- 1Joint Laboratory of Artificial Intelligence for Critical Care Medicine, Department of Critical Care Medicine, Institutes for Systems Genetics, Frontiers Science Center for Disease-Related Molecular Network, West China Hospital, Sichuan University, Chengdu, China
- 2School of Pharmaceutical Sciences, Lovely Professional University, Phagwara, Punjab, India
Diabetes-related pathophysiological alterations and various female reproductive difficulties were common in pregnant women with gestational diabetes mellitus (GDM), who had 21.1 million live births. Preeclampsia (PE), which increases maternal and fetal morbidity and mortality, affects approximately 3%–5% of pregnancies worldwide. Nevertheless, it is unclear what triggers PE and GDM to develop. Therefore, the development of novel moderator therapy approaches is a crucial advancement. Chemokines regulate physiological defenses and maternal-fetal interaction during healthy and disturbed pregnancies. Chemokines regulate immunity, stem cell trafficking, anti-angiogenesis, and cell attraction. CXC chemokines are usually inflammatory and contribute to numerous reproductive disorders. Fractalkine (CX3CL1) may be membrane-bound or soluble. CX3CL1 aids cell survival during homeostasis and inflammation. Evidence reveals that CXC and CX3CL1 chemokines and their receptors have been the focus of therapeutic discoveries for clinical intervention due to their considerable participation in numerous biological processes. This review aims to give an overview of the functions of CXC and CX3CL1 chemokines and their receptors in the pathophysiology of PE and GDM. Finally, we examined stimulus specificity for CXC and CX3CL1 chemokine expression and synthesis in PE and GDM and preclinical and clinical trials of CXC-based PE and GDM therapies.
1 Introduction
After 20 weeks of gestation, preeclampsia (PE), a severe pregnancy complication, is primarily characterized by hypertension and proteinuria. The global incidence of PE ranges between 3% and 10% (Steegers et al., 2010). PE, which occurs approximately in 3%–5% of pregnancies worldwide, is a significant contributor to maternal and fetal morbidity and mortality, high healthcare expenses, and up to 25% of low-birthweight preterm infants (Mol et al., 2016; Robillard et al., 2019). Hence, PE endangers the safety of both mother and infant during the perinatal period. Nonetheless, the etiology and pathogenesis of PE remain unclear, and the typical recommended treatment strategy is still inadequate. The previous description of the onset and progression of PE’s clinical symptoms can be described as follows (Huppertz, 2008; Duley, 2009): Stage 1 is when the mother and the allogeneic embryo do not have complete immune tolerance for each other when an embryo is implanted (Chaiworapongsa et al., 2014); Stage 2 of PE is described by placental hypoxia and syncytiotrophoblasts (STBs) dysplasia, which establishes the pathological basis for PE by resulting from diminished trophoblast invasion in the first trimester; and stage 3 is defined by placental ischemia and hypoxia, which result in severe metabolic problems, improper spiral artery remodeling, placental apoptosis, and an imbalance in the immune response (Redman et al., 2014). Thus, a maternal immune system’s instability is a critical component in the development of PE.
Gestational diabetes mellitus (GDM) affects women between 24 and 28 weeks of pregnancy and can adversely affect their health. According to estimates, pregnant women (aged 20–49) with some form of diabetes delivered 21.1 million live babies in 2021 (Guariguata et al., 2022). Globally, the incidence of GDM has rapidly increased in recent years, creating a severe issue for public health (Szmuilowicz et al., 2019).
Most studies found a correlation between GDM and PE in singleton pregnancies, indicating that better GDM management and medication may reduce the occurrence of PE, which is very advantageous to improving pregnancy outcomes. As a consequence, enhancing GDM management and therapy may lower PE occurrence. Table 1 shows the correlated studies among GDM and PE.
Previous research has demonstrated the critical roles that maternal immune regulators, such as the chemokines CXC and CX3CL1 (fractalkine), play in the interaction between PE and their surroundings (Liu et al., 2015; Lei et al., 2019; Szewczyk et al., 2021). Furthermore, Liu et al. (2022) indicated the connection between GDM and CXC chemokines. It is essential to consider these chemokines as potential targets or biological markers in the pathogenic process that precedes the development of PE and GDM (Liu et al., 2015, 2022). According to studies, PE and GDM have high levels of CXC chemokine expression, and there is a direct link between their expression and the pathophysiology of PE and GDM. Therefore, improving PE and GDM patients’ survival rates requires understanding the relationship between these parameters (CXC and CX3CL1), PE, and GDM progression. The roles of the chemokines CXC and CX3CL1 in PE and GDM have been intensively studied; however, the pathogenesis of PE and GDM’s underlying mechanisms is still unknown. Therefore, in this review, we will discuss how CXC and CX3CL1 chemokines affect PE and GDM and how they might be used to treat them.
Chemokines are transcriptional, low-molecular-weight peptides (8–10 kDa) primarily chemotactic for leukocytes (Baird et al., 2011). Based on the number of amino acids between the first and second cysteine residues in the peptide sequence, Chemokines are divided into four subfamilies (C, CC, CXC, and CXXXC) where X is any residue of an amino acid (Zlotnik and Yoshie, 2000). The chemokine family consists of two key subgroups, CXC and CC subtypes (Kawaguchi et al., 2019). CXC chemokines are short secretory proteins containing four highly conserved cysteine amino acid residues and one non-conserved amino acid residue separating the first two cysteines (Taub and Oppenheim, 1994). Depending on whether or not the NH2-terminus contains the Glu-Leu-Arg (ELR) motif, representatives of the CXC chemokine family are classified into two categories: ELR+/ELR-members (Dhawan and Richmond, 2002). The ELR + includes CXCL1 to CXCL3 and CXCL5 to CXCL8 members. The ELR-members are CXCL4, CXCL9 to CXCL14, and CXCL16 (Strieter et al., 1995; Lee et al., 2018; Li et al., 2020b).
In 1997, Bazan et al. (1997), Pan et al. (1997) published the first descriptions of the chemokine CX3CL1. On human chromosome 16, CX3CL1 includes three additional amino-acid residues in addition to the first two cysteine residues. The sole member of the CX3C (delta) subfamily is CX3CL1 (Stievano et al., 2004). Non-hematopoietic chemokine CX3CL1 has two different forms: a transmembrane protein with a long, mucin-like stalk attached to the chemokine domain and a soluble peptide liberated from the cell surface through proteolytic cleavage (Bowen et al., 2002). Human CX3CL1’s soluble chemokine domain attracts monocytes, T cells, and natural killer (NK) cells but not neutrophils. CX3CL1 membrane-bound functions include promoting leukocyte linkage and adhesion and activating target cells. Many inflammatory diseases, including asthma, rheumatoid arthritis, and osteoarthritis, have elevated levels of soluble CX3CL1, which is responsible for leukocyte, NK cell, and T-cell chemotaxis in inflammation. Therefore, in contrast to chemotaxis, the primary function of CX3CL1 is immune system modulation (D’Haese et al., 2012). CX3CL1, the sole endogenous ligand that CX3CR1 binds to, exhibits considerable therapy potential (Lu et al., 2022a). Table 2 displays the leading role of CXC chemokines in the progression and development of PE and GDM.
PE and GDM have inflammation as a defining feature. Numerous studies have shown that inflammation is crucial to the pathogenesis of PE and GDM (Yin et al., 2014; Nguyen-ngo et al., 2020a). Most CXC chemokines are inflammatory (Liong et al., 2018). Fakhr and others (Fakhr et al., 2022) reported that placental villi exhibit a preeclamptic-like phenotype due to the inflammatory mediator TNF-α. Furthermore, a disruption in the nuclear factor kappa B (NF-kB) signaling pathways balance in patients with PE results in aberrant placental trophoblast cell death (Aban et al., 2004; Caballero et al., 2013). A study found that CXCL3/CXCR2 participates in the tumor necrosis factor (TNF) and NF-κB signaling pathway significantly and is downregulated in PE (Rong et al., 2021), which indicated that the abnormal expression of CXCL3 induces decidual stromal cells dysfunction. In addition, Huang and others (Huang et al., 2019) reported that in the presence of excessive proinflammatory stimuli such as TNF-α, interleukin-1 beta (IL-1β), and interferon-gamma (IFN-γ), signaling through mitogen-activated protein kinase 1/2 (MAPK1/2), jun N-terminal kinase (JNK), and NF-kB pathways induces CX3CL1/CXCL10 production (Figure 1). Thus, it is likely that early pregnancy in women results in higher tissue levels of these chemokines, eventually developing PE. In addition, PE vascular smooth muscle cells treated with interleukin-17A (IL-17A) produced more neutrophil-related chemokines, such as CXCL8, CXCL5, and CXCL6. These chemokines cause inflammation (Walsh et al., 2022), which indicates that IL-17A inflammatory stimuli could be responsible for the vascular infiltration of neutrophils in PE. Furthermore, in vitro, Nguyen-ngo et al. (2019), Nguyen-ngo et al. (2022) used human placental and adipose tissue samples. They treated them with TNF and lipopolysaccharides (LPS) to induce a GDM-like environment. TNF and LPS treatment significantly increased the expression of inflammatory CXC chemokines such as CXCL1/5/8/9/10, which indicated that TNF and LPS were inflammatory stimuli that stimulated the proinflammatory chemokines in GDM. Thus, inflammatory stimuli like IL-1-β, TNF-α, IL-1, IFN-γ, and LPS can induce the expression and synthesis of CXC and CX3CL1 chemokines even though these inflammatory chemokines are not constitutively expressed (Pinheiro et al., 2013; Huang et al., 2019; Nguyen-ngo et al., 2022; Walsh et al., 2022). However, as shown in Table 3, a single stimulus may only impact a small number of particular chemokines.
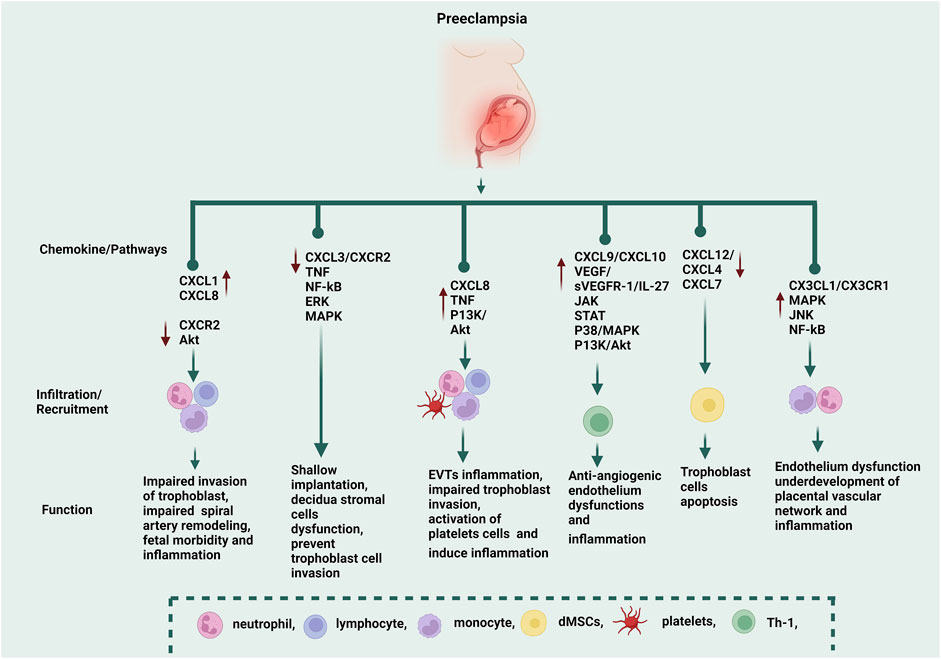
FIGURE 1. The functions of CXC and CX3CL1 chemokines in the progression of PE. CXCL1 and CXCL8 recruit neutrophils, monocytes, and lymphocytes, while CXCR2 is downregulated during PE via the AKT pathway, and these CXC chemokines induce impaired invasion of the trophoblast, impaired spiral artery remodeling, fetal morbidity, and inflammation. Through the TNF, NF-kB, ERK, and MAPK pathways, CXCL3/CXCR2 causes shallow implantation, decidua stromal cell dysfunction, and trophoblast cell invasion. CXCL8 recruits neutrophils, lymphocytes, monocytes, and platelets via TNF and P13K/Akt pathways, which induce extravillous trophoblasts (EVTs) inflammation, impaired trophoblast invasion, and activation of platelet cells (which induce inflammation). CXCL9 and CXCL10 are anti-angiogenic chemokines. CXCL10 recruits Th-1 via the IL-27/JAK, P38/MAPK, P13K, and Akt pathways, which induce inflammation. CXC10 is correlated to vascular growth factor (VEGF/sVEGFR-1), and the excessive secretion of these molecules induces endothelium dysfunction. CXCL12, CXCR4, and CXCR7 recruit decidual mesenchymal stem cells (dMSCs), which induce trophoblast cell apoptosis. CX3CL1/CX3CR1 recruits monocytes and neutrophils via MAPK, JNK, and NF-kB pathways, which induce endothelium dysfunction and inflammation. CX3CL1 was negatively correlated to the vascular/extravascular tissue index, which indicated that CX3CL1 was involved in the underdevelopment of the placental vascular network. ↑ The red arrow symbol shows upregulation, while the ↓ arrow shows downregulation.
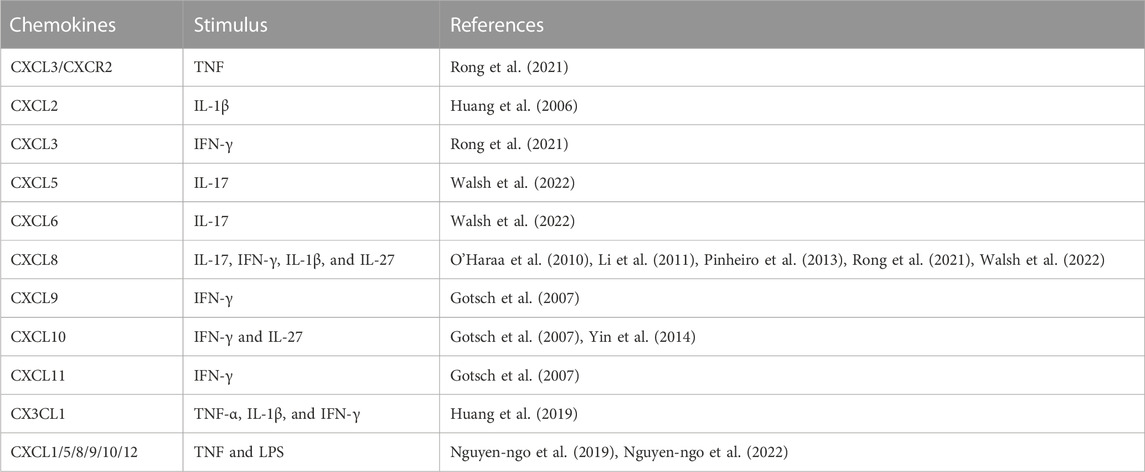
TABLE 3. Specificity of the stimulus for CXC and CX3CL1 chemokines expression and synthesis during PE and GDM.
Successful pregnancy and health depend on the homeostatic balance between systemic and local pro- and anti-angiogenic factors (Rana et al., 2012). In addition, numerous studies have demonstrated that the pathophysiology of PE depends on the appropriate balance between angiogenic and antiangiogenic mediators (Molvarec et al., 2010). Vascular endothelial growth factor (VEGF), which has six isoforms—VEGF-A, B, C, D, and placental growth factor (PlGF)—is the primary regulator of angiogenesis (Heinke et al., 2012). These mediators bind to and activate VEGFR-1, 2, and 3 (Karaman et al., 2018). The binding patterns of VEGF-A and VEGFR-2, VEGF-B, and PlGF are specific to VEGFR-1, while VEGF-C and VEGF-D bind to VEGFR-3 and impact the lymphangiogenic pathway (Karaman et al., 2018).
Zhang et al. (2019) reported that increased tumor growth factor-beta (TGF-β) levels from decidual Treg cells inhibited dNK cell function by decreasing IFN-γ/CXCL8/CD107a expressions. They found considerable positive relationships between IFN-γ and CD107a expression in dNK during preterm and term pregnancy but not in PE. PE had a strong negative connection between VEGF and CD107a expression, showing that dNK’s angiogenic and cytotoxic capabilities are conditionally regulated during pregnancy. Additionally, there is a correlation between GDM-PE and VEGF expression in clinical studies. Compared to the standard group, the GDM-PE group has different levels of VEGF and receptors. Moreover, the PE and GDM-PE groups had higher levels of fms-like tyrosine kinase-1 (FLT-1) expression (Dong, 2019; Krishnasamy et al., 2019; Sugimoto et al., 2019; Ren et al., 2020; Al-Ofi et al., 2021; Alqudah et al., 2021; Atallah et al., 2021; Bolatai et al., 2022). These results indicated that the upregulation of VEGF/FLT-1 in GDM-PE induces endothelium dysfunction. Thus, we consider VEGF and its receptors linked to these two pregnancy problems. The two diseases may be correlated because endothelial damage impacts VEGF and its receptor in GDM and PE. Together, both diseases have comparable cumulative risks. VEGF and its receptor alterations cause aberrant blood vessel proliferation and persistent hypoxia.
It is believed that VEGF imbalance precedes maternal-fetal complement progression. PE is caused by maternal pathogenesis preceding the placenta (Ying and Hongyan, 2020; Liu et al., 2021). VEGF antagonistic receptor irregularities are typically the primary symptom of a predisposition to PE. This receptor is often called soluble FLT-1 (Cindrova-Davies et al., 2011). It is one of the direct causes of the symptomatic proteinuria and hypertension that accompany PE in pregnancy. In general, an increase in sFLT-1 will combine with other endothelial factors to hinder binding, diminish normal placental villi infiltration and the capacity to stimulate vascular growth, and worsen vascular activity throughout the PE process during pregnancy. As a result, alterations in VEGF and its associated tyrosine kinase receptors can be observed in pregnancy problems (Bolatai et al., 2022). However, more case data is needed to demonstrate the link between VEGF and its receptors and GDM and PE mentioned in earlier studies.
Several studies have linked PE to higher levels of inflammatory signals and lower levels of immune regulatory markers. A Mexican study found significantly lower levels of regulatory T cells (Tregs) in the serum of women with varying degrees of PE severity. Increased levels of inflammatory signals such as interleukin-6 (IL-6), TNF-α, and interleukin-8 (IL-8), as well as anti-angiogenesis chemokines such as CXCL10 and CXCL9, were found among those with severe PE (Moreno-eutimio et al., 2014). In addition, Gotsch et al. (2007) also noted that CXCL10 exhibits anti-angiogenic behaviors, which are hallmarks of PE. They suggest that increased maternal serum concentrations of CXCL10 could contribute to establishing an anti-angiogenic state when combined with sVEGFR-1 because the anti-angiogenic effects of the serum from pregnant women with PE can be reversed in the endothelial cell tube formation bioassay whenever VEGF and PIGF are administered (Maynard et al., 2003). The administration of sVEGFR-1 to pregnant animals can also result in clinical symptoms of PE, such as proteinuria and hypertension (Maynard et al., 2003). Therefore, the authors speculate that increased maternal CXCL10 concentrations, in conjunction with sVEGF-R1, may aid in the establishment of an anti-angiogenic state in the context of their finding that CXCL10 exhibits anti-angiogenic properties, which is a unique feature of PE (Figure 1) (Gotsch et al., 2007).
Dijmărescu et al. (2021) describe the angiogenic and anti-angiogenic factors as helpful indicators for PE diagnosis and prognosis. Compared to the mild PE group, VEGF and CXCL10 concentrations in the severe PE group showed a stronger correlation with indices. The serum levels of CXCL10 were statistically higher in the severe PE group than in the mild PE group, demonstrating a substantial association between VEGF and CXCL10. The results showed that VEGF and CXCL10 were the biomarkers that best corresponded with indices that assessed the severity of PE.
CX3CL1 can promote integrin-dependent trophoblast migration and induce angiogenesis through the VEGF/hypoxia-inducible factor-1 alpha (HIF-1α) pathway (Hannan et al., 2006; Ryu et al., 2008; Szukiewicz et al., 2014). There is evidence that angiotensin II deregulation of CX3CL1 occurs in early-onset PE. As a result, it may play a role in the proinflammatory trophoblast-monocyte interaction (Siwetz et al., 2015; Nonn et al., 2019). Szewczyk et al. (2021) discovered considerably higher CX3CL1 serum levels and placental expression in PE patients and a negative correlation with the vascular/extravascular tissue index. The authors postulated that severe underdevelopment of the placental vascular network in PE is associated with alterations in CX3CL1 expression and distribution.
2 Role of CXC chemokines in the pathogenesis of PE
2.1 CXCL1/CXCR2
The secretion of CXC chemokines, such as CXCL1 and CXCL8, was dramatically increased when placental trophoblast cells were exposed to high doses of glucose (25 and 50 mM) (Han et al., 2015). These findings suggested that first-trimester trophoblast cells were in a pro-inflammatory state because of the excessive glucose deposition. These data show the trophoblast’s pleiotropic response to hyperglycemia, which may help to explain the strong association between diabetes and PE.
Due to reduced trophoblast invasion and aberrant vascular remodeling, it may prolong placental oxidative stress, which in turn may exacerbate the dysfunction of trophoblasts that is a defining feature of PE (Anton et al., 2013; Fu et al., 2013). The research also demonstrated that IL-1β induces CXCL1 expression, which aids trophoblast invasion (Baston-buest et al., 2017). In addition, microRNAs (miRNAs) are small, non-coding, single-stranded RNA that regulate gene expression at the post-transcriptional and transcriptional levels to a lesser extent (O’Brien et al., 2018). Hayder et al. (2021) recently revealed that overexpression of miR-210-3p lowered the mRNA levels of CXCL1 and CXCL8. These chemokines may invade extravillous trophoblasts (EVT) and enlist immune cells at the sites where the spiral arteries are remodeling during PE. These results imply that overexpression of miR-210-3p could result in impaired maternal spiral artery remodeling, which would contribute to the pathogenesis of PE, or that miR-210-3p regulates spiral artery remodeling events by inhibiting the expression of these chemokines (CXCL1 and CXCL8).
Neonatal patients, especially preterm newborns, have poor chemotaxis, which may be a factor in their increased risk of sepsis. Two chemokines are essential for leukocyte movement: CXCL1 and CXCL8 (Greer et al., 1989; Sullivan et al., 2002). Following neutrophil, monocyte, and leukocyte activation, plasma levels of CXCL1 and CXCL8 rise, which operate as immune system modulating factors (Mellembakken et al., 2001a, 2001b; Faulhaber et al., 2011). During PE, the numerous activations of chemokines (CXCL1 and CXCL8), which turn on neutrophils, monocytes, and leukocytes in the fetus, may affect fetal morbidity. In addition, the CXCL1 methylated gene is implicated in the pathogenesis of PE (Jia et al., 2012).
Wu et al. (2016) observed that CXCR2 mRNA and protein expression levels were considerably lower in PE placentas than in normal controls. The authors also showed that low levels of CXCR2 in the placenta may contribute to the pathogenesis of PE via downregulation of matrix metalloproteinase-2 (MMP-2) and matrix metalloproteinase-9 (MMP-9) via the Akt pathway, which impairs the invasion of the trophoblast (Wu et al., 2016). These results suggest that lower levels of CXCR2, which inhibit trophoblast invasion by decreasing MMP-2 and 9, may play a role in the development of PE. Furthermore, recent studies demonstrated significant abnormalities/downregulated in CXCR2 expression during PE (Wang et al., 2019b; Meeuwsen et al., 2020; Zhang et al., 2020). Further research elucidating the pathways that involve CXCR2 signaling, such as the Akt pathway, may provide new knowledge on the impact of CXCR2 in PE patients.
2.2 CXCL2
In a recent study, Ma et al. (2023) extracted decidual stromal cells from PE patient tissue and transfected them with miR-455-3p inhibitors to study their function. The study discovered that miR-455-3p inhibitor transfection raised CXC chemokines such as CXCL2, CXCL3, and CXCL8. The translation level was also examined by assessing CXC chemokine expression in decidual stromal cell-derived culture media (CM). Transfection of miR-455–3p inhibitors increased CXCL2, CXCL3, and CXCL8 expression in decidual stromal cells-derived CM. These findings demonstrate that the downregulation of miR-455-3p enhances CXCL2, CXCL3, and CXCL8 production in decidual stromal cells. In addition, Zhou et al. (2023) revealed that in PE decidual stromal cells, downregulation of miR-92a enhances the production of CXCL2, CXCL3, and CXCL8. Interferon regulatory factor-3 (IRF3) may control the levels of CXC chemokines like CXCL2, CXCL3, and CXCL8 in the decidual stromal cells of PE directly or indirectly. miR-92 may bind to the 3′UTR of IRF3, promote its downregulation, and eventually regulate the secretion of CXC chemokines in these cells. These CXC chemokines are overexpressed in PE patients, which causes inflammation. Thus, the latest studies indicated that miRNAs play a vital role in the pathophysiology of PE in decidual stromal cells.
CXCL2, responsible for monocyte and macrophage chemoattraction and activation, was also elevated in PE placental tissue. According to a study, IL-1 dramatically increases CXCL2 mRNA and protein expression in first-trimester decidual cells (Huang et al., 2006). Likewise, references indicated that CXCL2 is upregulated in PE patients and the blood pressure high/5 (BPH/5) female mouse model of PE (Yan et al., 2013; Batts et al., 2021). Interestingly, Johnston and others (Johnston et al., 2021) observed that CXCL2 mRNA expression was considerably lower in PE hepatic transaminases low platelets (HELLP) mouse model syndrome. The decreasing trend of CXCL2 during late pregnancy is unknown. To determine if these inflammatory mediators’ expression changes during normal gestation, comparing these mouse strains to other mouse strains or whether the BPH/5 female mouse represents a model of fatty liver disease associated with pregnancy rather than HELLP syndrome will be necessary.
2.3 CXCL3/CXCR2
Another crucial proinflammatory cytokine, CXCL3, attracts neutrophils and macrophages, which aids in developing inflammation-related colorectal adenoma (Payne and Cornelius, 2002). CXCL3 attracts and activates CXCR1 and CXCR2 receptors, which are present on the surface of endothelial, epithelial, and colloid cells. Consequently, this is crucial for the natural development of inflammation and angiogenesis (Russo et al., 2014). Coincidentally, these modules are also very closely related to the emergence of PE. More importantly, a previous study found that exogenous CXCL3 protein may boost trophoblast migration and proliferation, and placental CXCL3 levels in the severe PE group were significantly lower than in the mild PE and regular groups, suggesting that CXCL3 is pathologically linked to severe PE (Wang et al., 2018). Plasma CXCL3 levels and placental CXCL3 expression significantly differed in women with severe PE. CXCL3 had a role in trophoblast invasion, which showed that it was involved in shallow implantation (Gui et al., 2014). The placental expression levels of CXCL3, which are detected in STBs and vascular endothelium, were lowered in cases of severe PE. In vitro, HTR-8/SV-Neo cell invasion and proliferation were both induced by exogenous CXCL3. In addition, a recent bioinformatics study revealed that CXCL3/CXCR2 are involved in the TNF signaling and the NF-kB signaling pathway and that CXCL3 is strongly expressed in normal decidual macrophages but dramatically downregulated in PE patients (Rong et al., 2021), as a result, the aberrant expression of these differentially expressed genes (DEGs) (CXCL3) has an impact on the dysfunction of the decidual stromal cells, which revealed the interactions between immune cells in the decidua.
Additionally, Liao et al. (2022) discovered that demethylation (TARID), a new long non-coding RNA (lncRNA) linked to PE, plays a part in the onset and progression of PE by modulating the CXCL3, ERK, or MAPK pathways. TARID stimulates trophoblast movement, invasion, and tube formation. The downregulation of TARID expression during PE mediates the CXCL3, extracellular signal-regulated kinase (ERK), or MAPK pathways, which may prevent trophoblast invasion and spiral artery remodeling by impairing cell growth, invasion, and tube formation (Liao et al., 2022).
2.4 CXCL6
A recent bioinformatic study found that the hub gene (most closely associated with disease) CXCL6 functions as a PE biomarker and prediction model in patients (Lin et al., 2022). MicroRNA (MiR-101) was shown to be considerably reduced in PE placentas and was found to be involved in the regulation of several apoptotic proteins under endoplasmic reticulum stress (Zou et al., 2014). MiR-101 also suppresses trophoblast HTR-8/SVneo cell migration and invasion by targeting CXCL6 in PE (Zhang et al., 2021).
The Y153H, C allele of the transcription factor STOX1 (STORKHEAD-BOX1 PROTEIN 1) has been associated with the early utero-vascular etiology of placental diseases as well as the onset or progression of PE (Pinarbasi et al., 2020; Dunk et al., 2021). A subsequent study by the same group raises the possibility that the STOX1 Tyr153His mutation in the extravillous trophoblast may contribute to preterm birth and PE. Utero-vascular remodeling is a compromised process that preterm birth and PE share (Dunk et al., 2021). The researchers used primary EVT explant, placental decidual coculture models, and EVT-like cell lines to transfect the variant for functional validation. EVT and EVT-like cells transfected with the STOX1 Tyr153His mutation released lower IL-6 and IL-8 but higher levels of CXCL6 and tumor necrosis factor-related apoptosis-inducing ligand (TRAIL) than control wild-type EVT cells and EVT-like cells. Some of these genes, like CXCL6, were higher in the endothelial cells of mice that overexpressed STOX1A. They were linked to inflammation and cellular stress (Miralles et al., 2019), indicating STOX1A’s functional significance in the development of PE.
2.5 CXCL8
In a bioinformatic study, the Kyoto Encyclopedia of Genes and Genomes (KEGG) pathway enrichment analysis revealed that CXCL8 was upregulated via phosphatidylinositol 3-kinase (PI3K)/protein kinase B (AKT) signaling pathways in PE, which might assist inflammation and impaired trophoblast invasion during PE (Jiang et al., 2020). In addition, interferon-stimulated gene 15 (ISG15) serves as a crucial regulator of proinflammatory cytokine expression in EVTs during PE, and HTR8/SVneo cells with silencing of ISG15 had elevated levels of CXCL8 mRNA (Ozmen et al., 2022).
TNF-α stimulates the release of CXCL8 and other proinflammatory cytokines by endothelial cells (O’Haraa et al., 2010). There is evidence that TNF-α levels in maternal serum from PE-complicated pregnancies are higher than in normotensive pregnancies (Szarka et al., 2010). Shaw and others (Shaw et al., 2016) found that TNF-α was a significant factor in endothelial cells releasing and activating the proinflammatory cytokine CXCL8. This suggests that blocking TNF activity could help reduce the effects of maternal endothelial dysfunction in PE.
During the development and progression of PE, platelets may promote blood coagulation and initiate inflammation. Platelets may also play a role in the inflammatory response in PE (Wang et al., 2017). However, in preeclamptic women, the degree of inflammation is related to the degree of platelet activation. This tends to be linked to processes that alter cytokine levels, including CXCL8 (Sahin et al., 2014).
Pinheiro and his team discovered that women with severe PE had more CXCL8 in their blood than pregnant women with normal blood pressure. In the same study, they discovered a significant correlation between CXCL8 and IFN-γ in severely preeclamptic individuals and postulated that this could play a role in the pathophysiology of PE (Pinheiro et al., 2013). Alteration in chemokine levels, such as CXCL8, may, in our opinion, directly impact the endothelium of the maternal systemic vasculature. Furthermore, studies found higher CXCL8 serum concentrations in PE patients, implying that CXCL8 plays a role in the pathogenesis of PE (Gigraci et al., 2010; Garcia et al., 2018; Valencia-Ortega et al., 2019). Hence, targeting CXCL8 through developing PE therapeutic techniques may result in significant clinical applications in PE. CXCL8 may function as a biomarker for PE patients.
2.6 CXCL9, CXCL10, and CXCL11
CXCL9, CXCL10, and CXCL11 mRNA expression in the chorioamniotic membranes from PE was also higher in chronic chorioamnionitis cases than in those without chronic chorioamnionitis (Kim et al., 2010). Another comparative study reported that CXCL9 and CXCL10 were associated with inflammation in PE patients, which were found to be increased in PE patients (Brien et al., 2020).
IFN-γ-induced Th1 cytokine milieu also releases these CXC chemokines (Gotsch et al., 2007). CXCL10 and CXCL11 appear to be part of a Th1-like inflammatory response in PE, with CXCL10 potentially more reliable as a biomarker in PE patients (Boij et al., 2012).
According to Yin et al. (2014), IL-27 may have a critical role in PE. IL-27 was reported to increase CXCL10 expression in trophoblastic cells via activating the Janus kinase/signal transducers and activators of transcription (JAK/STAT), p38 MAPK, and PI3K-Akt signaling pathways (Figure 1). Understanding and treating PE might improve through elucidating the connections between IL-27 and CXCL10. Prior research has shown that women with PE have higher concentrations of CXCL10 and CXCL9 (Oliveira et al., 2011; Haedersdal et al., 2013; Chedraui et al., 2015; Kalinderis and Papanikolaou, 2015; Darakhshan et al., 2019b; Li et al., 2020a; Dijmărescu et al., 2021).
2.7 CXCL12 and CXCL16
Regarding the function of CXC12, CXCR4, and CXCR7 in PE, reports are inconsistent. Prior studies have documented elevated levels of CXCL12 in the placenta of patients with PE compared to the average control population (Schanz et al., 2011; Hwang et al., 2012). Nevertheless, several studies have demonstrated that the placentas of patients with severe PE have downregulated levels of CXCL12 and its receptors, CXCR4 and CXCR7 (Kim et al., 2014; Lu et al., 2016). CXCL12 has also been shown in further investigations to reduce the apoptosis rate of term trophoblast cells (Lu et al., 2016, 2020). Therefore, the downregulation of CXC12, CXCR4, and CXCR7 may interfere with trophoblast apoptosis and contribute to the development of severe PE. Interestingly, pregnant women with PE had greater levels of CXCL12 in their mid-trimester amniotic fluid; however, the precise mechanism is unknown (Tseng et al., 2009). Moreover, CXCL12/CXCR4 signaling influences several disorders linked to pregnancy, especially PE (Ao et al., 2020).
It has been demonstrated that bone morphogenetic protein 9 (BMP9) regulates the processes involved in endothelial dysfunction (Long et al., 2015). Yang and others (Yang et al., 2023) revealed that serum from PE patients had lower levels of CXCL12 and CXCR4 expression and that there was a positive correlation between BMP9 and these expression levels. In HTR-8/SVneo cells, overexpression of BMP9 increased the levels of CXCL12 and CXCR4. By activating the CXCL12/CXCR4 pathway, BMP9 promoted the migration and invasion of HTR-8/SVneo cells and inhibited apoptosis. It seems that BMP9 might be a biomarker for PE because it controls the CXCL12/CXCR4 axis. Lei et al. (2019) found that the CXCL12/CXCR4 axis can mediate the migration behavior of decidua-derived mesenchymal stem cells (dMSCs). dMSCs can migrate to the decidua layer along a CXCL12 concentration gradient in patients with PE, suggesting that it may play a role in the development and progression of PE. Finally, these data imply that the CXCL12/CXCR7/CXCR4 axis may be a critical molecular insight into PE that needs further investigation. Remarkably, in PE, the chemokines also exhibit their effects on decidual immune cells (Valencia-Ortega et al., 2019).
In STOX1 mice with PE, Miralles et al. (2019) examined the cytokine plasma profile and discovered aberrant CXCL16 expression. However, findings showed that CXCL16/CXCR6 expression was similar in PE patients and average pregnant women (tested at 35 weeks) (Schanz et al., 2011). One possible explanation for these conflicting results is that the tests were conducted at various gestational weeks. In addition, CXCL16 levels in the blood increased considerably in PE women at weeks 22–24, 30-32, and 36–38. In contrast, peripheral blood mononuclear cells (PBMC) mRNA expression of CXCL16 decreased (Lekva et al., 2017). Furthermore, numerous studies showed that the CXCL16 levels are significantly greater in PE patients (Estensen et al., 2015; Panagodage et al., 2016; Tok et al., 2019; Shi et al., 2020; Li et al., 2021). To date, no study has directly reported that aberrant CXCL16 chemokine expression is the underlying etiology of PE. These findings, taken together, demonstrate aberrant CXCL16 expression in the PBMC of PE patients; however, CXCL16 expression at the maternal-fetal interface during PE has not yet been investigated. Therefore, the fundamental mechanisms remain unknown. CXCL16 is overexpressed at the maternal-fetal interface and has biological processes, such as promoting trophoblast invasion (Zhang et al., 2022a). PE can be induced by inadequate trophoblast invasion and uterine spiral artery remodeling (Wang et al., 2020a). Hence, it is feasible that abnormal CXCL16 expression is connected to PE. However, further reliability and validity research are needed to reveal the specific mechanism.
3 Role of CX3CL1 chemokine in the pathogenesis of PE
Previous studies showing the role of CX3CL1 in the feto-maternal interaction in the process of placental invasion during PE indicated that CX3CL1 may be associated with missed abortion (Hannan et al., 2014; Usta et al., 2018). In a recent study, significantly increased content of CX3CR1+ cells in the decidua of PE patients and an increase of CX3CR1-positive cells may related to colonization of the decidua by monocytes (CX3CR1 was used as a marker of the macrophages of monocytic origin) attracted by inflammatory signals from circulation (Vishnyakova et al., 2021). These results reflect profound PE-associated alterations in the decidua, including the pronounced endothelial dysfunction attraction of the CX3CR1-positive effector cells.
According to Kudryashova et al. (2019), there is a correlation between low levels of CD62L+, CD99+, CXCR1+ neutrophils, serum CXCL8, and adversely affected blood vessel elastic properties. In addition, another study found that CX3CR1+ monocyte and neutrophil levels were higher in PE patients (Panova et al., 2018). These findings clarified that, possibly, in PE, activated endothelial cells themselves regulate how tightly neutrophils adhere to the endothelium. One of the main mechanisms of inflammation is the interaction between the chemokine receptors expressed by the cells and the chemokines produced in the affected area.
The above studies show that CX3CL1 acts as an inflammatory mediator in various cell types involved in PE. It remains to be seen whether CX3CL1 is required for human inflammatory cell survival. Finally, several lines of evidence point out that CX3CL1 is another player in the pathogenesis of PE that could be a valuable therapeutic target. A better understanding of the mechanism and timing of CX3CL1 actions may assist with determining the kind of PE pathology that should be targeted therapeutically. The chemokines CXC and CX3CL1 regulate the onset and progression of PE through several particular pathways, shown in Figure 1.
4 Role of CXCLs in the pathogenesis of GDM
4.1 CXCL1 and GDM
In a human study, serum levels of angiogenic (CXCL1 and CXCL12) were higher in gestational diabetes mellitus mothers (GDMM). Interestingly, newborns delivered by GDMM had higher levels of CXCL1 and CXCL12 but lower levels of angiostasis (CXCL9 and CXCL10) (Darakhshan et al., 2019a). This study speculated that increased angiogenesis chemokines (CXCL1 and CXCL12) might be involved in endothelium damage in GDM patients. In addition, a recent study revealed GDM exposure in male and female offspring mice. Whereas female offspring-specific elevated levels of proinflammatory and neutrophil chemoattractants chemokine and cytokine (CXCL1 and IL-1β) in lung lavage (Pascoe et al., 2022). Due to elevated neutrophil chemoattractants (CXCL1, IL-β), this allergen exposure may result in a rise in neutrophil recruitment to the lung. These proinflammatory changes could make female offspring of GDM mothers more susceptible to lung damage and inflammation triggered by neutrophils after allergen inhalation, which could result in asthma. Furthermore, a bioinformatic study found that T1D, T2D, and GDM patients have DEGs CXC chemokines (CXCL1, 2, 3, 5, 10, and 12) and receptor CXCR4 (Evangelista et al., 2014). This suggests that the prediction of GDM, prior to its onset, should be based on multiple markers (CXC chemokines) rather than a single marker.
4.2 CXCL8 and GDM
Human chorionic membrane-derived stem cells (CMSCs) derived from GDM patients exhibit high levels of inflammatory indicators such as CXCL8. According to Chen et al. (2019). CXCL8 was crucial to the inflammatory process during GDM. In addition, Li et al. (2020c) recently reported that GDM women exhibited an increased risk of neonatal infection because of an abnormal placenta. Further, the high-glucose environment activated the toll-like receptor 4 (TLR4)/myeloid differentiation primary response 88 (MyD88)/NF-kB pathway in GDM placentas. It induced the secretion of the CXCL8/IL-8. Inflammation caused by GDM breaks the homeostasis in the placenta, and autophagy balance is also destroyed by maintaining cell self-renewal and homeostasis. Autophagy was increased in GDM compared with ordinary women (Li et al., 2020c). Furthermore, Zhang and others (Zhang et al., 2017) also reported that inflammatory biomarkers, including NF-kB and CXCL8, had significant differences between GDM and healthy pregnancies. This phenomenon showed that the placentas of GDM women were in an inflammatory environment. The increased inflammatory mediators might promote intraplacental inflammatory cascades by specific gene transcription or translation.
GDM increases cancer risk in children. Bioinformatic analysis showed that cancer-related pathways, such as those in cancer and acute myeloid leukemia, were significantly enriched in umbilical cord blood mononuclear cells from GDM patients. CXCL8 was the top DEG between GDM and controls. The authors found only monocyte and granulocyte DEGs enriched in the cancer pathway, with CXCL8 being the common DEG between GDM and control groups. In GDM, the number of CXCL8+ IL1B + monocytes and CD16+ granulocytes increased. In the high-glucose stimulation experiment, CXCL8+IL1B + monocytes also increased significantly. These findings suggest that maternal GDM reprograms fetal monocytes and granulocytes with high CXCL8 expression, which could boost teenage cancer risk (Yin et al., 2022).
4.3 CXCL9, CXCL10, and GDM
The bioinformatic study revealed that CXCL9/10 was significantly enriched in the TLR signaling pathway, leading to speculation that it is a crucial gene that participates in the pathogenesis of GDM by regulating the progress of the TLR signaling pathway and may thus play a critical role in the pathogenesis of GDM by regulating the inflammatory pathway (Wang et al., 2019a). In addition, studies reported that CXCL10 is upregulation associated with the birth of a macrosomic child. Together, these results suggest that CXCL10 could be used as a predictive marker for pregnancy complications in GDM (Pan et al., 2021a; Tagoma et al., 2022).
4.4 CXCL16 and GDM
Lekva et al. (2017) showed that in the early stages of pregnancy, the levels of CXCL16 mRNA in PBMC were lower than in healthy pregnant women. On the other hand, the levels of CXCL16 in the blood of GDM patients were higher. Furthermore, apolipoprotein B (apoB) and low-density lipoprotein cholesterol (LDL-C) positively correlated with increased levels of CXCL16. CXCL16 also works as a scavenger receptor on macrophages, boosting LDL-C oxidation and internalization (Barlic et al., 2009), which may play an important role in the inflammatory response due to lipid accumulation. Therefore, the elevation in circulatory levels of CXCL16 in patients with GDM may be associated with abnormal lipid metabolism/dyslipidemia, although the specific mechanism remains unclear. Further studies are required to explain how the expression of CXCL16 mRNA in the PBMCs of patients with GDM decreases at a certain point during pregnancy.
5 Role of CX3CL1 in the pathogenesis of GDM
According to array gene expression analysis, CX3CL1/CX3CR1 was consistently markedly elevated in human endothelial cells in hyperglycemia status (Schiano et al., 2019). In GDM, higher levels of CX3CL1/CX3CR1 in the placenta are linked to increased insulin resistance (IR) and an inflammatory response. This is because circulating CX3CL1 in GDM can correlate to IR markers and proinflammatory progranulin during pregnancy (Ebert et al., 2014; Schiano et al., 2019).
TLRs recognize maintained molecular patterns and play a critical role in regulating innate immune responses and inflammation by activating inflammatory mediators such as the inflammatory transcription factor NF-kB (Medzhitov, 2001). There are increased levels of TLR2 and TLR4 in PBMC from women with GDM (Kuzmicki et al., 2013). TLR activation may play a role in the proinflammatory consequences of high glucose since it significantly increases the expression of TLR2 and TLR4 in human monocytes, which in turn activates NF-kB and secretes more proinflammatory chemokines, such as CX3CL1 (Kuzmicki et al., 2013).
In the above studies, GDM patients had altered CXC and CX3CL1 chemokines. This review study summarized the most recent studies on the CXC and CX3CL1 chemokine molecular mechanisms that cause GDM pathophysiology. This enabled insights into future difficulties and possibilities for distinct clinical and therapeutic properties and consequences for GDM. The crucial role of CXC and CX3CL1 chemokines via various pathways involved in the onset and progression of GDM is illustrated in Figure 2.
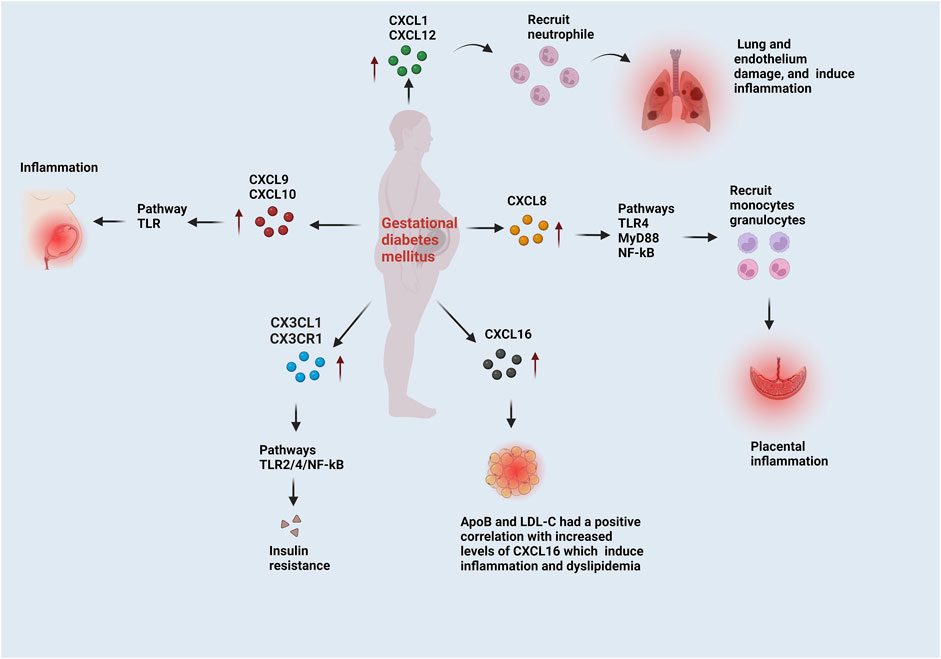
FIGURE 2. The functions of CXC and CX3CL1 chemokines in the progression of GDM. CXCL1 and CXCL12 recruit neutrophils, which induce lung and endothelium damage and inflammation. CXCL8 follows the TLR4/MyD88/NF-kB pathways and recruit’s monocytes and granulocytes, which induce placental inflammation. CXCL9 and CXCL10 induce inflammation via the TRL pathway. Adipose tissue biomarkers, like low-density lipoprotein cholesterol (LDL-C) and apolipoprotein B (ApoB), were correlated to CXCL6. These biomarkers are involved in dyslipidemia, a problem with adipose tissue. The CX3CL1/CX3CR1 is correlated to the insulin resistance markers. In GDM, CX3CL1/CX3CR1 leads to insulin resistance through the TLR2/4 and NF-kB pathways.
6 Other hypertension-related disorders
6.1 Cardiovascular disease (CVD)
There is a documented clinically significant correlation between elevated blood pressure and cardiovascular disease (CVD) risk, including atherosclerosis and cardiac fibrosis (Perumareddi, 2019; Fuchs and Whelton, 2020). Lu et al. (2022b) reported that CXC chemokines play a vital role in the progression and development of atherosclerosis and cardiofibrosis. However, some studies reported that some CXC chemokines work as anti-CVD.
Research has indicated a negative relationship between coronary artery disease (CAD) severity and CXCL5 plasma levels. Furthermore, several aggregates of CXCL5 and CXCR2 were found in coronary atherosclerotic plaques, indicating a potential protective function of CXCL5 against CAD (Ravi et al., 2017). In addition, animal studies have demonstrated that Apoe−/− mice with CXCL5 inhibition accumulate foam cells and macrophages in atherosclerotic plaques and decrease collagen content. This finding suggests that CXCL5 could reduce the progression of atherosclerosis by preventing macrophage accumulation and foam cell formation (Rousselle et al., 2013).
Considering the substantial evidence suggesting CXCL12 had a preventive function in atherosclerosis when Apoe−/− mice were given intravenous CXCL12. Akhtar et al. (2013) noticed that although the lesion size did not alter considerably, the fibrous cap of the diseased plaques in the mice thickened, and smooth muscle cells increased. In addition, plasma CXCL12 levels were lower in advanced atherosclerotic mice than in normal mice, CAD patients, and healthy individuals. This implies that CXCL12 might have actions that prevent atherosclerosis (Damås et al., 2002; Xu et al., 2011). Furthermore, CXCL16 works as an oxLDL clearance receptor that fights atherosclerosis, and phagocytosis of oxLDL by macrophages was reduced in CXCL16-deficient animals. CXCL16−/−/LDLR−/− mice developed accelerated atherosclerotic lesions. This evidence supports CXCL16’s antiatherosclerotic properties (Aslanian and Charo, 2006).
CXCL8 is pro-inflammatory in cardiac fibrosis, and pursuing MI-elevated CXCL9 expression stimulates fibroblast migration and proliferation. In contrast to CXCL9, CXCL10 can prevent fibroblast migration (Dobaczewski and Frangogiannis, 2009; Turner et al., 2011; Lin et al., 2019). The anti-fibrotic properties of CXCL10 and its receptor, CXCR3, prevent fibroblast migration. It may additionally play a role in lymphocyte recruitment (Frangogiannis et al., 2001; Bujak et al., 2009; Saxena et al., 2014; Li and Frangogiannis, 2021). A study found that CXCR4 antagonists could prevent cardiofibrosis in mice with type I and type II diabetes. Furthermore, CXCL12 therapy causes the proliferation and hypertrophy of rodents’ cardiac fibroblasts (CFs) and promotes CF collagen production. This outcome was more significant in hypertension models (Jackson et al., 2017; Wang et al., 2020b). Moreover, CXCL12 may promote repair and delay cardiac fibrosis. Inhibiting the scavenger receptor, CXCR7 caused the mice’s fibrosis process to slow down significantly (Chu et al., 2015). CXCL12 may promote cardiac fibrosis by attracting inflammatory cells that express CXCR4, which, in response, induces local inflammation and CF activation (Menhaji-Klotz et al., 2018).
CX3CL1 is rapidly generated following myocardial damage and chemoattracts monocytes and macrophages that express the CX3CR1 receptor (Boag et al., 2015). Since macrophages play an essential role in tissue fibrosis, it has been postulated that CX3CL1 plays a significant role in fibrotic remodeling. In vivo research on the CX3CL1/CX3CR1 axis’s function in fibrosis revealed that CX3CL1/CX3CR1 signaling prevents macrophage-driven fibrogenesis in a hepatic fibrosis model (Karlmark et al., 2010). While CX3CR1+ macrophages are abundant in the remodeling and infarcted myocardium (Nahrendorf et al., 2007), the significance of CX3CL1/CX3CL1 in cardiac fibrosis has not been systematically investigated. Furthermore, in a model of unilateral nephrectomy followed by angiotensin II infusion, CX3CR1 deletion did not affect myocardial fibrosis (Ahadzadeh et al., 2018).
6.2 Diabetes mellitus
Our recent comprehensive review study demonstrated that most of the CXC chemokines family members are upregulated during diabetes and play a vital role in the development and progression of diabetes (Ullah et al., 2023). Furthermore, numerous cell types, including fibroblasts, endothelial cells, and monocytes, release CXCL10. Patients with type 2 diabetes mellitus (T2DM) have been found to have higher levels of CXCL10 in meta-analysis research (Pan et al., 2021b). This is feasible because CXCL10 interacts with the chemokine receptor CXCR3 and TLR4 (Schulthess et al., 2009; Sajadi et al., 2013). Furthermore, CXCL10 has been linked to other processes, including stimulating T-lymphocytes and monocytes/macrophages. Additionally, clinical research has demonstrated that T2DM patients release greater levels of CXCL10 than the control group (Sajadi et al., 2013). Therefore, the interferon gamma-inducible CXCL10 is consequently believed to be a significant factor in initiating the death of b cells. CXCL10 can also reduce the viability of B cells and hinder insulin secretion. The particular process may involve the ability of the b cells stimulated by CXCL10 to maintain the activation of Akt, JNK, and cleavage of p21-activated protein kinase 2 (PAK-2), thereby altering the Akt signals from proliferative to apoptotic. It was TLR4 that mediated these effects (Tang et al., 2000; Schulthess et al., 2009).
Compared to T2DM patients, clinical investigations have demonstrated a substantial decrease in plasma CX3CL1 levels in the control group (Baldane et al., 2018). T2DM is strongly linked to CX3CL1, which is known to mediate leukocyte chemotaxis, adhesion, and survival, resulting in chronic adipose inflammation. CX3CL1 may be crucial in attracting monocytes to adipose tissue, which subsequently causes IR and systemic inflammation (Cefalu, 2011). The mechanism of leukocyte chemotaxis and adhesion mediated by CX3CL1 may impact T2DM and adipocyte dysfunction.
Finally, the studies described above suggested that hypertension-related disorders such as PE, GDM, CVDs, and T2DM alter specific CXC chemokines, including CXCL5, CXC10/CXCR3, CXCL12/CXCR4, CXCL16/CXCR6, and CX3CL1/CX3CR1. These CXC chemokines play a critical role in anti-CVDs, whereas in PE, GDM, and T2DM, they cause inflammation, the anti-angiogenesis process, damaged trophoblastic cells, dyslipidemia, and IR. Additional research is necessary to clarify the mechanisms underlying the interactions between CXC chemokines and hypertension-related diseases.
7 Therapeutic strategies to target CXC chemokines in PE and GDM
Wu et al. (2016) reported that CXCR2 regulated the expression of p-Akt, MMP-2, and MMP-9. CXCR2 inhibition with MK-2206 (2HC) suppressed trophoblast invasion by downregulating MMP-2 and MMP-9 levels via the Akt signaling pathway. CXCR2 inhibition reduced the inflammatory mediators such as MMP-2 and MMP9 during PE. In the placenta, nobiletin-added combination therapy also suppressed placental AKT and activated MAPK ERK1/2 during GDM. It also inhibited expression of CXCL8 secretion in placental tissue and VAT (Lacorte et al., 2022). These findings suggest that nobiletin works by activating the NF-kB, AKT, and MAPK/ERK1/2 pathways to enhance insulin sensitivity in tissues that have been impaired by inflammation and proinflammatory chemokines.
Furthermore, since CX3CL1 levels in the blood are linked to indicators of insulin resistance in people with GDM, researchers investigated whether resveratrol therapy might reduce CX3CL1/CX3CR1 production. Interestingly, in light of their results, the authors reported the therapeutic effects of resveratrol on reducing hyperglycemia and reductions in CX3CL1 production (Szukiewicz et al., 2017). Thus, pregnant women with metabolic disorders and euglycemia should benefit most from resveratrol’s anti-inflammatory effects on placental CX3CL1/CX3CR1 signaling. A comprehensive summary of the subsequent clinical and laboratory CXC chemokine and receptors (CXCRs) and CX3CL1 investigations in PE and GDM can be found in Table 4. In a few clinical and experimental studies on PE and GDM, CXC chemokines and receptors CXCRs and CX3CL1 are being investigated. The techniques required to target CXC chemokines and receptors CXCRs and CX3CL1 will reveal their potential use in PE and GDM.
8 Conclusions and future perspectives
We tried to clarify the expression, underlying molecular processes, origins, and essential roles of CXC and CX3CL1 chemokines in PE and GDM. In particular, CXCL1, 8, 10, 12, 16, and CX3CL1 play a vital role in the inflammation, endothelium dysfunctions, IR, and antiangiogenic processes during PE and GDM. In the studies mentioned above, PE and GDM patients had altered CXC and CX3CL1 chemokines. This review paper summarized the latest CXC and CX3CL1 chemokine molecular mechanisms underlying PE and GDM pathophysiology. This enabled insights into future difficulties and possibilities for distinct clinical and therapeutic properties. Consequences for PE and GDM, CXC and CX3CL1 chemokines inhibitors or drugs prevent the emergence and occurrence of severe PE and GDM, which may aid in improving the diagnosis and treatment of PE and GDM.
However, our knowledge of the complicated communication mechanism between CXC and CX3CL1 chemokines and their receptors is still lacking, which may limit the development of novel PE and GDM treatments. Each chemokine and receptor should be fully and immediately addressed to guarantee therapeutic relevance. Thus, more clinical and preclinical studies are needed to determine the molecular mechanism and whether an anti-inflammatory strategy targeting CXC and CX3CL1 chemokines and receptors could prevent PE and GDM progression.
Author contributions
AU: Conceptualization, Data curation, Formal Analysis, Investigation, Methodology, Software, Writing–original draft, Writing–review and editing. JZ: Formal Analysis, Investigation, Writing–review and editing. RS: Formal Analysis, Writing–review and editing, Investigation. BS: Conceptualization, Data curation, Formal Analysis, Funding acquisition, Investigation, Methodology, Project administration, Resources, Supervision, Visualization, Writing–review and editing.
Funding
The authors declare financial support was received for the research, authorship, and/or publication of this article. This work was supported by the National Natural Science Foundation of China (32070671, 32270690), the COVID-19 research projects of West China Hospital Sichuan University (Grant no. HX-2019-nCoV-057) as well as the regional innovation cooperation between Sichuan and Guangxi Provinces (2020YFQ0019).
Acknowledgments
West China Hospital Sichuan University financially supported this study. BioRender.com was used to create the figures.
Conflict of interest
The authors declare that the research was conducted in the absence of any commercial or financial relationships that could be construed as a potential conflict of interest.
Publisher’s note
All claims expressed in this article are solely those of the authors and do not necessarily represent those of their affiliated organizations, or those of the publisher, the editors and the reviewers. Any product that may be evaluated in this article, or claim that may be made by its manufacturer, is not guaranteed or endorsed by the publisher.
References
Aban, M., Cinel, L., Arslan, M., Dilek, U., Kaplanoglu, M., Arpaci, R., et al. (2004). Expression of nuclear factor-kappa B and placental apoptosis in pregnancies complicated with intrauterine growth restriction and preeclampsia: an immunohistochemical study. Tohoku J. Exp. Med. 204, 195–202. doi:10.1620/tjem.204.195
Accortt, E., Mirocha, J., Zhang, D., Kilpatrick, S. J., Libermann, T., and Karumanchi, S. A. (2023). Perinatal mood and anxiety disorders: biomarker discovery using plasma proteomics. Am. J. Obstetrics Gynecol. 229, 166.e1–166.e16. doi:10.1016/j.ajog.2023.01.012
Agarwal, P., Brar, N., Morriseau, T. S., Kereliuk, S. M., Fonseca, M. A., Cole, L. K., et al. (2019). Gestational diabetes adversely affects pancreatic Islet architecture and function in the male rat Offspring. Endocrinology 160, 1907–1925. doi:10.1210/en.2019-00232
Ahadzadeh, E., Rosendahl, A., Czesla, D., Steffens, P., Prüßner, L., Meyer-Schwesinger, C., et al. (2018). The chemokine receptor CX3CR1 reduces renal injury in mice with angiotensin ii-induced hypertension. Am. J. Physiology - Ren. Physiology 315, F1526–F1535. doi:10.1152/ajprenal.00149.2018
Akhtar, S., Gremse, F., Kiessling, F., Weber, C., and Schober, A. (2013). CXCL12 promotes the stabilization of atherosclerotic lesions mediated by smooth muscle progenitor cells in apoe-deficient mice. Arteriosclerosis, Thrombosis, Vasc. Biol. 33, 679–686. doi:10.1161/ATVBAHA.112.301162
Al-ajlan, A., Al-musharaf, S., Fouda, M. A., Krishnaswamy, S., Wani, K., Aljohani, N. J., et al. (2018). Lower vitamin D levels in Saudi pregnant women are associated with higher risk of developing GDM. BMC Pregnancy Childbirth 18, 86. doi:10.1186/s12884-018-1723-3
Al-Ofi, E., Alrafiah, A., Maidi, S., Almaghrabi, S., and Hakami, N. (2021). Altered expression of angiogenic biomarkers in pregnancy associated with gestational diabetes. Int. J. General Med. 14, 3367–3375. doi:10.2147/IJGM.S316670
Alqudah, A., Eastwood, K. A., Jerotic, D., Todd, N., Hoch, D., McNally, R., et al. (2021). FKBPL and SIRT-1 are downregulated by diabetes in pregnancy impacting on angiogenesis and endothelial function. Front. Endocrinol. 12, 650328. doi:10.3389/fendo.2021.650328
Anton, L., Olarerin-george, A. O., Schwartz, N., Srinivas, S., Bastek, J., Hogenesch, J. B., et al. (2013). miR-210 inhibits trophoblast invasion and is a serum biomarker for preeclampsia. Am. J. Pathology 183, 1437–1445. doi:10.1016/j.ajpath.2013.07.021
Ao, D., Li, D.-J., and Li, M.-Q. (2020). CXCL12 in normal and pathological pregnancies: a review. Am. J. reproductive Immunol. 84, e13280. doi:10.1111/aji.13280
Aslanian, A. M., and Charo, I. F. (2006). Targeted disruption of the scavenger receptor and chemokine CXCL16 accelerates atherosclerosis. Circulation 114, 583–590. doi:10.1161/CIRCULATIONAHA.105.540583
Atallah, R., Gindlhuber, J., Platzer, W., Bärnthaler, T., Tatzl, E., Toller, W., et al. (2021). Sucnr1 is expressed in human placenta and mediates angiogenesis: significance in gestational diabetes. Int. J. Mol. Sci. 22, 12048. doi:10.3390/ijms222112048
Baird, A., Gray, S. G., and Byrne, K. J. O. (2011). 2 Expression of the CXC (ELR+) receptor, CXCR2, is reactivated by epigenetic means in non-small cell lung cancer. PLOS ONE 6, 1–11. doi:10.1016/s0169-5002(11)70002-2
Baldane, S., Ipekci, S. H., Ekin, A., Abusoglu, S., Unlu, A., and Kebapcilar, L. (2018). Evaluation of fractalkine (FKN) and secreted frizzled-related protein 4 (SFRP-4) serum levels in patients with prediabetes and type 2 diabetes. Bratislava Med. J. 119, 112–115. doi:10.4149/BLL_2018_021
Barlic, J., Zhu, W., and Murphy, P. M. (2009). Atherogenic lipids induce high-density lipoprotein uptake and cholesterol efflux in human macrophages by up-regulating transmembrane chemokine CXCL16 without engaging CXCL16-dependent cell adhesion. J. Immunol. 182, 7928–7936. doi:10.4049/jimmunol.0804112
Baston-buest, D. M., Altergot-ahmad, O., Pour, S. J., Krüssel, J., Markert, U. R., Fehm, T. N., et al. (2017). Syndecan-1 acts as an important regulator of CXCL1 expression and cellular interaction of human endometrial stromal and trophoblast cells. Mediat. Inflamm. 2017, 8379256. doi:10.1155/2017/8379256
Batts, T., Langhor, I., Moeller, C., Liu, C., Sones, J., and Johnston, A. (2021). Hepatic steatosis precedes pregnancy in the BPH/5 preeclampsia-like mouse model. Fed. Am. Soc. Exp. Biol. 35, 1–8. doi:10.1096/fasebj.2021.35.S1.03307
Bazan, J. F., Bacon, K. B., Hardiman, G., Wang, W., Soo, K., Rossi, D., et al. (1997). A new class of membrane-bound chemokine with a CX3C motif. Nature 385, 640–644. doi:10.1038/385640a0
Boag, S. E., Das, R., Shmeleva, E. V., Bagnall, A., Egred, M., Howard, N., et al. (2015). T lymphocytes and fractalkine contribute to myocardial ischemia/reperfusion injury in patients. J. Clin. Investigation 125, 3063–3076. doi:10.1172/JCI80055
Boij, R., Svensson, J., Nilsson-ekdahl, K., Sandholm, K., Lindahl, T. L., Ernerudh, J., et al. (2012). Biomarkers of coagulation, inflammation, and angiogenesis are independently associated with preeclampsia. Am. J. Reproductive Immunol. 68, 258–270. doi:10.1111/j.1600-0897.2012.01158.x
Bolatai, A., He, Y., and Wu, N. (2022). Vascular endothelial growth factor and its receptors regulation in gestational diabetes mellitus and eclampsia. J. Transl. Med. 20, 400. doi:10.1186/s12967-022-03603-4
Bowen, J. M., Chamley, L., Mitchell, M. D., and Keelan, J. A. (2002). Cytokines of the placenta and extra-placental membranes: biosynthesis, secretion and roles in establishment of pregnancy in women. Placenta 23, 239–256. doi:10.1053/plac.2001.0781
Brien, M.-E., Boufaied, I., Bernard, N., Forest, J.-C., Giguere, Y., and Girard, S. (2020). Specific inflammatory profile in each pregnancy complication: a comparative study. Am. Jounral Reproductive Immunol. 84, e13316. doi:10.1111/aji.13316
Bujak, M., Dobaczewski, M., Gonzalez-Quesada, C., Xia, Y., Leucker, T., Zymek, P., et al. (2009). Induction of the CXC chemokine interferon-γ-inducible protein 10 regulates the reparative response following myocardial infarction. Circulation Res. 105, 973–983. doi:10.1161/CIRCRESAHA.109.199471
Caballero, I., Al Ghareeb, S., Basatvat, S., Sánchez-López, J. A., Montazeri, M., Maslehat, N., et al. (2013). Human trophoblast cells modulate endometrial cells nuclear factor κB response to flagellin in vitro. PLOS ONE 8, e39441. doi:10.1371/journal.pone.0039441
Cefalu, W. T. (2011). Fractalkine: a cellular link between adipose tissue inflammation and vascular pathologies. Diabetes 60, 1380–1382. doi:10.2337/db11-0239
Chaiworapongsa, T., Chaemsaithong, P., Yeo, L., and Roberto, R. (2014). Pre-eclampsia part 1: current understanding of its pathophysiology. Nat. Rev. Nephrol. 10, 466–480. doi:10.1038/nrneph.2014.102
Chedraui, P., Sol, E. J., Faustino, R. P., Schatz, F., Kayisli, U., Escobar, G. S., et al. (2015). Umbilical cord plasma interferon-induced protein 10 (IP-10) and interferon-induced T-cell alpha chemoattractant (ITAC) levels are lower in women with severe preeclampsia. J. Perinat. Med. 44, 291–294. doi:10.1515/jpm-2014-0371
Chen, L., Merkhan, M. M., Forsyth, N. R., and Wu, P. (2019). Chorionic and amniotic membrane-derived stem cells have distinct, and gestational diabetes mellitus independent, proliferative, differentiation, and immunomodulatory capacities. Stem Cell Res. 40, 101537. doi:10.1016/j.scr.2019.101537
Chen, L., Wang, C., Forsyth, N. R., and Wu, P. (2020). Transcriptional profiling reveals altered biological characteristics of chorionic stem cells from women with gestational diabetes. Stem Cell Res. Ther. 11, 319. doi:10.1186/s13287-020-01828-y
Chu, P. Y., Walder, K., Horlock, D., Williams, D., Nelson, E., Byrne, M., et al. (2015). CXCR4 antagonism attenuates the development of diabetic cardiac fibrosis. PLOS ONE 10, 0133616. doi:10.1371/journal.pone.0133616
Cindrova-Davies, T., Sanders, D. A., Burton, G. J., and Charnock-Jones, D. S. (2011). Soluble FLT1 sensitizes endothelial cells to inflammatory cytokines by antagonizing VEGF receptor-mediated signalling. Cardiovasc. Res. 89, 671–679. doi:10.1093/cvr/cvq346
Cui, J., Chen, X., Lin, S., Li, L., Fan, J., Hou, H., et al. (2020). MiR-101-containing extracellular vesicles bind to BRD4 and enhance proliferation and migration of trophoblasts in preeclampsia. Stem Cell Res. Ther. 11, 231. doi:10.1186/s13287-020-01720-9
Damås, J. K., Wæhre, T., Yndestad, A., Ueland, T., Müller, F., Eiken, H. G., et al. (2002). Stromal cell-derived factor-1alpha in unstable angina: potential antiinflammatory and matrix-stabilizing effects. Circulation 106, 36–42. doi:10.1161/01.CIR.0000020001.09990.90
Darakhshan, S., Fatehi, A., Hassanshahi, G., Mahmoodi, S., Hashemi Seyed, M., and Karimabad Noroozi, M. (2019a). Serum concentration of angiogenic (CXCL1, CXCL12) and angiostasis (CXCL9, CXCL10) CXC chemokines are differentially altered in normal and gestational diabetes mellitus associated pregnancies. J. Diabetes & Metabolic Disord. 18, 371–378. doi:10.1007/s40200-019-00421-2
Darakhshan, S., Hassanshahi, G., Mo, Z., Soltani, B., and Karimabad, M. N. (2019b). CXCL9/CXCL10 angiostasis CXC-chemokines in parallel with the CXCL12 as an angiogenesis CXC-chemokine are variously expressed in pre-eclamptic- women and their neonates. Pregnancy Hypertens. 17, 36–42. doi:10.1016/j.preghy.2019.05.001
D’Haese, J. G., Friess, H., and Ceyhan, G. O. (2012). Therapeutic potential of the chemokine-receptor duo fractalkine/CX3CR1: an update. Expert Opin. Ther. Targets 16, 613–618. doi:10.1517/14728222.2012.682574
Dhawan, P., and Richmond, A. (2002). Role of CXCL1 in tumorigenesis of melanoma. J. Leukoc. Biol. 72, 9–18. doi:10.1189/jlb.72.1.9
Dijmărescu, A. L., Boldeanu, L., Radu, M., Rotaru, I., Siminel, M. A., Maria Magdalena Manolea, S. C. V., et al. (2021). The potential value of diagnostic and predictive serum biomarkers for preeclampsia. Romanian J. Morphol. Embryology 62, 981–989. doi:10.47162/RJME.62.4.10
Dobaczewski, M., and Frangogiannis, N. G. (2009). Chemokines and cardiac fibrosis. Inflammation 1, 391–405. doi:10.2741/s33
Dong, P. ping (2019). Association of vascular endothelial growth factor expression and polymorphisms with the risk of gestational diabetes mellitus. J. Clin. Laboratory Analysis 33, e22686. doi:10.1002/jcla.22686
Dong, Y., Betancourt, A., Belfort, M. A., and Yallampalli, C. (2022). Lipid dysfunction and adrenomedullin expression in omental versus subcutaneous adipose tissues in diabetic pregnancies. PLOS ONE 17, e0265419. doi:10.1371/journal.pone.0265419
Duley, L. (2009). The global impact of pre-eclampsia and eclampsia. Seminars Perinatology 33, 130–137. doi:10.1053/j.semperi.2009.02.010
Dunk, C. E., Dijk, M. V., Choudhury, R., Wright, T. J., Cox, B., Leavey, K., et al. (2021). Functional evaluation of STOX1 (STORKHEAD-BOX PROTEIN 1) in placentation, preeclampsia, and preterm birth. Hypertension 77, 475–490. doi:10.1161/HYPERTENSIONAHA.120.15619
Ebert, T., Hindricks, J., Kralisch, S., Lossner, U., Jessnitzer, B., Richter, J., et al. (2014). Serum levels of fractalkine are associated with markers of insulin resistance in gestational diabetes. Diabet. Med. 31, 1014–1017. doi:10.1111/dme.12451
Estensen, M., Grindheim, G., Remme, E. W., Godang, K., Henriksen, T., Aukrust, P., et al. (2015). Elevated inflammatory markers in preeclamptic pregnancies, but no relation to systemic arterial stiffness. Pregnancy Hypertens. An Int. J. Women’s Cardiovasc. Health 5, 325–329. doi:10.1016/j.preghy.2015.09.003
Evangelista, A. F., Collares, C. V. A., Xavier, D. J., Macedo, C., Manoel-caetano, F. S., Rassi, D. M., et al. (2014). Integrative analysis of the transcriptome profiles observed in type 1, type 2 and gestational diabetes mellitus reveals the role of inflammation. BMC Med. Genomics 7, 28. doi:10.1186/1755-8794-7-28
Fakhr, Y., Koshti, S., Habibyan, Y. B., Webster, K., and Hemmings, D. G. (2022). Tumor necrosis factor-α induces a preeclamptic-like phenotype in placental villi via sphingosine kinase 1 activation. Int. J. Mol. Sci. 23, 3750. doi:10.3390/ijms23073750
Faulhaber, F. R. S., Silveira, R. C., Vargas, A. P., and Procianoy, R. S. (2011). Chemokines plasma levels in preterm newborns of preeclamptic mothers. Cytokine 56, 515–519. doi:10.1016/j.cyto.2011.07.012
Frangogiannis, N. G., Mendoza, L. H., Lewallen, M., Michael, L. H., Smith, C. W., and Entman, M. L. (2001). Induction and suppression of interferon-inducible protein 10 in reperfused myocardial infarcts may regulate angiogenesis. Fed. Am. Soc. Exp. Biol. 15, 1428–1430. doi:10.1096/fj.00-0745fje
Fu, G., Brki, J., Hayder, H., and Peng, C. (2013). MicroRNAs in human placental development and pregnancy complications. Int. J. Mol. Sci. 14, 5519–5544. doi:10.3390/ijms14035519
Fu, M., Zhang, X., Liu, C., Lyu, J., Liu, X., Zhong, S., et al. (2023). Phenotypic and functional alteration of CD45+ immune cells in the decidua of preeclampsia patients analyzed by mass cytometry (CyTOF). Front. Immunol. 13, 1047986. doi:10.3389/fimmu.2022.1047986
Fuchs, F. D., and Whelton, P. K. (2020). High blood pressure and cardiovascular disease. Hypertension 75, 285–292. doi:10.1161/HYPERTENSIONAHA.119.14240
Garcia, M. D. S., Mobley, Y., Henson, J., Davies, M., Skariah, A., Dambaeva, S., et al. (2018). Early pregnancy immune biomarkers in peripheral blood may predict preeclampsia. J. Reproductive Immunol. 125, 25–31. doi:10.1016/j.jri.2017.10.048
Giambrone, A. B., Logue, O. C., Shao, Q., Bidwell, G. L., and Warrington, J. P. (2019). Perinatal micro-bleeds and neuroinflammation in e19 rat fetuses exposed to utero-placental ischemia. Int. J. Mol. Sci. 20, 4051. doi:10.3390/ijms20164051
Gigraci, T., Celik, H., Avci, B., Yavuz, E., Alper, T., and Malatyalioglu, E. (2010). Maternal and umbilical serum levels of interleukin-6, interleukin-8, and tumor necrosis factor-alpha in normal pregnancies and in pregnancies complicated by preeclampsia. J. Maternal-Fetal Neonatal Med. 23, 880–886. doi:10.3109/14767051003774942
Gotsch, F., Romero, R., Friel, L., Kusanovic, J. P., Erez, O., Than, N. G., et al. (2007). CXCL10/IP-10: a missing link between inflammation and anti-angiogenesis in preeclampsia? J. Maternal-Fetal Neonatal Med. 20, 777–792. doi:10.1080/14767050701483298
Greer, I. A., Haddad, N. G., Dawes, J., Johnstone, F. D., and Calder, A. A. (1989). Neutrophil activation in pregnancy-induced hypertension. Br. J. Obstetric Gynaecol. 96, 978–982. doi:10.1111/j.1471-0528.1989.tb03358.x
Guariguata, K. O. L., Barengo, N. ., Lopez-Doriga, P. R., Sacre, J. W., Karuranga, S., Sun, H., et al. (2022). IDF diabetes Atlas: global estimates of undiagnosed diabetes in adults for 2021. Diabetes Res. Clin. Pract. 183, 109118. doi:10.1016/j.diabres.2021.109118
Gui, S., Ni, S., Jia, J., Gong, Y., Gao, L., Zhang, L., et al. (2014). Inconformity of CXCL3 plasma level and placenta expression in preeclampsia and its effect on trophoblast viability and invasion. PLOS ONE 9, e114408. doi:10.1371/journal.pone.0114408
Haedersdal, S., Salvig, J. D., Aabye, M., Thorball, C. W., Ruhwald, M., Ladelund, S., et al. (2013). Inflammatory markers in the second trimester prior to clinical onset of preeclampsia, intrauterine growth restriction, and spontaneous preterm birth. Inflammation 36, 907–913. doi:10.1007/s10753-013-9619-x
Halvatsiotis, P., Chrelias, C., Kassanos, D., Domali, E., Gazouli, M., Georgios, K., et al. (2019). Methylation profile of genes involved in inflammation, in the blood from pregnancies with maternal preeclampsia due to untreated gestational diabetes mellitus. Horm. (Athens) 2, 173–178. doi:10.1007/s42000-019-00111-x
Han, C. S., Herrin, M. A., Pitruzzello, M. C., Mulla, M. J., Werner, E. F., Pettker, C. M., et al. (2015). Glucose and metformin modulate human first trimester trophoblast function: a model and potential therapy for diabetes-associated uteroplacental insufficiency. Am. J. Reproductive Immunol. 73, 362–371. doi:10.1111/aji.12339
Hannan, N. J., Bambang, K., Kaitu’u-Lino, T. J., Konje, J. C., and Tong, S. (2014). A bioplex analysis of cytokines and chemokines in first trimester maternal plasma to screen for predictors of miscarriage. PLOS ONE 9, e93320. doi:10.1371/journal.pone.0093320
Hannan, N. J., Jones, R. L., White, C. A., and Salamonsen, L. A. (2006). The chemokines, CX3CL1, CCL14, and CCL4, promote human trophoblast migration at the feto-maternal interface. Biol. Reproduction 74, 896–904. doi:10.1095/biolreprod.105.045518
Hayder, H., Fu, G., Nadeem, L., Brien, J. A. O., Lye, S. J., and Peng, C. (2021). Overexpression of miR-210-3p impairs extravillous trophoblast functions associated with uterine spiral artery remodeling. Int. J. Mol. Sci. 22, 3961. doi:10.3390/ijms22083961
He, L., Zhan, F., Lu, L., Zhang, X., and Wu, J. (2023). Role of necroptosis and immune infiltration in preeclampsia: novel insights from bioinformatics analyses. BMC Pregnancy Childbirth 23, 495. doi:10.1186/s12884-023-05821-0
Heinke, J., Patterson, C., and Moser, M. (2012). Life is a pattern: vascular assembly within the embryo. Front. Biosci. 4, 2269–2288. doi:10.2741/541
Heydarlou, H., Eghabl-Fard, S., Ahmadi, M., Aghebati-Maleki, L., Dolati, S., Ali Akbar Movassaghpour, F. J.-N., et al. (2018). Investigation of follicular helper T cells, as a novel player,in preeclampsia. Jounral Cell. Biochem. 120, 3845–3852. doi:10.1002/jcb.27666
Howell, K. R., and Powell, T. L. (2017). Effects of maternal obesity on placental function and fetal development. Reproduction 153, R97–R123. doi:10.1530/REP-16-0495
Huang, S. J., Chen, C., Buchwalder, L., Yu, Y., Piao, L., Huang, C., et al. (2019). Regulation of CX3CL1 expression in human first-trimester decidual cells: implications for preeclampsia. Reprod. Sci. 26, 1256–1265. doi:10.1177/1933719118815592
Huang, S. J., Schatz, F., Masch, R., Rahman, M., Buchwalder, L., Niven-Fairchild, T., et al. (2006). Regulation of chemokine production in response to pro-inflammatory cytokines in first trimester decidual cells. J. Reproductive Immunol. 72, 60–73. doi:10.1016/j.jri.2006.03.002
Huppertz, B. (2008). Placental origins of preeclampsia: challenging the current hypothesis. Hypertension 51, 970–975. doi:10.1161/HYPERTENSIONAHA.107.107607
Hwang, H. S., Kwon, H. S., Sohn, I. S., Park, Y. W., and Ykim, oung H. (2012). Increased CXCL12 expression in the placentae of women with pre-eclampsia. Eur. J. Obstetrics Gynecol. Reproductive Biol. 160, 137–141. doi:10.1016/j.ejogrb.2011.10.007
Jackson, E. K., Zhang, Y., Gillespie, D. D., Zhu, X., Cheng, D., and Jackson, T. C. (2017). SDF-1α (stromal cell-derived factor 1α) induces cardiac fibroblasts, renal microvascular smooth muscle cells, and glomerular mesangial cells to proliferate, cause hypertrophy, and produce collagen. J. Am. Heart Assoc. 6, e007253. doi:10.1161/JAHA.117.007253
Jia, R., Zhang, X., Hu, P., Liu, X., Hua, X., Wang, X. I. N., et al. (2012). Screening for differential methylation status in human placenta in preeclampsia using a CpG island plus promoter microarray. Int. J. Mol. Med. 30, 133–141. doi:10.3892/ijmm.2012.983
Jiang, R., Wang, T., Zhou, F., Yao, Y., He, J., and Xu, D. (2020). Bioinformatics-based identi fi cation of miRNA-lncRNA-and mRNA-associated ceRNA networks and potential biomarkers for preeclampsia. Medicine 99, e22985. doi:10.1097/MD.0000000000022985
Johnston, A. N., Batts, T. L., Langohr, I. M., Moeller, C., Liu, C., and Sones, J. L. (2021). The BPH/5 mouse model of superimposed preeclampsia is not a model of HELLP syndrome. Biology 10, 1179. doi:10.3390/biology10111179
Kalinderis, M., Papanikolaou, A., Kalinderi, K., Vyzantiadis, T. A., Ioakimidou, A., and Tarlatzis, B. C. (2015). Serum levels of leptin and IP-10 in preeclampsia compared to controls. Archives Gynecol. Obstetrics 292, 343–347. doi:10.1007/s00404-015-3659-4
Kapustin, R. V., Drobintseva, A. O., Alekseenkova, E. N., Onopriychuk, A. R., Arzhanova, O. N., Polyakova, V. O., et al. (2019). Placental protein expression of kisspeptin-1 (KISS1) and the kisspeptin-1 receptor (KISS1R) in pregnancy complicated by diabetes mellitus or preeclampsia. Archives Gynecol. Obstetrics 301, 437–445. doi:10.1007/s00404-019-05408-1
Karaman, S., Leppänen, V. M., and Alitalo, K. (2018). Vascular endothelial growth factor signaling in development and disease. Dev. Camb. 145, dev151019. doi:10.1242/dev.151019
Karlmark, K. R., Zimmermann, H. W., Roderburg, C., Gassler, N., Wasmuth, H. E., Luedde, T., et al. (2010). The fractalkine receptor CX₃CR1 protects against liver fibrosis by controlling differentiation and survival of infiltrating hepatic monocytes. Hepatology 52, 1769–1782. doi:10.1002/hep.23894
Kawaguchi, N., Zhang, T.-T., and Nakanishi, and T. (2019). Involvement of CXCR4 in normal and abnormal development. Cells 8, 185–214. doi:10.3390/cells8020185
Kim, C. J., Romero, R., Yoo, J. P. K. W., Dong, Z., Topping, V., Gotsch, F., et al. (2010). The frequency, clinical significance, and pathological features of chronic chorioamnionitis: a lesion associated with spontaneous preterm birth. Mod. Pathol. 23, 1000–1011. doi:10.1038/modpathol.2010.73
Kim, D., Kim, J., Yoon, J. H., Ghim, J., Yea, K., Song, P., et al. (2014). CXCL12 secreted from adipose tissue recruits macrophages and induces insulin resistance in mice. Diabetologia 57, 1456–1465. doi:10.1007/s00125-014-3237-5
Kiyokoba, R., Uchiumi, T., Yagi, M., Toshima, T., Tsukahara, S., Fujita, Y., et al. (2022). Mitochondrial dysfunction-induced high hCG associated with development of fetal growth restriction and pre-eclampsia with fetal growth restriction. Sci. Rep. 12, 4056. doi:10.1038/s41598-022-07893-y
Krishnasamy, S., Ravi, V., Rajaraman, B., Kumar, S., Dhevasena, C. S., Pathak, A., et al. (2019). Role of VEGF 165b/VEGF TOTAL ratio in gestational diabetes mellitus. Gynecol. Endocrinol. 0, 811–814. doi:10.1080/09513590.2019.1595576
Kudryashova, A. V., Panova, I. A., and Rokotyanskaya, E. A. (2019). The role of neutrofils in the pathogenesis of preeclampsia. Russ. J. Immunol. 22, 350–352. doi:10.31857/S102872210006624-1
Kuzmicki, M., Telejko, B., Wawrusiewicz-kurylonek, N., Lipinska, D., Pliszka, J., Wilk, J., et al. (2013). The expression of genes involved in NF-κB activation in peripheral blood mononuclear cells of patients with gestational diabetes. Eur. J. Endocrinol. 168, 419–427. doi:10.1530/EJE-12-0654
Lacorte, L. H., Yanga, J. F., Samson, K. S., Aclibon, Y. K., Buenaventura, J. N., Francis, J., et al. (2022). Efficacy of flavonoids in increasing insulin sensitivity among pregnant women with gestational diabetes mellitus: a systematic review. Asian J. Biol. Life Sci. 11, 285–293. doi:10.5530/ajbls.2022.11.38
Ladd-acosta, C., Vang, E., Barrett, E. S., Bulka, C. M., Bush, N. R., Cardenas, A., et al. (2023). Analysis of pregnancy complications and epigenetic gestational age of newborns. JAMA Netw. Open 6, e230672. doi:10.1001/jamanetworkopen.2023.0672
Lee, N., Nikfarjam, M., and He, H. (2018). Functions of the CXC ligand family in the pancreatic tumor microenvironment. Pancreatology 18, 705–716. doi:10.1016/j.pan.2018.07.011
Lei, G. Q., Wu, Z. Y., Jiang, W. B., Luo, J., Xu, H., Yun, Z., et al. (2019). Effect of CXCL12/CXCR4 on migration of decidua -derived mesenchymal stem cells from pregnancies with preeclampsia. Am. Jounral Reproductive Immunol. 82, e13180. doi:10.1111/aji.13180
Lekva, T., Lyle, R., Cecilie, M., Roland, P., Friis, C., Bianchi, D. W., et al. (2016). Gene expression in term placentas is regulated more by spinal or epidural anesthesia than by late-onset preeclampsia or gestational diabetes mellitus. Sci. Rep. 6, 29715. doi:10.1038/srep29715
Lekva, T., Michelsen, A. E., Aukrust, P., Cecilie, M., Roland, P., Henriksen, T., et al. (2017). CXC chemokine ligand 16 is increased in gestational diabetes mellitus and preeclampsia and associated with lipoproteins in gestational diabetes mellitus at 5 years follow-up. Diabetes & Vasc. Dis. Res. 14, 525–533. doi:10.1177/1479164117728011
Lekva, T., Sugulle, M., Moe, K., Redman, C., Dechend, R., and Staff, A. C. (2020). Multiplex analysis of circulating maternal cardiovascular biomarkers comparing preeclampsia subtypes. Hypertension 75, 1513–1522. doi:10.1161/HYPERTENSIONAHA.119.14580
Li, L., Ling, B., Mei, J., Wang, Y., Zhang, J., and Zhao, X. (2021). Expression and significance of serum soluble fms-like tyrosine kinase 1 (sFlt-1), CXC chemokine ligand 16 (CXCL16), and lipocalin 2 (LCN-2) in pregnant women with preeclampsia. Ann. Palliat. Med. 10, 7866–7871. doi:10.21037/apm-21-1553
Li, M., Wu, Z., Yang, H., and Huang, S. J. (2011). NFκB and JNK/MAPK activation mediates the production of major macrophage- or dendritic cell-recruiting chemokine in human first trimester decidual cells in response to proinflammatory stimuli. J. Clin. Endocrinol. Metabolism 96, 2502–2511. doi:10.1210/jc.2011-0055
Li, N., He, F., Gao, H., Ge, Y., Fan, X., Zhang, J., et al. (2020a). Elevated cell-free fetal DNA contributes to placental inflammation and antiangiogenesis via AIM2 and IFI16 during pre-eclampsia. J. Cell. Physiology 235, 9577–9588. doi:10.1002/jcp.29766
Li, R., and Frangogiannis, N. G. (2021). Chemokines in cardiac fibrosis. Curr. Opin. Physiology 19, 80–91. doi:10.1016/j.cophys.2020.10.004
Li, Y., Liang, M., Lin, Y., Lv, J., Chen, M., Zhou, P., et al. (2020b). Transcriptional expressions of CXCL9/10/12/13 as prognosis factors in breast cancer. J. Oncol. 2020, 4270957–4271015. doi:10.1155/2020/4270957
Li, Y., Long, D., Liu, J., Qiu, D., Wang, J., Cheng, X., et al. (2020c). Gestational diabetes mellitus in women increased the risk of neonatal infection via in fl ammation and autophagy in the placenta. Medicine 99, e22152. doi:10.1097/MD.0000000000022152
Liao, L., Liu, M., Gao, Y., Wei, X., Yin, Y., Gao, L., et al. (2022). The long noncoding RNA TARID regulates the CXCL3/ERK/MAPK pathway in trophoblasts and is associated with preeclampsia. Reproductive Biol. Endocrinol. 20, 159. doi:10.1186/s12958-022-01036-8
Lin, C. F., Su, C. J., Liu, J. H., Chen, S. T., Huang, H. L., and Pan, S. L. (2019). Potential effects of CXCL9 and CCL20 on cardiac fibrosis in patients with myocardial infarction and isoproterenol-treated rats. J. Clin. Med. 8, 659. doi:10.3390/jcm8050659
Lin, J., Meng, Y., Song, M., and Gu, W. (2022). Network-based analysis reveals novel biomarkers in peripheral blood of patients with preeclampsia. Front. Mol. Biosci. 9, 757203–757214. doi:10.3389/fmolb.2022.757203
Liong, S., Barker, G., and Lappas, and M. (2018). Bromodomain protein BRD4 is increased in human placentas from women with early-onset preeclampsia. Reproduction 155, 573–582. doi:10.1530/REP-17-0744
Liu, H., Liu, A., Kaminga, A. C., Mcdonald, J., Wen, S. W., and Pan, X. (2022). Chemokines in gestational diabetes mellitus. Front. Immunol. 13, 705852. doi:10.3389/fimmu.2022.705852
Liu, L., Zhang, X., Rong, C., Rui, C., Ji, H., Qian, Y., et al. (2014). Distinct DNA methylomes of human placentas between pre-eclampsia and gestational diabetes mellitus. Cell. Physiology Biochem. 34, 1877–1889. doi:10.1159/000366386
Liu, X., Dai, L. I., and Zhou, R. (2015). Association between preeclampsia and the CXC chemokine family (Review). Exp. Ther. Med. 9, 1572–1576. doi:10.3892/etm.2015.2337
Liu, Y., Ren, M., Bi, X., Fu, Y., Jing, X., Zhang, H., et al. (2021). A systematic review on the application of vascular endothelial growth factors in preeclampsia. Ann. Palliat. Med. 10, 9259–9266. doi:10.21037/apm-21-2109
Long, L., Ormiston, M. L., Yang, X., Southwood, M., Gräf, S., Machado, R. D., et al. (2015). Selective enhancement of endothelial BMPR-II with BMP9 reverses pulmonary arterial hypertension. Nat. Med. 21, 777–785. doi:10.1038/nm.3877
Lu, J., Wang, Z., Ren, L., Sun, Y., Cao, Y., Xiong, Y., et al. (2020). Expression and functional analysis of CXCL12 and its receptors in human term trophoblast cells. Reprod. Sci. 27, 46–54. doi:10.1007/s43032-019-00134-0
Lu, J., Zhou, W., Ren, L., and Zhang, Y. (2016). CXCR4, CXCR7, and CXCL12 are associated with trophoblastic cells apoptosis and linked to pathophysiology of severe preeclampsia. Exp. Mol. Pathology 100, 184–191. doi:10.1016/j.yexmp.2015.12.013
Lu, M., Zhao, W., Han, S., Lin, X., Xu, T., Tan, Q., et al. (2022a). Activation of the human chemokine receptor CX3CR1 regulated by cholesterol. Sci. Adv. 8, eabn8048. doi:10.1126/sciadv.abn8048
Lu, X., Wang, Z., Ye, D., Feng, Y., Liu, M., Xu, Y., et al. (2022b). The role of CXC chemokines in cardiovascular diseases. Fontiers Pharmacol. 12, 765768. doi:10.3389/fphar.2021.765768
Ma, C., Zhao, M., Wang, X., Zhong, W., Zhang, Y., and Xu, L. (2023). Downregulation of miR-455–3p in decidual cells promotes macrophage polarization and suppresses trophoblasts invasion. Exp. Cell Res. 425, 113510. doi:10.1016/j.yexcr.2023.113510
Maynard, S. E., Min, J.-Y., Merchan, J., Lim, K.-H., Li, J., Mondal, S., et al. (2003). Excess placental soluble fms-like tyrosine kinase 1 (sFlt1) may contribute to endothelial dysfunction, hypertension, and proteinuria in preeclampsia. J. Clin. Investigation 111, 649–658. doi:10.1172/JCI17189
Mcelwain, C. J., Tuboly, E., Mccarthy, F. P., and Mccarthy, C. M. (2020). Mechanisms of endothelial dysfunction in pre-eclampsia and gestational diabetes mellitus: windows into future cardiometabolic health. Front. Endocrinol. 11, 655. doi:10.3389/fendo.2020.00655
Medzhitov, R. (2001). Toll-like receptors and innate immunity. Nat. Rev. Immunol. 1, 135–145. doi:10.1038/35100529
Meeuwsen, J. A. L., Vries, J.De, Zoet, G. A., Franx, A., Fauser, B. C. J. M., Maas, A. H. E. M., et al. (2020). Circulating neutrophils do not predict subclinical coronary artery disease in women with former preeclampsia. Cells 9, 468. doi:10.3390/cells9020468
Mellembakken, J. R., Aukrust, P., Hestdal, K., Ueland, T., Åbyholm, T., and Videm, V. (2001a). Chemokines and leukocyte activation in the fetal circulation during preeclampsia. Hypertension 38, 394–398. doi:10.1161/01.hyp.38.3.394
Mellembakken, J. R., Solum, N. O., Ueland, T., Videm, V., and Aukrust, P. (2001b). Increased concentrations of soluble CD40 ligand, RANTES and GRO- alpha in preeclampsia – possible role of platelet activation. Thrombosis Haemostasis 86, 1272–1276. doi:10.1055/s-0037-1616061
Menhaji-Klotz, E., Hesp, K. D., Londregan, A. T., Kalgutkar, A. S., Piotrowski, D. W., Boehm, M., et al. (2018). Discovery of a novel small-molecule modulator of C-X-C chemokine receptor type 7 as a treatment for cardiac fibrosis. J. Med. Chem. 61, 3685–3696. doi:10.1021/acs.jmedchem.8b00190
Miralles, F., Collinot, H., Boumerdassi, Y., Ducat, A., Duché, A., Renault, G., et al. (2019). Long-term cardiovascular disorders in the STOX1 mouse model of preeclampsia. Sci. Rep. 9, 11918. doi:10.1038/s41598-019-48427-3
Mistry, S. K., Das Gupta, R., Alam, S., Kaur, K., Shamim, A. A., and Puthussery, S. (2021). Gestational diabetes mellitus (GDM) and adverse pregnancy outcome in South Asia: a systematic review. Endocrinol. Diabetes Metabolism 4, e00285. doi:10.1002/edm2.285
Mol, B. W. J., Roberts, C. T., Thangaratinam, S., Magee, L. A., De Groot, C. J. M., and Hofmeyr, G. J. (2016). Pre-eclampsia. Lancet 387, 999–1011. doi:10.1016/S0140-6736(15)00070-7
Molvarec, A., Szarka, A., Walentin, S., Szucs, E., Nagy, B., and Jr, J. R. (2010). Circulating angiogenic factors determined by electrochemiluminescence immunoassay in relation to the clinical features and laboratory parameters in women with pre-eclampsia. Hypertens. Res. 33, 892–898. doi:10.1038/hr.2010.92
Moreno-eutimio, M. A., Tovar-rodríguez, J. M., Vargas-avila, K., Nieto-velázquez, N. G., Frías-de-león, M. G., Sierra-martinez, M., et al. (2014). Increased serum levels of inflammatory mediators and low frequency of regulatory T cells in the peripheral blood of preeclamptic Mexican women. BioMed Res. Int. 8, 413249. doi:10.1155/2014/413249
Nahrendorf, M., Swirski, F. K., Aikawa, E., Stangenberg, L., Wurdinger, T., Figueiredo, J. L., et al. (2007). The healing myocardium sequentially mobilizes two monocyte subsets with divergent and complementary functions. J. Exp. Med. 204, 3037–3047. doi:10.1084/jem.20070885
Negi, M., Mulla, M. J., Han, C. S., and Abrahams, V. M. (2020). Allopurinol inhibits excess glucose-induced trophoblast IL-1β and ROS production. Reproduction 159, 73–80. doi:10.1530/REP-19-0422
Nguyen-ngo, C., Lappas, M., Salomon, C., Quak, S., Lai, A., Willcox, J. C., et al. (2020a). Nobiletin exerts anti-diabetic and anti-inflammatory effects in an in vitro human model and in vivo murine model of gestational diabetes. Clin. Sci. 134, 571–592. doi:10.1042/CS20191099
Nguyen-ngo, C., Perkins, A. V., and Lappas, M. (2022). Selenium prevents inflammation in human placenta and adipose tissue in vitro: implications for metabolic diseases of pregnancy associated with inflammation. Nutrients 14, 3286–3319. doi:10.3390/nu14163286
Nguyen-ngo, C., Willcox, J. C., and Lappas, M. (2019). Anti-Diabetic, anti-inflammatory, and anti-oxidant effects of naringenin in an in vitro human model and an in vivo murine model of gestational diabetes mellitus. Gestation. Diabetes 19, 1900224. doi:10.1002/mnfr.201900224
Nguyen-ngo, C., Willcox, J. C., and Lappas, M. (2020b). Anti-inflammatory effects of phenolic acids punicalagin and curcumin in human placenta and adipose tissue. Placenta 100, 1–12. doi:10.1016/j.placenta.2020.08.002
Nonn, O., Güttler, J., Forstner, D., Maninger, S., Zadora, J., Balogh, A., et al. (2019). Placental CX3CL1 is deregulated by angiotensin II and contributes to a pro-inflammatory trophoblast-monocyte interaction. Int. J. Mol. Sci. 20, 641. doi:10.3390/ijms20030641
O’Brien, J., Hayder, H., Zayed, Y., and Peng, C. (2018). Overview of microRNA biogenesis, mechanisms of actions, and circulation. Front. Endocrinol. 9, 402. doi:10.3389/fendo.2018.00402
O’Haraa, A. M., Bhattacharyyaa, A., Baia, J., Mifflinb, R. C., Ernsta, P. B., Mitrac, S., et al. (2010). Tumor necrosis factor (TNF)-alpha-induced IL-8 expression in gastric epithelial cells: role of reactive oxygen species and AP endonuclease-1/redox factor (Ref)-1. Cytokine 46, 359–369. doi:10.1016/j.cyto.2009.03.010
Oliveira, L. D., Olsen, N., Câmara, S., Peraçoli, J. C., Peraçoli, M. T., Moron, A. F., et al. (2011). sFlt-1 and IP-10 in women with early-onset preeclampsia. Pregnancy Hypertens. An Int. J. Women’s Cardiovasc. Health 1, 129–131. doi:10.1016/j.preghy.2011.02.002
Ozmen, A., Guzeloglu-kayisli, O., Tabak, S., Guo, X., Semerci, N., Nwabuobi, C., et al. (2022). Preeclampsia is associated with reduced ISG15 levels impairing extravillous trophoblast invasion. Front. Cell Dev. Biol. 10, 898088. doi:10.3389/fcell.2022.898088
Pan, X., Jin, X., Wang, J., Hu, Q., and Dai, B. (2021a). Placenta inflammation is closely associated with gestational diabetes mellitus. Am. J. Transl. Res. 13, 4068–4079.
Pan, X., Kaminga, A. C., Wen, S. W., and Liu, A. (2021b). Chemokines in prediabetes and type 2 diabetes: a meta-analysis. Front. Immunol. 12, 622438. doi:10.3389/fimmu.2021.622438
Pan, Y., Lloyd, C., Zhou, H., Dolich, S., Deeds, J., Gonzalo, J., et al. (1997). Neurotactin, a membrane-anchored chemokine upregulated in brain inflammation. Nature 387, 611–617. doi:10.1038/42491
Panagodage, S., Yong, H. E. J., Silva, D., Borg, A. J., Kalionis, B., Brennecke, S. P., et al. (2016). Low-dose acetylsalicylic acid treatment modulates the production of cytokines and improves trophoblast function in an in vitro model of early-onset preeclampsia. Am. J. Pathology 186, 3217–3224. doi:10.1016/j.ajpath.2016.08.010
Panova, I. A., Kudryashova, A. V., Rokotyanskaya, E. A., Malyshkina, A. I., Pareyshvili, V. V., and Bogatova, I. K. (2018). Clinical value of changes of the transmigration activity of leukocytes in the diagnosis of severe preeclampsia. Akusherstvo i Ginekologiya/Obstetrics Gynecol. 8, 77–84. doi:10.18565/aig.2018.8.77-84
Pascoe, C. D., Basu, S., Schwartz, J., Fonseca, M., Kahnamoui, S., Jha, A., et al. (2022). Maternal diabetes promotes offspring lung dysfunction and in flammation in a sex-dependent manner. Am. J. Physiology-Lung Cell. Mol. Physiology 322, 373–384. doi:10.1152/ajplung.00425.2021
Payne, A. S., and Cornelius, L. A. (2002). The role of chemokines in melanoma tumor growth and metastasis. J. Investigative Dermatology 118, 915–922. doi:10.1046/j.1523-1747.2002.01725.x
Perumareddi, P. (2019). Prevention of hypertension related to cardiovascular disease. Prim. Care - Clin. Office Pract. 46, 27–39. doi:10.1016/j.pop.2018.10.005
Pinarbasi, E., Cekin, N., Bildirici, A. E., Akin, S., and Yanik, A. (2020). STOX1 gene Y153H polymorphism is associated with early-onset preeclampsia in Turkish population. Gene 754, 144894. doi:10.1016/j.gene.2020.144894
Pinheiro, M. B., Martins-filho, O. A., Paula, A., Mota, L., Alpoim, P. N., Godoi, L. C., et al. (2013). Severe preeclampsia goes along with a cytokine network disturbance towards a systemic inflammatory state. Cytokine 62, 165–173. doi:10.1016/j.cyto.2013.02.027
Qin, S., Wang, Y., Wang, S., Ning, B., Huai, J., and Yang, H. (2022). Gut microbiota in women with gestational diabetes mellitus has potential impact on metabolism in pregnant mice and their offspring. Front. Microbiol. 13, 870422. doi:10.3389/fmicb.2022.870422
Rana, S., Powe, C. E., Salahuddin, S., Verlohren, S., Perschel, F. H., Levine, R. J., et al. (2012). Angiogenic factors and the risk of adverse outcomes in women with suspected preeclampsia. Circulation 125, 911–919. doi:10.1161/CIRCULATIONAHA.111.054361
Ravi, S., Schuck, R. N., Hilliard, E., Lee, C. R., Dai, X., Lenhart, K., et al. (2017). Clinical evidence supports a protective role for CXCL5 in coronary artery disease. Am. J. Pathology 187, 2895–2911. doi:10.1016/j.ajpath.2017.08.006
Redman, C. W., Sargent, I. L., and Staff, A. C. (2014). IFPA senior award lecture: making sense of pre-eclampsia - two placental causes of preeclampsia? Placenta 28, S20–S25. doi:10.1016/j.placenta.2013.12.008
Ren, Z., Zhe, D., Li, Z., Sun, X.-P., Yang, K., and Lin, L. (2020). Study on the correlation and predictive value of serum pregnancy-associated plasma protein A, triglyceride and serum 25- hydroxyvitamin D levels with gestational diabetes mellitus. World J. Clin. Cases 8, 864–873. doi:10.12998/wjcc.v8.i5.864
Robillard, P., Dekker, G., Bouteiller, P. L., Scioscia, M., Hulsey, T. C., Hospital, L. M., et al. (2019). Preeclampsia and the 20th century: “Le siècle des Lumières.”. Pregnancy Hypertens. 13, 107–109. doi:10.1016/j.preghy.2018.05.013
Rong, M., Yan, X., Zhang, H., Zhou, C., and Zhang, C. (2021). Dysfunction of decidual macrophages is a potential risk factor in the occurrence of preeclampsia. Front. Immunol. 12, 655655. doi:10.3389/fimmu.2021.655655
Rousselle, A., Qadri, F., Leukel, L., Yilmaz, R., Fontaine, J. F., Sihn, G., et al. (2013). CXCL5 limits macrophage foam cell formation in atherosclerosis. J. Clin. Investigation 123, 1343–1347. doi:10.1172/JCI66580
Rout, M., and Lulu, S. S. (2019). Molecular and disease association of gestational diabetes mellitus affected mother and placental datasets reveal a strong link between insulin growth factor (IGF) genes in amino acid transport pathway: a network biology approach. J. Cell Biochem. 120, 1577–1587. doi:10.1002/jcb.27418
Roy, R., Roy, R., Nguyen-ngo, C., and Lappas, M. (2020). Short-chain fatty acids as novel therapeutics for gestational diabetes. J. Mol. Endocrinol. 65, 21–34. doi:10.1530/JME-20-0094
Russo, R. C., Garcia, C. C., Teixeira, M. M., and Amaral, F. A. (2014). The CXCL8/IL-8 chemokine family and its receptors in inflammatory diseases. Expert Rev. Clin. Immunol. 5, 593–619. doi:10.1586/1744666X.2014.894886
Ryu, J., Lee, C., Hong, K., Shin, J., Lim, S., Park, C., et al. (2008). Activation of fractalkine/CX3CR1 by vascular endothelial cells induces angiogenesis through VEGF-A/KDR and reverses hindlimb ischaemia. Cardiovasc. Res. 78, 333–340. doi:10.1093/cvr/cvm067
Sahin, S., Ozakpinar, O. B., Eroglu, M., Tulunay, A., Ciraci, E., Uras, F., et al. (2014). The impact of platelet functions and inflammatory status on the severity of preeclampsia. J. Maternal - Fetal & Neonatal Med. 28, 643–648. doi:10.3109/14767058.2014.927860
Sajadi, S. M. A., Khoramdelazad, H., Hassanshahi, G., Rafatpanah, H., Hosseini, J., Mahmoodi, M., et al. (2013). Plasma levels of CXCL1 (GRO-alpha) and CXCL10 (IP-10) are elevated in type 2 diabetic patients: evidence for the involvement of inflammation and angiogenesis/angiostasis in this disease state. Clin. Lab. 59, 133–137. doi:10.7754/Clin.Lab.2012.120225
Saxena, A., Bujak, M., Frunza, O., Dobaczewski, M., Gonzalez-Quesada, C., Lu, B., et al. (2014). CXCR3-independent actions of the CXC chemokine CXCL10 in the infarcted myocardium and in isolated cardiac fibroblasts are mediated through proteoglycans. Cardiovasc. Res. 103, 217–227. doi:10.1093/cvr/cvu138
Schanz, A., Winn, V. D., Fisher, S. J., Blumenstein, M., Heiss, C., Hess, A. P., et al. (2011). Pre-eclampsia is associated with elevated CXCL12 levels in placental syncytiotrophoblasts and maternal blood. Eur. J. Obstetrics Gynecol. Reproductive Biol. 157, 32–37. doi:10.1016/j.ejogrb.2011.02.023
Schiano, C., Grimaldi, V., Franzese, M., Nigris, F. D., Donatelli, F., Napoli, C., et al. (2019). Non-nutritional sweeteners effects on endothelial vascular function. Toxicol. Vitro 62, 104694. doi:10.1016/j.tiv.2019.104694
Schulthess, F. T., Paroni, F., Sauter, N. S., Shu, L., Ribaux, P., Haataja, L., et al. (2009). CXCL10 impairs β cell function and viability in diabetes through TLR4 signaling. Cell Metab. 9, 125–139. doi:10.1016/j.cmet.2009.01.003
Shaw, J., Tang, Z., Schneider, H., Salje, K., Hansson, S. R., and Guller, S. (2016). Inflammatory processes are specifically enhanced in endothelial cells by placental-derived TNF- alpha: implications in preeclampsia (PE). Placenta 43, 1–8. doi:10.1016/j.placenta.2016.04.015
Shi, J.-W., Yang, H.-L., Fan, D.-X., Yang, S.-L., Qiu, X.-M., Wang, Y., et al. (2020). The role of CXC chemokine ligand 16 in physiological and pathological pregnancies. Am. Jounral Reproductive Immunol. 83, e13223–e13228. doi:10.1111/aji.13223
Shin, G. S. R. L., Joe, Y. S., Kim, S. J., and Shin, J. C. (2010). Cytokine-related genes and oxidation-related genes detected in preeclamptic placentas. Arch. Gynecol. Obstet. 282, 363–369. doi:10.1007/s00404-009-1222-x
Siwetz, M., Dieber-Rotheneder, M., Cervar-Zivkovic, M., Kummer, D., Kremshofer, J., Weiss, G., et al. (2015). Placental fractalkine is up-regulated in severe early-onset preeclampsia. Am. J. Pathology 185, 1334–1343. doi:10.1016/j.ajpath.2015.01.019
Steegers, E. A. P., Von Dadelszen, P., Duvekot, J. J., and Pijnenborg, R. (2010). Pre-eclampsia. Lancet 376, 631–644. doi:10.1016/S0140-6736(10)60279-6
Stievano, L., Piovan, E., and Amadori, A. (2004). C and CX3C chemokines: cell sources and physiopathological implications. Crit. Rev. Immunol. 24, 205–228. doi:10.1615/critrevimmunol.v24.i3.40
Strieter, R. M., Polverini, P. J., Kunkel, S. L., Arenberg, D. A., Burdick, M. D., Kasper, J., et al. (1995). The functional role of the ELR motif in CXC chemokine-mediated angiogenesis. J. Biol. Chem. 270, 27348–27357. doi:10.1074/jbc.270.45.27348
Sugimoto, M., Kondo, M., Kamimoto, Y., Ikeda, T., Cutler, A., Mariya, A., et al. (2019). Changes in VEGF-related factors are associated with presence of inflammatory factors in carbohydrate metabolism disorders during pregnancy. PLOS ONE 14, e0220650. doi:10.1371/journal.pone.0220650
Sullivan, S. E., Staba, S. L., Gersting, J. A., Hutson, A. D., Theriaque, D., Christensen, R. D., et al. (2002). Circulating concentrations of chemokines in cord blood, neonates, and adults. Pediatr. Res. 51, 653–657. doi:10.1203/00006450-200205000-00018
Sun, X., Qu, T., He, X., Yang, X., Guo, N., Mao, Y., et al. (2018). Screening of differentially expressed proteins from syncytiotrophoblast for severe early-onset preeclampsia in women with gestational diabetes mellitus using tandem mass tag quantitative proteomics. BMC Pregnancy Childbirth 18, 437. doi:10.1186/s12884-018-2066-9
Sunjaya, A. P., and Sunjaya, A. F. (2018). Diabetes in pregnancy and infant mortality: link with glycemic control. Diabetes & Metabolic Syndrome Clin. Res. Rev. 12, 1031–1037. doi:10.1016/j.dsx.2018.06.019
Szarka, A., János Rigó, J. R., Lázár, L., Beko, G., and Molvarec, A. (2010). Circulating cytokines, chemokines and adhesion molecules in normal pregnancy and preeclampsia determined by multiplex suspension array. BMC Immunol. 11, 59. doi:10.1186/1471-2172-11-59
Szewczyk, G., Pyzlak, M., Pankiewicz, K., Szczerba, E., Stangret, A., Szukiewicz, D., et al. (2021). The potential association between a new angiogenic marker fractalkine and a placental vascularization in preeclampsia. Archives Gynecol. Obstetrics 304, 365–376. doi:10.1007/s00404-021-05966-3
Szmuilowicz, E. D., Josefson, J. L., and Metzger, B. E. (2019). Gestational diabetes mellitus. Endocrinol. Metab. Clin. North Am. 48, 479–493. doi:10.1016/j.ecl.2019.05.001
Szukiewicz, D., Kochanowski, J., Mittal, T. K., Pyzlak, M., Szewczyk, G., and Cendrowski, K. (2014). CX3CL1 (fractalkine) and TNFα production by perfused human placental lobules under normoxic and hypoxic conditions in vitro: the importance of CX3CR1 signaling. Inflamm. Res. 63, 179–189. doi:10.1007/s00011-013-0687-z
Szukiewicz, D., Pyzlak, M., Szewczyk, G., Stangret, A., Trojanowski, S., Bachanek, M., et al. (2017). High glucose level disturbs the resveratrol-evoked curtailment of CX3CL1/CX3CR1 signaling in human placental circulation. Mediat. Inflamm. 2017, 9853108. doi:10.1155/2017/9853108
Tagoma, A., Haller-kikkatalo, K., Oras, A., Roos, K., Kirss, A., Uibo, R., et al. (2022). Plasma cytokines during pregnancy provide insight into the risk of diabetes in the gestational diabetes risk group. J. Diabetes Investigation 13, 1596–1606. doi:10.1111/jdi.13828
Tang, Y., Zhou, H., Chen, A., Pittman, R. N., and Field, J. (2000). The Akt proto-oncogene links Ras to Pak and cell survival signals. J. Biol. Chem. 275, 9106–9109. doi:10.1074/jbc.275.13.9106
Taub, D., and Oppenheim, J. (1994). Chemokines, inflammation and the immune system. Ther. Immunol. 1, 229–246.
Tok, A., Seyithanoğlu, M., Ozer, A., Erkayıran, U., Çelebi, A., and Çelebi, A. (2019). The serum level of soluble CXCL16 is increased in preeclampsia and associated with hepatic/renal damage. J. Maternal-Fetal Neonatal Med. 34, 1435–1440. doi:10.1080/14767058.2019.1638361
Tseng, J., Chen, Y., Hsieh, Y., and Chou, M. (2009). Elevated amniotic fluid stromal cell-derived factor-1alpha (SDF-1alpha) concentration in mid-gestation as a predictor of adverse birth outcomes. J. Chin. Med. Assoc. 72, 638–642. doi:10.1016/S1726-4901(09)70446-0
Turner, N. A., Das, A., O’Regan, D. J., Ball, S. G., and Porter, K. E. (2011). Human cardiac fibroblasts express ICAM-1, E-selectin and CXC chemokines in response to proinflammatory cytokine stimulation. Int. J. Biochem. Cell Biol. 43, 1450–1458. doi:10.1016/j.biocel.2011.06.008
Ullah, A., Ud Din, A., Ding, W., Shi, Z., Pervaz, S., and Shen, B. (2023). A narrative review: cXC chemokines influence immune surveillance in obesity and obesity-related diseases: type 2 diabetes and nonalcoholic fatty liver disease. Rev. Endocr. Metabolic Disord. 4, 611–631. doi:10.1007/s11154-023-09800-w
Usta, A., Turan, G., Usta, C. S., Avci, E., and Adali, E. (2018). Placental fractalkine immunoreactivity in preeclampsia and its correlation with histopathological changes in the placenta and adverse pregnancy outcomes. J. Maternal-Fetal Neonatal Med. 33, 806–815. doi:10.1080/14767058.2018.1505854
Valencia-Ortega, J., Zárate, A., Saucedo, R., Hernández-Valencia, M., Cruz, J. G., and Puello, E. (2019). Placental proinflammatory state and maternal endothelial dysfunction in preeclampsia. Gynecol. Obstetric Investigation 84, 12–19. doi:10.1159/000491087
Vishnyakova, P., Poltavets, A., Nikitina, M., Muminova, K., Potapova, A., Vtorushina, V., et al. (2021). Preeclampsia: inflammatory signature of decidual cells in early manifestation of disease. Placenta 104, 277–283. doi:10.1016/j.placenta.2021.01.011
Walsh, S. W., Nugent, W. H., Archer, K. J., Dulaimi, M. A. L., Washington, S. L., and Strauss, J. F. (2022). Epigenetic regulation of interleukin-17-related genes and their potential roles in neutrophil vascular infiltration in preeclampsia. Reprod. Sci. 29, 154–162. doi:10.1007/s43032-021-00605-3
Wang, H., Wang, P., Liang, X., Li, W., Yang, M., Ma, J., et al. (2020a). Down-regulation of endothelial protein C receptor promotes preeclampsia by affecting actin polymerization. J. Cell. Mol. Med. 24, 3370–3383. doi:10.1111/jcmm.15011
Wang, H., Wang, T., Dai, L., Cao, W., Ye, L., Gao, L., et al. (2018). Effects of CXCL3 on migration, invasion,proliferation and tube formation of trophoblast cells. Placenta 66, 47–56. doi:10.1016/j.placenta.2018.05.004
Wang, W., Wu, C., Luo, R., and Zhang, Z. (2020b). CXCR4 antagonist alleviates proliferation and collagen synthesis of cardiac fibroblasts induced by TGF-β1. General Physiology Biophysics 39, 187–194. doi:10.4149/gpb_2019051
Wang, Y., Yu, H., Liu, F., and Song, X. (2019a). Analysis of key genes and their functions in placental tissue of patients with gestational diabetes mellitus. Reproductive Biol. Endocrinol. 17, 104. doi:10.1186/s12958-019-0546-z
Wang, Y., Zhou, B., and Zhou, R. (2019b). Association between polymorphisms in CXCR2 gene and preeclampsia. Mol ecular Genet. Genomic Med. 7, e578. doi:10.1002/mgg3.578
Wang, Z. M., Zhu, Q. Y., Zhang, J. F., Wu, J. L., Yang, R., and Wang, D. M. (2017). Changes of platelet parameters in early severe preeclampsia. Clin. Exp. Obstetrics Gynecol. 44, 259–263. doi:10.12891/ceog3430.2017
Wong, V. W., Chong, S., Jalaludin, B., Russell, H., and Depczynski, B. (2014). Urine albumin – creatinine ratio in women with gestational diabetes: its link with glycaemic status. Aust. N. Z. J. Obstetrics Gynaecol. 54, 529–533. doi:10.1111/ajo.12243
Wu, D., Chen, X., Wang, L., Chen, F., Cen, H., and Shi, L. (2019). Hypoxia-induced microRNA-141 regulates trophoblast apoptosis, invasion, and vascularization by blocking CXCL12β/CXCR2/4 signal transduction. Biomed. Pharmacother. 116, 108836. doi:10.1016/j.biopha.2019.108836
Wu, D., Hong, H., Huang, X., Huang, L., He, Z., Fang, Q., et al. (2016). CXCR2 is decreased in preeclamptic placentas and promotes human trophoblast invasion through the Akt signaling pathway. Placenta 43, 17–25. doi:10.1016/j.placenta.2016.04.016
Xu, Q., Wang, J., He, J., Zhou, M., Adi, J., Webster, K. A., et al. (2011). Impaired CXCR4 expression and cell engraftment of bone marrow-derived cells from aged atherogenic mice. Atherosclerosis 219, 92–99. doi:10.1016/j.atherosclerosis.2011.07.118
Yan, Y.-H., Zheng, Y., Yu, L., Han, J., Han, X., Li, L., et al. (2013). Screening for preeclampsia pathogenesis related genes. Eur. J. Pharmacol. Sci. 17, 3083–3094.
Yang, X., Ren, L., Chen, X., Pang, Y., Jia, B., Sun, J., et al. (2023). BMP9 maintains the phenotype of HTR-8/Svneo trophoblast cells by activating the SDF1/CXCR4 pathway. BMC Mol. Cell Biol. 24, 24. doi:10.1186/s12860-023-00487-0
Yang, Y., and Wu, N. (2022). Gestational diabetes mellitus and preeclampsia: correlation and influencing factors. Front. Cardiovasc. Med. 9, 831297. doi:10.3389/fcvm.2022.831297
Yin, M., Zhang, Y., Wang, J., and Li, X. (2022). Comment on Marcoux et al. Varying Impact of Gestational Diabetes Mellitus on Incidence of Childhood Cancers: an Age-Stratified Retrospective Cohort Study. Diabetes Care 2022;45:1177-1183. Diabetes Care 45, 153–154. doi:10.2337/dc22-0954
Yin, N., Zhang, H., Luo, X., Ding, Y., Xiao, X., Liu, X., et al. (2014). IL-27 activates human trophoblasts to express IP-10 and IL-6: implications in the immunopathophysiology of preeclampsia. Mediat. Inflamm. 2014, 926875–926910. doi:10.1155/2014/926875
Ying, Y., and Hongyan, L. (2020). Research on the relationship between vascular endothelial growth factor imbalance and complement deposition in preeclampsia. Chin. J. Comp. Med. 30, 70–76. doi:10.3969/j.issn.1671-7856.2020.04.011
Zhang, J., Chi, H., Xiao, H., Tian, X., Wang, Y., Yun, X., et al. (2017). Interleukin 6 (IL-6) and tumor necrosis factor α (TNF-α) single nucleotide polymorphisms (SNPs), inflammation and metabolism in gestational diabetes mellitus in inner Mongolia. Med. Sci. Monit. 23, 4149–4157. doi:10.12659/MSM.903565
Zhang, J., Dunk, C. E., Shynlova, O., Caniggia, I., and Lye, S. J. (2019). TGFb1 suppresses the activation of distinct dNK subpopulations in preeclampsia. EBioMedicine 39, 531–539. doi:10.1016/j.ebiom.2018.12.015
Zhang, S., Ding, J., Zhang, Y., Liu, S., Yang, J., and Yin, T. (2022a). Regulation and function of chemokines at the maternal–fetal interface. Front. Cell Dev. Biol. 10, 826053. doi:10.3389/fcell.2022.826053
Zhang, S., Wang, Y., Li, J., Zhong, Q., and Li, Y. (2021). MiR-101 inhibits migration and invasion of trophoblast HTR-8/SVneo cells by targeting CXCL6 in preeclampsia. Minerva Medica 112, 302–303. doi:10.23736/S0026-4806.19.06225-6
Zhang, W., Zhao, X., and Li, L. (2022b). Downregulation of circ_0001578 promotes gestational diabetes mellitus by inducing placental inflammation via the NF-κB and JNKs pathways. Front. Endocrinol. 13, 657802. doi:10.3389/fendo.2022.657802
Zhang, Y., Liu, X., Jia, J., Wang, T., Li, L., and Zhou, R. (2020). A potential role for CXCR2 in early-onset preeclampsia: placental CXCR2 expression is related to increased blood pressure and serum LDH levels. Maternal-Fetal Med. 2, 148–153. doi:10.1097/FM9.0000000000000050
Zhou, H., Wang, H., Liu, X., Liu, B., Che, Y., and Han, R. (2023). Downregulation of miR-92a in decidual stromal cells suppresses migration ability of trophoblasts by promoting macrophage polarization. DNA Cell Biol. 42, 507–514. doi:10.1089/dna.2022.0510
Zlotnik, A., and Yoshie, O. (2000). Chemokines: a new classification system and their role in immunity. Immunity 12, 121–127. doi:10.1016/s1074-7613(00)80165-x
Zou, Y., Jiang, Z., Yu, X., Zhang, Y., Sun, M., Wang, W., et al. (2014). MiR-101 regulates apoptosis of trophoblast HTR-8/SVneo cells by targeting endoplasmic reticulum (ER) protein 44 during preeclampsia. J. Hum. Hypertens. 28, 610–616. doi:10.1038/jhh.2014.35
Glossary
Keywords: preeclampsia, gestational diabetes mellitus, CXC chemokines, fractalkine (CX3CL1), inflammation, therapeutic discoveries
Citation: Ullah A, Zhao J, Singla RK and Shen B (2023) Pathophysiological impact of CXC and CX3CL1 chemokines in preeclampsia and gestational diabetes mellitus. Front. Cell Dev. Biol. 11:1272536. doi: 10.3389/fcell.2023.1272536
Received: 04 August 2023; Accepted: 09 October 2023;
Published: 19 October 2023.
Edited by:
Rodrigo Azevedo Loiola, Oroxcell, FranceReviewed by:
Olga Lilia Garibay-Cerdenares, Autonomous University of Guerrero, MexicoThoihen Meitei Heikrujam, University at Buffalo, United States
Gustavo Henrique Oliveira Da Rocha, Fraunhofer Institute for Cell Therapy and Immunology (IZI), Germany
Copyright © 2023 Ullah, Zhao, Singla and Shen. This is an open-access article distributed under the terms of the Creative Commons Attribution License (CC BY). The use, distribution or reproduction in other forums is permitted, provided the original author(s) and the copyright owner(s) are credited and that the original publication in this journal is cited, in accordance with accepted academic practice. No use, distribution or reproduction is permitted which does not comply with these terms.
*Correspondence: Bairong Shen, bairong.shen@scu.edu.cn