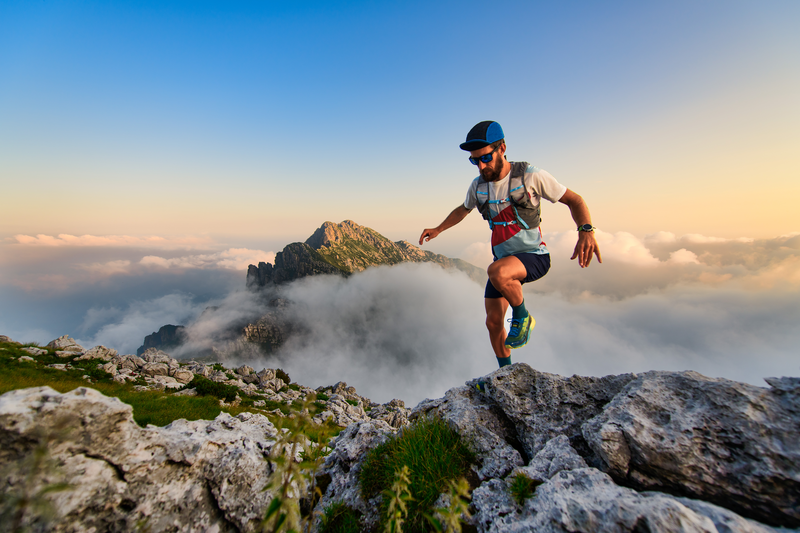
94% of researchers rate our articles as excellent or good
Learn more about the work of our research integrity team to safeguard the quality of each article we publish.
Find out more
EDITORIAL article
Front. Cell Dev. Biol. , 21 November 2023
Sec. Stem Cell Research
Volume 11 - 2023 | https://doi.org/10.3389/fcell.2023.1326727
This article is part of the Research Topic Three-dimensional/3D Stem Cell Culture Systems View all 8 articles
Editorial on the Research Topic
Three-dimensional/3D stem cell culture systems
In the 20th century, the advent of cell culture techniques revolutionised the understanding of cellular mechanisms in physiological or pathological conditions. However, traditional bi-dimensional (2D) culture in a petri dish lacks important cell-cell and cell-extracellular matrix (ECM) interactions, culminating in an artificial microenvironment. In this way, cells embedded in three-dimensional (3D) matrices bring more cell interactions and improve cell response by using many materials to mimic the ECM, mainly collagens. Since the isolation and characterisation of the embryonic and adult stem cells and cell reprogramming, the modern 3D cell culture strategies try to recreate the tissue-specific organisation by self-assembling cells in a multicellular aggregates scaffold-based or scaffold-free way. In the last decade, the transition from traditional 2D to 3D cell culture increased substantially, and we can see massive progress in obtaining spheroids and very complex organoids and assembloids (Bissell, 2017; Jedrzejczak-Silicka, 2017). The European Association for Cancer Research (EARC), which coined the term “Goodbye Flat Biology,” initiated a series of conferences in 2014 to discuss the evolution of 3D microenvironment in the cancer context. Associated with the evolution of bioprinting techniques, we can now print structured tissue-like grafts for Tissue Engineering applications or develop new in vitro platforms for drug development or Precision Medicine. Also, dynamic cell culture (perfusion) methods from macro to micro scales boost the biophysical and biomechanical properties of the 3D constructions. This Research Topic presents six papers (reviews and original research) that will permeate all these subjects.
Over the last 70 years, different cell culture methods for skin reconstruction have been developed, such as 3D skin equivalents by cells-ladden in biomimetic matrices and, more recently, 3D bioprinting (Souci and Denesvre, 2021). Such models will be in demand not only for drug testing but also for testing cosmetics and household chemicals, modeling skin diseases, studies of absorption of substances through the skin and others. Here, Santos et al. compared the commitment potential of umbilical cord stem cells (a type of mesenchymal stem cell) into keratinocytes in a 3D in vitro skin model composed of dermal equivalent (collagen type I + dermal fibroblasts) or commercial porcine skin decellularised matrix, both in an air-liquid interface assay. The dermal equivalent induced more expression of epidermal markers and cell differentiation, resulting in skin organotypic cultures. This platform is a promise for skin biology studies and in vitro toxicological assays.
According to Atala’s classification of anatomical organs, skin is the simplest flat organ. This explains the many in vitro skin models and skin equivalents. Full-fledged models of more complex tubular and solid organs like the intestine, kidney or lung, are not yet available. The generation of miniaturised organs and tissues in vitro was possible after the crucial knowledge of pluripotent stem cells (embryonic and reprogrammed) and approaches for differentiation towards mature cell lineages. It gained force from the seminal study on gut organoid done by Sato et al. (Sato et al., 2009). Then, organoids will be applied to developmental biology, drug development, precision medicine, toxicology and disease modelling studies in various organs/tissues. Here, Xu et al. update the main advances and challenges in organoid models based on iPSCs (inducible pluripotent stem cells), such as the brain, liver, kidney, heart and lung (mainly to understand the mechanism of the COVID-19).
Cerebral and brain organoids, or “mini-brains,” were one of the first organoids made (human pluripotent stem cells) (Lancaster et al., 2013). Here, Horánszky et al. showed the effects of bisphenol A (BPA), a well-known endocrine-disrupting compound, in a new long-term human iPSC spheroid-induced neural stem cell differentiation model. BPA treatment (repeated-dose exposure with sub-cytotoxicity) reduced spheroid size and decreased cell proliferation but did not affect cells after 21 days in culture. Moreover, proteomic analysis confirmed these findings. It is a new method for investigating neurotoxicity in the earliest stages of central nervous system development.
The kidney is one of the most complex organs in terms of different cell types and multiple nephron segments. The first kidney organoid or tubuloid models have been established only from human embryonic stem cells (Takasato et al., 2014), iPSC (Taguchi et al., 2014), or adult/progenitor stem cells (Schutgens et al., 2019). Here, Hanhof et al. showed, for the first time, a duct-enriched 3D tubuloid from a mouse kidney primary cell culture, representing the collecting tube (CD), the most distal part of the nephron. This model forms a single layer of polarised epithelium in 3D. The upregulation of gene and protein expression of specific collecting duct markers, such as water channel AQP2 and the sodium channel ENaC, was confirmed. Also, the response to the pharmacological agents in calcium uptake has successfully assessed the physiological function. This model is a powerful tool for studying CD physiology and modelling many renal pathologies.
Bone is a complex organ which is composed of many different types of cells, including bone-forming cells (osteoblasts), bone-homeostasis cells (osteocytes), and bone-resorbing cells (osteoclasts). These cells reside on the surface or inside distinct bone architectures (trabeculae or cortical) and are exposed to internal and external stimuli. Also, bone is formed by organic (non-mineralised ECM—osteoid) and inorganic fractions (mineralised ECM with hydroxyapatite crystals) given different physiological microenvironments. In this way, the reconstruction of bone in vitro is very challenging, in which 2D cell culture brings poor results, and the primary way to study bone biology is in vivo models. Here, Lipreri et al. show how different 3D in vitro fluidic perfused platforms can mimic bone microenvironment at the macro/mili/microscale. These approaches will drive bone biology studies in the coming years.
Many advances have been achieved using 3D cell culture models, including organoids; however, they lack the physiological system in static cell culture mode. In this way, microfluidic devices can connect different organs/tissues on a microscale, bringing the microphysiology, named organ-on-a-chip. The first was developed in 2010 by recapitulating the lung alveolus (Huh et al., 2010). Here, Baptista et al. focused on converging organoid, 3D bioprinting and microfluidic technologies (named 3D organ-on-a-chip) to boost drug development and pharmacological screening.
KP: Conceptualization, Writing–original draft, Writing–review and editing. JG: Conceptualization, Writing–review and editing. LB: Conceptualization, Writing–review and editing. AM: Conceptualization, Writing–review and editing. AR: Conceptualization, Writing–review and editing. EK: Conceptualization, Writing–review and editing.
The author(s) declare that no financial support was received for the research, authorship, and/or publication of this article.
The authors declare that the research was conducted in the absence of any commercial or financial relationships that could be construed as a potential conflict of interest.
All claims expressed in this article are solely those of the authors and do not necessarily represent those of their affiliated organizations, or those of the publisher, the editors and the reviewers. Any product that may be evaluated in this article, or claim that may be made by its manufacturer, is not guaranteed or endorsed by the publisher.
Bissell, M. J. (2017). Goodbye flat biology – time for the 3rd and the 4th dimensions. J. Cell Sci. 130, 3–5. doi:10.1242/jcs.200550
Huh, D., Matthews, B. D., Mammoto, A., Montoya-Zavala, M., Hsin, H. Y., and Ingber, D. E. (2010). Reconstituting organ-level lung functions on a chip. Science 328, 1662–1668. doi:10.1126/science.1188302
Jedrzejczak-Silicka, M. (2017). History of cell culture. Available at: https://api.semanticscholar.org/CorpusID:56568576.
Lancaster, M. A., Renner, M., Martin, C.-A., Wenzel, D., Bicknell, L. S., Hurles, M. E., et al. (2013). Cerebral organoids model human brain development and microcephaly. Nature 501, 373–379. doi:10.1038/nature12517
Sato, T., Vries, R. G., Snippert, H. J., van de Wetering, M., Barker, N., Stange, D. E., et al. (2009). Single Lgr5 stem cells build crypt-villus structures in vitro without a mesenchymal niche. Nature 459, 262–265. doi:10.1038/nature07935
Schutgens, F., Rookmaaker, M. B., Margaritis, T., Rios, A., Ammerlaan, C., Jansen, J., et al. (2019). Tubuloids derived from human adult kidney and urine for personalized disease modeling. Nat. Biotechnol. 37, 303–313. doi:10.1038/s41587-019-0048-8
Souci, L., and Denesvre, C. (2021). 3D skin models in domestic animals. Vet. Res. 52, 21. doi:10.1186/s13567-020-00888-5
Taguchi, A., Kaku, Y., Ohmori, T., Sharmin, S., Ogawa, M., Sasaki, H., et al. (2014). Redefining the in vivo origin of metanephric nephron progenitors enables generation of complex kidney structures from pluripotent stem cells. Cell Stem Cell 14, 53–67. doi:10.1016/j.stem.2013.11.010
Keywords: stem cells, spheroids, organoids, microfluidics, organ-on-a-chip, 3D models, 3D bioprinting, bone
Citation: Paiva KBS, Granjeiro JM, Baptista LS, Marques AP, Ribeiro AR and Koudan E (2023) Editorial: Three-dimensional/3D stem cell culture systems. Front. Cell Dev. Biol. 11:1326727. doi: 10.3389/fcell.2023.1326727
Received: 23 October 2023; Accepted: 14 November 2023;
Published: 21 November 2023.
Edited and Reviewed by:
Valerie Kouskoff, The University of Manchester, United KingdomCopyright © 2023 Paiva, Granjeiro, Baptista, Marques, Ribeiro and Koudan. This is an open-access article distributed under the terms of the Creative Commons Attribution License (CC BY). The use, distribution or reproduction in other forums is permitted, provided the original author(s) and the copyright owner(s) are credited and that the original publication in this journal is cited, in accordance with accepted academic practice. No use, distribution or reproduction is permitted which does not comply with these terms.
*Correspondence: Katiucia Batista Silva Paiva, katipaiva@usp.br
Disclaimer: All claims expressed in this article are solely those of the authors and do not necessarily represent those of their affiliated organizations, or those of the publisher, the editors and the reviewers. Any product that may be evaluated in this article or claim that may be made by its manufacturer is not guaranteed or endorsed by the publisher.
Research integrity at Frontiers
Learn more about the work of our research integrity team to safeguard the quality of each article we publish.