- 1Department of Nephrology, Yueyang Hospital of Integrated Traditional Chinese and Western Medicine, Shanghai University of Traditional Chinese Medicine, Shanghai, China
- 2Department of Nephrology, Ruijin Hospital, Shanghai Jiao Tong University School of Medicine, Shanghai, China
- 3Department of Medicine, Shanghai Hospital of Civil Aviation Administration of China, Shanghai, China
Endocytosis is a crucial component of many pathological conditions. The proximal tubules are responsible for reabsorbing the majority of filtered water and glucose, as well as all the proteins filtered through the glomerular barrier via endocytosis, indicating an essential role in kidney diseases. Genetic mutations or acquired insults could affect the proximal tubule endocytosis processes, by disturbing or overstressing the endolysosomal system and subsequently activating different pathways, orchestrating renal fibrosis. This paper will review recent studies on proximal tubular endocytosis affected by other diseases and factors. Endocytosis plays a vital role in the development of renal fibrosis, and renal fibrosis could also, in turn, affect tubular endocytosis.
Introduction
Endocytosis is a process of internalizing extracellular material intimately connected to diverse cellular functions, including signal transduction, cytoskeleton structure, and transcriptional regulation (Ellinger and Pietschmann, 2016). Several endocytic routes exist in eukaryotic cells, mainly receptor-mediated endocytosis and fluid-phased endocytosis. Receptor-mediated endocytosis involves clathrin-mediated and clathrin-independent endocytosis. The major endocytic way to internalize many cargoes is clathrin-mediated endocytosis (Kaksonen and Roux, 2018; Briant et al., 2020). Although clathrin-mediated endocytosis occurs in several renal cell types, endocytosis can also occur by non-clathrin-coated vesicles, including caveolae that contain a coat protein, caveolin (Zhuang et al., 2011; Srivastav et al., 2019). In kidneys, different cell types involve different endocytic pathways with other cellular functions. Glomerular cells, including endothelial cells, mesangial cells, and podocytes, all have the function of endocytosis, whereas tubular epithelial cells and collecting duct cells also have the same function (Singhal et al., 2000; Prabakaran et al., 2012; Wang et al., 2014; Inoue and Ishibe, 2015; Peng et al., 2016; Martin et al., 2018; Moriyama et al., 2019). This review mainly focuses on the endocytosis of proximal tubular epithelial cells and its relationship with renal fibrosis.
The proximal tubules reabsorb most filtered water and glucose (Eshbach and Weisz, 2017). The S1 and S2 segments of the proximal tubules internalize all the proteins filtered through the glomerular filtration barrier, including albumin and low molecular weight (LMW) proteins (Polesel and Hall, 2019). These LMW proteins include hormones (PTH, insulin, EGF, leptin, thyroglobulin), vitamin carrier proteins (transcobalamin-vitamin B12, vitamin D-binding protein (DBP), retinol-binding protein, folate-binding protein), enzymes (cathepsin B, plasminogen, urokinase, lysozyme), lipoproteins, cell surface antigen components (β2-microglobulin), immunoglobulin light chains, as well as drugs and toxins (aminoglycosides, gentamicin) (van der Wijst et al., 2019). The megalin (encoded by the LRP2 gene), cubulin (encoded by the CUBN gene), and amnionless (encoded by AMN gene) complex, expressed at the brush border of the tubular epithelial cells, are the primary multi-ligand receptors in the receptor-mediated endocytosis in tubular epithelial cells (De et al., 2014). These receptors are constitutively internalized in clathrin-coated pits (CCPs) into small apical endocytic endosomes (AEEs) that fuse with large apical vacuoles (AVs) (Shipman and Weisz, 2020). The soluble contents of vacuoles are delivered to lysosomes, whereas membrane receptors, megalin, and cubilin are recycled to the apical surface (Smith et al., 2019; Shipman and Weisz, 2020).
Currently, endocytosis has been regarded as an essential component of many pathological conditions (Marzolo and Farfan, 2011). Increasing studies have focused on the role of tubular endocytosis in kidney disease development and progression. It is noted that various genetic mutations and acquired insults can affect PT endolysosomal function, leading to different cellular pathway activations and triggering proinflammatory and profibrotic responses, resulting in renal fibrosis (Dizin et al., 2013).
Dent Disease
Dent disease is a rare genetic proximal tubulopathy (Gianesello et al., 2021). Mutations in the CLCN5 and OCRL genes are known to cause Dent disease. Hoopes et al. first classified Dent Disease into three types, mutations in the CLCN5 gene as Dent Disease type 1, mutations in OCRL gene as Dent Disease type 2, and no mutations in either gene are categorized as Dent Disease type 3 (Hoopes et al., 2004).
Dent disease type 1 is a heterotypic X-linked disease caused by mutations in CLCN5, which encodes the electrogenic Cl−/H+ exchanger CLC-5, localized in early endosomes of the proximal tubule (PT) (Gianesello et al., 2020). Phenotypic features commonly include low molecular weight proteinuria (LMWP), hypercalciuria, focal global sclerosis, calcium nephrolithiasis, nephrocalcinosis, and renal failure (Gorvin et al., 2013; Blanchard et al., 2016; Anglani et al., 2019). Disruption of the mouse Clcn5 gene causes proteinuria by strongly reducing apical proximal tubular endocytosis, and both receptor-mediated and fluid-phase endocytosis and endosomal acidification are affected (Piwon et al., 2000). Reduced expressions of megalin and cubilin were also observed in Dent disease models (Christensen et al., 2003; Shipman and Weisz, 2020). Weisz et al. also discovered that decreased proximal tubule receptor expression and tubular proteinuria in Dent disease are primarily mediated by delayed early endosome maturation as a result of defective acidification and decreased [Cl -] accumulation (Shipman et al., 2023).
Lowe syndrome/Dent disease type 2 is caused by mutations in the phosphatidylinositol 4,5-bisphosphate (PtdIns4,5P(2)) 5-phosphatase OCRL, characterized by congenital cataracts, central hypotonia, and renal proximal tubular dysfunction (Suchy et al., 1995; De Matteis et al., 2017). Studies have shown that OCRL is localized to various endocytic compartments, suggesting impairments in the endocytic pathway as a possible disease mechanism (Sharma et al., 2015). Vicinanza et al. showed that OCRL controls early endosome (EE) function via its 5-phosphatase activity (Vicinanza et al., 2011). The further study analyzed that the defective receptor-mediated endocytosis was due to a megalin misplacement, in which it localizes in the endosomes instead of the brush border of the proximal tubular cells (Festa et al., 2019). Depletion of OCRL also impairs the recycling of multiple classes of receptors, including the megalin (Vicinanza et al., 2011). Using proteomic and metabonomic studies, Vilasi et al. demonstrated that tubular reabsorption of plasma-derived proteins was similarly reduced in Dent’s disease and Lowe syndrome, indicating that it is indistinguishable to identify Lowe syndrome and Dent’s disease (Vilasi et al., 2007).
Furthermore, Norden et al. demonstrated that tubular epithelial cells could not reabsorb a wide variety of polypeptides, hormones (insulin, growth hormone, insulin-like growth factor-1), and chemokines (monocyte chemoattractant protein-1) due to endocytosis impairment (Norden et al., 2001). Meanwhile, gene set enrichment analysis revealed that kidney development, anion homeostasis, organic acid transport, extracellular matrix organization, and cell migration biological processes are most likely involved in Dent Disease pathophysiology (Duran et al., 2021). Interestingly, in the presence of a defect in endocytosis, considerable compensatory reabsorption occurs further along the PT, presumably to limit the loss of proteins and their cargo into the urine (Polesel et al., 2022). These may partially imply the pathogenesis of tubulointerstitial fibrosis and may be one of the underlying mechanism contributing to the progressive renal failure in Dent disease (van Berkel et al., 2017).
Cystic fibrosis
Cystic fibrosis is an autosomal recessive disease. It is a potentially lethal multisystem disease resulting from the accumulation of thick mucus that obstructs the airways, pancreatic ducts, intestine, bile ducts, and genital tract (Rowe et al., 2005). The disease-causing gene CFTR encodes a chloride channel and is also expressed in endosomes of proximal tubule cells (Riordan et al., 1989). CFTR mutations cause a major trafficking defect of endocytosis in proximal tubule cells, resulting in lysosomal dysfunction, oxidative stress, and tubular dedifferentiation/proliferation (Devuyst and Luciani, 2015). Further studies indicated that CFTR expression was downregulated in the kidney tissues of UUO murine model and CKD patients. Dysfunction or downregulation of CFTR in renal epithelial cells leads to alteration of genes in volved in EMT and kidney fibrosis (Zhang et al., 2017). Taken together, CFTR may affect endocytotic pathways but also involve in other classical profibrotic pathways. These changes combined together may affect long-term renal function, triggering tubulointerstitial injury and chronic kidney disease (Devuyst and Luciani, 2015).
Cystinosis
Cystinosis is an autosomal recessive disease caused by mutations in the CTNS gene that encodes the lysosomal protein cystinosin, leading to an accumulation of cystine in all organs (Wilmer et al., 2010). Cystinosis could cause loss of expression of megalin/cubulin and impairment in endocytosis in PTCs(Gaide Chevronnay et al., 2014). Late-stage cystinosis progressed to kidney failure characterized by PTC atrophy with interstitial and glomerular fibrosis (Cherqui and Courtoy, 2017). A recent study also demonstrated that genetic ablation of the megalin/LRP2 pathway in cystinotic kidneys could suppress cystine accumulation and crystal deposition, and preserve kidney function from progression into renal fibrosis, indicating endocytosis impairment is the main pathway affecting cystinotic kidney injuries (Janssens et al., 2019). Using CRISPR/Cas9 technology, Krohn et al. generated Ctns−/− rats characterized by progressive cystine accumulation in the kidney, proximal tubule dysfunction, tubulointerstitial fibrosis, and kidney failure. Further primary cultures of proximal tubules from Ctns−/− rat kidneys confirmed the critical mechanism of cystinosis involved renal fibrosis, including cystine overload, leading to reduced endocytic uptake, increased proliferation, and defective lysosomal dynamics and autophagy (Zhang et al., 2017). Luciani et al. published a series of papers on the mechanisms of cystinosis involving tubular dysfunction. They found that in cystinosis, lysosomal dysfunction impairs autophagy’s ability to clear damaged mitochondria, resulting in oxidative stress that stimulates phosphorylation of tight junction ZO-1 by Gα12/Src and triggers a signaling cascade involving ZO-1-associated Y-box factor ZONAB, leading to tubular cell proliferation and transport defects (Festa et al., 2018). They also observed that defective cystine mobilization from lysosomes could divert PT cells toward growth and proliferation and disrupt their functions. The the primary mechanism is the activation of regulator-Rag GTPase-dependent recruitment of mTORC1 (mechanistic target of rapamycin complex 1) (Berquez et al., 2023). Altogether, these studies provide insightful information on the mechanism of cystinosis-related renal fibrosis, indicating reduced endocytosis and lysosomal and autophagic defects, leading to renal fibrosis.
Ciliopathies
Ciliopathies are a group of diseases with mutations in ciliary-associated proteins. Many of these mutations manifest as renal ciliopathies, characterized by kidney dysfunction resulting from aberrant or ciliary functions, including polycystic kidney disease, nephronophthisis, et al. (McConnachie et al., 2021). In ADPKD, Obermuller et al. observed that loss of chloride channel CIC-5 and the albumin receptor megalin led to proteinuria in ADPKD (cy/+) rats, and the impairment in endocytosis could further reduce the efficacy of certain gene therapy (Obermuller et al., 2001; Witzgall et al., 2002). Endocytosis was further characterized as one of the shared gene pathways in ADPKD by the intra-species combined analysis (Chatterjee et al., 2017). Endocytic uptake was evident in megalin-positive cysts but only in those that remained connected to the renal tubular system (Nielsen et al., 2021). Altogether, many factors contributed to ADPKD induced renal fibrosis, and endocytic impairment occurred in ADPKD, leading to proteinuria, which may further partially orchestrate the development of renal fibrosis in ADPKD disease.
Light chain kidney disease
Most kidney disorders associated with myeloma are caused by the excess production of monoclonal light chains, and renal involvement is almost always accompanied by light chain proteinuria (Batuman, 2007; Heher et al., 2013). Regular light chains are filtered through the glomerulus, endocytosed by the proximal tubule cells through multiligand endocytic receptors megalin/cubilin, and degraded in lysosome (Sanders, 2011). When significant amounts of light chains are generated by multiple myeloma and filtered through the glomerulus, the proximal tubular endocytic process is overloaded. Cell stress responses that include lysosomal alterations, phosphorylation of MAPKs, nuclear transcription factors NF-kB, Toll-like receptors, and STAT1, leading to the production of proinflammatory cytokines including TNFα, MCP-1 and Il1 β, and profibrotic cytokines like TGF-β1 (Batuman, 2007; Luciani et al., 2016; Ying et al., 2019; Sirac et al., 2021). Intracellular H2O2 induced by endocytosis of monoclonal free light chains oxidizes and activates c-Src, promoting the release of MCP-1 (Basnayake et al., 2010). As a result, these proximal tubule alterations often progress to severe tubulointerstitial kidney disease, the most common type of kidney involvement responsible for end-stage renal failure seen in myeloma patients (Batuman, 2007). It has also been shown that silencing megalin and cubilin genes may inhibit the uptake of myeloma light chain, subsequently suppressing inflammation in PTECs, and ameliorating nephrotoxicity (Li et al., 2008). Overall, endocytic overload of the light chain is the starting point, followed by a series of intracellular pathways activation, including pro-inflammatory and profibrotic pathways. Endocytosis overload caused by excess light chain production is the leading key role in tubular injuries and renal fibrosis in light chain kidney disease.
Obesity-related renal disease
Obesity is significantly associated with the progression and development of chronic kidney disease (Kovesdy et al., 2017). It is demonstrated that megalin-mediated endocytosis involves the uptake of lipid-toxic glomerular-filtered substances, affecting the function of the endosome/lysosome system and resulting in autophagy impairment, followed by the increased production of profibrotic and inflammatory mediators in PTECs, which activates interstitial fibrocytes/pericytes to induce renal fibrosis (Kuwahara et al., 2016). It is believed that megalin could be a therapeutic target for obesity-related CKD. In obesity-related renal disease, numerous factors contribute to the development and progression of CKD, including insulin resistance, lipotoxicity, dysregulation of adipocytokine secretion, contribution of different fat depots, etc., (Sandino et al., 2022). Endocytosis impairment in tubule may partially contribute to CKD progression.
Anti-brush border antibody renal disease
Anti-brush border antibody (ABBA) renal disease is identified as a unique and likely under-reported cause of severe and progressive renal tubular injury. In ABBA, circulating autoantibodies to the tubular brush border protein LRP2/megalin deposit in the TBM are characterized by IgG- and LRP2-containing immune complexes (Larsen et al., 2018; Dvanajscak et al., 2020). The disease course rapidly progresses to end-stage renal disease (ESRD) partially through endocytic impairment (Campbell et al., 2020).
DM/DN affected tubular endocytosis
Numerous studies have been conducted to determine how tubular endocytosis is affected by Diabetes and Diabetic nephropathy. Interestingly, in early to mid-T2DM/DN, several groups found increases in megalin expression and albumin endocytosis due to the activation of insulin intracellular signaling, including PI3K/AKT, PKB, and mTOR pathways in TECs(Brunskill et al., 1998; Caruso-Neves et al., 2006; Hosojima et al., 2009; Koral et al., 2014; Peruchetti et al., 2014; Coffey et al., 2015; Bryniarski et al., 2018). Oxidative stress also increases megalin expression in the early stage of DM(Kurosaki et al., 2018). Other studies found that high glucose concentrations (HG) inhibited megalin expression (Tojo et al., 2001; Zhou et al., 2011). The mechanism is mainly because glucose is diverted to the hexosamine biosynthetic pathway (HBP), increasing O-GlcNAcylation of several intracellular proteins, including PKB (Peruchetti et al., 2018). PKB O-GlcNAcylation decreases PKB activity, leading to decreases in megalin expression and inhibition of the albumin endocytosis (Peruchetti et al., 2018). Then, as DN advances and glomerular selectivity decreases, PTECs are exposed to compounds like albumin, advanced glycation end products, and fatty acids bound to albumin, usually absent from glomerular filtrate (Saito et al., 2005a; Saito et al., 2005b; Abbate et al., 2006; Birn and Christensen, 2006; Tesch, 2008; Reidy et al., 2014; Slyne et al., 2015; Kuwahara et al., 2016; De et al., 2017). Excessive megalin/cubilin-mediated endocytosis overwhelms lysosomal clearance in PTECs and activates apoptosis, NLRP3 inflammation, and potentially tubulointerstitial fibrosis (Christensen et al., 2012; Liu et al., 2015; Kuwahara et al., 2016; De et al., 2017). As widely recognized, abundant factors are involved in DKD; in summary, tubular endocytosis is also greatly affected by DN/DKD, further adding to DKD progression.
High salt diet-induced tubular endosomal injuries
Hypertensive individuals are at a higher risk for developing chronic kidney disease (CKD) characterized by interstitial fibrosis (Pugh et al., 2019). Tubular dysfunction has been observed in hypertensive patients and animal models (Wang et al., 2009; Landgraf et al., 2011), indicating the critical role of the tubule in hypertension-induced CKD. Further study revealed that a high salt diet reduced receptor-mediated endocytosis and the expression of megalin, as compared to a regular salt diet, leading to increases in pro-inflammatory and profibrotic factors (Silva-Aguiar et al., 2018; Teixeira et al., 2020). In addition, in vitro study also showed that incubation of LLC-PK1 cells with higher NaCl concentration decreased both albumin endocytosis and megalin expression (Teixeira et al., 2020). The underlying mechanism is also related to the increases in O-GlcNAcylation (Silva-Aguiar et al., 2018). In this part, these studies suggested that rather than commonly regarded that the high salt diet could increase blood pressure and indirectly cause renal injuries, high salt could also directly affect tubular endocytosis, leading to tubular injuries, resulting in renal fibrosis.
Tubular reabsorption of albumin
Albumin is filtered by the glomeruli and reabsorbed by the proximal tubular cells through receptor-mediated endocytosis (Fatah et al., 2018). Megalin, cubulin, and AMN are all important since deficiency of any of the three proteins was shown to induce albuminuria in patients (Zeng et al., 2017; Bedin et al., 2020). Internalization of albumin by endocytosis is followed by transcytosis or undergo catabolism via lysosomal degradation (Gorriz and Martinez-Castelao, 2012; Molitoris et al., 2022). Low doses of albumin are protective. However, an albumin overload decreases the megalin expression and increases albumin endocytosis; overstressing the endocytic system is injurious to renal proximal tubule cells (Caruso-Neves et al., 2006). Albumin overload activates the mTORC2/PKB pathway leading to albumin-induced apoptosis (Caruso-Neves et al., 2006). Endocytosis of albumin induces Matrix Metalloproteinase-9 (MMP9) by activating the ERK signaling pathway in RTECs(Chen et al., 2017). Albumin endocytosis of PTECs could also lead to the activation of MCP-1 expression (Wang et al., 1997) and accumulation of collagen I, III, and IV (Wohlfarth et al., 2003). Since proximal tubular cells exposed to albuminuria exhibit a proinflammatory and profibrotic response, changes in proximal tubule (PT) albumin endocytosis play an essential role in developing tubular interstitial injuries (Teixeira et al., 2019). However, recently, another study revealed that serum, but not its major protein component albumin, induced tubular injury and secretion of proinflammatory cytokines (Lidberg et al., 2022). Altogether, further studies are required to elucidate the role of albumin in tubular epithelial injuries.
Renal fibrosis and proximal tubular endocytosis
Renal fibrosis is the final manifestation of every type of chronic kidney disease (CKD) (Zhong et al., 2017). The hallmark of renal fibrosis is characterized by increased deposition of extracellular matrix (ECM), resulting in kidney function loss, leading to end-stage renal failure (Liu, 2006).
Profibrotic factors downregulate megalin expression and affects endocytosis
TGF-β1 (transforming growth factor-β) has been identified as a central mediator in renal fibrosis (Meng et al., 2015). Interestingly, TGF-β1 downregulates megalin protein expression and reduces albumin endocytosis (Gekle et al., 2003). Further study showed that transcription factors SMAD2/3, the primary downstream pathway of TGF-β1, decrease megalin mRNA expression by binding directly to SBEs in the human megalin (Meng et al., 2015; Cabezas et al., 2019). Angiotensin II, another crucial role of renal fibrosis (Saldanha da Silva et al., 2017), also decreased megalin expression (Mezzano et al., 2001; Hosojima et al., 2009), and the in vivo inhibition of the angiotensin receptor (AT1) with Losartan protected against the reduction of megalin observed in the kidneys of proteinuria animal model (Marzolo and Farfan, 2011). Megalin also plays an important role in rhabdomyolysis-induced AKI, and megalin interference and inhibition could ameliorate rhabdomyolysis-induced AKI (Matsushita et al., 2021).
Decreases in Dab2 exacerbates renal fibrosis
Notch is involved in kidney development and kidney injuries (Sirin and Susztak, 2012). Tubular epithelial notch reactivation also contributes to epithelial injuries and fibrosis development (Sirin and Susztak, 2012). Schutte-Nutgen et al. performed genome-wide transcriptome analysis to identify that the top downregulated gene of Notch signaling is Disabled-2 (Dab2) (Schutte-Nutgen et al., 2019). Dab2 is a cytoplasmic adaptor protein that binds to the cytoplasmic tail of the multiligand endocytic receptor megalin (Nagai et al., 2005). Dab2 is involved in endocytic regulation and albumin endocytosis (Koral and Erkan, 2012). Knockout of Dab2 in mice disrupts formation of endocytic vesicles, increases the excretion of vitamin D-binding protein, and reduces resorption of megalin (Morris et al., 2002; Nagai et al., 2005). Qiu et al. demonstrated that genetic lowering of Dab2 expression in kidney tubules protected mice from renal fibrosis (Qiu et al., 2018). These data suggest that Dab2 plays a versatile role in the kidney and impacts renal fibrosis.
Loss of manba affects endo-lysosomal system of TECs and enhanced fibrotic response
MANBA (beta-mannosidase) is a lysosomal enzyme mainly expressed in the lysosome of TECs. Gu et al. showed that Manba knockout mice indicated a defect in lysosome function, leading to changes in receptor-mediated endocytosis and fluid-phase endocytosis and blockage in autophagy, followed by activation of the inflammasome and an enhanced fibrotic response after toxic injuries (Gu et al., 2021).
Rab7 is involved in renal fibrosis through multiple mechanisms
Moreover, Rab7, a late endosome-/lysosome-associated small GTPase and an essential member of the Rab family, play critical roles in the endocytic processes (Zhang et al., 2009). Rab7 participates in multiple regulation mechanisms in endosomal sorting, lysosome biogenesis, and phagocytosis (Zhang et al., 2009). Xu et al. showed that, in the Rab7-knock-in mice, the degree of renal fibrosis was milder than in WT mice on the seventh day of the UUO model. However, it became more severe 14 days after UUO(Xu et al., 2020). Liu et al. demonstrated that upregulation of Rab7 relieved epithelial-mesenchymal transition (EMT) and apoptosis in albumin-treated TECs (Liu et al., 2018). The study also shows that autophagy regulates MMP-2 activity in a RAB7-dependent manner (Liu et al., 2018). Another study showed that long-term albumin stimulation combined with overexpression of Rab7 could decrease MMP-2 activity, exacerbate renal tubular injury, and accelerate the development of Tubular interstitial fibrosis (Liu et al., 2021). These results indicated that Rab7 is closely associated with renal fibrosis progression and development.
Taken together, for the last part, we reviewed the recent studies on the cytokines like TGFβ1 and Angiotensin II and genes such as Dab2, Rab7, and Manba, affecting tubular endocytosis, eventually causing renal fibrosis. All these data also indicated that tubular endocytosis plays a crucial role in renal fibrosis.
Concluding remarks
Overall, various genetic mutations and acquired insults, including high salt, high glucose, light chains, etc., could trigger tubular endocytosis injuries (Figure 1). As a result, tubular endocytosis impairment is either a causal factor or a contributing factor involved in renal fibrosis. Meanwhile, renal fibrosis exacerbates tubular endocytosis injuries in a feedback mechanism, leading to a vicious cycle (Figure 1). But current studies still lack precise mechanisms of the relationship between endocytosis and renal fibrosis, and further studies are needed to elucidate the specific role of endocytosis in renal fibrosis. This review also indicates that the endosomal pathway is an important therapeutic target for abrogating renal fibrosis, and megalin and several other genes may be a potential mechanism for drug targeting in renal fibrosis if initiated early in the disease.
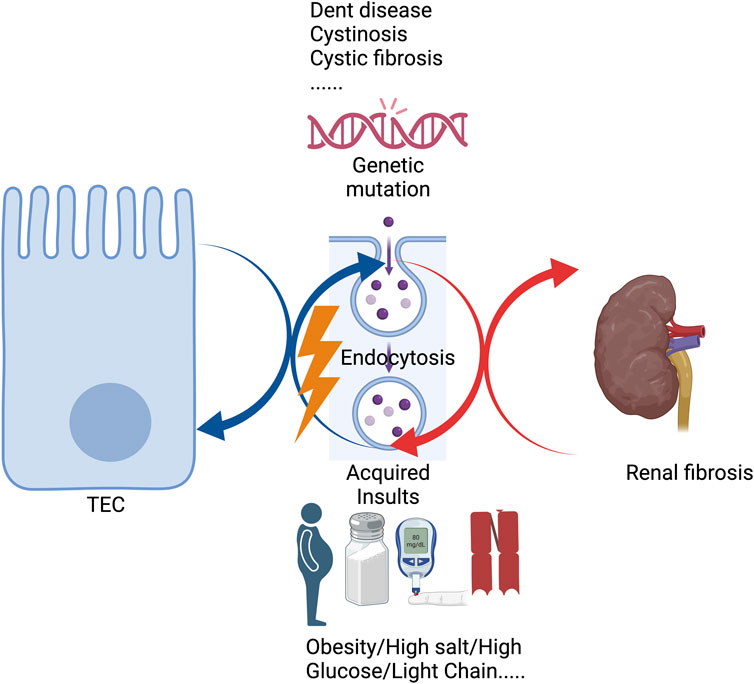
FIGURE 1. Genetic mutations or acquired insults affect tubular endocytosis, leading to renal fibrosis.
Author contributions
XG and MC conceived, wrote, and edited the manuscript. All authors contributed to the article and approved the submitted version.
Funding
This work was supported by Shanghai Yueyang Hospital Program No. 2201yyjm01, 2022 Shanghai “Science and Technology Innovation Action Plan” biopharmaceutical technology support special project No. 23S11900500, Shanghai Jiao Tong University School of Medicine, 2022 Integrated Traditional Chinese and Western Medicine Research Platform No. 2022zxy003 to XG.
Conflict of interest
The authors declare that the research was conducted in the absence of any commercial or financial relationships that could be construed as a potential conflict of interest.
Publisher’s note
All claims expressed in this article are solely those of the authors and do not necessarily represent those of their affiliated organizations, or those of the publisher, the editors and the reviewers. Any product that may be evaluated in this article, or claim that may be made by its manufacturer, is not guaranteed or endorsed by the publisher.
References
Abbate, M., Zoja, C., and Remuzzi, G. (2006). How does proteinuria cause progressive renal damage? J. Am. Soc. Nephrol. 17 (11), 2974–2984. doi:10.1681/ASN.2006040377
Anglani, F., Gianesello, L., Beara-Lasic, L., and Lieske, J. (2019). Dent disease: A window into calcium and phosphate transport. J. Cell Mol. Med. 23 (11), 7132–7142. doi:10.1111/jcmm.14590
Basnayake, K., Ying, W. Z., Wang, P. X., and Sanders, P. W. (2010). Immunoglobulin light chains activate tubular epithelial cells through redox signaling. J. Am. Soc. Nephrol. 21 (7), 1165–1173. doi:10.1681/ASN.2009101089
Batuman, V. (2007). Proximal tubular injury in myeloma. Contrib. Nephrol. 153, 87–104. doi:10.1159/000096762
Bedin, M., Boyer, O., Servais, A., Li, Y., Villoing-Gaude, L., Tete, M. J., et al. (2020). Human C-terminal CUBN variants associate with chronic proteinuria and normal renal function. J. Clin. Invest. 130 (1), 335–344. doi:10.1172/JCI129937
Berquez, M., Chen, Z., Festa, B. P., Krohn, P., Keller, S. A., Parolo, S., et al. (2023). Lysosomal cystine export regulates mTORC1 signaling to guide kidney epithelial cell fate specialization. Nat. Commun. 14 (1), 3994. doi:10.1038/s41467-023-39261-3
Birn, H., and Christensen, E. I. (2006). Renal albumin absorption in physiology and pathology. Kidney Int. 69 (3), 440–449. doi:10.1038/sj.ki.5000141
Blanchard, A., Curis, E., Guyon-Roger, T., Kahila, D., Treard, C., Baudouin, V., et al. (2016). Observations of a large Dent disease cohort. Kidney Int. 90 (2), 430–439. doi:10.1016/j.kint.2016.04.022
Briant, K., Redlingshofer, L., and Brodsky, F. M. (2020). Clathrin's life beyond 40: connecting biochemistry with physiology and disease. Curr. Opin. Cell Biol. 65, 141–149. doi:10.1016/j.ceb.2020.06.004
Brunskill, N. J., Stuart, J., Tobin, A. B., Walls, J., and Nahorski, S. (1998). Receptor-mediated endocytosis of albumin by kidney proximal tubule cells is regulated by phosphatidylinositide 3-kinase. J. Clin. Invest. 101 (10), 2140–2150. doi:10.1172/JCI1923
Bryniarski, M. A., Yee, B. M., Jaffri, I., Chaves, L. D., Yu, J. A., Guan, X., et al. (2018). Increased megalin expression in early type 2 diabetes: role of insulin-signaling pathways. Am. J. Physiol. Ren. Physiol. 315 (5), F1191–F1207. doi:10.1152/ajprenal.00210.2018
Cabezas, F., Farfan, P., and Marzolo, M. P. (2019). Participation of the SMAD2/3 signalling pathway in the down regulation of megalin/LRP2 by transforming growth factor beta (TGF-ß1). PLoS One 14 (5), e0213127. doi:10.1371/journal.pone.0213127
Campbell, R. E., Uhlenhopp, D., Shaw, M., Dai, D. F., and Sekar, A. (2020). Anti-brush border antibody (ABBA)-associated renal disease. QJM 113 (8), 561–562. doi:10.1093/qjmed/hcaa015
Caruso-Neves, C., Pinheiro, A. A., Cai, H., Souza-Menezes, J., and Guggino, W. B. (2006). PKB and megalin determine the survival or death of renal proximal tubule cells. Proc. Natl. Acad. Sci. U. S. A. 103 (49), 18810–18815. doi:10.1073/pnas.0605029103
Chatterjee, S., Verma, S. P., and Pandey, P. (2017). Profiling conserved biological pathways in autosomal dominant polycystic kidney disorder (ADPKD) to elucidate key transcriptomic alterations regulating cystogenesis: A cross-species meta-analysis approach. Gene 627, 434–450. doi:10.1016/j.gene.2017.06.059
Chen, X., Cobbs, A., George, J., Chima, A., Tuyishime, F., and Zhao, X. (2017). Endocytosis of albumin induces matrix Metalloproteinase-9 by activating the ERK signaling pathway in renal tubule epithelial cells. Int. J. Mol. Sci. 18 (8), 1758. doi:10.3390/ijms18081758
Cherqui, S., and Courtoy, P. J. (2017). The renal fanconi syndrome in cystinosis: pathogenic insights and therapeutic perspectives. Nat. Rev. Nephrol. 13 (2), 115–131. doi:10.1038/nrneph.2016.182
Christensen, E. I., Birn, H., Storm, T., Weyer, K., and Nielsen, R. (2012). Endocytic receptors in the renal proximal tubule. Physiol. (Bethesda) 27 (4), 223–236. doi:10.1152/physiol.00022.2012
Christensen, E. I., Devuyst, O., Dom, G., Nielsen, R., Van der Smissen, P., Verroust, P., et al. (2003). Loss of chloride channel ClC-5 impairs endocytosis by defective trafficking of megalin and cubilin in kidney proximal tubules. Proc. Natl. Acad. Sci. U. S. A. 100 (14), 8472–8477. doi:10.1073/pnas.1432873100
Coffey, S., Costacou, T., Orchard, T., and Erkan, E. (2015). Akt links insulin signaling to albumin endocytosis in proximal tubule epithelial cells. PLoS One 10 (10), e0140417. doi:10.1371/journal.pone.0140417
De Matteis, M. A., Staiano, L., Emma, F., and Devuyst, O. (2017). The 5-phosphatase OCRL in Lowe syndrome and Dent disease 2. Nat. Rev. Nephrol. 13 (8), 455–470. doi:10.1038/nrneph.2017.83
De, S., Kuwahara, S., Hosojima, M., Ishikawa, T., Kaseda, R., Sarkar, P., et al. (2017). Exocytosis-mediated urinary full-length megalin excretion is linked with the pathogenesis of diabetic nephropathy. Diabetes 66 (5), 1391–1404. doi:10.2337/db16-1031
De, S., Kuwahara, S., and Saito, A. (2014). The endocytic receptor megalin and its associated proteins in proximal tubule epithelial cells. Membr. (Basel) 4 (3), 333–355. doi:10.3390/membranes4030333
Devuyst, O., and Luciani, A. (2015). Chloride transporters and receptor-mediated endocytosis in the renal proximal tubule. J. Physiol. 593 (18), 4151–4164. doi:10.1113/JP270087
Dizin, E., Hasler, U., Nlandu-Khodo, S., Fila, M., Roth, I., Ernandez, T., et al. (2013). Albuminuria induces a proinflammatory and profibrotic response in cortical collecting ducts via the 24p3 receptor. Am. J. Physiol. Ren. Physiol. 305 (7), F1053–F1063. doi:10.1152/ajprenal.00006.2013
Duran, M., Burballa, C., Cantero-Recasens, G., Butnaru, C. M., Malhotra, V., Ariceta, G., et al. (2021). Novel Dent Disease 1 cellular models reveal biological processes underlying ClC-5 loss-of-function. Hum. Mol. Genet. 30, 1413–1428. doi:10.1093/hmg/ddab131
Dvanajscak, Z., Murphy, J. D., Larsen, C. P., and Padala, S. A. (2020). Anti-brush border antibody disease (Anti-LRP2 nephropathy) associated with lupus nephritis. Kidney Int. Rep. 5 (9), 1590–1594. doi:10.1016/j.ekir.2020.06.025
Ellinger, I., and Pietschmann, P. (2016). Endocytosis in health and disease-a thematic issue dedicated to Renate Fuchs. Wien Med. Wochenschr 166 (7-8), 193–195. doi:10.1007/s10354-016-0454-1
Eshbach, M. L., and Weisz, O. A. (2017). Receptor-mediated endocytosis in the proximal tubule. Annu. Rev. Physiol. 79, 425–448. doi:10.1146/annurev-physiol-022516-034234
Fatah, H., Benfaed, N., Chana, R. S., Chunara, M. H., Barratt, J., Baines, R. J., et al. (2018). Reduced proximal tubular expression of protein endocytic receptors in proteinuria is associated with urinary receptor shedding. Nephrol. Dial. Transpl. 33 (6), 934–943. doi:10.1093/ndt/gfx321
Festa, B. P., Berquez, M., Gassama, A., Amrein, I., Ismail, H. M., Samardzija, M., et al. (2019). OCRL deficiency impairs endolysosomal function in a humanized mouse model for Lowe syndrome and Dent disease. Hum. Mol. Genet. 28 (12), 1931–1946. doi:10.1093/hmg/ddy449
Festa, B. P., Chen, Z., Berquez, M., Debaix, H., Tokonami, N., Prange, J. A., et al. (2018). Impaired autophagy bridges lysosomal storage disease and epithelial dysfunction in the kidney. Nat. Commun. 9 (1), 161. doi:10.1038/s41467-017-02536-7
Gaide Chevronnay, H. P., Janssens, V., Van Der Smissen, P., N'Kuli, F., Nevo, N., Guiot, Y., et al. (2014). Time course of pathogenic and adaptation mechanisms in cystinotic mouse kidneys. J. Am. Soc. Nephrol. 25 (6), 1256–1269. doi:10.1681/ASN.2013060598
Gekle, M., Knaus, P., Nielsen, R., Mildenberger, S., Freudinger, R., Wohlfarth, V., et al. (2003). Transforming growth factor-beta1 reduces megalin- and cubilin-mediated endocytosis of albumin in proximal-tubule-derived opossum kidney cells. J. Physiol. 552 (2), 471–481. doi:10.1113/jphysiol.2003.048074
Gianesello, L., Del Prete, D., Ceol, M., Priante, G., Calo, L. A., and Anglani, F. (2020). From protein uptake to dent disease: an overview of the CLCN5 gene. Gene 747, 144662. doi:10.1016/j.gene.2020.144662
Gianesello, L., Del Prete, D., Anglani, F., and Calo, L. A. (2021). Genetics and phenotypic heterogeneity of dent disease: the dark side of the moon. Hum. Genet. 140 (3), 401–421. doi:10.1007/s00439-020-02219-2
Gorriz, J. L., and Martinez-Castelao, A. (2012). Proteinuria: detection and role in native renal disease progression. Transpl. Rev. Orl. 26 (1), 3–13. doi:10.1016/j.trre.2011.10.002
Gorvin, C. M., Wilmer, M. J., Piret, S. E., Harding, B., van den Heuvel, L. P., Wrong, O., et al. (2013). Receptor-mediated endocytosis and endosomal acidification is impaired in proximal tubule epithelial cells of Dent disease patients. Proc. Natl. Acad. Sci. U. S. A. 110 (17), 7014–7019. doi:10.1073/pnas.1302063110
Gu, X., Yang, H., Sheng, X., Ko, Y. A., Qiu, C., Park, J., et al. (2021). Kidney disease genetic risk variants alter lysosomal beta-mannosidase (MANBA) expression and disease severity. Sci. Transl. Med. 13 (576), eaaz1458. doi:10.1126/scitranslmed.aaz1458
Heher, E. C., Rennke, H. G., Laubach, J. P., and Richardson, P. G. (2013). Kidney disease and multiple myeloma. Clin. J. Am. Soc. Nephrol. 8 (11), 2007–2017. doi:10.2215/CJN.12231212
Hoopes, R. R., Raja, K. M., Koich, A., Hueber, P., Reid, R., Knohl, S. J., et al. (2004). Evidence for genetic heterogeneity in Dent's disease. Kidney Int. 65 (5), 1615–1620. doi:10.1111/j.1523-1755.2004.00571.x
Hosojima, M., Sato, H., Yamamoto, K., Kaseda, R., Soma, T., Kobayashi, A., et al. (2009). Regulation of megalin expression in cultured proximal tubule cells by angiotensin II type 1A receptor- and insulin-mediated signaling cross talk. Endocrinology 150 (2), 871–878. doi:10.1210/en.2008-0886
Inoue, K., and Ishibe, S. (2015). Podocyte endocytosis in the regulation of the glomerular filtration barrier. Am. J. Physiol. Ren. Physiol. 309 (5), F398–F405. doi:10.1152/ajprenal.00136.2015
Janssens, V., Gaide Chevronnay, H. P., Marie, S., Vincent, M. F., Van Der Smissen, P., Nevo, N., et al. (2019). Protection of cystinotic mice by kidney-specific megalin ablation supports an endocytosis-based mechanism for nephropathic cystinosis progression. J. Am. Soc. Nephrol. 30 (11), 2177–2190. doi:10.1681/ASN.2019040371
Kaksonen, M., and Roux, A. (2018). Mechanisms of clathrin-mediated endocytosis. Nat. Rev. Mol. Cell Biol. 19 (5), 313–326. doi:10.1038/nrm.2017.132
Koral, K., and Erkan, E. (2012). PKB/Akt partners with Dab2 in albumin endocytosis. Am. J. Physiol. Ren. Physiol. 302 (8), F1013–F1024. doi:10.1152/ajprenal.00289.2011
Koral, K., Li, H., Ganesh, N., Birnbaum, M. J., Hallows, K. R., and Erkan, E. (2014). Akt recruits Dab2 to albumin endocytosis in the proximal tubule. Am. J. Physiol. Ren. Physiol. 307 (12), F1380–F1389. doi:10.1152/ajprenal.00454.2014
Kovesdy, C. P., Furth, S. L., Zoccali, C., and World Kidney Day Steering, C. (2017). Obesity and kidney disease: hidden consequences of the epidemic. Can. J. Kidney Health Dis. 4, 2054358117698669. doi:10.1177/2054358117698669
Kurosaki, Y., Imoto, A., Kawakami, F., Yokoba, M., Takenaka, T., Ichikawa, T., et al. (2018). Oxidative stress increases megalin expression in the renal proximal tubules during the normoalbuminuric stage of diabetes mellitus. Am. J. Physiol. Ren. Physiol. 314 (3), F462–F470. doi:10.1152/ajprenal.00108.2017
Kuwahara, S., Hosojima, M., Kaneko, R., Aoki, H., Nakano, D., Sasagawa, T., et al. (2016). Megalin-mediated tubuloglomerular alterations in high-fat diet-induced kidney disease. J. Am. Soc. Nephrol. 27 (7), 1996–2008. doi:10.1681/ASN.2015020190
Landgraf, S. S., Wengert, M., Silva, J. S., Zapata-Sudo, G., Sudo, R. T., Takiya, C. M., et al. (2011). Changes in angiotensin receptors expression play a pivotal role in the renal damage observed in spontaneously hypertensive rats. Am. J. Physiol. Ren. Physiol. 300 (2), F499–F510. doi:10.1152/ajprenal.00384.2010
Larsen, C. P., Trivin-Avillach, C., Coles, P., Collins, A. B., Merchant, M., Ma, H., et al. (2018). LDL receptor-related protein 2 (megalin) as a target antigen in human kidney anti-brush border antibody disease. J. Am. Soc. Nephrol. 29 (2), 644–653. doi:10.1681/ASN.2017060664
Li, M., Balamuthusamy, S., Simon, E. E., and Batuman, V. (2008). Silencing megalin and cubilin genes inhibits myeloma light chain endocytosis and ameliorates toxicity in human renal proximal tubule epithelial cells. Am. J. Physiol. Ren. Physiol. 295 (1), F82–F90. doi:10.1152/ajprenal.00091.2008
Lidberg, K. A., Muthusamy, S., Adil, M., Mahadeo, A., Yang, J., Patel, R. S., et al. (2022). Serum protein exposure activates a core regulatory Program driving human proximal tubule injury. J. Am. Soc. Nephrol. 33 (5), 949–965. doi:10.1681/ASN.2021060751
Liu, D., Wen, Y., Tang, T. T., Lv, L. L., Tang, R. N., Liu, H., et al. (2015). Megalin/Cubulin-Lysosome-mediated albumin reabsorption is involved in the tubular cell activation of NLRP3 inflammasome and tubulointerstitial inflammation. J. Biol. Chem. 290 (29), 18018–18028. doi:10.1074/jbc.M115.662064
Liu, L., Li, Y., Wang, Z., Ding, F., Cheng, Z., Xu, Q., et al. (2018). Rab7 empowers renal tubular epithelial cells with autophagy-mediated protection against albumin-induced injury. Exp. Cell Res. 370 (2), 198–207. doi:10.1016/j.yexcr.2018.06.019
Liu, L., Xu, Q., Zhang, L., Sun, H., Ding, F., Li, Y., et al. (2021). Fe3O4 magnetic nanoparticles ameliorate albumin-induced tubulointerstitial fibrosis by autophagy related to Rab7. Colloids Surf. B Biointerfaces 198, 111470. doi:10.1016/j.colsurfb.2020.111470
Liu, Y. (2006). Renal fibrosis: new insights into the pathogenesis and therapeutics. Kidney Int. 69 (2), 213–217. doi:10.1038/sj.ki.5000054
Luciani, A., Sirac, C., Terryn, S., Javaugue, V., Prange, J. A., Bender, S., et al. (2016). Impaired lysosomal function underlies monoclonal light chain-associated renal fanconi syndrome. J. Am. Soc. Nephrol. 27 (7), 2049–2061. doi:10.1681/ASN.2015050581
Martin, C. E., Petersen, K. A., Aoudjit, L., Tilak, M., Eremina, V., Hardy, W. R., et al. (2018). ShcA adaptor protein promotes nephrin endocytosis and is upregulated in proteinuric nephropathies. J. Am. Soc. Nephrol. 29 (1), 92–103. doi:10.1681/ASN.2017030285
Marzolo, M. P., and Farfan, P. (2011). New insights into the roles of megalin/LRP2 and the regulation of its functional expression. Biol. Res. 44 (1), 89–105. doi:10.4067/S0716-97602011000100012
Matsushita, K., Mori, K., Saritas, T., Eiwaz, M. B., Funahashi, Y., Nickerson, M. N., et al. (2021). Cilastatin ameliorates rhabdomyolysis-induced AKI in mice. J. Am. Soc. Nephrol. 32 (10), 2579–2594. doi:10.1681/ASN.2020030263
McConnachie, D. J., Stow, J. L., and Mallett, A. J. (2021). Ciliopathies and the kidney: A review. Am. J. Kidney Dis. 77 (3), 410–419. doi:10.1053/j.ajkd.2020.08.012
Meng, X. M., Tang, P. M., Li, J., and Lan, H. Y. (2015). TGF-β/Smad signaling in renal fibrosis. Front. Physiol. 6, 82. doi:10.3389/fphys.2015.00082
Mezzano, S. A., Ruiz-Ortega, M., and Egido, J. (2001). Angiotensin II and renal fibrosis. Hypertension 38 (2), 635–638. doi:10.1161/hy09t1.094234
Molitoris, B. A., Sandoval, R. M., Yadav, S. P. S., and Wagner, M. C. (2022). Albumin uptake and processing by the proximal tubule: physiological, pathological, and therapeutic implications. Physiol. Rev. 102 (4), 1625–1667. doi:10.1152/physrev.00014.2021
Moriyama, T., Karasawa, K., Hasegawa, F., Uchida, K., and Nitta, K. (2019). Sertraline reduces albuminuria by interfering with caveolae-mediated endocytosis through glomerular endothelial and epithelial cells. Am. J. Nephrol. 50 (6), 444–453. doi:10.1159/000503917
Morris, S. M., Tallquist, M. D., Rock, C. O., and Cooper, J. A. (2002). Dual roles for the Dab2 adaptor protein in embryonic development and kidney transport. EMBO J. 21 (7), 1555–1564. doi:10.1093/emboj/21.7.1555
Nagai, J., Christensen, E. I., Morris, S. M., Willnow, T. E., Cooper, J. A., and Nielsen, R. (2005). Mutually dependent localization of megalin and Dab2 in the renal proximal tubule. Am. J. Physiol. Ren. Physiol. 289 (3), F569–F576. doi:10.1152/ajprenal.00292.2004
Nielsen, M. L., Mundt, M. C., Lildballe, D. L., Rasmussen, M., Sunde, L., Torres, V. E., et al. (2021). Functional megalin is expressed in renal cysts in a mouse model of adult polycystic kidney disease. Clin. Kidney J. 14 (11), 2420–2427. doi:10.1093/ckj/sfab088
Norden, A. G., Lapsley, M., Lee, P. J., Pusey, C. D., Scheinman, S. J., Tam, F. W., et al. (2001). Glomerular protein sieving and implications for renal failure in Fanconi syndrome. Kidney Int. 60 (5), 1885–1892. doi:10.1046/j.1523-1755.2001.00016.x
Obermuller, N., Kranzlin, B., Blum, W. F., Gretz, N., and Witzgall, R. (2001). An endocytosis defect as a possible cause of proteinuria in polycystic kidney disease. Am. J. Physiol. Ren. Physiol. 280 (2), F244–F253. doi:10.1152/ajprenal.2001.280.2.F244
Peng, F. F., Xiao, Z. L., Chen, H. M., Chen, Y., Zhou, J., Yu, H., et al. (2016). Parathyroid hormone inhibits TGF-β/Smad signaling and extracellular matrix proteins upregulation in rat mesangial cells. Biochem. Biophys. Res. Commun. 478 (3), 1093–1098. doi:10.1016/j.bbrc.2016.08.073
Peruchetti, D. B., Cheng, J., Caruso-Neves, C., and Guggino, W. B. (2014). Mis-regulation of mammalian target of rapamycin (mTOR) complexes induced by albuminuria in proximal tubules. J. Biol. Chem. 289 (24), 16790–16801. doi:10.1074/jbc.M114.549717
Peruchetti, D. B., Silva-Aguiar, R. P., Siqueira, G. M., Dias, W. B., and Caruso-Neves, C. (2018). High glucose reduces megalin-mediated albumin endocytosis in renal proximal tubule cells through protein kinase B O-GlcNAcylation. J. Biol. Chem. 293 (29), 11388–11400. doi:10.1074/jbc.RA117.001337
Piwon, N., Gunther, W., Schwake, M., Bosl, M. R., and Jentsch, T. J. (2000). ClC-5 Cl- -channel disruption impairs endocytosis in a mouse model for Dent's disease. Nature 408 (6810), 369–373. doi:10.1038/35042597
Polesel, M., and Hall, A. M. (2019). Axial differences in endocytosis along the kidney proximal tubule. Am. J. Physiol. Ren. Physiol. 317 (6), F1526–F1530. doi:10.1152/ajprenal.00459.2019
Polesel, M., Kaminska, M., Haenni, D., Bugarski, M., Schuh, C., Jankovic, N., et al. (2022). Spatiotemporal organisation of protein processing in the kidney. Nat. Commun. 13 (1), 5732. doi:10.1038/s41467-022-33469-5
Prabakaran, T., Nielsen, R., Satchell, S. C., Mathieson, P. W., Feldt-Rasmussen, U., Sorensen, S. S., et al. (2012). Mannose 6-phosphate receptor and sortilin mediated endocytosis of alpha-galactosidase A in kidney endothelial cells. PLoS One 7 (6), e39975. doi:10.1371/journal.pone.0039975
Pugh, D., Gallacher, P. J., and Dhaun, N. (2019). Management of hypertension in chronic kidney disease. Drugs 79 (4), 365–379. doi:10.1007/s40265-019-1064-1
Qiu, C., Huang, S., Park, J., Park, Y., Ko, Y. A., Seasock, M. J., et al. (2018). Renal compartment-specific genetic variation analyses identify new pathways in chronic kidney disease. Nat. Med. 24 (11), 1721–1731. doi:10.1038/s41591-018-0194-4
Reidy, K., Kang, H. M., Hostetter, T., and Susztak, K. (2014). Molecular mechanisms of diabetic kidney disease. J. Clin. Invest. 124 (6), 2333–2340. doi:10.1172/JCI72271
Riordan, J. R., Rommens, J. M., Kerem, B., Alon, N., Rozmahel, R., Grzelczak, Z., et al. (1989). Identification of the cystic fibrosis gene: cloning and characterization of complementary DNA. Science 245 (4922), 1066–1073. doi:10.1126/science.2475911
Rowe, S. M., Miller, S., and Sorscher, E. J. (2005). Cystic fibrosis. N. Engl. J. Med. 352 (19), 1992–2001. doi:10.1056/NEJMra043184
Saito, A., Takeda, T., Hama, H., Oyama, Y., Hosaka, K., Tanuma, A., et al. (2005a). Role of megalin, a proximal tubular endocytic receptor, in the pathogenesis of diabetic and metabolic syndrome-related nephropathies: protein metabolic overload hypothesis. Nephrol. Carlt. 10, S26–S31. doi:10.1111/j.1440-1797.2005.00453.x
Saito, A., Takeda, T., Sato, K., Hama, H., Tanuma, A., Kaseda, R., et al. (2005b). Significance of proximal tubular metabolism of advanced glycation end products in kidney diseases. Ann. N. Y. Acad. Sci. 1043, 637–643. doi:10.1196/annals.1333.072
Saldanha da Silva, A. A., Rodrigues Prestes, T. R., Lauar, A. O., Finotti, B. B., and Simoes, E. S. A. C. (2017). Renin angiotensin system and cytokines in chronic kidney disease: clinical and experimental evidence. Protein Pept. Lett. 24 (9), 799–808. doi:10.2174/0929866524666170818160809
Sanders, P. W. (2011). Light chain-mediated tubulopathies. Contrib. Nephrol. 169, 262–269. doi:10.1159/000313959
Sandino, J., Martin-Taboada, M., Medina-Gomez, G., Vila-Bedmar, R., and Morales, E. (2022). Novel insights in the physiopathology and management of obesity-related kidney disease. Nutrients 14 (19), 3937. doi:10.3390/nu14193937
Schutte-Nutgen, K., Edeling, M., Mendl, G., Krahn, M. P., Edemir, B., Weide, T., et al. (2019). Getting a notch closer to renal dysfunction: activated notch suppresses expression of the adaptor protein disabled-2 in tubular epithelial cells. FASEB J. 33 (1), 821–832. doi:10.1096/fj.201800392RR
Sharma, S., Skowronek, A., and Erdmann, K. S. (2015). The role of the Lowe syndrome protein OCRL in the endocytic pathway. Biol. Chem. 396 (12), 1293–1300. doi:10.1515/hsz-2015-0180
Shipman, K. E., Baty, C. J., Long, K. R., Rbaibi, Y., Cowan, I. A., Gerges, M., et al. (2023). Impaired endosome maturation mediates tubular proteinuria in dent disease cell culture and mouse models. J. Am. Soc. Nephrol. 34 (4), 619–640. doi:10.1681/ASN.0000000000000084
Shipman, K. E., and Weisz, O. A. (2020). Making a dent in dent disease. Funct. (Oxf) 1 (2), zqaa017. doi:10.1093/function/zqaa017
Silva-Aguiar, R. P., Bezerra, N. C. F., Lucena, M. C., Sirtoli, G. M., Sudo, R. T., Zapata-Sudo, G., et al. (2018). O-GlcNAcylation reduces proximal tubule protein reabsorption and promotes proteinuria in spontaneously hypertensive rats. J. Biol. Chem. 293 (33), 12749–12758. doi:10.1074/jbc.RA118.001746
Singhal, P. C., Gupta, S., Sharma, P., Shah, H., Shah, N., and Patel, P. (2000). Receptor mediated endocytosis by mesangial cells modulates transmigration of macrophages. Inflammation 24 (6), 519–532. doi:10.1023/a:1007073306394
Sirac, C., Batuman, V., and Sanders, P. W. (2021). The proximal tubule toxicity of immunoglobulin light chains. Kidney Int. Rep. 6 (5), 1225–1231. doi:10.1016/j.ekir.2021.02.026
Sirin, Y., and Susztak, K. (2012). Notch in the kidney: development and disease. J. Pathol. 226 (2), 394–403. doi:10.1002/path.2967
Slyne, J., Slattery, C., McMorrow, T., and Ryan, M. P. (2015). New developments concerning the proximal tubule in diabetic nephropathy: in vitro models and mechanisms. Nephrol. Dial. Transpl. 30 (4), iv60–67. doi:10.1093/ndt/gfv264
Smith, C. P., Lee, W. K., Haley, M., Poulsen, S. B., Thevenod, F., and Fenton, R. A. (2019). Proximal tubule transferrin uptake is modulated by cellular iron and mediated by apical membrane megalin-cubilin complex and transferrin receptor 1. J. Biol. Chem. 294 (17), 7025–7036. doi:10.1074/jbc.RA118.006390
Srivastav, R. K., Ansari, T. M., Prasad, M., Vishwakarma, V. K., Bagga, P., and Ahsan, F. (2019). Caveolins; an assailant or an ally of various cellular disorders. Drug Res. (Stuttg) 69 (8), 419–427. doi:10.1055/a-0850-6518
Suchy, S. F., Olivos-Glander, I. M., and Nussabaum, R. L. (1995). Lowe syndrome, a deficiency of phosphatidylinositol 4,5-bisphosphate 5-phosphatase in the Golgi apparatus. Hum. Mol. Genet. 4 (12), 2245–2250. doi:10.1093/hmg/4.12.2245
Teixeira, D. E., Peruchetti, D. B., Silva, L. S., Silva-Aguiar, R. P., Oquendo, M. B., Silva-Filho, J. L., et al. (2019). Lithium ameliorates tubule-interstitial injury through activation of the mTORC2/protein kinase B pathway. PLoS One 14 (4), e0215871. doi:10.1371/journal.pone.0215871
Teixeira, D. E., Peruchetti, D. B., Souza, M. C., das Gracas Henriques, M. G., Pinheiro, A. A. S., and Caruso-Neves, C. (2020). A high salt diet induces tubular damage associated with a pro-inflammatory and pro-fibrotic response in a hypertension-independent manner. Biochim. Biophys. Acta Mol. Basis Dis. 1866 (11), 165907. doi:10.1016/j.bbadis.2020.165907
Tesch, G. H. (2008). MCP-1/CCL2: a new diagnostic marker and therapeutic target for progressive renal injury in diabetic nephropathy. Am. J. Physiol. Ren. Physiol. 294 (4), F697–F701. doi:10.1152/ajprenal.00016.2008
Tojo, A., Onozato, M. L., Ha, H., Kurihara, H., Sakai, T., Goto, A., et al. (2001). Reduced albumin reabsorption in the proximal tubule of early-stage diabetic rats. Histochem Cell Biol. 116 (3), 269–276. doi:10.1007/s004180100317
van Berkel, Y., Ludwig, M., van Wijk, J. A. E., and Bokenkamp, A. (2017). Proteinuria in dent disease: a review of the literature. Pediatr. Nephrol. 32 (10), 1851–1859. doi:10.1007/s00467-016-3499-x
van der Wijst, J., Belge, H., Bindels, R. J. M., and Devuyst, O. (2019). Learning physiology from inherited kidney disorders. Physiol. Rev. 99 (3), 1575–1653. doi:10.1152/physrev.00008.2018
Vicinanza, M., Di Campli, A., Polishchuk, E., Santoro, M., Di Tullio, G., Godi, A., et al. (2011). OCRL controls trafficking through early endosomes via PtdIns4,5P₂-dependent regulation of endosomal actin. EMBO J. 30 (24), 4970–4985. doi:10.1038/emboj.2011.354
Vilasi, A., Cutillas, P. R., Maher, A. D., Zirah, S. F., Capasso, G., Norden, A. W., et al. (2007). Combined proteomic and metabonomic studies in three genetic forms of the renal Fanconi syndrome. Am. J. Physiol. Ren. Physiol. 293 (2), F456–F467. doi:10.1152/ajprenal.00095.2007
Wang, X., Armando, I., Upadhyay, K., Pascua, A., and Jose, P. A. (2009). The regulation of proximal tubular salt transport in hypertension: an update. Curr. Opin. Nephrol. Hypertens. 18 (5), 412–420. doi:10.1097/MNH.0b013e32832f5775
Wang, Y. B., Leroy, V., Maunsbach, A. B., Doucet, A., Hasler, U., Dizin, E., et al. (2014). Sodium transport is modulated by p38 kinase-dependent cross-talk between ENaC and Na,K-ATPase in collecting duct principal cells. J. Am. Soc. Nephrol. 25 (2), 250–259. doi:10.1681/ASN.2013040429
Wang, Y., Chen, J., Chen, L., Tay, Y. C., Rangan, G. K., and Harris, D. C. (1997). Induction of monocyte chemoattractant protein-1 in proximal tubule cells by urinary protein. J. Am. Soc. Nephrol. 8 (10), 1537–1545. doi:10.1681/ASN.V8101537
Wilmer, M. J., Emma, F., and Levtchenko, E. N. (2010). The pathogenesis of cystinosis: mechanisms beyond cystine accumulation. Am. J. Physiol. Ren. Physiol. 299 (5), F905–F916. doi:10.1152/ajprenal.00318.2010
Witzgall, R., Kranzlin, B., Gretz, N., and Obermuller, N. (2002). Impaired endocytosis may represent an obstacle to gene therapy in polycystic kidney disease. Kidney Int. 61 (1), S132–S137. doi:10.1046/j.1523-1755.2002.0610s1132.x
Wohlfarth, V., Drumm, K., Mildenberger, S., Freudinger, R., and Gekle, M. (2003). Protein uptake disturbs collagen homeostasis in proximal tubule-derived cells. Kidney Int. Suppl (84), S103–S109. doi:10.1046/j.1523-1755.63.s84.13.x
Xu, Q., Liu, L., Yang, Y., Wang, Z., Cai, Y., Hong, T., et al. (2020). Effects of Rab7 gene up-regulation on renal fibrosis induced by unilateral ureteral obstruction. Braz J. Med. Biol. Res. 53 (4), e9220. doi:10.1590/1414-431X20209220
Ying, W. Z., Li, X., Rangarajan, S., Feng, W., Curtis, L. M., and Sanders, P. W. (2019). Immunoglobulin light chains generate proinflammatory and profibrotic kidney injury. J. Clin. Invest. 129 (7), 2792–2806. doi:10.1172/JCI125517
Zeng, B., Chen, G. L., Garcia-Vaz, E., Bhandari, S., Daskoulidou, N., Berglund, L. M., et al. (2017). ORAI channels are critical for receptor-mediated endocytosis of albumin. Nat. Commun. 8 (1), 1920. doi:10.1038/s41467-017-02094-y
Zhang, J. T., Wang, Y., Chen, J. J., Zhang, X. H., Dong, J. D., Tsang, L. L., et al. (2017). Defective CFTR leads to aberrant beta-catenin activation and kidney fibrosis. Sci. Rep. 7 (1), 5233. doi:10.1038/s41598-017-05435-5
Zhang, M., Chen, L., Wang, S., and Wang, T. (2009). Rab7: roles in membrane trafficking and disease. Biosci. Rep. 29 (3), 193–209. doi:10.1042/BSR20090032
Zhong, J., Yang, H. C., and Fogo, A. B. (2017). A perspective on chronic kidney disease progression. Am. J. Physiol. Ren. Physiol. 312 (3), F375–F384. doi:10.1152/ajprenal.00266.2016
Zhou, L., Liu, F., Huang, X. R., Liu, F., Chen, H., Chung, A. C., et al. (2011). Amelioration of albuminuria in ROCK1 knockout mice with streptozotocin-induced diabetic kidney disease. Am. J. Nephrol. 34 (5), 468–475. doi:10.1159/000332040
Keywords: endocytosis, renal fibrosis, megalin, cubulin, tubule epithelial cell
Citation: Chen M and Gu X (2023) Emerging roles of proximal tubular endocytosis in renal fibrosis. Front. Cell Dev. Biol. 11:1235716. doi: 10.3389/fcell.2023.1235716
Received: 06 June 2023; Accepted: 11 September 2023;
Published: 20 September 2023.
Edited by:
Bin Yang, University of Leicester, United KingdomReviewed by:
Miriam Zacchia, University of Campania Luigi Vanvitelli, ItalyAndrew Hall, University of Zurich, Switzerland
Copyright © 2023 Chen and Gu. This is an open-access article distributed under the terms of the Creative Commons Attribution License (CC BY). The use, distribution or reproduction in other forums is permitted, provided the original author(s) and the copyright owner(s) are credited and that the original publication in this journal is cited, in accordance with accepted academic practice. No use, distribution or reproduction is permitted which does not comply with these terms.
*Correspondence: Xiangchen Gu, Z2V0dHlndWd1QDEyNi5jb20=