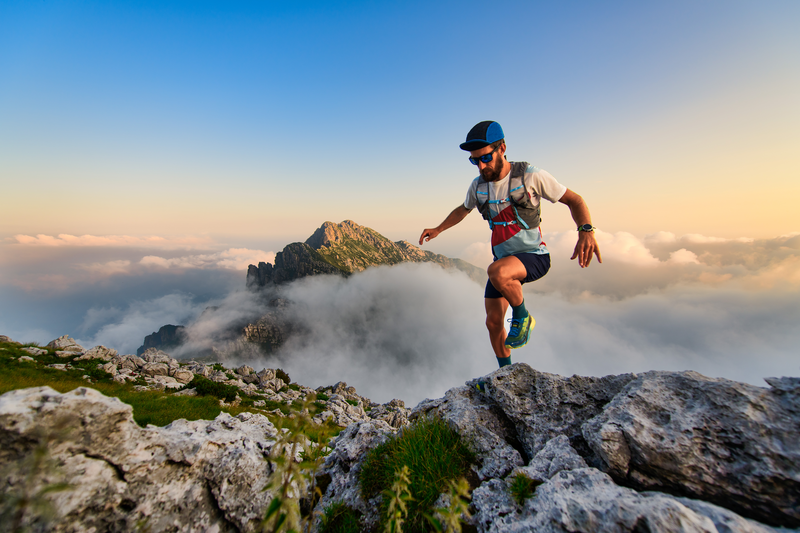
94% of researchers rate our articles as excellent or good
Learn more about the work of our research integrity team to safeguard the quality of each article we publish.
Find out more
MINI REVIEW article
Front. Cell Dev. Biol. , 12 October 2023
Sec. Molecular and Cellular Pathology
Volume 11 - 2023 | https://doi.org/10.3389/fcell.2023.1271014
This article is part of the Research Topic Precise Diagnosis of Acute and Chronic Wound Repair View all 4 articles
Poor palatal wound healing after cleft palate repair could lead to unfavorable prognosis such as oronasal fistula (ONF), which might affect the patient’s velopharyngeal function as well as their quality of life. Thus, restoring poor palatal wound healing for avoiding the occurrence of ONF should be considered the key to postoperative care after cleft palate repair. This review provided current insights in the preclinical study of poor palatal wound healing after cleft palate repair. This review comprehensively introduced the animal model establishment for palatal wound healing and related ONF, including the models by mice, rats, piglets, and dogs, and then demonstrated the aspects for investigating poor palatal wound healing and related treatments, including possible signaling pathways that could be involved in the formation of poor palatal wound healing, the related microbiota changes because of the deformity of palatal structure, and the studies for potential therapeutic strategies for palatal wound healing and ONF. The purpose of this review was to show the state of the art in preclinical studies about palatal wound healing after cleft palate repair and to show the promising aspects for better management of palatal wound healing.
Palatal wound healing happened after cleft palate repair and trauma and tumor excision (Majid, 2008; Sahoo et al., 2016; Alonso et al., 2019). Poor palatal wound healing could lead to oronasal fistula (ONF), resulting from extensive tension, infection, or hematoma (Hu and Rousso Joseph, 2020; Pollard et al., 2020). Oronasal fistula (ONF) was a common complication after cleft palate repair, which manifested as abnormal communication between oral and nasal cavities ranging from the alveolar process to the uvula (Honnebier et al., 2000; Mahajan et al., 2018; Alonso et al., 2019; Pollard et al., 2020), which could cause food reflux, unclear pronunciation and impaired velopharyngeal function, which affected the patient’s quality of life (Mahajan et al., 2018; Garg et al., 2019; Huang et al., 2023b). The incidence of ONF after cleft palate repair was up to 55% and the recurrence rate of ONF was up to 43% due to the cleft width, Veau types, and surgical techniques (Shankar et al., 2018; Sakran et al., 2022; Sakran et al., 2023a; Sakran et al., 2023b; Sakran et al., 2023c; Huang et al., 2023a). Thus, prevention of poor palatal wound healing should be an important mission to be completed.
In the situation of cleft palate repair, how to avoid poor palatal wound healing was always confusing, and the solutions were limited. Relaxing incision could help decrease the happening of poor palatal wound healing but might lead to further problems like inhibition of the maxillofacial growth (Becker and Hansson, 2013; Ogata et al., 2017; Mahajan et al., 2018; Liu et al., 2022). Thus, figuring out novel therapeutic strategies for preventing poor palatal wound healing and after cleft palate repair should be important in this field, and preclinical studies were essential. In this review, we first introduced the animal models that could be used for studying palatal wound healing, and then discussed current insights into the current aspects in the cause of poor palatal wound healing and related potential therapeutic strategies.
To understand palatal wound healing after cleft palate repair, animal studies were necessary. Here we showed the animal models that had been used in Table 1.
Take the approach of Ballestas et al. (2019) as an example (Figures 1A1–A3). An ophthalmologic cautery was used to create a 1.5-mm full-thickness mucosal injury on the hard palate. A similar approach was reported as well (Keswani et al., 2013). The differences were in the choice of mice and the tools used to create palatal wounds. A cautery excise was used to create a 1.5-mm full-thickness mucosal wound on the hard palate. In subsequent experiments, the formation of ONF was also confirmed by observing histological sections.
FIGURE 1. Studies on poor palatal wound healing and oronasal fistula after cleft palate repair (A) Animal models for studying poor palatal wound healing and oronasal fistula: (A1–A3) Changes in palatal wound in mice created with cautery over 7 days, (A1) Palatal wound on day 3, (A2) Palatal wound on day 5, (A3) Palatal wound on day 7 (Ballestas et al., 2019); (A4) Oronasal fistula on piglet’s palate 2 weeks after creation (Kesting et al., 2010); (A5) Four full-thickness defects on dog’s palate, mucosa and periosteum were removed and the palatal bone was exposed (Ayvazyan et al., 2011). (B) Common sites of ONF: (B1) A small ONF located at the anterior palate; (B2) A large ONF located at the anterior palate; (B3) ONF with alveolar cleft; (B4) ONF located at the junction of the hard and soft palate. (C) Mechanical force-related signaling pathways: Related signaling pathways activated by mechanotransduction were the Gridin/AKT signaling pathway, the TGF-β signaling pathway, the hippo signaling pathway and the RhoA/ROCK pathway (Created with BioRender.com). (D) The process of palatal wound healing: The process of palatal wound healing includes stages of hemostasis, inflammatory response, proliferation, and remodeling (Created with BioRender.com). (E) Four ways to reduce the inflammatory response by inhibiting NF-kB pathways, including inhibition of IKK kinase activity, inhibition of protease activity such as the 26S proteasome complex, inhibition of nuclear translocation prevented RelA and p50 from entering the nucleus, and inhibition of DNA binding to prevent the transcription process (Liu et al., 2017). (F) MMP-1 expression was higher in the group injected with Lactobacillus reuteri extracts (test group) on days 3 and 5 (F2, F4) than in the group injected with 0.9% NaCl (NS group) (F1, F3) (Han et al., 2019).
A 3-mm biopsy punch was used to create a standardized circular wound on the central area of the hard palate, exposing the bare bone (Zhu et al., 2015). The wounds of the rats were recorded with stereoscopic microscope images 1 week after surgery.
A 10-mm stainless steel biopsy punch was used to create a full-thickness wound in the hard palate of piglets (Figure 1A4) (Kirschner et al., 2006). The edges of the bony palate and nasal septum were extended 2 mm to allow for mucosal reepithelialization about the periphery of the wounds. This method of creating wounds was also described in other publications, and the only difference is the size of the palatal wound (Kesting et al., 2010; Rohleder et al., 2013). ONF formation could be verified by morphological observation 2 weeks after the creation of the defect and was also verified when tissue sections were made for observation 5 weeks later.
A biopsy punch was used to create standardized full-thickness wounds in the central area of the hard palate (Figure 1A5) (Ayvazyan et al., 2011). A rasp was used to remove the mucosa and periosteum to expose the bone. Photographs and tissue sections made during the experiment verified the final formation of ONF.
Overall, the differences between these methods were the sizes of the wounds and the instruments used. The two key points in creating a palatal wound capable of forming a final ONF were wound size and controlling asphyxia due to excessive bleeding during the procedure. A wound that was too small would not form a poor palatal healing. A wound that was too large would lead to difficulty in feeding, which would lead to excessive mortality and would not be conducive to subsequent experiments. The choice of instruments would determine the amount of intraoperative bleeding, which could affect death due to asphyxia. Currently, there were two main instruments, biopsy punch, and ophthalmologic cautery. The ophthalmic cautery was superior to the biopsy punch in reducing intraoperative bleeding because of its ability to stop bleeding at high temperatures, but it lacked in controlling the size of the wound and needed to be supplemented with other measuring tools.
As preventing poor palatal wound healing after cleft palate repair was the key to avoid ONF (Hu and Rousso Joseph, 2020; Wu et al., 2020; Li et al., 2021), the mechanisms for causing poor palatal wound healing should be explored. Extensive tension and infection have been found that contribute to the relatively high rate of poor wound healing (Ha et al., 2020; Hu and Rousso Joseph, 2020).
Clinically, a common site of poor palatal wound healing was the anterior palate, mainly due to the anatomical complexity of this area, particularly on the nasal side, where an inadequate issue often led to the failure of closure (Figures 1B1–B3). Another common site was the junction of the hard palate and soft palate, a site often considered to be the area of greatest tension (Figure 1B4) (Raoul and Ferri, 2007; Garg et al., 2019; Ha et al., 2020).Thus adequate tension reduction was the most crucial point in preventing poor palatal wound healing and tension-free suturing was a fundamental principle of cleft palate surgery (Agir et al., 2015; Elsherbiny and Grant, 2017).
Excessive tissue tension was an excessive mechanical force on the wound, which could affect wound healing (Huang et al., 2023a).The healing process of the oral mucosa included stages of hemostasis, inflammatory response, proliferation, and maturation (Guo and DiPietro, 2010). Excessive tissue tension might affect the proliferation and maturation stages. During the proliferation and maturation stages, the extracellular matrix (ECM) played a crucial role and was fundamental to the formation and function of soft connective tissues, which was affected by mechanical force (Leoni et al., 2015). Thus, we assumed that mechanotransduction could play an essential role in poor palatal wound healing after cleft palate repair.
The study about mechanotransduction in poor palatal wound healing was lacking, but we introduced some potential mechanisms that could be involved in poor palatal wound healing that need further investigation (Figure 1C). Transmembrane receptors on the cell membrane, mainly integrins, linked the extracellular to the intracellular, and intracellular integrin-linked proteins attached integrins to the cytoskeleton (CSK), and transmitted mechanical loads or signals within the cell (Hynes, 1992; Yin et al., 2022). The CSK stimulated by mechanical force went through complex morphological and biochemical changes and could trigger the transduction of mechanical signals into the cell (Hynes, 1992; Yin et al., 2022).
Related signaling pathways activated by CSK alterations were the Gridin/AKT signaling pathway, the TGF-β signaling pathway, the hippo signaling pathway, and the RhoA/ROCK pathway. It was found that Gridin family proteins were involved in regulating the activation of signaling pathways due to mechanical force (Enomoto et al., 2005), initiating the activation of the downstream signaling molecule Akt (ENOMOTO et al., 2006), and causing cytoskeletal reorganization (Bolcato-Bellemin et al., 2000a). Activated Transforming growth factor β1 (TGF-β1) induced the differentiation of epithelial cells into fibroblasts, inhibited collagenase release, and induced fibrillogenic effect (Bolcato-Bellemin et al., 2000b). Hippo signaling was an evolutionarily conserved pathway that controlled organ size by regulating cell proliferation, apoptosis, and stem cell self-renewal. YAP and TAZ were the two main downstream effector molecules of this pathway, which could induce the expression of genes that promoted cell proliferation and inhibited apoptosis (Toma et al., 2021).
Another common cause of poor palatal wound healing after the cleft palate repair was infection (Figure 1D). Sadhu et al. have reported that postoperative infection caused poor palatal wound healing, especially in adults (Sadhu, 2009). A methicillin-resistant Staphylococcus aureus infection with untreated candidiasis leading to poor palatal wound healing has also been reported (Palla et al., 2019). Unfortunately, the studies did not release the relationship between these infections and poor palatal wound healing in detail.
Infection could lead to inflammation, and inflammation could make wound healing more difficult and eventually lead to ONF. Thus, investigations on the mechanisms of infection and inflammation on poor palatal wound healing should be carried out and can be the potential therapeutic target for preventing poor palatal wound healing. Although the study about the roles and mechanisms of infection and inflammation in poor palatal wound healing was lacking, we introduced some potential mechanisms that could be involved in poor palatal wound healing.
In the inflammatory response, macrophages acted as the dominant cell type during the inflammatory phase of oral wound healing (Koh and DiPietro, 2011). M1 macrophages secreted cytokines, including interleukin-1 (IL-1), interleukin-6 (IL-6), fibroblast growth factor (FGF), platelet-derived growth factor (PDGF), epidermal growth factor (EGF) and TGF-β(Politis et al., 2016; Huang et al., 2022), which coordinated the movement of keratinocytes and fibroblasts towards the wound surface (Gallucci et al., 2000; Eming et al., 2007a). M2 macrophages promoted proliferation and healing and continued to secrete interleukin-10 (IL-10). M2 macrophages also helped to upregulate endogenous anti-inflammatory cytokine levels and downregulated pro-inflammatory cytokine levels previously secreted at the wound surface (Eming et al., 2007b). Thus, abnormal inflammatory response could result in the formation of chronic wound and lead to ONF.
The oral mucosa was a barrier to microorganisms, and the oral cavity was a second habitat for microorganisms. Microorganisms played a crucial role in maintaining the dynamic balance, protecting the oral cavity, and preventing diseases (Wade, 2013). ONF was an abnormal structure in the oral cavity, which led to the abnormal airway structure and might alter the colonization of microflora in the oral cavity. We also presumed that specific microorganisms might colonize and secrete specific substances on the wound surface, leading to poor wound healing and the formation of ONF after cleft palate repair, making it more susceptible to associated diseases such as respiratory infections.
Related studies have focused on the relationship between cleft palate and dental caries (Cheng et al., 2007), periodontal disease (Costa et al., 2003; PERDIKOGIANNI et al., 2009), and changes in the oral microflora before and after cleft palate repair (Arief et al., 2005). Although no specific microbiological studies associated with palatal wound healing were found, the abnormal morphology and function of the oral cavity in patients with ONF created an oral environment that could be different from that of healthy individuals, just like cleft palate.
Therefore, these abnormal changes were likely to affect alterations in the number and composition of the oral microflora because of cleft and abnormal oral structure that could lead to the happening of postoperative poor palatal wound healing. These studies had a certain reference value. In a study on the oral microflora of infants with complete cleft lip and palate versus soft cleft palate, it was shown that infants with complete cleft lip and palate had a significant increase in the development of oral microflora with commensal and potentially pathogenic microorganisms (Machorowska-Pieniążek et al., 2017). Studies found that patients with cleft palate were more likely to develop periodontal disease, which was reflected in the higher plaque index, mild gingivitis, deeper periodontal pockets, and looseness of teeth (Costa et al., 2003; PERDIKOGIANNI et al., 2009). The correlation between cleft palate and caries was also reported, and it indicated that patients with cleft palate were more susceptible to caries (Cheng et al., 2007). However, another study demonstrated that the differences in the composition of the dental plaque microflora in patients with cleft palate had no statistical significance compared to healthy individuals (Funahashi et al., 2019). Changes in the oral microflora of cleft palate patients before and after surgery have also received attention. It was reported that the number of colonies was significantly lower in children with cleft palates after surgery (Arief et al., 2005).
Again, as described above in relation to the study of cleft palate and microflora, even though there was no study on poor palatal wound healing as well as ONF and related oral microflora, we could speculate that abnormal structures in the oral cavity of patients with ONF, such as oronasal connection, may predispose the patients to certain diseases, so it is important to figure out so that we can intervene further.
Nutrition of nerves, distribution of blood vessels and mechanical force could impact the normal palatal wound healing (Miron et al., 2017; Najeeb et al., 2017; Iglesias-Bartolome et al., 2018), so the drugs affecting these factors have been investigated.
During the wound healing process, platelets regulated the homeostasis phase through vascular obliteration and facilitated fibrin clot formation (Guo and DiPietro, 2010), and stimulated the proliferation and activation of cells involved in the healing process, such as fibroblasts, neutrophils, macrophages and stem cells. Growth factors such as platelet-derived growth factor (PDGF), adhesion molecules, and angiogenic factors were released and activated by platelets (Nurden, 2011). Therefore, platelet-rich fibrins (PRFs) regarded as a second-generation platelet concentrate was used to accelerate wound healing (Miron et al., 2016). It was demonstrated that PRFs could induce earlier complete wound epithelialization (Ozcan et al., 2017). In addition, PRFs contained an important component, fibrins, which acted as a bridging molecule that supplied a tridimensional matrix, so that fibroblasts and endothelial cells permeated within this fibrins network. Advanced platelet-rich fibrins (A-PRFs) also hasted palatal wound healing process in a prospective randomized study (Vanessa et al., 2020).
Recently, fingolimod hydrochloride (FTY720), an immunomodulatory drug was used to enhance the healing of palatal wound by increasing the accumulation of regenerating monocytes and macrophages on the wound surface (Awojoodu et al., 2013; Olingy et al., 2017). In animal studies of palatal wound healing, it was demonstrated that the use of FTY720 could promote wound healing and prevent poor palatal wound healing and the development of ONF after cleft palate repair (Ballestas et al., 2019). It also reported that FTY720 increased the expression of SOX2, which could increase the migration and proliferation of keratinocytes and leads to rapid wound healing (Ballestas et al., 2019). Studies have demonstrated that FTY720 reduced the expression of several pro-inflammatory interleukins, including IL-1, IL-4 and IL-6 and promoted the expression of the regenerative IL-10, which improved oral wound healing (King et al., 2013).
For palatal wounds where ONFs have developed, surgeons often used a three-layer repair surgery, with the extra layer allowing for as much tension-free suturing as possible (El-Kassaby et al., 2014; Emodi et al., 2018). In recent years, many studies have used an acellular dermal matrix (ADM) to promoting palatal wound healing and repair ONF(Agir et al., 2015; Cassi and Massei, 2015). The use of ADM was first reported by Kirschner in 2006 (Kirschner et al., 2006). The ADM acted as a scaffold for host fibroblast migration and retained its basement membrane complex to facilitate attachment of the surface epithelium (Zhang et al., 2014). At the same time, it could solve the problems of insufficient tissue and scar formation (Agir et al., 2015). A study reported the use of collagen membrane to aid the closure of ONF and showed the effectiveness of good results of this surgery in repairing large ONF(Atherton and Boorman, 2016). However, it has been reported that it could not promote mucoperiosteum regeneration (Srivastava et al., 2001; Ophof et al., 2004; Liu et al., 2023).
Electrical stimulation (ES) has been applied to treat many chronic wounds and some other diseases, showing promising results. In dentistry, it has been investigated in periodontitis (Kaynak et al., 2005; Tomofuji et al., 2013), orthodontic tooth movement (Qamruddin et al., 2017; Mistry et al., 2020) and bony defects (Kaynak et al., 2005). One study on the ES effect on the palate wound healing reported that it could accelerate the process of palate wound healing, especially in the early stages (Ferreira et al., 2021). The results showed that the level of IL-6, IL-10, TNF-α and VEGF was lower than the sham ES group (Ferreira et al., 2021). However, the mechanisms by which cells respond to ES were not figured out. One possible pathway to explain the reduced inflammation by ES could be the NF-kB suppression activity (Figure 1E) (Kaur et al., 2011; Liu et al., 2017). According to the NF-kB signaling, ES could contribute to increased angiogenesis, collagen matrix formation, contraction of the wound and reepithelization during the proliferative phase (Hunckler and De Mel, 2017).
The balance of oral pathogenic bacteria and probiotics also played a crucial role in maintaining the healthy status of the human host. It seemed that adjusting the balance of oral microflora was an effective way to promote wound healing. It was reported that the wound healing process was delayed due to the disturbances of microflora adhesion on the wound (Lindley et al., 2016). MSCs were considered a promising method that might promote tissue regeneration and wound healing due to the potential of multilineage differentiation and self-renewal properties (Park et al., 2018; Tsai et al., 2018). A study reported that the imbalanced oral microflora could impair the proliferation capability of mesenchymal stem cells (MSCs) and delay palatal wound healing (Figure 1F1–F4) (Han et al., 2019). Lactobacillus reuteri extracts were found to activate the potentials of GMSCs and enhance the wound healing process by regulating the PI3K/AKT/β-catenin/TGFβ1 pathway (Han et al., 2019). However, these studies only illustrated that unbalanced microflora could delay wound healing but did not show that balanced microflora would promote wound healing. A study revealed that the balance between oral pathogenic bacteria and probiotics could activate the functions of MSCs, which provided promising ideas and methods for the prevention and treatment of oral diseases (Han et al., 2020).
Poor wound healing after cleft palate repair would lead to ONF. In this review, we introduced the animal models for studying palatal wound healing and demonstrated the promising aspects for better managing palatal wound healing. Future research will focus on the molecular mechanisms of poor palatal wound healing, followed by the development of new materials and treatments.
JC: Data curation, Investigation, Methodology, Resources, Writing–original draft, Writing–review and editing. RY: Data curation, Formal Analysis, Methodology, Writing–original draft. JY: Data curation, Methodology, Resources, Writing–original draft. BS: Conceptualization, Supervision, Writing–original draft, Writing–review and editing. HH: Conceptualization, Funding acquisition, Investigation, Supervision, Validation, Writing–original draft, Writing–review and editing.
The author(s) declare financial support was received for the research, authorship, and/or publication of this article. This work was supported by the National Natural Science Foundation of China (82301148), the Research and Development Program, West China Hospital of Stomatology, Sichuan University (RD-02-202107), Sichuan Province Science and Technology Support Program (2022NSFSC0743), and Sichuan Postdoctoral Science Foundation (TB2022005).
The authors declare that the research was conducted in the absence of any commercial or financial relationships that could be construed as a potential conflict of interest.
All claims expressed in this article are solely those of the authors and do not necessarily represent those of their affiliated organizations, or those of the publisher, the editors and the reviewers. Any product that may be evaluated in this article, or claim that may be made by its manufacturer, is not guaranteed or endorsed by the publisher.
Agir, H., Eren, G. G., and Yasar, E. K. (2015). Acellular dermal matrix use in cleft palate and palatal fistula repair: a potential benefit? J. Craniofacial Surg. 26, 1517–1522. doi:10.1097/SCS.0000000000001814
Alonso, V., Abuin, A. S., Duran, C., Gomez, O., Miguez, L., and Molina, M. E. (2019). Three-layered repair with a collagen membrane and a mucosal rotational flap reinforced with fibrine for palatal fistula closure in children. Int. J. Pediatr. Otorhinolaryngol. 127, 109679. doi:10.1016/J.IJPORL.2019.109679
Arief, E. M., Mohamed, Z., and Idris, F. M. (2005). Study of viridans streptococci and Staphylococcus species in cleft lip and palate patients before and after surgery. Cleft Palate Craniofacial J. 42, 277–279. doi:10.1597/04-083r.1
Atherton, D. D., and Boorman, J. G. (2016). Use of a purified collagen membrane to aid closure of palatal fistulae. J. Plastic, Reconstr. Aesthetic Surg. 69, 1003–1007. doi:10.1016/j.bjps.2016.02.009
Ayvazyan, A., Morimoto, N., Kanda, N., Takemoto, S., Kawai, K., Sakamoto, Y., et al. (2011). Collagen-gelatin scaffold impregnated with bFGF accelerates palatal wound healing of palatal mucosa in dogs. J. Surg. Res. 171, e247–e257. doi:10.1016/j.jss.2011.06.059
Awojoodu, A. O., Ogle, M. E., Sefcik, L. S., Bowers, D. T., Martin, K., Brayman, K. L., et al. (2013). Sphingosine 1-phosphate receptor 3 regulates recruitment of anti-inflammatory monocytes to microvessels during implant arteriogenesis. Proc Natl Acad Sci U S A. 110 (34), 13785–90. doi:10.1073/pnas.1221309110
Ballestas, S. A., Turner, T. C., Kamalakar, A., Stephenson, Y. C., Willett, N. J., Goudy, S. L., et al. (2019). Improving hard palate wound healing using immune modulatory autotherapies. Acta Biomater. 91, 209–219. doi:10.1016/J.ACTBIO.2019.04.052
Becker, M., and Hansson, E. (2013). Low rate of fistula formation after Sommerlad palatoplasty with or without lateral incisions: an analysis of risk factors for formation of fistulas after palatoplasty. J. plastic, Reconstr. aesthetic Surg. 66, 697–703. doi:10.1016/j.bjps.2013.01.031
Bolcato-Bellemin, A. L., Elkaim, R., Abehsera, A., Fausser, J. L., Haikel, Y., and Tenenbaum, H. (2000a). Expression of mRNAs encoding for alpha and beta integrin subunits, MMPs, and TIMPs in stretched human periodontal ligament and gingival fibroblasts. J. Dent. Res. 79, 1712–1716. doi:10.1177/00220345000790091201
Bolcato-Bellemin, A. L., Elkaim, R., Abehsera, A., Fausser, J. L., Haikel, Y., and Tenenbaum, H. (2000b). Expression of mRNAs encoding for alpha and beta integrin subunits, MMPs, and TIMPs in stretched human periodontal ligament and gingival fibroblasts. J. Dent. Res. 79, 1712–1716. doi:10.1177/00220345000790091201
Cassi, L. C., and Massei, A. (2015). The use of acellular dermal matrix in the closure of oronasal fistulae after cleft palate repair. Plastic Reconstr. Surgery–Global Open 3, e341. doi:10.1097/GOX.0000000000000301
Cheng, L. L., Moor, S. L., and Ho, C. T. C. (2007). Predisposing factors to dental caries in children with cleft lip and palate: a review and strategies for early prevention. Cleft Palate Craniofacial J. 44, 67–72. doi:10.1597/05-112
Costa, B., de Oliveira Lima, J., Gomide, M. R., and Pereira da Silva Rosa, O. (2003). Clinical and microbiological evaluation of the periodontal status of children with unilateral complete cleft lip and palate. Cleft Palate Craniofacial J. 40, 585–589. doi:10.1597/01-083
El-Kassaby, M. A., Khalifah, M. A. A.-J., Metwally, S. A., and Abd ElKader, K. A. E. (2014). Acellular dermal matrix allograft: an effective adjunct to oronasal fistula repair in patients with cleft palate. Ann. Maxillofac. Surg. 4, 158–161. doi:10.4103/2231-0746.147108
Eming, S. A., Werner, S., Bugnon, P., Wickenhauser, C., Siewe, L., Utermöhlen, O., et al. (2007a). Accelerated wound closure in mice deficient for interleukin-10. Am. J. Pathol. 170, 188–202. doi:10.2353/AJPATH.2007.060370
Eming, S. A., Werner, S., Bugnon, P., Wickenhauser, C., Siewe, L., Utermöhlen, O., et al. (2007b). Accelerated wound closure in mice deficient for interleukin-10. Am. J. Pathol. 170, 188–202. doi:10.2353/ajpath.2007.060370
Emodi, O., Ginini, J. G., van Aalst, J. A., Shilo, D., Naddaf, R., Aizenbud, D., et al. (2018). Cleft palate fistula closure utilizing acellular dermal matrix. Plast. Reconstr. Surg. Glob. Open 6, e1682. doi:10.1097/GOX.0000000000001682
Enomoto, A., Murakami, H., Asai, N., Morone, N., Watanabe, T., Kawai, K., et al. (2005). Akt/PKB regulates actin organization and cell motility via girdin/APE. Dev. Cell 9, 389–402. doi:10.1016/j.devcel.2005.08.001
Enomoto, A., Ping, J., and Takahashi, M. (2006). Girdin, a novel actin-binding protein, and its family of proteins possess versatile functions in the Akt and wnt signaling pathways. Ann. N. Y. Acad. Sci. 1086, 169–184. doi:10.1196/annals.1377.016
Elsherbiny, A., and Grant, J. H. (2017). Total palatal mobilization and multilamellar suturing technique improves outcome for palatal fistula repair. Ann Plast Surg. 79 (6), 566–570. doi:10.1097/SAP.0000000000001216
Ferreira, C. L., Neves Jardini, M. A., Moretto Nunes, C. M., Bernardo, D. V., Viana Casarin, R. C., dos Santos Gedraite, E., et al. (2021). Electrical stimulation enhances early palatal wound healing in mice. Arch. Oral Biol. 122, 105028. doi:10.1016/J.ARCHORALBIO.2020.105028
Funahashi, K., Shiba, T., Watanabe, T., Muramoto, K., Takeuchi, Y., Ogawa, T., et al. (2019). Functional dysbiosis within dental plaque microbiota in cleft lip and palate patients. Prog. Orthod. 20, 11. doi:10.1186/s40510-019-0265-1
Gallucci, R. M., Simeonova, P. P., Matheson, J. M., Kommineni, C., Guriel, J. L., Sugawara, T., et al. (2000). Impaired cutaneous wound healing in interleukin-6-deficient and immunosuppressed mice. FASEB J. 14, 2525–2531. doi:10.1096/fj.00-0073com
Garg, R., Shah, S., Uppal, S., and Mittal, R. K. (2019). A statistical analysis of incidence, etiology, and management of palatal fistula. Natl. J. Maxillofac. Surg. 10, 43–46. doi:10.4103/njms.NJMS_42_18
Guo, S., and DiPietro, L. A. (2010). Factors affecting wound healing. J. Dent. Res. 89, 219–229. doi:10.1177/0022034509359125
Ha, J. H., Jeong, Y., Koo, Y. T., Jeon, S., Chung, J., and Kim, S. (2020). Effect of collagen matrix on postoperative palatal fistula in cleft palate repair. Sci. Rep. 10, 15236. doi:10.1038/s41598-020-72046-y
Han, N., Jia, L., Guo, L., Su, Y., Luo, Z., Du, J., et al. (2020). Balanced oral pathogenic bacteria and probiotics promoted wound healing via maintaining mesenchymal stem cell homeostasis. Stem Cell Res. Ther. 11, 61. doi:10.1186/s13287-020-1569-2
Han, N., Jia, L., Su, Y., Du, J., Guo, L., Luo, Z., et al. (2019). Lactobacillus reuteri extracts promoted wound healing via PI3K/AKT/β-catenin/TGFβ1 pathway. Stem Cell Res. Ther. 10, 243. doi:10.1186/s13287-019-1324-8
Honnebier, M. B. O. M., Johnson, D. S., Parsa, A. A., Dorian, A., and Parsa, F. D. (2000). Closure of palatal fistula with a local mucoperiosteal flap lined with buccal mucosal graft. Cleft Palate Craniofacial J. 37, 127–129. doi:10.1597/1545-1569_2000_037_0127_copfwa_2.3.co_2
Hu, J., and Rousso Joseph, J. S. L. (2020). Revision surgery of the cleft palate. Semin. Plast. Surg. 34, 120–128. doi:10.1055/s-0040-1709429
Huang, H., Li, J., Li, C., Yin, H., Wu, M., Wang, Y., et al. (2023a). Sommerlad–Furlow modified palatoplasty: a retrospective study. J. Cranio-Maxillofacial Surg. 51, 238–245. doi:10.1016/J.JCMS.2023.04.004
Huang, H., Pan, W., Wang, Y., Kim, H. S., Shao, D., Huang, B., et al. (2022). Nanoparticulate cell-free DNA scavenger for treating inflammatory bone loss in periodontitis. Nat. Commun. 13, 5925. doi:10.1038/s41467-022-33492-6
Huang, H., Zheng, O., Wang, D., Yin, J., Wang, Z., Ding, S., et al. (2023b). ChatGPT for shaping the future of dentistry: the potential of multi-modal large language model. Int. J. Oral Sci. 15, 29. doi:10.1038/s41368-023-00239-y
Hunckler, J., and De Mel, A. (2017). A current affair: electrotherapy in wound healing. J. Multidiscip. Healthc. 10, 179–194. doi:10.2147/JMDH.S127207
Hynes, R. O. (1992). Integrins: versatility, modulation, and signaling in cell adhesion. Cell 69, 11–25. doi:10.1016/0092-8674(92)90115-S
Iglesias-Bartolome, R., Uchiyama, A., Molinolo, A. A., Abusleme, L., Brooks, S. R., Callejas-Valera, J. L., et al. (2018). Transcriptional signature primes human oral mucosa for rapid wound healing. Sci. Transl. Med. 10, eaap8798. doi:10.1126/scitranslmed.aap8798
Kaur, S., Lyte, P., Garay, M., Liebel, F., Sun, Y., Liu, J.-C., et al. (2011). Galvanic zinc–copper microparticles produce electrical stimulation that reduces the inflammatory and immune responses in skin. Arch. Dermatol Res. 303, 551–562. doi:10.1007/s00403-011-1145-9
Kaynak, D., Meffert, R., Günhan, M., and Günhan, Ö. (2005). A histopathologic investigation on the effects of electrical stimulation on periodontal tissue regeneration in experimental bony defects in dogs. J. Periodontol. 76, 2194–2204. doi:10.1902/jop.2005.76.12.2194
Kesting, M. R., Loeffelbein, D. J., Classen, M., Slotta-Huspenina, J., Hasler, R. J., Jacobsen, F., et al. (2010). Repair of oronasal fistulas with human amniotic membrane in minipigs. Br. J. Oral Maxillofac. Surg. 48, 131–135. doi:10.1016/j.bjoms.2009.04.025
Keswani, S. G., Balaji, S., Le, L. D., Leung, A., Parvadia, J. K., Frischer, J., et al. (2013). Role of salivary vascular endothelial growth factor (VEGF) in palatal mucosal wound healing. Wound Repair Regen. 21, 554–562. doi:10.1111/wrr.12065
King, A., Balaji, S., Le, L. D., Crombleholme, T. M., and Keswani, S. G. (2013). Regenerative wound healing: the role of interleukin-10. Adv. Wound Care (New Rochelle) 3, 315–323. doi:10.1089/wound.2013.0461
Kirschner, R. E., Cabiling, D. S., Slemp, A. E., Siddiqi, F., LaRossa, D. D., and Losee, J. E. (2006). Repair of oronasal fistulae with acellular dermal matrices. Plast. Reconstr. Surg. 118, 1431–1440. doi:10.1097/01.prs.0000239612.35581.c3
Koh, T. J., and DiPietro, L. A. (2011). Inflammation and wound healing: the role of the macrophage. Expert Rev. Mol. Med. 13, e23. doi:10.1017/S1462399411001943
Leoni, G., Neumann, P.-A., Sumagin, R., Denning, T. L., and Nusrat, A. (2015). Wound repair: role of immune–epithelial interactions. Mucosal Immunol. 8, 959–968. doi:10.1038/mi.2015.63
Li, Y., Wu, M., Yang, C., Tsauo, C., Li, C., Liu, R., et al. (2021). Evaluation of fistula rates in three cleft palate techniques without relaxing incisions. J. Cranio-Maxillofacial Surg. 49, 456–461. doi:10.1016/j.jcms.2021.01.022
Lindley, L. E., Stojadinovic, O., Pastar, I., and Tomic-Canic, M. (2016). Biology and biomarkers for wound healing. Plast. Reconstr. Surg. 138, 18S–28S. doi:10.1097/PRS.0000000000002682
Liu, T., Zhang, L., Joo, D., and Sun, S.-C. (2017). NF-κB signaling in inflammation. Signal Transduct. Target Ther. 2, 17023. doi:10.1038/sigtrans.2017.23
Liu, Y., Zhang, S., Sakran, K. A., Yin, J., Lan, M., Yang, C., et al. (2022). Observation of palatal wound healing process following various degrees of mucoperiosteal and bone trauma in a young rat model. Biol. (Basel) 11, 1142. doi:10.3390/biology11081142
Liu, Y., Zhang, S., Sakran, K. A., Yin, J., Lan, M., Yang, C., et al. (2023). Observation of palatal wound healing process following various degrees of mucoperiosteal and bone trauma in a young rat model. Biol. (Basel) 11, 1142. doi:10.3390/biology11081142
Machorowska-Pieniążek, A., Mertas, A., Skucha-Nowak, M., Tanasiewicz, M., and Morawiec, T. (2017). A comparative study of oral microbiota in infants with complete cleft lip and palate or cleft soft palate. Biomed. Res. Int. 2017, 1460243. doi:10.1155/2017/1460243
Mahajan, A., Singh, S. M., and Kumar Prakash, R. K. K. (2018). A retrospective analysis of incidence and management of palatal fistula. Indian J. Plastic Surg. 51, 298–305. doi:10.4103/ijps.IJPS_84_18
Majid, O. W. (2008). Persistent oronasal fistula after primary management of facial gunshot injuries. Br. J. Oral Maxillofac. Surg. 46, 50–52. doi:10.1016/j.bjoms.2006.09.016
Miron, R. J., Fujioka-Kobayashi, M., Bishara, M., Zhang, Y., Hernandez, M., and Choukroun, J. (2016). Platelet-rich fibrin and soft tissue wound healing: a systematic review. Tissue Eng. Part B Rev. 23, 83–99. doi:10.1089/ten.teb.2016.0233
Miron, R. J., Zucchelli, G., Pikos, M. A., Salama, M., Lee, S., Guillemette, V., et al. (2017). Use of platelet-rich fibrin in regenerative dentistry: a systematic review. Clin. Oral Investig. 21, 1913–1927. doi:10.1007/s00784-017-2133-z
Mistry, D., Dalci, O., Papageorgiou, S. N., Darendeliler, M. A., and Papadopoulou, A. K. (2020). The effects of a clinically feasible application of low-level laser therapy on the rate of orthodontic tooth movement: a triple-blind, split-mouth, randomized controlled trial. Am. J. Orthod. Dentofac. Orthop. 157, 444–453. doi:10.1016/j.ajodo.2019.12.005
Najeeb, S., Khurshid, Z., Agwan, M. A. S., Ansari, S. A., Zafar, M. S., and Matinlinna, J. P. (2017). Regenerative potential of platelet rich fibrin (PRF) for curing intrabony periodontal defects: a systematic review of clinical studies. Tissue Eng. Regen. Med. 14, 735–742. doi:10.1007/s13770-017-0079-5
Nurden, A. T. (2011). Platelets, inflammation and tissue regeneration. Thromb. Haemost. 105, S13–S33. doi:10.1160/THS10-11-0720
Ogata, H., Sakamoto, Y., and Kishi, K. (2017). Cleft palate repair without lateral relaxing incision. Plast. Reconstr. Surg. Glob. Open 5, e1256. doi:10.1097/GOX.0000000000001256
Olingy, C. E., San Emeterio, C. L., Ogle, M. E., Krieger, J. R., Bruce, A. C., and Pfau, D. D. (2017). Non-classical monocytes are biased progenitors of wound healing macrophages during soft tissue injury. Sci Rep. 7 (1), 447. doi:10.1038/s41598-017-00477-1
Ophof, R., Maltha, J. C., Von Den Hoff, J. W., and Kuijpers-Jagtman, A.-M. (2004). Histologic evaluation of skin-derived and collagen-based substrates implanted in palatal wounds. Wound Repair Regen. 12, 528–538. doi:10.1111/j.1067-1927.2004.012505.x
Ozcan, M., Ucak, O., Alkaya, B., Keceli, S., Seydaoglu, G., and Haytac, M. C. (2017). Effects of platelet-rich fibrin on palatal wound healing after free gingival graft harvesting: a comparative randomized controlled clinical trial. Int. J. Periodontics Restor. Dent. 37, e270–e278. doi:10.11607/prd.3226
Palla, S., Shekar, V., and Reddy, J. R. C. (2019). Methicillin-resistant Staphylococcus aureus superinfection over untreated candidiasis resulting in oronasal fistula. Indian J. Dermatol Venereol. Leprol. 87, 60–61. doi:10.4103/ijdvl.IJDVL_573_18
Park, S.-R., Kim, J.-W., Jun, H.-S., Roh, J. Y., Lee, H.-Y., and Hong, I.-S. (2018). Stem cell secretome and its effect on cellular mechanisms relevant to wound healing. Mol. Ther. 26, 606–617. doi:10.1016/j.ymthe.2017.09.023
Perdikogianni, H., Papaioannou, W., Nakou, M., Oulis, C., and Papagiannoulis, L. (2009). Periodontal and microbiological parameters in children and adolescents with cleft lip and/or palate. Int. J. Paediatr. Dent. 19, 455–467. doi:10.1111/j.1365-263X.2009.01020.x
Politis, C., Schoenaers, J., Jacobs, R., and Agbaje, J. O. (2016). Wound healing problems in the mouth. Front. Physiol. 7, 507. doi:10.3389/fphys.2016.00507
Pollard, S. H., Skirko, J. R., Dance, D., Reinemer, H., Yamashiro, D., Lyon, N. F., et al. (2020). Oronasal fistula risk after palate repair. Cleft Palate Craniofacial J. 58, 35–41. doi:10.1177/1055665620931707
Qamruddin, I., Alam, M. K., Mahroof, V., Fida, M., Khamis, M. F., and Husein, A. (2017). Effects of low-level laser irradiation on the rate of orthodontic tooth movement and associated pain with self-ligating brackets. Am. J. Orthod. Dentofac. Orthop. 152, 622–630. doi:10.1016/j.ajodo.2017.03.023
Raoul, G., and Ferri, J. (2007). Oronasal fistula in sequels of labialalveolarvelopalatine clefts. Rev. Stomatol. Chir. Maxillofac. 108, 321–328. doi:10.1016/j.stomax.2007.06.008
Rohleder, N. H., Loeffelbein, D. J., Feistl, W., Eddicks, M., Wolff, K.-D., Gulati, A., et al. (2013). Repair of oronasal fistulae by interposition of multilayered amniotic membrane allograft. Plast. Reconstr. Surg. 132, 172–181. doi:10.1097/PRS.0b013e3182910b50
Sadhu, P. (2009). Oronasal fistula in cleft palate surgery. Indian J. Plastic Surg. 42, S123–S128. doi:10.4103/0970-0358.57203
Sahoo, N. K., Desai, A. P., Roy, I. D., and Kulkarni, V. (2016). Oro-nasal communication. J. Craniofacial Surg. 27, e529–e533. doi:10.1097/SCS.0000000000002815
Sakran, K. A., Wu, M., Alkebsi, K., Mashrah, M. A., Al-Rokhami, R. K., Wang, Y., et al. (2023a). The sommerlad–furlow modified palatoplasty technique: postoperative complications and implicating factors. Laryngoscope 133, 822–829. doi:10.1002/lary.30385
Sakran, K. A., Wu, M., Yin, H., Wang, Y., Li, C., Alkebsi, K., et al. (2023b). Evaluation of postoperative outcomes in two cleft palate repair techniques without relaxing incisions. Plast. Reconstr. Surg., 10–1097. doi:10.1097/PRS.0000000000010230
Sakran, K. A., Yin, J., Yang, R., Alkebsi, K., Elayah, S. A., Al-Rokhami, R. K., et al. (2023c). Evaluation of late cleft palate repair by a modified technique without relaxing incisions. J. Stomatol. Oral Maxillofac. Surg. 124, 101403. doi:10.1016/j.jormas.2023.101403
Sakran, K. A., Yin, J., Yang, R., Elayah, S. A., Alkebsi, K., Zhang, S., et al. (2022). Early cleft palate repair by a modified technique without relaxing incisions. Cleft Palate-Craniofacial J., 105566562211352. doi:10.1177/10556656221135288
Shankar, V. A., Snyder-Warwick, A., Skolnick, G. B., Woo, A. S., and Patel, K. B. (2018). Incidence of palatal fistula at time of secondary alveolar cleft reconstruction. Cleft Palate Craniofacial J. 55, 999–1005. doi:10.1597/16-179
Srivastava, A., DeSagun, E. Z., Jennings, L. J., Sethi, S., Phuangsab, A., Hanumadass, M., et al. (2001). Use of porcine acellular dermal matrix as a dermal substitute in rats. Ann. Surg. 233, 400–408. doi:10.1097/00000658-200103000-00015
Suragimath, G., Krishnaprasad, K. R., Moogla, S., Sridhara, S. U., and Raju, S. (2010). Effect of carbonated drink on excisional palatal wound healing: a study on Wistar rats. Indian J. Dent. Res. 21, 330–333. doi:10.4103/0970-9290.70789
Toma, A. I., Fuller, J. M., Willett, N. J., and Goudy, S. L. (2021). Oral wound healing models and emerging regenerative therapies. Transl. Res. 236, 17–34. doi:10.1016/j.trsl.2021.06.003
Tomofuji, T., Ekuni, D., Azuma, T., Irie, K., Endo, Y., Kasuyama, K., et al. (2013). Effects of electrical stimulation on periodontal tissue remodeling in rats. J. Periodontal Res. 48, 177–183. doi:10.1111/j.1600-0765.2012.01518.x
Tsai, H.-W., Wang, P.-H., and Tsui, K.-H. (2018). Mesenchymal stem cell in wound healing and regeneration. J. Chin. Med. Assoc. 81, 223–224. doi:10.1016/j.jcma.2017.06.011
Vanessa, S., João, B., Luís, P., José João, M., and Alves Ricardo, F. M. (2020). Effect of A-PRF application on palatal wound healing after free gingival graft harvesting: a prospective randomized study. Eur. J. Dent. 14, 63–69. doi:10.1055/s-0040-1702259
Wade, W. G. (2013). The oral microbiome in health and disease. Pharmacol. Res. 69, 137–143. doi:10.1016/J.PHRS.2012.11.006
Wu, M., Zhu, Z.-B., Shi, B., Gong, C.-X., Zhang, B.-H., and Li, Y. (2020). Palatal fistula rate after Huaxi Sommerlad-Furlow palatoplasty. Hua Xi Kou Qiang Yi Xue Za Zhi 38, 166–169. doi:10.7518/hxkq.2020.02.010
Yin, J., Zhang, S., Yang, C., Wang, Y., Shi, B., Zheng, Q., et al. (2022). Mechanotransduction in skin wound healing and scar formation: potential therapeutic targets for controlling hypertrophic scarring. Front. Immunol. 13, 1028410. doi:10.3389/fimmu.2022.1028410
Zhang, B., Li, J., Sarma, D., Zhang, F., and Chen, J. (2014). The use of heterogeneous acellular dermal matrix in the closure of hard palatal fistula. Int. J. Pediatr. Otorhinolaryngol. 78, 75–78. doi:10.1016/J.IJPORL.2013.10.053
Keywords: mechanism, oronasal fistula, oral microflora, treatments, wound healing
Citation: Chen J, Yang R, Yin J, Shi B and Huang H (2023) Current insights in the preclinical study of palatal wound healing and oronasal fistula after cleft palate repair. Front. Cell Dev. Biol. 11:1271014. doi: 10.3389/fcell.2023.1271014
Received: 01 August 2023; Accepted: 03 October 2023;
Published: 12 October 2023.
Edited by:
Ramani Ramchandran, Medical College of Wisconsin, United StatesReviewed by:
Ming Cai, Shanghai Jiao Tong University, ChinaCopyright © 2023 Chen, Yang, Yin, Shi and Huang. This is an open-access article distributed under the terms of the Creative Commons Attribution License (CC BY). The use, distribution or reproduction in other forums is permitted, provided the original author(s) and the copyright owner(s) are credited and that the original publication in this journal is cited, in accordance with accepted academic practice. No use, distribution or reproduction is permitted which does not comply with these terms.
*Correspondence: Hanyao Huang, aHVhbmdoYW55YW9fY25Ac2N1LmVkdS5jbg==
†These authors have contributed equally to this work and share first authorship
Disclaimer: All claims expressed in this article are solely those of the authors and do not necessarily represent those of their affiliated organizations, or those of the publisher, the editors and the reviewers. Any product that may be evaluated in this article or claim that may be made by its manufacturer is not guaranteed or endorsed by the publisher.
Research integrity at Frontiers
Learn more about the work of our research integrity team to safeguard the quality of each article we publish.