- 1Bethune First Clinical School of Medicine, The First Hospital of Jilin University, Changchun, China
- 2Department of General Surgery, The Second Hospital of Jilin University, Changchun, China
- 3Department of Otolaryngology Head and Neck Surgery, China-Japan Union Hospital of Jilin University, Changchun, China
- 4Department of Clinical Pharmacy, The First Hospital of Jilin University, Changchun, China
Cancer-associated fibroblasts (CAFs), a class of stromal cells in the tumor microenvironment (TME), play a key role in controlling cancer cell invasion and metastasis, immune evasion, angiogenesis, and resistance to chemotherapy. CAFs mediate their activities by secreting soluble chemicals, releasing exosomes, and altering the extracellular matrix (ECM). Exosomes contain various biomolecules, such as nucleic acids, lipids, and proteins. microRNA (miRNA), a 22–26 nucleotide non-coding RNA, can regulate the cellular transcription processes. Studies have shown that miRNA-loaded exosomes secreted by CAFs engage in various regulatory communication networks with other TME constituents. This study focused on the roles of CAF-derived exosomal miRNAs in generating cancer malignant characteristics, including immune modulation, tumor growth, migration and invasion, epithelial-mesenchymal transition (EMT), and treatment resistance. This study thoroughly examines miRNA’s dual regulatory roles in promoting and suppressing cancer. Thus, changes in the CAF-derived exosomal miRNAs can be used as biomarkers for the diagnosis and prognosis of patients, and their specificity can be used to develop newer therapies. This review also discusses the pressing problems that require immediate attention, aiming to inspire researchers to explore more novel avenues in this field.
1 Introduction
The International Agency for Research on Cancer predicted that the global incidence of cancer will rise by 75% by 2030, reaching 22.2 million new cases (Thun et al., 2010). According to a recent editorial published in the Journal of the American Medical Association, cancer is estimated to cost $25.2 trillion between 2020 and 2050, 0.55% of the world’s yearly gross domestic product (Lopes, 2023). Cancer is a complex disease involving alterations in the genome with multiple mutations, leading to uncontrolled cell proliferation and morphological changes (Graham and Sottoriva, 2017). Most malignancies present numerous malignant characteristics, including uncontrolled reproductive capacity, enhanced invasion and metastasis, increased angiogenesis, cell death resistance, immune surveillance evasion, metabolic reprogramming, and treatment resistance (Curtius et al., 2018; Wu et al., 2019). The extent of these behaviors is determined by the interactions between different components of the tumor microenvironment (TME) (Lee and Cheah, 2019).
TME consists of immune cells (such as T and B lymphocytes, natural killer (NK) cells, and tumor-associated macrophages) and stromal cells (fibroblasts, mesenchymal stromal cells, pericytes, and adipocytes), which are present in the extracellular matrix (ECM) (Malla et al., 2021; Ali et al., 2022; Tiwari et al., 2022). The importance of cancer-associated fibroblasts (CAFs), a crucial part of TME, in tumor control cannot be disregarded.
CAFs, a diverse group of interstitial cells, can be classified into various subtypes based on the differential expression of specific biomarkers, each having unique functions and roles (Zhang M. et al., 2022). Fibroblasts are usually quiescent in normal tissues but can be activated during tissue injury (Raju et al., 2022). One of the primary sources of CAFs is the presence of these activated fibroblasts that are seen near cancer cells. CAFs are a type of mesenchymal cells exhibiting high levels of elasticity, flexibility, and universality. They are actively engaged in cancer development by enhancing immune evasion, inducing angiogenesis, encouraging/suppressing chemotherapy resistance, and facilitating/inhibiting cancer cell invasion and metastasis through intricate interactions with other cell types in the TME (Chen and Song, 2019). One significant way CAFs operate is through exosomes (Yang et al., 2017; Pan et al., 2022a). For instance, they can mediate the proliferation and invasion of bladder cancer cells (Yan et al., 2020).
Almost every cell type in the human body can release exosomes, a subtype of extracellular vesicles (EVs) (Doyle and Wang, 2019). Exosomes, having an average diameter of 30–150 nm, were initially identified by Trams et al. In 1981 as cell-shedding vesicles that could be separated from various normal and malignant cells (Trams et al., 1981). These membrane-based vesicles were identified and given the formal term “exosomes” by Johnstone et al. in 1987 (Johnstone et al., 1987). Presently, exosomes are included into the small extracellular vesicles category according to MISEV guidelines and current consensus in the field. Exosomes contain proteins, lipids, and nucleic acids that can be transferred between cells. This transfer can activate various signaling pathways and regulate the biological functions of tumor cells. These exosomes have potential applications in tumor detection and management (Kalluri and LeBleu, 2020; Shao et al., 2020; Zhang et al., 2022b). In essence, the potential impacts of the contents of exosomes determine the manifestation of its regulatory influence.
The human body contains significant amounts of microRNA (miRNAs), vital in regulating the biological genome. Their expression profiles in cells vary between the normal and disease states in humans, suggesting that miRNAs can serve as disease biomarkers and have garnered growing interest (He et al., 2020; Hill and Tran, 2021; Liu J. et al., 2023). miRNAs significantly impact various essential biological processes, such as cell differentiation, apoptosis, proliferation, metabolism, and differentiation. They have also been linked to several illnesses, including malignancies (Iacona and Lutz, 2019; Li B. et al., 2021; Pan G. et al., 2023; Pan Z. et al., 2023). Exosomes provide an optimal environment for carrying miRNA because miRNA is unstable when it exists alone in vitro and can be degraded by RNA enzymes in the human body (Qiu et al., 2022). Several studies have pointed out that miRNAs in CAF-derived exosomes play an essential role in tumor regulation, and these miRNAs can interact with different cells in the TME through exosomes (Villegas-Pineda et al., 2021). The impact of extracellular miRNAs secreted by immune cells, cancer cells, and other cell types in TME has been briefly outlined by others and will not be further discussed in this work (Alizadeh et al., 2019; Xin et al., 2021; Hao et al., 2023). This review focuses on the function of CAF-derived exosomal miRNAs in cancer progression. Furthermore, it points out their prospects as tools for diagnosing and treating patients with cancer, in addition to acknowledging the pressing problems in the current research.
2 CAFs
CAFs are a type of stromal cells in the TME that can produce ECM related to cancer growth, invasion, and metastasis. Unlike normal fibroblasts, CAFs have been reprogrammed by the cancer cells and surrounding TME to promote tumor progression (Sahai et al., 2020). Activated fibroblasts, increased microvascular density, inflammatory cell count, and altered ECM composition are the salient characteristics of mesenchymal TME (Huang et al., 2021; Bilotta et al., 2022; Jayaram and Phillips, 2024). Since CAFs are a form of interstitial cells with high universality, plasticity, and elasticity, they actively participate in cancer development through intricate interactions with other cell types in the TME (Mao et al., 2021). The characteristics and interactions of CAFs with different cell types may undergo dynamic changes as tumor participants. Fibroblast activating protein (FAP) and α-smooth muscle actin (α-SMA) expression are commonly used to identify CAFs (Geng et al., 2021). CAF’s effect on tumors is currently under extensive investigation, as it plays a crucial role in the development and advancement of cancer. Consequently, it is considered a highly promising target for cancer treatment.
2.1 Sources of CAFs
Generally, fibroblasts are not endothelial, immune, or epithelial cells but mesenchymal cells (Wei et al., 2021). Hence, fibroblasts present inside or close to the tumor can be considered CAFs (Papait et al., 2022). However, the exact cellular origin and function of fibroblasts remain unclear and difficult to determine because of their phenotypic and functional heterogeneity.
According to the findings of published studies, the primary sources of CAFs are as follows: (1) resident fibroblasts (O’Connor et al., 2023; Buechler and Turley, 2018); (2) bone marrow mesenchymal stem cells (Liubomirski et al., 2019); (3) vascular adventitia and smooth muscle cells (Zeltz et al., 2019); (4) endothelial cells (Chu et al., 2022; Shinkawa et al., 2022); (5) human adipose tissue-derived stem cells (Sato et al., 2023); (6) stationary pancreatic stellate cells (Morgan et al., 2023) and hepatic stellate cells (Yin et al., 2013; Wang S. S. et al., 2021; Sankar et al., 2023); and (7) cancer stem cells (Najafi et al., 2019) (Figure 1). Understanding the origins of different CAFs can shed light on their functions and phenotypes. This will contribute to advancing research on targeted tumor therapies involving CAF-derived cells.
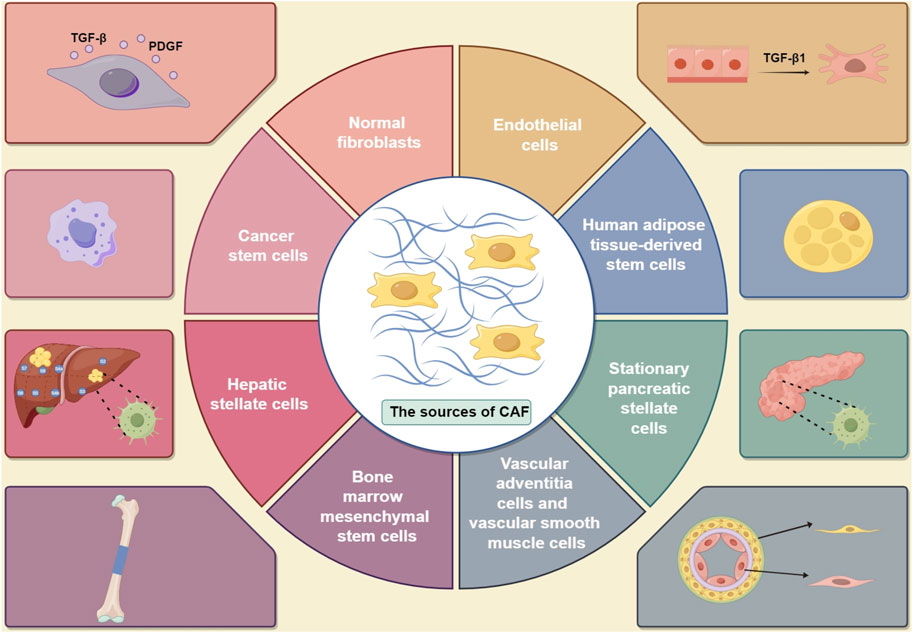
Figure 1. Sources of CAFs in tumors, including (1) normal fibroblasts, which can be influenced by TGF-β and PDGF (O’Connor et al., 2023; Buechler and Turley, 2018); (2) cancer stem cells (Liubomirski et al., 2019); (3) vascular adventitia and vascular smooth muscle cells (Zeltz et al., 2019); (4) endothelial cells, which can be activated by TGF- β1 (Chu et al., 2022; Shinkawa et al., 2022); (5) human adipose tissue-derived stem cells (Sato et al., 2023); (6) stationary pancreatic stellate cells (Morgan et al., 2023); (7) hepatic stellate cells (Wang S. S. et al., 2021); and (8) bone marrow mesenchymal stem cells (TGF- β: transforming growth factor-β; TGF- β1: transforming growth factor-β1; PDGF: platelet-derived growth factor). (By Figdraw).
2.2 Heterogeneity of CAFs
Studies have indicated that heterogeneity in CAFs primarily manifests through variations in cellular phenotype. The phenotypic changes in CAFs exhibit temporal and geographical features, which involve the development of distinct fibroblast phenotypes and the differentiation of phenotypes of the same fibroblast in different tissue regions (Sahai et al., 2020; Zhang et al., 2022c). The advancement in single-cell RNA sequencing technology has enabled the statistical evaluation of transcriptome variations at the cellular level (Li X. et al., 2022; Luo et al., 2022). This analysis technique reveals the coexistence of various fibroblast populations in CAFs, forming different subgroups (Mezawa and Orimo, 2022). The main subgroups of CAFs include (1) mCAF, derived from resident tissue fibroblasts, mainly present in the periphery of tumors, involved in immune suppression (Zhang Z. et al., 2020); (2) dCAF, originating from tumor epithelial stroma, located close to cancer cells in the early stages of tumors, promoting tumor cell migration (Ohlund et al., 2017); (3) vCAF, converted from perivascular cells. In the early stages of tumor development, vCAFs are located near blood vessels, but during tumor evolution, they are located within the stromal compartment, promoting angiogenesis (Bartoschek et al., 2018; Mezawa and Orimo, 2022); (4) cCAF, overlapping with vCAF and exhibiting strong proliferative ability (Wang et al., 2022a); (5) iCAF, associated with inflammation, characterized by immune regulatory molecules, involved in tumor metastasis, immune suppression, migration, and other processes (Chen Z. et al., 2020); (6) myCAF, derived from myofibroblasts (Ohlund et al., 2017; Peltier et al., 2022), involved in tumor cell metastasis and drug resistance (Das et al., 2020); (7) apCAF, the antigen-presenting CAF population, capable of presenting antigens to T cells. However, due to the lack of necessary co-stimulatory molecules such as CD80, CD86, and CD40, they can hardly induce T cell clonal proliferation. Existing studies hypothesize that apCAFs act as decoy receptors, inhibiting immune reactions in the tumor microenvironment (Elyada et al., 2019). Furthermore, other CAF subtypes, including CD146+ CAFs (Brechbuhl et al., 2017), α-SMA+ CAFs (McAndrews et al., 2022), asporin+ CAFs (Maris et al., 2015), and versican+ CAFs (Fanhchaksai et al., 2016), also play tumor-suppressive roles. In summary, there are significant variations in the overall survival rates, immune cell counts, and response rates to immunotherapy among different subtypes (Wang et al., 2022a).
Markers used to identify different subtypes of CAF are: (1) surface markers such as podoplanin (PDPN), fibroblast activating protein α (FAP-α), platelet-derived growth factor receptor α or β (PDGFR α or β), TGF-β receptor I/II (TGF-βR I/II), epidermal growth factor receptor (EGFR), fibroblast growth factor receptor (FGFR), and bone morphogenetic protein receptor I/II (BMPR I/II); (2) intracellular markers such as α-SMA, actin-α cardiac muscle (ACTA2), S100A4, fibroblast specific protein-1 (FSP-1), vimentin (VIM), desmin, and transgelin (TAGLN); (3) extracellular biomarkers such as collagen 1a1 (COL1A1), COL1A2, lumican (LUM), decorin (DCN), microfibril associated protein 5 (MFAP5), fibronectin, tenacin-C, periostin, and remodeling enzymes: lysyl oxidase (LOX), lysyl oxidase-like 1 (LOXL 1), and matrix metalloproteinase (MMP); and (4) growth factors and cytokines: TGF-β, vascular endothelial growth factor (VEGF), platelet-derived growth factor (PDGF), fibroblast growth factor (FGF), paternally expressed gene 2 (PEG2), connective tissue growth factor (CTGF), stromal cell-derived factor-1 (SDF-1), and WNT, and so on (Dzobo and Dandara, 2020; Aden et al., 2023; Xu et al., 2023).
Additional research stemming from the diversity of CAFs includes whether the development of cancer or mutations in tumor suppressor genes within tumor cells can lead to the transformation of one subtype into another or the emergence of new subtypes. Furthermore, the precise mechanisms and interactions through which different subtypes of CAFs regulate tumors are currently being investigated.
2.3 Functions of CAFs in TME
TME is a complex network of cells embedded in the ECM, playing a crucial role in tumor formation. These include immunological and endothelial cells, adipocytes, tumor cells, and CAFs. The presence of activated fibroblasts, higher density of microvascularization, inflammatory cell count, and altered ECM components are the key characteristics of TME (Rimal et al., 2022). Of all the mesenchymal cells that make up TME, CAFs are the most common cells (Fang et al., 2023). The contributions of CAFs vary according to tumor type. For example, pancreatic adenocarcinoma displays a highly reactive stroma where CAFs are thought to play a prominent role in tumor progression. But, in other tumor types, their presence is quite restricted (Geng et al., 2021; Aden et al., 2023). So far, their role in tumor development has not been fully understood.
CAFs have higher contractility, increased proliferation rate, and greater expression of α-SMA than normal fibroblasts, suggesting its undeniable effect on tumor genesis and development (Affo et al., 2017). According to recent studies, CAFs can produce ECM in the TME that sustains tumors, encourages pre-tumor epithelial cell growth, expansion, and diffusion, fosters the emergence of newly malignant cells, and significantly impacts the progression of different organ tumors (Wang Y. et al., 2022; Sun et al., 2022). Through indirect cellular contact, CAFs can also control the biological activity of other mesenchymal and tumor cells, reshape and synthesize ECM, and release many regulatory factors, which can affect the development and progression of tumors (Zhang M. et al., 2022; Wu et al., 2022). In addition, it can reprogram lipid metabolism, release lipid metabolites, get absorbed into tumor cells, encourage migration, and so forth (Gong et al., 2020). Other mechanisms include immune regulation and extracellular secretion (Peng et al., 2023). CAFs have been shown to play an immunosuppressive role in the TME through the secretion of several key regulatory factors, including cytokines and chemokines. Cytokines involved include TGF-β, IL-6, IL-33, TGF-β-induced gene (TGFBI), cardiotrophin-like cytokine factor 1 (CLCF1), TNF, and IL-1β (Limoge et al., 2017; Karakasheva et al., 2018; Shani et al., 2020; Sato et al., 2021; Xiang et al., 2022). Chemokines such as CXCL12, CCL2, CCL17, and CXCL16 are also involved (Chun et al., 2015; Xiang et al., 2022; Zheng and Hao, 2023). The following text will elaborate on how exosomes secrete and govern malignancies.
Recently, researchers have become interested in CAFs that exhibit an aging phenotype. Under stress conditions, these cells can exhibit a senescence-associated secretory phenotype (SASP), leading to cancer progression and chemotherapy resistance. An interesting study in a mouse model found that CAFs with SASP can promote the formation of peritoneal tumors through the JAK/STAT3 signaling pathway. Furthermore, the presence of CAFs with SASP was discovered in the ascitic fluid of gastric cancer (GC) patients with peritoneal metastasis. The results suggest that CAFs with SASP may promote peritoneal dissemination of primary tumors and cancer progression (Yasuda et al., 2021). Another study demonstrated that the proliferative potential of pancreatic cancer (PC) may be associated with p53-mediated cellular senescence and CAFs with SASP (Higashiguchi et al., 2023). However, the role of aged CAFs in metastatic lesions and the molecular mechanisms induced by inflammation-related SASP still require further investigation.
In general, CAFs impact tumor formation in TME through invasion and metastasis promotion, angiogenesis, stem cell properties of cancer cells, and resistance to chemotherapy and radiation therapy. However, the unique internal mechanics of CAFs are not fully understood.
3 Exosome
3.1 Structure of exosome
In recent years, exosomes have garnered increasing attention in tumor research due to their role in transporting different active compounds from cells, influencing immune escape, tumorigenesis, and TME reconstruction (Dzobo and Dandara, 2020). Other types of cells can secrete exosomes, which are extracellular vesicles with a diameter ranging from 30 to 150 nm. They can be found in blood, breast milk, urine, nasal secretions, pleural effusion, bronchoalveolar lavage, and ascites, among other body fluids. Exosomes have a highly stable structure as their membrane lipid bilayers have rich ceramide, sphingomyelin, cholesterol content, and numerous transmembrane proteins. Exosomes contain proteins, lipids, DNA, and RNA (mRNA, miRNA, and other non-coding RNAs), which are crucial to the biological functions of exosomes (Affo et al., 2017; Rimal et al., 2022; Fang et al., 2023). Exosomes originate from within cells, and their biological processes primarily involve two main pathways: the endosomal and multivesicular body pathways (Arya et al., 2024). They mainly regulate receptor cells by two mechanisms. First, they interact with the target cell receptors and trigger associated signaling pathways. Second, their contents are released following endocytosis or fusion with the target cell’s plasma membrane, resulting in modifications to the receptor cell’s protein translation and related gene expression levels (Zhu et al., 2020; Rajabi et al., 2022).
3.2 Role of exosomes in tumor progression
Exosomes take part in growth and carcinogenesis through multiple processes. (1) They can enhance cellular communication with target cells by directly interacting with extracellular receptors to transmit signals or fusing with cell membranes to absorb or internalize them (Gurung et al., 2021). (2) Substantial evidence suggests that exosomes produced by tumors can accelerate tumor cell growth and alter the migration direction of recipient malignant cells. (3) Through metastatic treatment resistance, exosomes can guarantee communication between tumors (Mashouri et al., 2019). (4) In controlled conditions, the exosomes secreted by epithelial cells can act as messengers, delivering inflammatory signals to immune cells throughout the body (Yang et al., 2021). (5) Exosomes can regulate the immune system through molecular transport and signal transduction (Xie et al., 2022). They can also regulate immune cell activity, which in turn stimulates the immune system to release tumor cells (Taha et al., 2019; Daassi et al., 2020). (6) They can induce fibroblasts to express matrix metalloproteinases, facilitate matrix remodeling, and eventually encourage tumor invasion (Bai et al., 2023). (7) Exosomes may also have a role in forming CAFs (Wang et al., 2018). (8) Exosomes can also exert tumor suppressor effects due to the differences in their contents (Yang Z. et al., 2020).
Currently, studies examining the connection between exosomes and cancers are delving further to improve tumor diagnosis and treatment. Tumor and immune cells can create special exosomes that can be used directly in anti-tumor immunotherapy (Zhang et al., 2023). In the future, exosomes may also be best utilized as a cancer vaccine (He et al., 2018; Huda and Nurunnabi, 2022). They are also beneficial diagnostic markers, offering a new technique for early tumor diagnosis (Yang D. et al., 2020).
4 miRNA
4.1 Structure and production mechanism of miRNA
Since they have a greater prevalence in body tissues and fluids, significant impact on gene expression, and potential applications as disease biomarkers, miRNAs have become a fascinating topic for basic and translational biomedical research (Gjorgjieva et al., 2019). miRNA, a non-coding RNA of 22–26 nucleotides, constitutes 1% of the human genome’s total number of genes. By binding to the target gene’s untranslated 3′UTR region, it inhibits the target gene’s transcription, modifying gene expression levels and ultimately influencing intracellular homeostasis, a method by which eukaryotic cells control the gene transcription (Fuhrmann and Brune, 2022). miRNAs regulate many essential biological functions, including differentiation, apoptosis, proliferation, and metabolism. Several miRNAs have the potential to cause cancer and are strongly linked to tumor development, making them valuable as diagnostic markers and therapeutic targets (Breulmann et al., 2023). Primary miRNA, or pri-miRNA, is created when RNA polymerase II (RNA pol II) transcribes the miRNA genes inside and between genes. Drosha-DGCR8 then processes the pri-miRNA to pre-miRNA in the nucleus, which is then transported to the cytoplasm by Exportin5/RanGTP. This is the current understanding of the mechanism underlying the production and function of miRNAs. Dicer, a naturally occurring ribonuclease in the cytoplasm, further cleaves and converts the pre-miRNAs into mature, short, double-stranded RNA fragments (∼22 nt) called miRNAs. To exert its regulatory function, one strand of the mature miRNA duplex binds to the argonaute protein (AGO2) in RNA-induced silencing complex (RISC) and a complementary site in the 3′UTR of the target mRNA, resulting in translation inhibition. The second strand is degraded, leading to mRNA cleavage and generation of cytoplasmic processing bodies, known as P bodies (sites of mRNA decay and inhibition) (Iqbal et al., 2019; Long et al., 2023; Rezaee et al., 2023).
miRNA can exist in body fluids in various forms, and exosomes are one of the common packaging ways (Cortez et al., 2011). The sorting and packaging of miRNAs in exosomes is essential in comprehending vesicles’ role in cancer. Research has shown that stress-induced microenvironment can trigger miRNA trafficking and packaging into exosomes (Lee et al., 2017). Furthermore, evidence suggests that RNA-binding proteins play a synergistic role in the biogenesis of exosomes containing miRNAs. These include heterogeneous nuclear ribonucleoprotein-A2B1 (hnRNPA2B1) (Groot and Lee, 2020), MEX3C RNA binding protein (Lu et al., 2017), Y-box binding proteins (Shurtleff et al., 2016), Argonaute-2 (McKenzie et al., 2016), and membranous proteins such as caveolin-1 (Giannubilo et al., 2024).
4.2 Role of miRNA in tumors
The miRNAs expressed in cancer cells can participate in tumor progression through dual effects of carcinogenesis or tumor suppression (Hill and Tran, 2021). These processes can be achieved through epigenetic modifications, such as widespread genomic DNA hypomethylation (Ma et al., 2023) and histone acetylation (Liu Y. et al., 2022). In addition, transcription factors c-myc and p53 can also participate in tumor regulation through miRNA interactions (Parfenyev et al., 2021; Li Z. Y. et al., 2022; Kaller et al., 2022). The specific roles of miRNAs in tumors mainly include migration and invasion, tumor cell proliferation, and drug resistance. For example, miR-144-3p can induce iron deficiency by negatively regulating the expression of ZEB1, thereby inhibiting the proliferation, migration, and invasion of osteosarcoma (OS) cells (Jiang M. et al., 2023). miR-874-3p can participate in the migration, invasion, and proliferation of breast cancer cells by targeting voltage-dependent anion channel 1 (VDAC1) (Yang et al., 2023). miR-223-3p regulates ECT2 to promote GC proliferation, invasion, and metastasis through the Wnt/β-catenin signaling pathway (Li et al., 2023). Hence, it is evident that miRNAs are closely related to tumor development.
A large number of studies show that the expression profiles of miRNAs in cells under human disease conditions differ from those in normal conditions, indicating that miRNAs have the potential to become markers for disease diagnosis and provide a theoretical basis for disease liquid biopsy (Moro et al., 2023).
5 Function of CAF-derived exosomal miRNA in promoting tumorigenesis and development
5.1 Role in EMT
EMT was initially conceptualized as how tumor cells changed from an epithelial to a mesenchymal phenotype. According to recent studies, it also involves a continuous process known as partial EMT or intermediate mixed epithelial and mesenchymal (E/M) phenotypes (Taki et al., 2021). The process results in a loss of epithelial integrity and characteristics, the acquisition of mesenchymal properties, reduction in intercellular connections, decreased interactions with surrounding and stromal cells, and increased cell motility and migration (Dongre and Weinberg, 2019). Cancer overtakes this process to induce fundamental alterations in cell motility and shape, thereby facilitating invasion (Lu and Kang, 2019). Furthermore, research suggests that EMT orchestrates various complementary characteristics of cancer, including stem cell properties, tumorigenicity, metabolic reprogramming, treatment resistance, and the ability of tumor cells to adapt to changes in their microenvironment (Jiang et al., 2021; Qin et al., 2021; Manfioletti and Fedele, 2022). Numerous studies now demonstrate the involvement of miRNAs, along with factors such as vascular endothelial growth factor, WNT, Notch, PDGF, and epidermal growth factor, as well as external factors like alcohol and UV light, and pathological conditions including hypoxia in the TME, in the activation mechanism of the EMT process (Behbahani et al., 2017; Sugita et al., 2022; Yang et al., 2022).
Exosomal miRNAs generated from CAFs also play a role in this process. Globally, the second most prevalent cause of cancer-related mortality is colorectal cancer (CRC). Studies have demonstrated that exogenous miR-625-3p produced by CAFs may stimulate EMT in CRC cells by blocking the CELF2/WWOX pathway (Zhang Y. et al., 2022). Similarly, in CRC, exosomes produced from CAFs express more miR-92a-3p when the Wnt/β-catenin pathway is activated. Thus, this leads to EMT in CRC cells, inhibiting mitochondrial apoptosis and directly suppressing FBXW7 and MOAP1 (Hu et al., 2019), providing potential candidates for CRC prediction and treatment. Breast cancer tissue has low expression of tumor suppressor HOXA5. In vitro, its overexpression causes cancer cells to undergo EMT inhibition and accelerate apoptosis (Hussain et al., 2020; Lu et al., 2021). A study involving 122 patients with surgically removed cancerous tissues and corresponding paracancerous tissues revealed that miR-181d-5p in exosomes derived from CAFs can target transcription factors that bind to the HOXA5 promoter, thereby stimulating the growth of MCF-7 cells and inhibiting their apoptosis, thus playing a pivotal role in the environmental effects of tumors (Wang et al., 2020). Similarly, the exosomal miR-18b selectively binds to the transcription elongation factor TCEAL7’s 3′UTR region, activating NF-κB. It encourages nuclear Snails to become ectopically active, causing EMT in breast cancer cells (Yan et al., 2021). Furthermore, Josson et al. used laser capture anatomical microscopy to isolate the cancer-related prostate stromal fibroblasts and bone-related stromal models. The exosome-derived miR-409-3p and miR-409-5p specifically upregulated delta-like one homologous deiodinase, iodothyronine 3 (DLK1-DIO3), which was involved in the regulation of developmental and embryonic processes on human chromosome 14. These expressions inhibited genes suppressing tumor growth, such as Ras, promoting tumor induction and EMT in vitro and in vivo (Josson et al., 2015). Some studies have also reported on the EMT of tumors, as shown in Table 1 (Li et al., 2019) (Figure 2).
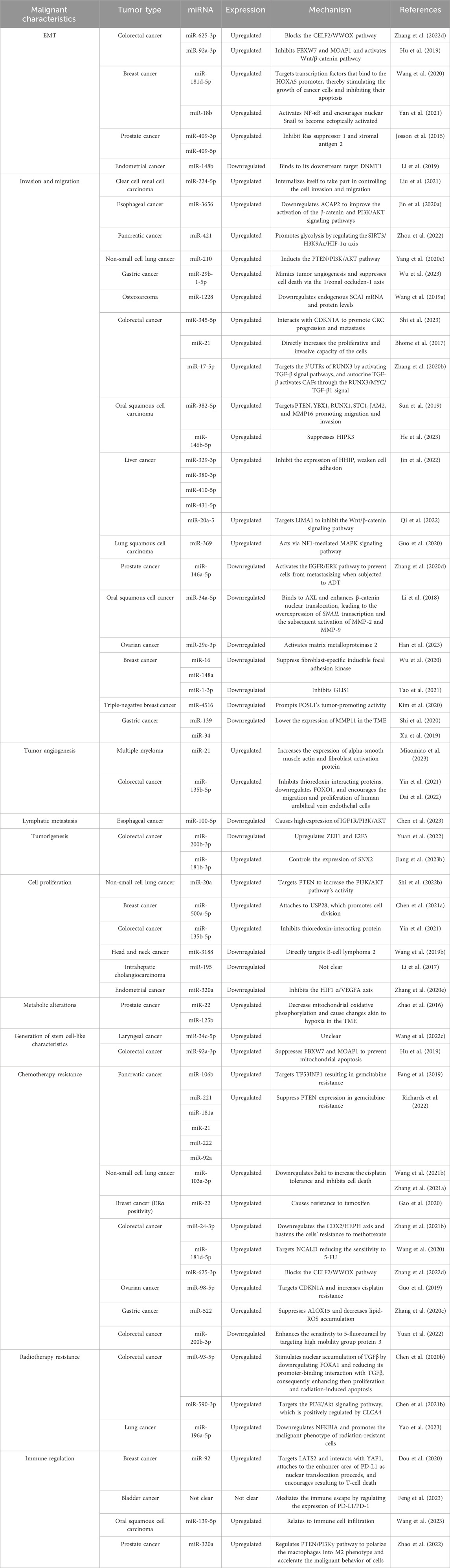
Table 1. Summary of CAF-derived exosomal miRNAs’ functions in the malignant characteristics of a tumor.
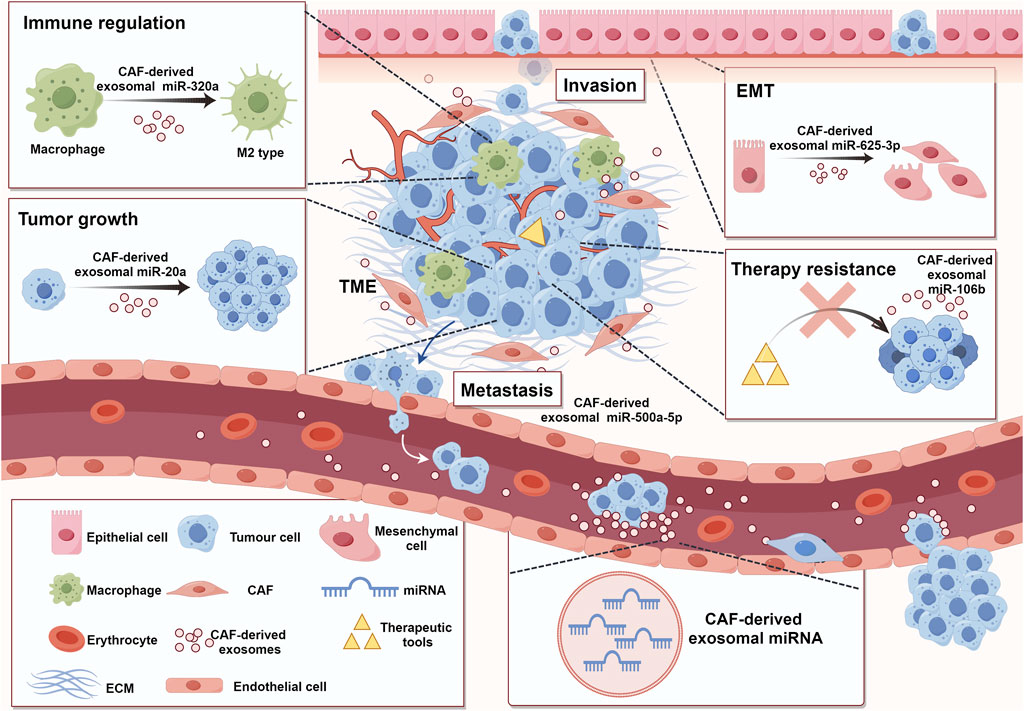
Figure 2. Functions of CAF-derived exosomal miRNA in promoting tumorigenesis and cancer development. TME comprises various cell types, including stromal cells, immune cells, tumor cells, and so on. All these cells were enveloped in ECM (Fang et al., 2023). CAFs, as an important component of TME, can participate in tumor regulation by secreting exosomal miRNAs (Wang J. W. et al., 2019). These miRNAs can take part in immune modulation (Zhao et al., 2022), tumor growth (Shi L. et al., 2022), migration and invasion (Chen B. et al., 2021), EMT (Zhang Y. et al., 2022), and treatment resistance (Fang et al., 2019) (ECM: extracellular matrix; TME: tumor microenvironment; EMT: epithelial-mesenchymal transition). (By Figdraw).
CAF-derived exosomes that affect tumor EMT are not only limited to miRNAs but also include related cytokines (Goulet et al., 2019; Al-Raimi et al., 2023), long-chain non-coding RNAs (Jin N. et al., 2020; Shi Z. et al., 2022; Zhang Y. et al., 2022), and proteins (Pan et al., 2022b; Liu X. et al., 2023). EMT is an important factor affecting tumor progression. Exploring its pathogenesis holds implications for the early diagnosis, treatment plans, and prognosis of patients with cancer. There is little research on the impact of CAF-derived exosomes on EMT, and further in-depth studies are needed in the future.
5.2 Role in tumor invasion and migration
As important indicators of the advancement of malignant tumors, invasion and migration have an impact on cancer prognosis and treatment. Wang et al. employed in vitro experiments and miRNA microarray analysis in an OS study to determine and validate the increase in miR-1228 levels in CAFs and their secreted exosomes, facilitating OS invasion and migration by downregulating endogenous SCAI mRNA and protein levels (Wang J. W. et al., 2019). Shi et al. found that miR-345-5p is a considerably elevated miRNA in exosomes derived from CAFs compared to exosomes obtained from normal fibroblasts. Through interaction with CDKN1A, exosomes mediate the transfer of miR-345-5p to CRC cells, promoting growth and metastasis (Shi et al., 2023). Simultaneously, CAF-derived exosomes are carriers of miR-21 that facilitate CRC transmission (Bhome et al., 2017). The ability to transfer highly expressed CAF-derived exosomal miRNAs to CRC cells, which then directly targets the 3′UTRs of the Runt-domain transcription factor 3 (RUNX3), was further demonstrated in an experiment. These exosomes also exhibited a higher miR-17-5p expression than exosomes derived from normal fibroblasts. Through its interaction with the MYC pro-oncogene and transforming growth factor-β1 (TGF-β1) at base pairs 1005–1296 promoter binding, RUNX3 promotes the progression of tumors by activating TGF-β signal pathways. Moreover, exosomal miR-17-5p is constantly released into CRC cells by autocrine TGF-β activating CAFs through the RUNX3/MYC/TGF-β1 signal, generating positive and negative feedback loops for CRC development (Zhang et al., 2020b). Oral squamous cell carcinoma (OSCC) is the most common malignant tumor in the head and neck region, with a high propensity for metastasis. Mauricio et al. in 2019 noted that extracellular vesicles formed from CAFs were crucial for encouraging OSCC cell migration and invasion (Dourado et al., 2019). In a subsequent investigation, Sun et al. found that OSCC overexpressed the CAF-derived exosomal miR-382-5p (Sun et al., 2019). According to a recent study, miR-146b-5p is also overexpressed in OSCC. Subsequent luciferase assay tests confirmed that it can specifically target the 3′UTR of HIPK3, leading to the suppression of HIPK3 and its involvement in the migration and invasion process of OSCC cells. (He et al., 2023). miRNAs in the DLK1-DIO3 imprinting area, including miR-329-3p, miR-380-3p, miR-410-5p, and miR-431-5p, were found to be upregulated in hepatocellular carcinoma (HCC) cells co-cultured with exosomes produced from CAFs. These miRNAs inhibited HHIP expression, weakened cell adhesion, and enabled cell migration and invasion (Jin et al., 2022). In HCC, miR-20a-5p has been demonstrated to have a comparable impact (Qi et al., 2022). Other studies about CAF-derived exosomal miRNAs’ role in invasion and migration are shown in Table 1 (Xu et al., 2019; Yang F. et al., 2020; Guo et al., 2020; Shi et al., 2020; Liu et al., 2021; Zhou et al., 2022; Wu et al., 2023).
Tumor angiogenesis is an important form of nutrient acquisition and metastasis in tumor cells (Bhat et al., 2021). Researchers have found that exosomal miRNAs derived from CAFs can also participate in the process of promoting tumor angiogenesis. For example, CAF-derived exosomes promote angiogenesis by delivering miR-21 to endothelial cells in multiple myeloma (Miaomiao et al., 2023). miR-135b-5p is upregulated, promoting angiogenesis in CRC cells by inhibiting thioredoxin-interacting proteins (Yin et al., 2021). Similarly, miR-135b-5p downregulates FOXO1 in colorectal adenocarcinoma and encourages the migration and proliferation of human umbilical vein endothelial cells, resulting in angiogenesis (Dai et al., 2022). Lymphatic metastasis is another important method of tumor metastasis besides hematogenous metastasis. The reduction of CAF-derived exosomal miR-100-5p in esophageal squamous cell carcinoma (ESCC) may promote the growth, migration, and invasion of tumor-related lymphatic endothelial cells by increasing the expression of IGF1R/PI3K/AKT, ultimately leading to the development of lymphatic vessels (Chen et al., 2023) (Figure 2).
5.3 Role in tumor growth
As the primary constituents of the cancer matrix, CAFs can secrete exosomes to affect the processes relevant to tumor growth regulation (Figure 2). The regulatory process of tumor growth includes aspects such as cell proliferation, reprogramming of cell metabolism, and obtaining the stem cell phenotype.
The exosomal miRNAs generated from CAFs influence the growth of tumor cells. In non-small cell lung cancer, exosomes derived from the CAFs express miR-20a more frequently, which serves as a conduit to infiltrate tumor cells. Targeting PTEN increases the PI3K/AKT pathway’s activity, promoting proliferation (Shi L. et al., 2022). Cui et al. conducted a study where they incubated ESCC cell lines (TE-1 and KYSE-150) with exosomes formed from CAFs. The results indicated that increased RIG-I/IFN-β expression could increase cell proliferation. Additionally, they observed that exosomes derived from CAFs prevented cell apoptosis (Cui et al., 2022). A previous study suggested that miRNAs could be crucial to cellular apoptosis, warranting further investigation in subsequent studies (Jin et al., 2021). In breast cancer, miR-500a-5p can migrate from CAFs to cancer cells via exosomes. Once inside the cancer cells, it attaches itself to USP28, a ubiquitin-specific peptidase, to promote cell division (Chen B. et al., 2021). It was discovered in CRC that miR-135b-5p stimulated tumor cell proliferation and angiogenesis (Yin et al., 2021). Furthermore, miR-181b-3p is involved in colorectal tumorigenesis (Jiang Y. et al., 2023).
The metabolism of tumor cells exhibits significant departures from that of healthy cells, a deviation that underlies the emergence of a wide array of malignant tumor symptoms (Lv et al., 2021; Zanotelli et al., 2021; Pavlova et al., 2022). In a study by Zhao et al., exosomes derived from prostate cancer (PCa) CAFs had higher levels of miR-22 and miR-125b, which decreased mitochondrial oxidative phosphorylation and caused changes akin to hypoxia in the TME that can result in metabolic alterations in cancer cells (Zhao et al., 2016).
Tumor cells have strong plasticity (Chiodi and Mondello, 2020). The generation of stem cell-like characteristics is an important driving factor for tumor cells to self-renew, have clonal tumor initiation ability, and possess long-term proliferation potential (Han et al., 2020; Huang et al., 2020; Suva and Tirosh, 2020; Kumar et al., 2022). In exosomes secreted by CAFs, miR-34c-5p expression was significantly reduced in laryngeal carcinoma. In vivo and in vitro research has shown that miR-34c-5p regulates the stem cell-like characteristics of laryngeal cancer cells (Wang et al., 2022c). When the Wnt/β-catenin pathway was activated in CRC, the expression of CAF-derived exosomal miR-92a-3p rose and directly suppressed FBXW7 and MOAP1 expression to prevent mitochondrial apoptosis, causing CRC cells to produce stem cell characteristics (Hu et al., 2019). This can significantly impact the cancerous characteristics of tumors, including their ability to proliferate, invade, alter their shape and form plate colonies, become tumorigenic, and express high levels of tumor stem cell genes that are important for tumor development (Machida, 2020).
5.4 Role in therapy resistance
Although there have been notable advancements in anti-cancer treatment, drug resistance associated with molecular and clinical recurrence remains prevalent. As a result, many patients resort to different treatment approaches, yet their prognosis remains unfavorable. Innate and/or acquired resistance mechanisms severely restrict the clinical efficacy of anti-cancer therapy (Hofmann et al., 2023). Thus, it is imperative to investigate the precise mechanisms of treatment resistance in clinical practice to create significant changes in tumor treatment modalities. Understanding these pathways may aid in forecasting the development of clinical drug resistance and identifying alternate therapeutic approaches. Exosomal miRNAs derived from CAFs have been implicated in tumor therapy resistance (Figure 2), providing valuable insights into resistance mechanisms from the TME perspective.
Exosomal miRNAs produced from CAFs may contribute to developing chemotherapy resistance in tumors. Gemcitabine (GEM) is frequently used to treat PC (Motoi et al., 2019; Beutel and Halbrook, 2023; Jiang X. et al., 2023). However, individuals with PC still face the difficulty of developing resistance to GEM (Liang et al., 2017). According to a study by Fang et al. on the role of exosomal miRNAs from CAFs in regulating drug resistance, CAFs are intrinsically resistant to GEM. Subsequent GEM therapy demonstrated elevated miR-106b levels in CAFs and CAF exosomes directly targeting TP53INP1, resulting in GEM resistance in cancer cells (Fang et al., 2019). Richards et al. identified five different forms of short RNAs in another experiment: miR-221, miR-181a, miR-21, miR-222, and miR-92a. During GEM therapy, these miRNAs considerably increased the production of CAF-derived exosomes targeting PTEN. In vitro investigations demonstrated that these CAF exosomes might suppress PTEN expression (Richards et al., 2022). The experiment found that CAFs derived from non-small cell lung cancer (NSCLC) exhibit inherent resistance to cisplatin treatment (Kryczka et al., 2021; Shi L. et al., 2022). CAF-derived exosomes play a role in developing chemotherapy resistance in NSCLC cells by moving from CAFs to NSCLC cells. However, this chemotherapy resistance can be reversed by suppressing the miR-103a-3p gene. Subsequent studies have shown that miR-103a-3p directly targets Bak1. Downregulating Bak1 increases cisplatin tolerance in NSCLC cells and inhibits cell death. Furthermore, miR-103a-3p can be packaged into exosomes by the RNA-binding protein PUM2 (Zhang T. et al., 2021; Wang H. et al., 2021). For breast cancer with estrogen receptor alpha (ERα) positivity, tamoxifen is still the most effective treatment. However, a significant proportion of patients still face metastases and recurrence, along with resistance to tamoxifen, which poses significant therapeutic challenges. In a previous study, Gao et al. found that CD63 + CAFs caused patients with breast cancer to become resistant to tamoxifen by secreting miR-22. This suggested that CD63 + CAFs could be a potential target for therapy to improve tamoxifen sensitivity (Gao et al., 2020). Further details about the role of therapy resistance are provided in Table 1 (Guo et al., 2019; Zhang H. et al., 2020; Gao et al., 2020; Zhang H. W. et al., 2021).
Radiotherapy, an important tumor treatment method, has demonstrated therapeutic effects influenced by miRNAs in CAF-derived exosomes. High miR-93-5p CAF-derived exosomes stimulate the nuclear accumulation of TGFβ by downregulating FOXA1 and reducing its promoter-binding interaction with TGFβ, consequently enhancing the proliferation and shielding of CRC SW480 cells from radiation-induced apoptosis (Chen X. et al., 2020). Another study found that miR-590-3p can boost the radiation resistance of CRC by targeting the PI3K/Akt signaling pathway, which is positively regulated by CLCA4 (Chen X. et al., 2021). In lung cancer, CAF-derived exosomal miR-196a-5p downregulates NFKBIA, promoting the malignant phenotype of radiation-resistant cells and contributing to radiation resistance (Yao et al., 2023).
Studying the mechanism of tumor therapy resistance mediated by CAF-derived exosomal miRNAs can provide new targets for improving the sensitivity of tumor cells to radiotherapy and chemotherapy. However, current studies mainly focus on resistance to radiotherapy and chemotherapy, while studies on biological targeted therapy, immunotherapy, and resistance caused by tumor cell heterogeneity have not yet emerged. Thus, these may be good research directions, necessitating further exploration in the future.
5.5 Role in immune regulation
Cancer is a complex ecosystem in which the interaction between cancer and host cells can affect the disease progression and treatment response. Besides cancer cells, immune cells are arguably the most complex players in solid tumors, and their activity can range from anti-tumorigenic to tumorigenic (Dumauthioz et al., 2018; Oliveira and Wu, 2023). Cancer cells employ diverse strategies to thwart immunological defenses as their tumors grow, including downregulating the antigen presentation pathway and triggering the production of immune checkpoint molecules that suppress immune responses (Jhunjhunwala et al., 2021). In addition, cancer cells exploit immune cells such as neutrophils, macrophages, and regulatory T cells to create an immunosuppressive TME (Lei et al., 2020). This, in turn, promotes immune escape, remodels the ECM, and supports cancer progression and treatment resistance. Therefore, abnormal immune responses are considered as markers for cancer (Khalaf et al., 2021; Mafi et al., 2021; Peng et al., 2022). Recent research has also demonstrated that miRNAs in exosomes produced from CAFs regulate the tumor immune system and offer therapeutic targets for cancer.
A recent experiment discovered a novel mechanism for inducing immunosuppression in the TME. Increased expression of miR-92 and greater levels of programmed cell death receptor ligand 1 (PD-L1) were detected in breast cancer cells treated with CAF-derived exosomes produced from human breast cancer cells (Feng et al., 2021). LATS2 is the miR-92 target gene, and immunoprecipitation has demonstrated the interaction between LATS2 and YAP1 (Jin D. et al., 2020). Tumor transcriptional activity was increased when YAP1 was attached to the enhancer area of PD-L1 as nuclear translocation proceeded. To a certain extent, this effect hampered the proliferation and strongly encouraged T-cell death (Dou et al., 2020). In bladder cancer, CAF-derived exosomes have also been confirmed to mediate the immune evasion of cancer cells by modulating PD-L1/PD-1 expression (Feng et al., 2023). However, the specific molecules that play a role in the exosomes still need to be further explored, and the participation of miRNAs is a potential research direction. The immune regulatory pathway may be a special mechanism by which CAFs promote OSCC proliferation. Wang et al. conducted bioinformatics analysis on foreign sequencing data from CAF sources and identified all the candidate genes related to tumor immunity. Hsa-miR-139-5p is one of the target genes, and its expression differences and prognostic significance are well-established in the TCGA dataset. The study found that has-miR-139-5p was related to immune cell infiltration, and its low expression promotes the development of OSCC, which is closely related to patient survival (Wang et al., 2023). M2-type macrophages are activated by cytokines such as IL-4 and IL-13. They have an enhanced ability to engulf particles due to the secretion of anti-inflammatory cytokines, including TGF-β and IL-10. These cytokines promote the generation of Th2 cells and contribute to immune regulation and angiogenesis processes. Consequently, they facilitate the rapid dissemination of tumors (Chen D. et al., 2021; Christofides et al., 2022). CAF-derived exosomal miR-320a has been shown to regulate the PTEN/PI3Kγ pathway to polarize the macrophages into M2 phenotype, promoting the malignant behavior of PCa cells (Zhao et al., 2022) (Figure 2). Tumor immune regulation is crucial for studying the mechanisms of tumor occurrence in the body, and it is of great significance in the development of tumor treatment methods, the generation of treatment resistance, and patient prognosis. CAF-derived exosomal miRNAs have shown excellent research value in this field. With the deepening of various studies, new targets for tumor treatment are likely to emerge.
6 Tumor inhibitory effect of CAF-derived exosomal miRNAs
Extensive research has demonstrated the considerable tumor-suppressive effects of several CAF-derived exosomal miRNAs across tumor types.
Androgen deprivation therapy (ADT) is the cornerstone treatment for advanced PCa (Shafi et al., 2013). Despite the initial good response, castration resistance and metastatic progression inevitably occur (Li Q. et al., 2021). Through the EGFR/ERK pathway, exosomal miR-146a-5p produced from CAFs can prevent PCa cells from metastasizing when treated with ADT (Zhang et al., 2020d). In OSCC, exosomes derived from CAFs exhibited reduced expression of miR-34a-5p. In xenograft trials, OSCC cell carcinogenesis can be prevented by overexpressing miR-34a-5p in CAFs. It was also demonstrated that miR-34a-5p could bind to its direct downstream target AXL, and prevent the proliferation and metastasis of OSCC cells (Li et al., 2018). Peritoneal metastases are frequent and an extensive hallmark of ovarian cancer (OC) (Pascual-Anton et al., 2021). Overexpression of miR-29c-3p in exosomes produced from CAF suppresses tumor metastasis by amplifying its impact on the direct target, matrix metalloproteinase 2 (MMP2) (Han et al., 2023). Studies on breast cancer have shown that the concentrated presence of miR-16 and miR-148a in exosomes derived from CAFs has beneficial effects on anti-tumor cell activity and anti-metastasis (Wu et al., 2020). Similarly, miR-4516 targeted FOSL1’s tumor-promoting activity to inhibit triple-negative breast cancer (Kim et al., 2020). CAF-derived exosomal miRNA also plays a certain role in enhancing drug sensitivity. For example, miR-200b-3p was upregulated in exosomes derived from hypoxic CAFs, improving the sensitivity of CRC to 5-fluorouracil by targeting high mobility group protein 3 (Yuan et al., 2022). In addition, miR-195 in CAF-derived exosomes improved the survival rate in rat models of intrahepatic cholangiocarcinoma (Li et al., 2017). The inhibition of miR-320a via the HIF1 α/VEGFA axis on endometrial cancer has also been verified (Zhang N. et al., 2020). miR-3188 has also been proven to engage in the proliferation of head and neck cancer cells (Wang X. et al., 2019), and miR-1-3p has been shown to inhibit migration and invasion of breast cancer cells (Tao et al., 2021).
The multifaceted roles of miRNA in cancer development and its treatment vary significantly among different types of tumors. Therefore, it is essential to understand the specific types and mechanisms of miRNA to effectively diagnose and treat tumors (Table 1).
7 The potential of CAF-derived exosomal miRNAs in diagnosis and treatment
CAF-derived exosomal miRNAs have corresponding roles in tumor cell growth, migration and invasion, EMT, immune regulation, and treatment. These findings indicate their rich potential in tumor early diagnosis, treatment, and prognosis prediction.
Regarding diagnosis, the distinct composition of miRNAs in exosomes from tumor and normal tissues allows for identifying biomarkers. This is possible because exosomes are found in various bodily fluids. Diagnostic criteria can be established by analyzing the miRNAs that are significantly altered in the specific tumors. The patient’s discomfort can be reduced using non-invasive technology to collect bodily fluids, separate exosomes for miRNA identification, and determine the type of tumor. Moreover, they can serve as an early detection method in scenarios where pathology and imaging fail to yield valuable insights regarding microtumors (Gerloff et al., 2022; Takizawa et al., 2022). Hiroshi and his colleagues demonstrated that matrix miR-21 is more crucial for the progression of GC than tumor cell miR-21 (Uozaki et al., 2014). Additionally, CAF-derived miRNAs help with clinical tumor staging to create more individualized diagnoses and treatment regimens. A study has demonstrated that miR-92a-3p is highly expressed in CAF-derived exosomes in liver cancer patients with a diameter greater than 5 cm, and it is more expressed in patients with BCLC B/c phase (Liu X. et al., 2022). A strong theoretical foundation for liquid biopsy can also be provided by investigating CAF-derived exosome miRNAs. Exosomes can potentially be therapeutic agents due to their superior biocompatibility and circulatory stability.
Exosome-derived miRNAs have attracted a lot of interest in tumor treatment studies due to the specificity of their structure and mode of action. However, recent research primarily focused on the exosomal miRNAs from tumor cells (Jabbari et al., 2020; Kok and Yu, 2020; Huang et al., 2022; Wan et al., 2022), with relatively little investigation into the origins of CAFs. Due to their direct effects on genetic material, it is evident that CAF-generated exosomal miRNAs have significant potential in tumor treatment, as indicated in this paper. Given their robust structure, exosomes can efficiently transport processed miRNAs as nanocarriers, decreasing the likelihood of degradation (Zhang et al., 2022e). This characteristic makes CAF-derived exosomes crucial for biological information transfer within TME (Peng et al., 2021). Following absorption by adjacent receptor cells, these receptor cells undergo a sequence of events, including controlling signal pathways and targeting particular genes. This method substantially impacts drug resistance, immunological response, metastasis, and tumor cell proliferation.
Based on the current evidence, exploring CAF-derived exosomes holds promise for the development of innovative methods for tumor diagnosis and therapy.
8 Discussion
Previous studies on cancers have primarily focused on tumor cells. Recently, researchers started to investigate the relationship between different components of TME and malignancies, in addition to cancer cells. CAF-derived exosomes, a key TME mediator, are essential for developing and spreading cancer and can be considered as a channel for information exchange within TME. Due to their influence on gene transcription and expression control, miRNAs—which are extensively found in body fluids and tissues—have also drawn much attention related to tumor growth studies (Gjorgjieva et al., 2019). In the last 5 years, there has been a notable shift in the emphasis of research concerning the effects of exosomal miRNAs derived from CAFs on tumors. Presently, a multitude of studies are in the advanced stages of development. It is known that exosomal miRNAs produced from CAFs have equivalent functions in the development, invasion, and migration of tumor cells apart from EMT, immunological control, and therapy. These imply that the miRNAs have rich development prospects in early tumor diagnosis, treatment, and prognosis prediction.
Nevertheless, research on the application of CAF-derived exosomes as biomarkers in diagnosis and treatment still faces some challenges. First, no established extraction method reliably produces exosomes with high levels of purity when it comes to exosome separation and purification techniques. Moreover, the integrity processing of exosomes obtained from the existing processes is not well-quantified, which may greatly affect their accuracy and efficiency in diagnosis. Second, the complex characteristics of TME require further investigation to determine the exact mechanism through which miRNAs obtained from CAF-derived exosomes interact with tumor cells, thereby influencing the behavior of cancerous tumors. In addition, more investigations are required to ascertain the tumor-specificity of miRNAs in CAF-derived exosomes extracted from various sources. Third, there is a lack of clear experimental evidence regarding the capacity of exosomes to consistently remain present in bodily fluids and generate potent therapeutic effects. Thus, CAF-derived exosomal miRNAs remain viable and efficient targets for cancer therapy due to their distinct function in malignancies.
9 Conclusion
This study focused on elucidating the functions of miRNAs produced from CAFs in promoting malignant features in tumors, including EMT, migration and invasion, tumor growth, treatment resistance, and immunomodulation. Additionally, we emphasized the inhibitory effect of CAF-derived exosomal miRNAs.
We emphasize that with comprehensive and creative research, the functions of CAF-derived exosomal miRNAs in tumor progression can be further clarified, providing a robust theoretical framework for clinical diagnosis and treatment applications. This will relieve patients' intense psychological and physical suffering, in addition to the substantial financial strain that malignant tumors impose.
Author contributions
ZG: Software, Visualization, Writing–original draft, Writing–review and editing. JnL: Writing–original draft, Writing–review and editing. JmL: Supervision, Writing–review and editing. NY: Writing–review and editing.
Funding
The author(s) declare that financial support was received for the research, authorship, and/or publication of this article. This work was supported by the Science and Technology Department of Jilin Province (grant number YDZJ202201ZYTS004) and the National Natural Science Foundation of China (grant number 32000953). All sources of funding received for the research have been submitted.
Acknowledgments
We used Figdraw (https://www.figdraw.com/#/) to create our figures.
Conflict of interest
The authors declare that the research was conducted in the absence of any commercial or financial relationships that could be construed as a potential conflict of interest.
Publisher’s note
All claims expressed in this article are solely those of the authors and do not necessarily represent those of their affiliated organizations, or those of the publisher, the editors and the reviewers. Any product that may be evaluated in this article, or claim that may be made by its manufacturer, is not guaranteed or endorsed by the publisher.
References
Aden, D., Zaheer, S., Ahluwalia, H., and Ranga, S. (2023). Cancer-associated fibroblasts: is it a key to an intricate lock of tumorigenesis? Cell Biol. Int. 47 (5), 859–893. doi:10.1002/cbin.12004
Affo, S., Yu, L. X., and Schwabe, R. F. (2017). The role of cancer-associated fibroblasts and fibrosis in liver cancer. Annu. Rev. Pathol. 12, 153–186. doi:10.1146/annurev-pathol-052016-100322
Ali, R., Shao, H., and Varamini, P. (2022). Potential nanotechnology-based therapeutics to prevent cancer progression through TME cell-driven populations. Pharmaceutics 15 (1), 112. doi:10.3390/pharmaceutics15010112
Alizadeh, M., Safarzadeh, A., Beyranvand, F., Ahmadpour, F., Hajiasgharzadeh, K., Baghbanzadeh, A., et al. (2019). The potential role of miR-29 in health and cancer diagnosis, prognosis, and therapy. J. Cell Physiol. 234 (11), 19280–19297. doi:10.1002/jcp.28607
Al-Raimi, H. A. I., Kong, J., Ran, Y., Zhu, L., Li, J., Liu, X., et al. (2023). Extracellular vesicles from carcinoma-associated fibroblasts promote EMT of salivary adenoid cystic carcinoma via IL-6. Arch. Med. Res. 54 (1), 27–36. doi:10.1016/j.arcmed.2022.12.005
Arya, S. B., Collie, S. P., and Parent, C. A. (2024). The ins-and-outs of exosome biogenesis, secretion, and internalization. Trends Cell Biol. 34 (2), 90–108. doi:10.1016/j.tcb.2023.06.006
Bai, X., Shao, J., Duan, T., Liu, X., Wang, M., Li, X., et al. (2023). Exo-miR-1290-induced by COX-2 overexpression promotes cancer-associated fibroblasts activation and tumor progression by CUL3-Nrf2 pathway in lung adenocarcinoma. Cell Commun. Signal 21 (1), 242. doi:10.1186/s12964-023-01268-0
Bartoschek, M., Oskolkov, N., Bocci, M., Lövrot, J., Larsson, C., Sommarin, M., et al. (2018). Spatially and functionally distinct subclasses of breast cancer-associated fibroblasts revealed by single cell RNA sequencing. Nat. Commun. 9 (1), 5150. doi:10.1038/s41467-018-07582-3
Behbahani, G. D., Ghahhari, N. M., Javidi, M. A., Molan, A. F., Feizi, N., and Babashah, S. (2017). MicroRNA-mediated post-transcriptional regulation of epithelial to mesenchymal transition in cancer. Pathol. Oncol. Res. 23 (1), 1–12. doi:10.1007/s12253-016-0101-6
Beutel, A. K., and Halbrook, C. J. (2023). Barriers and opportunities for gemcitabine in pancreatic cancer therapy. Am. J. Physiol. Cell Physiol. 324 (2), C540–C552. doi:10.1152/ajpcell.00331.2022
Bhat, S. M., Badiger, V. A., Vasishta, S., Chakraborty, J., Prasad, S., Ghosh, S., et al. (2021). 3D tumor angiogenesis models: recent advances and challenges. J. Cancer Res. Clin. Oncol. 147 (12), 3477–3494. doi:10.1007/s00432-021-03814-0
Bhome, R., Goh, R. W., Bullock, M. D., Pillar, N., Thirdborough, S. M., Mellone, M., et al. (2017). Exosomal microRNAs derived from colorectal cancer-associated fibroblasts: role in driving cancer progression. Aging (Albany NY) 9 (12), 2666–2694. doi:10.18632/aging.101355
Bilotta, M. T., Antignani, A., and Fitzgerald, D. J. (2022). Managing the TME to improve the efficacy of cancer therapy. Front. Immunol. 13, 954992. doi:10.3389/fimmu.2022.954992
Brechbuhl, H. M., Finlay-Schultz, J., Yamamoto, T. M., Gillen, A. E., Cittelly, D. M., Tan, A. C., et al. (2017). Fibroblast subtypes regulate responsiveness of luminal breast cancer to estrogen. Clin. Cancer Res. 23 (7), 1710–1721. doi:10.1158/1078-0432.CCR-15-2851
Breulmann, F. L., Hatt, L. P., Schmitz, B., Wehrle, E., Richards, R. G., Della Bella, E., et al. (2023). Prognostic and therapeutic potential of microRNAs for fracture healing processes and non-union fractures: a systematic review. Clin. Transl. Med. 13 (1), e1161. doi:10.1002/ctm2.1161
Buechler, M. B., and Turley, S. J. (2018). A short field guide to fibroblast function in immunity. Semin. Immunol. 35, 48–58. doi:10.1016/j.smim.2017.11.001
Chen, B., Sang, Y., Song, X., Zhang, D., Wang, L., Zhao, W., et al. (2021a). Exosomal miR-500a-5p derived from cancer-associated fibroblasts promotes breast cancer cell proliferation and metastasis through targeting USP28. Theranostics 11 (8), 3932–3947. doi:10.7150/thno.53412
Chen, C., Yang, C., Tian, X., Liang, Y., Wang, S., Wang, X., et al. (2023). Downregulation of miR-100-5p in cancer-associated fibroblast-derived exosomes facilitates lymphangiogenesis in esophageal squamous cell carcinoma. Cancer Med. 12 (13), 14468–14483. doi:10.1002/cam4.6078
Chen, D., Zhang, X., Li, Z., and Zhu, B. (2021c). Metabolic regulatory crosstalk between tumor microenvironment and tumor-associated macrophages. Theranostics 11 (3), 1016–1030. doi:10.7150/thno.51777
Chen, X., Liu, J., Zhang, Q., Liu, B., Cheng, Y., Zhang, Y., et al. (2020b). Exosome-mediated transfer of miR-93-5p from cancer-associated fibroblasts confer radioresistance in colorectal cancer cells by downregulating FOXA1 and upregulating TGFB3. J. Exp. Clin. Cancer Res. 39 (1), 65. doi:10.1186/s13046-019-1507-2
Chen, X., Liu, Y., Zhang, Q., Liu, B., Cheng, Y., Zhang, Y., et al. (2021b). Exosomal miR-590-3p derived from cancer-associated fibroblasts confers radioresistance in colorectal cancer. Mol. Ther. Nucleic Acids 24, 113–126. doi:10.1016/j.omtn.2020.11.003
Chen, X., and Song, E. (2019). Turning foes to friends: targeting cancer-associated fibroblasts. Nat. Rev. Drug Discov. 18 (2), 99–115. doi:10.1038/s41573-018-0004-1
Chen, Z., Zhou, L., Liu, L., Hou, Y., Xiong, M., Yang, Y., et al. (2020a). Single-cell RNA sequencing highlights the role of inflammatory cancer-associated fibroblasts in bladder urothelial carcinoma. Nat. Commun. 11 (1), 5077. doi:10.1038/s41467-020-18916-5
Chiodi, I., and Mondello, C. (2020). Life style factors, tumor cell plasticity and cancer stem cells. Mutat. Res. Rev. Mutat. Res. 784, 108308. doi:10.1016/j.mrrev.2020.108308
Christofides, A., Strauss, L., Yeo, A., Cao, C., Charest, A., and Boussiotis, V. A. (2022). The complex role of tumor-infiltrating macrophages. Nat. Immunol. 23 (8), 1148–1156. doi:10.1038/s41590-022-01267-2
Chu, X., Yang, Y., and Tian, X. (2022). Crosstalk between pancreatic cancer cells and cancer-associated fibroblasts in the tumor microenvironment mediated by exosomal MicroRNAs. Int. J. Mol. Sci. 23 (17), 9512. doi:10.3390/ijms23179512
Chun, E., Lavoie, S., Michaud, M., Gallini, C. A., Kim, J., Soucy, G., et al. (2015). CCL2 promotes colorectal carcinogenesis by enhancing polymorphonuclear myeloid-derived suppressor cell population and function. Cell Rep. 12 (2), 244–257. doi:10.1016/j.celrep.2015.06.024
Cortez, M. A., Bueso-Ramos, C., Ferdin, J., Lopez-Berestein, G., Sood, A. K., and Calin, G. A. (2011). MicroRNAs in body fluids--the mix of hormones and biomarkers. Nat. Rev. Clin. Oncol. 8 (8), 467–477. doi:10.1038/nrclinonc.2011.76
Cui, Y., Zhang, S., Hu, X., and Gao, F. (2022). Tumor-associated fibroblasts derived exosomes induce the proliferation and cisplatin resistance in esophageal squamous cell carcinoma cells through RIG-I/IFN-β signaling. Bioengineered 13 (5), 12462–12474. doi:10.1080/21655979.2022.2076008
Curtius, K., Wright, N. A., and Graham, T. A. (2018). An evolutionary perspective on field cancerization. Nat. Rev. Cancer 18 (1), 19–32. doi:10.1038/nrc.2017.102
Daassi, D., Mahoney, K. M., and Freeman, G. J. (2020). The importance of exosomal PDL1 in tumour immune evasion. Nat. Rev. Immunol. 20 (4), 209–215. doi:10.1038/s41577-019-0264-y
Dai, X., Xie, Y., and Dong, M. (2022). Cancer-associated fibroblasts derived extracellular vesicles promote angiogenesis of colorectal adenocarcinoma cells through miR-135b-5p/FOXO1 axis. Cancer Biol. Ther. 23 (1), 76–88. doi:10.1080/15384047.2021.2017222
Das, S., Shapiro, B., Vucic, E. A., Vogt, S., and Bar-Sagi, D. (2020). Tumor cell-derived IL1β promotes desmoplasia and immune suppression in pancreatic cancer. Cancer Res. 80 (5), 1088–1101. doi:10.1158/0008-5472.CAN-19-2080
Dongre, A., and Weinberg, R. A. (2019). New insights into the mechanisms of epithelial-mesenchymal transition and implications for cancer. Nat. Rev. Mol. Cell Biol. 20 (2), 69–84. doi:10.1038/s41580-018-0080-4
Dou, D., Ren, X., Han, M., Xu, X., Ge, X., Gu, Y., et al. (2020). Cancer-associated fibroblasts-derived exosomes suppress immune cell function in breast cancer via the miR-92/PD-L1 pathway. Front. Immunol. 11, 2026. doi:10.3389/fimmu.2020.02026
Dourado, M. R., Korvala, J., Åström, P., De Oliveira, C. E., Cervigne, N. K., Mofatto, L. S., et al. (2019). Extracellular vesicles derived from cancer-associated fibroblasts induce the migration and invasion of oral squamous cell carcinoma. J. Extracell. Vesicles 8 (1), 1578525. doi:10.1080/20013078.2019.1578525
Doyle, L. M., and Wang, M. Z. (2019). Overview of extracellular vesicles, their origin, composition, purpose, and methods for exosome isolation and analysis. Cells 8 (7), 727. doi:10.3390/cells8070727
Dumauthioz, N., Labiano, S., and Romero, P. (2018). Tumor resident memory T cells: new players in immune surveillance and therapy. Front. Immunol. 9, 2076. doi:10.3389/fimmu.2018.02076
Dzobo, K., and Dandara, C. (2020). Architecture of cancer-associated fibroblasts in tumor microenvironment: mapping their origins, heterogeneity, and role in cancer therapy resistance. OMICS 24 (6), 314–339. doi:10.1089/omi.2020.0023
Elyada, E., Bolisetty, M., Laise, P., Flynn, W. F., Courtois, E. T., Burkhart, R. A., et al. (2019). Cross-species single-cell analysis of pancreatic ductal adenocarcinoma reveals antigen-presenting cancer-associated fibroblasts. Cancer Discov. 9 (8), 1102–1123. doi:10.1158/2159-8290.CD-19-0094
Fang, Y., Zhou, W., Rong, Y., Kuang, T., Xu, X., Wu, W., et al. (2019). Exosomal miRNA-106b from cancer-associated fibroblast promotes gemcitabine resistance in pancreatic cancer. Exp. Cell Res. 383 (1), 111543. doi:10.1016/j.yexcr.2019.111543
Fang, Z., Meng, Q., Xu, J., Wang, W., Zhang, B., Liu, J., et al. (2023). Signaling pathways in cancer-associated fibroblasts: recent advances and future perspectives. Cancer Commun. (Lond) 43 (1), 3–41. doi:10.1002/cac2.12392
Fanhchaksai, K., Okada, F., Nagai, N., Pothacharoen, P., Kongtawelert, P., Hatano, S., et al. (2016). Host stromal versican is essential for cancer-associated fibroblast function to inhibit cancer growth. Int. J. Cancer 138 (3), 630–641. doi:10.1002/ijc.29804
Feng, R., Li, Z., Ge, G., Wang, C., Jia, Y., and Ouyang, J. (2023). Cancer-associated fibroblast-derived extracellular vesicles mediate immune escape of bladder cancer via PD-L1/PD-1 expression. Endocr. Metab. Immune Disord. Drug Targets 23 (11), 1410–1420. doi:10.2174/1871530323666230228124125
Feng, S., Sun, H., and Zhu, W. (2021). MiR-92 overexpression suppresses immune cell function in ovarian cancer via LATS2/YAP1/PD-L1 pathway. Clin. Transl. Oncol. 23 (3), 450–458. doi:10.1007/s12094-020-02439-y
Fuhrmann, D. C., and Brune, B. (2022). A graphical journey through iron metabolism, microRNAs, and hypoxia in ferroptosis. Redox Biol. 54, 102365. doi:10.1016/j.redox.2022.102365
Gao, Y., Li, X., Zeng, C., Liu, C., Hao, Q., Li, W., et al. (2020). CD63(+) cancer-associated fibroblasts confer tamoxifen resistance to breast cancer cells through exosomal miR-22. Adv. Sci. (Weinh) 7 (21), 2002518. doi:10.1002/advs.202002518
Geng, X., Chen, H., Zhao, L., Hu, J., Yang, W., Li, G., et al. (2021). Cancer-associated fibroblast (CAF) heterogeneity and targeting therapy of CAFs in pancreatic cancer. Front. Cell Dev. Biol. 9, 655152. doi:10.3389/fcell.2021.655152
Gerloff, D., Kewitz-Hempel, S., Hause, G., Ehrenreich, J., Golle, L., Kingreen, T., et al. (2022). Comprehensive analyses of miRNAs revealed miR-92b-3p, miR-182-5p and miR-183-5p as potential novel biomarkers in melanoma-derived extracellular vesicles. Front. Oncol. 12, 935816. doi:10.3389/fonc.2022.935816
Giannubilo, S. R., Cecati, M., Marzioni, D., and Ciavattini, A. (2024). Circulating miRNAs and preeclampsia: from implantation to epigenetics. Int. J. Mol. Sci. 25 (3), 1418. doi:10.3390/ijms25031418
Gjorgjieva, M., Sobolewski, C., Dolicka, D., Correia de Sousa, M., and Foti, M. (2019). miRNAs and NAFLD: from pathophysiology to therapy. Gut 68 (11), 2065–2079. doi:10.1136/gutjnl-2018-318146
Gong, J., Lin, Y., Zhang, H., Liu, C., Cheng, Z., Yang, X., et al. (2020). Reprogramming of lipid metabolism in cancer-associated fibroblasts potentiates migration of colorectal cancer cells. Cell Death Dis. 11 (4), 267. doi:10.1038/s41419-020-2434-z
Goulet, C. R., Champagne, A., Bernard, G., Vandal, D., Chabaud, S., Pouliot, F., et al. (2019). Cancer-associated fibroblasts induce epithelial-mesenchymal transition of bladder cancer cells through paracrine IL-6 signalling. BMC Cancer 19 (1), 137. doi:10.1186/s12885-019-5353-6
Graham, T. A., and Sottoriva, A. (2017). Measuring cancer evolution from the genome. J. Pathol. 241 (2), 183–191. doi:10.1002/path.4821
Groot, M., and Lee, H. (2020). Sorting mechanisms for MicroRNAs into extracellular vesicles and their associated diseases. Cells 9 (4), 1044. doi:10.3390/cells9041044
Guo, H., Ha, C., Dong, H., Yang, Z., Ma, Y., and Ding, Y. (2019). Cancer-associated fibroblast-derived exosomal microRNA-98-5p promotes cisplatin resistance in ovarian cancer by targeting CDKN1A. Cancer Cell Int. 19, 347. doi:10.1186/s12935-019-1051-3
Guo, L., Li, B., Yang, J., Shen, J., Ji, J., and Miao, M. (2020). Fibroblast-derived exosomal microRNA-369 potentiates migration and invasion of lung squamous cell carcinoma cells via NF1-mediated MAPK signaling pathway. Int. J. Mol. Med. 46 (2), 595–608. doi:10.3892/ijmm.2020.4614
Gurung, S., Perocheau, D., Touramanidou, L., and Baruteau, J. (2021). The exosome journey: from biogenesis to uptake and intracellular signalling. Cell Commun. Signal 19 (1), 47. doi:10.1186/s12964-021-00730-1
Han, J., Won, M., Kim, J. H., Jung, E., Min, K., Jangili, P., et al. (2020). Cancer stem cell-targeted bio-imaging and chemotherapeutic perspective. Chem. Soc. Rev. 49 (22), 7856–7878. doi:10.1039/d0cs00379d
Han, Q., Tan, S., Gong, L., Li, G., Wu, Q., Chen, L., et al. (2023). Omental cancer-associated fibroblast-derived exosomes with low microRNA-29c-3p promote ovarian cancer peritoneal metastasis. Cancer Sci. 114 (5), 1929–1942. doi:10.1111/cas.15726
Hao, C., Sheng, Z., Wang, W., Feng, R., Zheng, Y., Xiao, Q., et al. (2023). Tumor-derived exosomal miR-148b-3p mediates M2 macrophage polarization via TSC2/mTORC1 to promote breast cancer migration and invasion. Thorac. Cancer 14 (16), 1477–1491. doi:10.1111/1759-7714.14891
He, B., Zhao, Z., Cai, Q., Zhang, Y., Zhang, P., Shi, S., et al. (2020). miRNA-based biomarkers, therapies, and resistance in Cancer. Int. J. Biol. Sci. 16 (14), 2628–2647. doi:10.7150/ijbs.47203
He, C., Zheng, S., Luo, Y., and Wang, B. (2018). Exosome theranostics: biology and translational medicine. Theranostics 8 (1), 237–255. doi:10.7150/thno.21945
He, L., Guo, J., Fan, Z., Yang, S., Zhang, C., Cheng, B., et al. (2023). Exosomal miR-146b-5p derived from cancer-associated fibroblasts promotes progression of oral squamous cell carcinoma by downregulating HIPK3. Cell Signal 106, 110635. doi:10.1016/j.cellsig.2023.110635
Higashiguchi, M., Murakami, H., Akita, H., Kobayashi, S., Takahama, S., Iwagami, Y., et al. (2023). The impact of cellular senescence and senescence-associated secretory phenotype in cancer-associated fibroblasts on the malignancy of pancreatic cancer. Oncol. Rep. 49 (5), 98. doi:10.3892/or.2023.8535
Hill, M., and Tran, N. (2021). miRNA interplay: mechanisms and consequences in cancer. Dis. Model Mech. 14 (4), dmm047662. doi:10.1242/dmm.047662
Hofmann, W. K., Trumpp, A., and Muller-Tidow, C. (2023). Therapy resistance mechanisms in hematological malignancies. Int. J. Cancer 152 (3), 340–347. doi:10.1002/ijc.34243
Hu, J. L., Wang, W., Lan, X. L., Zeng, Z. C., Liang, Y. S., Yan, Y. R., et al. (2019). CAFs secreted exosomes promote metastasis and chemotherapy resistance by enhancing cell stemness and epithelial-mesenchymal transition in colorectal cancer. Mol. Cancer 18 (1), 91. doi:10.1186/s12943-019-1019-x
Huang, J., Zhang, L., Wan, D., Zhou, L., Zheng, S., Lin, S., et al. (2021). Extracellular matrix and its therapeutic potential for cancer treatment. Signal Transduct. Target Ther. 6 (1), 153. doi:10.1038/s41392-021-00544-0
Huang, S., Dong, M., and Chen, Q. (2022). Tumor-derived exosomes and their role in breast cancer metastasis. Int. J. Mol. Sci. 23 (22), 13993. doi:10.3390/ijms232213993
Huang, T., Song, X., Xu, D., Tiek, D., Goenka, A., Wu, B., et al. (2020). Stem cell programs in cancer initiation, progression, and therapy resistance. Theranostics 10 (19), 8721–8743. doi:10.7150/thno.41648
Huda, M. N., and Nurunnabi, M. (2022). Potential application of exosomes in vaccine development and delivery. Pharm. Res. 39 (11), 2635–2671. doi:10.1007/s11095-021-03143-4
Hussain, I., Deb, P., Chini, A., Obaid, M., Bhan, A., Ansari, K. I., et al. (2020). HOXA5 expression is elevated in breast cancer and is transcriptionally regulated by estradiol. Front. Genet. 11, 592436. doi:10.3389/fgene.2020.592436
Iacona, J. R., and Lutz, C. S. (2019). miR-146a-5p: expression, regulation, and functions in cancer. Wiley Interdiscip. Rev. RNA 10 (4), e1533. doi:10.1002/wrna.1533
Iqbal, M. A., Arora, S., Prakasam, G., Calin, G. A., and Syed, M. A. (2019). MicroRNA in lung cancer: role, mechanisms, pathways and therapeutic relevance. Mol. Asp. Med. 70, 3–20. doi:10.1016/j.mam.2018.07.003
Jabbari, N., Akbariazar, E., Feqhhi, M., Rahbarghazi, R., and Rezaie, J. (2020). Breast cancer-derived exosomes: tumor progression and therapeutic agents. J. Cell Physiol. 235 (10), 6345–6356. doi:10.1002/jcp.29668
Jayaram, M. A., and Phillips, J. J. (2024). Role of the microenvironment in glioma pathogenesis. Annu. Rev. Pathol. 19, 181–201. doi:10.1146/annurev-pathmechdis-051122-110348
Jhunjhunwala, S., Hammer, C., and Delamarre, L. (2021). Antigen presentation in cancer: insights into tumour immunogenicity and immune evasion. Nat. Rev. Cancer 21 (5), 298–312. doi:10.1038/s41568-021-00339-z
Jiang, M., Jike, Y., Liu, K., Gan, F., Zhang, K., Xie, M., et al. (2023a). Exosome-mediated miR-144-3p promotes ferroptosis to inhibit osteosarcoma proliferation, migration, and invasion through regulating ZEB1. Mol. Cancer 22 (1), 113. doi:10.1186/s12943-023-01804-z
Jiang, X., Ma, Y., Wang, T., Zhou, H., Wang, K., Shi, W., et al. (2023c). Targeting UBE2T potentiates gemcitabine efficacy in pancreatic cancer by regulating pyrimidine metabolism and replication stress. Gastroenterology 164 (7), 1232–1247. doi:10.1053/j.gastro.2023.02.025
Jiang, Y., Han, Q., Zhao, H., and Zhang, J. (2021). Promotion of epithelial-mesenchymal transformation by hepatocellular carcinoma-educated macrophages through Wnt2b/β-catenin/c-Myc signaling and reprogramming glycolysis. J. Exp. Clin. Cancer Res. 40 (1), 13. doi:10.1186/s13046-020-01808-3
Jiang, Y., Qiu, Q., Jing, X., Song, Z., Zhang, Y., Wang, C., et al. (2023b). Cancer-associated fibroblast-derived exosome miR-181b-3p promotes the occurrence and development of colorectal cancer by regulating SNX2 expression. Biochem. Biophys. Res. Commun. 641, 177–185. doi:10.1016/j.bbrc.2022.12.026
Jin, A. L., Ding, L., Yang, W. J., Liu, T., Chen, W., Li, T., et al. (2022). Exosomal microRNAs in the DLK1-DIO3 imprinted region derived from cancer-associated fibroblasts promote progression of hepatocellular carcinoma by targeting hedgehog interacting protein. BMC Gastroenterol. 22 (1), 505. doi:10.1186/s12876-022-02594-2
Jin, D., Guo, J., Wu, Y., Yang, L., Wang, X., Du, J., et al. (2020b). m(6)A demethylase ALKBH5 inhibits tumor growth and metastasis by reducing YTHDFs-mediated YAP expression and inhibiting miR-107/LATS2-mediated YAP activity in NSCLC. Mol. Cancer 19 (1), 40. doi:10.1186/s12943-020-01161-1
Jin, N., Bu, W., Li, X., Liu, L., and Wang, Z. (2020a). Long non-coding RNA TIRY promotes tumor metastasis by enhancing epithelial-to-mesenchymal transition in oral cancer. Exp. Biol. Med. (Maywood) 245 (7), 585–596. doi:10.1177/1535370220903673
Jin, Y., Meng, Q., Zhang, B., Xie, C., Chen, X., Tian, B., et al. (2021). Cancer-associated fibroblasts-derived exosomal miR-3656 promotes the development and progression of esophageal squamous cell carcinoma via the ACAP2/PI3K-AKT signaling pathway. Int. J. Biol. Sci. 17 (14), 3689–3701. doi:10.7150/ijbs.62571
Johnstone, R. M., Adam, M., Hammond, J. R., Orr, L., and Turbide, C. (1987). Vesicle formation during reticulocyte maturation. Association of plasma membrane activities with released vesicles (exosomes). J. Biol. Chem. 262 (19), 9412–9420. doi:10.1016/s0021-9258(18)48095-7
Josson, S., Gururajan, M., Sung, S. Y., Hu, P., Shao, C., Zhau, H. E., et al. (2015). Stromal fibroblast-derived miR-409 promotes epithelial-to-mesenchymal transition and prostate tumorigenesis. Oncogene 34 (21), 2690–2699. doi:10.1038/onc.2014.212
Kaller, M., Hünten, S., Siemens, H., and Hermeking, H. (2022). Analysis of the p53/microRNA network in cancer. Adv. Exp. Med. Biol. 1385, 187–228. doi:10.1007/978-3-031-08356-3_7
Kalluri, R., and LeBleu, V. S. (2020). The biology, function, and biomedical applications of exosomes. Science 367 (6478), eaau6977. doi:10.1126/science.aau6977
Karakasheva, T. A., Tang, Q., Qiao, E., Waldron, T. J., and Soni, M. (2018). IL-6 mediates cross-talk between tumor cells and activated fibroblasts in the tumor microenvironment. Cancer Res. 78 (17), 4957–4970. doi:10.1158/0008-5472.CAN-17-2268
Khalaf, K., Hana, D., Chou, J. T. T., Singh, C., Mackiewicz, A., and Kaczmarek, M. (2021). Aspects of the tumor microenvironment involved in immune resistance and drug resistance. Front. Immunol. 12, 656364. doi:10.3389/fimmu.2021.656364
Kim, J. E., Kim, B. G., Jang, Y., Kang, S., Lee, J. H., and Cho, N. H. (2020). The stromal loss of miR-4516 promotes the FOSL1-dependent proliferation and malignancy of triple negative breast cancer. Cancer Lett. 469, 256–265. doi:10.1016/j.canlet.2019.10.039
Kok, V. C., and Yu, C. C. (2020). Cancer-derived exosomes: their role in cancer biology and biomarker development. Int. J. Nanomedicine 15, 8019–8036. doi:10.2147/IJN.S272378
Kryczka, J., Czarnecka-Chrebelska, K. H., and Brzeziańska-Lasota, E. (2021). Molecular mechanisms of chemoresistance induced by cisplatin in NSCLC cancer therapy. Int. J. Mol. Sci. 22 (16), 8885. doi:10.3390/ijms22168885
Kumar, V. E., Nambiar, R., De Souza, C., Nguyen, A., Chien, J., and Lam, K. S. (2022). Targeting epigenetic modifiers of tumor plasticity and cancer stem cell behavior. Cells 11 (9), 1403. doi:10.3390/cells11091403
Lee, H., Zhang, D., Wu, J., Otterbein, L. E., and Jin, Y. (2017). Lung epithelial cell-derived microvesicles regulate macrophage migration via MicroRNA-17/221-induced integrin β1 recycling. J. Immunol. 199 (4), 1453–1464. doi:10.4049/jimmunol.1700165
Lee, S. S., and Cheah, Y. K. (2019). The interplay between MicroRNAs and cellular components of tumour microenvironment (TME) on non-small-cell lung cancer (NSCLC) progression. J. Immunol. Res. 2019, 3046379. doi:10.1155/2019/3046379
Lei, X., Lei, Y., Li, J. K., Du, W. X., Li, R. G., Yang, J., et al. (2020). Immune cells within the tumor microenvironment: biological functions and roles in cancer immunotherapy. Cancer Lett. 470, 126–133. doi:10.1016/j.canlet.2019.11.009
Li, B., Cao, Y., Sun, M., and Feng, H. (2021a). Expression, regulation, and function of exosome-derived miRNAs in cancer progression and therapy. FASEB J. 35 (10), e21916. doi:10.1096/fj.202100294RR
Li, B. L., Lu, W., Qu, J. J., Ye, L., Du, G. Q., and Wan, X. P. (2019). Loss of exosomal miR-148b from cancer-associated fibroblasts promotes endometrial cancer cell invasion and cancer metastasis. J. Cell Physiol. 234 (3), 2943–2953. doi:10.1002/jcp.27111
Li, L., Liu, P., He, C., and Xu, C. (2023). miRNA-223-3p regulates ECT2 to promote proliferation, invasion, and metastasis of gastric cancer through the Wnt/β-catenin signaling pathway. J. Cancer Res. Clin. Oncol. 149 (1), 121–134. doi:10.1007/s00432-022-04453-9
Li, L., Piontek, K., Ishida, M., Fausther, M., Dranoff, J. A., Fu, R., et al. (2017). Extracellular vesicles carry microRNA-195 to intrahepatic cholangiocarcinoma and improve survival in a rat model. Hepatology 65 (2), 501–514. doi:10.1002/hep.28735
Li, Q., Wang, M., Hu, Y., Zhao, E., Li, J., Ren, L., et al. (2021b). MYBL2 disrupts the Hippo-YAP pathway and confers castration resistance and metastatic potential in prostate cancer. Theranostics 11 (12), 5794–5812. doi:10.7150/thno.56604
Li, X., Sun, Z., Peng, G., Xiao, Y., Guo, J., Wu, B., et al. (2022a). Single-cell RNA sequencing reveals a pro-invasive cancer-associated fibroblast subgroup associated with poor clinical outcomes in patients with gastric cancer. Theranostics 12 (2), 620–638. doi:10.7150/thno.60540
Li, Y. Y., Tao, Y. W., Gao, S., Zheng, J. M., and Zhang, S. E. (2018). Cancer-associated fibroblasts contribute to oral cancer cells proliferation and metastasis via exosome-mediated paracrine miR-34a-5p. EBioMedicine 36, 209–220. doi:10.1016/j.ebiom.2018.09.006
Li, Z. Y., Xie, Y., Deng, M., Zhu, L., Wu, X., Li, G., et al. (2022b). c-Myc-activated intronic miR-210 and lncRNA MIR210HG synergistically promote the metastasis of gastric cancer. Cancer Lett. 526, 322–334. doi:10.1016/j.canlet.2021.11.006
Liang, C., Shi, S., Meng, Q., Liang, D., Ji, S., Zhang, B., et al. (2017). Complex roles of the stroma in the intrinsic resistance to gemcitabine in pancreatic cancer: where we are and where we are going. Exp. Mol. Med. 49 (12), e406. doi:10.1038/emm.2017.255
Limoge, M., Safina, A., Beattie, A., Kapus, L., Truskinovsky, A. M., and Bakin, A. V. (2017). Tumor-fibroblast interactions stimulate tumor vascularization by enhancing cytokine-driven production of MMP9 by tumor cells. Oncotarget 8 (22), 35592–35608. doi:10.18632/oncotarget.16022
Liu, J., Zhang, F., Wang, J., and Wang, Y. (2023a). MicroRNA-mediated regulation in lung adenocarcinoma: signaling pathways and potential therapeutic implications (Review). Oncol. Rep. 50 (6), 211. doi:10.3892/or.2023.8648
Liu, X., Cheng, D., Cui, M., Qu, F., Yu, J., Tang, Z., et al. (2022b). Exosome marker proteins of tumor-associated fibroblasts and exosome-derived miR-92a-3p act as potential biomarkers for liver cancer. Crit. Rev. Eukaryot. Gene Expr. 32 (1), 49–57. doi:10.1615/CritRevEukaryotGeneExpr.2021039570
Liu, X., Li, J., Yang, X., Kong, J., and Qi, D. (2023b). Carcinoma-associated fibroblast-derived lysyl oxidase-rich extracellular vesicles mediate collagen crosslinking and promote epithelial-mesenchymal transition via p-FAK/p-paxillin/YAP signaling. Int. J. Oral Sci. 15 (1), 32. doi:10.1038/s41368-023-00236-1
Liu, Y., Fu, W., Cao, X., Li, S., Xiong, T., Zhang, X., et al. (2021). Delivery of miR-224-5p by exosomes from cancer-associated fibroblasts potentiates progression of clear cell renal cell carcinoma. Comput. Math. Methods Med. 2021, 5517747. doi:10.1155/2021/5517747
Liu, Y., Shi, M., He, X., Cao, Y., Liu, P., Li, F., et al. (2022a). LncRNA-PACERR induces pro-tumour macrophages via interacting with miR-671-3p and m6A-reader IGF2BP2 in pancreatic ductal adenocarcinoma. J. Hematol. Oncol. 15 (1), 52. doi:10.1186/s13045-022-01272-w
Liubomirski, Y., Lerrer, S., Meshel, T., Rubinstein-Achiasaf, L., Morein, D., Wiemann, S., et al. (2019). Tumor-stroma-inflammation networks promote pro-metastatic chemokines and aggressiveness characteristics in triple-negative breast cancer. Front. Immunol. 10, 757. doi:10.3389/fimmu.2019.00757
Long, Y. F., Chow, S. K. H., Cui, C., Wong, R. M. Y., Zhang, N., Qin, L., et al. (2023). Does exercise influence skeletal muscle by modulating mitochondrial functions via regulating MicroRNAs? A systematic review. Ageing Res. Rev. 91, 102048. doi:10.1016/j.arr.2023.102048
Lopes, G. (2023). The global economic cost of cancer-estimating it is just the first step. JAMA Oncol. 9 (4), 461–462. doi:10.1001/jamaoncol.2022.7133
Lu, P., Li, H., Singh, R. N., Bishop, C. E., and Chen, X. (2017). MEX3C interacts with adaptor-related protein complex 2 and involves in miR-451a exosomal sorting. PLoS One 12 (10), e0185992. doi:10.1371/journal.pone.0185992
Lu, W., and Kang, Y. (2019). Epithelial-mesenchymal plasticity in cancer progression and metastasis. Dev. Cell 49 (3), 361–374. doi:10.1016/j.devcel.2019.04.010
Lu, X., Duan, J., Zhou, R., and Xu, Y. (2021). MiR-301b-3p promotes the occurrence and development of breast cancer cells via targeting HOXA5. Crit. Rev. Eukaryot. Gene Expr. 31 (3), 35–44. doi:10.1615/CritRevEukaryotGeneExpr.2021038215
Luo, H., Xia, X., Huang, L. B., An, H., Cao, M., Kim, G. D., et al. (2022). Pan-cancer single-cell analysis reveals the heterogeneity and plasticity of cancer-associated fibroblasts in the tumor microenvironment. Nat. Commun. 13 (1), 6619. doi:10.1038/s41467-022-34395-2
Lv, H., Lv, G., Chen, C., Zong, Q., Jiang, G., Ye, D., et al. (2021). NAD(+) metabolism maintains inducible PD-L1 expression to drive tumor immune evasion. Cell Metab. 33 (1), 110–127 e5. doi:10.1016/j.cmet.2020.10.021
Ma, L., Li, C., Yin, H., Huang, J., Yu, S., Zhao, J., et al. (2023). The mechanism of DNA methylation and miRNA in breast cancer. Int. J. Mol. Sci. 24 (11), 9360. doi:10.3390/ijms24119360
Machida, K. (2020). Cell fate, metabolic reprogramming and lncRNA of tumor-initiating stem-like cells induced by alcohol. Chem. Biol. Interact. 323, 109055. doi:10.1016/j.cbi.2020.109055
Mafi, S., Mansoori, B., Taeb, S., Sadeghi, H., Abbasi, R., Cho, W. C., et al. (2021). mTOR-mediated regulation of immune responses in cancer and tumor microenvironment. Front. Immunol. 12, 774103. doi:10.3389/fimmu.2021.774103
Malla, R. R., Padmaraju, V., Marni, R., and Kamal, M. A. (2021). Natural products: potential targets of TME related long non-coding RNAs in lung cancer. Phytomedicine 93, 153782. doi:10.1016/j.phymed.2021.153782
Manfioletti, G., and Fedele, M. (2022). Epithelial-mesenchymal transition (EMT) 2021. Int. J. Mol. Sci. 23 (10), 5848. doi:10.3390/ijms23105848
Mao, X., Xu, J., Wang, W., Liang, C., Hua, J., Liu, J., et al. (2021). Crosstalk between cancer-associated fibroblasts and immune cells in the tumor microenvironment: new findings and future perspectives. Mol. Cancer 20 (1), 131. doi:10.1186/s12943-021-01428-1
Maris, P., Blomme, A., Palacios, A. P., Costanza, B., Bellahcène, A., Bianchi, E., et al. (2015). Asporin is a fibroblast-derived TGF-β1 inhibitor and a tumor suppressor associated with good prognosis in breast cancer. PLoS Med. 12 (9), e1001871. doi:10.1371/journal.pmed.1001871
Mashouri, L., Yousefi, H., Aref, A. R., Ahadi, A. M., Molaei, F., and Alahari, S. K. (2019). Exosomes: composition, biogenesis, and mechanisms in cancer metastasis and drug resistance. Mol. Cancer 18 (1), 75. doi:10.1186/s12943-019-0991-5
McAndrews, K. M., Chen, Y., Darpolor, J. K., Zheng, X., Yang, S., Carstens, J. L., et al. (2022). Identification of functional heterogeneity of carcinoma-associated fibroblasts with distinct IL6-mediated therapy resistance in pancreatic cancer. Cancer Discov. 12 (6), 1580–1597. doi:10.1158/2159-8290.CD-20-1484
McKenzie, A. J., Hoshino, D., Hong, N. H., Cha, D. J., Franklin, J. L., Coffey, R. J., et al. (2016). KRAS-MEK signaling controls Ago2 sorting into exosomes. Cell Rep. 15 (5), 978–987. doi:10.1016/j.celrep.2016.03.085
Mezawa, Y., and Orimo, A. (2022). Phenotypic heterogeneity, stability and plasticity in tumor-promoting carcinoma-associated fibroblasts. FEBS J. 289 (9), 2429–2447. doi:10.1111/febs.15851
Miaomiao, S., Xiaoqian, W., Yuwei, S., Chao, C., Chenbo, Y., Yinghao, L., et al. (2023). Cancer-associated fibroblast-derived exosome microRNA-21 promotes angiogenesis in multiple myeloma. Sci. Rep. 13 (1), 9671. doi:10.1038/s41598-023-36092-6
Morgan, A., Griffin, M., Kameni, L., Wan, D. C., Longaker, M. T., and Norton, J. A. (2023). Medical biology of cancer-associated fibroblasts in pancreatic cancer. Biol. (Basel) 12 (8), 1044. doi:10.3390/biology12081044
Moro, G., Fratte, C. D., Normanno, N., Polo, F., and Cinti, S. (2023). Point-of-Care testing for the detection of MicroRNAs: towards liquid biopsy on a chip. Angew. Chem. Int. Ed. Engl. 62 (51), e202309135. doi:10.1002/anie.202309135
Motoi, F., Kosuge, T., Ueno, H., Yamaue, H., Satoi, S., Sho, M., et al. (2019). Randomized phase II/III trial of neoadjuvant chemotherapy with gemcitabine and S-1 versus upfront surgery for resectable pancreatic cancer (Prep-02/JSAP05). Jpn. J. Clin. Oncol. 49 (2), 190–194. doi:10.1093/jjco/hyy190
Najafi, M., Farhood, B., and Mortezaee, K. (2019). Cancer stem cells (CSCs) in cancer progression and therapy. J. Cell Physiol. 234 (6), 8381–8395. doi:10.1002/jcp.27740
O’Connor, R. A., Martinez, B. R., Koppensteiner, L., Mathieson, L., and Akram, A. R. (2023). Cancer-associated fibroblasts drive CXCL13 production in activated T cells via TGF-beta. Front. Immunol. 14, 1221532. doi:10.3389/fimmu.2023.1221532
Ohlund, D., Handly-Santana, A., Biffi, G., Elyada, E., Almeida, A. S., Ponz-Sarvise, M., et al. (2017). Distinct populations of inflammatory fibroblasts and myofibroblasts in pancreatic cancer. J. Exp. Med. 214 (3), 579–596. doi:10.1084/jem.20162024
Oliveira, G., and Wu, C. J. (2023). Dynamics and specificities of T cells in cancer immunotherapy. Nat. Rev. Cancer 23 (5), 295–316. doi:10.1038/s41568-023-00560-y
Pan, G., Jiang, B., Yi, Z., Yin, J., and Liu, Y. (2023a). Exosomal miR-105-5p derived from bladder cancer stem cells targets for GPR12 to promote the malignancy of bladder cancer. BMC Urol. 23 (1), 155. doi:10.1186/s12894-023-01326-2
Pan, S., Deng, Y., Fu, J., Zhang, Y., Zhang, Z., and Qin, X. (2022a). N6-methyladenosine upregulates miR-181d-5p in exosomes derived from cancer-associated fibroblasts to inhibit 5-FU sensitivity by targeting NCALD in colorectal cancer. Int. J. Oncol. 60 (2), 14. doi:10.3892/ijo.2022.5304
Pan, S., Lai, L. A., Simeone, D. M., Dawson, D. W., Yan, Y., Crnogorac-Jurcevic, T., et al. (2022b). Proteomes of extracellular vesicles from pancreatic cancer cells and cancer-associated fibroblasts. Pancreas 51 (7), 790–799. doi:10.1097/MPA.0000000000002115
Pan, Z., Yun, H., Xiao, Y., Tong, F., Liu, G., Zhang, G., et al. (2023b). MiR-934 exacerbates malignancy of gastric cancer cells by targeting ZFP36. Iran. J. Public Health 52 (8), 1720–1729. doi:10.18502/ijph.v52i8.13411
Papait, A., Romoli, J., Stefani, F. R., Chiodelli, P., Montresor, M. C., Agoni, L., et al. (2022). Fight the cancer, hit the CAF. Cancers (Basel) 14 (15), 3570. doi:10.3390/cancers14153570
Parfenyev, S., Singh, A., Fedorova, O., Daks, A., Kulshreshtha, R., and Barlev, N. A. (2021). Interplay between p53 and non-coding RNAs in the regulation of EMT in breast cancer. Cell Death Dis. 12 (1), 17. doi:10.1038/s41419-020-03327-7
Pascual-Anton, L., Cardeñes, B., Sainz de la Cuesta, R., González-Cortijo, L., López-Cabrera, M., Cabañas, C., et al. (2021). Mesothelial-to-Mesenchymal transition and exosomes in peritoneal metastasis of ovarian cancer. Int. J. Mol. Sci. 22 (21), 11496. doi:10.3390/ijms222111496
Pavlova, N. N., Zhu, J., and Thompson, C. B. (2022). The hallmarks of cancer metabolism: still emerging. Cell Metab. 34 (3), 355–377. doi:10.1016/j.cmet.2022.01.007
Peltier, A., Seban, R. D., Buvat, I., Bidard, F. C., and Mechta-Grigoriou, F. (2022). Fibroblast heterogeneity in solid tumors: from single cell analysis to whole-body imaging. Semin. Cancer Biol. 86 (3), 262–272. doi:10.1016/j.semcancer.2022.04.008
Peng, K., Zhang, Y., Liu, D., and Chen, J. (2023). MMP2 is a immunotherapy related biomarker and correlated with cancer-associated fibroblasts infiltrate in melanoma. Cancer Cell Int. 23 (1), 26. doi:10.1186/s12935-023-02862-5
Peng, L., Wang, D., Han, Y., Huang, T., He, X., Wang, J., et al. (2021). Emerging role of cancer-associated fibroblasts-derived exosomes in tumorigenesis. Front. Immunol. 12, 795372. doi:10.3389/fimmu.2021.795372
Peng, Q., Zhou, Y., Oyang, L., Wu, N., Tang, Y., Su, M., et al. (2022). Impacts and mechanisms of alternative mRNA splicing in cancer metabolism, immune response, and therapeutics. Mol. Ther. 30 (3), 1018–1035. doi:10.1016/j.ymthe.2021.11.010
Qi, Y., Wang, H., Zhang, Q., Liu, Z., Wang, T., Wu, Z., et al. (2022). CAF-released exosomal miR-20a-5p facilitates HCC progression via the LIMA1-mediated β-catenin pathway. Cells 11 (23), 3857. doi:10.3390/cells11233857
Qin, M. Y., Huang, S. Q., Zou, X. Q., Zhong, X. B., Yang, Y. F., Zhang, Y. T., et al. (2021). Drug-containing serum of rhubarb-astragalus capsule inhibits the epithelial-mesenchymal transformation of HK-2 by downregulating TGF-β1/p38MAPK/Smad2/3 pathway. J. Ethnopharmacol. 280, 114414. doi:10.1016/j.jep.2021.114414
Qiu, S., Xie, L., Lu, C., Gu, C., Xia, Y., Lv, J., et al. (2022). Gastric cancer-derived exosomal miR-519a-3p promotes liver metastasis by inducing intrahepatic M2-like macrophage-mediated angiogenesis. J. Exp. Clin. Cancer Res. 41 (1), 296. doi:10.1186/s13046-022-02499-8
Rajabi, H., Konyalilar, N., Erkan, S., Mortazavi, D., Korkunc, S. K., Kayalar, O., et al. (2022). Emerging role of exosomes in the pathology of chronic obstructive pulmonary diseases; destructive and therapeutic properties. Stem Cell Res. Ther. 13 (1), 144. doi:10.1186/s13287-022-02820-4
Raju, S., Shukla, J., and Kumar, R. (2022). Fibroblast activation protein inhibitor theranostics. Pet. Clin. 17 (3), 453–464. doi:10.1016/j.cpet.2022.03.005
Rezaee, D., Saadatpour, F., Akbari, N., Zoghi, A., Najafi, S., Beyranvand, P., et al. (2023). The role of microRNAs in the pathophysiology of human central nervous system: a focus on neurodegenerative diseases. Ageing Res. Rev. 92, 102090. doi:10.1016/j.arr.2023.102090
Richards, K. E., Xiao, W., Hill, R., and On Behalf Of The Usc Pancreas Research Team, (2022). Cancer-associated fibroblasts confer gemcitabine resistance to pancreatic cancer cells through PTEN-targeting miRNAs in exosomes. Cancers (Basel) 14 (11), 2812. doi:10.3390/cancers14112812
Rimal, R., Desai, P., Daware, R., Hosseinnejad, A., Prakash, J., Lammers, T., et al. (2022). Cancer-associated fibroblasts: origin, function, imaging, and therapeutic targeting. Adv. Drug Deliv. Rev. 189, 114504. doi:10.1016/j.addr.2022.114504
Sahai, E., Astsaturov, I., Cukierman, E., DeNardo, D. G., Egeblad, M., Evans, R. M., et al. (2020). A framework for advancing our understanding of cancer-associated fibroblasts. Nat. Rev. Cancer 20 (3), 174–186. doi:10.1038/s41568-019-0238-1
Sankar, K., Pearson, A. N., Worlikar, T., Perricone, M. D., Holcomb, E. A., Mendiratta-Lala, M., et al. (2023). Impact of immune tolerance mechanisms on the efficacy of immunotherapy in primary and secondary liver cancers. Transl. Gastroenterol. Hepatol. 8, 29. doi:10.21037/tgh-23-11
Sato, R., Imamura, K., Semba, T., Tomita, Y., Saeki, S., Ikeda, K., et al. (2021). TGFβ signaling activated by cancer-associated fibroblasts determines the histological signature of lung adenocarcinoma. Cancer Res. 81 (18), 4751–4765. doi:10.1158/0008-5472.CAN-20-3941
Sato, S., Hiruma, T., Koizumi, M., Yoshihara, M., Nakamura, Y., Tadokoro, H., et al. (2023). Bone marrow adipocytes induce cancer-associated fibroblasts and immune evasion, enhancing invasion and drug resistance. Cancer Sci. 114 (6), 2674–2688. doi:10.1111/cas.15786
Shafi, A. A., Yen, A. E., and Weigel, N. L. (2013). Androgen receptors in hormone-dependent and castration-resistant prostate cancer. Pharmacol. Ther. 140 (3), 223–238. doi:10.1016/j.pharmthera.2013.07.003
Shani, O., Vorobyov, T., Monteran, L., Lavie, D., Cohen, N., Raz, Y., et al. (2020). Fibroblast-derived IL33 facilitates breast cancer metastasis by modifying the immune microenvironment and driving type 2 immunity. Cancer Res. 80 (23), 5317–5329. doi:10.1158/0008-5472.CAN-20-2116
Shao, J., Zaro, J., and Shen, Y. (2020). Advances in exosome-based drug delivery and tumor targeting: from tissue distribution to intracellular fate. Int. J. Nanomedicine 15, 9355–9371. doi:10.2147/IJN.S281890
Shi, L., Wang, Z., Geng, X., Zhang, Y., and Xue, Z. (2020). Exosomal miRNA-34 from cancer-associated fibroblasts inhibits growth and invasion of gastric cancer cells in vitro and in vivo. Aging (Albany NY) 12 (9), 8549–8564. doi:10.18632/aging.103157
Shi, L., Zhu, W., Huang, Y., Zhuo, L., Wang, S., Chen, S., et al. (2022b). Cancer-associated fibroblast-derived exosomal microRNA-20a suppresses the PTEN/PI3K-AKT pathway to promote the progression and chemoresistance of non-small cell lung cancer. Clin. Transl. Med. 12 (7), e989. doi:10.1002/ctm2.989
Shi, W., Liu, Y., Qiu, X., Yang, L., and Lin, G. (2023). Cancer-associated fibroblasts-derived exosome-mediated transfer of miR-345-5p promotes the progression of colorectal cancer by targeting CDKN1A. Carcinogenesis 44 (4), 317–327. doi:10.1093/carcin/bgad014
Shi, Z., Jiang, T., Cao, B., Sun, X., and Liu, J. (2022a). CAF-derived exosomes deliver LINC01410 to promote epithelial-mesenchymal transition of esophageal squamous cell carcinoma. Exp. Cell Res. 412 (2), 113033. doi:10.1016/j.yexcr.2022.113033
Shinkawa, T., Ohuchida, K., and Nakamura, M. (2022). Heterogeneity of cancer-associated fibroblasts and the tumor immune microenvironment in pancreatic cancer. Cancers (Basel) 14 (16), 3994. doi:10.3390/cancers14163994
Shurtleff, M. J., Temoche-Diaz, M. M., Karfilis, K. V., and Schekman, R. (2016). Y-box protein 1 is required to sort microRNAs into exosomes in cells and in a cell-free reaction. Elife 5, e19276. doi:10.7554/eLife.19276
Sugita, B. M., Rodriguez, Y., Fonseca, A. S., Nunes Souza, E., Kallakury, B., Cavalli, I. J., et al. (2022). MiR-150-5p overexpression in triple-negative breast cancer contributes to the in vitro aggressiveness of this breast cancer subtype. Cancers (Basel) 14 (9), 2156. doi:10.3390/cancers14092156
Sun, H., Wang, X., Wang, X., Xu, M., and Sheng, W. (2022). The role of cancer-associated fibroblasts in tumorigenesis of gastric cancer. Cell Death Dis. 13 (10), 874. doi:10.1038/s41419-022-05320-8
Sun, L. P., Xu, K., Cui, J., Yuan, D. Y., Zou, B., Li, J., et al. (2019). Cancer-associated fibroblast-derived exosomal miR-382-5p promotes the migration and invasion of oral squamous cell carcinoma. Oncol. Rep. 42 (4), 1319–1328. doi:10.3892/or.2019.7255
Suva, M. L., and Tirosh, I. (2020). The glioma stem cell model in the era of single-cell genomics. Cancer Cell 37 (5), 630–636. doi:10.1016/j.ccell.2020.04.001
Taha, E. A., Ono, K., and Eguchi, T. (2019). Roles of extracellular HSPs as biomarkers in immune surveillance and immune evasion. Int. J. Mol. Sci. 20 (18), 4588. doi:10.3390/ijms20184588
Taki, M., Abiko, K., Ukita, M., Murakami, R., Yamanoi, K., Yamaguchi, K., et al. (2021). Tumor immune microenvironment during epithelial-mesenchymal transition. Clin. Cancer Res. 27 (17), 4669–4679. doi:10.1158/1078-0432.CCR-20-4459
Takizawa, S., Matsuzaki, J., and Ochiya, T. (2022). Circulating microRNAs: challenges with their use as liquid biopsy biomarkers. Cancer Biomark. 35 (1), 1–9. doi:10.3233/CBM-210223
Tao, S., Li, H., Ma, X., Ma, Y., He, J., Gao, Y., et al. (2021). Elevating microRNA-1-3p shuttled by cancer-associated fibroblasts-derived extracellular vesicles suppresses breast cancer progression and metastasis by inhibiting GLIS1. Cancer Gene Ther. 28 (6), 634–648. doi:10.1038/s41417-020-00244-x
Thun, M. J., DeLancey, J. O., Center, M. M., Jemal, A., and Ward, E. M. (2010). The global burden of cancer: priorities for prevention. Carcinogenesis 31 (1), 100–110. doi:10.1093/carcin/bgp263
Tiwari, A., Trivedi, R., and Lin, S. Y. (2022). Tumor microenvironment: barrier or opportunity towards effective cancer therapy. J. Biomed. Sci. 29 (1), 83. doi:10.1186/s12929-022-00866-3
Trams, E. G., Lauter, C. J., Salem, N., and Heine, U. (1981). Exfoliation of membrane ecto-enzymes in the form of micro-vesicles. Biochim. Biophys. Acta 645 (1), 63–70. doi:10.1016/0005-2736(81)90512-5
Uozaki, H., Morita, S., Kumagai, A., Aso, T., Soejima, Y., Takahashi, Y., et al. (2014). Stromal miR-21 is more important than miR-21 of tumour cells for the progression of gastric cancer. Histopathology 65 (6), 775–783. doi:10.1111/his.12491
Villegas-Pineda, J. C., Lizarazo-Taborda, M. D. R., Ramírez-de-Arellano, A., and Pereira-Suárez, A. L. (2021). Exosomal miRNAs and lncRNAs: the modulator keys of cancer-associated fibroblasts in the genesis and progression of malignant neoplasms. Front. Cell Dev. Biol. 9, 717478. doi:10.3389/fcell.2021.717478
Wan, Y. H., Liu, Q. S., Wan, S. S., and Wang, R. W. (2022). Colorectal cancer-derived exosomes and modulation KRAS signaling. Clin. Transl. Oncol. 24 (11), 2074–2080. doi:10.1007/s12094-022-02877-w
Wang, H., Huang, H., Wang, L., Liu, Y., Wang, M., Zhao, S., et al. (2021b). Cancer-associated fibroblasts secreted miR-103a-3p suppresses apoptosis and promotes cisplatin resistance in non-small cell lung cancer. Aging (Albany NY) 13 (10), 14456–14468. doi:10.18632/aging.103556
Wang, H., Wei, H., Wang, J., Li, L., Chen, A., and Li, Z. (2020). MicroRNA-181d-5p-Containing exosomes derived from CAFs promote EMT by regulating CDX2/HOXA5 in breast cancer. Mol. Ther. Nucleic Acids 19, 654–667. doi:10.1016/j.omtn.2019.11.024
Wang, J., Guan, X., Zhang, Y., Ge, S., Zhang, L., Li, H., et al. (2018). Exosomal miR-27a derived from gastric cancer cells regulates the transformation of fibroblasts into cancer-associated fibroblasts. Cell Physiol. Biochem. 49 (3), 869–883. doi:10.1159/000493218
Wang, J. W., Wu, X. F., Gu, X. J., and Jiang, X. H. (2019a). Exosomal miR-1228 from cancer-associated fibroblasts promotes cell migration and invasion of osteosarcoma by directly targeting SCAI. Oncol. Res. 27 (9), 979–986. doi:10.3727/096504018X15336368805108
Wang, M., Feng, R., Chen, Z., Shi, W., Li, C., Liu, H., et al. (2022a). Identification of cancer-associated fibroblast subtype of triple-negative breast cancer. J. Oncol. 2022, 6452636. doi:10.1155/2022/6452636
Wang, M., Zhuoma, C., Liu, X., Huang, Q., Cai, G., Sang, G., et al. (2022c). Loss of exosomal miR-34c-5p in cancer-associated fibroblast for the maintenance of stem-like phenotypes of laryngeal cancer cells. Head. Neck 44 (11), 2437–2451. doi:10.1002/hed.27155
Wang, S. S., Tang, X. T., Lin, M., Yuan, J., Peng, Y. J., Yin, X., et al. (2021a). Perivenous stellate cells are the main source of myofibroblasts and cancer-associated fibroblasts formed after chronic liver injuries. Hepatology 74 (3), 1578–1594. doi:10.1002/hep.31848
Wang, W. Z., Cao, X., Bian, L., Gao, Y., Yu, M., Li, Y. T., et al. (2023). Analysis of mRNA-miRNA interaction network reveals the role of CAFs-derived exosomes in the immune regulation of oral squamous cell carcinoma. BMC Cancer 23 (1), 591. doi:10.1186/s12885-023-11028-5
Wang, X., Qin, X., Yan, M., Shi, J., Xu, Q., Li, Z., et al. (2019b). Loss of exosomal miR-3188 in cancer-associated fibroblasts contributes to HNC progression. J. Exp. Clin. Cancer Res. 38 (1), 151. doi:10.1186/s13046-019-1144-9
Wang, Y., Liang, H., and Zheng, J. (2022b). Exosomal microRNAs mediating crosstalk between cancer cells and cancer-associated fibroblasts in the tumor microenvironment. Pathol. Res. Pract. 239, 154159. doi:10.1016/j.prp.2022.154159
Wei, K., Nguyen, H. N., and Brenner, M. B. (2021). Fibroblast pathology in inflammatory diseases. J. Clin. Invest. 131 (20), e149538. doi:10.1172/JCI149538
Wu, C., Gu, J., Gu, H., Zhang, X., Zhang, X., and Ji, R. (2022). The recent advances of cancer associated fibroblasts in cancer progression and therapy. Front. Oncol. 12, 1008843. doi:10.3389/fonc.2022.1008843
Wu, C., Li, D., Cheng, X., Gu, H., Qian, Y., and Feng, L. (2023). Downregulation of cancer-associated fibroblast exosome-derived miR-29b-1-5p restrains vasculogenic mimicry and apoptosis while accelerating migration and invasion of gastric cancer cells via immunoglobulin domain-containing 1/zonula occluden-1 axis. Cell Cycle 22 (17), 1807–1826. doi:10.1080/15384101.2023.2231740
Wu, C. H., Silvers, C. R., Messing, E. M., and Lee, Y. F. (2019). Bladder cancer extracellular vesicles drive tumorigenesis by inducing the unfolded protein response in endoplasmic reticulum of nonmalignant cells. J. Biol. Chem. 294 (9), 3207–3218. doi:10.1074/jbc.RA118.006682
Wu, H. J., Hao, M., Yeo, S. K., and Guan, J. L. (2020). FAK signaling in cancer-associated fibroblasts promotes breast cancer cell migration and metastasis by exosomal miRNAs-mediated intercellular communication. Oncogene 39 (12), 2539–2549. doi:10.1038/s41388-020-1162-2
Xiang, X., Niu, Y. R., Wang, Z. H., Ye, L. L., Peng, W. B., and Zhou, Q. (2022). Cancer-associated fibroblasts: vital suppressors of the immune response in the tumor microenvironment. Cytokine Growth Factor Rev. 67, 35–48. doi:10.1016/j.cytogfr.2022.07.006
Xie, Q. H., Zheng, J. Q., Ding, J. Y., Wu, Y. F., Liu, L., Yu, Z. L., et al. (2022). Exosome-mediated immunosuppression in tumor microenvironments. Cells 11 (12), 1946. doi:10.3390/cells11121946
Xin, L., Wu, Y., Liu, C., Zeng, F., Wang, J. L., Wu, D. Z., et al. (2021). Exosome-mediated transfer of lncRNA HCG18 promotes M2 macrophage polarization in gastric cancer. Mol. Immunol. 140, 196–205. doi:10.1016/j.molimm.2021.10.011
Xu, G., Zhang, B., Ye, J., Cao, S., Shi, J., Zhao, Y., et al. (2019). Exosomal miRNA-139 in cancer-associated fibroblasts inhibits gastric cancer progression by repressing MMP11 expression. Int. J. Biol. Sci. 15 (11), 2320–2329. doi:10.7150/ijbs.33750
Xu, Y., Li, W., Lin, S., Liu, B., Wu, P., and Li, L. (2023). Fibroblast diversity and plasticity in the tumor microenvironment: roles in immunity and relevant therapies. Cell Commun. Signal 21 (1), 234. doi:10.1186/s12964-023-01204-2
Yan, L., Wang, P., Fang, W., and Liang, C. (2020). Cancer-associated fibroblasts-derived exosomes-mediated transfer of LINC00355 regulates bladder cancer cell proliferation and invasion. Cell Biochem. Funct. 38 (3), 257–265. doi:10.1002/cbf.3462
Yan, Z., Sheng, Z., Zheng, Y., Feng, R., Xiao, Q., Shi, L., et al. (2021). Cancer-associated fibroblast-derived exosomal miR-18b promotes breast cancer invasion and metastasis by regulating TCEAL7. Cell Death Dis. 12 (12), 1120. doi:10.1038/s41419-021-04409-w
Yang, D., Zhang, W., Zhang, H., Zhang, F., Chen, L., Ma, L., et al. (2020b). Progress, opportunity, and perspective on exosome isolation - efforts for efficient exosome-based theranostics. Theranostics 10 (8), 3684–3707. doi:10.7150/thno.41580
Yang, F., Ning, Z., Liu, W., Shao, C., and Shu, Y. (2017). Exosomal miRNAs and miRNA dysregulation in cancer-associated fibroblasts. Mol. Cancer 16 (1), 148. doi:10.1186/s12943-017-0718-4
Yang, F., Yan, Y., Yang, Y., Hong, X., Wang, M., Yang, Z., et al. (2020c). MiR-210 in exosomes derived from CAFs promotes non-small cell lung cancer migration and invasion through PTEN/PI3K/AKT pathway. Cell Signal 73, 109675. doi:10.1016/j.cellsig.2020.109675
Yang, H., Wang, Z., Hu, S., Chen, L., Li, W., and Yang, Z. (2023). miRNA-874-3p inhibits the migration, invasion and proliferation of breast cancer cells by targeting VDAC1. Aging (Albany NY) 15 (3), 705–717. doi:10.18632/aging.204474
Yang, X., Qin, C., Zhao, B., Wang, Y., and Li, Z. (2022). Long noncoding RNA and circular RNA: two rising stars in regulating epithelial-mesenchymal transition of pancreatic cancer. Front. Oncol. 12, 910678. doi:10.3389/fonc.2022.910678
Yang, Y., Yuan, L., Du, X., Zhou, K., Qin, L., Wang, L., et al. (2021). Involvement of epithelia-derived exosomes in chronic respiratory diseases. Biomed. Pharmacother. 143, 112189. doi:10.1016/j.biopha.2021.112189
Yang, Z., Shi, J., Xie, J., Wang, Y., Sun, J., Liu, T., et al. (2020a). Large-scale generation of functional mRNA-encapsulating exosomes via cellular nanoporation. Nat. Biomed. Eng. 4 (1), 69–83. doi:10.1038/s41551-019-0485-1
Yao, F., Shi, W., Fang, F., Lv, M. Y., Xu, M., Wu, S. Y., et al. (2023). Exosomal miR-196a-5p enhances radioresistance in lung cancer cells by downregulating NFKBIA. Kaohsiung J. Med. Sci. 39 (6), 554–564. doi:10.1002/kjm2.12673
Yasuda, T., Koiwa, M., Yonemura, A., Miyake, K., Kariya, R., Kubota, S., et al. (2021). Inflammation-driven senescence-associated secretory phenotype in cancer-associated fibroblasts enhances peritoneal dissemination. Cell Rep. 34 (8), 108779. doi:10.1016/j.celrep.2021.108779
Yin, C., Evason, K. J., Asahina, K., and Stainier, D. Y. R. (2013). Hepatic stellate cells in liver development, regeneration, and cancer. J. Clin. Invest. 123 (5), 1902–1910. doi:10.1172/JCI66369
Yin, H., Yu, S., Xie, Y., Dai, X., Dong, M., Sheng, C., et al. (2021). Cancer-associated fibroblasts-derived exosomes upregulate microRNA-135b-5p to promote colorectal cancer cell growth and angiogenesis by inhibiting thioredoxin-interacting protein. Cell Signal 84, 110029. doi:10.1016/j.cellsig.2021.110029
Yuan, H., Chen, B., Chai, R., Gong, W., Wan, Z., Zheng, B., et al. (2022). Loss of exosomal micro-RNA-200b-3p from hypoxia cancer-associated fibroblasts reduces sensitivity to 5-flourouracil in colorectal cancer through targeting high-mobility group box 3. Front. Oncol. 12, 920131. doi:10.3389/fonc.2022.920131
Zanotelli, M. R., Zhang, J., and Reinhart-King, C. A. (2021). Mechanoresponsive metabolism in cancer cell migration and metastasis. Cell Metab. 33 (7), 1307–1321. doi:10.1016/j.cmet.2021.04.002
Zeltz, C., Alam, J., Liu, H., Erusappan, P. M., Hoschuetzky, H., Molven, A., et al. (2019). α11β1 integrin is induced in a subset of cancer-associated fibroblasts in desmoplastic tumor stroma and mediates in vitro cell migration. Cancers (Basel) 11 (6), 765. doi:10.3390/cancers11060765
Zhang, H., Deng, T., Liu, R., Ning, T., Yang, H., Liu, D., et al. (2020c). CAF secreted miR-522 suppresses ferroptosis and promotes acquired chemo-resistance in gastric cancer. Mol. Cancer 19 (1), 43. doi:10.1186/s12943-020-01168-8
Zhang, H. W., Shi, Y., Liu, J. B., Wang, H. M., Wang, P. Y., Wu, Z. J., et al. (2021b). Cancer-associated fibroblast-derived exosomal microRNA-24-3p enhances colon cancer cell resistance to MTX by down-regulating CDX2/HEPH axis. J. Cell Mol. Med. 25 (8), 3699–3713. doi:10.1111/jcmm.15765
Zhang, M., Guan, W. B., Li, J. L., Li, L. X., Wang, K. Z., Wang, R. F., et al. (2022a). Cancer-associated fibroblasts subtypes and role in invasion and metastasis of gastric cancer. Neoplasma 69 (6), 1277–1288. doi:10.4149/neo_2022_220513N511
Zhang, N., Wang, Y., Liu, H., and Shen, W. (2020e). Extracellular vesicle encapsulated microRNA-320a inhibits endometrial cancer by suppression of the HIF1α/VEGFA axis. Exp. Cell Res. 394 (2), 112113. doi:10.1016/j.yexcr.2020.112113
Zhang, T., Zhang, P., and Li, H. X. (2021a). CAFs-derived exosomal miRNA-130a confers cisplatin resistance of NSCLC cells through PUM2-dependent packaging. Int. J. Nanomedicine 16, 561–577. doi:10.2147/IJN.S271976
Zhang, X., Han, C., Du, B., Zhang, W., and He, G. (2022e). Isolation and identification of adipose stem cell exosomes and the study of its potential as drug delivery carrier in vitro. Appl. Biochem. Biotechnol. 194 (6), 2594–2603. doi:10.1007/s12010-022-03835-6
Zhang, X., Xu, Y., Ma, L., Yu, K., Niu, Y., Xu, X., et al. (2022b). Essential roles of exosome and circRNA_101093 on ferroptosis desensitization in lung adenocarcinoma. Cancer Commun. (Lond) 42 (4), 287–313. doi:10.1002/cac2.12275
Zhang, X., Zhang, Y., Qiu, X., Cai, J., Yang, Z., and Song, F. (2022c). Extracellular vesicles derived from lung cancer cells induce transformation of normal fibroblasts into lung cancer-associated fibroblasts and promote metastasis of lung cancer by delivering lncRNA HOTAIR. Stem Cells Int. 2022, 3805013. doi:10.1155/2022/3805013
Zhang, Y., Luo, J., Gui, X., Zheng, Y., Schaar, E., Liu, G., et al. (2023). Bioengineered nanotechnology for nucleic acid delivery. J. Control Release 364, 124–141. doi:10.1016/j.jconrel.2023.10.034
Zhang, Y., Wang, S., Lai, Q., Fang, Y., Wu, C., Liu, Y., et al. (2020b). Cancer-associated fibroblasts-derived exosomal miR-17-5p promotes colorectal cancer aggressive phenotype by initiating a RUNX3/MYC/TGF-β1 positive feedback loop. Cancer Lett. 491, 22–35. doi:10.1016/j.canlet.2020.07.023
Zhang, Y., Yin, C., Wei, C., Xia, S., Qiao, Z., Zhang, X. W., et al. (2022d). Exosomal miR-625-3p secreted by cancer-associated fibroblasts in colorectal cancer promotes EMT and chemotherapeutic resistance by blocking the CELF2/WWOX pathway. Pharmacol. Res. 186, 106534. doi:10.1016/j.phrs.2022.106534
Zhang, Y., Zhao, J., Ding, M., Su, Y., Cui, D., Jiang, C., et al. (2020d). Loss of exosomal miR-146a-5p from cancer-associated fibroblasts after androgen deprivation therapy contributes to prostate cancer metastasis. J. Exp. Clin. Cancer Res. 39 (1), 282. doi:10.1186/s13046-020-01761-1
Zhang, Z., Gao, Z., Rajthala, S., Sapkota, D., Dongre, H., Parajuli, H., et al. (2020a). Metabolic reprogramming of normal oral fibroblasts correlated with increased glycolytic metabolism of oral squamous cell carcinoma and precedes their activation into carcinoma associated fibroblasts. Cell Mol. Life Sci. 77 (6), 1115–1133. doi:10.1007/s00018-019-03209-y
Zhao, H., Yang, L., Baddour, J., Achreja, A., Bernard, V., Moss, T., et al. (2016). Tumor microenvironment derived exosomes pleiotropically modulate cancer cell metabolism. Elife 5, e10250. doi:10.7554/eLife.10250
Zhao, M., Zhuang, A., and Fang, Y. (2022). Cancer-associated fibroblast-derived exosomal miRNA-320a promotes macrophage M2 polarization in vitro by regulating PTEN/PI3Kγ signaling in pancreatic cancer. J. Oncol. 2022, 9514697. doi:10.1155/2022/9514697
Zheng, J., and Hao, H. (2023). The importance of cancer-associated fibroblasts in targeted therapies and drug resistance in breast cancer. Front. Oncol. 13, 1333839. doi:10.3389/fonc.2023.1333839
Zhou, B., Lei, J. H., Wang, Q., Qu, T. F., Cha, L. C., Zhan, H. X., et al. (2022). Cancer-associated fibroblast-secreted miR-421 promotes pancreatic cancer by regulating the SIRT3/H3K9Ac/HIF-1α axis. Kaohsiung J. Med. Sci. 38 (11), 1080–1092. doi:10.1002/kjm2.12590
Zhu, L., Sun, H. T., Wang, S., Huang, S. L., Zheng, Y., Wang, C. Q., et al. (2020). Isolation and characterization of exosomes for cancer research. J. Hematol. Oncol. 13 (1), 152. doi:10.1186/s13045-020-00987-y
Glossary
Keywords: cancer-associated fibroblasts-derived exosomal miRNA, cancer malignant characteristics, dual regulatory functions, diagnosis, prognosis, therapy
Citation: Gou Z, Li J, Liu J and Yang N (2024) The hidden messengers: cancer associated fibroblasts—derived exosomal miRNAs as key regulators of cancer malignancy. Front. Cell Dev. Biol. 12:1378302. doi: 10.3389/fcell.2024.1378302
Received: 29 January 2024; Accepted: 08 April 2024;
Published: 17 April 2024.
Edited by:
Mingxi Yao, Southern University of Science and Technology, ChinaReviewed by:
Susana García-Silva, Spanish National Cancer Research Center, SpainElisa Tamariz, Universidad Veracruzana, Mexico
Copyright © 2024 Gou, Li, Liu and Yang. This is an open-access article distributed under the terms of the Creative Commons Attribution License (CC BY). The use, distribution or reproduction in other forums is permitted, provided the original author(s) and the copyright owner(s) are credited and that the original publication in this journal is cited, in accordance with accepted academic practice. No use, distribution or reproduction is permitted which does not comply with these terms.
*Correspondence: Na Yang, eWFuZ25Aamx1LmVkdS5jbg==