- 1Department of Life Sciences and Systems Biology, University of Turin, Turin, Italy
- 2IIGM-Italian Institute for Genomic Medicine, IRCCS, Candiolo, TO, Italy
- 3Candiolo Cancer Institute, FPO-IRCCS, Candiolo Cancer (IT), Torino, Italy
- 4Department of Medical Biotechnology, Fasa University of Medical Sciences, Fasa, Iran
- 5Department of Medical Genetics, Fasa University of Medical Sciences, Fasa, Iran
- 6Noncommunicable Diseases Research Center, Fasa University of Medical Sciences, Fasa, Iran
- 7Pharmaceutical Sciences Research Center, Ardabil University of Medical Sciences, Ardabil, Iran
Patients with breast cancer show altered expression of genes within the pectoralis major skeletal muscle cells of the breast. Through analyses of The Cancer Genome Atlas (TCGA)-breast cancer (BRCA), we identified three previously uncharacterized putative novel tumor suppressor genes expressed in normal muscle cells, whose expression was downregulated in breast tumors. We found that NEDD4 binding protein 2-like 1 (N4BP2L1), pleckstrin homology domain-containing family A member 4 (PLEKHA4), and brain-enriched guanylate kinase-associated protein (BEGAIN) that are normally highly expressed in breast myoepithelial cells and smooth muscle cells were significantly downregulated in breast tumor tissues of a cohort of 50 patients with this cancer. Our data revealed that the low expression of PLEKHA4 in patients with menopause below 50 years correlated with a higher risk of breast cancer. Moreover, we identified N4BP2L1 and BEGAIN as potential biomarkers of HER2-positive breast cancer. Furthermore, low BEGAIN expression in breast cancer patients with blood fat, heart problems, and diabetes correlated with a higher risk of this cancer. In addition, protein and RNA expression analysis of TCGA-BRCA revealed N4BP2L1 as a promising diagnostic protein biomarker in breast cancer. In addition, the in silico data of scRNA-seq showed high expression of these genes in several cell types of normal breast tissue, including breast myoepithelial cells and smooth muscle cells. Thus, our results suggest their possible tumor-suppressive function in breast cancer and muscle development.
1 Introduction
Breast cancer is among the most common cancers and a leading cause of cancer-related deaths among women in the world (IARC, 2022; “Statistics on preventable cancers” [Internet]; Cancer Research, 2015 [cited 2020 June 15]; Zemni et al., 2022). Currently, we are experiencing a rapid increase in new cases (Shiovitz and Korde, 2015; Giaquinto et al., 2022; Canadian Cancer Statistics, 2023; Kratzer et al., 2023). Its incidence has been continuously growing despite screening for some genes reported to be correlated with the progression of the disease, including BRCA1 and 2 DNA repair associated (BRCA1 and BRCA2), phosphatase and tensin homolog (PTEN), ATM serine/threonine kinase (ATM), partner and localizer of BRCA2 (PALB2), and checkpoint kinase 2 (CHEK2) (Shiovitz and Korde, 2015). Considerably, many patients diagnosed with breast cancer experience metastasis and recurrence (Luo et al., 2018; Giaquinto et al., 2022) even after successful surgical resections and therapies (Siegel et al., 2017). Although several potential biomarkers involved in breast cancer development and metastasis have been reported, it is essential to deeply identify the pathways and processes underlying the progression of breast cancer and new prognostic and diagnostic RNA and protein biomarkers correlated with this cancer.
The pectoralis, a type of skeletal muscle, attaches the front of the chest wall to the upper extremities. On each side of the breastbone, two such muscles are present, namely, the pectoralis major and pectoralis minor (Sanchez et al., 2014). Understanding the tumor involvement of the pectoralis muscle in breast cancer before the surgical operation may change the management of surgery (Harris et al., 1992; Lagios, 1992; DiBiase et al., 1998; Obedian and Haffty, 2000; Park et al., 2000; Lee et al., 2014).
The role of mammary myoepithelial cells in breast tumors remains largely understood. Nonetheless, recent studies have suggested that these cells may regulate the transition from non-invasive to invasive cancer and the activity of stem cells. It has been reported that patients with pectoralis muscle invasion have a worse prognosis (Orel et al., 1994; Kerslake et al., 1995; Kazama et al., 2005; Sanchez et al., 2014; Mango et al., 2015; Mango et al., 2015; Bohlen et al., 2018; Pasquero et al., 2020; Myers et al., 2021). A study conducted by the E. Pistilli group focused on individuals with early-stage non-metastatic breast tumors. They found that there were changes in the expression of genes associated with muscle protein homeostasis in the pectoralis muscle of these patients (Bohlen et al., 2018). RNA sequencing (RNA-seq) on biopsies of skeletal muscle obtained from breast tumors compared with biopsies from individuals without cancer demonstrated a significant decrease in the levels of gene expression inside the breast tumor tissues in comparison to the non-tumor samples. They observed compromised canonical pathways essential for oxidative phosphorylation and defective mitochondrial function in the pectoralis muscle of their breast tumor patients. Thus, this suggested that skeletal muscle exhibited a comprehensive response to the growth of breast tumors. Tumor suppressors are persistently expressed in all or almost all myoepithelial cells in the normal ductal system (Man and Sang, 2004; Li and Man, 2009; Xu et al., 2009). In certain instances, cytoplasmic expression of TP63 in the corresponding epithelial cells, representing more aggressiveness and invasiveness, was connected with the absence of TP63 expression in myoepithelial cells (Zou et al., 1994; Barbareschi et al., 2001; Man et al., 2005; Man and Nieburgs, 2006; Xu et al., 2009; Hsiao et al., 2010; Man, 2010).
The goal of the current study is to investigate differential mRNA expression in patients with breast invasive carcinoma (BRCA) extracted from The Cancer Genome Atlas (TCGA). This involves univariate survival analysis, selection of uncharacterized genes showing downregulation and significant association with overall survival (OS) in BRCA patients as potential tumor suppressor genes, validation using quantitative reverse transcriptase polymerase chain reaction (RT-qPCR), in silico analysis of their gene set enrichment analysis (GSEA) using Enrichr [11], single-cell RNA sequencing (scRNA-seq) from the breast tissue and muscle cells during developmental stages, assessment of total RNA and protein expression in normal and breast cancer tissues, and evaluation of differential expression in various patients’ statuses of TCGA-BRCA.
Through database analyses of breast tumors, we identified new putative tumor-suppressive genes, including NEDD4 binding protein 2-like 1 (N4BP2L1), pleckstrin homology domain-containing family A member 4 (PLEKHA4), and brain-enriched guanylate kinase-associated protein (BEGAIN), and validated their decreased expression by RT-qPCR in our cohort of 50 patients with breast tumors and the expression in the muscle cells by RT-qPCR on muscle cells differentiated from H9 human embryonic stem cells (hESCs).
2 Materials and methods
In our study, we considered experimental methods and bioinformatic analyses, as described in Figure 1. In each of the following sections, we explain them in detail.
2.1 Gene expression analysis in TCGA-BRCA
2.1.1 Collection of TCGA-BRCA data and its differential mRNA expression levels using GDCRNATools
We used the R/Bioconductor package GDCRNATools (Li et al., 2018) to investigate, first, the differential gene expression of patients with BRCA from TCGA. The gene expression data from tumors and their matched adjacent non-tumor tissue were analyzed as described previously (Li et al., 2018). The following functions were applied to the TCGA-BRCA dataset: gdcParseMetadata, gdcFilterDuplicate, gdcFilterSampleType, gdcVoomNormalization, gdcDEAnalysis, and gdcDEReport functions, as reported in a previous study (Dastsooz et al., 2021). In our analysis, we considered 1,222 RNA sequencing data and 1,097 clinical data from the TCGA-BRCA dataset. In the next step, the univariate survival analysis was carried out by applying the gdcSurvivalAnalysis function from the same package (Kaplan–Meier division of patients into high- and low-expression groups). Among the differentially expressed genes, we looked for those with significant OS, and finally, we selected those downregulated genes (as possible tumor suppressor genes) that have not been reported or poorly understood in BRCA patients for further analyses.
2.1.2 Expression analysis of the selected TCGA-BRCA genes across the different patients’ statuses and other TCGA cancers using TACCO and UALCAN web resources
To strengthen the possible role of three uncharacterized tumor-suppressive genes in breast cancer, we used different databases for extracting TCGA data, including the TACCO web server (http://tacco.life.nctu.edu.tw) (Chou et al., 2019) for the investigation of gene expression profiling across all TCGA cancers and UALCAN (https://ualcan.path.uab.edu/index.html) (Chandrashekar et al., 2017; Chandrashekar et al., 2022) for the analysis of gene expression in RNA and protein levels in different statuses of patients with breast tumor, including tumor stages, subclasses, histologic subtypes, and nodal metastasis. UALCAN used data from the Clinical Proteomic Tumor Analysis Consortium (CPTAC, https://proteomics.cancer.gov/programs/cptac) (Edwards et al., 2015) and International Cancer Proteogenome Consortium (ICPC, https://icpc.cancer.gov/portal/) datasets.
2.2 Gene ontology analysis of N4BP2L1, PLEKHA4, and BEGAIN using Enrichr
We used the Enrichr web-based tool (Kuleshov et al., 2016) to search the “biological process” sub-ontology of Gene Ontology (GO: BP) for N4BP2L1, PLEKHA4, and BEGAIN. This tool helped analyze the link between proteins (e.g., their interactions or co-regulation associations), assuming that proteins with the same annotations (or relations) of GO: BP terms have interactions with each other or are functionally connected.
2.3 N4BP2L1, PLEKHA4, and BEGAIN mRNA expression in a cohort of Iranian patients with breast cancer
2.3.1 Breast cancer samples, RNA isolation, and cDNA synthesis assay
In the current study, 50 primary tumors and their paired normal tissues adjacent to the tumor were collected from Iranian patients with breast cancer from Shahid Faghihi Hospital (Shiraz, Fars, Iran). The patients enrolled in our study did not have chemotherapy or radiotherapy before the surgical operation. Fresh tissue samples were snap-frozen in liquid nitrogen and stored at −80°C until future processing. It should be noted that all tumors were diagnosed and validated by histopathological examination. We collected written informed consent from all patients before the start of our studies, and the Research Ethics Committee of the Fasa University of Medical Sciences (ethical code: IR FUMS. REC.1397.143) provided ethical approval for the study, thereby granting the necessary authorization for the research to be conducted adhering to established ethical guidelines and principles. The clinical and demographic data from the patients were collected using a standard questionnaire, as reported in our previous study (Mansoori et al., 2021). As shown in Supplementary Table S1, different statuses (the study variables) of our cohort were as follows: age, tumor size, estrogen receptor, progesterone receptor, HER-2 receptor, nuclear grade, histologic grade, lymph nodes metastasis, histologic type of invasive carcinoma, marital status, cut point of BMI, age of menarche, regular or irregular menstruation, age of menopause, menopausal status, history of uterine surgery, number of pregnancies, age at FFTP, breastfeeding duration, number of abortions, abortion history, family history of cancer, disease history, chest radiograph history, hormone therapy history, duration of OCP consumption, OCP consumption, vitamin D consumption, regular sleep, exercising, deodorant use, hair dye use, and cosmetics use. As shown in Supplementary Table S1, for each variable, the patients were divided into different groups, and then we investigated the correlation between each variable and the expression of the selected genes in our cohort. The experiment was carried out with the comprehension and consent of the human participants, along with a declaration that the ethical committee in charge had approved the experiments. The administration of anesthetics or surgical procedures and the provision of evidence demonstrating the implementation of all feasible measures to prevent animal distress at every stage of the experiment were ensured.
Total RNA was prepared from 50 primary tumors and their paired normal tissues adjacent to the tumor of the Iranian patients with breast cancer using TRIzol (Invitrogen, Thermo Fisher Scientific) based on the manufacturer’s recommendations. We measured their concentration using a spectrophotometer and determined their quality by agarose gel electrophoresis. Then, we performed complementary DNA (cDNA) synthesis using a Thermo Fisher Scientific ™ First Strand cDNA Synthesis Kit (Fermentas, Cat. No: K1622). All clinical data on our patients are provided in Supplementary Table S1. The RNA and clinical data from these samples have also been used in our previous study (Mansoori et al., 2021).
2.3.2 RT-qPCR analysis
The real-time PCR reaction was prepared for the test, internal control samples, and negative control in duplicate using RealQ Plus 2x Master Mix Green with
2.3.3 Statistical analysis of experiments related to breast cancer samples
The mean and standard deviation were used for presenting ΔΔCt, and the median was used for fold change. ΔΔCt ‘s comparison between the tumors and adjacent non-tumor tissues was performed using the paired sample t-test, and the comparison of log fold changes between these samples was performed using the Mann–Whitney test. The association between the expression of these genes and clinicopathologic and demographic factors was analyzed using the Mann–Whitney and Kruskal–Wallis tests. According to the median, log fold changes were divided into two groups of high and low expressions, and the chi-square test and independent t-test were used to compare these groups. IBM SPSS 26 statistical software was applied for data analyses. The degree of a p-value less than 0.05 was defined as statistically significant.
2.4 Analysis of N4BP2L1, PLEKHA4, and BEGAIN involvement in breast tissue and muscle cells
2.4.1 Expression analysis of these genes in normal public bulk RNA-seq, single-cell RNA-seq, and protein databases
We retrieved the expression of N4BP2L1, PLEKHA4, and BEGAIN at bulk and single-cell RNA-seq levels from the GTEx database (Uhlen et al., 2005; Consortium, 2013; Carithers and Moore, 2015; Uhlen et al., 2015; Karlsson et al., 2021) using the Human Protein Atlas (https://www.proteinatlas.org). The protein expression levels were also available as immunohistochemistry of breast tissues in the Human Protein Atlas (Uhlen et al., 2005).
2.4.2 PLEKHA4, N4BP2L1, and BEGAIN expression across developmental stages using the Human Skeletal Muscle Atlas
In this analysis, we used the data from the Human Skeletal Muscle Atlas (https://aprilpylelab.com/datasets/) (https://doi.org/10.1016/j.stem.2020.04.017) to investigate the expression of these genes in different in vivo muscle developmental stages. The data contain single-cell RNA sequencing of human skeletal muscle tissues from the embryonic, fetal, and postnatal stages (Xi et al., 2020).
2.4.3 PLEKHA4, N4BP2L1, and BEGAIN expression in mammary myoepithelial cells compared to luminal epithelial cells using GREIN tools
In another part of our study, we investigated the expression levels of N4BP2L1, PLEKHA4, and BEGAIN in myoepithelial (MEP) cells compared with luminal epithelial (LEP) cells isolated from primary breast organoids and also the expression pattern of recognized tumor suppressor genes, including TP63 and SERPINB5, among others. Since organoids are simple tissue-engineered cell-based in vitro models that recapitulate many aspects of the complex structure and function of the corresponding in vivo tissue, we analyzed this model. The GSE182338 data were analyzed using online GREIN tools (GEO RNA-seq Experiments Interactive Navigator, http://www.ilincs.org/apps/grein/?gse=GSE182338) (Mahi et al., 2019). This web-based tool offers alternatives for exploring and analyzing GEO RNA-seq data that are easy to use. It is supported by several existing processed datasets and the back-end computational pipeline to consistently process the RNA-seq data. GSE182338 data contain transcriptional profiling of human mammary LEP and MEP cells from different samples, including the primary breast organoids. We analyzed differential expression in organoids prepared from samples with an age/risk status considered normal risk for individuals <30 years (Sayaman, 2021).
2.4.4 Expression analysis of the selected genes in muscle cells
In our study, we used a protocol described by Shelton et al. (2016) to differentiate H9 hESCs into skeletal muscle cells. H9 hESCs were grown and maintained in TeSR™-E8™ (STEMCELL Technologies) in 6-well plates coated with 0.5% Corning Matrigel® Growth Factor Reduced (GFR) Basement Membrane Matrix, Phenol Red-Free (Cat. No: 356231), prepared in PBS 1X, or advanced DMEM/F12 (Gibco). The cells were grown in an incubator at 37°C with 5% CO2 and 5% O2. The ES media was changed daily, and when the cells reached 70%–80% confluency with healthy pluripotent colonies, they were dissociated into single cells to form cell clusters, with 6–15 cells in each cluster on the day of differentiation.
The H9 hESCs were differentiated in a 12-well plate coated with 3% Corning Matrigel with the protocol described by Shelton, M et al. Our differentiation stages were performed for 46 days (D46, terminal stage), and we collected cells for RNA extraction and RT-qPCR at different muscle stages (D0: undifferentiated, D2: mesoderm stage, D7: somite stage, D30: skeletal muscle progenitors’ (SMPs’) stage, and D46: terminally differentiated stage). RT-qPCR for the T (Brachyury) gene (TBXT) on day 2, paired box 3 (PAX3) on day 7, and myogenin (MYOG) on day 30 (the PUM1 gene was considered an internal reference gene, Table 1) and immunofluorescent (IF) staining for MF20 (myosin heavy chain protein) on D46 were performed to confirm the differentiation process and presence of muscle cells. Regarding the MF20 IF assay, the first antibody was mouse DSHB (Developmental Studies Hybridoma Bank) AB_2147781, and the secondary antibody was anti-mouse Alexa Fluor 488, goat Invitrogen A11001. RNA extraction was carried out by QIAzol reagent (QIAGEN), and the RNA quality was determined using the Agilent Bioanalyzer 2100 (RNA integrity number ranging from 8 to 10). We then investigated the relative expression levels of PLEKHA4, N4BP2L1, and BEGAIN by RT-qPCR in the muscle cells compared to hESCs.
2.5 Disease association analysis of PLEKHA4, N4BP2L1, and BEGAIN using the CTD dataset
Moreover, we used the CTD dataset (Davis et al., 2023) (https://ctdbase.org) for finding any possible association between these genes and diseases. This dataset contains curated information related to interactions between chemical compounds and the gene/protein, associations of chemicals with diseases, and genes with diseases, combined with functional and pathway data. CTD includes curated and inferred chemical–disease associations. The curated one is retrieved from the publications, while the inferred relationships are created through curated interactions between chemical compounds and genes (for example, chemical compound H has a relationship with cancer I since chemical compound H shows a curated interaction with gene L, and gene L has a curated relationship with cancer I) (Barabasi and Albert, 1999; Li and Liang, 2009; King et al., 2012).
3 Results
3.1 Differential gene expression analysis of TCGA-BRCA samples
We performed a differential gene expression analysis of normal versus tumor samples collected in TCGA-BRCA through the GDCRNATools package, resulting in several differentially expressed genes (Supplementary Table S2). We found that the expression of some of them was correlated with patient prognosis (Supplementary Table S3). Our analysis revealed some downregulated genes, including N4BP2L1, BEGAIN, and PLEKHA4, and some upregulated genes, such as SLC35A2, DONSON, BRI3BP, NDUFAF6, C2CD4D, and SEZ6L2, which have not been reported or poorly understood in breast cancer (Table 2). Considering the expression data and patient OS, we proceeded by investigating the genes with lower expression and a possible tumor-suppressive role (Table 3).
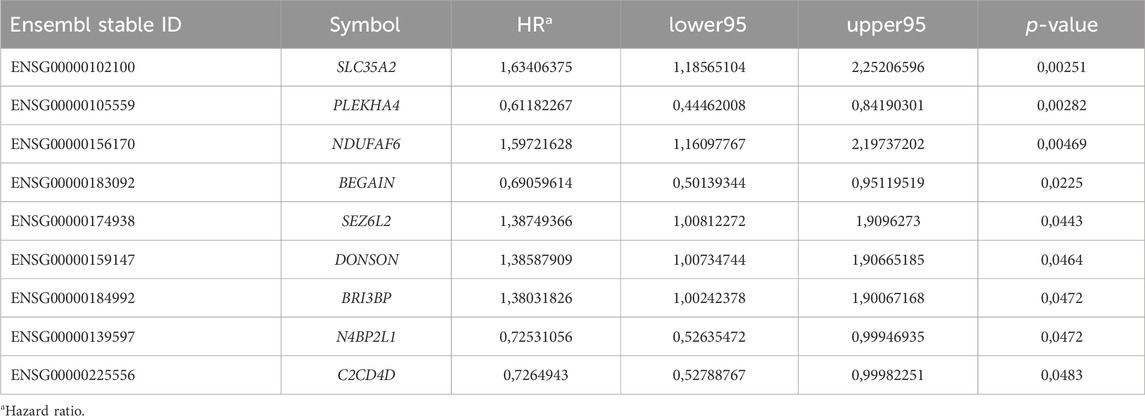
Table 2. Poorly understood proto-oncogenes and tumor suppressor genes annotated in the TCGA-BRCA dataset with significant OS.
3.2 N4BP2L1, PLEKHA4, and BEGAIN expression across all TCGA cancers and different statuses of TCGA-BRCA patients
We retrieved TCGA data from all tumors to show the possible involvement of N4BP2L1, PLEKHA4, and BEGAIN not only in breast cancer but also in other cancers. Using different databases to extract TCGA data, we found that these genes were significantly downregulated in several tumors, with the most significant difference in breast cancer (Supplementary Table S4). Using the TACCO web server, it was found that N4BP2L1 showed lower expression in cancers of the breast, lung, liver, uterine, head–neck, stomach, prostate, colon, bladder, kidney (chromophobe), brain (glioblastoma multiforme), pancreas, cervix, and endometrium (Supplementary Table S4). Regarding PLEKHA4, it revealed decreased expression levels in tumors of the breast, liver, lung, kidney (chromophobe), uterine, bladder, and head–neck (Supplementary Table S4). BEGAIN showed downregulation in cancers of the breast, uterine, stomach, prostate, colon, bladder, thyroid, kidney (kidney renal papillary cell carcinoma and chromophobe), and brain (Glioblastoma multiforme) (Supplementary Table S4). These data, representing the downregulation of these genes in several cancers, may indicate their important roles in tumorigenesis. Therefore, we analyzed their expression in different patients’ statuses in TCGA-BRCA.
In the next step, we looked for their expression in different tumor stages of TCGA-BRCA. As shown in Supplementary Figure S1, panel 1, all three genes, N4BP2L1, PLEKHA4, and BEGAIN, had a significantly decreased expression in all tumor stages (1, 2, 3, and 4) compared to their matched normal tissues. Regarding N4BP2L1, the later tumor stage 4 also showed significantly lower expression compared to stage 1 (p-value: 0.0157). Concerning BEGAIN, tumor stage 4 revealed significantly more downregulation compared to stages 1, 2, and 3 (p-values: 0.00066, 0.0037, and 0.0022, respectively). Stage IV (4) tumors are usually considered metastatic forms. Therefore, these results represent their more probable role in tumor metastasis.
We then analyzed their expression in the three main breast cancer subclasses, and these genes had a significant downregulation in all the subclasses compared to normal tissues, including luminal types (N4BP2L1: p-value <1E-12; PLEKHA4: p-value = 1.62E-12; and BEGAIN: p-value = 1.49-E08), HER2-positive (N4BP2L1: p-value = 1.62E-12; PLEKHA4: p-value = 1.62E-12; and BEGAIN: p-value = 4.3-E15), and triple-negative (N4BP2L1: p-value <1E-12; PLEKHA4: p-value = 5.7E-10; and BEGAIN: p-value = 0.0011) (Supplementary Figure S1; panel 2). It is worth noting that their expression in the HER2-positive subclass was lower than in the other two subclasses, followed by the triple-negative and luminal categories. These data may represent the possible involvement of these genes in all three different subclasses of breast cancer, mainly the HER2-positive one.
After that, we evaluated the expression of these genes in all triple-negative subclasses of TCGA-BRCA, and they revealed downregulation in most of these subclasses compared to normal tissues (Supplementary Figure S1; panel 3). Our results found that N4BP2L1 had lower expression in all subclasses, PLEKHA4 had decreased expression in almost all, except for TNBC-basal-like 2 (BL2) and -unspecified (UNS), and BEGAIN had downregulation in TNBC-basal-like 1 (BL1), TNBC-immunomodulatory (IM), and TNBC-luminal androgen receptor (LAR). As seen in Supplementary Figure S1, panel 3, N4BP2L1 and PLEKHA4 genes also had significant downregulation in TNBC-MSL (TNBC mesenchymal stem-like) and mainly TNBC-M (TNBC mesenchymal), which originate from the mesoderm involved in the muscle differentiation process.
Next, we investigated N4BP2L1, PLEKHA4, and BEGAIN expression in TCGA-BRCA based on histologic subtypes (IDC: infiltrating ductal carcinoma, mixed histology, medullary carcinoma, INOS: infiltrating carcinoma NOS, ILC: infiltrating lobular carcinoma, mucinous carcinoma, and metaplastic carcinoma). As seen in Supplementary Figure S1, panel 4, the data showed a significant N4BP2L1 downregulation in all subtypes (regarding INOS, it represented NA, not applicable, for the three genes due to its low sample size); significantly, PLEKHA4 showed lower expression in all subtypes except for metaplastic ones, and significantly decreased expression of BEGAIN was found only in IDC, ILC, and mixed subtypes compared to normal tissues. As shown in this panel, several breast cancer subtypes revealed significant downregulation of these genes compared to normal tissue as well as each other, indicating their possible role in specific subtypes. Based on these findings, these genes may be involved in different histologic breast tumor subtypes, mainly for N4BP2L1 and PLEKHA4, with differential expression in most of them. Since N4BP2L1 also had significantly lower expression in metaplastic breast cancer (p-value = 1.7E-12), it may indicate its involvement in breast cancer metastasis, as mentioned in the earlier sections.
We also analyzed the possible involvement of these genes in the nodal metastasis statuses of TCGA-BRCA (N0: no regional lymph node metastasis, N1: metastases in 1–3 axillary lymph nodes, N2: metastases in 4–9 axillary lymph nodes, and N3: metastases in 10 or more axillary lymph nodes). The TCGA data showed their significant downregulation in all statuses compared to their normal counterparts (Supplementary Figure S1; panel 5). PLEKHA4 and BEGAIN revealed significantly decreased expression not only in N0 compared to the normal but also in other comparisons as follows: PLEKHA4 lower expression in N1 (p-value = 0.038) and N2 (p-value = 0.017) compared to N3, and BEGAIN downregulation in N2 compared to N0 (p-value = 0.0021), N1 (p-value = 0.013), and N3 (p-value = 0.037). Since studies have shown that non-regional lymph node (NRLN) metastases can be considered in the prognosis evaluation and clinical management of cancers (Pond et al., 2014; James et al., 2015), assessing these genes together may be considered a prognostic biomarker set. This is because the downregulation of N4BP2L1 is not changed in N1, N2, and N3 compared to N0 regional lymph node metastasis, while others have alterations. Altogether, these data may represent the possible role of these genes in breast cancer metastasis (Supplementary Figure S1; panel 5).
3.3 GO-associated processes of N4BP2L1, BEGAIN, and PLEKHA4
We interrogated the Enrichr web tool with our uncharacterized tumor suppressor genes and found their involvement in different biological processes. Interestingly, these genes may have a direct or indirect role in myogenesis based on Enrichr GO: BP (Figure 2). In particular, PLEKHA4 is involved in the regulation of the Wnt signaling pathway, which is critical for the induction of mesoderm and muscle lineage. The significant Enrichr GO: BP terms associated with PLEKHA4 included the positive regulation of the Wnt signaling pathway, planar cell polarity pathway (GO:2000096), regulation of the Wnt signaling pathway, planar cell polarity pathway (GO:2000095), positive regulation of the non-canonical Wnt signaling pathway (GO:2000052), positive regulation of the canonical Wnt signaling pathway (GO:0090263), phosphatidylinositol metabolic process (GO:0046488), and phosphatidylinositol biosynthetic process (GO:0006661) (Figure 2). BEGAIN can also be involved in muscle differentiation since it has a function in the regulation of postsynaptic neurotransmitter receptor activity (GO:0098962 and GO:0099601) and the modulation of chemical synaptic transmission (GO:0050804) (Figure 2), with an important role in neuromuscular junctions (NMJs), a cellular synapse between a motor neuron and a skeletal muscle fiber (Vilmont et al., 2016). It also plays a role in the regulation of the nervous system process (GO:0031644) (Figure 2). Regarding N4BP2L1, although Enrichr did not reveal any biological processes for this gene (Figure 2), through literature mining (https://www.genecards.org) (Stelzer et al., 2016), we found that this gene is reported to be involved in inflammatory leiomyosarcoma, which originated from muscle tissues. Therefore, for the first time, we propose its role in the muscle process and also breast cancer.
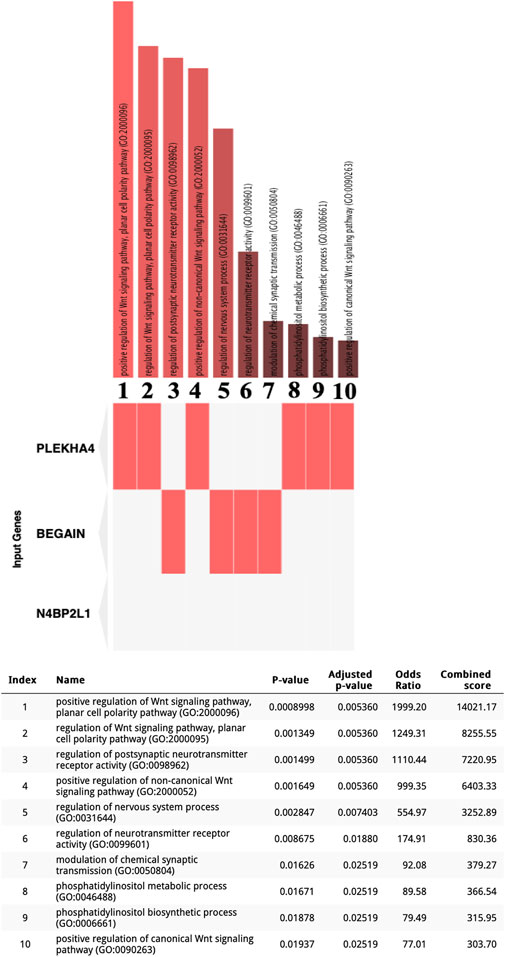
Figure 2. Enrichr GO: BP terms for three uncharacterized tumor suppressor genes in the TCGA-BRCA dataset. Significant GO: BP terms were only found for BEGAIN and PLEKHA4.
3.4 N4BP2L1, BEGAIN, and PLEKHA4 expression in 50 Iranian patients with breast cancer and their correlations with the patients’ statuses
In the next step, we considered the uncharacterized genes with a low OS and a lower expression in TCGA-BRCA tumor samples compared to their normal counterparts in the dataset (Tables 2 and 3). We confirmed the downregulation of N4BP2L1, BEGAIN, and PLEKHA4 in our cohort of 50 patients with breast cancer using RT-qPCR (Figure 3A). As described in Supplementary Table S1, for each variable, our cases were divided into different groups, and then we analyzed their correlation with the mRNA expression of the selected genes.
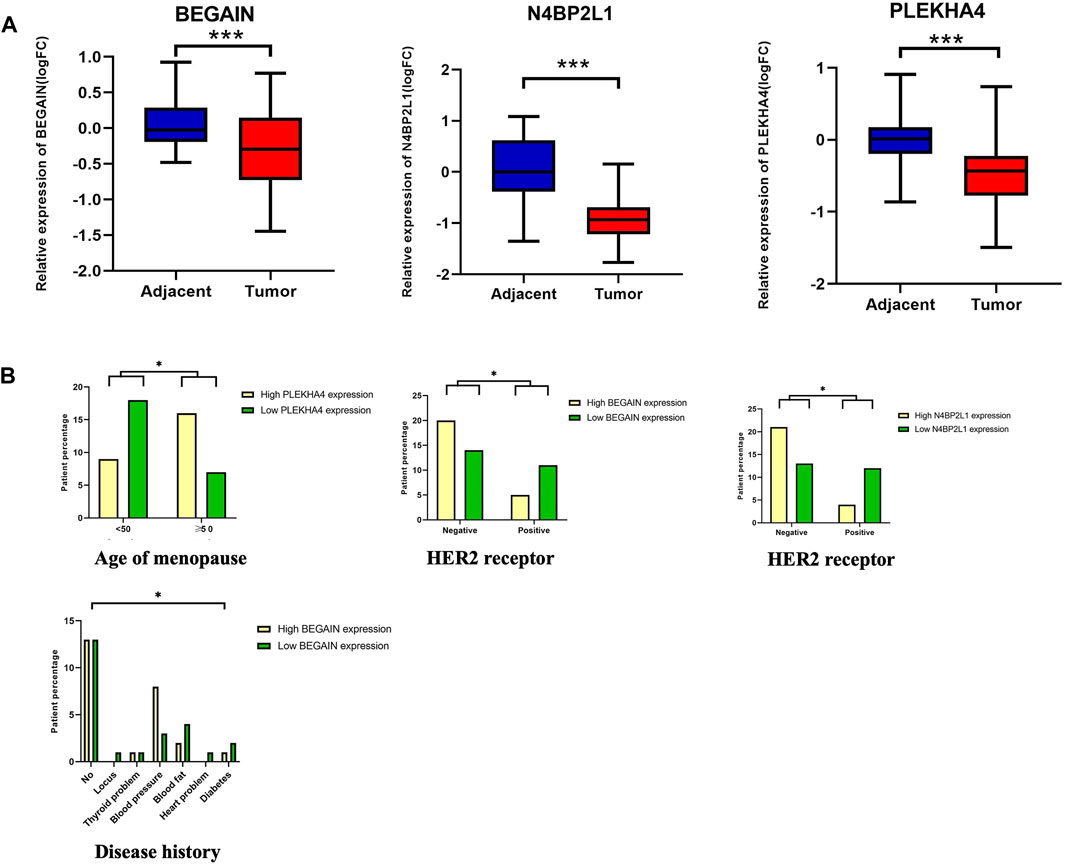
Figure 3. (A) Downregulation of N4BP2L1, BEGAIN, and PLEKHA4 in 50 patients using RT-qPCR. (B) Correlation between PLEKHA4 expression and the percentage of patients with breast cancer at different ages of menopause (Mann–Whitney test), a correlation between N4BP2L1 and BEGAIN expression (Kruskal–Wallis test) and HER2-positive (Mann–Whitney test), and a correlation between BEGAIN expression and disease history of our patients with breast cancer. The box and whisker plot (10–90 percentile) of the relative expression levels of genes (ns, p > 0.05; *,p ≤ 0.05; **,p ≤ 0.01; ***,p ˂0.001) were plotted using GraphPad Prism 9.3.1.
When we compared the relative expression of these genes, grouping the patients according to the age of the onset of menopause, PLEKHA4 revealed a significant difference (Mann–Whitney test, p-value <0.05) (Figure 3B; Supplementary Table S1, Panel A). Our results showed that 69.6% of patients with an age of menopause onset below 50 years or after were in the higher PLEKHA4 expression group, while 30.4% of the patients were in the lower PLEKHA4 expression group. However, for patients with an age of menopause onset before 50 years, 66.7% of them were in the lower PLEKHA4 expression group, and 33.3% of the patients were in the higher PLEKHA4 expression group. Therefore, patients with an age of menopause onset below 50 who had lower expression of PLEKHA4 were at a higher risk of breast cancer, and this gene could be correlated with the age of menopause in patients with breast cancer. Moreover, our study showed that 75% and 69% of breast cancer patients who were HER2-positive had lower N4BP2L1 and BEGAIN expressions, respectively. Therefore, these two genes can be proposed as potential biomarkers of HER2-positive breast cancer (Supplementary Table S1; panels B and C). Furthermore, breast cancer patients with high blood fat, heart problems, diabetes, and locus together with low BEGAIN expression were at a higher risk of this cancer (Figure 3B; Supplementary Table S1; panel C).
3.5 N4BP2L1, PLEKHA4, and BEGAIN protein expression in TCGA-BRCA and other datasets
We also looked for the protein expression of these genes in TCGA breast cancer tissues. As shown in Supplementary Figure S2, panel A, N4BP2L1 showed weak expression in most of the samples (12 out of 18, 66%). However, PLEKHA4 had weak expression only in one sample out of 30 samples (Supplementary Figure S2; panel B), and BEGAIN protein revealed moderate expression in all 20 samples tested (Supplementary Figure S2; panel C). In addition to the protein expression of these genes in TCGA-BRCA, we investigated their expression in the CPTAC using UALCAN. As seen in Supplementary Figure S2, panel D, the N4BP2L1 protein showed highly significant lower expression (p-value: 4.56E-05) in 125 primary breast tumors compared to their matched normal tissues. Moreover, as shown in Supplementary Figure S2, panel E, its protein downregulation was found in breast cancer stages 1, 2, and 3; tumor subclasses of luminal, HER2-positive, and TNBC; histological subtypes of IDC and mixed ones; pathways of HIPPO, WNT, mTOR, RTK, P53/RB, and other ones; SWI-SNF complex status of patients; MYC/MYCN altered condition; and chromatin modifier altered patients’ statuses. Therefore, based on these protein expression data, the N4BP2L1 protein may be considered a promising protein biomarker in breast cancer.
3.6 N4BP2L1, BEGAIN, and PLEKHA4 expression at the normal single-cell RNA level and total RNA and protein expression levels
In the next step, we investigated the bulk and single-cell RNA expression levels of these genes and their protein abundance by interrogating the Human Protein Atlas (https://www.proteinatlas.org) and GTEx (https://gtexportal.org/home/) databases. In particular, single-cell RNA data could help reveal which cell types within breast tissue express these genes, giving important hints for their function elucidation. We selected normal breast tissues from the GTEx database and observed that N4BP2L1 had an RNA expression of 25.7 normalized transcripts per million (nTPM) in GTEx. The Human Protein Atlas database reports a medium protein immunostaining score in myoepithelial and glandular cells (Supplementary Figure S2; panel F). PLEKHA4 RNA expression was 31.7 nTPM in GTEx, and its immunostaining score is high in myoepithelial and medium in glandular cells (Supplementary Figure S2; panel G). BEGAIN RNA expression showed 1.7 nTPM in GTEx, and its protein expression analysis is pending and has not been performed yet. These data revealed that these genes are expressed in the breast tissue in bulk RNA and by protein immunohistochemistry.
Single-cell RNA sequencing extracted from the Human Protein Atlas revealed the different expression patterns of these genes in different cell types grouped into 25 clusters of breast tissues as follows (Figure 4): regarding N4BP2L1, its higher expression was observed in clusters 16, C-16 (nTPM: 75.2, breast glandular cells), C-2 (nTPM: 69.5, T-cells), and C-0 (nTPM: 50.3, T-cells) (Figure 4A). Moreover, it had expression in breast myoepithelial cells C-20 (nTPM: 23) and smooth muscle cells C-14 (nTPM: 33.2), revealing its involvement in muscle cells. In most of the cell clusters, it showed a notable expression that can represent its role in these cells in breast tissue.
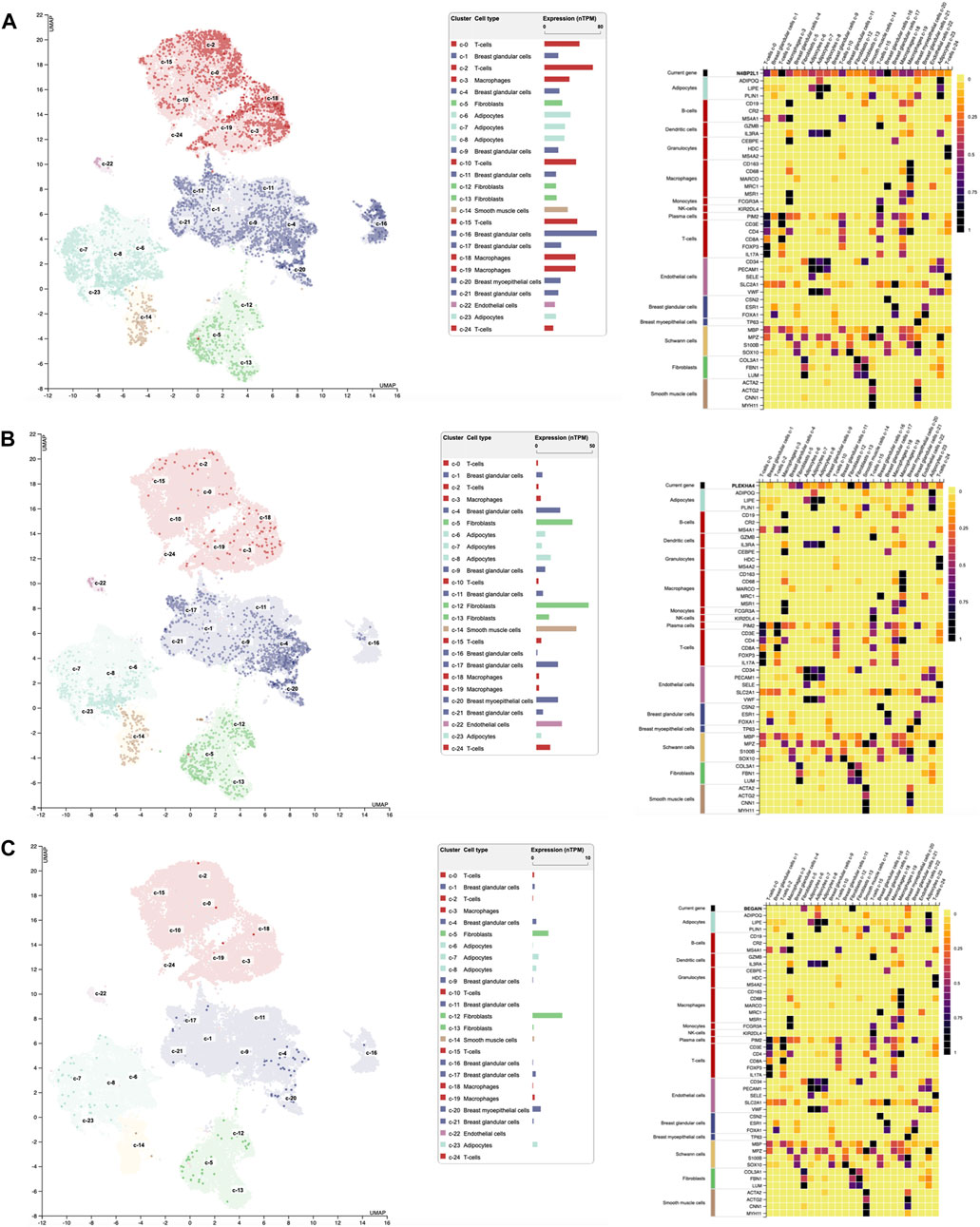
Figure 4. Single-cell RNA expression of breast tissue extracted from the Human Protein Atlas; (A–C) N4BP2L1, PLEKHA4, and BEGAIN expressions, respectively, in different cell types of breast tissue.
In relation to PLEKHA4, its higher expression was in clusters C-12 (nTPM: 47.1, fibroblasts, mesenchymal cells), C-14 (nTPM: 36.2, smooth muscle cells), and C-5 (nTPM: 32.7, fibroblasts, mesenchymal cells) (Figure 4B). Since we observed PLEKHA4 expression in myoepithelial cells (C-20, nTPM: 19.4) and smooth muscle cells, we conclude that it may have a function in muscle cells. This gene is also expressed in other cells of breast tissue, such as endothelial cells (C-22) and breast glandular cells (such as C-4 and C-17), among others. In the previous section, our data from Enrichr GO: BP showed PLEKHA4 involvement in the regulation of the Wnt signaling pathway (important in the mesenchymal stage); therefore, it is reliable to see its higher expression in fibroblasts, as mesenchymal cells, of breast tissues.
BEGAIN revealed higher expression in clusters C-12 (nTPM: 5.4, fibroblasts, mesenchymal cells), C-5 (nTPM: 2.9, fibroblasts, mesenchymal cells), and C-20 (nTPM: 1.5, myoepithelial cells). Its expression in the smooth muscle cells was 0.3 nTPM (Figure 4C). Higher expression of BEGAIN in fibroblasts, mesenchymal cells, and breast tissue suggests its possible role in the muscle process.
The highest expression of PLEKHA4 and BEGAIN was in mesenchymal cells, which was 103 nTPM and 5.4 nTPM, respectively. However, the notable expression of N4BP2L1 was in both mesenchymal cells (fibroblasts) and myoepithelial cells with the same level, 75.2 nTPM. These expression data can suggest their important roles in different cell types of breast tissue, mainly muscle cells and their progenitors, as found in our muscle cell differentiation data explained in the next section. Moreover, we observed the notably higher expression of PLEKHA4 with respect to N4BP2L1 and BEGAIN in smooth muscle cells in RNA single-cell sequencing data extracted from the Human Protein Atlas database, as found in our muscle cells differentiated from hESCs.
3.7 PLEKHA4, N4BP2L1, and BEGAIN expression across in vivo developmental stages
Based on the data retrieved from the human muscle atlas, PLEKHA4 is expressed in all muscle developmental stages, both in the hind limb muscle and myogenic subset, and its expression is higher than that of the other two genes. N4BP2L1 also showed expression in the muscle developmental stages, except for the embryonic week 6–7 myogenic subset. We found that BEGAIN is mainly expressed in hind limb muscle in all of these developmental stages. The scRNA-seq data could separate different cell types in each muscle developmental stage, and the expression of these genes was different in each subset given in Table 4; Supplementary Figure S3. Moreover, using the scRNA-seq data of the in vitro MS protocol, the protocol used in our study, we found that these genes were also expressed in these muscle cells (Figure 5). The scRNA-seq data of the MS protocol distinguished different cell types, including epithelium, skeletal development, and skeletal muscle cells. These three genes showed expression in these subsets, with the highest expression for PLEKHA4, followed by N4BP2L1 and BEGAIN (Figure 5).
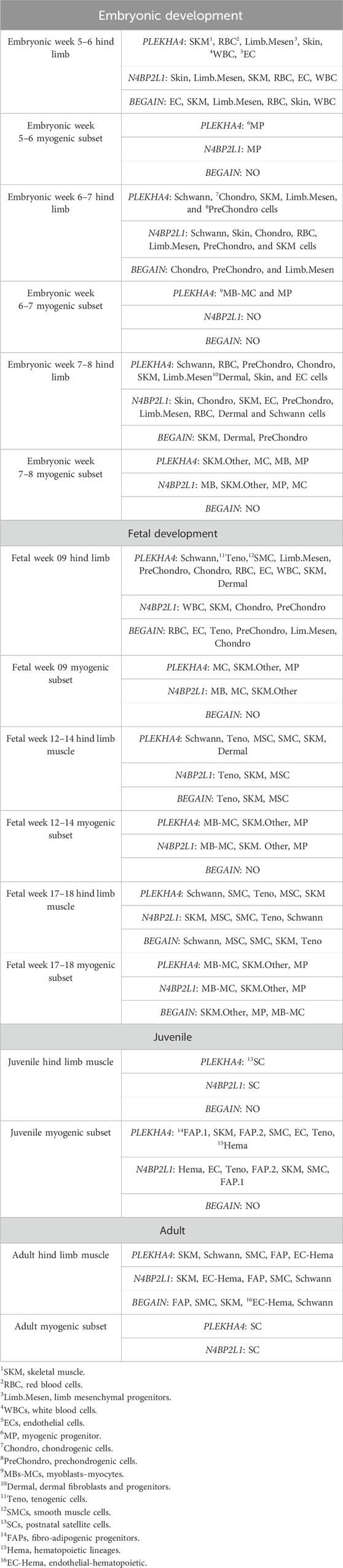
Table 4. PLEKHA4, N4BP2L1, and BEGAIN expression in different cell types of in vivo muscle developmental stages.
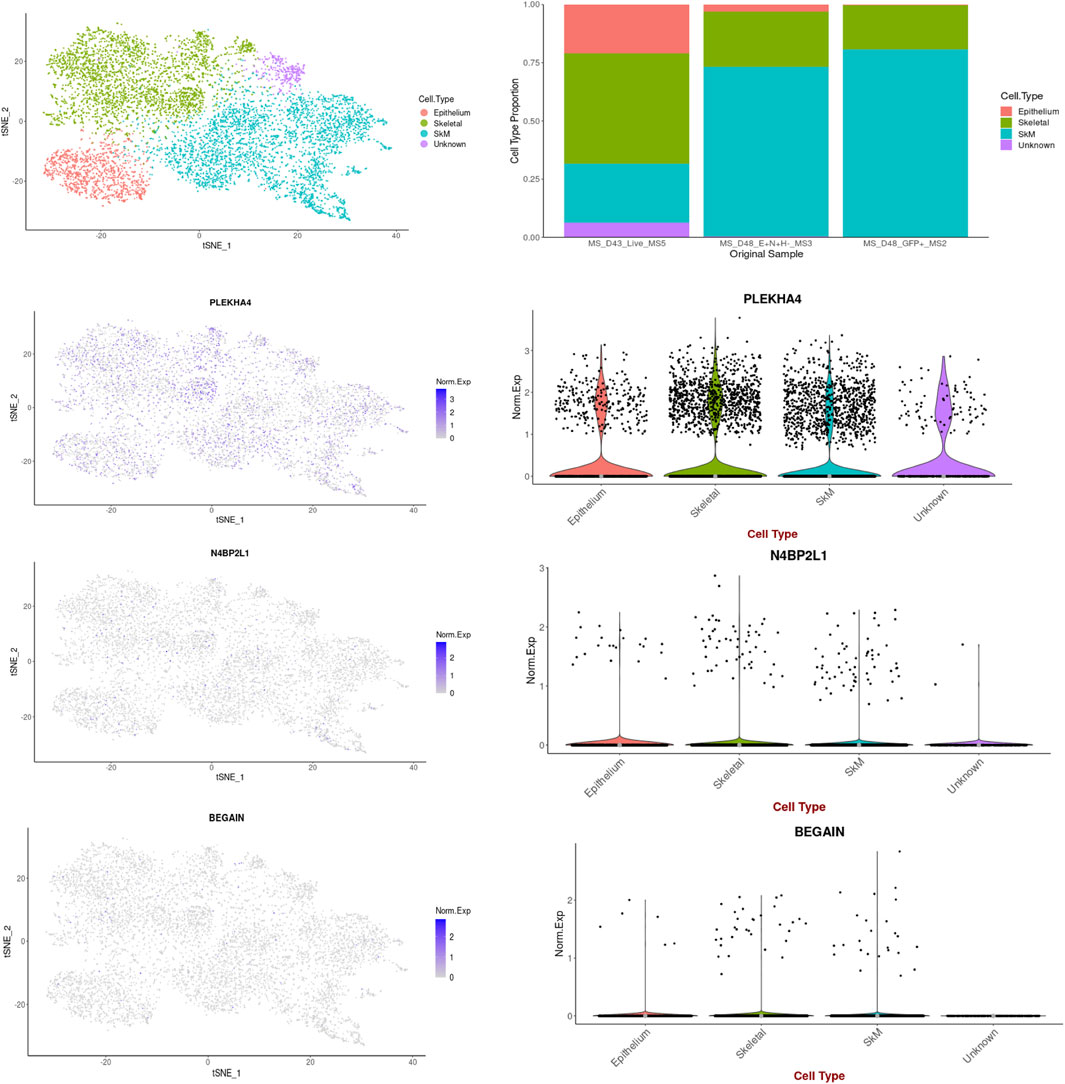
Figure 5. PLEKHA4, N4BP2L1, and BEGAIN expressions in muscle cells retrieved from the scRNA-seq data of the in vitro MS protocol.
3.8 Higher expression of PLEKHA4 and BEGAIN in MEP cells in comparison with LEP cells
Our results revealed statistically significant higher expression of PLEKHA4 (logFC: 1.81 and FDR: 0.0006) and BEGAIN (logFC: 2.76 and FDR: 0.00003) in MEP compared to LEP cells. However, for N4BP2L1, the expression was not different (logFC: 0.164 and FDR: 0.93) between these two cell types of organoids, representing their possible function in both of these cells (Figure 6; Table 5).
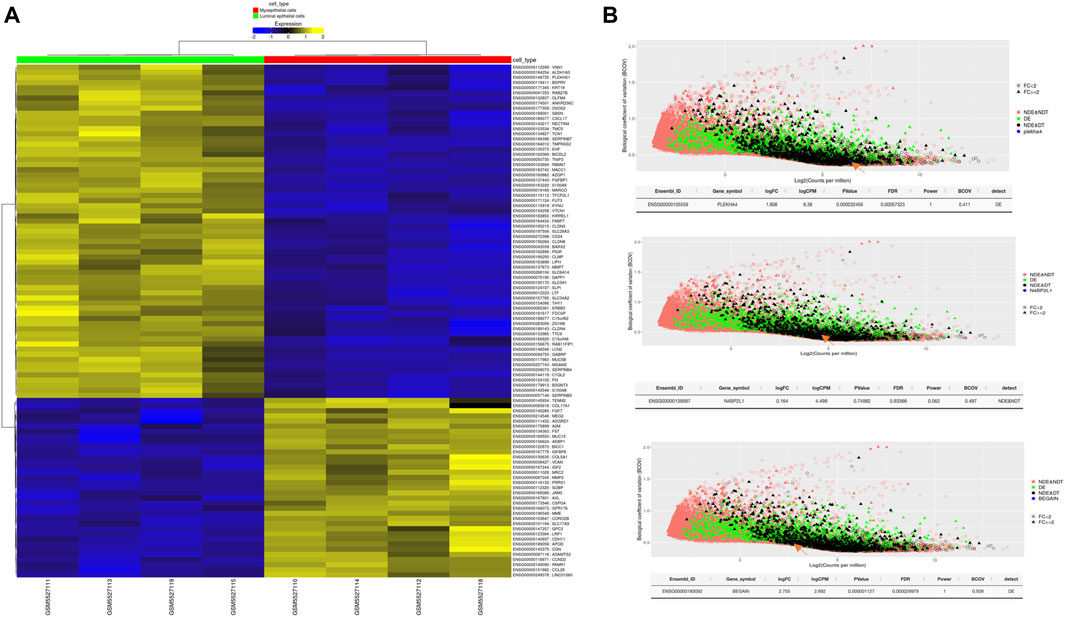
Figure 6. (A) Heatmap of differentially expressed genes between MEP and LEP cells isolated from primary breast organoids. (B) Power analysis of our proposed genes involved in muscle cells and breast cancer. The statistical power for each of the genes to be detected as differentially expressed between the selected groups is estimated. The plot shows the effect of BCOV and mean coverage on the detectability of the genes. logCPM, log counts per million; BCOV, biological coefficient of variation.
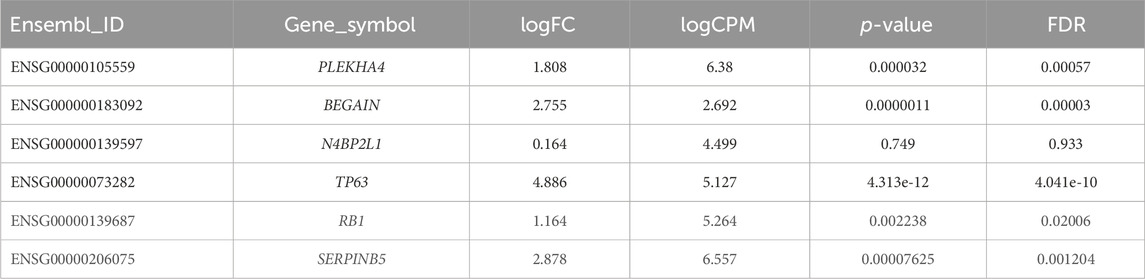
Table 5. Differential expression of some important tumor suppressor genes and our proposed genes between MEP and LEP cells isolated from primary breast organoid.
We also identified higher expression of some important tumor suppressor genes as expected, such as TP63 (logFC:4.886 and FDR:4.041e-10), RB1 (logFC:1.164 and FDR:0.02), and SERPINB5 (logFC:2.88 and FDR:0.0012) in MEP cells compared with LEP cells (Table 5). Therefore, the higher expression of these genes in normal MEP compared to LEP cells should be considered for future functional studies to find their exact roles in these cells and breast tumors.
3.9 N4BP2L1, PLEKHA4, and BEGAIN upregulation in in vitro muscle cells differentiated from hESCs
To evaluate the involvement of N4BP2L1, PLEKHA4, and BEGAIN genes in muscle cell development, we investigated their expression levels by performing RT-qPCR on muscle cells differentiated from hESCs (D0: stem stage and D46: terminally differentiated stage). The muscle differentiation process was confirmed by controlling the expression of specific stage marker genes, including TBXT (paraxial mesoderm stage marker) on day 2, PAX3 (the somite stage marker) on day 7, MYOG (a marker of skeletal muscle progenitors’ stage) on day 30 using qRT-PCR (Figure 7A), and myosin heavy chain (detected by MF20 antibody) by IF staining on day 46 (Figure 7B), confirming the expected behavior of stage-specific markers.
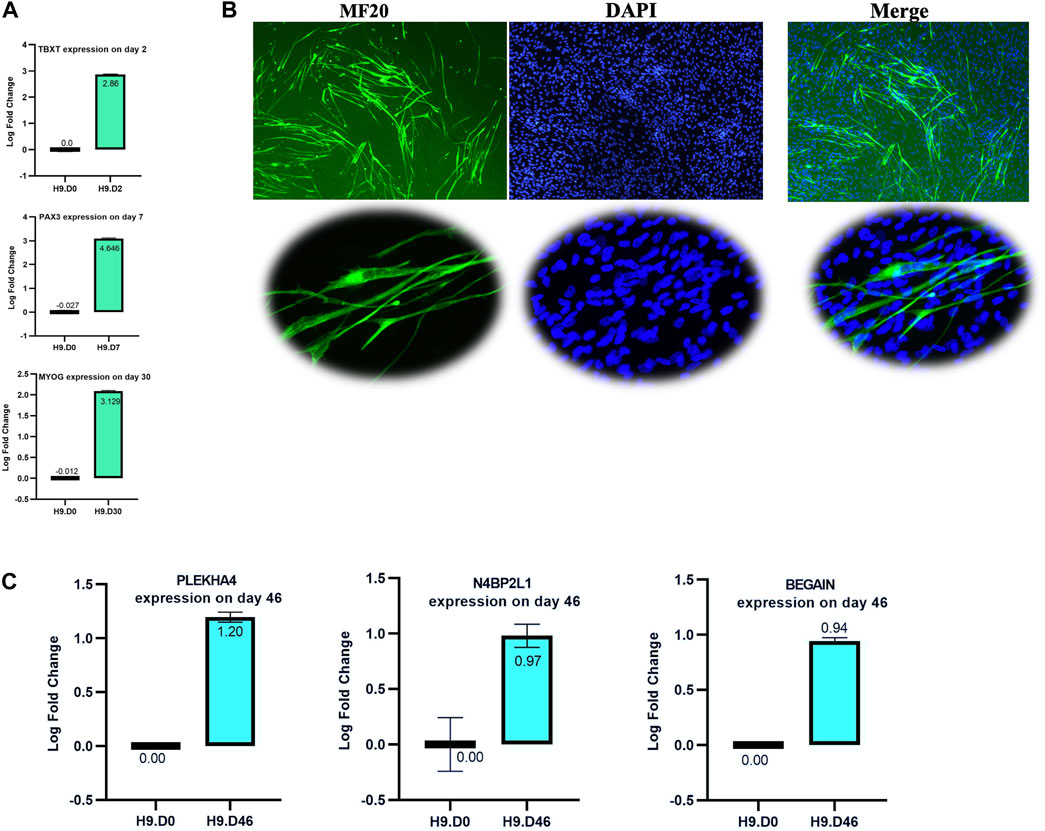
Figure 7. Validation of the muscle differentiation process. (A) RT-qPCR of selected stage marker genes shows a higher relative expression: TBXT on day 2, PAX3 on day 7, and MYOG on day 30. (B) Immunofluorescence staining of the myosin heavy chain using the MF20 antibody on day 46 of the muscle cell process. The lower image shows some multinucleated cells in our muscle cell process. (C) RT-qPCR analysis of N4BP2L1, PLEKHA4, and BEGAIN expression in muscle cells differentiated from hESCs.
In our muscle differentiation data, we found higher expression of N4BP2L1, PLEKHA4, and BEGAIN using RT-qPCR in muscle cells compared to H9 hESCs, as shown in Figure 7C. Among these genes, only PLEKHA4 functions as a transcription factor that promotes Wnt signaling through the inhibition of cytoplasmic protein disheveled (DVL) degradation (Shami Shah et al., 2019). Our data represent the role of N4BP2L1, PLEKHA4, and BEGAIN in muscle cells and breast tissue given their higher expression level in these cells compared to hESCs and their lower expression in breast cancer, representing their function as tumor-suppressive genes.
4 Discussion
In the current study, we found that PLEKHA4, BEGAIN, and N4BP2L1 correlated with patient prognosis as possible tumor suppressors with a role in muscle cells. Our result revealed a PLEKHA4 expression correlation with the age of menopause in our cohort of patients with breast cancer (Supplementary Table S1; panel A). Furthermore, in this cohort, we showed N4BP2L1 and BEGAIN as potential biomarkers of HER2-positive breast cancer (Supplementary Table S1; panels B and C). By searching Enrichr, we identified the involvement of these genes in biological processes critical for muscle cell differentiation (Figure 2).
Using the gene expression analysis of these genes in different patient statuses of TCGA-BRCA, our data could propose their function in tumorigenesis. The TCGA data analysis revealed significant downregulation of N4BP2L1, PLEKHA4, and BEGAIN genes not only in breast cancer but also in other main cancers (Supplementary Table S4). Moreover, all three genes had a significantly decreased expression in all TCGA-BRCA tumor stages compared to their matched normal tissues, representing their probable role in tumor metastasis (Supplementary Figure S1; panel 1). Furthermore, these genes showed significant downregulation in all subclasses of TCGA-BRCA compared to normal tissues (Supplementary Figure S1; panel 2), with the highest significant value for the HER2-positive subclass. Additionally, they had downregulation in various TNBC subclasses compared to normal tissues. Since N4BP2L1 and PLEKHA4 genes also had significantly decreased expression in TNBC-MSL and TNBC-M originated from mesoderm lineage, essential for the muscle differentiation process (Supplementary Figure S1; panel 3), it may indicate that their involvement is not only in breast cancer but also in muscle development. In addition, based on the histologic subtypes of TCGA-BRCA, we found significantly lower expression of these genes in their subtypes (Supplementary Figure S1; panel 4). N4BP2L1 may also play an important role in metaplastic breast cancer due to its significantly lower expression in this subtype compared to normal. Moreover, the analysis of these genes in nodal metastasis statuses of TCGA-BRCA revealed their significant downregulation in all statuses compared to the normal one, representing their probable function in breast cancer metastasis (Supplementary Figure S1; panel 5). Since NRLN metastases are considered in the prognosis assessment and clinical control of cancers, we proposed PLEKHA4 and BEGAIN as a prognostic biomarker set.
To extend the possible functionality of N4BP2L1 as a protein biomarker in breast cancer, we could find its weak expression in most of the TCGA-BRCA tumor samples (66%) (Supplementary Figure S2; panel A), its significantly lower expression in primary breast tumors of the CPTAC dataset compared to their matched normal tissues (Supplementary Figure S2; panel D), and its downregulation in different breast tumor subclasses, histological subtypes, pathways, and patients’ statues (Supplementary Figure S2; panel E).
The bulk RNA-seq data and protein expression data of these uncharacterized tumor suppressor genes in normal breast tissues collected in the GTEx databases revealed a high level of expression for PLEKHA4 and a considerable level for N4BP2L1 and BEGAIN. The single-cell RNA-seq data available from the Human Protein Atlas uncovered the different expression patterns of these genes in different cell types of breast tissue. Notably, these genes were expressed in mesenchymal cells, breast myoepithelial cells, and smooth muscle cells, indicating their possible involvement in muscle cells (Figure 4), as found by their upregulation in muscle cells differentiated from hESCs and also scRNA-seq data of in vivo muscle developmental stages (Figure 7C and 5; Supplementary Figure S3).
As mentioned in the result section, at the single-cell RNA level, we found that PLEKHA4 exhibited high expression not only in cells related to the early muscle stages and their precursors but also showed the highest expression in smooth muscle cells, C-14 (nTPM: 36.2) (Figure 4). Moreover, as expected in our muscle differentiation process, it had higher expression in terminally differentiated muscle cells (D46) (Figure 7C), representing its probable role in these cells.
In addition to the above-mentioned data, based on the data extracted from the CTD dataset, all these genes showed an association with both breast cancer and muscular disorders (Supplementary Table S5), which strengthens their possible function in these disorders.
PLEKHA4 is encoded for a plasma membrane-localized signaling protein that functions as a positive regulator of DVL protein levels through the inhibition of its polyubiquitination. This gene is a positive modulator of Wnt signaling pathways (canonical, β-catenin-dependent and non-canonical, β-catenin-independent ones) by the recruitment of the CUL3-KLHL12 E3 ligase complex to the plasma membrane, which reduces DVL protein ubiquitination, helps in increasing the DVL levels, and subsequently enhances and strengthens Wnt signaling pathways (Angers et al., 2006; Shami Shah et al., 2019). Its expression levels in our muscle differentiation stages can indicate its role in this pathway, and its downregulation in our breast cancer samples and also in TCGA-BRCA can show its role in this cancer.
N4BP2L1 is a target gene of FoxO1 and has an important function in the insulin signaling pathway due to its role in GLUT4-mediated glucose uptake, supporting its key role in adipocyte differentiation (Watanabe et al., 2019; Watanabe et al., 2021). Since the insulin signaling pathway is involved with the skeletal muscle (Ijuin et al., 2015) and breast cancer (Yee et al., 2020), N4BP2L1 can be involved in this process and breast cancer, as found in our study, with its upregulation during muscle differentiation and its downregulation in our breast cancer samples, as well as TCGA-BRCA at both the RNA and protein levels.
One study in sheep showed that BEGAIN is ubiquitously expressed in different tissues, such as skeletal muscle, at all times of development. Moreover, in the ovine, it was found as a paternally expressed gene in the ovine DLK1-GTL2-imprinted domain, expressed in the brain, kidney, liver, and skeletal muscle (Smit et al., 2005). BEGAIN was reported to play an important role in the transmission of pathological pain through the activation of the spinal cord NMDA receptor (NMDAR) in its inner lamina II (Katano et al., 2016). Therefore, based on our findings, its higher expression during muscle cell differentiation stages compared to hESCs can show its involvement in muscle cells, and its downregulation in our breast samples and TCGA-BRCA can represent its role in this cancer as a tumor suppressor gene.
In conclusion, our results proposed the important functions of these genes in muscle cells and breast tissue due to their notable upregulation in these cells compared to hESCs, their expression in muscle cells during in vivo muscle development, and their significant downregulation in different statuses of patients with breast cancer, indicating their function as possible tumor-suppressive genes.
Data availability statement
In the current study, some publicly available datasets were analyzed for the involvement of genes in cancers including TCGA, Genecards, Human Protein Atlas, and Enrichr. The raw data can be found here: https://tcga-data.nci.nih.gov/tcga/, https://www.genecards.org, https://www.proteinatlas.org, and https://maayanlab.cloud/Enrichr/. Concerning the GDCRNATools, we used the R codes from the following link: https://github.com/Jialab-UCR/GDCRNATools. Regarding data of in vivo human skeletal muscle development and primary breast organoids, we used the publicly available dataset aprilpylelab (https://aprilpylelab.com/datasets/) and GREIN tools (http://www.ilincs.org/apps/grein/?gse=GSE182338), respectively.
Ethics statement
The studies involving humans were approved by the Ethics Committee of the Fasa University of Medical Sciences, Fasa, Fars, Iran. The studies were conducted in accordance with the local legislation and institutional requirements. The participants provided their written informed consent to participate in this study.
Author contributions
HD: conceptualization, data curation, formal analysis, methodology, writing–original draft, and writing–review and editing. FA: methodology, writing–review and editing. AL: writing–review and editing, data curation, and formal analysis. CC: data curation, formal analysis, and writing–review and editing. VP: writing–review and editing and conceptualization. EM: methodology and writing–review and editing. ZF: methodology, writing–review and editing. YM: methodology, resources, and writing–review and editing. HH-A: investigation and writing–review and editing. LC: investigation, validation, writing–review and editing. SO: conceptualization, funding acquisition, resources, supervision, validation, and writing–review and editing.
Funding
The author(s) declare that financial support was received for the research, authorship, and/or publication of this article. This research was funded by AIRC IG 2022, grant number ID 27155, and IIGM institutional fund.
Acknowledgments
The authors thank all members of the laboratory for useful discussion.
Conflict of interest
The authors declare that the research was conducted in the absence of any commercial or financial relationships that could be construed as a potential conflict of interest.
Publisher’s note
All claims expressed in this article are solely those of the authors and do not necessarily represent those of their affiliated organizations, or those of the publisher, the editors, and the reviewers. Any product that may be evaluated in this article, or claim that may be made by its manufacturer, is not guaranteed or endorsed by the publisher.
Supplementary material
The Supplementary Material for this article can be found online at: https://www.frontiersin.org/articles/10.3389/fcell.2024.1295403/full#supplementary-material
References
Angers, S., Thorpe, C. J., Biechele, T. L., Goldenberg, S. J., Zheng, N., MacCoss, M. J., et al. (2006). The KLHL12-Cullin-3 ubiquitin ligase negatively regulates the Wnt-beta-catenin pathway by targeting Dishevelled for degradation. Nat. Cell Biol. 8 (4), 348–357. doi:10.1038/ncb1381
Barabasi, A. L., and Albert, R. (1999). Emergence of scaling in random networks. Science 286 (5439), 509–512. doi:10.1126/science.286.5439.509
Barbareschi, M., Pecciarini, L., Cangi, M. G., Macri, E., Rizzo, A., Viale, G., et al. (2001). p63, a p53 homologue, is a selective nuclear marker of myoepithelial cells of the human breast. Am. J. Surg. Pathol. 25 (8), 1054–1060. doi:10.1097/00000478-200108000-00010
Bohlen, J., McLaughlin, S. L., Hazard-Jenkins, H., Infante, A. M., Montgomery, C., Davis, M., et al. (2018). Dysregulation of metabolic-associated pathways in muscle of breast cancer patients: preclinical evaluation of interleukin-15 targeting fatigue. J. Cachexia Sarcopenia Muscle 9 (4), 701–714. doi:10.1002/jcsm.12294
Canadian Cancer Statistics (2023). A 2022 special report on cancer prevalence. Health Promot Chronic Dis. Prev. Can. 43 (1), 49. doi:10.24095/hpcdp.43.1.05
Cancer Research (2015). Statistics on preventable cancers. Available at: https://www.cancerresearchuk.org/health-professional/cancer-statistics/risk/preventable-cancers.
Carithers, L. J., and Moore, H. M. (2015). The genotype-tissue expression (GTEx) project. Biopreserv Biobank 13 (5), 307–308. doi:10.1089/bio.2015.29031.hmm
Chandrashekar, D. S., Bashel, B., Balasubramanya, S. A. H., Creighton, C. J., Ponce-Rodriguez, I., Chakravarthi, B., et al. (2017). UALCAN: a portal for facilitating tumor subgroup gene expression and survival analyses. Neoplasia 19 (8), 649–658. doi:10.1016/j.neo.2017.05.002
Chandrashekar, D. S., Karthikeyan, S. K., Korla, P. K., Patel, H., Shovon, A. R., Athar, M., et al. (2022). UALCAN: an update to the integrated cancer data analysis platform. Neoplasia 25, 18–27. doi:10.1016/j.neo.2022.01.001
Chou, P. H., Liao, W. C., Tsai, K. W., Chen, K. C., Yu, J. S., and Chen, T. W. (2019). TACCO, a database connecting transcriptome alterations, pathway alterations and clinical outcomes in cancers. Sci. Rep. 9 (1), 3877. doi:10.1038/s41598-019-40629-z
Consortium, G. T. (2013). The genotype-tissue expression (GTEx) project. Nat. Genet. 45 (6), 580–585. doi:10.1038/ng.2653
Dastsooz, H., Alizadeh, A., Habibzadeh, P., Nariman, A., Hosseini, A., Mansoori, Y., et al. (2021). LncRNA-miRNA-mRNA networks of gastrointestinal cancers representing common and specific LncRNAs and mRNAs. Front. Genet. 12, 791919. doi:10.3389/fgene.2021.791919
Davis, A. P., Wiegers, T. C., Johnson, R. J., Sciaky, D., Wiegers, J., and Mattingly, C. J. (2023). Comparative toxicogenomics database (CTD): update 2023. Nucleic Acids Res. 51 (D1), D1257–D1262. doi:10.1093/nar/gkac833
DiBiase, S. J., Komarnicky, L. T., Schwartz, G. F., Xie, Y., and Mansfield, C. M. (1998). The number of positive margins influences the outcome of women treated with breast preservation for early stage breast carcinoma. Cancer 82 (11), 2212–2220. doi:10.1002/(sici)1097-0142(19980601)82:11<2212::aid-cncr16>3.3.co;2-l
Edwards, N. J., Oberti, M., Thangudu, R. R., Cai, S., McGarvey, P. B., Jacob, S., et al. (2015). The CPTAC data portal: a resource for cancer proteomics research. J. Proteome Res. 14 (6), 2707–2713. doi:10.1021/pr501254j
Gaffo, E., Boldrin, E., Dal Molin, A., Bresolin, S., Bonizzato, A., Trentin, L., et al. (2019). Circular RNA differential expression in blood cell populations and exploration of circRNA deregulation in pediatric acute lymphoblastic leukemia. Sci. Rep. 9 (1), 14670. doi:10.1038/s41598-019-50864-z
Giaquinto, A. N., Sung, H., Miller, K. D., Kramer, J. L., Newman, L. A., Minihan, A., et al. (2022). Breast cancer statistics, 2022. CA Cancer J. Clin. 72 (6), 524–541. doi:10.3322/caac.21754
Harris, J. R., Lippman, M. E., Veronesi, U., and Willett, W. (1992). Breast cancer (2). N. Engl. J. Med. 327 (6), 390–398. doi:10.1056/NEJM199208063270606
Hsiao, Y. H., Su, Y. A., Tsai, H. D., Mason, J. T., Chou, M. C., and Man, Y. G. (2010). Increased invasiveness and aggressiveness in breast epithelia with cytoplasmic p63 expression. Int. J. Biol. Sci. 6 (5), 428–442. doi:10.7150/ijbs.6.428
IARC (2022). Breast cancer fact sheet. Available at: https://gco.iarc.fr/today/data/factsheets/cancers/20-Breast-fact-sheet.pdf.
Ijuin, T., Hatano, N., Hosooka, T., and Takenawa, T. (2015). Regulation of insulin signaling in skeletal muscle by PIP3 phosphatase, SKIP, and endoplasmic reticulum molecular chaperone glucose-regulated protein 78. Biochim. Biophys. Acta 1853 (12), 3192–3201. doi:10.1016/j.bbamcr.2015.09.009
James, N. D., Spears, M. R., Clarke, N. W., Dearnaley, D. P., De Bono, J. S., Gale, J., et al. (2015). Survival with newly diagnosed metastatic prostate cancer in the "docetaxel era": data from 917 patients in the control arm of the STAMPEDE trial (MRC PR08, CRUK/06/019). Eur. Urol. 67 (6), 1028–1038. doi:10.1016/j.eururo.2014.09.032
Karlsson, M., Zhang, C., Mear, L., Zhong, W., Digre, A., Katona, B., et al. (2021). A single-cell type transcriptomics map of human tissues. Sci. Adv. 7 (31), eabh2169. doi:10.1126/sciadv.abh2169
Katano, T., Fukuda, M., Furue, H., Yamazaki, M., Abe, M., Watanabe, M., et al. (2016). Involvement of brain-enriched guanylate kinase-associated protein (BEGAIN) in chronic pain after peripheral nerve injury. eNeuro 3 (5). doi:10.1523/ENEURO.0110-16.2016
Kazama, T., Nakamura, S., Doi, O., Suzuki, K., Hirose, M., and Ito, H. (2005). Prospective evaluation of pectoralis muscle invasion of breast cancer by MR imaging. Breast Cancer 12 (4), 312–316. doi:10.2325/jbcs.12.312
Kerslake, R. W., Carleton, P. J., Fox, J. N., Imrie, M. J., Cook, A. M., Read, J. R., et al. (1995). Dynamic gradient-echo and fat-suppressed spin-echo contrast-enhanced MRI of the breast. Clin. Radiol. 50 (7), 440–454. doi:10.1016/s0009-9260(05)83159-9
King, B. L., Davis, A. P., Rosenstein, M. C., Wiegers, T. C., and Mattingly, C. J. (2012). Ranking transitive chemical-disease inferences using local network topology in the comparative toxicogenomics database. PLoS One 7 (11), e46524. doi:10.1371/journal.pone.0046524
Kratzer, T. B., Jemal, A., Miller, K. D., Nash, S., Wiggins, C., Redwood, D., et al. (2023). Cancer statistics for American Indian and Alaska Native individuals, 2022: including increasing disparities in early onset colorectal cancer. CA Cancer J. Clin. 73 (2), 120–146. doi:10.3322/caac.21757
Kuleshov, M. V., Jones, M. R., Rouillard, A. D., Fernandez, N. F., Duan, Q., Wang, Z., et al. (2016). Enrichr: a comprehensive gene set enrichment analysis web server 2016 update. Nucleic Acids Res. 44 (W1), W90–W97. doi:10.1093/nar/gkw377
Lagios, M. D. (1992). Pathologic features related to local recurrence following lumpectomy and irradiation. Semin. Surg. Oncol. 8 (3), 122–128. Available at: https://www.ncbi.nlm.nih.gov/pubmed/1496221.
Lee, S. C., Jain, P. A., Jethwa, S. C., Tripathy, D., and Yamashita, M. W. (2014). Radiologist's role in breast cancer staging: providing key information for clinicians. Radiographics 34 (2), 330–342. doi:10.1148/rg.342135071
Li, H., and Liang, S. (2009). Local network topology in human protein interaction data predicts functional association. PLoS One 4 (7), e6410. doi:10.1371/journal.pone.0006410
Li, J. H., and Man, Y. G. (2009). Dual usages of single Wilms' tumor 1 immunohistochemistry in evaluation of breast tumors: a preliminary study of 30 cases. Cancer Biomark. 5 (3), 109–116. doi:10.3233/CBM-2009-0595
Li, R., Qu, H., Wang, S., Wei, J., Zhang, L., Ma, R., et al. (2018). GDCRNATools: an R/Bioconductor package for integrative analysis of lncRNA, miRNA and mRNA data in GDC. Bioinformatics 34 (14), 2515–2517. doi:10.1093/bioinformatics/bty124
Luo, Z., Cai, Q., Zhao, Y., Wang, X., Fu, S., and Zhai, L. (2018). Late distant recurrence of breast carcinoma and metastasis to the main bronchus and choroid: a case report. Med. Baltim. 97 (20), e10754. doi:10.1097/MD.0000000000010754
Mahi, N. A., Najafabadi, M. F., Pilarczyk, M., Kouril, M., and Medvedovic, M. (2019). GREIN: an interactive web platform for Re-analyzing GEO RNA-seq data. Sci. Rep. 9 (1), 7580. doi:10.1038/s41598-019-43935-8
Man, Y. G. (2010). Tumor cell budding from focally disrupted tumor capsules: a common pathway for all breast cancer subtype derived invasion? J. Cancer 1, 32–37. doi:10.7150/jca.1.32
Man, Y. G., and Nieburgs, H. E. (2006). A subset of cell clusters with malignant features in morphologically normal-appearing and hyperplastic tissues. Cancer Detect Prev. 30 (3), 239–247. doi:10.1016/j.cdp.2006.04.006
Man, Y. G., and Sang, Q. X. (2004). The significance of focal myoepithelial cell layer disruptions in human breast tumor invasion: a paradigm shift from the "protease-centered" hypothesis. Exp. Cell Res. 301 (2), 103–118. doi:10.1016/j.yexcr.2004.08.037
Man, Y. G., Zhang, Y., Shen, T., Zeng, X., Tauler, J., Mulshine, J. L., et al. (2005). cDNA expression profiling reveals elevated gene expression in cell clusters overlying focally disrupted myoepithelial cell layers: implications for breast tumor invasion. Breast Cancer Res. Treat. 89 (2), 199–208. doi:10.1007/s10549-004-2049-6
Mango, V. L., Kaplan, J., Sung, J. S., Moskowitz, C. S., Dershaw, D. D., and Morris, E. A. (2015). Breast carcinoma in augmented breasts: MRI findings. AJR Am. J. Roentgenol. 204 (5), W599–W604. doi:10.2214/AJR.14.13221
Mansoori, H., Darbeheshti, F., Daraei, A., Mokhtari, M., Tabei, M. B., Abdollahzadeh, R., et al. (2021). Expression signature of lncRNA APTR in clinicopathology of breast cancer: its potential oncogenic function in dysregulation of ErbB signaling pathway. Gene Rep. 23, 101116. doi:10.1016/j.genrep.2021.101116
McNeill, R. E., Miller, N., and Kerin, M. J. (2007). Evaluation and validation of candidate endogenous control genes for real-time quantitative PCR studies of breast cancer. BMC Mol. Biol. 8, 107. doi:10.1186/1471-2199-8-107
Myers, K. S., Stern, E., Ambinder, E. B., and Oluyemi, E. T. (2021). Breast cancer abutting the pectoralis major muscle on breast MRI: what are the clinical implications? Br. J. Radiol. 94 (1119), 20201202. doi:10.1259/bjr.20201202
Obedian, E., and Haffty, B. G. (2000). Negative margin status improves local control in conservatively managed breast cancer patients. Cancer J. Sci. Am. 6 (1), 28–33. Available at: https://www.ncbi.nlm.nih.gov/pubmed/10696736.
Orel, S. G., Schnall, M. D., LiVolsi, V. A., and Troupin, R. H. (1994). Suspicious breast lesions: MR imaging with radiologic-pathologic correlation. Radiology 190 (2), 485–493. doi:10.1148/radiology.190.2.8284404
Park, C. C., Mitsumori, M., Nixon, A., Recht, A., Connolly, J., Gelman, R., et al. (2000). Outcome at 8 years after breast-conserving surgery and radiation therapy for invasive breast cancer: influence of margin status and systemic therapy on local recurrence. J. Clin. Oncol. 18 (8), 1668–1675. doi:10.1200/JCO.2000.18.8.1668
Pasquero, G., Surace, A., Ponti, A., Bortolini, M., Tota, D., Mano, M. P., et al. (2020). Role of magnetic resonance imaging in the evaluation of breast cancer response to neoadjuvant chemotherapy. Vivo 34 (2), 909–915. doi:10.21873/invivo.11857
Pond, G. R., Sonpavde, G., de Wit, R., Eisenberger, M. A., Tannock, I. F., and Armstrong, A. J. (2014). The prognostic importance of metastatic site in men with metastatic castration-resistant prostate cancer. Eur. Urol. 65 (1), 3–6. doi:10.1016/j.eururo.2013.09.024
Sanchez, E. R., Sanchez, R., and Moliver, C. (2014). Anatomic relationship of the pectoralis major and minor muscles: a cadaveric study. Aesthetic Surg. J. 34 (2), 258–263. doi:10.1177/1090820x13519643
Sayaman, R. W. M. M., Senapati, P., Shalabi, S., Zirbes, A., Todhunter, M. E., Seewaldt, V., et al. (2021). Epigenetic changes with age primes mammary luminal epithelia for cancer initiation. bioRxiv. doi:10.1101/2021.02.12.430777
Shami Shah, A., Batrouni, A. G., Kim, D., Punyala, A., Cao, W., Han, C., et al. (2019). PLEKHA4/kramer attenuates dishevelled ubiquitination to modulate Wnt and planar cell polarity signaling. Cell Rep. 27 (7), 2157–2170. doi:10.1016/j.celrep.2019.04.060
Shelton, M., Kocharyan, A., Liu, J., Skerjanc, I. S., and Stanford, W. L. (2016). Robust generation and expansion of skeletal muscle progenitors and myocytes from human pluripotent stem cells. Methods 101, 73–84. doi:10.1016/j.ymeth.2015.09.019
Shiovitz, S., and Korde, L. A. (2015). Genetics of breast cancer: a topic in evolution. Ann. Oncol. 26 (7), 1291–1299. doi:10.1093/annonc/mdv022
Siegel, R. L., Miller, K. D., Fedewa, S. A., Ahnen, D. J., Meester, R. G. S., Barzi, A., et al. (2017). Colorectal cancer statistics, 2017. Ca-a Cancer J. Clin. 67 (3), 177–193. doi:10.3322/caac.21395
Smit, M. A., Tordoir, X., Gyapay, G., Cockett, N. E., Georges, M., and Charlier, C. (2005). BEGAIN: a novel imprinted gene that generates paternally expressed transcripts in a tissue- and promoter-specific manner in sheep. Mamm. Genome 16 (10), 801–814. doi:10.1007/s00335-004-2415-z
Stelzer, G., Rosen, N., Plaschkes, I., Zimmerman, S., Twik, M., Fishilevich, S., et al. (2016). The GeneCards suite: from gene data mining to disease Genome sequence analyses. Curr. Protoc. Bioinforma. 54 (1), 30 31–31 30 33. doi:10.1002/cpbi.5
Uhlen, M., Bjorling, E., Agaton, C., Szigyarto, C. A., Amini, B., Andersen, E., et al. (2005). A human protein atlas for normal and cancer tissues based on antibody proteomics. Mol. Cell Proteomics 4 (12), 1920–1932. doi:10.1074/mcp.M500279-MCP200
Uhlen, M., Fagerberg, L., Hallstrom, B. M., Lindskog, C., Oksvold, P., Mardinoglu, A., et al. (2015). Proteomics. Tissue-based map of the human proteome. Science 347 (6220), 1260419. doi:10.1126/science.1260419
Vilmont, V., Cadot, B., Ouanounou, G., and Gomes, E. R. (2016). A system for studying mechanisms of neuromuscular junction development and maintenance. Development 143 (13), 2464–2477. doi:10.1242/dev.130278
Vo, J. N., Cieslik, M., Zhang, Y., Shukla, S., Xiao, L., Zhang, Y., et al. (2019). The landscape of circular RNA in cancer. Cell 176 (4), 869–881. doi:10.1016/j.cell.2018.12.021
Watanabe, K., Matsumoto, A., Tsuda, H., and Iwamoto, S. (2021). N4BP2L1 interacts with dynactin and contributes to GLUT4 trafficking and glucose uptake in adipocytes. J. Diabetes Investig. 12 (11), 1958–1966. doi:10.1111/jdi.13623
Watanabe, K., Yokota, K., Yoshida, K., Matsumoto, A., and Iwamoto, S. (2019). A novel upstream transcription factor 1 target gene N4bp2l1 that regulates adipogenesis. Biochem. Biophys. Rep. 20, 100676. doi:10.1016/j.bbrep.2019.100676
Xi, H., Langerman, J., Sabri, S., Chien, P., Young, C. S., Younesi, S., et al. (2020). A human skeletal muscle atlas identifies the trajectories of stem and progenitor cells across development and from human pluripotent stem cells. Cell Stem Cell 27 (1), 181–185. doi:10.1016/j.stem.2020.06.006
Xu, Z., Wang, W., Deng, C. X., and Man, Y. G. (2009). Aberrant p63 and WT-1 expression in myoepithelial cells of pregnancy-associated breast cancer: implications for tumor aggressiveness and invasiveness. Int. J. Biol. Sci. 5 (1), 82–96. doi:10.7150/ijbs.5.82
Yee, L. D., Mortimer, J. E., Natarajan, R., Dietze, E. C., and Seewaldt, V. L. (2020). Metabolic health, insulin, and breast cancer: why oncologists should care about insulin. Front. Endocrinol. (Lausanne) 11, 58. doi:10.3389/fendo.2020.00058
Zemni, I., Kacem, M., Dhouib, W., Bennasrallah, C., Hadhri, R., Abroug, H., et al. (2022). Breast cancer incidence and predictions (Monastir, Tunisia: 2002-2030): a registry-based study. PLoS One 17 (5), e0268035. doi:10.1371/journal.pone.0268035
Keywords: breast cancer, muscle cells, myoepithelial cells, the cancer genome atlas, breast cancer, tumor suppressor, biomarker
Citation: Dastsooz H, Anselmi F, Lauria A, Cicconetti C, Proserpio V, Mohammadisoleimani E, Firoozi Z, Mansoori Y, Haghi-Aminjan H, Caizzi L and Oliviero S (2024) Involvement of N4BP2L1, PLEKHA4, and BEGAIN genes in breast cancer and muscle cell development. Front. Cell Dev. Biol. 12:1295403. doi: 10.3389/fcell.2024.1295403
Received: 16 September 2023; Accepted: 22 April 2024;
Published: 24 May 2024.
Edited by:
Shyamala Maheswaran, Massachusetts General Hospital and Harvard Medical School, United StatesReviewed by:
Ana Paula Zen Petisco Fiore, New York University, United StatesRamiro Magno, Pattern Institute, Portugal
Copyright © 2024 Dastsooz, Anselmi, Lauria, Cicconetti, Proserpio, Mohammadisoleimani, Firoozi, Mansoori, Haghi-Aminjan, Caizzi and Oliviero. This is an open-access article distributed under the terms of the Creative Commons Attribution License (CC BY). The use, distribution or reproduction in other forums is permitted, provided the original author(s) and the copyright owner(s) are credited and that the original publication in this journal is cited, in accordance with accepted academic practice. No use, distribution or reproduction is permitted which does not comply with these terms.
*Correspondence: Salvatore Oliviero, oliviero.salvatore@iigm.it, salvatore.oliviero@unito.it