- 1IRCCS Humanitas Research Hospital, Milan, Italy
- 2Milan Unit, Istituto di Ricerca Genetica e Biomedica (IRGB), National Research Council, Milan, Italy
Pre-B cell leukemia factor 1 (PBX1) is a Three Aminoacid Loop Extension (TALE) homeodomain-containing transcription factor playing crucial roles in organ pattering during embryogenesis, through the formation of nuclear complexes with other TALE class and/or homeobox proteins to regulate target genes. Its contribution to the development of several organs has been elucidated mainly through the study of murine knockout models. A crucial role for human development has been recently highlighted through the discovery of different de novo pathogenic PBX1 variants in children affected by developmental defects. In the adult, PBX1 is expressed in selected tissues such as in the brain, in the gastro-intestinal and urinary systems, or in hematopoietic stem and progenitor cells, while in other organs is barely detectable. When involved in the t(1;19) chromosomal translocation it acts as an oncogene, since the resulting fusion protein drives pre-B cell leukemia, due to the induction of target genes not normally targeted by the native protein. Its aberrant expression has been associated to tumor development, progression, or therapy-resistance as in breast cancer, ovarian cancer or myeloproliferative neoplasm (MPN). On the other hand, in colorectal cancer PBX1 functions as a tumor suppressor, highlighting its context-dependent role. We here discuss differences and analogies of PBX1 roles during embryonic development and in cancer, focusing mainly on the most recent discoveries.
Introduction
PBX1 belongs to a class of homeobox transcription factors called TALE, due to a Three Aminoacid Loop Extension domain located in the homeodomain. The class is composed of two families, the MEINOX (which includes MEIS and PREP proteins) and the PBC (which includes PBX1-4). All TALE transcription factors contain an atypical homeodomain, which allows binding to DNA and to other transcription factors, and two protein-protein interacting domains, which mediate binding to a large variety of HOX proteins and/or to mutually exclusive MEINOX proteins. Like other TALE members, PBX1 is also able to recruit chromatin accessibility regulators, such as the SWI/SNF complexes, and co-repressors, such as histone deacetylases (Longobardi et al., 2014). Therefore, depending on the context, PBX1 can either promote or repress transcription of target genes. According to the current view, context-specific roles are provided by interaction with selected cofactors that confer tissue or cell specificity, by the cis-regulatory landscape, and by the co-expression of other transcription factors that are part of the same gene regulatory network. In addition to acting in concert with different HOX and other TALE-homeodomain partners, PBX1 is also found in “HOX-less” domains (Capellini et al., 2011; Losa et al., 2018). Examples of non-HOX partners are MYOD, whose interaction with the PBX1-MEIS1 complex drives transcription of the Myogenin gene during skeletal muscle differentiation (Berkes et al., 2004), or SMAD4, which, in complex with PBX1 and PREP1, mediates Activin-dependent expression of the FSHβ gene (Bailey et al., 2004), important for ovulation and spermatogenesis. Moreover, in accordance with its expression in embryonic stem cells (ESC) (Chan et al., 2009), PBX1 has been proposed as a “pioneer factor” (Sagerström, 2004), although unequivocal demonstration is still missing; it marks selected genes for transcriptional activation through specific histone binding in closed chromatin, to increase DNA access for other transcription factors and determining cell fate changes, such as in the context of specification of the skeletal muscle and neuronal lineages (Grebbin and Schulte, 2017).
PBX1 has a widespread distribution in many tissues and in different steps of development (Selleri et al., 2019). In human adults it is mainly expressed in glandular tissues, female tissues, the bladder and in subsets of hematopoietic cells (Nagel et al., 2021; Mary et al., 2022). Different isoforms are produced due to alternative splicing, although the function of some of them is unknown. PBX1a is mainly expressed in the brain and in adulthood, PBX1b is typical of the embryo, while PBX1d is expressed in CD4+ T cells. Some PBX1 functions are shared with other PBX family members, mainly PBX2, while others are peculiar of PBX1 (Capellini et al., 2006; Selleri et al., 2019).
PBX1 has long been known as a developmental regulator; its role has been dissected mainly through constitutive and conditional mouse models, as well as through compound mutants of PBX1 and one of the other PBC family members or one of his molecular partners. However, there is increasing evidence that its functions during murine development are conserved in humans (Slavotinek et al., 2017). On the other hand, PBX1 aberrant expression is linked to cancer in several of the tissues in which it plays a role during development, as discussed below.
PBX1 as a developmental regulator
Several studies of Pbx-mutant mouse embryos have revealed PBX1 as a crucial developmental regulator. Among the other PBC family members, only PBX1 is absolutely required for embryonic development since other PBX knockout embryos are viable. PBX1 role has been linked to hematopoietic development (DiMartino et al., 2001), neuronal and cardiovascular patterning (Chang et al., 2008; Vitobello et al., 2011; Sgadò et al., 2012), lung (Li et al., 2014) and diaphragm formation (Russell et al., 2012), pancreas development (Kim et al., 2002), spleen ontogeny (Brendolan et al., 2005), urogenital differentiation (Schnabel et al., 2003a; Schnabel et al., 2003b), face morphogenesis (Losa et al., 2018), skeletal and limb patterning (Selleri et al., 2001; Capellini et al., 2011), among others. More recently, its role in limb morphogenesis including the cell type (mesoderm progenitors) and the time window (initiation of hindlimb bud development) that requires PBX function has been further elucidated through a multi-omics approach (Losa et al., 2023). PBX1 also controls self-renewal and pluripotency of human ESCs by directly regulating NANOG expression (Chan et al., 2009); it is downregulated during ESC early differentiation, but expressed again in ESC–derived hematopoietic stem/progenitor cells (Oshima et al., 2011).
Several of the functions of PBX1 during development that have been discovered through murine models have some correspondence in human developmental anomalies caused by de novo PBX1 heterozygous mutations or aberrant expression. A comprehensive overview of all known human developmental defects due to PBX1 mutations is excellently presented in Mary et al. (Mary et al., 2022). So far, at least forty patients with PBX1 heterozygous mutations or deletions have been described. This number is likely set to rise thanks to the increasing use of whole-exome sequencing techniques. For example, missense variations cause lung hypoplasia, cardiac malformations, and sexual developmental defects; truncating variants are at the basis of deafness or cryptorchidism. Most frequently, PBX1 germline variations are associated with kidney syndromic anomalies, often called CAKUTHED for Congenital Anomalies of the Kidney and Urinary Tract (the most frequent birth defects) with or without Hearing loss, abnormal Ears, or Developmental delay; most commonly renal hypoplasia, sometimes with ocular manifestations (Safgren et al., 2022). Other described developmental defects include face, head, and skeletal anomalies. Mutations can be found in one of the protein-binding domains or in the homeodomain, affecting the ability to form heterodimers with protein partners or to bind DNA, respectively. Other mutations affect the nuclear localization or the nuclear export signals, causing cytoplasmic retention (Mary et al., 2022).
PBX1 exerts its role as a developmental regulator also when expressed in non-embryonic tissues. At the maternal-fetal interface, a subset of decidual natural killer (dNK) cells expresses PBX1 at high level (Zhou et al., 2020). In these cells, PBX1 directly regulates the transcriptional expression of growth-promoting factors including Pleiotrophin and Osteoglycin, to allow proper murine fetal growth. Reduced PBX1 activity in human dNK is frequent in women with a history of unexplained recurrent spontaneous abortion, suggesting that the function of PBX1 in dNK cells is conserved in human.
PBX1 as a regulator of tissue homeostasis
PBX1 acts to establish the proper timing of gene expression also during differentiation of specific cell types beyond morphogenesis and fetal development. For example, in the adult subventricular zone (SVZ) it acts as early regulator of neurogenic cell fate decision and of survival of newly generated neurons, and as pioneer factor for SVZ neurogenesis (Grebbin et al., 2016). In the bone, PBX1, expressed at high levels in osteoprogenitors also in the adult, has been proposed as an attenuator of osteoblast genes transcription through recruitment of chromatin remodeling proteins to the promoters of the osteoblast-related genes osteocalcin and bone sialoprotein. This allows correct gene expression timing and results in matrix maturation and mineral deposition only in fully differentiated osteoblasts, in which PBX1 is no longer expressed, and not in precursors (Gordon et al., 2011), thus contributing to maintaining bone homeostasis. In the hair follicle, PBX1 promotes proliferation, facilitates DNA damage repair, and attenuates senescence and apoptosis of mesenchymal stromal cells (MSCs) (Liu et al., 2019). The homeostasis of the immune system also relies on PBX1 since, together with PREP1, PBX1 mediates the transcriptional activation of IL10 in phagocytes stimulated by apoptotic cells, thus favoring suppression of autoimmunity (Chung et al., 2007). On the other hand, the dominant negative splicing isoform PBX1d, which lacks the DNA and Hox-binding domains, has been recently described as an autoimmunity (Lupus) susceptibility gene that impairs the balance between regulatory and follicular helper CD4+ T cells (Li et al., 2020). This isoform is less stable (Park et al., 2023) and has different DNA binding and co-factor recruitment ability relative to the normal isoform; one of its downstream effectors is CD44, a marker of CD4+ T cell activation (Niu et al., 2017).
A further example of regulation of tissue homeostasis by PBX1 is its function in early hematopoiesis, as detailed below.
PBX1 in the hematopoietic system
The role of PBX1 within the hematopoietic system is time- and context-dependent and only seemingly controversial. During embryonic and fetal development it promotes proliferation of stem and progenitor cells and is therefore required for both primitive (Pillay et al., 2010) and definitive hematopoiesis (DiMartino et al., 2001). However, PBX1 role in the developing hematopoietic system is not limited to promoting proliferation in the embryo, but also to specify the Megakaryocyte (Mk) lineage fate and red blood cell development, in complex with its partner MEIS1, through induction of the GATA1 master regulator of erythropoiesis, at the expenses of myeloid induction (Pillay et al., 2010). PBX1 promotes Mk induction also in vitro from induced pluripotent stem cells (Cullmann et al., 2021) and from human CD34+ cells through direct regulation of PF4 (Okada et al., 2003). PBX1/MEIS1-mediated GATA1 induction has been recently shown to be indirect and to occur through promoting HIF1α transcription (Chung et al., 2021), which is known to regulate erythropoiesis (Zhang et al., 2012). Moreover, PBX1 is essential for lymphoid development starting from the common lymphoid progenitor stage, as demonstrated by elegant RAG1-deficient blastocyst complementation assays (Sanyal et al., 2007). This role is maintained in the adult, impacting on B, T and NK cell number (Xu et al., 2020) and it starts at the level of lymphoid priming in Hematopoietic stem cells (HSCs) (Ficara et al., 2008), although is not apparently linked to proliferation. Similarly, the control of the myeloid vs. erythroid/Mk skewing is maintained in the adult, both in the mouse (Ficara et al., 2013; Muggeo et al., 2021), in which PBX1 is expressed at higher levels in a subgroup of HSCs with Mk potential (Wilson et al., 2015), and in the human (Wang et al., 2022), whereas proliferation promotion does not appear to occur in the adult hematopoietic system at least in steady state. The transition to the adult hematopoietic system is characterized by HSCs becoming quiescent for most of the time, so that their pool is protected by genotoxic stimuli. In this context, rather than acting on proliferation, PBX1 role is linked to preserve HSC potential towards lymphoid, erythroid, and platelet fate at the expenses of other myeloid cell types. Indeed, its absence results in premature myeloid differentiation (Ficara et al., 2013), which might be mediated by downregulating miR-127 (Crisafulli et al., 2019). Interestingly, aberrant expression of PBX1 contributes to the development of cancer involving those lineages (see below).
Overall, PBX1 promotes cell renewal. In some context or cell types, this translates into promoting proliferation; in other contexts, such as in HSCs, preservation of self-renewal capacity is achieved by limiting proliferation.
PBX1 as cancer contributor
PBX1 functions in the adult are less studied compared to its roles during development. However, its aberrant expression is linked to many different types of cancer.
PBX1 contributes to the development of cancer involving the tissues and cell lineages that it normally regulates during development (Table 1; Figure 1). Examples of affected lineages within the hematopoietic system are B cells, HSCs and Mk-Erythrocyte Progenitors (MEP). The t (Russell et al., 2012; Longobardi et al., 2014) translocation that occurs in pro- or pre-B cells generates the E2A-PBX1 chimeric transcription factor (also named TCF3::PBX1) that contains the N-terminal transactivation domain of the lymphoid lineage E2A transcription factor fused to the C-terminal DNA-binding homeodomain of PBX1. This initiating event, followed by a secondary mutation, results in pediatric B Acute Lymphoblastic Leukemia (B-ALL), accounting for 5%–10% of pediatric ALL (Yin et al., 2023); adult cases have also been reported (Burmeister et al., 2023). E2A-PBX1-dependent gene activation and leukemic cell growth rely on the interaction of the fusion protein with the Mediator complex through the MED1 subunit (Lee et al., 2021) and on RUNX1 coactivation through direct binding to the PBX1 homeodomain (Pi et al., 2020). Moreover, E2A-PBX1-mediated oncogenesis occurs through self-oligomerization, so that dimerization with protein partners that normally stabilize and regulate PBX1 import into the nucleus become dispensable (Lin et al., 2019). E2A-PBX1 fusions have been found prenatally, which might explain the highest occurrence of this type of B-ALL in children (Hein et al., 2019). E2A-PBX1 fusion in isolated cases of B-lymphoblastic lymphomas (B-LBL), rare subtypes of non-Hodgkin lymphoma seen primarily in children or young adults, have also been recently reported (Kubota-Tanaka et al., 2019; Okura et al., 2020). Other genomic alterations occurring in B cells are PBX1 duplications, which give rise to Multiple Myeloma (Trasanidis et al., 2022) or to Hodgkin lymphoma (Nagel et al., 2021; Nagel et al., 2023).
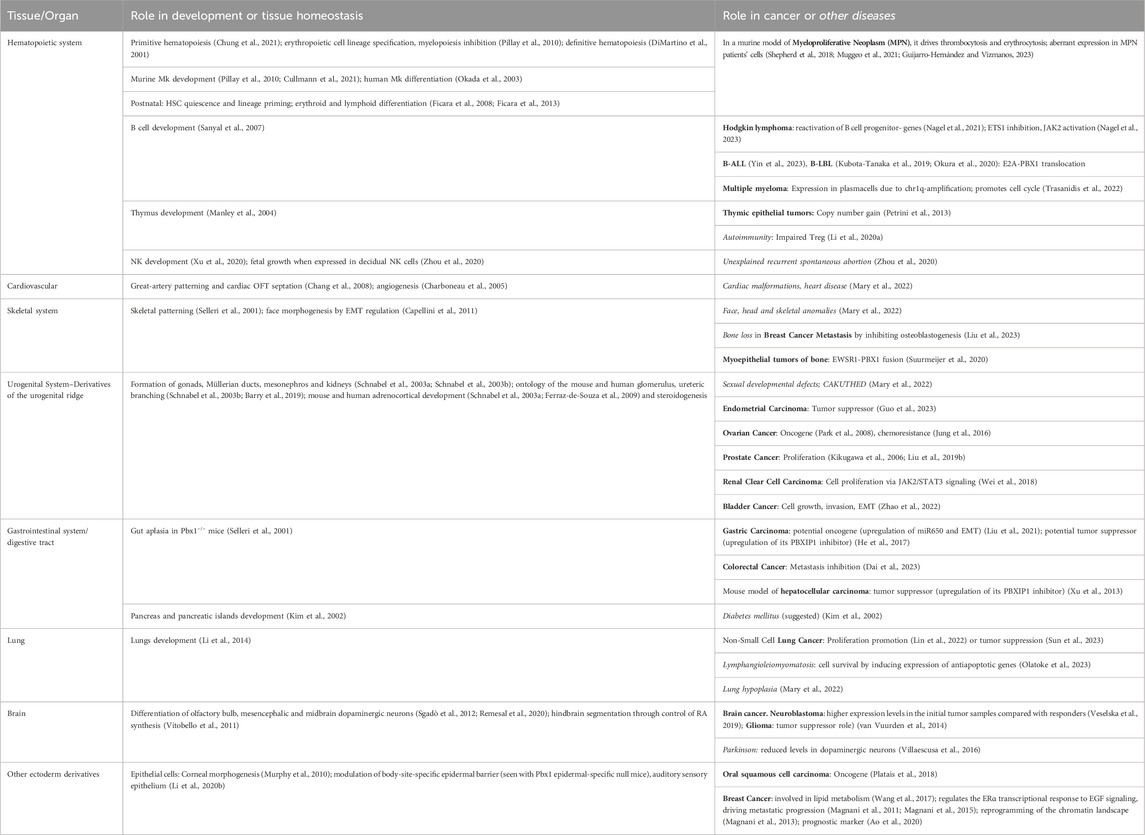
TABLE 1. Summary of the role of PBX1 during development, mostly discovered through the analysis of mutant mice or embryos, and role in cancer or other diseases affecting the same tissues whose development depend on PBX1. Most diseases present in the table are discussed or quoted in the text. We apologize for the organs, cells, or diseases that we skipped for brevity.
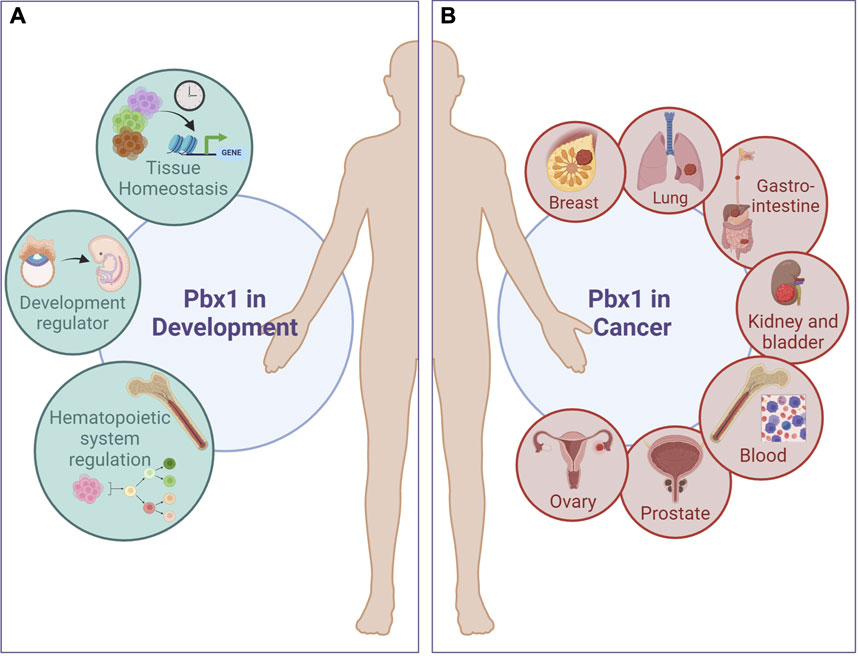
FIGURE 1. Pbx1 functions in development and cancer. PBX1 regulates the development of several type of cancers involving tissues and cell lineages that are normally regulated during development by PBX1 itself. (A), Green circles represent PBX1 physiological functions; (B), red circles depict some of the tumors in which PBX1 promotes carcinogenesis. A more comprehensive list of PBX1-dependent tumors is present in Table 1. Figure was created with biorender.com.
PBX1 may contribute to other hematopoietic cancers also in the absence of genomic alterations, for example, if overexpressed or if expressed in HSCs or MEPs in the presence of other driver mutations, such as the somatic V617F mutation in the JAK2 gene typical of myeloproliferative neoplasm (MPN). MPN are heterogeneous blood malignancies associated with increased risk of thrombotic events and of leukemic transformation. They are characterized by the aberrant proliferation of one or more myeloid lineages and progressive bone marrow fibrosis (Coltro et al., 2021). In particular, the polycythemia vera and the essential thrombocythemia subtypes are distinguished by an excess of red blood cells or of platelets, respectively, although the mutation occurs at the HSC or at the progenitors’ level, altering their fate. Since PBX1 is one of the key factors regulating the balance between self-renewal and differentiation in postnatal HSCs (Ficara et al., 2008), and given its action in preserving lymphoid, erythroid and Mk potential (Ficara et al., 2013; Wang et al., 2022), it is not surprising that it exerts a role in blood malignancies in which the cells of origin are HSCs or hematopoietic progenitors, as in myeloid neoplasms. By exploiting an MPN mouse model bearing the JAK2V617F mutation, we demonstrated that PBX1 expression in JAK2V617F HSCs is necessary to sustain MPN (Muggeo et al., 2021), in accordance with a previous report indicating that its overexpression in JAK2V617F HSCs contributes to sustaining an MPN phenotype in animal models (Shepherd et al., 2018). In silico analysis revealed an inverse correlation between the genes differentially expressed (DE) in human MPN and those DE in Pbx1-null HSCs (Muggeo et al., 2021), providing further evidence of the involvement of PBX1 in human MPN. In addition, PBXIP1 (PBX homeobox interacting protein 1), which codes for a protein that inhibits the transcriptional activation potential of PBX1 by preventing its binding to DNA, is downregulated in MPN patients (Guijarro-Hernández and Vizmanos, 2023). The positive regulation of STAT3 transcription by PBX1 binding to its promoter that has been reported in other tissues (Jung et al., 2016) might represent the underlying mechanism through which its expression contributes to MPN development/maintenance; STAT3, part of the JAK/STAT pathway that acts as an effector of the mutations causing MPN, is widely expressed within the hematopoietic system and its transcription is downregulated in the absence of PBX1 in purified HSCs (Ficara et al., 2008).
The action of another PBX1-MEIS1 downstream effector, the previously quoted HIF1α, might represent another mechanism through which PBX1 exert its role in MPN cells; indeed, HIF1α has been recently proposed as a new potential therapeutic target in these diseases (Baumeister et al., 2020). HIF-1α is a master transcriptional regulator of the response to decreased oxygen levels; it has been shown to regulate HSC quiescence and erythroid differentiation within their hypoxic niche (Takubo et al., 2010; Zhang et al., 2012). HSCs utilize glycolysis as main source of energy prior to maturation and HIF1α is the key transcriptional regulator of glycolytic metabolism in these cells, thus regulating their energy metabolism and differentiation.
Other PBX1 downstream effectors in MPN are the Mk marker CD61, which is downregulated upon PBX1 deletion in the murine MPN model, and the novel early myeloid differentiation marker Embigin, which is instead upregulated upon PBX1 deletion (Muggeo et al., 2021), although direct regulation has not been demonstrated.
Besides the hematopoietic system, PBX1 is involved in several solid tumors. In some instances, increased expression of PBX1 is linked to proliferation promotion, as in breast cancer. Breast cancer represents the major malignancy in women and in approximately two-thirds of cases the pathology is driven by the estrogen receptor ERα (Tyson et al., 2011). By acting as a pioneer factor, PBX1 is essential for the ERα-mediated transcriptional response, promoting greater tumor proliferation and aggressiveness (Magnani et al., 2011; Magnani et al., 2015). A positive correlation of PBX1 and ERα expression levels in breast cancer has been demonstrated. Moreover, depletion of PBX1 inhibits tumor proliferation in the presence of estrogenic stimuli (Magnani et al., 2011).
In addition to acting on primary tumor cells, PBX1 also plays a role in promoting metastasis. In 20%–30% of cases, ERα-positive breast cancer patients treated with endocrine therapy eventually relapse and progress to metastatic disease (Musgrove and Sutherland, 2009). The acquisition of resistance to endocrine therapy is a long-term sequential process accompanied by an important transcriptional reprogramming of the cell, suggesting that remodeling of the chromatin landscape may play a central role in this process. In this context, PBX1 was shown to promote resistance to endocrine therapy in vitro by controlling the expression of several genes implicated in this process (Magnani et al., 2013).
Metastasis, including bone metastasis, is the main cause of disease-related mortality in breast cancer. When breast cancer cells colonize the bone, they release cytokines such as osteopontin and RANKL, which act on osteoclast precursors promoting their maturation into bone resorbing osteoclasts (Granata et al., 2022). In addition, exosomes released by breast cancer cells contain reduced levels of miR-6881-3p, recently shown to target PBX1 in osteoblasts thus negatively regulating the expression of its downstream effectors. This results in reduced osteoblastogenesis that likely further contributes to bone loss, although this mechanism has not been shown in vivo yet (Liu et al., 2023). Therefore, PBX1 upregulation is involved in driving tumorigenesis and metastasis in breast cancer by acting on malignant cells as well as in the tumor microenvironment.
The association between PBX1 and estrogen signaling was also confirmed in the estrogen-mediated bladder cancer, in which PBX1 expression levels were positively related to tumor size, lymph node metastasis and poorer survival (Zhao et al., 2022).
Other examples of solid tumors in which PBX1 promotes proliferation include high grade clear renal carcinoma, in which PBX1 was positively related to cell cycle progression and proliferation through the JAK2/STAT3 pathway (Wei et al., 2018). In prostate cancer cell lines, PBX1-HOXC8 heterocomplex formation was shown to drive cell growth (Kikugawa et al., 2006); PBX1-mediated induction of cell proliferation and resistance against anti-cancer drugs was shown also in the patients’ cells (Liu Y. et al., 2019). In ovarian cancer, the association of PBX1 and proliferation was observed to be NOTCH3-dependent (Park et al., 2008), and PBX1-mediated chemoresistance to be related to PBX1 binding to STAT3 promoter, positively regulating its transcription (Jung et al., 2016). Along with these, PBX1 over-expression led to a higher number of in vitro colonies in gastric cancer cell lines (He et al., 2017); PBX1 was indeed found to be upregulated in patients’ samples and to promote gastric cancer cell proliferation and invasion through promoting miR-650 transcription (Liu et al., 2021).
Despite PBX1 is a transcription factor, lacking intrinsic enzymatic activity, it has recently been considered as a therapeutic target. One strategy is to destabilize its binding to the DNA or to prevent the formation of hetero-homeodomain PBX1-containing transcriptional complexes, thanks to one or more small molecules. This approach proved to be successful in different cancer cell lines and in an in vivo model of ovarian cancer (Shen et al., 2021; Trasanidis et al., 2022). Another strategy is the use of short peptide antagonists able to interfere with PBX-HOX interactions, recently shown to be effective in vitro and in vivo on lymphangioleiomyomatosis, a rare lung disease that depends on PBX1-HOXD11 interaction (Olatoke et al., 2023). Whether these approaches represent the basis for generating novel drugs to cure human cancer is still to be demonstrated.
PBX1 as tumor suppressor
In some cancers, decreased expression of PBX1 favors malignancy. This is the case of endometrial carcinoma, in which reduced expression of PBX1 causes increased WNT signaling owing to downregulation of its direct target SFRP4, a known WNT pathway inhibitor (Guo et al., 2023). A similar mechanism occurs in colorectal cancer. In this tumor, lack of PBX1 was shown to cause absence of DCDC2 suppression, resulting in increased WNT signaling and increased spindle function, which in turn lead to cell proliferation and metastasis (Dai et al., 2023). In non-small cell lung cancer PBX1 was recently shown to inhibit tumor growth; in patients’ tissues it is indeed downregulated through binding to the ubiquitin ligase TRIM6, which drives PBX1 proteasomal degradation (Sun et al., 2023). However, this is in contrast with a previous report demonstrating a positive role of PBX1 in cell cycle progression (Lin et al., 2022). Contradictory roles of PBX1 are also present in the framework of gastric cancer. At variance with the previously quoted tumor-inducing role in this type of cancer (He et al., 2017; Liu et al., 2021), other authors reported overexpression of the PBX1 inhibitor PBXIP1 in gastric cancer patients, leading to repressed PBX1 transcriptional activity and promotion of cell proliferation, migration, and invasion (Feng et al., 2015). Other cancers in which PBX1 exert a tumor suppressor role are liver cancer (Xu et al., 2013), glioma (van Vuurden et al., 2014) and oral carcinoma (Platais et al., 2018).
Conclusion
PBX1 is an important player in development, adult tissue homeostasis and cancer. It can either promote proliferation, as in embryonic/fetal growth and in tumor progression, or act as a brake on cell expansion as in the hematopoietic system or in tumors in which it functions as tumor suppressor. In murine models, its absence results in premature differentiation in different cell types including those belonging to the hematopoietic system (Ficara et al., 2008; Ficara et al., 2013), vascular (Hurtado et al., 2015) and bone tissues (Selleri et al., 2001). Its pleiotropic role, which depends on the cellular context, requires tight regulation. Indeed, both increased or decreased expression leads to disruption of cellular homeostasis and disease, although the mechanisms are not completely clear and deserve further studies.
Author contributions
LC: Visualization, Writing–original draft. MB: Visualization, Writing–original draft. ML: Writing–original draft. CS: Writing–review and editing. FF: Conceptualization, Funding acquisition, Project administration, Supervision, Writing–original draft, Writing–review and editing.
Funding
The author(s) declare financial support was received for the research, authorship, and/or publication of this article. Funding was received from Cariplo and Telethon Foundations, Grant no. 2022-0576, Project no. GJC21072 and from AIRC Investigator Grant—IG 2023 no. 29245, both to FF. CS received funding from the Italian Ministry of Health, Grant no. RF-2018-12367680.
Conflict of interest
The authors declare that the research was conducted in the absence of any commercial or financial relationships that could be construed as a potential conflict of interest.
The author(s) declared that they were an editorial board member of Frontiers, at the time of submission. This had no impact on the peer review process and the final decision.
Publisher’s note
All claims expressed in this article are solely those of the authors and do not necessarily represent those of their affiliated organizations, or those of the publisher, the editors and the reviewers. Any product that may be evaluated in this article, or claim that may be made by its manufacturer, is not guaranteed or endorsed by the publisher.
References
Ao, X., Ding, W., Ge, H., Zhang, Y., Ding, D., and Liu, Y. (2020). PBX1 is a valuable prognostic biomarker for patients with breast cancer. Exp. Ther. Med. 20 (1), 385–394. doi:10.3892/etm.2020.8705
Bailey, J. S., Rave-Harel, N., McGillivray, S. M., Coss, D., and Mellon, P. L. (2004). Activin regulation of the follicle-stimulating hormone beta-subunit gene involves Smads and the TALE homeodomain proteins Pbx1 and Prep1. Mol. Endocrinol. 18 (5), 1158–1170. doi:10.1210/me.2003-0442
Barry, D. M., McMillan, E. A., Kunar, B., Lis, R., Zhang, T., Lu, T., et al. (2019). Molecular determinants of nephron vascular specialization in the kidney. Nat. Commun. 10 (1), 5705. doi:10.1038/s41467-019-12872-5
Baumeister, J., Chatain, N., Hubrich, A., Maié, T., Costa, I. G., Denecke, B., et al. (2020). Hypoxia-inducible factor 1 (HIF-1) is a new therapeutic target in JAK2V617F-positive myeloproliferative neoplasms. Leukemia 34 (4), 1062–1074. doi:10.1038/s41375-019-0629-z
Berkes, C. A., Bergstrom, D. A., Penn, B. H., Seaver, K. J., Knoepfler, P. S., and Tapscott, S. J. (2004). Pbx marks genes for activation by MyoD indicating a role for a homeodomain protein in establishing myogenic potential. Mol. Cell 14 (4), 465–477. doi:10.1016/s1097-2765(04)00260-6
Brendolan, A., Ferretti, E., Salsi, V., Moses, K., Quaggin, S., Blasi, F., et al. (2005). A Pbx1-dependent genetic and transcriptional network regulates spleen ontogeny. Development 132 (13), 3113–3126. doi:10.1242/dev.01884
Burmeister, T., Gröger, D., Gökbuget, N., Spriewald, B., Starck, M., Elmaagacli, A., et al. (2023). Molecular characterization of TCF3::PBX1 chromosomal breakpoints in acute lymphoblastic leukemia and their use for measurable residual disease assessment. Sci. Rep. 13 (1), 15167. doi:10.1038/s41598-023-42294-9
Capellini, T. D., Di Giacomo, G., Salsi, V., Brendolan, A., Ferretti, E., Srivastava, D., et al. (2006). Pbx1/Pbx2 requirement for distal limb patterning is mediated by the hierarchical control of Hox gene spatial distribution and Shh expression. Development 133 (11), 2263–2273. doi:10.1242/dev.02395
Capellini, T. D., Zappavigna, V., and Selleri, L. (2011). Pbx homeodomain proteins: TALEnted regulators of limb patterning and outgrowth. Dev. Dyn. 240 (5), 1063–1086. doi:10.1002/dvdy.22605
Chan, K. K., Zhang, J., Chia, N. Y., Chan, Y. S., Sim, H. S., Tan, K. S., et al. (2009). KLF4 and PBX1 directly regulate NANOG expression in human embryonic stem cells. Stem Cells 27 (9), 2114–2125. doi:10.1002/stem.143
Chang, C. P., Stankunas, K., Shang, C., Kao, S. C., Twu, K. Y., and Cleary, M. L. (2008). Pbx1 functions in distinct regulatory networks to pattern the great arteries and cardiac outflow tract. Development 135 (21), 3577–3586. doi:10.1242/dev.022350
Charboneau, A., East, L., Mulholland, N., Rohde, M., and Boudreau, N. (2005). Pbx1 is required for Hox D3-mediated angiogenesis. Angiogenesis 8 (4), 289–296. doi:10.1007/s10456-005-9016-7
Chung, E. Y., Liu, J., Homma, Y., Zhang, Y., Brendolan, A., Saggese, M., et al. (2007). Interleukin-10 expression in macrophages during phagocytosis of apoptotic cells is mediated by homeodomain proteins Pbx1 and Prep-1. Immunity 27 (6), 952–964. doi:10.1016/j.immuni.2007.11.014
Chung, H. Y., Lin, B. A., Lin, Y. X., Chang, C. W., Tzou, W. S., Pei, T. W., et al. (2021). Meis1, Hi1α, and GATA1 are integrated into a hierarchical regulatory network to mediate primitive erythropoiesis. FASEB J. 35 (10), e21915. doi:10.1096/fj.202001044RRR
Coltro, G., Loscocco, G. G., and Vannucchi, A. M. (2021). Classical Philadelphia-negative myeloproliferative neoplasms (MPNs): a continuum of different disease entities. Int. Rev. Cell Mol. Biol. 365, 1–69. doi:10.1016/bs.ircmb.2021.09.001
Crisafulli, L., Muggeo, S., Uva, P., Wang, Y., Iwasaki, M., Locatelli, S., et al. (2019). MicroRNA-127-3p controls murine hematopoietic stem cell maintenance by limiting differentiation. Haematologica 104 (9), 1744–1755. doi:10.3324/haematol.2018.198499
Cullmann, K., Jahn, M., Spindler, M., Schenk, F., Manukjan, G., Mucci, A., et al. (2021). Forming megakaryocytes from murine-induced pluripotent stem cells by the inducible overexpression of supporting factors. Res. Pract. Thromb. Haemost. 5 (1), 111–124. doi:10.1002/rth2.12453
Dai, W., Liu, Y., Zhang, T., Huang, Z., Xu, X., Zhao, Z., et al. (2023). Spindle function and Wnt pathway inhibition by PBX1 to suppress tumor progression via downregulating DCDC2 in colorectal cancer. Oncogenesis 12 (1), 3. doi:10.1038/s41389-023-00448-4
DiMartino, J. F., Selleri, L., Traver, D., Firpo, M. T., Rhee, J., Warnke, R., et al. (2001). The Hox cofactor and proto-oncogene Pbx1 is required for maintenance of definitive hematopoiesis in the fetal liver. Blood 98 (3), 618–626. doi:10.1182/blood.v98.3.618
Feng, Y., Li, L., Zhang, X., Zhang, Y., Liang, Y., Lv, J., et al. (2015). Hematopoietic pre-B cell leukemia transcription factor interacting protein is overexpressed in gastric cancer and promotes gastric cancer cell proliferation, migration, and invasion. Cancer Sci. 106 (10), 1313–1322. doi:10.1111/cas.12754
Ferraz-de-Souza, B., Martin, F., Mallet, D., Hudson-Davies, R. E., Cogram, P., Lin, L., et al. (2009). CBP/p300-interacting transactivator, with Glu/Asp-rich C-terminal domain, 2, and pre-B-cell leukemia transcription factor 1 in human adrenal development and disease. J. Clin. Endocrinol. Metab. 94 (2), 678–683. doi:10.1210/jc.2008-1064
Ficara, F., Crisafulli, L., Lin, C., Iwasaki, M., Smith, K. S., Zammataro, L., et al. (2013). Pbx1 restrains myeloid maturation while preserving lymphoid potential in hematopoietic progenitors. J. Cell Sci. 126 (Pt 14), 3181–3191. doi:10.1242/jcs.125435
Ficara, F., Murphy, M. J., Lin, M., and Cleary, M. L. (2008). Pbx1 regulates self-renewal of long-term hematopoietic stem cells by maintaining their quiescence. Cell Stem Cell 2 (5), 484–496. doi:10.1016/j.stem.2008.03.004
Gordon, J. A., Hassan, M. Q., Koss, M., Montecino, M., Selleri, L., van Wijnen, A. J., et al. (2011). Epigenetic regulation of early osteogenesis and mineralized tissue formation by a HOXA10-PBX1-associated complex. Cells Tissues Organs 194 (2-4), 146–150. doi:10.1159/000324790
Granata, V., Crisafulli, L., Nastasi, C., Ficara, F., and Sobacchi, C. (2022). Bone marrow niches and tumour cells: lights and shadows of a mutual relationship. Front. Immunol. 13, 884024. doi:10.3389/fimmu.2022.884024
Grebbin, B. M., Hau, A. C., Groß, A., Anders-Maurer, M., Schramm, J., Koss, M., et al. (2016). Pbx1 is required for adult subventricular zone neurogenesis. Development 143 (13), 2281–2291. doi:10.1242/dev.128033
Grebbin, B. M., and Schulte, D. (2017). PBX1 as pioneer factor: a case still open. Front. Cell Dev. Biol. 5, 9. doi:10.3389/fcell.2017.00009
Guijarro-Hernández, A., and Vizmanos, J. L. (2023). Transcriptomic comparison of bone marrow CD34 + cells and peripheral blood neutrophils from ET patients with JAK2 or CALR mutations. BMC Genom Data 24 (1), 40. doi:10.1186/s12863-023-01142-5
Guo, L., Chen, H., Chen, J., Gao, C., Fu, X., Zhou, S., et al. (2023). PBX1-promoted SFRP4 transcription inhibits cell proliferation and epithelial-mesenchymal transition in endometrial carcinoma. Tissue Cell 82, 102083. doi:10.1016/j.tice.2023.102083
He, C., Wang, Z., Zhang, L., Yang, L., Li, J., Chen, X., et al. (2017). A hydrophobic residue in the TALE homeodomain of PBX1 promotes epithelial-to-mesenchymal transition of gastric carcinoma. Oncotarget 8 (29), 46818–46833. doi:10.18632/oncotarget.17473
Hein, D., Dreisig, K., Metzler, M., Izraeli, S., Schmiegelow, K., Borkhardt, A., et al. (2019). The preleukemic TCF3-PBX1 gene fusion can be generated in utero and is present in ≈0.6% of healthy newborns. Blood 134 (16), 1355–1358. doi:10.1182/blood.2019002215
Hurtado, R., Zewdu, R., Mtui, J., Liang, C., Aho, R., Kurylo, C., et al. (2015). Pbx1-dependent control of VMC differentiation kinetics underlies gross renal vascular patterning. Development 142 (15), 2653–2664. doi:10.1242/dev.124776
Jung, J. G., Shih, I. M., Park, J. T., Gerry, E., Kim, T. H., Ayhan, A., et al. (2016). Ovarian cancer chemoresistance relies on the stem cell reprogramming factor PBX1. Cancer Res. 76 (21), 6351–6361. doi:10.1158/0008-5472.CAN-16-0980
Kikugawa, T., Kinugasa, Y., Shiraishi, K., Nanba, D., Nakashiro, K., Tanji, N., et al. (2006). PLZF regulates Pbx1 transcription and Pbx1-HoxC8 complex leads to androgen-independent prostate cancer proliferation. Prostate 66 (10), 1092–1099. doi:10.1002/pros.20443
Kim, S. K., Selleri, L., Lee, J. S., Zhang, A. Y., Gu, X., Jacobs, Y., et al. (2002). Pbx1 inactivation disrupts pancreas development and in Ipf1-deficient mice promotes diabetes mellitus. Nat. Genet. 30 (4), 430–435. doi:10.1038/ng860
Kubota-Tanaka, M., Osumi, T., Miura, S., Tsujimoto, H., Imamura, T., Nishimura, A., et al. (2019). B-lymphoblastic lymphoma with TCF3-PBX1 fusion gene. Haematologica 104 (1), e35–e37. doi:10.3324/haematol.2018.199885
Lee, Y. L., Ito, K., Pi, W. C., Lin, I. H., Chu, C. S., Malik, S., et al. (2021). Mediator subunit MED1 is required for E2A-PBX1-mediated oncogenic transcription and leukemic cell growth. Proc. Natl. Acad. Sci. U. S. A. 118 (6), e1922864118. doi:10.1073/pnas.1922864118
Li, J., Zhang, T., Ramakrishnan, A., Fritzsch, B., Xu, J., Wong, E. Y. M., et al. (2020b). Dynamic changes in cis-regulatory occupancy by Six1 and its cooperative interactions with distinct cofactors drive lineage-specific gene expression programs during progressive differentiation of the auditory sensory epithelium. Nucleic Acids Res. 48 (6), 2880–2896. doi:10.1093/nar/gkaa012
Li, W., Elshikha, A. S., Cornaby, C., Teng, X., Abboud, G., Brown, J., et al. (2020a). T cells expressing the lupus susceptibility allele Pbx1d enhance autoimmunity and atherosclerosis in dyslipidemic mice. JCI Insight 5 (11), e138274. doi:10.1172/jci.insight.138274
Li, W., Lin, C. Y., Shang, C., Han, P., Xiong, Y., Lin, C. J., et al. (2014). Pbx1 activates Fgf10 in the mesenchyme of developing lungs. Genesis 52 (5), 399–407. doi:10.1002/dvg.22764
Lin, C. H., Wang, Z., Duque-Afonso, J., Wong, S. H., Demeter, J., Loktev, A. V., et al. (2019). Oligomeric self-association contributes to E2A-PBX1-mediated oncogenesis. Sci. Rep. 9 (1), 4915. doi:10.1038/s41598-019-41393-w
Lin, Z., Li, Y., Han, X., Fu, Z., Tian, Z., and Li, C. (2022). Targeting SPHK1/PBX1 Axis induced cell cycle arrest in non-small cell lung cancer. Int. J. Mol. Sci. 23 (21), 12741. doi:10.3390/ijms232112741
Liu, F., Shi, J., Zhang, Y., Lian, A., Han, X., Zuo, K., et al. (2019a). NANOG attenuates hair follicle-derived mesenchymal stem cell senescence by upregulating PBX1 and activating AKT signaling. Oxid. Med. Cell Longev. 2019, 4286213. doi:10.1155/2019/4286213
Liu, J., Wang, L., Li, J., and Xu, Y. (2021). Upregulation of microRNA-650 by PBX1 is correlated with the development of Helicobacter pylori-associated gastric carcinoma. Neoplasma 68 (2), 262–272. doi:10.4149/neo_2020_200806N823
Liu, Y., Xu, X., Lin, P., He, Y., Zhang, Y., Cao, B., et al. (2019b). Inhibition of the deubiquitinase USP9x induces pre-B cell homeobox 1 (PBX1) degradation and thereby stimulates prostate cancer cell apoptosis. J. Biol. Chem. 294 (12), 4572–4582. doi:10.1074/jbc.RA118.006057
Liu, Z., Zhang, N., Xin, B., Shi, Y., Liang, Z., Wan, Y., et al. (2023). Exosomes from LSD1 knockdown breast cancer cells activate osteoclastogenesis and inhibit osteoblastogenesis. Int. J. Biol. Macromol. 235, 123792. doi:10.1016/j.ijbiomac.2023.123792
Longobardi, E., Penkov, D., Mateos, D., De Florian, G., Torres, M., and Blasi, F. (2014). Biochemistry of the tale transcription factors PREP, MEIS, and PBX in vertebrates. Dev. Dyn. 243 (1), 59–75. doi:10.1002/dvdy.24016
Losa, M., Barozzi, I., Osterwalder, M., Hermosilla-Aguayo, V., Morabito, A., Chacón, B. H., et al. (2023). A spatio-temporally constrained gene regulatory network directed by PBX1/2 acquires limb patterning specificity via HAND2. Nat. Commun. 14 (1), 3993. doi:10.1038/s41467-023-39443-z
Losa, M., Risolino, M., Li, B., Hart, J., Quintana, L., Grishina, I., et al. (2018). Face morphogenesis is promoted by Pbx-dependent EMT via regulation of Snail1 during frontonasal prominence fusion. Development 145 (5), dev157628. doi:10.1242/dev.157628
Magnani, L., Ballantyne, E. B., Zhang, X., and Lupien, M. (2011). PBX1 genomic pioneer function drives ERα signaling underlying progression in breast cancer. PLoS Genet. 7 (11), e1002368. doi:10.1371/journal.pgen.1002368
Magnani, L., Patten, D. K., Nguyen, V. T., Hong, S. P., Steel, J. H., Patel, N., et al. (2015). The pioneer factor PBX1 is a novel driver of metastatic progression in ERα-positive breast cancer. Oncotarget 6 (26), 21878–21891. doi:10.18632/oncotarget.4243
Magnani, L., Stoeck, A., Zhang, X., Lánczky, A., Mirabella, A. C., Wang, T. L., et al. (2013). Genome-wide reprogramming of the chromatin landscape underlies endocrine therapy resistance in breast cancer. Proc. Natl. Acad. Sci. U. S. A. 110 (16), E1490–E1499. doi:10.1073/pnas.1219992110
Manley, N. R., Selleri, L., Brendolan, A., Gordon, J., and Cleary, M. L. (2004). Abnormalities of caudal pharyngeal pouch development in Pbx1 knockout mice mimic loss of Hox3 paralogs. Dev. Biol. 276 (2), 301–312. doi:10.1016/j.ydbio.2004.08.030
Mary, L., Leclerc, D., Gilot, D., Belaud-Rotureau, M. A., and Jaillard, S. (2022). The TALE never ends: a comprehensive overview of the role of PBX1, a TALE transcription factor, in human developmental defects. Hum. Mutat. 43 (9), 1125–1148. doi:10.1002/humu.24388
Muggeo, S., Crisafulli, L., Uva, P., Fontana, E., Ubezio, M., Morenghi, E., et al. (2021). PBX1-directed stem cell transcriptional program drives tumor progression in myeloproliferative neoplasm. Stem Cell Rep. 16 (11), 2607–2616. doi:10.1016/j.stemcr.2021.09.016
Murphy, M. J., Polok, B. K., Schorderet, D. F., and Cleary, M. L. (2010). Essential role for Pbx1 in corneal morphogenesis. Invest. Ophthalmol. Vis. Sci. 51 (2), 795–803. doi:10.1167/iovs.08-3327
Musgrove, E. A., and Sutherland, R. L. (2009). Biological determinants of endocrine resistance in breast cancer. Nat. Rev. Cancer 9 (9), 631–643. doi:10.1038/nrc2713
Nagel, S., Meyer, C., and Pommerenke, C. (2023). Establishment of the lymphoid ETS-code reveals deregulated ETS genes in Hodgkin lymphoma. PLoS One 18 (7), e0288031. doi:10.1371/journal.pone.0288031
Nagel, S., Pommerenke, C., Meyer, C., MacLeod, R. A. F., and Drexler, H. G. (2021). Establishment of the TALE-code reveals aberrantly activated homeobox gene PBX1 in Hodgkin lymphoma. PLoS One 16 (2), e0246603. doi:10.1371/journal.pone.0246603
Niu, Y., Sengupta, M., Titov, A. A., Choi, S. C., and Morel, L. (2017). The PBX1 lupus susceptibility gene regulates CD44 expression. Mol. Immunol. 85, 148–154. doi:10.1016/j.molimm.2017.02.016
Okada, Y., Nagai, R., Sato, T., Matsuura, E., Minami, T., Morita, I., et al. (2003). Homeodomain proteins MEIS1 and PBXs regulate the lineage-specific transcription of the platelet factor 4 gene. Blood 101 (12), 4748–4756. doi:10.1182/blood-2002-02-0380
Okura, E., Saito, S., Natsume, T., Morita, D., Tanaka, M., Kiyokawa, N., et al. (2020). Multiple heterogeneous bone invasions of B lymphoblastic lymphoma with the TCF3/PBX1 fusion gene: a case report. Int. J. Hematol. 111 (2), 163–165. doi:10.1007/s12185-019-02794-9
Olatoke, T., Wagner, A., Astrinidis, A., Zhang, E. Y., Guo, M., Zhang, A. G., et al. (2023). Single-cell multiomic analysis identifies a HOX-PBX gene network regulating the survival of lymphangioleiomyomatosis cells. Sci. Adv. 9 (19), eadf8549. doi:10.1126/sciadv.adf8549
Oshima, M., Endoh, M., Endo, T. A., Toyoda, T., Nakajima-Takagi, Y., Sugiyama, F., et al. (2011). Genome-wide analysis of target genes regulated by HoxB4 in hematopoietic stem and progenitor cells developing from embryonic stem cells. Blood 117 (15), e142–e150. doi:10.1182/blood-2010-12-323212
Park, J. T., Shih, I. M., and Wang, T. L. (2008). Identification of Pbx1, a potential oncogene, as a Notch3 target gene in ovarian cancer. Cancer Res. 68 (21), 8852–8860. doi:10.1158/0008-5472.CAN-08-0517
Park, Y. P., Roach, T., Soh, S., Zeumer-Spataro, L., Choi, S. C., Ostrov, D. A., et al. (2023). Molecular mechanisms of lupus susceptibility allele PBX1D. J. Immunol. 211 (5), 727–734. doi:10.4049/jimmunol.2300362
Petrini, I., Wang, Y., Zucali, P. A., Lee, H. S., Pham, T., Voeller, D., et al. (2013). Copy number aberrations of genes regulating normal thymus development in thymic epithelial tumors. Clin. Cancer Res. 19 (8), 1960–1971. doi:10.1158/1078-0432.CCR-12-3260
Pi, W. C., Wang, J., Shimada, M., Lin, J. W., Geng, H., Lee, Y. L., et al. (2020). E2A-PBX1 functions as a coactivator for RUNX1 in acute lymphoblastic leukemia. Blood 136 (1), 11–23. doi:10.1182/blood.2019003312
Pillay, L. M., Forrester, A. M., Erickson, T., Berman, J. N., and Waskiewicz, A. J. (2010). The Hox cofactors Meis1 and Pbx act upstream of gata1 to regulate primitive hematopoiesis. Dev. Biol. 340 (2), 306–317. doi:10.1016/j.ydbio.2010.01.033
Platais, C., Radhakrishnan, R., Niklander Ebensperger, S., Morgan, R., Lambert, D. W., and Hunter, K. D. (2018). Targeting HOX-PBX interactions causes death in oral potentially malignant and squamous carcinoma cells but not normal oral keratinocytes. BMC Cancer 18 (1), 723. doi:10.1186/s12885-018-4622-0
Remesal, L., Roger-Baynat, I., Chirivella, L., Maicas, M., Brocal-Ruiz, R., Pérez-Villalba, A., et al. (2020). PBX1 acts as terminal selector for olfactory bulb dopaminergic neurons. Development 147 (8), dev186841. doi:10.1242/dev.186841
Russell, M. K., Longoni, M., Wells, J., Maalouf, F. I., Tracy, A. A., Loscertales, M., et al. (2012). Congenital diaphragmatic hernia candidate genes derived from embryonic transcriptomes. Proc. Natl. Acad. Sci. U. S. A. 109 (8), 2978–2983. doi:10.1073/pnas.1121621109
Safgren, S. L., Olson, R. J., Pinto E Vairo, F., Bothun, E. D., Hanna, C., Klee, E. W., et al. (2022). De novo PBX1 variant in a patient with glaucoma, kidney anomalies, and developmental delay: an expansion of the CAKUTHED phenotype. Am. J. Med. Genet. A 188 (3), 919–925. doi:10.1002/ajmg.a.62576
Sagerström, C. G. (2004). PbX marks the spot. Dev. Cell 6 (6), 737–738. doi:10.1016/j.devcel.2004.05.015
Sanyal, M., Tung, J. W., Karsunky, H., Zeng, H., Selleri, L., Weissman, I. L., et al. (2007). B-cell development fails in the absence of the Pbx1 proto-oncogene. Blood 109 (10), 4191–4199. doi:10.1182/blood-2006-10-054213
Schnabel, C. A., Godin, R. E., and Cleary, M. L. (2003b). Pbx1 regulates nephrogenesis and ureteric branching in the developing kidney. Dev. Biol. 254 (2), 262–276. doi:10.1016/s0012-1606(02)00038-6
Schnabel, C. A., Selleri, L., and Cleary, M. L. (2003a). Pbx1 is essential for adrenal development and urogenital differentiation. Genesis 37 (3), 123–130. doi:10.1002/gene.10235
Selleri, L., Depew, M. J., Jacobs, Y., Chanda, S. K., Tsang, K. Y., Cheah, K. S., et al. (2001). Requirement for Pbx1 in skeletal patterning and programming chondrocyte proliferation and differentiation. Development 128 (18), 3543–3557. doi:10.1242/dev.128.18.3543
Selleri, L., Zappavigna, V., and Ferretti, E. (2019). Building a perfect body': control of vertebrate organogenesis by PBX-dependent regulatory networks. Genes Dev. 33 (5-6), 258–275. doi:10.1101/gad.318774.118
Sgadò, P., Ferretti, E., Grbec, D., Bozzi, Y., and Simon, H. H. (2012). The atypical homeoprotein Pbx1a participates in the axonal pathfinding of mesencephalic dopaminergic neurons. Neural Dev. 7, 24. doi:10.1186/1749-8104-7-24
Shen, Y. A., Jung, J., Shimberg, G. D., Hsu, F. C., Rahmanto, Y. S., Gaillard, S. L., et al. (2021). Development of small molecule inhibitors targeting PBX1 transcription signaling as a novel cancer therapeutic strategy. iScience 24 (11), 103297. doi:10.1016/j.isci.2021.103297
Shepherd, M. S., Li, J., Wilson, N. K., Oedekoven, C. A., Belmonte, M., Fink, J., et al. (2018). Single-cell approaches identify the molecular network driving malignant hematopoietic stem cell self-renewal. Blood 132 (8), 791–803. doi:10.1182/blood-2017-12-821066
Slavotinek, A., Risolino, M., Losa, M., Cho, M. T., Monaghan, K. G., Schneidman-Duhovny, D., et al. (2017). De novo, deleterious sequence variants that alter the transcriptional activity of the homeoprotein PBX1 are associated with intellectual disability and pleiotropic developmental defects. Hum. Mol. Genet. 26 (24), 4849–4860. doi:10.1093/hmg/ddx363
Sun, Y., Lin, P., Zhou, X., Ren, Y., He, Y., Liang, J., et al. (2023). TRIM26 promotes non-small cell lung cancer survival by inducing PBX1 degradation. Int. J. Biol. Sci. 19 (9), 2803–2816. doi:10.7150/ijbs.81726
Suurmeijer, A. J. H., Dickson, B. C., Swanson, D., Zhang, L., Sung, Y. S., Fletcher, C. D., et al. (2020). A morphologic and molecular reappraisal of myoepithelial tumors of soft tissue, bone, and viscera with EWSR1 and FUS gene rearrangements. Genes Chromosom. Cancer 59 (6), 348–356. doi:10.1002/gcc.22835
Takubo, K., Goda, N., Yamada, W., Iriuchishima, H., Ikeda, E., Kubota, Y., et al. (2010). Regulation of the HIF-1alpha level is essential for hematopoietic stem cells. Cell Stem Cell 7 (3), 391–402. doi:10.1016/j.stem.2010.06.020
Trasanidis, N., Katsarou, A., Ponnusamy, K., Shen, Y. A., Kostopoulos, I. V., Bergonia, B., et al. (2022). Systems medicine dissection of chr1q-amp reveals a novel PBX1-FOXM1 axis for targeted therapy in multiple myeloma. Blood 139 (13), 1939–1953. doi:10.1182/blood.2021014391
Tyson, J. J., Baumann, W. T., Chen, C., Verdugo, A., Tavassoly, I., Wang, Y., et al. (2011). Dynamic modelling of oestrogen signalling and cell fate in breast cancer cells. Nat. Rev. Cancer 11 (7), 523–532. doi:10.1038/nrc3081
van Vuurden, D. G., Aronica, E., Hulleman, E., Wedekind, L. E., Biesmans, D., Malekzadeh, A., et al. (2014). Pre-B-cell leukemia homeobox interacting protein 1 is overexpressed in astrocytoma and promotes tumor cell growth and migration. Neuro Oncol. 16 (7), 946–959. doi:10.1093/neuonc/not308
Veselska, R., Jezova, M., Kyr, M., Mazanek, P., Chlapek, P., Dobrotkova, V., et al. (2019). Comparative analysis of putative prognostic and predictive markers in neuroblastomas: high expression of PBX1 is associated with a poor response to induction therapy. Front. Oncol. 9, 1221. doi:10.3389/fonc.2019.01221
Villaescusa, J. C., Li, B., Toledo, E. M., Rivetti di Val Cervo, P., Yang, S., Stott, S. R., et al. (2016). A PBX1 transcriptional network controls dopaminergic neuron development and is impaired in Parkinson's disease. EMBO J. 35 (18), 1963–1978. doi:10.15252/embj.201593725
Vitobello, A., Ferretti, E., Lampe, X., Vilain, N., Ducret, S., Ori, M., et al. (2011). Hox and Pbx factors control retinoic acid synthesis during hindbrain segmentation. Dev. Cell 20 (4), 469–482. doi:10.1016/j.devcel.2011.03.011
Wang, J., Shidfar, A., Ivancic, D., Ranjan, M., Liu, L., Choi, M. R., et al. (2017). Overexpression of lipid metabolism genes and PBX1 in the contralateral breasts of women with estrogen receptor-negative breast cancer. Int. J. Cancer 140 (11), 2484–2497. doi:10.1002/ijc.30680
Wang, Y., Xie, X., Zhang, C., Su, M., Gao, S., Wang, J., et al. (2022). Rheumatoid arthritis, systemic lupus erythematosus and primary Sjögren's syndrome shared megakaryocyte expansion in peripheral blood. Ann. Rheum. Dis. 81 (3), 379–385. doi:10.1136/annrheumdis-2021-220066
Wei, X., Yu, L., and Li, Y. (2018). PBX1 promotes the cell proliferation via JAK2/STAT3 signaling in clear cell renal carcinoma. Biochem. Biophys. Res. Commun. 500 (3), 650–657. doi:10.1016/j.bbrc.2018.04.127
Wilson, N. K., Kent, D. G., Buettner, F., Shehata, M., Macaulay, I. C., Calero-Nieto, F. J., et al. (2015). Combined single-cell functional and gene expression analysis resolves heterogeneity within stem cell populations. Cell Stem Cell 16 (6), 712–724. doi:10.1016/j.stem.2015.04.004
Xu, X., Fan, Z., Kang, L., Han, J., Jiang, C., Zheng, X., et al. (2013). Hepatitis B virus X protein represses miRNA-148a to enhance tumorigenesis. J. Clin. Invest. 123 (2), 630–645. doi:10.1172/JCI64265
Xu, X., Zhou, Y., Fu, B., Zhang, J., Dong, Z., Zhang, X., et al. (2020). PBX1 promotes development of natural killer cells by binding directly to the Nfil3 promoter. FASEB J. 34 (5), 6479–6492. doi:10.1096/fj.202000121R
Yin, H., Wang, J., Tan, Y., Jiang, M., Zhang, H., and Meng, G. (2023). Transcription factor abnormalities in B-ALL leukemogenesis and treatment. Trends Cancer 9 (10), 855–870. doi:10.1016/j.trecan.2023.06.004
Zhang, F. L., Shen, G. M., Liu, X. L., Wang, F., Zhao, Y. Z., and Zhang, J. W. (2012). Hypoxia-inducible factor 1-mediated human GATA1 induction promotes erythroid differentiation under hypoxic conditions. J. Cell Mol. Med. 16 (8), 1889–1899. doi:10.1111/j.1582-4934.2011.01484.x
Zhao, Y., Che, J., Tian, A., Zhang, G., Xu, Y., Li, S., et al. (2022). PBX1 participates in estrogen-mediated bladder cancer progression and chemo-resistance affecting estrogen receptors. Curr. Cancer Drug Targets 22 (9), 757–770. doi:10.2174/1568009622666220413084456
Zhou, Y., Fu, B., Xu, X., Zhang, J., Tong, X., Wang, Y., et al. (2020). PBX1 expression in uterine natural killer cells drives fetal growth. Sci. Transl. Med. 12 (537), eaax1798. doi:10.1126/scitranslmed.aax1798
Glossary
Keywords: PBX1, TALE, development, cancer, t(1;19), Myeloproliferative Neoplasm (MPN), Hematopoietic stem cells (HSC)
Citation: Crisafulli L, Brindisi M, Liturri MG, Sobacchi C and Ficara F (2024) PBX1: a TALE of two seasons—key roles during development and in cancer. Front. Cell Dev. Biol. 12:1372873. doi: 10.3389/fcell.2024.1372873
Received: 18 January 2024; Accepted: 29 January 2024;
Published: 09 February 2024.
Edited by:
Grazia Fazio, University of Milano-Bicocca, ItalyReviewed by:
Babhrubahan Roy, University of Michigan, United StatesCopyright © 2024 Crisafulli, Brindisi, Liturri, Sobacchi and Ficara. This is an open-access article distributed under the terms of the Creative Commons Attribution License (CC BY). The use, distribution or reproduction in other forums is permitted, provided the original author(s) and the copyright owner(s) are credited and that the original publication in this journal is cited, in accordance with accepted academic practice. No use, distribution or reproduction is permitted which does not comply with these terms.
*Correspondence: Francesca Ficara, francesca.ficara@humanitasresearch.it
†These authors have contributed equally to this work