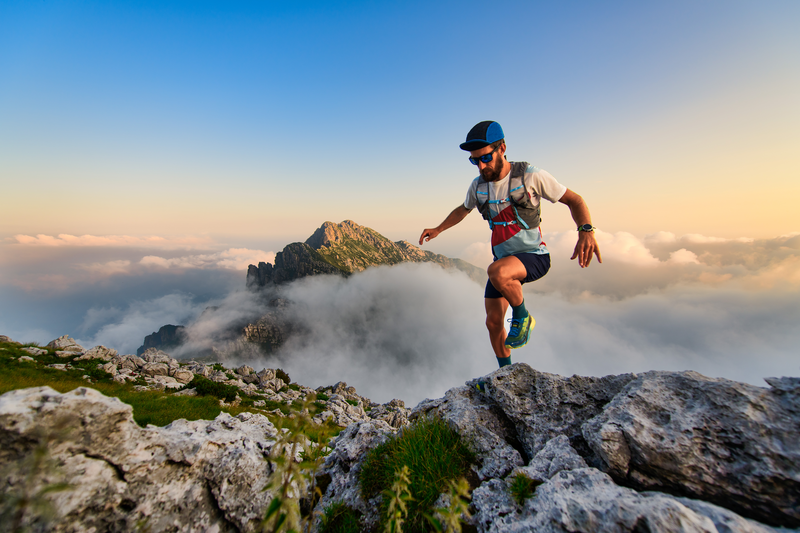
95% of researchers rate our articles as excellent or good
Learn more about the work of our research integrity team to safeguard the quality of each article we publish.
Find out more
OPINION article
Front. Cell Dev. Biol. , 06 December 2023
Sec. Evolutionary Developmental Biology
Volume 11 - 2023 | https://doi.org/10.3389/fcell.2023.1320209
This article is part of the Research Topic The Future of the Homology Concept under Novel Evolutionary Paradigms View all 5 articles
Ctenophores or comb jellies possess one of the most unique neural organizations of enigmatic origins; and there are no recognized homologies to any other phylum. The recent integrative (Moroz et al., 2014) and comparative genomics (Ryan et al., 2013; Whelan et al., 2015; Whelan et al., 2017; Li et al., 2021; Whelan and Halanych, 2023), especially cross-phyla chromosome level synteny (Schultz et al., 2023), analyses strongly confirmed a surprising hypothesis that morphologically and behaviorally complex ctenophores are descendants of the earliest metazoan branch, followed by simpler nerveless sponges (Porifera) and Placozoa (Figure 1). Moreover, the molecular deciphering of neural toolkits in ctenophores reveals their unique molecular organization (Moroz, 2015), including reduced representation of canonical bilaterian neurogenic and synaptic gene complement, distinct molecular profiling of ctenophore neurons as well as the apparent lack of classical low molecular weight transmitters (Moroz et al., 2014; Moroz et al., 2020). It is possible to state that ctenophores use remarkably different chemical language for intercellular communications with a unique (mostly unknown) subset of signal molecules as the hallmark of their neural architecture.
FIGURE 1. Phylogeny of animals with ctenophores as the sister lineage to the rest of Metazoa. Comparative analyses suggest convergent evolution of neurons, synapses, muscles, and mesoderm (see text for details). Possible origins of microRNA and HOX gene cluster are indicated (Moroz et al., 2014).
Specifically, both the complement of neurotransmitter synthetic enzymes and, most importantly, direct microchemical analyses of neurotransmitters themselves (Moroz et al., 2014; Moroz et al., 2020) indicate that acetylcholine, serotonin, dopamine, noradrenaline, adrenaline, and histamine are not produced by ctenophores studied so far, including Pleurobrachia and Mnemiopsis (Moroz et al., 2014; Moroz and Kohn, 2016). Furthermore, initial pharmacological tests also failed to observe noticeable behavioral effects of these low molecular weight “classical” transmitters (Moroz et al., 2014; Norekian and Moroz, 2023). Thus, we concluded that monoamines and acetylcholine are true bilaterian innovations (Moroz and Kohn, 2015; 2016; Moroz et al., 2021b), later confirmed with the additional comparative survey of synthetic and metabolic enzymes (Goulty et al., 2023). Glutamate was initially proposed as a neuromuscular transmitter and a possible interneuronal transmitter in ctenophores (Moroz et al., 2014; Moroz, 2015; Moroz et al., 2020; Moroz et al., 2021a). In contrast, ctenophores (including Pleurobrachia and Mnemiopsis with two sequenced genomes at that time) developed several dozen small signaling peptides and neuropeptides, which have no detectable homologs outside Ctenophora (Moroz et al., 2014; Moroz and Kohn, 2016) with two possible exceptions (Yañez-Guerra et al., 2022).
The obtained interdisciplinary evidence leads to the conclusion that ctenophores independently developed neural systems (Moroz, 2014; Moroz et al., 2014) and independently evolved synaptic organization (Moroz and Kohn, 2016; Moroz et al., 2021b). Therefore, ctenophore neurons are not homologous to cnidarian and bilaterian neurons. Thus, we attempted to refine and broaden the definition of neurons and also used terms of alternative neural and integrative systems (Moroz et al., 2021b; Moroz and Romanova, 2022). In other words, neurons are synaptically coupled polarized and highly heterogenous secretory cells at the top of behavioral hierarchies with learning capabilities; and we postulated that neurons are functional rather than genetic categories (Moroz and Kohn, 2016).
In summary, ctenophore neurons result from convergent evolution with their very own array of chemical transmitters, including ctenophore-specific neuropeptides. Recent immunohistochemical and pharmacological experiments confirmed this hypothesis and showed specific distribution and behavioral effects of ctenophore-specific neurotransmitters in Mnemiopsis (Sachkova et al., 2021) and Bolinopsis (Hayakawa et al., 2022). The overall assessment was that ctenophores broadly used chemical (volume) and more localized synaptic signaling as the dominant way of interneuronal communications with more than 100 signaling molecules (Moroz et al., 2021b). Earlier transmission electron microscopy data identified unique chemical synapses across structures and species in ctenophores, as summarized by Mari Luz Hernandez-Nicaise (Hernandez-Nicaise, 1991), see also Figure 2C.
FIGURE 2. Syncytium-like neuroid systems in tentacles of Pleurobrachia bachei (from (Norekian and Moroz, 2019b)). Scanning electron microscopy of the polygonal neural net in the tentacle pocket. (A) The upper epithelium layer is peeled off in some areas (arrows), revealing the underlying layer of the neural network. (B) The neural syncytial-type network consists of different polygonal units, neural cell bodies, and anastomosed neural processes (yellow arrows). (C) Neural nets and unique synaptic organization in ctenophores (modified from (Hernandez-Nicaise, 1991; Moroz, 2015)). (1) The basic features of synapses in ctenophores. The generalized asymmetrical synapse. (2) Symmetrical neurite-to-neurite synapse in Beroe. Scale bar: 100 nm. (3) The asymmetrical synapse between a neurite and an epithelial cell (ep) in the epidermis of Pleurobrachia. Scale bar: 200 nm. (4) Soma-to-soma reciprocal synapse in the epithelium of Bolina hydatina. Scale bar: 100 nm. c.v., cytoplasmic vesicles; co, dense coat on the postsynaptic membrane; e.r., endoplasmic reticulum; g, Golgi complex; l, intracleft dense line; M, mesoglea; mi, mitochondrion; mt, microtubules; n, nucleus; p, presynaptic dense projections; r, ribosomes; s.v., synaptic vesicle. (5) The schematic diagram of the subepithelial nerve system of a generalized cydippid (the aboral view). Images are reproduced and adapted from Hernandez-Nicaise (Hernandez-Nicaise, 1991) with permission from Wiley-Liss, Inc. (D) Subepithelial neural net in Pleuronrachia bachei stained with tubulin antibody (from (Norekian and Moroz, 2019b)). Neural net consists of polygonal units of different shapes and sizes. There are individual neurons (white arrows) with clearly visible neurites (yellow arrows). The segments of the neural net are composed of tightly packed thin processes, rather than a single thick axon.Scale bars: (A) 100 μm; (C) 20 μm, (D) 50 μm.
Recent and remarkable ultrastructural data with volume microscopy validate the uniqueness of neural systems and synapses in ctenophores (Sachkova et al., 2021; Burkhardt et al., 2023), further reinforcing our earlier hypothesis of their independent origins (Moroz and Kohn, 2016). However, besides the canonical neural organization with distinct synapses, ctenophores likely possess syncytial-type connectivity in some neuronal populations, such as components of subepithelial nerve net and possibly in the gut (Burkhardt et al., 2023). This 3D electron microscopy reconstruction of neural nets highlighted an apparent “resurrection” of the original Golgi’s reticular theory (Burkhardt et al., 2023). Furthermore, the initial perception of the novel volume microscopic data might be that non-[chemical]synaptic transmission is the distinct characteristic of ctenophore organization in general (Dunn, 2023; Lenharo, 2023), in contrast to other animals and the Cajal’s neuronal doctrine. Moreover, recent discussions and news releases might represent these ultrastructural data as evidence that all ctenophore neurons form the neuroid-type syncytium and have reduced chemical transmission across all neural circuits. Or this situation might be viewed as the predominance of syncytial organization for electrical propagation of signals vs. chemical transmitter-mediated signaling. Experimental functional exploration is needed to understand the cellular bases of ctenophore behaviors.
Toward this discussion, I think that ctenophore neural communications are primarily chemical, with deep ancestry of chemical signaling at the base of animal and neural organization. Here, I summarize this viewpoint and the prospects for future studies.
The Neuron Doctrine postulated anatomical and functional identities of individual neurons as the foundation of any neural organization, stressing morphological and physiological discontinuity of neurons in central and peripheral neural systems. Nevertheless, in his vision of the Neuron Doctrine, Raymon y Cajal wisely considered that “neuronal discontinuity… could sustain some exceptions” (Cajal, 1995; Bullock et al., 2005). Coupling cells and neurites into functional syncytia might occur with and without electrical synapses. Ctenophores present an exceptional opportunity to readdress 130-year-old concepts of neuronal architectures.
There are three groups of questions. i) How universal are ctenophore neural syncytia during development and across species? ii) Is syncytial organization unique to ctenophore neurons? iii) What are relationships between neuroid syncytia and chemical signaling with distinct secretory machinery in behavioral integrations of ctenophores? Interdisciplinary comparative studies would be needed to address these questions experimentally.
Burkhard and others performed their remarkable 3D electron microscopy observations on small, just-hatching larval/juvenile animals of the lobate ctenophore, Mnemiopsis leidyi (Sachkova et al., 2021; Burkhardt et al., 2023), with developing neural systems consisting of a few dozen putative neurons (Norekian and Moroz, 2021). Whether or not the syncytial organization is preserved within a greater neuronal diversity in adult Mnemiopsis must be determined.
First, the neural syncytium within some ctenophore neural nets is possible and likely exists in other species, such as the cydippid Pleurobrachia bachei (e.g., Figure 1C in (Moroz et al., 2014)). For example, we did observe such architecture within the nerve net of tentacle pockets (Norekian and Moroz, 2019b) of adult Pleurobrachia (Figures 2A,B), the species with an estimated ∼10,000 individual neurons (Norekian and Moroz, 2016; 2019b). Nevertheless, most subepithelial neural nets in Pleurobrachia and more than ten other investigated species have neurons with two or more neurites within their orthogons (Figure 2D, see details in (Norekian and Moroz, 2016;2019b;a;2020), and in contrast to one neurite of studied microscopic Mnemiopsis, suggesting that different types of communications are involved.
Second, although syncytial types of networks are relatively rare, neuroid-type syncytia, similar to these found in ctenophores, were observed in the representatives of at least six animal phyla. However, this list can be expanded since most “minor” phyla remain unexplored. Syncytial-like neural nets might exist in the colonial polyp Velella (Mackie, 1960; Mackie et al., 1988) [Cnidaria]. In the cephalopod stellate ganglion, neuronal processes are fused to form giant axons (Young, 1939). Neuronal membrane fusion was also reported in gastropod molluscs, annelids [leeches], nematodes, and mammals (Oren-Suissa et al., 2010; Giordano-Santini et al., 2016; Giordano-Santini et al., 2020). Specifically, neurite and synaptic fusion occur during neural development and neuroplasticity in Drosophila (Yu and Schuldiner, 2014) [Arthropoda] and mammals (Faust et al., 2021) [Chordata], likely contributing to metabolic coupling, fast propagating, axon and dendrite pruning, and integration of signaling.
Third, based on published data, only a limited fraction of ctenophore neurons make a syncytial nerve net (Burkhardt et al., 2023). In the recent reconstruction, only 5 of 33 studied neurons in the early stages of Mnemiopsis can form a syncytium with fused plasma membranes (Burkhardt et al., 2023). Still, these characterized neurons revealed diverse chemical synapses with characteristic ctenophore-specific presynaptic triads of organelles arranged in layers of synaptic vesicles, endoplasmic reticulum, and mitochondrion (Hernandez-Nicaise, 1973; Hernandez-Nicaise, 1991).
Burkhard and others did not report chemical synapses between subepithelial neurons; however, 3D reconstruction revealed chemical synapses from subepithelial neurons to multiple effector cells such as ciliated structures—polster cells in combs (Burkhardt et al., 2023). Furthermore, 4 of 5 studied populations of sensory neurons make morphologically recognized synapses to subepithelial and mesogleal neurons as well as among themselves and comb cells (Burkhardt et al., 2023), confirming the widespread distribution of chemical synapses within neural systems of Mnemiopsis.
It is worth noting that all ctenophore neurons and their neurites contained a diversity of secretory vesicles, suggesting recruitments of multiple neurotransmitters with possible co-localization of signal molecules within the same neuron. The presented ultramicroscopic images indicate about 60–70 sites with dense-core vesicles within a 2-3 neuronal soma diameter area (Burkhardt et al., 2023), suggesting that even this anastomosed subnet can be a neurosecretory system without identified gap junctions among subepithelial neurons. Indeed, the anastomosed neurites contain endogenous neuropeptides, e.g., ML02736a (Sachkova et al., 2021) as possible secretory products of these nets.
Structural constraints of the discovered syncytial-like net are equally essential in understanding the directional propagation of neural signaling in ctenophores. Burkhard and others visualized distinct “blebbed or ‘pearls-on-a-string’ morphology” of neurites in the subepithelial layer with a chain of secretory vesicles (Burkhardt et al., 2023). Of note, secretory vesicles are separated by extremely narrow (∼50–60 nm) cytoplasmatic bridges, sufficient for few microtubules to pass through. A similar type of organization was also recently observed in some rodent axons (Griswold et al., 2023). How these vesicles are transported to these locations or maintained is unclear. How, for example, electrical signals can be propagated along these ultranarrow channels with apparently high resistance are unanswered questions. Saltatory electrical conduction combined with the volume release of neurosecretory molecules might occur. Unfortunately, the majority of signal molecules are unknown in the ctenophore lineage. The current subset of transmitters includes i) L-/D Glutamate (Moroz et al., 2014; Moroz et al., 2020) and ii) glycine as a potential agonist of some ionotropic glutamate receptors in ctenophores (Alberstein et al., 2015; Yu et al., 2016), iii) gaseous nitric oxide (NO) (Moroz et al., 2023), plus iv) several ctenophore-specific neuropeptides (Moroz et al., 2014; Sachkova et al., 2021; Hayakawa et al., 2022), and possibly some catecholamines (Townsend and Sweeney, 2019). Many surprises are expected with apparently alternative chemical “syntax” and even the chemical “alphabet” of signaling molecules in this still very enigmatic lineage of basal metazoans.
1. Ctenophore nets are structurally and molecularly unique compared to other metazoans. The syncytial-type organization occurs in neural nets within the subepithelium, the gut of Mnemiopsis (Burkhardt et al., 2023), and the Pleurobrachia tentacle pocket (Norekian and Moroz, 2019b). These ultrastructural data provide additional support for the convergent nature of ctenophore neurons (Moroz et al., 2014; Moroz and Kohn, 2016).
2. Unique tripartite synapses, unique molecular neural and synaptic toolkits, unique expression of transcription factors, and diversity of unique ctenophore-specific neuropeptides, plus deficiency of bilaterian + cnidarian low molecular weight transmitters, are arguments for the hypothesis of independent origins of ctenophore neural systems, as proposed earlier (Moroz, 2009; Moroz, 2014; Moroz et al., 2014; Moroz, 2021).
3. Whether the syncytial organization of some ctenophore larval neurons is a primarily or secondary traits remains to be determined by ongoing comparative analyses of other ctenophore species. More likely, neuroid syncytia are evolutionarily derived events and relatively rare specializations for particular functions, as evident from other fused neurons in some cnidarians and bilaterians.
4. The directionality of neuronal signaling in ctenophores is evident from the behaviors of these animals as ambush or active predators. Existing information favors the predominance of chemical signaling in ctenophores and its essential role in neuronal integration and behavioral control to be further explored. For example, suppression of synaptic transmission in high magnesium solutions eliminated the coordinated activity of cilia in intact and semi-intact ctenophore preparations (Norekian and Moroz, 2023).
5. The emerging peptidergic nature of the ctenophore neural systems (Moroz et al., 2014; Sachkova et al., 2021; Hayakawa et al., 2022) is consistent with the hypothesis that neurons evolved from secretory cells (Moroz, 2009; 2014; 2021). Moreover, the astonishing diversity and higher information capacity of classical synapses (Moroz and Romanova, 2021) and volume transmission indicate that chemical signaling is the hallmark of neural and other integrative systems regardless of their origins (Moroz et al., 2021b; Norekian and Moroz, 2021; Moroz and Romanova, 2022). Cajal’s neuronal doctrine applies to ctenophores in full and “…could sustain some exceptions” (Cajal, 1995; Bullock et al., 2005) as secondary specializations to be investigated functionally.
6. Finally, in addition to neuronal systems, the ctenophore evolved several parallel electrical conductive systems in the ciliated furrows via gap junctions formed by at least 12 innexins (Hernandez-Nicaise, 1968; Tamm, 1973; Tamm, 1982; Tamm, 1984; Tamm and Moss, 1985; Hernandez-Nicaise, 1991; Tamm and Tamm, 2002; Moroz et al., 2014; Tamm, 2014; Moroz and Kohn, 2016; Norekian and Moroz, 2020). We might also expect the presence of alternative integrative (electrical and chemical) systems in this still enigmatic group of early-branching metazoans.
LM: Conceptualization, Funding acquisition, Investigation, Resources, Validation, Writing–original draft, Writing–review and editing.
The author(s) declare financial support was received for the research, authorship, and/or publication of this article. This work was supported by the National Science Foundation (IOS-1557923) and, in part, by the National Institute of Neurological Disorders and Stroke of the National Institutes of Health under Award Number R01NS114491.
The author declares that the research was conducted in the absence of any commercial or financial relationships that could be construed as a potential conflict of interest.
All claims expressed in this article are solely those of the authors and do not necessarily represent those of their affiliated organizations, or those of the publisher, the editors and the reviewers. Any product that may be evaluated in this article, or claim that may be made by its manufacturer, is not guaranteed or endorsed by the publisher.
The content is solely the author’ responsibility and does not necessarily represent the official views of the National Institutes of Health.
Alberstein, R., Grey, R., Zimmet, A., Simmons, D. K., and Mayer, M. L. (2015). Glycine activated ion channel subunits encoded by ctenophore glutamate receptor genes. Proc. Natl. Acad. Sci. U. S. A. 112, E6048–E6057. doi:10.1073/pnas.1513771112
Bullock, T. H., Bennett, M. V., Johnston, D., Josephson, R., Marder, E., and Fields, R. D. (2005). Neuroscience. The neuron doctrine, redux. Science 310, 791–793. doi:10.1126/science.1114394
Burkhardt, P., Colgren, J., Medhus, A., Digel, L., Naumann, B., Soto-Angel, J. J., et al. (2023). Syncytial nerve net in a ctenophore adds insights on the evolution of nervous systems. Science 380, 293–297. doi:10.1126/science.ade5645
Cajal, S. R. Y. (1995). Histology of the nervous system of man and vertebrates. New York: Oxford Univ. Press.
Dunn, C. (2023). Neurons that connect without synapses. Science 380, 241–242. doi:10.1126/science.adh0542
Faust, T. E., Gunner, G., and Schafer, D. P. (2021). Mechanisms governing activity-dependent synaptic pruning in the developing mammalian CNS. Nat. Rev. Neurosci. 22, 657–673. doi:10.1038/s41583-021-00507-y
Giordano-Santini, R., Kaulich, E., Galbraith, K. M., Ritchie, F. K., Wang, W., Li, Z., et al. (2020). Fusogen-mediated neuron-neuron fusion disrupts neural circuit connectivity and alters animal behavior. Proc. Natl. Acad. Sci. U. S. A. 117, 23054–23065. doi:10.1073/pnas.1919063117
Giordano-Santini, R., Linton, C., and Hilliard, M. A. (2016). Cell-cell fusion in the nervous system: alternative mechanisms of development, injury, and repair. Semin. Cell Dev. Biol. 60, 146–154. doi:10.1016/j.semcdb.2016.06.019
Goulty, M., Botton-Amiot, G., Rosato, E., Sprecher, S. G., and Feuda, R. (2023). The monoaminergic system is a bilaterian innovation. Nat. Commun. 14, 3284. doi:10.1038/s41467-023-39030-2
Griswold, J. M., Bonilla-Quintana, M., Pepper, R., Lee, C. T., Raychaudhuri, S., Ma, S., et al. (2023). Membrane mechanics dictate axonal morphology and function. bioRxiv, 2023, Available at: https://doi.org/10.1101/2023.07.20.549958.
Hayakawa, E., Guzman, C., Horiguchi, O., Kawano, C., Shiraishi, A., Mohri, K., et al. (2022). Mass spectrometry of short peptides reveals common features of metazoan peptidergic neurons. Nat. Ecol. Evol. 6, 1438–1448. doi:10.1038/s41559-022-01835-7
Hernandez-Nicaise, M. L. (1968). Specialized connexions between nerve cells and mesenchymal cells in ctenophores. Nature 217, 1075–1076. doi:10.1038/2171075a0
Hernandez-Nicaise, M. L. (1973). The nervous system of ctenophores. III. Ultrastructure of synapses. J. Neurocytol. 2, 249–263. doi:10.1007/BF01104029
Hernandez-Nicaise, M.-L. (1991). “Ctenophora,” in Microscopic anatomy of invertebrates: Placozoa, Porifera, Cnidaria, and Ctenophora. Editors F. W. F. W Harrison, and J. A Westfall (New York: Wiley), 359–418.
Lenharo, M. (2023). Comb jellies' unique fused neurons challenge evolution ideas. Nature 2023. doi:10.1038/d41586-023-01381-7
Li, Y., Shen, X. X., Evans, B., Dunn, C. W., and Rokas, A. (2021). Rooting the animal tree of life. Mol. Biol. Evol. 38, 4322–4333. doi:10.1093/molbev/msab170
Mackie, G. O. (1960). The structure of the nervous system in Velella. J. Cell Sci. s3-101, 119–131. doi:10.1242/jcs.s3-101.54.119
Mackie, G. O., Singla, C. L., and Arkett, S. A. (1988). On the nervous system of Velella (Hydrozoa: chondrophora). J. Morphol. 198, 15–23. doi:10.1002/jmor.1051980103
Moroz, L. L. (2009). On the independent origins of complex brains and neurons. Brain Behav. Evol. 74, 177–190. doi:10.1159/000258665
Moroz, L. L. (2014). The genealogy of genealogy of neurons. Commun. Integr. Biol. 7, e993269. doi:10.4161/19420889.2014.993269
Moroz, L. L. (2015). Convergent evolution of neural systems in ctenophores. J. Exp. Biol. 218, 598–611. doi:10.1242/jeb.110692
Moroz, L. L. (2021). Multiple origins of neurons from secretory cells. Front. Cell Dev. Biol. 9, 669087. doi:10.3389/fcell.2021.669087
Moroz, L. L., Kocot, K. M., Citarella, M. R., Dosung, S., Norekian, T. P., Povolotskaya, I. S., et al. (2014). The ctenophore genome and the evolutionary origins of neural systems. Nature 510, 109–114. doi:10.1038/nature13400
Moroz, L. L., and Kohn, A. B. (2015). Unbiased view of synaptic and neuronal gene complement in ctenophores: are there pan-neuronal and pan-synaptic genes across Metazoa? Integr. Comp. Biol. 55, 1028–1049. doi:10.1093/icb/icv104
Moroz, L. L., and Kohn, A. B. (2016). Independent origins of neurons and synapses: insights from ctenophores. Philos. Trans. R. Soc. Lond B Biol. Sci. 371, 20150041. doi:10.1098/rstb.2015.0041
Moroz, L. L., Mukherjee, K., and Romanova, D. Y. (2023). Nitric oxide signaling in ctenophores. Front. Neurosci. 17, 1125433. doi:10.3389/fnins.2023.1125433
Moroz, L. L., Nikitin, M. A., Policar, P. G., Kohn, A. B., and Romanova, D. Y. (2021a). Evolution of glutamatergic signaling and synapses. Neuropharmacology 199, 108740. doi:10.1016/j.neuropharm.2021.108740
Moroz, L. L., and Romanova, D. Y. (2021). Selective advantages of synapses in evolution. Front. Cell Dev. Biol. 9, 726563. doi:10.3389/fcell.2021.726563
Moroz, L. L., and Romanova, D. Y. (2022). Alternative neural systems: what is a neuron? (Ctenophores, sponges and placozoans). Front. Cell Dev. Biol. 10, 1071961. doi:10.3389/fcell.2022.1071961
Moroz, L. L., Romanova, D. Y., and Kohn, A. B. (2021b). Neural versus alternative integrative systems: molecular insights into origins of neurotransmitters. Philos. Trans. R. Soc. Lond B Biol. Sci. 376, 20190762. doi:10.1098/rstb.2019.0762
Moroz, L. L., Sohn, D., Romanova, D. Y., and Kohn, A. B. (2020). Microchemical identification of enantiomers in early-branching animals: lineage-specific diversification in the usage of D-glutamate and D-aspartate. Biochem. Biophys. Res. Commun. 527, 947–952. doi:10.1016/j.bbrc.2020.04.135
Norekian, T. P., and Moroz, L. L. (2016). Development of neuromuscular organization in the ctenophore Pleurobrachia bachei. J. Comp. Neurol. 524, 136–151. doi:10.1002/cne.23830
Norekian, T. P., and Moroz, L. L. (2019a). Neural system and receptor diversity in the ctenophore Beroe abyssicola. J. Comp. Neurol. 527, 1986–2008. doi:10.1002/cne.24633
Norekian, T. P., and Moroz, L. L. (2019b). Neuromuscular organization of the ctenophore Pleurobrachia bachei. J. Comp. Neurol. 527, 406–436. doi:10.1002/cne.24546
Norekian, T. P., and Moroz, L. L. (2020). Comparative neuroanatomy of ctenophores: neural and muscular systems in Euplokamis dunlapae and related species. J. Comp. Neurol. 528, 481–501. doi:10.1002/cne.24770
Norekian, T. P., and Moroz, L. L. (2021). Development of the nervous system in the early hatching larvae of the ctenophore Mnemiopsis leidyi. J. Morphol. 282, 1466–1477. doi:10.1002/jmor.21398
Norekian, T. P., and Moroz, L. L. (2023). Recording cilia activity in ctenophores: effects of nitric oxide and low molecular weight transmitters. Front. Neurosci. 17, 1125476. doi:10.3389/fnins.2023.1125476
Oren-Suissa, M., Hall, D. H., Treinin, M., Shemer, G., and Podbilewicz, B. (2010). The fusogen EFF-1 controls sculpting of mechanosensory dendrites. Science 328, 1285–1288. doi:10.1126/science.1189095
Ryan, J. F., Pang, K., Schnitzler, C. E., Nguyen, A. D., Moreland, R. T., Simmons, D. K., et al. (2013). The genome of the ctenophore Mnemiopsis leidyi and its implications for cell type evolution. Science 342, 1242592. doi:10.1126/science.1242592
Sachkova, M. Y., Nordmann, E. L., Soto-Angel, J. J., Meeda, Y., Gorski, B., Naumann, B., et al. (2021). Neuropeptide repertoire and 3D anatomy of the ctenophore nervous system. Curr. Biol. 31, 5274–5285.e6. doi:10.1016/j.cub.2021.09.005
Schultz, D. T., Haddock, S. H. D., Bredeson, J. V., Green, R. E., Simakov, O., and Rokhsar, D. S. (2023). Ancient gene linkages support ctenophores as sister to other animals. Nature 618, 110–117. doi:10.1038/s41586-023-05936-6
Tamm, S. (1973). Mechanisms of ciliary Co-ordination in ctenophores. J. Exp. Biol. 59, 231–245. doi:10.1242/jeb.59.1.231
Tamm, S. L. (1982). “Ctenophora,” in Electrical conduction and behavior in "simple" invertebrates (Oxford: Clarendon Press), 266–358.
Tamm, S. L. (1984). Mechanical synchronization of ciliary beating within comb plates of ctenophores. J. Exp. Biol. 113, 401–408. doi:10.1242/jeb.113.1.401
Tamm, S. L. (2014). Cilia and the life of ctenophores. Invertebr. Biol. 133, 1–46. doi:10.1111/ivb.12042
Tamm, S. L., and Moss, A. G. (1985). Unilateral ciliary reversal and motor responses during prey capture by the ctenophore Pleurobrachia. J. Exp. Biol. 114, 443–461. doi:10.1242/jeb.114.1.443
Tamm, S. L., and Tamm, S. (2002). Novel bridge of axon-like processes of epithelial cells in the aboral sense organ of ctenophores. J. Morphol. 254, 99–120. doi:10.1002/jmor.10019
Townsend, J. P., and Sweeney, A. M. (2019). Catecholic compounds in ctenophore colloblast and nerve net proteins suggest a structural role for DOPA-like molecules in an early-diverging animal lineage. Biol. Bull. 236, 55–65. doi:10.1086/700695
Whelan, N. V., and Halanych, K. M. (2023). Available data do not rule out Ctenophora as the sister group to all other Metazoa. Nat. Commun. 14, 711. doi:10.1038/s41467-023-36151-6
Whelan, N. V., Kocot, K. M., Moroz, L. L., and Halanych, K. M. (2015). Error, signal, and the placement of Ctenophora sister to all other animals. Proc. Natl. Acad. Sci. U. S. A. 112, 5773–5778. doi:10.1073/pnas.1503453112
Whelan, N. V., Kocot, K. M., Moroz, T. P., Mukherjee, K., Williams, P., Paulay, G., et al. (2017). Ctenophore relationships and their placement as the sister group to all other animals. Nat. Ecol. Evol. 1, 1737–1746. doi:10.1038/s41559-017-0331-3
Yañez-Guerra, L. A., Thiel, D., and Jékely, G. (2022). Premetazoan origin of neuropeptide signaling. Mol. Biol. Evol. 39, msac051. doi:10.1093/molbev/msac051
Young, Z. Y. (1939). Fused neurons and synaptic contacts in the giant nerve fibres of cephalopods. Philos. Trans. R. Soc. Lond. B. Biol. Sci. 229, 465–503. doi:10.1098/rstb.1939.0003
Yu, A., Alberstein, R., Thomas, A., Zimmet, A., Grey, R., Mayer, M. L., et al. (2016). Molecular lock regulates binding of glycine to a primitive NMDA receptor. Proc. Natl. Acad. Sci. U. S. A. 113, E6786–E6795. doi:10.1073/pnas.1607010113
Keywords: ctenophora, porifera, placozoa, cnidaria, nervous system evolution, neurotransmission, synapses, animal origins
Citation: Moroz LL (2023) Syncytial nets vs. chemical signaling: emerging properties of alternative integrative systems. Front. Cell Dev. Biol. 11:1320209. doi: 10.3389/fcell.2023.1320209
Received: 12 October 2023; Accepted: 22 November 2023;
Published: 06 December 2023.
Edited by:
Thiago André, University of Brasilia, BrazilReviewed by:
Ildiko M. L. Somorjai, University of St Andrews, United KingdomCopyright © 2023 Moroz. This is an open-access article distributed under the terms of the Creative Commons Attribution License (CC BY). The use, distribution or reproduction in other forums is permitted, provided the original author(s) and the copyright owner(s) are credited and that the original publication in this journal is cited, in accordance with accepted academic practice. No use, distribution or reproduction is permitted which does not comply with these terms.
*Correspondence: Leonid L. Moroz, bW9yb3pAd2hpdG5leS51ZmwuZWR1
Disclaimer: All claims expressed in this article are solely those of the authors and do not necessarily represent those of their affiliated organizations, or those of the publisher, the editors and the reviewers. Any product that may be evaluated in this article or claim that may be made by its manufacturer is not guaranteed or endorsed by the publisher.
Research integrity at Frontiers
Learn more about the work of our research integrity team to safeguard the quality of each article we publish.