipid emulsion inhibits lung tumors in mice. Mol. Ther. 19 (6), 1116–1122. doi:10.1038/mt.2011.48
Tsai, T. H., Gow, C. H., Liu, Y. N., Tsai, M. F., Chang, T. H., Wu, S. G., et al. (2023). MiR-503 pleiotropically regulates epithelial-mesenchymal transition and targets PTK7 to control lung cancer metastasis. Cancer Med. 12 (13), 14511–14525. doi:10.1002/cam4.6116
Valadi, H., Ekström, K., Bossios, A., Sjöstrand, M., Lee, J. J., and Lötvall, J. O. (2007). Exosome-mediated transfer of mRNAs and microRNAs is a novel mechanism of genetic exchange between cells. Nat. Cell Biol. 9 (6), 654–659. doi:10.1038/ncb1596
Van Roosbroeck, K., and Calin, G. A. (2017). Cancer hallmarks and MicroRNAs: the therapeutic connection. Adv. Cancer Res. 135, 119–149. doi:10.1016/bs.acr.2017.06.002
Vidal, S. J., Rodriguez-Bravo, V., Galsky, M., Cordon-Cardo, C., and Domingo-Domenech, J. (2014). Targeting cancer stem cells to suppress acquired chemotherapy resistance. Oncogene 33 (36), 4451–4463. doi:10.1038/onc.2013.411
Vishwamitra, D., Li, Y., Wilson, D., Manshouri, R., Curry, C. V., Shi, B., et al. (2012). MicroRNA 96 is a post-transcriptional suppressor of anaplastic lymphoma kinase expression. Am. J. Pathol. 180 (5), 1772–1780. doi:10.1016/j.ajpath.2012.01.008
Wang, C., Wang, X., Liang, H., Wang, T., Yan, X., Cao, M., et al. (2013b). miR-203 inhibits cell proliferation and migration of lung cancer cells by targeting PKCα. PLoS One 8 (9), e73985. doi:10.1371/journal.pone.0073985
Wang, D., Cao, Q., Qu, M., Xiao, Z., Zhang, M., and Di, S. (2017). MicroRNA-616 promotes the growth and metastasis of non-small cell lung cancer by targeting SOX7. Oncol. Rep. 38 (4), 2078–2086. doi:10.3892/or.2017.5854
Wang, H., Tang, Z., Duan, J., Zhou, C., Xu, K., and Mu, H. (2022c). Cancer-released exosomal circular RNA circ_0008717 promotes cell tumorigenicity through microRNA-1287-5p/P21-activated kinase 2 (PAK2) axis in non-small cell lung cancer. Bioengineered 13 (4), 8937–8949. doi:10.1080/21655979.2022.2056822
Wang, K., Song, X., Li, X., Zhang, Z., Xie, L., and Song, X. (2022e). Plasma SNORD83A as a potential biomarker for early diagnosis of non-small-cell lung cancer. Future Oncol. 18 (7), 821–832. doi:10.2217/fon-2021-1278
Wang, L., Wang, D., Xu, Z., Qiu, Y., Chen, G., and Tan, F. (2023b). Circ_0010235 confers cisplatin resistance in lung cancer by upregulating E2F7 through absorbing miR-379-5p. Thorac. Cancer 14 (20), 1946–1957. doi:10.1111/1759-7714.14941
Wang, M., Yu, F., Li, P., and Wang, K. (2020a). Emerging function and clinical significance of exosomal circRNAs in cancer. Mol. Therapy-Nucleic Acids 21, 367–383. doi:10.1016/j.omtn.2020.06.008
Wang, M., Yu, F., Li, P., and Wang, K. (2020b). Emerging function and clinical significance of exosomal circRNAs in cancer. Mol. Ther. Nucleic Acids 21, 367–383. doi:10.1016/j.omtn.2020.06.008
Wang, P., Ren, Z., and Sun, P. (2012b). Overexpression of the long non-coding RNA MEG3 impairs in vitro glioma cell proliferation. J. Cell Biochem. 113 (6), 1868–1874. doi:10.1002/jcb.24055
Wang, Q., Xiao, G., Jiang, X., and Li, C. (2023a). lncRNA PCBP1-AS1 mediated downregulation of ITGAL as a prognostic biomarker in lung adenocarcinoma. Aging (Albany NY) 15 (10), 4510–4523. doi:10.18632/aging.204756
Wang, Q., Zhang, W., Yin, D., Tang, Z., Zhang, E., and Wu, W. (2022a). Gene amplification-driven lncRNA SNHG6 promotes tumorigenesis via epigenetically suppressing p27 expression and regulating cell cycle in non-small cell lung cancer. Cell Death Discov. 8 (1), 485. doi:10.1038/s41420-022-01276-y
Wang, R., Li, G., Zhuang, G., Sun, S., and Song, Z. (2019a). Overexpression of microRNA-423-3p indicates poor prognosis and promotes cell proliferation, migration, and invasion of lung cancer. Diagn Pathol. 14 (1), 53. doi:10.1186/s13000-019-0831-3
Wang, R., Liu, H., Dong, M., Huang, D., and Yi, J. (2022d). Exosomal hsa_circ_0000519 modulates the NSCLC cell growth and metastasis via miR-1258/RHOV axis. Open Med. (Wars) 17 (1), 826–840. doi:10.1515/med-2022-0428
Wang, S., Wang, T., Liu, D., and Kong, H. (2020c). LncRNA MALAT1 aggravates the progression of non-small cell lung cancer by stimulating the expression of COMMD8 via targeting miR-613. Cancer Manag. Res. 12, 10735–10747. doi:10.2147/CMAR.S263538
Wang, W., Sun, H., Zhu, T., and Zhang, H. (2022b). Circ_0002476 regulates cell growth, invasion, and mtDNA damage in non-small cell lung cancer by targeting miR-1182/TFAM axis. Thorac. Cancer 13 (20), 2867–2878. doi:10.1111/1759-7714.14631
Wang, X., Cao, L., Wang, Y., Liu, N., and You, Y. (2012a). Regulation of let-7 and its target oncogenes (Review). Oncol. Lett. 3 (5), 955–960. doi:10.3892/ol.2012.609
Wang, X., Ling, C., Bai, Y., and Zhao, J. (2011). MicroRNA-206 is associated with invasion and metastasis of lung cancer. Anat. Rec. Hob. 294 (1), 88–92. doi:10.1002/ar.21287
Wang, Y., Xu, R., Zhang, D., Lu, T., Yu, W., Wo, Y., et al. (2019b). Circ-ZKSCAN1 regulates FAM83A expression and inactivates MAPK signaling by targeting miR-330-5p to promote non-small cell lung cancer progression. Transl. Lung Cancer Res. 8 (6), 862–875. doi:10.21037/tlcr.2019.11.04
Wang, Y. Y., Ren, T., Cai, Y. Y., and He, X. Y. (2013a). MicroRNA let-7a inhibits the proliferation and invasion of nonsmall cell lung cancer cell line 95D by regulating K-Ras and HMGA2 gene expression. Cancer Biother Radiopharm. 28 (2), 131–137. doi:10.1089/cbr.2012.1307
Wang, Z., Lin, M., He, L., Qi, H., Shen, J., and Ying, K. (2021). Exosomal lncRNA SCIRT/miR-665 transferring promotes lung cancer cell metastasis through the inhibition of HEYL. J. Oncol. 2021, 9813773. doi:10.1155/2021/9813773
Wang, Z. H., Ye, L. L., Xiang, X., Wei, X. S., Niu, Y. R., Peng, W. B., et al. (2023c). Circular RNA circFBXO7 attenuates non-small cell lung cancer tumorigenesis by sponging miR-296-3p to facilitate KLF15-mediated transcriptional activation of CDKN1A. Transl. Oncol. 30, 101635. doi:10.1016/j.tranon.2023.101635
Wei, J., Jia, A., Ma, L., Wang, Y., Qiu, L., and Xiao, B. (2020). MicroRNA-16 inhibits the proliferation and metastasis of human lung cancer cells by modulating the expression of YAP1. J. buon 25 (2), 862–868.
Wei, J., Yu, H., Liu, T., Wang, Z., Lang, C., and Pan, Y. (2023). FOXA1-induced LINC00621 promotes lung adenocarcinoma progression via activating the TGF-β signaling pathway. Thorac. Cancer 14 (21), 2026–2037. doi:10.1111/1759-7714.14986
Wei, S., Zheng, Y., Jiang, Y., Li, X., Geng, J., Shen, Y., et al. (2019). The circRNA circPTPRA suppresses epithelial-mesenchymal transitioning and metastasis of NSCLC cells by sponging miR-96-5p. EBioMedicine 44, 182–193. doi:10.1016/j.ebiom.2019.05.032
Wilson, M. M., Weinberg, R. A., Lees, J. A., and Guen, V. J. (2020). Emerging mechanisms by which EMT programs control stemness. Trends Cancer 6 (9), 775–780. doi:10.1016/j.trecan.2020.03.011
Wolfers, J., Lozier, A., Raposo, G., Regnault, A., Théry, C., Masurier, C., et al. (2001). Tumor-derived exosomes are a source of shared tumor rejection antigens for CTL cross-priming. Nat. Med. 7 (3), 297–303. doi:10.1038/85438
Wu, C., Yang, J., Lin, X., Li, R., and Wu, J. (2023b). miR-508-5p serves as an anti-oncogene by targeting S100A16 to regulate AKT signaling and epithelial-mesenchymal transition process in lung adenocarcinoma cells. Am. J. Med. Sci. 365 (6), 520–531. doi:10.1016/j.amjms.2023.02.014
Wu, D., Deng, S., Li, L., Liu, T., Zhang, T., Li, J., et al. (2021). TGF-β1-mediated exosomal lnc-MMP2-2 increases blood-brain barrier permeability via the miRNA-1207-5p/EPB41L5 axis to promote non-small cell lung cancer brain metastasis. Cell Death Dis. 12 (8), 721. doi:10.1038/s41419-021-04004-z
Wu, D. M., Deng, S. H., Liu, T., Han, R., Zhang, T., and Xu, Y. (2018). TGF-β-mediated exosomal lnc-MMP2-2 regulates migration and invasion of lung cancer cells to the vasculature by promoting MMP2 expression. Cancer Med. 7 (10), 5118–5129. doi:10.1002/cam4.1758
Wu, J., Liu, C., and Yu, G. (2023c). Downregulation of circ_PLXND1 inhibits tumorigenesis of non-small cell lung carcinoma via miR-1287-5p/ERBB3 axis. Thorac. Cancer 14 (17), 1543–1555. doi:10.1111/1759-7714.14897
Wu, K. L., Tsai, Y. M., Lien, C. T., Kuo, P. L., and Hung, A. J. Y. (2019). The roles of MicroRNA in lung cancer. Int. J. Mol. Sci. 20 (7), 1611. doi:10.3390/ijms20071611
Wu, X., Zhang, J., Zhang, X., Xiang, M., Xu, Z., and Cao, Z. (2023a). Prognostic value of miR-219-5p in relation to mortality in patients with small cell lung cancer: a retrospective, observational cohort study in China. BMJ Open 13 (3), e064700. doi:10.1136/bmjopen-2022-064700
Xia, S., and Wang, C. (2023). Hsa_circ_0003220 drives chemoresistance of human NSCLC cells by modulating miR-489-3p/IGF1. Int. J. Genomics 2023, 8845152. doi:10.1155/2023/8845152
Xia, S., and Zhang, Z. (2022). Circular RNA hsa_circ_0000317 inhibits non-small cell lung cancer progression through regulating microRNA-494-3p/phosphatase and tensin homolog deleted on chromosome 10 axis. Clin. (Sao Paulo) 77, 100086. doi:10.1016/j.clinsp.2022.100086
Xia, Y., Zhu, Y., Zhou, X., and Chen, Y. (2014). Low expression of let-7 predicts poor prognosis in patients with multiple cancers: a meta-analysis. Tumour Biol. 35 (6), 5143–5148. doi:10.1007/s13277-014-1663-0
Xie, H., Chen, J., Lv, X., Zhang, L., Wu, J., Ge, X., et al. (2020). Clinical value of serum and exhaled breath condensate miR-186 and IL-1β levels in non-small cell lung cancer. Technol. Cancer Res. Treat. 19, 1533033820947490. doi:10.1177/1533033820947490
Xie, H., Yao, J., Wang, Y., and Ni, B. (2022). Exosome-transmitted circVMP1 facilitates the progression and cisplatin resistance of non-small cell lung cancer by targeting miR-524-5p-METTL3/SOX2 axis. Drug Deliv. 29 (1), 1257–1271. doi:10.1080/10717544.2022.2057617
Xie, Q., Yu, Z., Lu, Y., Fan, J., and Ni, Y. (2019). microRNA-148a-3p inhibited the proliferation and epithelial-mesenchymal transition progression of non-small-cell lung cancer via modulating Ras/MAPK/Erk signaling. J. Cell Physiol. 234 (8), 12786–12799. doi:10.1002/jcp.27899
Xing, L., Ning, Z., Jun, D., Lu, Z., Xin Ting, F., Yu, W., et al. (2020). MicroRNA-138 targets SOX4 to regulate the proliferation and metastasis of human lung cancer cells. J. buon 25 (2), 835–841.
Xiong, J., Xing, S., Dong, Z., Niu, L., Xu, Q., Li, Y., et al. (2021). miR-654-3p suppresses cell viability and promotes apoptosis by targeting RASAL2 in non-small-cell lung cancer. Mol. Med. Rep. 23 (2), 124. doi:10.3892/mmr.2020.11763
Xu, R., He, H., Wang, Y., Peng, Q., Mei, K., Liu, Y., et al. (2023). LncRNA AK001796 promotes cell proliferation via acting as a ceRNA of miR-150 in hepatocellular carcinoma. Genet. Mol. Biol. 46 (2), e20220277. doi:10.1590/1678-4685-GMB-2022-0277
Xu, X., Tao, R., Sun, L., and Ji, X. (2020). Exosome-transferred hsa_circ_0014235 promotes DDP chemoresistance and deteriorates the development of non-small cell lung cancer by mediating the miR-520a-5p/CDK4 pathway. Cancer Cell Int. 20 (1), 552. doi:10.1186/s12935-020-01642-9
Xu, Z., Liu, X., Wang, H., Li, J., Dai, L., Li, J., et al. (2018). Lung adenocarcinoma cell-derived exosomal miR-21 facilitates osteoclastogenesis. Gene 666, 116–122. doi:10.1016/j.gene.2018.05.008
Xue, M., Hong, W., Jiang, J., Zhao, F., and Gao, X. (2020). Circular RNA circ-LDLRAD3 serves as an oncogene to promote non-small cell lung cancer progression by upregulating SLC1A5 through sponging miR-137. RNA Biol. 17 (12), 1811–1822. doi:10.1080/15476286.2020.1789819
Xue, X., Liu, Y., Wang, Y., Meng, M., Wang, K., Zang, X., et al. (2016). MiR-21 and MiR-155 promote non-small cell lung cancer progression by downregulating SOCS1, SOCS6, and PTEN. Oncotarget 7 (51), 84508–84519. doi:10.18632/oncotarget.13022
Xue, Y., Ni, T., Jiang, Y., and Li, Y. (2017). Long noncoding RNA GAS5 inhibits tumorigenesis and enhances radiosensitivity by suppressing miR-135b expression in non-small cell lung cancer. Oncol. Res. 25 (8), 1305–1316. doi:10.3727/096504017X14850182723737
Xue, Y. B., Ding, M. Q., Xue, L., and Luo, J. H. (2019). CircAGFG1 sponges miR-203 to promote EMT and metastasis of non-small-cell lung cancer by upregulating ZNF281 expression. Thorac. Cancer 10 (8), 1692–1701. doi:10.1111/1759-7714.13131
Yamada, H., Yanagisawa, K., Tokumaru, S., Taguchi, A., Nimura, Y., Osada, H., et al. (2008). Detailed characterization of a homozygously deleted region corresponding to a candidate tumor suppressor locus at 21q11-21 in human lung cancer. Genes, Chromosomes Cancer 47 (9), 810–818. doi:10.1002/gcc.20582
Yan, J., Zhu, J., Zhu, X., Liu, H., and Chen, G. (2022). Circ_0092012 knockdown restrains non-small cell lung cancer progression by inhibiting cell malignant phenotype and immune escape through microRNA-635/programmed death ligand 1 axis. Bioengineered 13 (5), 13929–13943. doi:10.1080/21655979.2022.2080386
Yang, F., Pei, Y., Xu, W., and Rong, L. (2022a). hsa_circ_0003176 suppresses the progression of non-small-cell lung cancer via regulating miR-182-5p/RBM5 Axis. Dis. Markers 2022, 8402116. doi:10.1155/2022/8402116
Yang, F., Yan, Y., Yang, Y., Hong, X., Wang, M., Yang, Z., et al. (2020b). MiR-210 in exosomes derived from CAFs promotes non-small cell lung cancer migration and invasion through PTEN/PI3K/AKT pathway. Cell Signal 73, 109675. doi:10.1016/j.cellsig.2020.109675
Yang, H., Feng, X., and Tong, X. (2023c). Long noncoding RNA POU6F2-AS2 contributes to the aggressiveness of nonsmall-cell lung cancer via microRNA-125b-5p-mediated E2F3 upregulation. Aging (Albany NY) 15 (7), 2689–2704. doi:10.18632/aging.204639
Yang, J., Liu, X., Sun, Y., Zhang, X., Zhao, Y., Zhang, H., et al. (2023a). ING5 overexpression upregulates miR-34c-5p/Snail1 to inhibit EMT and invasion of lung cancer cells. Acta Biochim. Biophys. Sin. (Shanghai) 55 (5), 809–817. doi:10.3724/abbs.2023074
Yang, J., Yang, C., and Li, P. (2023d). circ-IARS depletion inhibits the progression of non-small-cell lung cancer by circ-IARS/miR-1252-5p/HDGF ceRNA pathway. Open Med. (Wars) 18 (1), 20220613. doi:10.1515/med-2022-0613
Yang, K., Zhang, J., and Bao, C. (2021). Exosomal circEIF3K from cancer-associated fibroblast promotes colorectal cancer (CRC) progression via miR-214/PD-L1 axis. BMC Cancer 21 (1), 933. doi:10.1186/s12885-021-08669-9
Yang, L. Z., Lei, C. C., Zhao, Y. P., Sun, H. W., Yu, Q. H., Yang, E. J., et al. (2020a). MicroRNA-34c-3p target inhibiting NOTCH1 suppresses chemosensitivity and metastasis of non-small cell lung cancer. J. Int. Med. Res. 48 (3), 300060520904847. doi:10.1177/0300060520904847
Yang, Q., Wang, M., Xu, J., Yu, D., Li, Y., Chen, Y., et al. (2023b). LINC02159 promotes non-small cell lung cancer progression via ALYREF/YAP1 signaling. Mol. Cancer 22 (1), 122. doi:10.1186/s12943-023-01814-x
Yang, X., Li, M., Zhao, Y., Tan, X., Su, J., and Zhong, X. (2022b). Hsa_circ_0079530/AQP4 Axis is related to non-small cell lung cancer development and radiosensitivity. Ann. Thorac. Cardiovasc Surg. 28 (5), 307–319. doi:10.5761/atcs.oa.21-00237
Yang, X., Yu, F., Huang, G., Ni, Y., Zhang, T., Zou, Z., et al. (2023e). Exosomal miR-133a-3p promotes the growth and metastasis of lung cancer cells following incomplete microwave ablation. Int. J. Hyperth. 40 (1), 2190065. doi:10.1080/02656736.2023.2190065
Yao, J., Zhang, H. Y., Gu, S., Zou, J. L., Zhang, Q., and Qu, R. C. (2023). Circular RNA-AnnexinA7 accelerates cisplatin resistance in non-small cell lung cancer via modulating microRNA-545-3p to mediate Cyclin D1. Acta Biochim. Pol. 70 (2), 295–304. doi:10.18388/abp.2020_6539
Yin, Y., Cai, X., Chen, X., Liang, H., Zhang, Y., Li, J., et al. (2014). Tumor-secreted miR-214 induces regulatory T cells: a major link between immune evasion and tumor growth. Cell Res. 24 (10), 1164–1180. doi:10.1038/cr.2014.121
You, X., Vlatkovic, I., Babic, A., Will, T., Epstein, I., Tushev, G., et al. (2015). Neural circular RNAs are derived from synaptic genes and regulated by development and plasticity. Nat. Neurosci. 18 (4), 603–610. doi:10.1038/nn.3975
Yu, F., Liang, M., Huang, Y., Wu, W., Zheng, B., and Chen, C. (2021). Hypoxic tumor-derived exosomal miR-31-5p promotes lung adenocarcinoma metastasis by negatively regulating SATB2-reversed EMT and activating MEK/ERK signaling. J. Exp. Clin. Cancer Res. 40 (1), 179. doi:10.1186/s13046-021-01979-7
Yu, N., Gong, H., Chen, W., and Peng, W. (2023). CircRNA ZKSCAN1 promotes lung adenocarcinoma progression by miR-185-5p/TAGLN2 axis. Thorac. Cancer 14 (16), 1467–1476. doi:10.1111/1759-7714.14889
Yu, X., Liu, D., Wang, L., and Wang, L. (2022). LncRNA LOC285758 induced non-small cell lung cancer development through up-regulating CDK6 by sponge adsorption of miRNA-204. Iran. J. Public Health 51 (9), 2117–2127. doi:10.18502/ijph.v51i9.10567
Yuan, D., Xu, J., Wang, J., Pan, Y., Fu, J., Bai, Y., et al. (2016). Extracellular miR-1246 promotes lung cancer cell proliferation and enhances radioresistance by directly targeting DR5. Oncotarget 7 (22), 32707–32722. doi:10.18632/oncotarget.9017
Yuan, Y., Yu, P., Shen, H., Xing, G., and Li, W. (2023). LncRNA FOXD2-AS1 increased proliferation and invasion of lung adenocarcinoma via cell-cycle regulation. Pharmgenomics Pers. Med. 16, 99–109. doi:10.2147/PGPM.S396866
Zang, X., Gu, J., Zhang, J., Shi, H., Hou, S., Xu, X., et al. (2020). Exosome-transmitted lncRNA UFC1 promotes non-small-cell lung cancer progression by EZH2-mediated epigenetic silencing of PTEN expression. Cell Death Dis. 11 (4), 215. doi:10.1038/s41419-020-2409-0
Zhang, F., Sang, Y., Chen, D., Wu, X., Wang, X., Yang, W., et al. (2021c). M2 macrophage-derived exosomal long non-coding RNA AGAP2-AS1 enhances radiotherapy immunity in lung cancer by reducing microRNA-296 and elevating NOTCH2. Cell Death Dis. 12 (5), 467. doi:10.1038/s41419-021-03700-0
Zhang, H., Chen, X., Zong, B., Yuan, H., Wang, Z., Wei, Y., et al. (2018a). Gypenosides improve diabetic cardiomyopathy by inhibiting ROS-mediated NLRP 3 inflammasome activation. J. Cell. Mol. Med. 22 (9), 4437–4448. doi:10.1111/jcmm.13743
Zhang, J., Hua, X., Qi, N., Han, G., Yu, J., Yu, Y., et al. (2020a). MiR-27b suppresses epithelial-mesenchymal transition and chemoresistance in lung cancer by targeting Snail1. Life Sci. 254, 117238. doi:10.1016/j.lfs.2019.117238
Zhang, J., Li, S., Li, L., Li, M., Guo, C., Yao, J., et al. (2015a). Exosome and exosomal microRNA: trafficking, sorting, and function. Genomics Proteomics Bioinforma. 13 (1), 17–24. doi:10.1016/j.gpb.2015.02.001
Zhang, J., Mou, Y., Shen, H., Song, J., and Li, Q. (2023a). LINC00638 promotes the progression of non-small cell lung cancer by regulating the miR-541-3p/IRS1/PI3K/Akt axis. Heliyon 9 (6), e16999. doi:10.1016/j.heliyon.2023.e16999
Zhang, J.-g., Wang, J. j., Zhao, F., Liu, Q., Jiang, K., and Yang, G. h. (2010a). MicroRNA-21 (miR-21) represses tumor suppressor PTEN and promotes growth and invasion in non-small cell lung cancer (NSCLC). Clin. Chim. Acta 411 (11), 846–852. doi:10.1016/j.cca.2010.02.074
Zhang, L., Liu, H., Long, Y., and Zhang, Y. (2023b). Overexpression of LINC00607 inhibits cell growth and aggressiveness by regulating the miR-1289/EFNA5 axis in non-small-cell lung cancer. Open Med. (Wars) 18 (1), 20230649. doi:10.1515/med-2023-0649
Zhang, L., Zhang, S., Yao, J., Lowery, F. J., Zhang, Q., Huang, W. C., et al. (2015b). Microenvironment-induced PTEN loss by exosomal microRNA primes brain metastasis outgrowth. Nature 527 (7576), 100–104. doi:10.1038/nature15376
Zhang, N., Nan, A., Chen, L., Li, X., Jia, Y., Qiu, M., et al. (2020c). Circular RNA circSATB2 promotes progression of non-small cell lung cancer cells. Mol. Cancer 19 (1), 101. doi:10.1186/s12943-020-01221-6
Zhang, W., Cai, X., Yu, J., Lu, X., Qian, Q., and Qian, W. (2018b). Exosome-mediated transfer of lncRNA RP11-838N2.4 promotes erlotinib resistance in non-small cell lung cancer. Int. J. Oncol. 53 (2), 527–538. doi:10.3892/ijo.2018.4412
Zhang, X., Gejman, R., Mahta, A., Zhong, Y., Rice, K. A., Zhou, Y., et al. (2010b). Maternally expressed gene 3, an imprinted noncoding RNA gene, is associated with meningioma pathogenesis and progression. Cancer Res. 70 (6), 2350–2358. doi:10.1158/0008-5472.CAN-09-3885
Zhang, X., Guo, H., Bao, Y., Yu, H., Xie, D., and Wang, X. (2019b). Exosomal long non-coding RNA DLX6-AS1 as a potential diagnostic biomarker for non-small cell lung cancer. Oncol. Lett. 18 (5), 5197–5204. doi:10.3892/ol.2019.10892
Zhang, X., Hamblin, M. H., and Yin, K. J. (2017). The long noncoding RNA Malat1: its physiological and pathophysiological functions. RNA Biol. 14 (12), 1705–1714. doi:10.1080/15476286.2017.1358347
Zhang, X., Sai, B., Wang, F., Wang, L., Wang, Y., Zheng, L., et al. (2019a). Hypoxic BMSC-derived exosomal miRNAs promote metastasis of lung cancer cells via STAT3-induced EMT. Mol. Cancer 18 (1), 40. doi:10.1186/s12943-019-0959-5
Zhang, X. Q., Song, Q., and Zeng, L. X. (2023d). Circulating hsa_circ_0072309, acting via the miR-100/ACKR3 pathway, maybe a potential biomarker for the diagnosis, prognosis, and treatment of brain metastasis from non-small-cell lung cancer. Cancer Med. 12, 18005–18019. doi:10.1002/cam4.6371
Zhang, Y., Jia, D. D., Zhang, Y. F., Cheng, M. D., Zhu, W. X., Li, P. F., et al. (2021b). The emerging function and clinical significance of circRNAs in Thyroid Cancer and Autoimmune Thyroid Diseases. Int. J. Biol. Sci. 17 (7), 1731–1741. doi:10.7150/ijbs.55381
Zhang, Y., and Ma, H. (2023). LncRNA HOXD-AS2 regulates miR-3681-5p/DCP1A axis to promote the progression of non-small cell lung cancer. J. Thorac. Dis. 15 (3), 1289–1301. doi:10.21037/jtd-23-153
Zhang, Y., Mi, Y., and He, C. (2023c). 2-methoxyestradiol restrains non-small cell lung cancer tumorigenesis through regulating circ_0010235/miR-34a-5p/NFAT5 axis. Thorac. Cancer 14 (22), 2105–2115. doi:10.1111/1759-7714.14993
Zhang, Y., Zhang, T., Li, P., Xu, J., and Wang, Z. (2021a). Long noncoding RNA TFAP2A-AS1 exerts promotive effects in non-small cell lung cancer progression via controlling the microRNA-548a-3p/CDK4 axis as a competitive endogenous RNA. Oncol. Res. 29 (2), 129–139. doi:10.32604/or.2022.03563
Zhang, Z. Y., Gao, X. H., Ma, M. Y., Zhao, C. L., Zhang, Y. L., and Guo, S. S. (2020b). CircRNA_101237 promotes NSCLC progression via the miRNA-490-3p/MAPK1 axis. Sci. Rep. 10 (1), 9024. doi:10.1038/s41598-020-65920-2
Zhao, H., Wang, Z., Wu, G., Lu, Y., Zheng, J., Zhao, Y., et al. (2023b). Role of MicroRNA-214 in dishevelled1-modulated β-catenin signalling in non-small cell lung cancer progression. J. Cancer 14 (2), 239–249. doi:10.7150/jca.80291
Zhao, H., Wei, H., He, J., Wang, D., Li, W., Wang, Y., et al. (2020b). Propofol disrupts cell carcinogenesis and aerobic glycolysis by regulating circTADA2A/miR-455-3p/FOXM1 axis in lung cancer. Cell Cycle 19 (19), 2538–2552. doi:10.1080/15384101.2020.1810393
Zhao, J. (2016). Cancer stem cells and chemoresistance: the smartest survives the raid. Pharmacol. Ther. 160, 145–158. doi:10.1016/j.pharmthera.2016.02.008
Zhao, L., Wu, X., Zhang, Z., Fang, L., Yang, B., and Li, Y. (2023a). ELF1 suppresses autophagy to reduce cisplatin resistance via the miR-152-3p/NCAM1/ERK axis in lung cancer cells. Cancer Sci. 114 (6), 2650–2663. doi:10.1111/cas.15770
Zhao, Q., Liu, J., Deng, H., Ma, R., Liao, J. Y., Liang, H., et al. (2020a). Targeting mitochondria-located circRNA SCAR alleviates NASH via reducing mROS output. Cell 183 (1), 76–93. doi:10.1016/j.cell.2020.08.009
Zhao, X., Lu, C., Chu, W., Zhang, B., Zhen, Q., Wang, R., et al. (2017). MicroRNA-124 suppresses proliferation and glycolysis in non-small cell lung cancer cells by targeting AKT-GLUT1/HKII. Tumour Biol. 39 (5), 1010428317706215. doi:10.1177/1010428317706215
Zhao, Z., Liu, J., Wang, C., Wang, Y., Jiang, Y., and Guo, M. (2014). MicroRNA-25 regulates small cell lung cancer cell development and cell cycle through cyclin E2. Int. J. Clin. Exp. Pathol. 7 (11), 7726–7734.
Zheng, L., Qi, Y. X., Liu, S., Shi, M. L., and Yang, W. P. (2016). miR-129b suppresses cell proliferation in the human lung cancer cell lines A549 and H1299. Genet. Mol. Res. 15 (4). doi:10.4238/gmr15048367
Zhou, C., Huang, C., Wang, J., Huang, H., Li, J., Xie, Q., et al. (2017). LncRNA MEG3 downregulation mediated by DNMT3b contributes to nickel malignant transformation of human bronchial epithelial cells via modulating PHLPP1 transcription and HIF-1α translation. Oncogene 36 (27), 3878–3889. doi:10.1038/onc.2017.14
Zhou, J., Wang, H., Sun, Q., Liu, X., Wu, Z., Wang, X., et al. (2021b). miR-224-5p-enriched exosomes promote tumorigenesis by directly targeting androgen receptor in non-small cell lung cancer. Mol. Ther. Nucleic Acids 23, 1217–1228. doi:10.1016/j.omtn.2021.01.028
Zhou, Q., Tang, X., Tian, X., Tian, J., Zhang, Y., Ma, J., et al. (2018). LncRNA MALAT1 negatively regulates MDSCs in patients with lung cancer. J. Cancer 9 (14), 2436–2442. doi:10.7150/jca.24796
Zhou, R., Xu, J., Wang, L., and Li, J. (2021a). LncRNA PRRT3-AS1 exerts oncogenic effects on nonsmall cell lung cancer by targeting microRNA-507/homeobox B5 axis. Oncol. Res. 29 (6), 411–423. doi:10.32604/or.2022.026236
Zhou, Y., Liu, H., Wang, R., and Zhang, M. (2023). Circ_0043256 upregulates KLF2 expression by absorbing miR-1206 to suppress the tumorigenesis of lung cancer. Thorac. Cancer 14 (7), 683–699. doi:10.1111/1759-7714.14794
Zhu, H., Yang, W., Cheng, Q., and Yang, S. (2022b). Circ_0010235 regulates HOXA10 expression to promote malignant phenotypes and radioresistance in non-small cell lung cancer cells via decoying miR-588. Balk. Med. J. 39 (4), 255–266. doi:10.4274/balkanmedj.galenos.2022.2022-2-50
Zhu, J., Cao, K., Zhang, P., and Ma, J. (2023a). LINC00669 promotes lung adenocarcinoma growth by stimulating the Wnt/β-catenin signaling pathway. Cancer Med. 12 (7), 9005–9023. doi:10.1002/cam4.5604
Zhu, J., Wang, F., Weng, Y., and Zhao, J. (2022c). Exosome-delivered circSATB2 targets the miR-330-5p/PEAK1 axis to regulate proliferation, migration and invasion of lung cancer cells. Thorac. Cancer 13 (21), 3007–3017. doi:10.1111/1759-7714.14652
Zhu, K., Ding, H., Wang, W., Liao, Z., Fu, Z., Hong, Y., et al. (2016). Tumor-suppressive miR-218-5p inhibits cancer cell proliferation and migration via EGFR in non-small cell lung cancer. Oncotarget 7 (19), 28075–28085. doi:10.18632/oncotarget.8576
Zhu, L., Sun, L., Xu, G., Song, J., Hu, B., Fang, Z., et al. (2022a). The diagnostic value of has_circ_0006423 in non-small cell lung cancer and its role as a tumor suppressor gene that sponges miR-492. Sci. Rep. 12 (1), 13722. doi:10.1038/s41598-022-17816-6
Zhu, X., Du, T., Chen, X., and Hu, P. (2023b). Circ-PDZD8 promotes cell growth and glutamine metabolism in non-small cell lung cancer by enriching LARP1 via sequestering miR-330-5p. Thorac. Cancer 14 (22), 2187–2197. doi:10.1111/1759-7714.15006
Zhu, X., Wan, J., You, X., Yang, W., and Zhao, L. (2023c). Circular non-coding RNA circ_0072088 serves as a ceRNA, targeting the miR-1225-5p/WT1 axis to regulate non-small cell lung cancer cell malignant behavior. Thorac. Cancer 14 (20), 1969–1979. doi:10.1111/1759-7714.14943
Zhuang, G., Wu, X., Jiang, Z., Kasman, I., Yao, J., Guan, Y., et al. (2012). Tumour-secreted miR-9 promotes endothelial cell migration and angiogenesis by activating the JAK-STAT pathway. Embo J. 31 (17), 3513–3523. doi:10.1038/emboj.2012.183
Keywords: lung cancer, non-coding RNA, microRNA, long non-coding RNA, circular RNA
Citation: Lv X, Yang L, Xie Y and Momeni MR (2024) Non-coding RNAs and exosomal non-coding RNAs in lung cancer: insights into their functions. Front. Cell Dev. Biol. 12:1397788. doi: 10.3389/fcell.2024.1397788
Received: 08 March 2024; Accepted: 02 May 2024;
Published: 27 May 2024.
Edited by:
Zhi Tian, University of South Florida, United StatesReviewed by:
Xiangxuan Zhao, China Medical University, ChinaCopyright © 2024 Lv, Yang, Xie and Momeni. This is an open-access article distributed under the terms of the Creative Commons Attribution License (CC BY). The use, distribution or reproduction in other forums is permitted, provided the original author(s) and the copyright owner(s) are credited and that the original publication in this journal is cited, in accordance with accepted academic practice. No use, distribution or reproduction is permitted which does not comply with these terms.
*Correspondence: Yunbo Xie, ZG9jdG9yOTJAaG9zcGl0YWwuY3FtdS5lZHUuY24=; Mohammad Reza Momeni, bW9tZW5pLm0xMjA5QGdtYWlsLmNvbQ==
†These authors have contributed equally to this work and share first authorship
Disclaimer: All claims expressed in this article are solely those of the authors and do not necessarily represent those of their affiliated organizations, or those of the publisher, the editors and the reviewers. Any product that may be evaluated in this article or claim that may be made by its manufacturer is not guaranteed or endorsed by the publisher.
Research integrity at Frontiers
Learn more about the work of our research integrity team to safeguard the quality of each article we publish.