- 1Hunan Key Laboratory of Cancer Metabolism, Hunan Cancer Hospital, Affiliated Cancer Hospital of Xiangya School of Medicine, Central South University, Changsha, Hunan, China
- 2Public Service Platform of Tumor Organoids Technology, Changsha, Hunan, China
- 3Hengyang Medical School, University of South China, Hengyang, Hunan, China
Organoids are a class of multicellular structures with the capability of self-organizing and the characteristic of original tissues, they are generated from stem cells in 3D culture in vitro. Organoids can mimic the occurrence and progression of original tissues and widely used in disease models in recent years. The ability of tumor organoids to retain characteristic of original tumors make them unique for tumorigenesis and cancer therapy. However, the history of organoid development and the application of organoid technology in cancer therapy are not well understood. In this paper, we reviewed the history of organoids development, the culture methods of tumor organoids establishing and the applications of organoids in cancer research for better understanding the process of tumor development and providing better strategies for cancer therapy. The standardization of organoids cultivation facilitated the large-scale production of tumor organoids. Moreover, it was found that combination of tumor organoids and other cells such as immune cells, fibroblasts and nervous cells would better mimic the microenvironment of tumor progression. This might be important developing directions for tumor organoids in the future.
Introduction
Cancer is now a major cause of death worldwide and an important barrier for extending life expectancy (Siegel et al., 2023). According to World Health Organization’s data about cancer statistics for 183 countries in 2019, the mortality caused by cancer was high among those who died before the age of 70, and in some countries it even ranked first (Sung et al., 2021). Studying tumor development process, developing tumor early diagnosis technology and improving tumor treatment efficiency are directions of current cancer research. Among them, the establishment of tumor model is the most critical factor (Balani et al., 2017; Drost and Clevers, 2018). Traditional 2 dimensional (2D) culture model of tumor cells had the characteristics of easy to handle and rapid to culture, which was widely used in tumor research and anti-tumor drug screening. However, there were two deficiencies in the 2D cells culture model, one was poor tumor heterogeneity, the other was new mutation produced during the culture process, which were the main reasons for poor efficiency of antitumor drugs in clinical trials (Kretzschmar, 2021). PDX (patient derived xenograft) model can effectively replicate tumor growth and preserve the heterogeneity of tumors in the tumor microenvironment, making it an ideal model for studying tumors and screening anti-tumor drugs. However, due to the low success rate of PDX model established, long time spent and huge costed, it was difficult to apply them on large scale applications (Ben-David et al., 2017; Byrne et al., 2017). The emergence of organoid model can greatly compensate for the shortcomings of 2D cell model and PDX model, and it has great potential in disease model constructing, anti-tumor drug screening and organ transplantation.
Organoids are multicellular mass formed by 3D (3 dimensional) culture of stem cells (including embryonic stem cells, induced pluripotent stem cells or adult tissue stem cells) in vitro, which have the ability to self-organize and self-renew. Furthermore, they can mimic the structure and function of the original tissues (Camp et al., 2017). Compared to traditional 2D cells model (e.g., cell lines, original cells), organoid model contains multiple cell compositions and 3D culture environments which better reflect the structural and functional characteristics of the original tissue so that they can maintain genetic and phenotypic stability during long-term expansion (Kaluthantrige Don and Huch, 2021). It largely overcomes the deficiency of stable genetic amplification arising from the process of 2D culture of cells. At the same time, the interaction and communication between cells and the pericellular matrix are preserved, which greatly reflects the growth of tissues in the body. Moreover, the organoid model is superior to PDX model because it is cultivated in vitro, it means that organoid model will be more cost-effective and rapidly in cultivation. The organoid technology had also been selected by several top journals as a major breakthrough in science (Rossi et al., 2018).
Currently, organoids had involved in many aspects of medicine and played an important role in basic and clinical medical research, especially in the study of tumorigenesis, tumor heterogeneity and tumor precision therapy. However, there were some shortcomings in the applications of organoids in tumors, such as the current inconsistency of tumor culture methods and poor reproducibility were the major drawbacks of organoids. In addition, the lack of fibroblasts and immune cells in the organoids lead to the inability of the organoids to truly reflect tumor microenvironment on tumor cells. This paper reviewed the history of organoids development, the establishment methods of tumor organoids, the applications of organoid technology in tumor treatment and the shortcomings of current tumor organoids. Moreover, we summarized the methods to compensate the deficiencies and discussed the future development directions of tumor organoids.
History of organoids development
Organoids were widely used in disease research in recent years, but the history of organoids development had undergone a long process of stagnation till the rapid development in the last decade (Figure 1). The origin of the organoids could be traced back to 1907, when H.V. Wilson, a professor at Baker Raleigh University, discovered mechanically separated sponge cells who could reassemble and self-organize into new functional sponge organisms under 3D culture (H.V.Wilson, 1907). This was the earliest report about organoid generation, however, the term “organoid” was first used to define cystic teratoma in a 2-month-old patient because it looked like an organ in appearance in 1946 (Smith and Cochrane, 1946). Subsequently, organoids were broadly defined as a class of cell clusters. In 1963, a blood vessel structure was observed in monolayers of cell cultures, suggesting that monolayers could also be cultured into different cellular compositions (Schneider et al., 1963). Nevertheless, the way in which organoids been induced was not well understood at that time. In 2002, researchers found that hepatocytes could spontaneously transform to biliary Epithelial Cells (BECs) under the condition of HGF and EGF addition (Michalopoulos et al., 2002). This discovery expanded the understanding of organoid induction and unlocked the key to organoid development rapidly. Additionally, in 2008, a study discovered that individual murine leucine-rich repeatcontaining G protein-coupled receptor 5 (LGR5+) intestinal stem cells could spontaneously form cortical tissue under certain conditions, and this process could be regulated by exogenous signals, this discovery laid the foundation for organoid development (Eiraku et al., 2008). Subsequently, a landmark study emerged in which researchers discovered that a single Lgr5+ stem cell could self-organize into an intestinal organ with intestinal crypt-villi structure through differentiation and self-assembly in vitro, suggesting that stem cells could self-organize and develop into functional organs under certain conditions (Sato et al., 2009). Since then, organoid researches entered a period of rapid development. In 2010, scientists found that Lgr5+ stem cells could be cultivated to form gastric organoids similar to pyloric epithelium in vitro, and noted that gastric organoids were important tools for studying gastric epithelial renewal, inflammation/disruption, and gastric cancer (Barker et al., 2010). In the same year, researchers discovered that single cell suspensions isolated from embryonic kidney tissues could transformed into a cell mass with the similar morphology and appropriate molecular markers to original tissues. Moreover, it connected distally to ureteral buds, marking the successful establishment of a kidney organoid (Unbekandt and Davies, 2010). One year later, human pluripotent stem cells were successfully grown to form intestinal organoids in vitro, and Wnt3A and FGF4 were discovered to be essential factors for intestinal organoids cultivation, which expanded the cell sources for organoids cultivation and accelerated the development of organoids (Spence et al., 2011). After that, retinal organoids developed from human pluripotent stem cells were successfully cultivation in 2013 (Bellapianta et al., 2022). In addition, it was also found that human pluripotent stem cells could spontaneously organize into brain organoids in matrigel (Lancaster et al., 2013). Since then, human prostate organoids, liver organoids, bile duct organoids, and lung organoids had been successfully cultivated (Chua et al., 2014; Tuveson and Clevers, 2019; Corro et al., 2020). Tumor organoids were also important direction for organoids development in recent year. Scientists successfully cultivated advanced prostate cancer organoids (Gao et al., 2014) and pancreatic cancer organoids (Boj et al., 2015) from biopsy tissue and circulating tumor cells. Thereafter, other tumor organoids such as glioblastomas organoids, colorectal cancer organoids, gastric cancer organoids and breast cancer organoids had been successfully cultivation (Hubert et al., 2016; Kretzschmar, 2021). Due to the absence of tumor microenvironment components such as immune cells, nerve cells and fibroblasts, tumor organoids are limited in their ability to mimic in vivo tumors. To explore the interplay between tumor organoids and their microenvironment, as well as the immune response of tumors, a co-culture system for tumor organoids was established (Yuan et al., 2022). Co-culturing mouse-derived gastric cancer organoids with autoimmune cells enables the investigation of PD-L1/PD-1 interactions within the tumor microenvironment (Chakrabarti et al., 2018). Additionally, researchers have co-cultured lymphocytes with tumor organoids to generate tumor-reactive T cells, which holds great potential for the immunotherapy of tumors (Dijkstra et al., 2018). Recently, a classical co-culture method of tumor organoids has been developed to predict the efficacy of precise treatment for gastric cancer patients, leading to an improved prognosis. This method utilizes tumor antigens to stimulate antigen-presenting dendritic cells (DCs), which are then co-cultured with CD8+ T cells in order to promote cytolysis and proliferation prior to co-culture with patient-derived gastric cancer organoids (Chakrabarti et al., 2021). Fibroblasts play a crucial role in the tumor-stromal interaction, co-culturing colorectal carcinoids with tumor-associated fibroblasts can enhance the plasticity of fibroblasts and provide insight into the role of tumor-associated fibroblasts in the progression of colorectal cancer (Luo et al., 2021). As the development of organoid technology, it is believed that more and more organoids will be successfully cultivated to help people better understand the pathogenesis of diseases and to provide ideal research models for disease treatment.
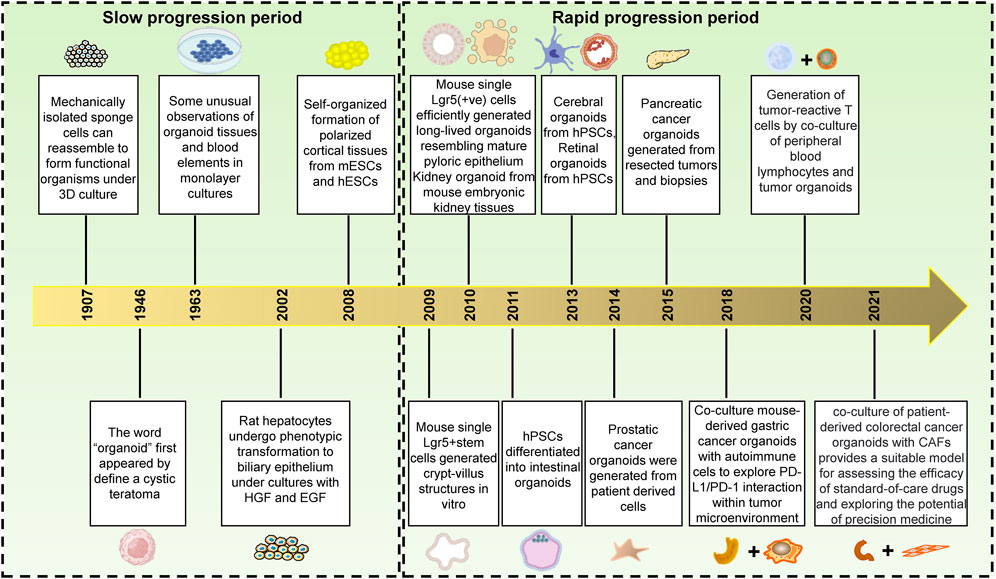
FIGURE 1. The history of organoids development. From 1907–2008, the development of organoids progressed slowly; however, from 2009 to present day, there has been a rapid advancement in organoid technology. This is evidenced by the initial successful culture of small intestinal crypt organoids and culminating in the maturation of tumor organoid co-culture. These milestones mark an increasingly widespread application of organoid technology within medicine.
The cultivation for tumor organoids
Tumors are important threat to human health and longevity, and it is also one of the hottest fields in medicine. Since the cultivation of small intestine organoids, organoid technology had been widely developed in biomedicine, and its great advantages been favored by tumor researchers. However, tumor organoids were difficult to cultivate, besides basal medium, numbers of cytokines were needed, furthermore, the cytokines needed for cultivating tumor organoids were varied between tumors (Figure 2) (Papapetrou, 2016).
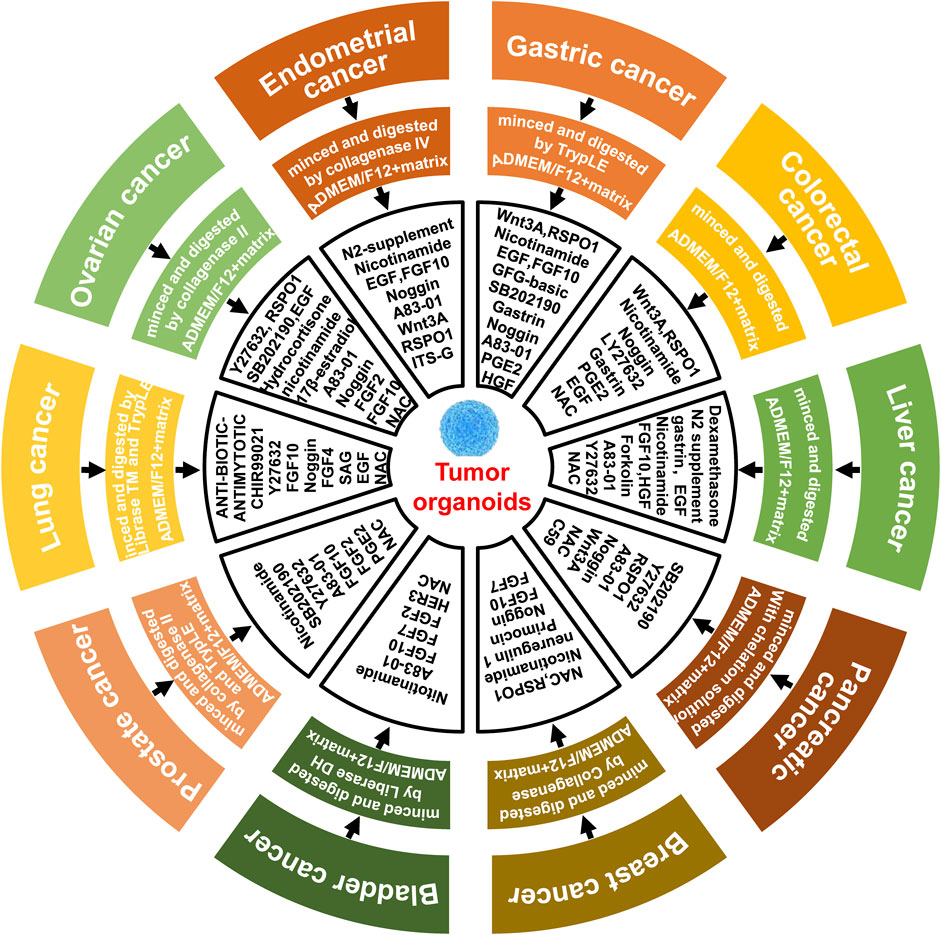
FIGURE 2. Summary of cytokines required for the cultivation of tumor organoids. In general, the establishment of organoid cultures involves the procurement of tumor tissue, followed by mechanical and enzymatic dissociation to obtain individual cells that are subsequently cultured in Advanced DMEM/F12 medium supplemented with basal membrane matrix and various cytokines. The specific cytokine requirements vary among different tumor types, including but not limited to gastric cancer (Vlachogiannis et al., 2018), colorectal cancer (van de Wetering et al., 2015), liver cancer (Broutier et al., 2017), pancreatic cancer (Seino et al., 2018), breast cancer (Sachs et al., 2018), bladder cancer (Whyard et al., 2020), prostate cancer (Zhou et al., 2021), lung cancer (Shi et al., 2020), ovarian cancer (Hill et al., 2018) and endometrial carcinoma (Boretto et al., 2019).
Next, we will make a brief summary of the current methods for tumor organoids cultivation. Gastric cancer is the fifth most common malignancy among new cases of tumors worldwide, and its mortality ranks the 4th among deaths caused by tumors (Sung et al., 2021). Yet the cultivation of gastric cancer organoids was complicated. Besides using ADMEM/F12 (Advanced Dulbecco’s Modified Eagles Medium with Nutrient Mixture F12 Hams) and basement membrane matrix, B27, EGF, Noggin, R-Spondin 1, Gastrin, FGF-10, FGF-basic, Wnt3A, Prostaglandin E2 (PGE2), Y-27632 (Rock inhibitor), Nicotinamide, A83-01 (inhibitor of TGF-β type I receptor), SB202190 (p38 MAPK inhibitor) and HGF should also be added to ensure the successful cultivation of gastric cancer organoids (Vlachogiannis et al., 2018). As for the culture of colorectal cancer organoids, besides human Advanced DMEM/F12 and basic membrane matrix, it was necessary to add B27, N-acetyl Cysteine, R-Spondin1, Nicotinamide, EGF, Gastrin, A83-01, SB202190, Prostaglandine E2 and LY27632 (van de Wetering et al., 2015). It showed that the cytokines to be added for organoid cultivation of different tumors were verified. Primary hepatocellular carcinoma, one of the highest mortality rate malignant tumors, was mainly divided into hepatocellular carcinoma and cholangiocellular carcinoma (Llovet et al., 2021). For hepatocellular carcinoma, dexamethasone was used in the cell culture medium instead of the conventional Noggin, R-spondin-1 and Wnt3A. in addition, Forkslolin was necessary. While in cholangiocytic hepatocellular carcinoma, the addition of R-spondin-1 was required to maintain cell growth, which further reflected the complexity of the tumor organoids cultivation (Broutier et al., 2017). Unlike other tumor organoids, removal of EGF and adding of Wnt inhibitors could achieve better success rate in the culture of pancreatic cancer organoids (Seino et al., 2018). Breast cancer is the highest incidence tumor among women, with increasing new cases each year. The culture medium for breast cancer organoids was slightly different from other tumor organoids, such as Wnt3A, which was necessary for the culture of other tumor organoids, but dispensable for breast cancer organoids cultivation; while Neuregulin 1 was essential for maintaining efficient regeneration and long-term expansion of breast cancer organoids. EGF has double-sided effect on breast cancer organoids cultivation, low concentration of EGF inhibited cell proliferation but promoted tumor organoids self-organizing while high concentration of EGF promoted cell proliferation but caused tumor organoids disintegration and loss function of self-organizing. In addition, SB202190 was another important determinant for the establishment of breast cancer organoids (Sachs et al., 2018). Most bladder cancers were uroepithelial cancers and most of them were non-muscle invasive bladder cancers which usually had a good prognosis with high cost of treatment (Humphrey et al., 2016). The cultivation of organoids helped to reduce the cost of bladder cancer treatment. It was mainly done by using CTOS medium (containing advanced Dulbecco’s modified Eagle medium/F-12, B27, A83-01, N-acetylcysteine and nitotinamide). Moreover, it is necessary to supplement FGF10, FGF7, FGF2 and HER3 (Whyard et al., 2020). Prostate cancer is one of the most common types of cancer in men. According to the data in 2020, the risk of prostate cancer in men was 7.3%, only less than lung cancer; at the meantime, the death rate of prostate cancer reached to 3.8%, which became a major threat to men’s health (Siegel et al., 2021; Sung et al., 2021). For prostate cancer organoid cultivation, A83-01, FGF10, FGF2, prostaglandin E2(PGE2), Nicotinamide and p38 inhibitor SB202190, N-acetylcysteine, B27 and Rho kinase inhibitor Y-27632 were added into the general organoid culture medium. However, for mice prostate cancer organoids, FGF10, FGF2, PGE2, Nicotinamide and SB202190 were not necessary (Zhou et al., 2021). Lung cancer is the most common malignant solid tumor worldwide. Due to the importance and special nature of the lung organ in the human body, the clinical manifestations of lung cancer in the early stage were not obvious, which made it easier to confuse with other infections and thus overlooked. Usually, the tumor had already progressed to the middle and late stage when confirmed; therefore, timely and accurately screening of appropriate anti-tumor drugs was particularly important for lung cancer patients (Nasim et al., 2019). The cultivation of lung cancer organoids greatly reduced the time for drug screening. In addition to the common cytokines for lung cancer organoid cultivation, Antibiotic-Antimycotic, CHIR99021, FGF4, SAG (Smoothened Ligand) and Y27632 were needed (Shi et al., 2020). Similar to lung cancer, ovarian cancer is also a type of malignant tumor without obvious early symptoms, and about 70% of ovarian cancer patients had progressed to advanced stages when diagnosed, resulting in a 5-year survival rate of less than 40% (Yang et al., 2021). Currently, there were several methods to culture ovarian cancer organoids, but the highest success rate was achieved by using a combination of matrix gel and Advanced DMEM/F12 basal medium supplemented with Glutamax, B27, Nicotinamide, EGF, FGF2, FGF10, A83-01, N-acetylcysteine, Noggin, Rspondin1, p38 inhibitor, Hydrocortisone, and Y27632, resulting in 80%–90% success rate of carcinoid culture (Hill et al., 2018). Moreover, tumor organoids have been successfully cultivation for kidney cancer, endometrial cancer, cervical cancer and glioma (Boretto et al., 2017; Boretto et al., 2019; Grassi et al., 2019; Maru et al., 2019; Jacob et al., 2020).
As we can see from the different methods of tumor organoid establishment described above, the initial raw material for tumor organoids cultivation were tumor cells obtained by mechanical or enzymatic digestion from cancer tissues, and then special medium were used to culture these isolated tumor cells to generate different tumor organoids. The culture medium of these different tumor organoids almost all contained the same basal medium and cell growth factors, but only a few growth factors varied in different tumor organoids, indicating different preferences for organoid formation and reflecting the complexity among tumors (Aboulkheyr Es et al., 2018). And it also implied that these cytokines were the determinants of tumor organoids to be cultivated successfully. Conversely, these cytokines might also play the same function through artificial addition or replacement, so that the culture of different tumor organoids could be standardized, and then the purpose of large-scale batch production of tumor organoids can be achieved and widely used in clinical studies for tumors.
Application of organoids in cancer
With the development and improvement of organoid technology, organoids were also widely used in the biomedical research especially in tumor research. The mechanism of tumorigenesis, the screening of anti-tumor drugs and the tumor precision therapy were three important directions and challenges in the field of oncology at present. Since their characteristic of spontaneously organization and preservation of genetic factors and tumor heterogeneity, tumor organoids were ideal tools for cancer research. In the following context, we reviewed the role of tumor organoids in tumorigenesis, screening of antitumor drugs, and tumor precision therapy (Figure 3).
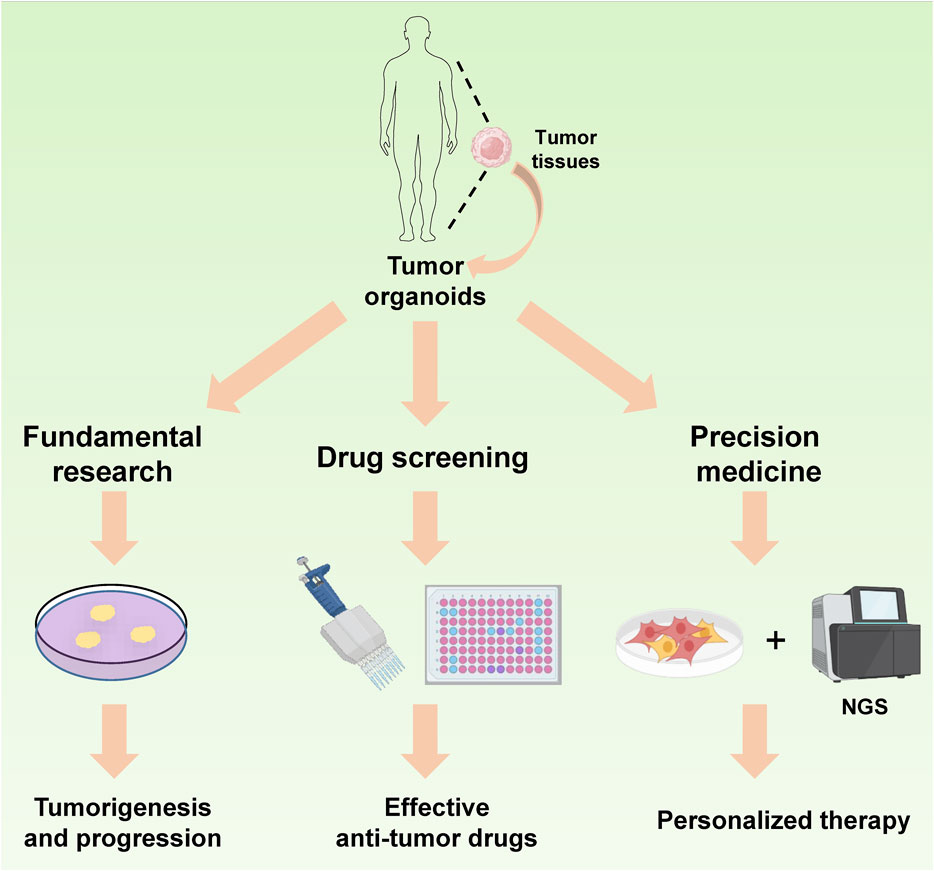
FIGURE 3. Application of organoids in cancer. The tumor organoids serve as an ideal model for investigating tumor progression. Moreover, owing to their inherited characteristics from parental tumor tissues and rapid culture ability, they can be effectively utilized for antitumor drug screening and play a crucial role in the precision treatment of cancer patients.
The role of organoids in the study of tumorigenesis mechanism
Tumor organoids can mimic the process of tumorigenesis, it implies that the mechanism of tumorigenesis could be studied through the cultivation of tumor organoids (Cimen Bozkus and Bhardwaj, 2021). Uhrf2 is high expressed in colorectal cancer, and its expression was positive associated with the grade and prognosis of colorectal cancer significantly (Lu et al., 2014). Uhrf2 deletion significantly reduced intestinal cancer organoid formation and reduced the number of tumor stem cells. Further study revealed that Uhrf2 could bind and SUMOylate TCF4, an important transcription factor downstream of the Wnt signaling pathway, thus stabilizing TCF4 expression and activating Wnt signaling, and finally Uhrf2 promoted the progression of intestinal cancer. Therefore, Uhrf2 was also considered as a target for the treatment of intestinal cancer (Muthusamy et al., 2020). Cancer-associated fibroblasts (CAF) are an important promoter in tumorigenesis, but the regulatory mechanism of tumor-associated fibroblasts is still unclear. Researchers found that tumor-secreted TGFβ and IL1 took an important role in the development of CAF heterogeneity via organoid model in pancreatic ductal adenocarcinoma. IL1 could produce inflammatory CAF by inducing LIF expression and activate downstream JAK/STAT signaling, while TGFβ antagonized this phenomenon by inhibiting IL-1R expression and promoting CAF differentiation into myofibroblasts (Biffi et al., 2019). In a bladder cancer organoid model, the used of the Wnt pathway activator CHIR99021 promoted bladder cancer cell proliferation and bladder cancer organoid formation significantly. In contrast, the use of RNAi to interfere the expression of β-catanin, an important protein in Wnt signaling, blocked bladder cancer organoid growth significantly, this confirmed the importance of Wnt signaling in bladder cancer growth (Yoshida et al., 2018). In an organoid model of intestinal cancer, researchers found that Wnt, Notch and Myb signaling took distinct roles in tumor development, it revealed pathway-dependent changes occurred during tumor initiation (Germann et al., 2014). By combining organoid technology and CRISPR/Cas9 technology, it is conductive to study the effect of gene mutations on tumorigenesis and development in a targeted manner. APC and TP53 genes are common mutated genes in intestinal cancer. When APC and TP53 are mutated in intestinal stem cells, a large number of aneuploidy cells can be found through the construction of organoid model, it was found that the organoids could develop into adenocarcinoma after tumor organoids transplanted into immunodeficient mice, this suggested that APC and TP53 gene mutations took an important driving role in the development of colorectal carcinogenesis. In contrast, transplantation of the same gene-edited organoid into the kidney capsule of mice resulted in tumor formation, albeit without metastasis to other organs, implying that the invasive behavior of tumors may necessitate involvement of additional genes (Matano et al., 2015). ARID1A mutations are among the most common gene aberrations in human cancers. The oncogenic consequences of ARID1A mutations in human cells are unclear due to the lack of forward genetic models. By combining gastric cancer organoids and CRISPR/Cas9 technologies, it was found that knockdown of ARID1A caused morphological dysplasia, tumorigenicity and mucus differentiation. In addition, the researchers identified BIRC5/survivin signaling as a key signal for early tumorigenesis caused by ARID1A knockdown (Lo et al., 2021). In CRC, researchers validated some CRC driver genes via utilizing CRISPR-Cas9 in instestinal tumor organoids and human CRC-derived organoids. They found that co-occurent mutations in receptors for activing and TGF-β synergistically promote tumorigenesis. Moreover, they found that Acvr1b, Acvr2a and Arid2 could function as tumor suppressor genes in CRC (Takeda et al., 2019). Another study had also showed that CRISPR-Cas9 technology could applied in patient-specific functional genomics screening, they screened and identified TGFBR2 as the most revalent tumor suppressor gene (Michels et al., 2020). It is indicated that the combination of organoid and gene editing technology could better investigate the role of tumor driver genes in the development of tumorigenesis.
The role of organoids in the screening of anti-tumor drugs
Drug screening is an important part of disease treatment, and it will spend lot of time and money to screen for an effective clinical drug, which mainly due to the extremely low success rate (Kondo and Inoue, 2019). A study analyzed 406,038 clinical trials and 21,143 compounds involved in this clinical trials between 2000 and 2015 and found that the overall clinical trial success rate was only 13.8%, while the success rate for antitumor drug screening was even lower, it was only 3.4% (Wong et al., 2019). Drug screening consists of three parts: the candidate compounds or drugs, the screening methods, and the drug screening model, and the drug screening model is the most critical part among them, which is the object of drug treatment (Pushpakom et al., 2019; Tyers and Wright, 2019). Since the first report on the use of leukemia animal model for drug screening in 1950, an increasing number of human tumors had been transplanted into mice to construct mouse models for the screening of antitumor chemotherapeutic drugs (Yoshida, 2020). The most common model was the transplantation of a tumor cell lines into a mouse subcutaneously. However, these models could not truly mimic the growth of tumors due to the single cellular component of tumor cell lines and the dominant growth of tumor cells after passing through generations, which were mostly used for the study of tumor pathogenesis (Hutchinson and Kirk, 2011). In addition, in situ tumor transplantation model had also been developed for antitumor drugs screening. The patient-derived tumor tissues were digested or simply cut into small pieces and inoculated into the subcutaneous of mouse, known as the PDX (patient-derived xenograft) model. But it had the deficiency of time-consuming and difficulty in constructing, moreover, the cost was huge to construct PDX model (Hidalgo et al., 2014). Similarly, traditional 2D culture model had deficiency of single composition and susceptibility during long-term culturing. They were not the ideal models for antitumor drugs screening (Pereira and Williams, 2007; Calvert et al., 2016).
In recent years, with the rapid development of tumor organoid technology, a new anti-tumor drug screening model had been established, it was called tumor organoid model. The tumor organoid model had the characteristics of short time consuming, inexpensive and better mimicked the growth of tumor. It could be used as an effective biological model for preclinical studies and is widely appreciated. A study on a non-small cell lung cancer organoid model found that organoids constructed from surgically resected primary non-small cell lung cancer tissues preserved the tumorigenic properties of the original tumor tissues, such as the cytological characteristics of the malignancy and xenograft formation. In addition, whole-exome sequencing and RNA sequencing also showed that the organoids preserved the mutations and copy number aberrations from the parental tumor tissues, demonstrating the consistency in tumor cell morphology and genetic specificity between organoids and parental tumor tissues. Furthermore, organoid model retains the same sensitivity to tumor-targeted therapy as the parental tumor tissues, which demonstrates the reliability of the tumor organoid model in anti-tumor drug screening (Shi et al., 2020). Another study on lung cancer organoids showed that a large number of lung cancer organoids could be generated simultaneously by combining organoid technology and integrated superhydrophobic microwell array chip (InSMAR-Chip) technology. It was sufficient to produce a clinically meaningful drug response within a week. The drug test result fitted well with xenografts from cancer tissues in tumor gene mutations and drug sensitivity, which also provided a viable assay for anti-tumor drug screening and an important direction for anti-tumor drug screening (Hu et al., 2021). The establishment of tumor organoid biobank had greatly facilitated the screening of antitumor drugs (Table 1). In colorectal cancer, 83 compounds had been screened simultaneously by constructing a colorectal cancer organoid biospecimen library. Cetuximab was found to be highly efficacious for KRAS wild-type organoids, while Nutlin-3a was also highly effective in killing TP53 wild-type organoids, it was consistent with the results in clinical trials (van de Wetering et al., 2015). In gastric cancer, 37 compounds were examined by constructing a library of patient-derived gastric cancer organoid biospecimens, Some new potent compounds such as Abemaciclib, Napabucasin and ATR inhibitor VE-822 had been identified and showed antitumor effects in clinical trials (Yan et al., 2018). In hepatocellular carcinoma, 129 classes of antitumor drugs were tested by constructing a library of liver cancer organoid samples. In addition to FDA-approved drugs for liver cancer treatment, liver cancer organoids were found to be significantly sensitive to some new anti-tumor drugs (for which liver cancer was not indicated) (Li et al., 2019). In breast cancer, some signaling pathway inhibitors were detected by combining breast cancer organoids and gene sequencing technology, it was found that the sensitivity of breast cancer organoids to drugs was consistent with in vivo models of transplanted tumors in breast cancer patients as well as patient-derived mice (Sachs et al., 2018). A screening of 45 compounds for breast cancer found that docetaxel got an excellent result (Guillen et al., 2022). Moreover, a satisfactory result was obtained by testing the sensitivity of tumor organoids to drugs in bladder cancer (Lee et al., 2018) and ovarian cancer (Jabs et al., 2017). In a pancreatic cancer organoid drug screening study, researchers screened 76 non-clinical compounds and found that PRMT5 inhibitor EZP015556 could be a potential candidate for MTAP-negative pancreatic cancer (Driehuis et al., 2019). In addition, the enormous potential of tumor organoids for oncological drug screening has been demonstrated in prostate cancer and endometrial cancer, either alone or in combination with other drugs (Kiyohara et al., 2016; Karkampouna et al., 2021). Genetic mutations are a frequent feature of colorectal cancer. A study of colorectal carcinoids with KRAS mutations showed that the combination of KRAS inhibitors and EGFR-MEK-ERK pathway inhibitors could significantly inhibited organoids growth, which was also validated in a normal colorectal organoid model with KRAS mutations introduced by CRISPR technology (Verissimo et al., 2016). In tumors, various mutations can also lead to variations in the sensitivity of tumors to drugs. In a test of drug sensitivity in lung cancer patients, the researchers screened 26 antitumor drugs using lung cancer organoids and found that organoids with mutations in EGFR responded to gefitinib and osimertinib differently (Chen J H et al., 2020).
These all indicated the reliability of tumor organoids in anti-tumor drug screening. In particular, the combination of organoid technology with high-throughput technologies such as chip technology would greatly expand the number of tumor samples while preserving tumor heterogeneity. It was beneficial for large-scale drug screening and improved the efficiency and scope of drug screening, organoids are important tool for future antitumor drug development and clinical application.
The role of organoids in tumor precision therapy
With the development of biomedicine, it was found that drug response of tumor patients varied even though drugs were treated at the same dose, especially for some target-specific antitumor drugs (Ward et al., 2021). Further studies revealed that there are various mutations in the tumor tissues of different tumor patients, which might be the reason why anti-tumor targeted drugs did not work well for partial patients (Chen and Chen, 2020). The precision therapy of tumors is an effective response to the current clinical treatment of tumors. However, because of the complexity and heterogeneity of tumors, detection of mutations in a single target alone did not truly reflect the complexity of mutations in tumors. Moreover, new mutations were producing when treated with targeted anti-tumor drugs because of tumor adaptability, which were harmful for tumor patients (Xu et al., 2022). The emergence of tumor organoid model can greatly alleviate this situation. Patient-derived xenografts (PDX) model is the most ideal preclinical diagnostic model for tumors, However, due to the lengthy and costly of PDX model and its low success rate, it is not the best choice. Moreover, PDX requires more tumor tissue as the material for construct a PDX model.
While tumor organoids are much shorter and less expensive to construct compared to PDX model, and the success rate of construction is much higher than that of PDX model (Shiihara and Furukawa, 2022). Moreover, the construction of tumor organoids model demands few tumors tissue samples, even only tumor puncture samples (needle biopsy) can meet the requirements. At the same time, tumor organoids can also greatly retain the characteristics of the parental tumor, a variety of anti-tumor drugs can be screened at the same time using tumor organoids model, so that the most suitable anti-tumor drugs for patients can be screened in a short time, which can greatly improve the therapeutic effect of tumor patients and prolong the life cycle of patients (Ren et al., 2022). In a study for prostate cancer organoid, targeting organoid model of prostate cancer, researchers had found that multiple FDA-approved drugs, including multi-kinase inhibitors (ponatinib, sunitinib, sorafenib), could be effectively tested to screen for the most appropriate therapeutic agent by constructing organoid model (Karkampouna et al., 2021). In another study of organoid biospecimen banking in lung cancer patients, researchers have found that the most suitable anti-tumor drugs could be selected by screening tumor organoids, because tumor organoids retain the genetic characteristics of parental tumor tissues. For example, for BRCA2-mutated organoids, the use of olaparib could achieve better results, while for EGFR-mutated organoids, erlotinib was better. When EGFR mutations and MET amplification exist at the same time, crizotinib was the best choice (Kim et al., 2019). In a study of colorectal cancer organoid, the researchers tested 85 compounds (including targeted therapy compounds) in intestinal tumor organoids to explore the sensitivity of tumor organoids to chemotherapy drugs. It was found that TP53-deficient colorectal organoids were highly resistant to MDM2 inhibitors, whereas RAS-mutated colorectal organoids were insensitive to EGFR inhibitors. Furthermore, RNF43-mutated colorectal organoids were particularly sensitive to inhibitors of Wnt signaling, suggesting a classical approach to RNF43-mutated colorectal cancer (Aboulkheyr Es et al., 2018). Additionally, organoid had enabled the screening of antitumor drugs with fewer side effects, thus effectively reduced the side effects during antitumor treatment. In an organoid culture for colorectal diseases, researchers had cultured colonic organoids from induced pluripotent stem cells from patients with familial adenomatous polyposis (FAP-iPSCs). Antibiotic geneticin was screened out by this drug screening platform, it can effectively target colorectal cancer organoids with abnormal Wnt activity, but does not affect the growth of normal APC-mutated colonic organoids. It exhibited the important role of tumor organoids in drug selection for colorectal diseases (Crespo et al., 2017). Tumor organoids had also shown their advantage in renal cell carcinoma. Multiple anti-tumor drugs such as sunitinib, pazopanib, cabozantinib, axitinib and sorafenib could be screened for tumor treatment by constructing patient-derived renal cell carcinoids (Kazama et al., 2021). Malignant rhabdomyosarcoma (MRT) is one of the most aggressive childhood malignancies, but there are currently no effective treatment options for malignant rhabdomyosarcoma and the prognosis is poor. By constructing an organoid model of malignant rhabdomyosarcoma and using the organoid model of normal tissue as a control, it could be used to screen for antitumor drugs that effectively inhibit malignant rhabdomyosarcoma. A neddylation inhibitor MLN4924 was screened out by this model and proved that a single dose of MLN4924 could significantly prolong survival of patients. Exhibiting the advantages of tumor organoid in screening antitumor drugs with few sides’ effects (Calandrini et al., 2021). In ovarian and breast cancers, organoids also offered advantage in screening antitumor drugs (Kopper et al., 2019; Chen P et al., 2021).
Apart from in situ tumors, tumor organoids can also be used for drug sensitivity analysis by culturing tumor cells in malignant effusions to form tumor organoid in vitro. An experimental study on malignant effusion specimens from patients with high-grade serous ovarian cancer showed that by recovering multicellular spheroids in malignant effusion and culturing organoids, effective therapeutic drugs could be screened for individualized treatment of patients via this organoids (Chen H et al., 2020). The association of genes with drugs took a key role in individualized and targeted tumor therapy. A strong correlation between gene mutations and the therapeutic effect of chemotherapeutic drugs had been demonstrated and referred to mutation-based drug sensitivity (MDS). Because genomic analysis was not sufficient to identify effective treatment options for most patients with advanced cancer, drug screening of tumor organoids can clarify unknown drug responses (Pauli et al., 2017).
Disadvantages of organoids in cancer therapy
Tumor organoids have the advantages including short culture time, low cost, and high construction success rate; however, they also have some deficiencies. For example, Although the overall success rate of tumor organoids construction was higher compared to the PDX tumor model, but they were uneven and depended on the type of tumors. In addition, it was also highly susceptible to contamination during the cultivation of tumor organoids (Dijkstra et al., 2020). Until now, there has been a lack of uniform standards for the cultivation of organoids, slight differences in organoid culture methods and culture conditions established by each laboratory result in diverse success rates. Furthermore, tumor organoids were built up in a shorter period of time compared to PDX model, it still took a short period of time for organoid model construction. When the tumor organoids were used for screening appropriate drugs or other analysis for patients those with mid to late-stage tumors, there was not much time to wait for the best drug match results (Veninga and Voest, 2021). Moreover, the cultivation of organoids requires special medium as well as the addition of different cytokines and inhibitors, which were also costly. In addition, among these inhibitors required for tumor organoids, some of them were also signaling pathway inhibitors, such as ALK inhibitor A83-01 and P38 inhibitor SB202190. And these inhibitors could be adverse factors for drug screening against related signaling pathways (Grassi et al., 2019; Veninga and Voest, 2021). Another problem was the lack of fibroblasts, immune cells and nerves in the tumor organoid model, they played an important role in tumor growth (Neal et al., 2018; Cattaneo et al., 2020; Kim et al., 2020). For example, tumor-associated fibroblasts could secrete cytokines and promote tumor growth and abnormal tumor-associated stroma (Chen Y et al., 2021), Immune cells, such as M2 macrophages, are also critical to the malignant progression of tumors (Entezari et al., 2022). Therefore, the organoid model could not display the tumor microenvironment completely and reality, it was more outstanding when immunotherapy drugs were screened (Xia et al., 2019). Matrix is an important support of most tumor organoids, most of them were cultured using animal-derived mechanisms or collagen, but these matrices contain many unknown growth factors in addition to laminin, collagenIV and entactin. These were the safety hazards in clinical applications (Gjorevski et al., 2016; Blondel and Lutolf, 2019).
Conclusion
Tumor organoid model was widely used in recent years, they were produced by using stem cell-derived tissues (embryonic stem cells, induced pluripotent stem cells, and tumor stem cells) and cultivated under specific conditions (Garreta et al., 2021). Organoids met the needs of biomedical research in many aspects, including disease development mechanism, drug screening, personalized therapy and tissue regeneration (Artegiani and Clevers, 2018). This paper reviewed the history of organoid, the methods for organoids establishment, the application of organoid in oncology (including tumor organoid in basic tumor research, the role of tumor organoid in drug screening and the role of organoid in tumor precision therapy) and discussed the shortcomings of current organoid in oncology research. We found that tumor organoids played an important role in exploring the occurrence and development of tumors. Whole genome sequencing and RNA sequencing have demonstrated that the organoid maintains the genetic characteristics of the original tumor tissue, including its genetic mutations and copy number variations. Additionally, the organoid exhibits histopathological features consistent with those of the parental tumor tissue (Romero-Calvo et al., 2019; Shi et al., 2020). They were the ideal model for tumor research as they could reflect the occurrence and development of tumors more realistically. However, there were few clinical trials were operated via tumor organoids, which might be related to the complicated and requirements of tumor organoid establishment. It is believed that the potential of tumor organoids in basic tumor research would be further explored with the development of organoid technology. As we all know, the screening of antitumor drugs was a long and costly process and conventional 2D cultured cells were widely used with less effective, resulting in a great waste of human and financial resources. Because long-term culturing would lose some genetic information and produce new mutations in tumor cells. Moreover, limited by the 2D culture dish, the 3D environment of tumor growth cannot be truly reflected as the target cells were relatively single, which cannot truly simulate the tumor progression. Therefore, most of the anti-tumor drugs were effective in 2D culture model but not in clinical trials (Ben-David et al., 2017; Byrne et al., 2017; Zhou et al., 2017). In contrast, tumor organoids had more advantage in drug screening because they cultured in a 3D growth environment that preserved the heterogeneity and growth characteristics of the origin tumor tissues, they could mimic the environment of tumor reality. Meanwhile, for tumor patients, especially those whose tumors had progressed to the middle or late stages, the tumor organoids could screen out the appropriate anti-tumor drugs quickly. Due to the ability of organoids to simultaneously screen a large number of antitumor drugs and their short screening period, they are capable of efficiently identifying the most suitable chemotherapy drugs for patients in a timely manner. Therefore, they are highly applicable for precision therapy in tumor treatment. However, we also found an important problem with the current tumor organoids technology-the success rate of building tumor organoids varied greatly among different tumor types and different subject groups (Drost and Clevers, 2018). The reason was that there was no uniform standard for the establishment of tumor organoids currently. The cytokines used for tumor organoid culture were varied among tumors and research groups, and it was difficult to construct tumor organoids on a large scale successfully, at the same time, it would limit the application of tumor organoids. Furthermore, the lack of tumor-associated fibroblasts, immune cells, nerve cells and blood vessels in tumor organoids could not fully mimic the tumor microenvironment, which was another important factor to restrict tumor organoids application (Workman et al., 2017; LeSavage et al., 2022). Fortunately, researchers were currently trying to solve this problem by co-culturing organoid cells with immune cells and fibroblasts, and had achieved good outcomes in tumor organoids (Cattaneo et al., 2020; Zhao et al., 2021; Sun et al., 2022). Overall, we summarized the role of organoid technology in tumor development, drug screening and precision therapy. Although there are still many problems of tumor organoids, it is believed that these problems will be overcome with the research progresses, and tumor organoids might be a powerful tool for tumor treatment.
Author contributions
XJ, LO, QP, XX, NW, ST, WY, JL, LX, MP, YT, XL, and MS collected the related paper and drafted the manuscript. YS, YZ, and QL participated in the design of the review and draft the manuscript. All authors contributed to the article and approved the submitted version.
Funding
This work was supported in part by grants from the following sources: the National Natural Science Foundation of China (82203233, 82202966, 82173142, 81972636, 81872281), the Natural Science Foundation of Hunan Province (2022JJ80078, 2020JJ5336), the Research Project of Health Commission of Hunan Province (202203034978, 202109031837, 20201020), Key Research and Development Program of Hunan Province (2022SK 2051), Hunan Provincial Science and Technology Department (2020TP1018), the Changsha Science and Technology Board (kh2201054), Ascend Foundation of National cancer center (NCC201909B06), Hunan Cancer Hospital Climb Plan (ZX2020001-3, YF2020002), and by China Postdoctoral Science Foundation (2022TQ0104, 2022M721118).
Conflict of interest
The authors declare that the research was conducted in the absence of any commercial or financial relationships that could be construed as a potential conflict of interest.
Publisher’s note
All claims expressed in this article are solely those of the authors and do not necessarily represent those of their affiliated organizations, or those of the publisher, the editors and the reviewers. Any product that may be evaluated in this article, or claim that may be made by its manufacturer, is not guaranteed or endorsed by the publisher.
Abbreviations
2D, 2 dimensional; BECs, biliary Epithelial Cells; CAF, Cancer-associated fibroblasts; FAP, familial adenomatous polyposis; InSMAR-Chip, integrated superhydrophobic microwell array chip; LGR5, leucine-rich repeatcontaining G protein-coupled receptor 5; MDS, mutation-based drug sensitivity; MRT, Malignant rhabdomyosarcoma; PDX, patient derived xenograft; PGE2, prostaglandin E2.
References
Aboulkheyr Es, H., Montazeri, L., Aref, A. R., Vosough, M., and Baharvand, H. (2018). Personalized cancer medicine: An organoid approach. Trends Biotechnol. 36, 358–371. doi:10.1016/j.tibtech.2017.12.005
Artegiani, B., and Clevers, H. (2018). Use and application of 3D-organoid technology. Hum. Mol. Genet. 27, R99–R107. doi:10.1093/hmg/ddy187
Balani, S., Nguyen, L. V., and Eaves, C. J. (2017). Modeling the process of human tumorigenesis. Nat. Commun. 8, 15422. doi:10.1038/ncomms15422
Barker, N., Huch, M., Kujala, P., van de Wetering, M., Snippert, H. J., van Es, J. H., et al. (2010). Lgr5(+ve) stem cells drive self-renewal in the stomach and build long-lived gastric units in vitro. Cell Stem Cell 6, 25–36. doi:10.1016/j.stem.2009.11.013
Bellapianta, A., Cetkovic, A., Bolz, M., and Salti, A. (2022). Retinal organoids and retinal prostheses: An overview. Int. J. Mol. Sci. 23, 2922. doi:10.3390/ijms23062922
Ben-David, U., Ha, G., Tseng, Y. Y., Greenwald, N. F., Oh, C., Shih, J., et al. (2017). Patient-derived xenografts undergo mouse-specific tumor evolution. Nat. Genet. 49, 1567–1575. doi:10.1038/ng.3967
Biffi, G., Oni, T. E., Spielman, B., Hao, Y., Elyada, E., Park, Y., et al. (2019). IL1-Induced JAK/STAT signaling is antagonized by TGFβ to shape CAF heterogeneity in pancreatic ductal adenocarcinoma. Cancer Discov. 9, 282–301. doi:10.1158/2159-8290.CD-18-0710
Blondel, D., and Lutolf, M. P. (2019). Bioinspired hydrogels for 3D organoid culture. Chim. (Aarau) 73, 81–85. doi:10.2533/chimia.2019.81
Boj, S. F., Hwang, C. I., Baker, L. A., Chio, , Engle, D. D., Corbo, V., et al. (2015). Organoid models of human and mouse ductal pancreatic cancer. Cell 160, 324–338. doi:10.1016/j.cell.2014.12.021
Boretto, M., Cox, B., Noben, M., Hendriks, N., Fassbender, A., Roose, H., et al. (2017). Development of organoids from mouse and human endometrium showing endometrial epithelium physiology and long-term expandability. Development 144, 1775–1786. doi:10.1242/dev.148478
Boretto, M., Maenhoudt, N., Luo, X., Hennes, A., Boeckx, B., Bui, B., et al. (2019). Patient-derived organoids from endometrial disease capture clinical heterogeneity and are amenable to drug screening. Nat. Cell Biol. 21, 1041–1051. doi:10.1038/s41556-019-0360-z
Broutier, L., Mastrogiovanni, G., Verstegen, M. M., Francies, H. E., Gavarro, L. M., Bradshaw, C. R., et al. (2017). Human primary liver cancer-derived organoid cultures for disease modeling and drug screening. Nat. Med. 23, 1424–1435. doi:10.1038/nm.4438
Byrne, A. T., Alferez, D. G., Amant, F., Annibali, D., Arribas, J., Biankin, A. V., et al. (2017). Interrogating open issues in cancer precision medicine with patient-derived xenografts. Nat. Rev. Cancer 17, 254–268. doi:10.1038/nrc.2016.140
Calandrini, C., van Hooff, S. R., Paassen, I., Ayyildiz, D., Derakhshan, S., Dolman, M. E. M., et al. (2021). Organoid-based drug screening reveals neddylation as therapeutic target for malignant rhabdoid tumors. Cell Rep. 36, 109568. doi:10.1016/j.celrep.2021.109568
Calvert, M. E., Ward, A. M., Wright, G. D., and Bard, F. (2016). New developments and novel applications in high throughput and high content imaging. Cytom. A 89, 705–707. doi:10.1002/cyto.a.22921
Camp, J. G., Sekine, K., Gerber, T., Loeffler-Wirth, H., Binder, H., Gac, M., et al. (2017). Multilineage communication regulates human liver bud development from pluripotency. Nature 546, 533–538. doi:10.1038/nature22796
Cattaneo, C. M., Dijkstra, K. K., Fanchi, L. F., Kelderman, S., Kaing, S., van Rooij, N., et al. (2020). Tumor organoid-T-cell coculture systems. Nat. Protoc. 15, 15–39. doi:10.1038/s41596-019-0232-9
Chakrabarti, J., Holokai, L., Syu, L., Steele, N., Chang, J., Dlugosz, A., et al. (2018). Mouse-derived gastric organoid and immune cell Co-culture for the study of the tumor microenvironment. Methods Mol. Biol. 1817, 157–168. doi:10.1007/978-1-4939-8600-2_16
Chakrabarti, J., Koh, V., So, J. B. Y., Yong, W. P., and Zavros, Y. (2021). A preclinical human-derived autologous gastric cancer organoid/immune cell Co-culture model to predict the efficacy of targeted therapies. J. Vis. Exp. 173, 61443. doi:10.3791/61443
Chen, H., Gotimer, K., De Souza, C., Tepper, C. G., Karnezis, A. N., Leiserowitz, G. S., et al. (2020). Short-term organoid culture for drug sensitivity testing of high-grade serous carcinoma. Gynecol. Oncol. 157, 783–792. doi:10.1016/j.ygyno.2020.03.026
Chen, H. W., and Chen, T. W. (2020). Genomic-guided precision therapy for soft tissue sarcoma. ESMO Open 5, e000626. doi:10.1136/esmoopen-2019-000626
Chen, J. H., Chu, X. P., Zhang, J. T., Nie, Q., Tang, W. F., Su, J., et al. (2020). Genomic characteristics and drug screening among organoids derived from non-small cell lung cancer patients. Thorac. Cancer 11, 2279–2290. doi:10.1111/1759-7714.13542
Chen, P., Zhang, X., Ding, R., Yang, L., Lyu, X., Zeng, J., et al. (2021). Patient-derived organoids can guide personalized-therapies for patients with advanced breast cancer. Adv. Sci. (Weinh) 8, e2101176. doi:10.1002/advs.202101176
Chen, Y., McAndrews, K. M., and Kalluri, R. (2021). Clinical and therapeutic relevance of cancer-associated fibroblasts. Nat. Rev. Clin. Oncol. 18, 792–804. doi:10.1038/s41571-021-00546-5
Chua, C. W., Shibata, M., Lei, M., Toivanen, R., Barlow, L. J., Bergren, S. K., et al. (2014). Single luminal epithelial progenitors can generate prostate organoids in culture. Nat. Cell Biol. 16, 951–954. doi:10.1038/ncb3047
Cimen Bozkus, C., and Bhardwaj, N. (2021). Tumor organoid-originated biomarkers predict immune response to PD-1 blockade. Cancer Cell 39, 1187–1189. doi:10.1016/j.ccell.2021.08.003
Corro, C., Novellasdemunt, L., and Li, V. S. W. (2020). A brief history of organoids. Am. J. Physiol. Cell Physiol. 319, C151–C165. doi:10.1152/ajpcell.00120.2020
Crespo, M., Vilar, E., Tsai, S. Y., Chang, K., Amin, S., Srinivasan, T., et al. (2017). Colonic organoids derived from human induced pluripotent stem cells for modeling colorectal cancer and drug testing. Nat. Med. 23, 878–884. doi:10.1038/nm.4355
Dijkstra, K. K., Cattaneo, C. M., Weeber, F., Chalabi, M., van de Haar, J., Fanchi, L. F., et al. (2018). Generation of tumor-reactive T cells by Co-culture of peripheral blood lymphocytes and tumor organoids. Cell 174, 1586–1598.e12. doi:10.1016/j.cell.2018.07.009
Dijkstra, K. K., Monkhorst, K., Schipper, L. J., Hartemink, K. J., Smit, E. F., Kaing, S., et al. (2020). Challenges in establishing pure lung cancer organoids limit their utility for personalized medicine. Cell Rep. 31, 107588. doi:10.1016/j.celrep.2020.107588
Driehuis, E., van Hoeck, A., Moore, K., Kolders, S., Francies, H. E., Gulersonmez, M. C., et al. (2019). Pancreatic cancer organoids recapitulate disease and allow personalized drug screening. Proc. Natl. Acad. Sci. U. S. A. 116, 26580–26590. doi:10.1073/pnas.1911273116
Drost, J., and Clevers, H. (2018). Organoids in cancer research. Nat. Rev. Cancer 18, 407–418. doi:10.1038/s41568-018-0007-6
Eiraku, M., Watanabe, K., Matsuo-Takasaki, M., Kawada, M., Yonemura, S., Matsumura, M., et al. (2008). Self-organized formation of polarized cortical tissues from ESCs and its active manipulation by extrinsic signals. Cell Stem Cell 3, 519–532. doi:10.1016/j.stem.2008.09.002
Entezari, M., Sadrkhanloo, M., Rashidi, M., Asnaf, S. E., Taheriazam, A., Hashemi, M., et al. (2022). Non-coding RNAs and macrophage interaction in tumor progression. Crit. Rev. Oncol. Hematol. 173, 103680. doi:10.1016/j.critrevonc.2022.103680
Gao, D., Vela, I., Sboner, A., Iaquinta, P. J., Karthaus, W. R., Gopalan, A., et al. (2014). Organoid cultures derived from patients with advanced prostate cancer. Cell 159, 176–187. doi:10.1016/j.cell.2014.08.016
Garreta, E., Kamm, R. D., Chuva de Sousa Lopes, S. M., Lancaster, M. A., Weiss, R., Trepat, X., et al. (2021). Rethinking organoid technology through bioengineering. Nat. Mater 20, 145–155. doi:10.1038/s41563-020-00804-4
Germann, M., Xu, H., Malaterre, J., Sampurno, S., Huyghe, M., Cheasley, D., et al. (2014). Tripartite interactions between Wnt signaling, Notch and Myb for stem/progenitor cell functions during intestinal tumorigenesis. Stem Cell Res. 13, 355–366. doi:10.1016/j.scr.2014.08.002
Gjorevski, N., Sachs, N., Manfrin, A., Giger, S., Bragina, M. E., Ordonez-Moran, P., et al. (2016). Designer matrices for intestinal stem cell and organoid culture. Nature 539, 560–564. doi:10.1038/nature20168
Grassi, L., Alfonsi, R., Francescangeli, F., Signore, M., De Angelis, M. L., Addario, A., et al. (2019). Organoids as a new model for improving regenerative medicine and cancer personalized therapy in renal diseases. Cell Death Dis. 10, 201. doi:10.1038/s41419-019-1453-0
Guillen, K. P., Fujita, M., Butterfield, A. J., Scherer, S. D., Bailey, M. H., Chu, Z., et al. (2022). A human breast cancer-derived xenograft and organoid platform for drug discovery and precision oncology. Nat. Cancer 3, 232–250. doi:10.1038/s43018-022-00337-6
Hidalgo, M., Amant, F., Biankin, A. V., Budinska, E., Byrne, A. T., Caldas, C., et al. (2014). Patient-derived xenograft models: An emerging platform for translational cancer research. Cancer Discov. 4, 998–1013. doi:10.1158/2159-8290.CD-14-0001
Hill, S. J., Decker, B., Roberts, E. A., Horowitz, N. S., Muto, M. G., Worley, M. J., et al. (2018). Prediction of DNA repair inhibitor response in short-term patient-derived ovarian cancer organoids. Cancer Discov. 8, 1404–1421. doi:10.1158/2159-8290.CD-18-0474
Hu, Y., Sui, X., Song, F., Li, Y., Li, K., Chen, Z., et al. (2021). Lung cancer organoids analyzed on microwell arrays predict drug responses of patients within a week. Nat. Commun. 12, 2581. doi:10.1038/s41467-021-22676-1
Hubert, C. G., Rivera, M., Spangler, L. C., Wu, Q., Mack, S. C., Prager, B. C., et al. (2016). A three-dimensional organoid culture system derived from human glioblastomas recapitulates the hypoxic gradients and cancer stem cell heterogeneity of tumors found in vivo. Cancer Res. 76, 2465–2477. doi:10.1158/0008-5472.CAN-15-2402
Humphrey, P. A., Moch, H., Cubilla, A. L., Ulbright, T. M., and Reuter, V. E. (2016). The 2016 WHO classification of tumours of the urinary system and male genital organs-Part B: Prostate and bladder tumours. Eur. Urol. 70, 106–119. doi:10.1016/j.eururo.2016.02.028
Hutchinson, L., and Kirk, R. (2011). High drug attrition rates-where are we going wrong? Nat. Rev. Clin. Oncol. 8, 189–190. doi:10.1038/nrclinonc.2011.34
Jabs, J., Zickgraf, F. M., Park, J., Wagner, S., Jiang, X., Jechow, K., et al. (2017). Screening drug effects in patient-derived cancer cells links organoid responses to genome alterations. Mol. Syst. Biol. 13, 955. doi:10.15252/msb.20177697
Jacob, F., Salinas, R. D., Zhang, D. Y., Nguyen, P. T. T., Schnoll, J. G., Wong, S. Z. H., et al. (2020). A patient-derived glioblastoma organoid model and biobank recapitulates inter- and intra-tumoral heterogeneity. Cell 180, 188–204.e22. doi:10.1016/j.cell.2019.11.036
Kaluthantrige Don, F., and Huch, M. (2021). Organoids, where we stand and where we go. Trends Mol. Med. 27, 416–418. doi:10.1016/j.molmed.2021.03.001
Karkampouna, S., La Manna, F., Benjak, A., Kiener, M., De Menna, M., Zoni, E., et al. (2021). Patient-derived xenografts and organoids model therapy response in prostate cancer. Nat. Commun. 12, 1117. doi:10.1038/s41467-021-21300-6
Kazama, A., Anraku, T., Kuroki, H., Shirono, Y., Murata, M., Bilim, V., et al. (2021). Development of patientderived tumor organoids and a drug testing model for renal cell carcinoma. Oncol. Rep. 46, 226. doi:10.3892/or.2021.8177
Kim, E., Choi, S., Kang, B., Kong, J., Kim, Y., Yoon, W. H., et al. (2020). Creation of bladder assembloids mimicking tissue regeneration and cancer. Nature 588, 664–669. doi:10.1038/s41586-020-3034-x
Kim, M., Mun, H., Sung, C. O., Cho, E. J., Jeon, H. J., Chun, S. M., et al. (2019). Patient-derived lung cancer organoids as in vitro cancer models for therapeutic screening. Nat. Commun. 10, 3991. doi:10.1038/s41467-019-11867-6
Kiyohara, Y., Yoshino, K., Kubota, S., Okuyama, H., Endo, H., Ueda, Y., et al. (2016). Drug screening and grouping by sensitivity with a panel of primary cultured cancer spheroids derived from endometrial cancer. Cancer Sci. 107, 452–460. doi:10.1111/cas.12898
Kondo, J., and Inoue, M. (2019). Application of cancer organoid model for drug screening and personalized therapy. Cells 8 (5), 470. doi:10.3390/cells8050470
Kopper, O., de Witte, C. J., Lohmussaar, K., Valle-Inclan, J. E., Hami, N., Kester, L., et al. (2019). An organoid platform for ovarian cancer captures intra- and interpatient heterogeneity. Nat. Med. 25, 838–849. doi:10.1038/s41591-019-0422-6
Kretzschmar, K. (2021). Cancer research using organoid technology. J. Mol. Med. Berl. 99, 501–515. doi:10.1007/s00109-020-01990-z
Lancaster, M. A., Renner, M., Martin, C. A., Wenzel, D., Bicknell, L. S., Hurles, M. E., et al. (2013). Cerebral organoids model human brain development and microcephaly. Nature 501, 373–379. doi:10.1038/nature12517
Lee, S. H., Hu, W., Matulay, J. T., Silva, M. V., Owczarek, T. B., Kim, K., et al. (2018). Tumor evolution and drug response in patient-derived organoid models of bladder cancer. Cell 173, 515–528.e17. doi:10.1016/j.cell.2018.03.017
LeSavage, B. L., Suhar, R. A., Broguiere, N., Lutolf, M. P., and Heilshorn, S. C. (2022). Next-generation cancer organoids. Nat. Mater 21, 143–159. doi:10.1038/s41563-021-01057-5
Li, L., Knutsdottir, H., Hui, K., Weiss, M. J., He, J., Philosophe, B., et al. (2019). Human primary liver cancer organoids reveal intratumor and interpatient drug response heterogeneity. JCI Insight 4, e121490. doi:10.1172/jci.insight.121490
Llovet, J. M., Kelley, R. K., Villanueva, A., Singal, A. G., Pikarsky, E., Roayaie, S., et al. (2021). Hepatocellular carcinoma. Nat. Rev. Dis. Prim. 7, 6. doi:10.1038/s41572-020-00240-3
Lo, Y. H., Kolahi, K. S., Du, Y., Chang, C. Y., Krokhotin, A., Nair, A., et al. (2021). A CRISPR/Cas9-Engineered ARID1A-deficient human gastric cancer organoid model reveals essential and nonessential modes of oncogenic transformation. Cancer Discov. 11, 1562–1581. doi:10.1158/2159-8290.CD-20-1109
Lu, S., Yan, D., Wu, Z., Jiang, T., Chen, J., Yuan, L., et al. (2014). Ubiquitin-like with PHD and ring finger domains 2 is a predictor of survival and a potential therapeutic target in colon cancer. Oncol. Rep. 31, 1802–1810. doi:10.3892/or.2014.3035
Luo, X., Fong, E. L. S., Zhu, C., Lin, Q. X. X., Xiong, M., Li, A., et al. (2021). Hydrogel-based colorectal cancer organoid co-culture models. Acta Biomater. 132, 461–472. doi:10.1016/j.actbio.2020.12.037
Maru, Y., Tanaka, N., Ebisawa, K., Odaka, A., Sugiyama, T., Itami, M., et al. (2019). Establishment and characterization of patient-derived organoids from a young patient with cervical clear cell carcinoma. Cancer Sci. 110, 2992–3005. doi:10.1111/cas.14119
Matano, M., Date, S., Shimokawa, M., Takano, A., Fujii, M., Ohta, Y., et al. (2015). Modeling colorectal cancer using CRISPR-Cas9-mediated engineering of human intestinal organoids. Nat. Med. 21, 256–262. doi:10.1038/nm.3802
Michalopoulos, G. K., Bowen, W. C., Mule, K., Lopez-Talavera, J. C., and Mars, W. (2002). Hepatocytes undergo phenotypic transformation to biliary epithelium in organoid cultures. Hepatology 36, 278–283. doi:10.1053/jhep.2002.34858
Michels, B. E., Mosa, M. H., Streibl, B. I., Zhan, T., Menche, C., Abou-El-Ardat, K., et al. (2020). Pooled in vitro and in vivo CRISPR-cas9 screening identifies tumor suppressors in human colon organoids. Cell Stem Cell 26, 782–792.e7. doi:10.1016/j.stem.2020.04.003
Muthusamy, T., Cordes, T., Handzlik, M. K., You, L., Lim, E. W., Gengatharan, J., et al. (2020). Serine restriction alters sphingolipid diversity to constrain tumour growth. Nature 586, 790–795. doi:10.1038/s41586-020-2609-x
Nasim, F., Sabath, B. F., and Eapen, G. A. (2019). Lung cancer. Med. Clin. North Am. 103, 463–473. doi:10.1016/j.mcna.2018.12.006
Neal, J. T., Li, X., Zhu, J., Giangarra, V., Grzeskowiak, C. L., Ju, J., et al. (2018). Organoid modeling of the tumor immune microenvironment. Cell 175, 1972–1988.e16. doi:10.1016/j.cell.2018.11.021
Papapetrou, E. P. (2016). Patient-derived induced pluripotent stem cells in cancer research and precision oncology. Nat. Med. 22, 1392–1401. doi:10.1038/nm.4238
Pauli, C., Hopkins, B. D., Prandi, D., Shaw, R., Fedrizzi, T., Sboner, A., et al. (2017). Personalized in vitro and in vivo cancer models to guide precision medicine. Cancer Discov. 7, 462–477. doi:10.1158/2159-8290.CD-16-1154
Pereira, D. A., and Williams, J. A. (2007). Origin and evolution of high throughput screening. Br. J. Pharmacol. 152, 53–61. doi:10.1038/sj.bjp.0707373
Pushpakom, S., Iorio, F., Eyers, P. A., Escott, K. J., Hopper, S., Wells, A., et al. (2019). Drug repurposing: Progress, challenges and recommendations. Nat. Rev. Drug Discov. 18, 41–58. doi:10.1038/nrd.2018.168
Ren, X., Chen, W., Yang, Q., Li, X., and Xu, L. (2022). Patient-derived cancer organoids for drug screening: Basic technology and clinical application. J. Gastroenterol. Hepatol. 37, 1446–1454. doi:10.1111/jgh.15930
Romero-Calvo, I., Weber, C. R., Ray, M., Brown, M., Kirby, K., Nandi, R. K., et al. (2019). Human organoids share structural and genetic features with primary pancreatic adenocarcinoma tumors. Mol. Cancer Res. 17, 70–83. doi:10.1158/1541-7786.MCR-18-0531
Rossi, G., Manfrin, A., and Lutolf, M. P. (2018). Progress and potential in organoid research. Nat. Rev. Genet. 19, 671–687. doi:10.1038/s41576-018-0051-9
Sachs, N., de Ligt, J., Kopper, O., Gogola, E., Bounova, G., Weeber, F., et al. (2018). A living biobank of breast cancer organoids captures disease heterogeneity. Cell 172, 373–386.e10. doi:10.1016/j.cell.2017.11.010
Sato, T., Vries, R. G., Snippert, H. J., van de Wetering, M., Barker, N., Stange, D. E., et al. (2009). Single Lgr5 stem cells build crypt-villus structures in vitro without a mesenchymal niche. Nature 459, 262–265. doi:10.1038/nature07935
Schneider, H., Muirhead, E. E., and Zydeck, F. A. (1963). Some unusual observations of organoid tissues and blood elements in monolayer cultures. Exp. Cell Res. 30, 449–459. doi:10.1016/0014-4827(63)90322-7
Seino, T., Kawasaki, S., Shimokawa, M., Tamagawa, H., Toshimitsu, K., Fujii, M., et al. (2018). Human pancreatic tumor organoids reveal loss of stem cell niche factor dependence during disease progression. Cell Stem Cell 22, 454–467.e6. doi:10.1016/j.stem.2017.12.009
Shi, R., Radulovich, N., Ng, C., Liu, N., Notsuda, H., Cabanero, M., et al. (2020). Organoid cultures as preclinical models of non-small cell lung cancer. Clin. Cancer Res. 26, 1162–1174. doi:10.1158/1078-0432.CCR-19-1376
Shiihara, M., and Furukawa, T. (2022). Application of patient-derived cancer organoids to personalized medicine. J. Pers. Med. 12, 789. doi:10.3390/jpm12050789
Siegel, R. L., Miller, K. D., Fuchs, H. E., and Jemal, A. (2021). Cancer statistics. CA Cancer J. Clin. 71, 7–33. doi:10.3322/caac.21654
Siegel, R. L., Miller, K. D., Wagle, N. S., and Jemal, A. (2023). Cancer statistics. CA Cancer J. Clin. 73, 17–48. doi:10.3322/caac.21763
Smith, E., and Cochrane, W. J. (1946). Cystic organoid teratoma: (Report of A case). Can. Med. Assoc. J. 55, 151–152.
Spence, J. R., Mayhew, C. N., Rankin, S. A., Kuhar, M. F., Vallance, J. E., Tolle, K., et al. (2011). Directed differentiation of human pluripotent stem cells into intestinal tissue in vitro. Nature 470, 105–109. doi:10.1038/nature09691
Sun, C. P., Lan, H. R., Fang, X. L., Yang, X. Y., and Jin, K. T. (2022). Organoid models for precision cancer immunotherapy. Front. Immunol. 13, 770465. doi:10.3389/fimmu.2022.770465
Sung, H., Ferlay, J., Siegel, R. L., Laversanne, M., Soerjomataram, I., Jemal, A., et al. (2021). Global cancer statistics 2020: GLOBOCAN estimates of incidence and mortality worldwide for 36 cancers in 185 countries. CA Cancer J. Clin. 71, 209–249. doi:10.3322/caac.21660
Takeda, H., Kataoka, S., Nakayama, M., Ali, M. A. E., Oshima, H., Yamamoto, D., et al. (2019). CRISPR-Cas9-mediated gene knockout in intestinal tumor organoids provides functional validation for colorectal cancer driver genes. Proc. Natl. Acad. Sci. U. S. A. 116, 15635–15644. doi:10.1073/pnas.1904714116
Tuveson, D., and Clevers, H. (2019). Cancer modeling meets human organoid technology. Science 364, 952–955. doi:10.1126/science.aaw6985
Tyers, M., and Wright, G. D. (2019). Drug combinations: A strategy to extend the life of antibiotics in the 21st century. Nat. Rev. Microbiol. 17, 141–155. doi:10.1038/s41579-018-0141-x
Unbekandt, M., and Davies, J. A. (2010). Dissociation of embryonic kidneys followed by reaggregation allows the formation of renal tissues. Kidney Int. 77, 407–416. doi:10.1038/ki.2009.482
van de Wetering, M., Francies, H. E., Francis, J. M., Bounova, G., Iorio, F., Pronk, A., et al. (2015). Prospective derivation of a living organoid biobank of colorectal cancer patients. Cell 161, 933–945. doi:10.1016/j.cell.2015.03.053
Veninga, V., and Voest, E. E. (2021). Tumor organoids: Opportunities and challenges to guide precision medicine. Cancer Cell 39, 1190–1201. doi:10.1016/j.ccell.2021.07.020
Verissimo, C. S., Overmeer, R. M., Ponsioen, B., Drost, J., Mertens, S., Verlaan-Klink, I., et al. (2016). Targeting mutant RAS in patient-derived colorectal cancer organoids by combinatorial drug screening. Elife 5, e18489. doi:10.7554/eLife.18489
Vlachogiannis, G., Hedayat, S., Vatsiou, A., Jamin, Y., Fernandez-Mateos, J., Khan, K., et al. (2018). Patient-derived organoids model treatment response of metastatic gastrointestinal cancers. Science 359, 920–926. doi:10.1126/science.aao2774
Ward, R. A., Fawell, S., Floc'h, N., Flemington, V., McKerrecher, D., and Smith, P. D. (2021). Challenges and opportunities in cancer drug resistance. Chem. Rev. 121, 3297–3351. doi:10.1021/acs.chemrev.0c00383
Whyard, T., Liu, J., Darras, F. S., Waltzer, W. C., and Romanov, V. (2020). Organoid model of urothelial cancer: Establishment and applications for bladder cancer research. Biotechniques 69, 193–199. doi:10.2144/btn-2020-0068
Wilson, H. V. (1907). On some phenomena of coalescence and regeneration in sponges. J. Elisha Mitchell Sci. Soc. 23, 245–258. doi:10.1002/jez.1400050204
Wong, C. H., Siah, K. W., and Lo, A. W. (2019). Estimation of clinical trial success rates and related parameters. Biostatistics 20, 273–286. doi:10.1093/biostatistics/kxx069
Workman, M. J., Mahe, M. M., Trisno, S., Poling, H. M., Watson, C. L., Sundaram, N., et al. (2017). Engineered human pluripotent-stem-cell-derived intestinal tissues with a functional enteric nervous system. Nat. Med. 23, 49–59. doi:10.1038/nm.4233
Xia, X., Li, F., He, J., Aji, R., and Gao, D. (2019). Organoid technology in cancer precision medicine. Cancer Lett. 457, 20–27. doi:10.1016/j.canlet.2019.04.039
Xu, H., Jiao, D., Liu, A., and Wu, K. (2022). Multi-omics in spinal cord injury: Diagnosis, prognosis, and treatment. J. Hematol. Oncol. 15, 58–70. doi:10.14715/cmb/2022.68.11.11
Yan, H. H. N., Siu, H. C., Law, S., Ho, S. L., Yue, S. S. K., Tsui, W. Y., et al. (2018). A comprehensive human gastric cancer organoid biobank captures tumor subtype heterogeneity and enables therapeutic screening. Cell Stem Cell 23, 882–897.e11. doi:10.1016/j.stem.2018.09.016
Yang, J., Huang, S., Cheng, S., Jin, Y., Zhang, N., and Wang, Y. (2021). Application of ovarian cancer organoids in precision medicine: Key challenges and current opportunities. Front. Cell Dev. Biol. 9, 701429. doi:10.3389/fcell.2021.701429
Yoshida, G. J. (2020). Applications of patient-derived tumor xenograft models and tumor organoids. J. Hematol. Oncol. 13, 4. doi:10.1186/s13045-019-0829-z
Yoshida, T., Sopko, N. A., Kates, M., Liu, X., Joice, G., McConkey, D. J., et al. (2018). Three-dimensional organoid culture reveals involvement of Wnt/β-catenin pathway in proliferation of bladder cancer cells. Oncotarget 9, 11060–11070. doi:10.18632/oncotarget.24308
Yuan, J., Li, X., and Yu, S. (2022). Cancer organoid co-culture model system: Novel approach to guide precision medicine. Front. Immunol. 13, 1061388. doi:10.3389/fimmu.2022.1061388
Zhao, H., Jiang, E., and Shang, Z. (2021). 3D Co-culture of cancer-associated fibroblast with oral cancer organoids. J. Dent. Res. 100, 201–208. doi:10.1177/0022034520956614
Zhou, J., Su, J., Fu, X., Zheng, L., and Yin, Z. (2017). Microfluidic device for primary tumor spheroid isolation. Exp. Hematol. Oncol. 6, 22. doi:10.1186/s40164-017-0084-3
Keywords: organoids, organoids development, establishing, application, cancer therapy
Citation: Jiang X, Oyang L, Peng Q, Liu Q, Xu X, Wu N, Tan S, Yang W, Han Y, Lin J, Xia L, Peng M, Tang Y, Luo X, Su M, Shi Y, Zhou Y and Liao Q (2023) Organoids: opportunities and challenges of cancer therapy. Front. Cell Dev. Biol. 11:1232528. doi: 10.3389/fcell.2023.1232528
Received: 31 May 2023; Accepted: 18 July 2023;
Published: 27 July 2023.
Edited by:
Dingxiao Zhang, Hunan University, ChinaReviewed by:
Xin Chen, Huazhong University of Science and Technology, ChinaYu Shu, Shanghai Jiao Tong University, China
Copyright © 2023 Jiang, Oyang, Peng, Liu, Xu, Wu, Tan, Yang, Han, Lin, Xia, Peng, Tang, Luo, Su, Shi, Zhou and Liao. This is an open-access article distributed under the terms of the Creative Commons Attribution License (CC BY). The use, distribution or reproduction in other forums is permitted, provided the original author(s) and the copyright owner(s) are credited and that the original publication in this journal is cited, in accordance with accepted academic practice. No use, distribution or reproduction is permitted which does not comply with these terms.
*Correspondence: Qianjin Liao, bWFyY2gtb25AMTI2LmNvbQ==; Yujuan Zhou, eXVqYW55X3pob3VAMTYzLmNvbQ==; Yingrui Shi, c2hpeWluZ3J1aUBhbGl5dW4uY29t
†These authors have contributed equally to this work