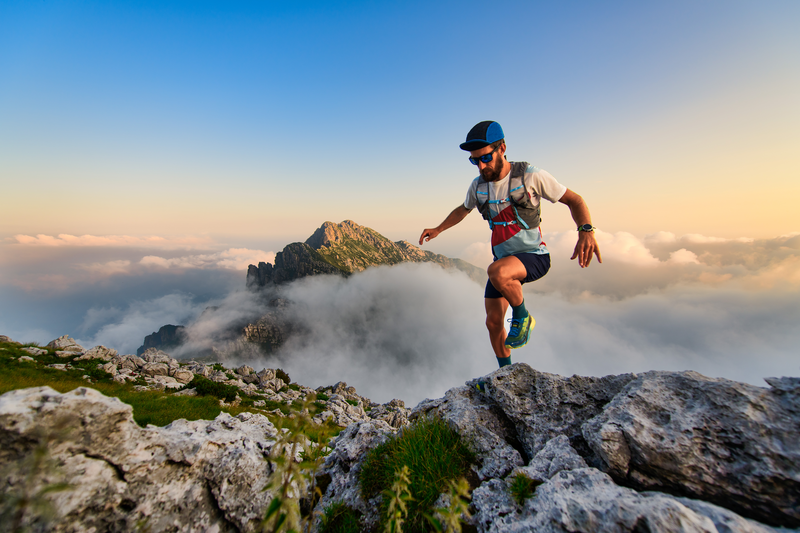
95% of researchers rate our articles as excellent or good
Learn more about the work of our research integrity team to safeguard the quality of each article we publish.
Find out more
REVIEW article
Front. Cell Dev. Biol. , 10 July 2023
Sec. Stem Cell Research
Volume 11 - 2023 | https://doi.org/10.3389/fcell.2023.1221175
This article is part of the Research Topic New Insights in Cellular and Molecular Biology of Cancer Stem Cells View all 6 articles
Breast cancer stem cells (BCSCs) represent a distinct subpopulation of cells with the ability to self-renewal and differentiate into phenotypically diverse tumor cells. The involvement of CSC in treatment resistance and cancer recurrence has been well established. Numerous studies have provided compelling evidence that the self-renewal ability of cancer stem cells is tightly regulated by specific signaling pathways, which exert critical roles to maintain an undifferentiated phenotype and prevent the differentiation of CSCs. Signaling pathways such as Wnt/β-catenin, NF-κB, Notch, Hedgehog, TGF-β, and Hippo have been implicated in the promotion of self-renewal of many normal and cancer stem cells. Given the pivotal role of BCSCs in driving breast cancer aggressiveness, targeting self-renewal signaling pathways holds promise as a viable therapeutic strategy for combating this disease. In this review, we will discuss the main signaling pathways involved in the maintenance of the self-renewal ability of BCSC, while also highlighting current strategies employed to disrupt the signaling molecules associated with stemness.
Inside tumor mass, a distinct subset of malignant cells possesses the remarkable ability to establish the intra-tumor heterogeneity and sustain tumor growth and metastasis. Such cells, defined as cancer stem cells (CSCs), exhibit distinctive characteristics, including extensive self-renewal and the ability to differentiate generating phenotypically diverse progeny. Breast cancer stem cells (BCSCs) drive tumor progression, metastatic dissemination, drug resistance, and cancer relapse (Lim et al., 2021).
CSCs exhibit a diverse array of functional and biological properties that distinguish them from non-cancer stem cells in tumors. CSCs are mainly characterized by the presence or absence of diverse stem cell markers whose levels are heterogeneously distributed among tumors. These markers range from cell surface protein, cytoplasmic enzymes, and nuclear transcription factors (Lim et al., 2021).
BCSCs are characterized by the high expression of CD44, ESA, and low or absent levels of CD24. In addition to CD44+/ESA+/CD24-cells, other studies have identified and isolated BCSCs using CD133, CD49f, PROCR, LGR5, ABCG2, among others. BCSCs also exhibit high levels of transcription factors such as SOX2, NANOG, and OCT4, which are crucial for maintaining stemness and preventing differentiation. Moreover, BCSCs present high activity of the aldehyde dehydrogenase 1 cytosolic enzyme (ALDH1) and high expression of ABC family transporters, which provide resistance to conventional cancer treatments (Wang et al., 2022) (Figure 1).
FIGURE 1. (A) Breast cancer stem cells represent a distinct subset of tumor cells characterized by their remarkable capacity for self-renewal and their ability to differentiate into non-BCSCs. (B) BCSCs can be distinguished by the high expression of BCSCs markers, low expression of differentiation markers, specific signaling pathways activity, resistance to conventional therapy and the high tumorigenesis potential in vivo.
Similarly, another crucial attribute that sustains breast cancer stem cells is their metabolic flexibility and adaptation. Most BCSCs display a heightened glycolytic rate, exhibit increased glucose uptake, and lactate production, and a decreased mitochondrial respiration. Recent evidence has indicated that BCSCs possess the ability to alternate between glycolysis and mitochondrial oxidative phosphorylation (OXPHOS) in the presence of oxygen, enabling them to facilitate tumor growth. This metabolic flexibility enables CSCs to engage in mitochondrial respiration and generate ATP, thereby conferring a survival advantage under conditions where glycolysis is compromised. Interestingly, proliferative BCSCs prefer the OXPHOS metabolism, while quiescent BCSCs opt for a glycolytic metabolism. In addition, CSCs also rely on mitochondrial fatty acid oxidation as an alternative energy source to maintain their survival, self-renewal, and chemoresistance properties. CSCs exhibit dysregulated fatty acid synthesis, which leads to increased lipid production and accumulation, thereby supporting the biosynthesis of macromolecules vital for cellular growth and division. Moreover, CSCs demonstrate distinctive alterations in glutamine metabolism, utilizing it as a carbon source to fuel energy production and sustain the biosynthesis of essential macromolecules. These distinctive metabolic features of CSCs enable them to adapt to the challenging conditions within the tumor microenvironment, promote self-renewal, and drive tumor progression (Gao and Dong, 2020).
Functionally, CSCs can grow tumors retaining the self-renewal ability in several serial passages even when they are transplanted in very low numbers. In addition, CSCs have the ability to grow in low adherence conditions and exhibit improved processes of invasion and metastasis (Zhang et al., 2022). The characterization of CSCs has been a key step in cancer research, huge efforts are undertaken to decipher the complex biology of CSCs. Tremendous efforts are being undertaken to further elucidate the unique features of CSCs. Novel therapeutic strategies are being proposed to eliminate the CSC fraction, overcome drug resistance, and prevent cancer relapse. Currently, one of the promising approaches to prevent CSC maintenance is based on blocking the various signaling pathways that maintain stemness in breast cancer.
This review aims to provide an overview of the main signaling pathways involved in the maintenance of BCSCs. We also summarized the current therapeutic advances to target BCSCs.
The properties of CSCs are modulated by intricate signaling pathways, which modulate the stemness, self-renewal, differentiation, proliferation, and survival of CSCs. The main signaling pathways involved in CSC maintenance include Notch, Wnt, Hedgehog, NF-KB, Hippo among others. These pathways play important roles in the invasion, metastasis, autophagy, and EMT. The signaling molecules and components of these pathways have been extensively studied as therapeutic target options to destroy CSCs (Figure 2).
FIGURE 2. BCSCs maintenance can be regulated by the activity of signaling pathways. In breast cancer, pivotal signaling pathways including Wnt, NF-κB, Notch Hedgehog, Hippo and TGF-β govern the self-renewal process of BCSCs.
The WNT signaling pathway is a highly complex and evolutive conserved pathway involved in the regulation of cell fate determination, cell polarity, and self-renewal of normal and CSCs. The Wnt family comprises 19 secreted cys-rich glycoproteins, which can activate more than 15 cell membrane receptors. This pathway is triggered through Wnt ligands, Frizzled receptors, and/or low-density lipoprotein-related protein (LRP) 5 and LRP6 coreceptors. WNT signaling typically employs two major pathways: canonical (mediated through β-catenin) and non-canonical pathways (independent of β-catenin) (Xu et al., 2020).
In the context of canonical Wnt signaling, non-active conditions enable the continuous phosphorylation of β-catenin mediated by the inhibitory complex of β-catenin, which includes AXIN, GSK3β, APC, and CK1. This phosphorylation triggers the proteasomal degradation of β-catenin through the E3 ubiquitin ligase β-TrCP. In contrast, under active conditions, Wnt ligands (Wnt1, Wnt2, Wnt3, Wnt3a, Wnt8b, Wnt10a, Wnt10b, among others) bind to their receptors and induce the phosphorylation of the co-receptor LRP5/6. This phosphorylation event recruits both the Dishelved protein (Dvl) and Axin, thus preventing the inhibition of β-catenin phosphorylation. Subsequently, the accumulation of β-catenin levels results in its nuclear translocation, where it binds to TCF/LEF transcription factors to activate the transcription of numerous Wnt target genes (Xu et al., 2020).
It has been reported that molecules such as LGR4/5/6 receptors enhance the Wnt/β-catenin signal by interacting with R-spondin ligands (RSPOs). In the absence of RSPOs, the E3 ubiquitin ligases ZNRF3 and RNF43 continuously ubiquitinate the Wnt receptor complex, thus inducing their degradation. The activation of LGR4/5/6 by RSPOs ligands neutralizes the ZNRF3 and RNF43 ligases allowing for the stabilization of surface Frizzled receptors and thus boosting the WNT signaling (Ter Steege and Bakker, 2021).
Non-canonical Wnt signaling is independent of β-catenin. This pathway is activated when Wnt ligands bind to Frizzled and other co-receptors such as ROR1, ROR2, or RYK. The non-canonical signaling induces the activation of PCP, RTK, or Ca++ signaling cascades. This pathway induces several downstream effectors, including multiple small Rho GTPases, Jnk, Src, NLK and heterotrimeric G proteins. Activation of this pathway results in transcriptional regulation and cytoskeletal rearrangement (Xu et al., 2020).
In breast cancer, both canonical and non-canonical WNT signaling participates in the regulation of CSCs. Breast tumors exhibit constitutive activation of the Wnt/β-catenin pathway, accompanied by increased nuclear stabilization of β-catenin. Even though Wnt signaling molecules harbor some mutations, several studies have identified that most alterations frequently occur in activators or inhibitors of the Wnt signaling pathway. Activators of the Wnt/β-catenin pathway such as Wnt and Dvl ligands are commonly amplified or overexpressed, while Wnt inhibitors such as FRP1, DKK1, and APC are commonly inactivated. (Lindvall et al., 2007; Feng et al., 2018). Sustained Wnt-β-catenin activation endows CSCs with self-renewal abilities, proliferation, invasion, and metastatic abilities (Katoh, 2017).
Several studies have highlighted the importance of the Wnt/β-catenin signal in the maintenance of different BCSCs subpopulations. The canonical pathway has been associated with self-renewal, mammosphere formation, migration, invasion, and drug resistance. Li et al., showed that BCSCs exhibiting elevated expression of the transcription factor Twist, are regulated through the Wnt/β-catenin signal (Li and Zhou, 2011). In addition, activation of Wnt/β-catenin triggered by histone deacetylase inhibitors, provokes an increment in the population of ALDH-positive BCSCs, resulting in an enhanced ability to grow spheres and to seed tumors (Debeb et al., 2012). Some other studies have found that different subsets of BCSCs depend on the Wnt/β-catenin signaling to maintain their properties and self-renewal potential (Zhao Z. et al., 2014; Siddharth et al., 2017; Zhu L. et al., 2019).
LGR4/5/6 receptors and their RSPO ligands induce stemness in breast cancer. These receptors have been involved in the maintenance of the stem cell phenotype through the potentiation of Wnt/β-catenin signaling. It has been shown that the LGR4 receptor promotes tumorigenesis, induces the epithelial-mesenchymal transition process, and favors a stem cell phenotype through the Wnt/β-catenin pathway (Yue et al., 2018). LGR5 also potentiates the Wnt/β-catenin signal resulting in the acquisition of a stem cell phenotype. Notably, LGR5 has been associated with a worse prognosis in patients with breast cancer (Yang et al., 2015; Hou et al., 2018; Chen and Xue, 2019). Similarly, LGR6 promotes the proliferation and metastasis of breast cancer cells through the Wnt/β-catenin signal. LGR6 is commonly overexpressed in triple-negative breast cancer tumors and has been associated with a worse prognosis in patients with this disease (Kong et al., 2020).
Besides the classic molecules participating in the Wnt/β-catenin signaling, various non-classical activators have been associated with the maintenance of stemness in breast cancer. Pigo2 protein (Pygopus Family PHD Finger 2) acts as a scaffold for the recruitment of β-catenin, allowing it to associate with histone methyl and acetyltransferases, favoring the transcription of genes associated with stemness, and thus increasing the BCSC fraction (Chen et al., 2010). Another study indicates that the cell cycle regulator p21 is capable of regulating the stemness of breast cancer cells through the Wnt/β-catenin signaling. p21 induces the expression of Cyclin D1 and TCF1, a transcription factor involved in the Wnt pathway, leading to the activation of the Wnt/β-catenin pathway and the induction of BCSCs self-renewal (Benard et al., 2019). Tang et al., showed that the enzyme β1, 4-Galactosyltransferase B (B4galT5) regulates the stemness phenotype of breast cancer. B4galT5 protects the Frizzled-1 receptor from degradation via the lysosome, allowing its membrane stabilization and favoring the Wnt/β-catenin pathway activation (Tang et al., 2020). The BOP1 molecule regulates drug resistance and stemness phenotype in triple-negative breast cancer cells. BOP1 allows the recruitment of the CBD protein, facilitating β-catenin acetylation, thus inducing its activation, and increasing the expression of stem cell markers such as CD133 and ALDH1A1 (Li et al., 2021). Similarly, salt-inducible kinase 2 (SIK2) is capable of regulating the stem cell phenotype of breast cancer by favoring the phosphorylation of the CK1 protein kinase and the co-receptor of the Wnt/LRP6 signaling, facilitating the Wnt/β-catenin activation (Rong et al., 2022).
Similarly, various non-coding RNAs have been associated with the maintenance of stemness in breast cancer through the Wnt/β-catenin signal. It has been well established that Wnt/β-catenin induces the expression of the lncRNA Lin28 to block let7 miRNA activity, thus promoting a stem cell phenotype in breast cancer cells (Cai et al., 2013). In addition, the microRNA mir-204 can activate the Wnt/β-catenin signal through the Sam68 protein, thus regulating stem cell self-renewal and tumorigenesis in SKBR3 and MCF7 breast cancer cell lines. (Wang et al., 2015). Eterno et al., showed that the protein AurkA can regulate the Wnt3a ligand levels by inhibiting mir-128 and thus regulating the stem cell phenotype in breast cancer (Eterno et al., 2016). It was discovered that the lncRNA LncCCAT1 promotes stemness in breast cancer by interacting with the miR-204/211, miR-148a/152, and Annexin A2, inducing the overexpression of TCF4 and favoring the activation of the Wnt/β-catenin signal (Tang et al., 2019). LUCAT1 is associated with advanced breast cancer tumors and is expressed mainly in the stem fraction, where it regulates the self-renewal of BCSCs by acting as a mir-5582-3p sponge, favoring the expression of TCF7L2 and promoting the Wnt/β-catenin signal. (Zheng et al., 2019). In addition, the mir-5188 inhibits the expression of FOXO1 which facilitates the ubiquitination of β-catenin. Blockage of FOXO1 by mir-5188 results in the accumulation of β-catenin and induces the activation of Wnt signaling thus promoting the self-renewal and maintenance of CSCs (Zou et al., 2019). The lncRNA THOR is also able to regulate stemness by interacting and stabilizing the β-catenin mRNA and increasing its expression, favoring the activation of the Wnt signaling (Wang et al., 2020). Taken together, these findings indicate that lncRNAs, miRNAs, and other molecules can influence canonical WNT/β-catenin signaling and this is involved in the maintenance and expansion of BCSCs.
Although less studied than the WNT/β-catenin pathway, non-canonical signaling also plays a role in the regulation of the BCSC fraction. In CSCs, the non-canonical pathway is commonly activated by Wnt5a, and other non-canonical ligands secreted from cancer or stromal cells. Wnt5a enhances the sphere formation efficiency by activating the non-canonical Wnt pathway in MMTV-Wnt1 mouse primary cells. The effects of Wnt5a on stemness depend on the receptor tyrosine kinase (ROR2) that binds Wnt5a and transduces the Wnt signal, thus inducing the activation of the Jun N-terminal kinase (JNK) (Many and Brown, 2014). Interestingly, breast cancer patients expressing ROR2 had shorter overall survival than those harboring tumors without ROR2 expression (Henry et al., 2015). Although Wnt5a can activate the Wnt/β-catenin in special circumstances (Mikels and Nusse, 2006; van Amerongen et al., 2008), some studies have shown that the effects of Wnt5a on stemness depend on the non-canonical Wnt and not on the canonical signaling (Many and Brown, 2014). Notably, Wnt5a is overexpressed specifically in basal breast cancer cell lines (MDA-MB-231 and BCap-37), which harbor a mesenchymal phenotype and a high proportion of CSCs. Remarkably, the inhibition of Wnt5a mediated by the disruption of Twist-BRD4 association suppresses CSC properties, reduces the invasion, and impairs tumorigenesis of basal breast cancer cells (Li and Zhou, 2011). Wnt5B has also been involved in the regulation of stemness via the interaction of the Fzd7 receptor and the subsequent activation of the non-canonical Wnt pathway. Fzd7 knockdown reduces the fraction of LGR5+ CSCs, suppresses tumorigenesis, and impairs metastasis. Mechanistically, Fzd7/Wnt5b regulates the expression of key intracellular molecules such as phosphorylated Stat3, Smad3, and Yap1 to induce EMT and stemness. Interestingly, Col6a1 is implicated in the Fzd7-Wnt5b signal and mediates the stemness effect of Fzd7/Wnt5b (Yin et al., 2020). Wnt5a and Wnt5b can also interact with Fzd2, a receptor that signals to the non-canonical via. Interestingly, Fzd2 and its ligands are overexpressed in high-grade tumors, and metastatic cancer cell lines, and high Fzf2 expression is associated with shortened overall survival, relapse-free survival, and distant metastasis-free survival (Gujral et al., 2014; Yin et al., 2020). Fzd2 also correlates with the expression of EMT markers and promotes EMT, drug resistance, and induces stemness via non-canonical Wnt signaling. Interestingly, Fzd2 knockdown impairs stemness, reduces the fraction of Lgr5+ CSC subpopulation, inhibits migration and invasion, impairs tumor growth, and enhances drug sensitivity (Gujral et al., 2014; Yin et al., 2020). Interestingly, the effect of Fzd2 in the induction of EMT and cell migration is mediated by the association with Stat3. Mechanistically, Fzd2 is phosphorylated on Tyr552 resulting in the binding of Fzd2 to the SH2 domain of Fyn kinase, which activates Star3 via the phosphorylation of Tyr705 (Gujral et al., 2014). Interestingly, Fzd2/Wnt5a/b regulate stemness by activating several oncogenic pathways including IL6/STAT3, Yap1 and TGF-b1/Smad3 (Yin et al., 2020).
NF-κB is a family of transcription factors that regulates the expression of genes associated with immune response, inflammation, survival, cell differentiation, and stemness. The family consists of five members: RelA (p65), RelB, c-Rel, NFκB1 (p105/p50), and NFκB2 (p100/p52). These proteins harbor a conserved N-terminal Rel homology domain (RHD) which facilitates homo or heterodimerization, nuclear localization, and DNA binding. In addition, only RelA, Relb, and c-Rel contain a C-terminal transactivation domain (TAD) which mediates coactivators interactions to promote gene transcription. Remarkably, p50 and p52 lack TAD domain, thus p50/p50, p50/p52 or p52/p52 dimers fail to activate gene expression. The precursor of p50 (p100) and p52 (p105) proteins contains ankyrin repeats which are proteolytically cleaved to generate the active p50 and p52 proteins (Vazquez-Santillan et al., 2015). Members of the NF-κB family possess the ability to generate distinct homo or heterodimers. The formation of NF-κB dimers varies depending on the specific cellular context, and the abundance of these molecules. For instance, the p50/RealA and p52/RelB dimers are known to be the major players in the canonical and non-canonical pathways, respectively. Similarly, the activation of the cRel/p50 heterodimer is a crucial element of the innate immune response and the subsequent eradication of pathogens (Courtine et al., 2012).
The NF-κB members are retained in the cytoplasm by a family of NF-κB inhibitor (IκB) proteins (IκBα, IκBβ, and IκBε) and other proteins harboring ankyrin repeats. Upon receiving an activation stimulus, NF-κB proteins form homo or heterodimers, which translocate to the nucleus and regulate the expression of their target genes (Vazquez-Santillan et al., 2015). NFκB is activated by two major signaling branches: the canonical and the non-canonical pathway.
The canonical signaling is rapidly inducible and independent of protein synthesis, this pathway is associated with immunological and inflammatory roles. This signal is activated by diverse external stimuli promoting inflammation, such as proinflammatory cytokines including tumor necrosis factor α (TNF-α), pathogen-associated molecular patterns (PAMPs), and damage-associated molecular patterns (DAMPs). The interaction of ligands with their receptors favors the recruitment of proteins leading to the activation of the inhibitory kappa B kinases (IKK) complex, consisting of the scaffold protein NF-κB essential modulator (NEMO) and two catalytic subunits, IKKα and IKKβ. Upon activation, the IKK complex catalyzes the phosphorylation of the NF-κB inhibitor (IκB) proteins, triggering IκB polyubiquitination and subsequent degradation in the proteasome. Under non-active conditions, IκB proteins bind to NF-κB dimers, sequestering NF-κB and preventing their nuclear translocation, thus IκB phosphorylation mediated by IKKs is essential to NF-κB nuclear translocation and to modulate the expression of target genes (Vazquez-Santillan et al., 2015).
In contrast to canonical signaling, the non-canonical pathway is slow, persistent, and depends on the novo protein synthesis. Non-canonical signaling is associated with the differentiation, development, and survival of immune cells. This pathway is activated by a number of ligands including CD40 ligand (CD40L), B cell activating factor (BAFF), receptor activator of nuclear factor kappa B ligand (RANKL), and lymphotoxin β (LTβ). These ligands interact and bind to a subset of receptors favoring the stabilization of the NF-B-inducing kinase (NIK), which in turn phosphorylates the IKK complex formed exclusively by IKKa homodimers. The IKK complex phosphorylates the p100 NF-κB protein provoking its partial degradation via the proteasome, which converts p100 to the active p52 form. P52 forms dimers with RelB or p65, which can translocate to the nucleic fraction and favors the expression of its target genes (Vazquez-Santillan et al., 2015).
In BCSCs, constitutive activation of the NF-κB pathway has been observed in MCF7 and MDA-MB-231 breast cancer cell lines. Both canonical and no canonical pathway participates in the chemoresistance, tumorigenesis, and self-renewal of BCSCs (Pratt et al., 2009; Liu et al., 2010; Kendellen et al., 2014; Jia et al., 2015; Kumar et al., 2021). Interestingly, accumulating evidence has shown that NF-κB activity is able to expand the BCSCs (Yamamoto et al., 2013; Kendellen et al., 2014) and regulate the expression of stem cell markers (Smith and Cai, 2012). It has been shown that canonical and non-canonical NF-κB signaling is required by CSCs to self-renew and to form tumors in murine in vivo models. Interestingly, NF-κB regulates stemness by promoting the epithelial to mesenchymal transition and the expression of inflammatory cytokines Interleukin 1B and interleukin 6 (Kendellen et al., 2014).
Canonical NF-κB signaling drives resistance to chemotherapy in quiescent BCSCs and its pharmacological blocking sensitizes breast tumors to chemotherapy (Kumar et al., 2021). The inhibition of IKKβ or NF-κB subunits disrupts mammosphere formation and impaired stemness of SUM149 (Kendellen et al., 2014). Interestingly, the super repressor mutant of the IKB protein inhibits the stem cell properties, reduces proliferation, and suppresses the clonogenicity and tumorigenic ability of breast tumor cells by constitutively inhibiting the canonical NF-κB pathway (Liu et al., 2010; Hinohara et al., 2012). IKKα, a kinase involved in both canonical and non-canonical signaling, also contributes to the maintenance of the BCSCs fraction. The employment of an inactive mutation of IKKα impairs self-renewal and delays tumor formation in MMTV-c-neu mice (Cao et al., 2007).
It has been well demonstrated that non-canonical NF-κB also exerts essential roles in the maintenance and self-renewal of BCSCs. The non-canonical NIK protein is preferentially expressed in BCSCs, increases the expression of stem cell markers, regulates the self-renewal and expansion, and promotes tumorigenesis of CSCs through IKKα (Zhang et al., 2013; Vazquez-Santillan et al., 2016). In the same way, the IKKε kinase is able to promote the stem cell phenotype of breast cancer cell lines including MCF7 (Orlova et al., 2019).
Furthermore, NF-κB ligands such as TNF-α and RANKL increase the proportion of BCSCs by promoting the activity of the NF-κB pathway. TNF-α induce the expression of TAZ through the non-canonical NF-κB pathway and increases the proportion of BCSCs. Mechanistically p52 binds to the promoter region of TAZ to favor its transcription. TNF-α/TAZ plays a crucial role in the maintenance of BCSCs (Liu W. et al., 2020). The receptor activator of nuclear factor kappa B ligand (RANKL) and its receptor (RANK) participate in the activation of the non-canonical NF-κB pathway. RANK expression levels have been associated with poor prognosis in breast cancer patients. Accumulating evidence has shown that the RANK receptor increases tumorigenesis, migration, epithelial-mesenchymal transition, resistance to therapy, and stemness in breast cancer (Palafox et al., 2012; Pfitzner et al., 2014; Renema et al., 2016; Cuyàs et al., 2017). Interestingly, the inhibition of RANKL by the RANK-Fc recombinant protein results in the reduction of breast cancer tumorigenesis and the induction of the differentiation of CSCs, suggesting that the RANKL/RANK signaling expand the CSC fraction by activating the NF-κB signaling (Yoldi et al., 2016).
NF-κB signaling also renders BCSCs with invasive and metastatic abilities. A study found that Lin28, a downstream effector of IKKβ, enhances the metastatic abilities of BCSCs. IKKβ inhibition reduced the expression of stem cell factors (LIN28, OCT4, SOX2, and NANOG) and eliminate the ability of CSCs to metastasize (Chen et al., 2015). EMT induction mediated by NF-κB signaling also contributes to the invasive, tumorigenic, and metastatic abilities of BCSCs. Inhibition of NF-κB reverts the EMT and decreases invasion, reduces metastasis, and restores cell sensitivity to chemotherapy (Asiedu et al., 2014; Kendellen et al., 2014).
A plethora of molecules activating the NF-κB signaling has been shown to regulate the BCSC fraction. Recent evidence found that the receptor GPR50 is highly expressed in BCSCs and regulates the activity of the NF-kB pathway, enabling CSCs to form spheres, proliferate and migrate. The heat shock protein Hsp27 participates in the regulation of the epithelial-mesenchymal transition process and promotes stemness through the NF-κB signal (Wei et al., 2011). Neuropilin 1 (NRP1) is expressed in BCSCs and induces stemness by stimulating the NF-κB signaling (Glinka et al., 2012). Another study showed that the transcription factor FOXA1 inhibits stemness by blocking the expression of Interleukin 6 through the inhibition of NF-κB recruitment to the IL6 promoter gen (Yamaguchi et al., 2017). Stromal cell-derived factor 1 (SFD-1) promotes the stem cell phenotype, cell proliferation, migration, and invasion through the NF-κB signal (Kong et al., 2016). Another study found that the let7 miRNA inhibits the ability of CSCs to form mammospheres and impairs tumorigenicity by disrupting the NF-κB and MAPK signaling, suggesting that let7 regulates the stem properties in breast cancer (Xu et al., 2015). The microRNAs 221/222 promote the stem cell phenotype through the inhibition of PTEN and the activation of the AKT/NF-κB/COX-2 pathway (Li et al., 2017).
The NF-κB pathway was initially characterized as an inductor of inflammation and a regulator of the immune system in normal processes and cancer. However, it has been recently shown as an essential signal for developmental processes and as an important promoter of stemness in breast cancer, making it an attractive target to deplete BCSCs and improve the prognosis of breast cancer patients.
Notch is an evolutionarily conserved signaling pathway exerting pivotal roles in proliferation, cell fate determination, differentiation, and stem cell maintenance. In mammals, this pathway consists of 5 notch ligands (Jagged1, Jagged2, Delta-like (DLL) 1, 3, and 4) and 4 notch receptors (Notch 1-4). The notch pathway is activated when the extracellular domain of the Notch receptor binds to Notch ligands. Upon ligand binding, Notch receptors undergo two proteolytic cleavages, the first cleavage is performed in the extracellular region and catalyzed by the ADAM family of metalloproteases, while the second occurs in the intracellular region mediated by the y-secretase enzyme complex (presenilin, nicastrin, PEN2, and APH1). These cleavages provoke the release of the Notch intracellular domain (NICD) from the membrane and the subsequent translocation into the nucleus, where it forms a complex with CSL and a member of the Mastermind (MAM) family of coactivators to regulate the transcription of Notch target genes (Miele et al., 2006; Harrison et al., 2010a).
It is well known that the notch signal is an important regulator of normal mammary stem cells (Farnie and Clarke, 2007). Aberrant expression of Notch receptors has been observed in breast cancer and is associated with poor prognosis. Notch 1 and Notch 4 are enriched in BCSCs compared to differentiated cells, both receptors have been reported to regulate breast cancer stem cells. Remarkably, Notch 4 exerts a stronger effect on the maintenance of BCSCs (CD44+/CD24-/ESA+). Notch 1 and 4 inhibition impair breast cancer stem cell activity by reducing ALDH activity thus reducing tumor growth and render CSCs resistant to drug therapy. This has been observed in MCF7, T47D and ZR75-1 breast cancer cell lines (Harrison et al., 2010b; Simões et al., 2015).
Notch signaling increases cell proliferation, angiogenesis, apoptosis, and stimulates drug resistance, EMT, metastasis, and increases BCSC numbers. Hyperactivation of notch signal activity has been found in BCSCs, this pathway regulates the properties of a large number of BCSCs. (Wu, 2007; Wong et al., 2012; Lagadec et al., 2013; Peng et al., 2014; D’Angelo et al., 2015; Acar et al., 2016; Pal et al., 2017; Kontomanolis et al., 2018).
A study reported that Notch signaling induces stemness by promoting the deacetylation and subsequent activation of ALDH1A1 (Zhao D. et al., 2014). Additionally, the Notch signal highly correlates with Ki-67 expression in BCSCs (Cui et al., 2015). Notch signal induced by radiation or hypoxia leads to an acquisition of a breast cancer stem cell phenotype (Xing et al., 2011; Wong et al., 2012; Lagadec et al., 2013).
Different molecules regulate stemness in breast cancer through the Notch signal. Majumder et al., demonstrated that Cox-2 is capable of inducing BCSCs with high ALDH activity by promoting Notch expression and activating Notch signaling in MCF7 and SKBR3 cell lines (Majumder et al., 2016). Another study revealed that Mel-18 blocks the Notch signal by inhibiting the expression of the Jagged-1 ligand and thus reducing BCSCs (CD44high/CD24low) in MCF7 cells (Won et al., 2012). Likewise, Garcia-Heredia et al., showed that Numbl inhibition induces Notch activity and promotes the acquisition of a stem cell phenotype in the T47D cell line (García-Heredia et al., 2016). Additionally, MAPK17 kinase interacts with NUMB, a notch inhibitor, and facilitates the activation of the Notch pathway which in turn expands the BCSC fraction (Garcia-Heredia et al., 2017). The SATB1 molecule is also able of activating the Notch signal and regulating the stem phenotype in breast cancer (Sun et al., 2015). Some microRNAs such as mir-129 decrease the Notch signal by suppressing the expression of Cyclin d1/DICER and thus inhibiting the stem cell phenotype. Mir526b-3p is commonly reduced in breast cancer, when expressed, it regulates the Hif2a/Notch signal and inhibits stemness (Yan et al., 2018). Mir34a also downregulates the Notch1 receptor, inhibits stemness, and renders cancer cells more sensitive to paclitaxel (Kang et al., 2015).
In line with previous observations, Notch signaling induces the expansion of the BCSCs, suggesting that inhibitors of this signaling system could decrease this cell population and improve therapy response in these tumors.
The Hedgehog pathway (HH) is a signaling system involved in tissue homeostasis, embryogenesis, development, and regeneration. Hedgehog molecules constitute a small family of secreted signaling proteins including Sonic Hedgehog (SHH), Indian Hedgehog (IHH), and Desert Hedgehog (DHH). The Patched receptor (PTCH), the transmembrane protein Smoothened (SMO), and Gli transcription factors (Gli 1, 2 and 3) along with HH ligands are the major players in Hedgehog signaling (Bhateja et al., 2019). The Gli code has been proposed as a phenomenon where the collaborative action of three transcription factors, namely Gli1, Gli2, and Gli3 with their respective activating and repressing functions, is necessary for the integration of the Hedgehog signaling pathway within the cells. The Gli code undergoes modifications when HH ligands are present, resulting in the transcription and activation of Gli1 and the inhibition of Gli2 and Gli3 processing (Ruiz i Altaba et al., 2007). Mechanistically, in the absence of HH ligands, the patched receptor inhibits the smoothened receptor, and gene expression is repressed by Gli1 and Gli2. Upon ligand binding to PTCH, the repression of SMO is relieved, allowing the activation of GLI proteins to facilitate the transcription of target genes (Bhateja et al., 2019).
The Hedgehog pathway is largely inactive in most postnatal tissues, but this is commonly activated in cancer. Recent finding has demonstrated that the Gli code not only regulates stemness but also plays a crucial role in tumor progression and the development of metastatic lesions (Ruiz i Altaba et al., 2007). This pathway favors tumor progression and is associated with aggressive tumors with high CSC content in breast cancer (Kasper et al., 2009; Zhao et al., 2016; Riobo-Del Galdo et al., 2019). Accumulating evidence indicates that Hedgehog signaling plays a role in the regulation of CSC properties by promoting self-renewal, stemness, and drug resistance in breast tumors (Liu et al., 2006; Tanaka et al., 2009; He et al., 2015; Sims-Mourtada et al., 2015).
Different studies revealed that PTCH1, GLI1, GLI2, and SMO are highly expressed in the CSC fraction and their expression reduces upon stem cell differentiation. Activation of Hedgehog signaling promotes tumorigenesis and metastasis, increases self-renewal, proliferation, and sphere forming-efficiency of BCSCs via SHH-mediated upregulation of the polycomb protein Bmi-1 (Liu et al., 2006; Wang L. et al., 2014).
Notably, CD24, a protein absent or low expressed in BCSCs, decreases the stem cell phenotype by inhibiting the expression of SHH and GLI1, and deactivating the Hedgehog pathway (Suyama et al., 2016). In addition, various molecules regulate stemness by potentiating the Hedgehog signal. Yuan Cao and collaborators showed that glutamic-pyruvic transaminase (GPT2) increases stemness by reducing α-ketoglutarate levels and inhibiting the enzyme proline hydroxylase 2 (PHD2) involved in the regulation of HIF1a stability. Accumulation of HIF1α levels results in the constitutive activation of the SHH signaling (Cao et al., 2017). Similarly, p63 regulates the expression levels of SHH, GLI2, and PTCHD1, thus facilitating their activity and expanding the number of BCSCs (Memmi et al., 2015). Otherwise, the transcription factor FOXC1 (Forkhead box C1 protein) mediates the activation of the SMO-independent Hedgehog signal by interacting and activating Gli2, thus inducing the activity of ALDH1 and promoting the self-renewal of CSCs in basal breast cancers (Han et al., 2015, 2016). Moreover, the ETV4 transcription factor also activates the Hedgehog signaling by promoting the expression of CXCR4 and thus enriching stemness by favoring the glycolytic activity of BCSCs (Zhu et al., 2021). The enzyme 24-dehydrocholesterol reductase (DHCR24) also expands the BCSC fraction through the activation of the HH signaling (Qiu et al., 2020). Another study found that tetraspanin 8 (TSPAN8) interacts with PTCH1, stabilizing its membrane location, and subsequently favoring BCSCs, drug resistance, and tumorigenesis by inducting the activity of hedgehog signaling (Zhu R. et al., 2019). Circ_DCAF6 RNA is also able to promote HH signaling by inducing GLI1 expression through sequestering mir-616-3p and thus expanding the CSC fraction (Ye et al., 2020).
Collectively, these data suggest that Hedgehog signaling induces stemness, regulates self-renewal, and participates in the CSC-driven propagation of breast cancer. Since HH signaling exerts profound implications in the expansion of CSCs, molecules disrupting this pathway are ideal therapeutic targets to reduce the fraction of BCSCs to achieve a durable clinical response.
Hippo signaling is an evolutive conserved pathway that regulates development, tissue homeostasis, and organ size. The hippo pathway consists of both a kinase cascade (MST and LATS) and a downstream transcriptional module (YAP and TAZ). The kinases are composed of MST1 and MST2, which phosphorylate and activate downstream kinases LATS1 and LATS2, and their scaffold MOB1A/B. The hippo signaling pathway is activated when MST1/2, LATS1/2, and MOB1A/B are phosphorylated. Hippo activation results in the inactivation of the transcriptional coactivators YAP and TAZ mediated by LAST1/2 phosphorylation. Phosphorylated of YAP and TAZ results in their localization in the cytoplasm through binding to 14-3-3 protein, followed by their degradation in a ubiquitin-proteasome-dependent manner. (Maugeri-Saccà and De Maria, 2016; Wu and Guan, 2021). Conversely, when the hippo pathway is inactivated, dephosphorylated YAP and TAZ translocate to the nucleus and through the TEAD family of transcription factors, induce gene expression.
Hippo signaling has been associated with normal mammary development. In cancer, YAP/TAZ act as oncogenes promoting proliferation, invasion, migration, epithelial-mesenchymal transition, metastasis, and BCSCs self-renewal (Shi et al., 2014; Maugeri-Saccà and De Maria, 2016). Accumulated evidence has shown that YAP/TAZ signaling regulates BCSC maintenance. TAZ is overexpressed in breast cancer and indispensable to promoting the self-renewal of BCSCs (Cordenonsi et al., 2011). Bartucci et al., (2015) shows that TAZ is an important mediator of metastasis, chemo-resistance and tumorigenesis of BCSCs. Chang et al., 2015 show that TAZ regulated BCSCs self-renewal through Laminin 511 matrix (Chang et al., 2015).
Other molecules can regulate BCSCs maintenance through Hippo/YAP/TAZ signaling. A recent study identify that FOXM1 is overexpressed in breast cancer and promotes proliferation, migration, and stemness through the Hippo signaling pathway (Sun et al., 2020). Another study shows that RUN1/3 acts as a negative regulator of YAP signaling and inhibits migration and stemness in breast cancer (Kulkarni et al., 2018). Mir-520b is also overexpressed in BCSCs and promotes stemness through the Hippo signaling (Zhang et al., 2019). Mir-125a regulated Hippo signaling through LIFR and promote BCSCs (Nandy et al., 2015). LncRNA SOX21-AS1 is overexpressed in breast cancer and promotes BCSCs, proliferation, invasion, and migration through promoting YAP nuclear translocation.
The TGF-β (Transforming growth factor beta) signaling pathway is a complex cellular signaling network that plays an important role in a variety of normal and pathological processes. TGF-β represents a family of soluble proteins including TGF-β1, TGF-β2 and TGF-β3, BMPs (bone morphogenic proteins), activin, growth differentiation factors (GDFs), nodal, and the müllerian inhibiting substance (MIS), which act through type I and II transmembrane serine-threonine receptors (Tzavlaki and Moustakas, 2020; Babyshkina et al., 2021).
The TGF-β signaling consists of two branches, a canonical pathway transduced via SMAD and a non-canonical pathway independent of SMAD proteins. These branches activate distinct target genes and frequently exhibit opposite functional roles. In canonical signaling, binding of TGF-β ligands induces the formation of a heterotetrameric active receptor complex (formed by a dimer of TGF-β and homodimers of both TGF-βRII and TGF-βRI) which results in the phosphorylation of TGF-βR1 by TGF-βR2. TGF-βI phosphorylates R-Smad proteins (Smad1/2/3/5/8), which form complexes with the common partner Smad (co-Smad; Smad4) and translocates to the nucleus to regulate the transcription of their target genes in conjunction with other DNA-binding transcription. Inhibitory SMADs such as SMAD6 and 7 (I-SMAD) can inhibit the signaling (Hata and Chen, 2016; Tzavlaki and Moustakas, 2020). In the non-canonical signaling also termed non-Smad pathways, TGF-β receptor complex phosphorylate alternative molecules such as TGF-β activated kinase 1 (TAK1), aPKC, Par6, Akt, and PI3K, which regulates several processes including apoptosis, proliferation, differentiation and migration (Zhang, 2017).
It is well known that TGF-β signaling is an important regulator of tumorigeneses by inducing epithelial-mesenchymal transition and regulating BCSCs maintenance. TGF-β ser 69 and 74 phosphorylation recruit SMAD3/p53 complex and regulate the transcription of BCSCs resistance genes (Zakharchenko et al., 2013). Zheng et al., (2014) showed that TGF-β2 expression correlates with the BCSCs marker ALDH1 and represents a bad prognosis. Another study showed that CD49Fhigh and CD61high BCSCs are regulated by TGF-β in HER2+ breast cancer. (Lo et al., 2012). Recent studies have shown that TGF-β is capable to induce epithelial-mesenchymal transition, invasion, and lung metastasis and regulates apoptosis and resistance of BCSCs (Konge et al., 2018; Xu et al., 2018; Zhang et al., 2018; Katsuno et al., 2019; Yadav and Shankar, 2019; Tsubakihara et al., 2022).
Different molecules can regulate BCSCs maintenance through TGF-β. Iwanaga et al., show that Six1 promotes BCSCs through TGF-β and MAPK in luminal breast cancer (Iwanaga et al., 2012). In claudin-low breast cancer, NEDD9 is necessary to promote the expansion of BCSCs mediated by TGFβ/Smad and Rho-actin-SRF-dependent signals (Bruna et al., 2012).
A recent study showed that PARP3 promotes stemness and TGFβ-dependent EMT by inducing the Snail-E-cadherin axis and facilitating the acquisition of cell motility (Karicheva et al., 2016). Recent evidence indicates that RAD18 promotes the stem-cell phenotype through the Hippo/YAP/TGF-β pathway, resulting in the activation of M2 tumor-associated macrophages in triple-negative breast cancer (Yan et al., 2022). In addition, macrophages are capable to induce ERK/TGF-β1 signaling and promote BCSCs (Kundu and Shankar, 2022). Histamine H4 agonists also promote BCSCs and epithelial-mesenchymal transition through TGF-β and Src signaling (Galarza et al., 2020). Aurora-A kinase mediates TGF-β activation and promotes ALDH-positive cells, self-renewal, and resistance in breast cancer (Jalalirad et al., 2021). ALG3 promotes radioresistance and stemness through TGF-βRII glycosylation (Sun et al., 2021) ILEI/LIFR regulates BCSCS through TGF-β (Woosley et al., 2019). It has been observed that Leptin 1 and COX2 promote BCSCs through TGF-β1 (Mishra et al., 2017; Tian et al., 2017).
It has been reported that Autophagy promotes BCSCs through STAT3 and TGF-β/SMAD signaling (Yeo et al., 2016). Hyaluronan promotes aggressiveness in tumors through induce BCSCs, EMT, snail, twist, and TGF-β signaling (Chanmee et al., 2014). TGF-β overexpresses PMEPA1 and increases BCSCs (Nie et al., 2015).
Since, several signaling pathways regulating CSCs are known to be dysregulated in CSCs and contribute to CSCs survival, inhibitors of these pathways have been developed and tested in preclinical and clinical studies. Targeting signaling pathways regulating CSCs is a promising therapeutic approach for cancer treatment. Various authors have evaluated the effect of signaling pathway inhibitors in BCSCs as an alternative therapeutic approach for treating breast cancer (Table 1).
Accumulated evidence has shown that Wnt/β-catenin inhibition decreases BCSCs, metastasis, and resistance to conventional therapy (Jang et al., 2015b; Shamsian et al., 2020; Abreu de Oliveira et al., 2021). A study in 2021 shows that antibodies against Frizzled-7 are capable of reducing invasion, migration, epithelial-mesenchymal transition, and breast cancer stem cell fraction in triple-negative breast cancer (Xie et al., 2021). Another study showed that apatinib is capable of reducing BCSC through Wnt/β-catenin inhibition. (Jiang et al., 2022). In the same way, the small molecule inhibitors PKF118–310 and CWP23228 decrease BCSCs by disrupting the interaction between β-catenin and Tcf/Lef transcription factors, thereby inhibiting Wnt target gene expression (Hallett et al., 2012; Jang et al., 2015a).
Furthermore, organic components such as diallyl trisulfide, ursolic acid, curcumin, plumbagin, prodigiosin, diosgenin, hydroxytyrosol, resveratrol, and oxymatrine are capable of inhibiting Wnt/β-catenin-induced BCSCs (Zhang et al., 2011; Fu et al., 2014; Wang et al., 2016; Bhuvanalakshmi et al., 2017; Li et al., 2018a, 2018b; Cruz-Lozano et al., 2019; Sakunrangsit and Ketchart, 2019; Mandal et al., 2021).
It is also well known that NF-κB inhibition impairs the self-renewal of BCSCs. A study in 2019 shows that nalbuphine is capable to inhibit AKT/NF-κB signaling and reduce BCSCs (Yu et al., 2019). Organic components such as 6-methoxymellein, anthocyanins, pterostilbene, eugenol, tanshinone IIA, sulforaphane, and machilin D are capable to inhibit NF-κB-induced BCSCs and drug resistance in breast cancer (Lin et al., 2013; Mak et al., 2013; Burnett et al., 2017; Islam et al., 2018; Liu R. et al., 2020; Paramanantham et al., 2020; Zhen et al., 2020). Furthermore, disulfiram and aspirin disrupt the NF-κB signaling by inhibiting the phosphorylation and degradation of IκBα (Yip et al., 2011; Saha et al., 2016). Sulconazole, also inhibits the translocation of NF-κB from cytoplasm to the nucleus, thus disrupting the NF-κB pathway. (Kastrati et al., 2017; Choi et al., 2019). Parthenolide, pyrrolidine dithiocarbamate, and its analog diethyldithiocarbamate were found to inhibit the NF-kB activity and diminish the proliferation and colony formation of BCSCs (Zhou et al., 2008). In vivo, PDTC was also able to disrupt tumor onset and growth, and its effect was enhanced by paclitaxel (Zhou et al., 2008).
Disruption of the notch signaling reduces BCSCs, resistance, and metastasis in vivo and in vitro in breast cancer. (Grudzien et al., 2010; McGowan et al., 2011; Simmons et al., 2012; Qiu et al., 2013; Peng et al., 2014). γ-secretase inhibitors reduce the CSCs population and inhibit self-renewal. A study shows that γ-secretase inhibitors in combination with ErbB1/2 inhibitors (lapatinib and gefitinib) decrease CSCs in preclinical models (Farnie et al., 2013). Other γ-secretase inhibitors such as MRK-003 and GSIXII inactivate Notch signaling and decrease BCSCs in mice (Kondratyev et al., 2012; Séveno et al., 2012). Furthermore, natural components such as psoralidin, celastrol y triptolide are capable to inhibit BCSCs and epithelial-mesenchymal transition by disrupting the Notch signaling pathway. (Pal et al., 2017; Ramamoorthy et al., 2021).
It is well demonstrated that Hedgehog inhibition reduces the BCSCs fraction, impairs self-renewal, and sensitizes CSCs to drug therapy. Gli1/2 inhibitors such as GANT61 and HPI-1 reduce proliferation, and migration, increase apoptosis and reduce BCSCs (Kurebayashi et al., 2017; Jeng et al., 2018). Furthermore, natural compounds such as nitidine chloride, huaier aqueous extract, physalin A, and genistein are capable to impaired migration, and invasion and reducing BCSCs through Hedgehog inhibition (Fan et al., 2013; Wang X. et al., 2014; Sun et al., 2016; Ko et al., 2021). Pharmacological components such as metformin, salinomycin, and thiostrepton inhibit Hedgehog signaling and the BCSCs population (Fan et al., 2015; Lu et al., 2015; Yang et al., 2016). In addition, many studies have shown that the inhibition of YAP, a transcription factor regulating BCSCs, reduces drug resistance in breast cancer (Guimei et al., 2020). Further, pharmacological components such as quinacrine are capable to reduce YAP and LATS1/2 expression in breast cancer (Darbankhales et al., 2020). Chlorpromazine inhibits YAP and decreases BCSCs resistance (Yang et al., 2019). Ciclesonide is capable to inhibit glucocorticoid receptor-dependent YAP signaling and decreasing BCSCs (Kim et al., 2020). The natural component physalin A also reduces YAP1 levels in breast cancer and impacts the proportion of BCSCs (Ko et al., 2021).
TGF-β inhibition may target CSCs by promoting their differentiation and blocking their expansion (Sulaiman et al., 2021). TGF-β inhibition mediated by LY2157299, an inhibitor of the TGF-β receptor 1 kinase, and a siRNA against SMAD4 blocks BCSCs expansion, impairs mammosphere formation and reduces drug resistance (Bhola et al., 2013). Vactosertib targets the kinase activity of ALK5 (Activin receptor-like kinase 5), which is a receptor for the TGF-β signaling pathway. By inhibiting ALK5, vactosertib can interfere with TGF-β signaling and reduces epithelial-mesenchymal transition, in vivo metastasis, and BCSC fraction through ROS reduction (Choi et al., 2022). ALK5 and EW-7197 inhibitors reduce paclitaxel-promoting epithelial-mesenchymal transition and BCSC numbers, suggesting that combined treatment of paclitaxel with TGF-β inhibitors attenuate breast cancer metastasis and BCSCs (Park et al., 2015). ZL170 inhibits proliferation, epithelial-mesenchymal transition, stemness, invasion, and migration via inhibition of TGF-β/BMP-SMAD pathways in triple-negative breast cancer (Di et al., 2019). Caffeic acid reduces BCSCs for induction of microRNA-148a. These microRNAs inhibit TGF-β/SAMD2 signaling (Li et al., 2015).
Collectively these data depicted inhibitors of signaling pathways showing promise for the treatment of breast cancer, and several agents are currently being tested either as monotherapy or in combination with other conventional therapies. Overall, targeting signaling pathways regulating CSCs is a promising therapeutic approach for cancer treatment, but further research is needed to overcome the challenges and optimize the clinical efficacy of this approach.
BCSCs are a group of cells within tumors with the ability to self-renew and differentiate into non-CSCs that form the bulk of the tumor. CSCs are responsible for tumor maintenance, resistance, and relapse in breast cancer patients. Targeting CSCs has emerged as a promising therapeutic approach for the treatment of cancer. In recent years, significant progress has been made in developing therapeutic interventions for targeting CSCs. These interventions include the use of small molecules to inhibit the signaling pathways that are esential for the maintenance and self-renewal of CSCs, such as the Wnt, NF-kB, Notch, Hedgehog, Hippo, and TGF-β signaling pathways. Recent approaches have revealed that the inhibition of key molecules involved in those signaling pathways reduces the CSC fraction and impairs self-renewal.
Although significant advancements have been achieved, there are still several challenges that need to be addressed. First, CSCs are heterogeneous and could rely on different signaling pathways depending on the tumor type and stage. Second, CSCs may adapt to the inhibition of a given single pathway by activating compensatory pathways. Third, some signaling pathways also play important roles in normal stem cells and tissues, which can lead to toxic side effects. Therefore, combination therapies targeting multiple pathways or combining CSC-targeting agents with conventional chemotherapy or radiotherapy could be more effective in eliminating CSCs and preventing tumor recurrence.
In conclusion, the development of CSC-targeted therapies represents an exciting area of research with the potential to revolutionize cancer treatment. Despite the challenges, progress has been made in developing therapeutic interventions that can effectively target CSCs. Continued research in this field is crucial for creating more effective and specific CSC-targeted therapies that can ultimately improve patient outcomes.
AO-R and KV-S drafted the manuscript and conceived the original idea. OT-J and KV-S reviewed and edited the manuscript. All authors contributed to the article and approved the submitted version.
KV-S is supported by a Women in Science Fellowship from LOREAL/UNESCO/AMC 2018, AO-R and OT-J are supported by a CONACYT PhD research scholarship.
This paper is part of the production obtained for the Doctoral degree of AO-R and OT-J at the Posgrado en Ciencias Biológicas, UNAM. We thank designer Javier de Jesus Cervantes Estrada for his excellent contribution to figure design.
The authors declare that the research was conducted in the absence of any commercial or financial relationships that could be construed as a potential conflict of interest.
All claims expressed in this article are solely those of the authors and do not necessarily represent those of their affiliated organizations, or those of the publisher, the editors and the reviewers. Any product that may be evaluated in this article, or claim that may be made by its manufacturer, is not guaranteed or endorsed by the publisher.
Abreu de Oliveira, W. A., Moens, S., El Laithy, Y., van der Veer, B. K., Athanasouli, P., Cortesi, E. E., et al. (2021). Wnt/β-Catenin inhibition disrupts carboplatin resistance in isogenic models of triple-negative breast cancer. Front. Oncol. 11, 705384. doi:10.3389/fonc.2021.705384
Acar, A., Simões, B. M., Clarke, R. B., and Brennan, K. (2016). A role for notch signalling in breast cancer and endocrine resistance. Stem Cells Int. 2016, 2498764. doi:10.1155/2016/2498764
Asiedu, M. K., Beauchamp-Perez, F. D., Ingle, J. N., Behrens, M. D., Radisky, D. C., and Knutson, K. L. (2014). AXL induces epithelial-to-mesenchymal transition and regulates the function of breast cancer stem cells. Oncogene 33, 1316–1324. doi:10.1038/onc.2013.57
Babyshkina, N., Dronova, T., Erdyneeva, D., Gervas, P., and Cherdyntseva, N. (2021). Role of TGF-β signaling in the mechanisms of tamoxifen resistance. Cytokine Growth Factor Rev. 62, 62–69. doi:10.1016/j.cytogfr.2021.09.005
Bartucci, M., Dattilo, R., Moriconi, C., Pagliuca, A., Mottolese, M., Federici, G., et al. (2015). TAZ is required for metastatic activity and chemoresistance of breast cancer stem cells. Oncogene 34, 681–690. doi:10.1038/onc.2014.5
Benard, O., Qian, X., Liang, H., Ren, Z., Suyama, K., Norton, L., et al. (2019). p21CIP1 promotes mammary cancer–initiating cells via activation of wnt/TCF1/CyclinD1 signaling. Mol. Cancer Res. 17, 1571–1581. doi:10.1158/1541-7786.MCR-18-1044
Bhateja, P., Cherian, M., Majumder, S., and Ramaswamy, B. (2019). The hedgehog signaling pathway: A viable target in breast cancer? Cancers (Basel) 11, 1126. doi:10.3390/cancers11081126
Bhola, N. E., Balko, J. M., Dugger, T. C., Kuba, M. G., Sánchez, V., Sanders, M., et al. (2013). TGF-β inhibition enhances chemotherapy action against triple-negative breast cancer. J. Clin. Invest. 123, 1348–1358. doi:10.1172/JCI65416
Bhuvanalakshmi, G., Basappa, , , Rangappa, K. S., Dharmarajan, A., Sethi, G., Kumar, A. P., et al. (2017). Breast cancer stem-like cells are inhibited by diosgenin, a steroidal saponin, by the attenuation of the Wnt β-catenin signaling via the Wnt antagonist secreted frizzled related protein-4. Front. Pharmacol. 8, 1–14. doi:10.3389/fphar.2017.00124
Bruna, A., Greenwood, W., Le Quesne, J., Teschendorff, A., Miranda-Saavedra, D., Rueda, O. M., et al. (2012). TGFβ induces the formation of tumour-initiating cells in claudin low breast cancer. Nat. Commun. 3, 1055. doi:10.1038/ncomms2039
Burnett, J. P., Lim, G., Li, Y., Shah, R. B., Lim, R., Paholak, H. J., et al. (2017). Sulforaphane enhances the anticancer activity of taxanes against triple negative breast cancer by killing cancer stem cells. Cancer Lett. 394, 52–64. doi:10.1016/j.canlet.2017.02.023
Cai, W. Y., Wei, T. Z., Luo, Q. C., Wu, Q. W., Liu, Q. F., Yang, M., et al. (2013). The wnt-β-catenin pathway represses let-7 microrna expression through transactivation of Lin28 to augment breast cancer stem cell expansion. J. Cell Sci. 126, 2877–2889. doi:10.1242/jcs.123810
Cao, Y., Lin, S. H., Wang, Y., Chin, Y. E., Kang, L., and Mi, J. (2017). Glutamic pyruvate transaminase GPT2 promotes tumorigenesis of breast cancer cells by activating sonic hedgehog signaling. Theranostics 7, 3021–3033. doi:10.7150/thno.18992
Cao, Y., Luo, J. L., and Karin, M. (2007). IkappaB kinase alpha kinase activity is required for self-renewal of ErbB2/Her2-transformed mammary tumor-initiating cells. Proc. Natl. Acad. Sci. U. S. A. 104, 15852–15857. doi:10.1073/pnas.0706728104
Chang, C., Goel, H. L., Gao, H., Pursell, B., Shultz, L. D., Greiner, D. L., et al. (2015). A laminin 511 matrix is regulated by Taz and functions as the ligand for the α6Bβ1 integrin to sustain breast cancer stem cells. Genes Dev. 29, 1–6. doi:10.1101/gad.253682.114
Chanmee, T., Ontong, P., Mochizuki, N., Kongtawelert, P., Konno, K., and Itano, N. (2014). Excessive hyaluronan production promotes acquisition of cancer stem cell signatures through the coordinated regulation of twist and the transforming growth factor β (TGF-β)-snail signaling axis. J. Biol. Chem. 289, 26038–26056. doi:10.1074/jbc.M114.564120
Chen, C., Cao, F., Bai, L., Liu, Y., Xie, J., Wang, W., et al. (2015). IKKβ enforces a LIN28B/TCF7L2 positive feedback loop that promotes cancer cell stemness and metastasis. Cancer Res. 75, 1725–1735. doi:10.1158/0008-5472.CAN-14-2111
Chen, J., Luo, Q., Yuan, Y., Huang, X., Cai, W., Li, C., et al. (2010). Pygo2 associates with MLL2 histone methyltransferase and GCN5 histone acetyltransferase complexes to augment wnt target gene expression and breast cancer stem-like cell expansion. Mol. Cell. Biol. 30, 5621–5635. doi:10.1128/mcb.00465-10
Chen, Z., and Xue, C. (2019). G-Protein-Coupled receptor 5 (LGR5) overexpression activates β-catenin signaling in breast cancer cells via protein kinase A. Med. Sci. Monit. Basic Res. 25, 15–25. doi:10.12659/MSMBR.912411
Choi, H. S., Kim, J. H., Kim, S. L., and Lee, D. S. (2019). Disruption of the nf-κb/il-8 signaling axis by sulconazole inhibits human breast cancer stem cell formation. Cells 8, 1007. doi:10.3390/cells8091007
Choi, J., Park, J., Cho, I., and Sheen, Y. (2022). Co-treatment with vactosertib, a novel, orally bioavailable activin receptor-like kinase 5 inhibitor, suppresses radiotherapy-induced epithelial-to-mesenchymal transition, cancer cell stemness, and lung metastasis of breast cancer. Radiol. Oncol. 56, 185–197. doi:10.2478/raon-2022-0012
Cordenonsi, M., Zanconato, F., Azzolin, L., Forcato, M., Rosato, A., Frasson, C., et al. (2011). The hippo transducer TAZ confers cancer stem cell-related traits on breast cancer cells. Cell 147, 759–772. doi:10.1016/j.cell.2011.09.048
Courtine, E., Cagnard, N., Mazzolini, J., Antona, M., Pène, F., Fitting, C., et al. (2012). Combined loss of cRel/p50 subunits of NF-κB leads to impaired innate host response in sepsis. Innate Immun. 18, 753–763. doi:10.1177/1753425912440296
Cruz-Lozano, M., González-González, A., Marchal, J. A., Muñoz-Muela, E., Molina, M. P., Cara, F. E., et al. (2019). Hydroxytyrosol inhibits cancer stem cells and the metastatic capacity of triple-negative breast cancer cell lines by the simultaneous targeting of epithelial-to-mesenchymal transition, Wnt/β-catenin and TGFβ signaling pathways. Eur. J. Nutr. 58, 3207–3219. doi:10.1007/s00394-018-1864-1
Cui, J., Li, P., Liu, X., Hu, H., and Wei, W. (2015). Abnormal expression of the notch and Wnt/β-catenin signaling pathways in stem-like ALDHhiCD44+ cells correlates highly with Ki-67 expression in breast cancer. Oncol. Lett. 9, 1600–1606. doi:10.3892/ol.2015.2942
Cuyàs, E., Corominas-Faja, B., Martín, M. M. S., Martin-Castillo, B., Lupu, R., Brunet, J., et al. (2017). BRCA1 haploinsufficiency cell-autonomously activates RANKL expression and generates denosumab-responsive breast cancerinitiating cells. Oncotarget 8, 35019–35032. doi:10.18632/oncotarget.16558
D’Angelo, R. C., Ouzounova, M., Davis, A., Choi, D., Tchuenkam, S. M., Kim, G., et al. (2015). Notch reporter activity in breast cancer cell lines identifies a subset of cells with stem cell activity. Mol. Cancer Ther. 14, 779–787. doi:10.1158/1535-7163.MCT-14-0228
Darbankhales, S., Mirfakhraie, R., Ghahremani, H., Asadolahi, M., Saket-Kisomi, K., Safakish, L., et al. (2020). Effects of quinacrine on expression of hippo signaling pathway components (LATS1, LATS2, and YAP) in human breast cancer stem cells. Asian Pac. J. Cancer Prev. 21, 3171–3176. doi:10.31557/APJCP.2020.21.11.3171
Debeb, B. G., Lacerda, L., Xu, W., Larson, R., Solley, T., Atkinson, R., et al. (2012). Histone deacetylase inhibitors stimulate dedifferentiation of human breast cancer cells through WNT/β-catenin signaling. Stem Cells 30, 2366–2377. doi:10.1002/stem.1219
Di, L., Liu, L. J., Yan, Y. M., Fu, R., Li, Y., Xu, Y., et al. (2019). Discovery of a natural small-molecule compound that suppresses tumor EMT, stemness and metastasis by inhibiting TGFβ/BMP signaling in triple-negative breast cancer. J. Exp. Clin. Cancer Res. 38, 134. doi:10.1186/s13046-019-1130-2
Eterno, V., Zambelli, A., Villani, L., Tuscano, A., Manera, S., Spitaleri, A., et al. (2016). AurkA controls self-renewal of breast cancer-initiating cells promoting wnt3a stabilization through suppression of MIR-128. Sci. Rep. 6, 28436. doi:10.1038/srep28436
Fan, C., Wang, Y., Liu, Z., Sun, Y., Wang, X., Wei, G., et al. (2015). Metformin exerts anticancer effects through the inhibition of the Sonic hedgehog signaling pathway in breast cancer. Int. J. Mol. Med. 36, 204–214. doi:10.3892/ijmm.2015.2217
Fan, P., Fan, S., Wang, H., Mao, J., Shi, Y., Ibrahim, M. M., et al. (2013). Genistein decreases the breast cancer stem-like cell population through Hedgehog pathway. Stem Cell Res. Ther. 4, 146. doi:10.1186/scrt357
Farnie, G., and Clarke, R. B. (2007). Mammary stem cells and breast cancer - role of notch signalling. Stem Cell Rev. 3, 169–175. doi:10.1007/s12015-007-0023-5
Farnie, G., Willan, P. M., Clarke, R. B., and Bundred, N. J. (2013). Combined inhibition of ErbB1/2 and notch receptors effectively targets breast ductal carcinoma in situ (DCIS) stem/progenitor cell activity regardless of ErbB2 status. PLoS One 8, e56840–e56847. doi:10.1371/journal.pone.0056840
Feng, Y., Spezia, M., Huang, S., Yuan, C., Zeng, Z., Zhang, L., et al. (2018). Breast cancer development and progression: Risk factors, cancer stem cells, signaling pathways, genomics, and molecular pathogenesis. Genes Dis. 5, 77–106. doi:10.1016/j.gendis.2018.05.001
Fu, Y., Chang, H., Peng, X., Bai, Q., Yi, L., Zhou, Y., et al. (2014). Resveratrol inhibits breast cancer stem-like cells and induces autophagy via suppressing Wnt/β-catenin signaling pathway. PLoS One 9, e102535–e102538. doi:10.1371/journal.pone.0102535
Galarza, T. E., Táquez Delgado, M. A., Mohamad, N. A., Martín, G. A., and Cricco, G. P. (2020). Histamine H4 receptor agonists induce epithelial-mesenchymal transition events and enhance mammosphere formation via Src and TGF-β signaling in breast cancer cells. Biochem. Pharmacol. 180, 114177. doi:10.1016/j.bcp.2020.114177
Gao, X., and Dong, Q.-Z. (2020). Advance in metabolism and target therapy in breast cancer stem cells. World J. Stem Cells 12, 1295–1306. doi:10.4252/wjsc.v12.i11.1295
Garcia-Heredia, J. M., Lucena-Cacace, A., Verdugo-Sivianes, E. M., Perez, M., and Carnero, A. (2017). The cargo protein MAP17 (PDZK1IP1) regulates the cancer stem cell pool activating the Notch pathway by abducting NUMB. Clin. Cancer Res. 23, 3871–3883. doi:10.1158/1078-0432.CCR-16-2358
García-Heredia, J. M., Verdugo Sivianes, E. M., Lucena-Cacace, A., Molina-Pinelo, S., and Carnero, A. (2016). Numb-Like (NumbL) downregulation increases tumorigenicity, cancer stem cell-like properties and resistance to chemotherapy. Oncotarget 7, 63611–63628. doi:10.18632/oncotarget.11553
Glinka, Y., Mohammed, N., Subramaniam, V., Jothy, S., and Prud’homme, G. J. (2012). Neuropilin-1 is expressed by breast cancer stem-like cells and is linked to NF-κB activation and tumor sphere formation. Biochem. Biophys. Res. Commun. 425, 775–780. doi:10.1016/j.bbrc.2012.07.151
Grudzien, P., Lo, S., Albain, K. S., Robinson, P., Rajan, P., Strack, P. R., et al. (2010). Inhibition of notch signaling reduces the stem-like population of breast cancer cells and prevents mammosphere formation. Anticancer Res. 30, 3853–3867. doi:10.1158/0008-5472.sabcs-106
Guimei, M., Alrouh, S., Saber-Ayad, M., Hafezi, S. A., Vinod, A., Rawat, S., et al. (2020). Inhibition of yes-associated protein-1 (Yap1) enhances the response of invasive breast cancer cells to the standard therapy. Breast Cancer Targets Ther. 12, 189–199. doi:10.2147/BCTT.S268926
Gujral, T. S., Chan, M., Peshkin, L., Sorger, P. K., Kirschner, M. W., and MacBeath, G. (2014). A noncanonical Frizzled2 pathway regulates epithelial-mesenchymal transition and metastasis. Cell 159, 844–856. doi:10.1016/j.cell.2014.10.032
Hallett, R. M., Kondratyev, M. K., Giacomelli, A. O., Nixon, A. M. L., Girgis-Gabardo, A., Ilieva, D., et al. (2012). Small molecule antagonists of the Wnt/β-catenin signaling pathway target breast tumor-initiating cells in a Her2/Neu mouse model of breast cancer. PLoS One 7, e33976. doi:10.1371/journal.pone.0033976
Han, B., Qu, Y., Jin, Y., Yu, Y., Deng, N., Wawrowsky, K., et al. (2015). FOXC1 activates smoothened-independent hedgehog signaling in basal-like breast cancer. Cell Rep. 13, 1046–1058. doi:10.1016/j.celrep.2015.09.063
Han, B., Qu, Y., Yu-Rice, Y., Johnson, J., and Cui, X. (2016). FOXC1-induced Gli2 activation: A non-canonical pathway contributing to stemness and anti-hedgehog resistance in basal-like breast cancer. Mol. Cell. Oncol. 3, e1131668. doi:10.1080/23723556.2015.1131668
Harrison, H., Farnie, G., Brennan, K. R., and Clarke, R. B. (2010a). Breast cancer stem cells: Something out of notching? Cancer Res. 70, 8973–8976. doi:10.1158/0008-5472.CAN-10-1559
Harrison, H., Farnie, G., Howell, S. J., Rock, R. E., Stylianou, S., Brennan, K. R., et al. (2010b). Regulation of breast cancer stem cell activity by signaling through the Notch4 receptor. Cancer Res. 70, 709–718. doi:10.1158/0008-5472.CAN-09-1681
Hata, A., and Chen, Y.-G. (2016). TGF-Β signaling from receptors to smads. Cold Spring Harb. Perspect. Biol. 8, a022061. doi:10.1101/cshperspect.a022061
He, M., Fu, Y., Yan, Y., Xiao, Q., Wu, H., Yao, W., et al. (2015). The Hedgehog signalling pathway mediates drug response of MCF-7 mammosphere cells in breast cancer patients. Clin. Sci. 129, 809–822. doi:10.1042/CS20140592
Henry, C., Quadir, A., Hawkins, N. J., Jary, E., Llamosas, E., Kumar, D., et al. (2015). Expression of the novel Wnt receptor ROR2 is increased in breast cancer and may regulate both β-catenin dependent and independent Wnt signalling. J. Cancer Res. Clin. Oncol. 141, 243–254. doi:10.1007/s00432-014-1824-y
Hinohara, K., Kobayashi, S., Kanauchi, H., Shimizu, S., Nishioka, K., Tsuji, E. I., et al. (2012). ErbB receptor tyrosine kinase/NF-κB signaling controls mammosphere formation in human breast cancer. Proc. Natl. Acad. Sci. U. S. A. 109, 6584–6589. doi:10.1073/pnas.1113271109
Hou, M. F., Chen, P. M., and Chu, P. Y. (2018). LGR5 overexpression confers poor relapse-free survival in breast cancer patients. BMC Cancer 18, 219. doi:10.1186/s12885-018-4018-1
Islam, S. S., Al-Sharif, I., Sultan, A., Al-Mazrou, A., Remmal, A., and Aboussekhra, A. (2018). Eugenol potentiates cisplatin anti-cancer activity through inhibition of ALDH-positive breast cancer stem cells and the NF-κB signaling pathway. Mol. Carcinog. 57, 333–346. doi:10.1002/mc.22758
Iwanaga, R., Wang, C. A., Micalizzi, D. S., Harrell, J. C., Jedlicka, P., Sartorius, C. A., et al. (2012). Expression of Six1 in luminal breast cancers predicts poor prognosis and promotes increases in tumor initiating cells by activation of extracellular signal-regulated kinase and transforming growth factor-beta signaling pathways. Breast Cancer Res. 14, R100. doi:10.1186/bcr3219
Jalalirad, M., Haddad, T. C., Salisbury, J. L., Radisky, D., Zhang, M., Schroeder, M., et al. (2021). Aurora-A kinase oncogenic signaling mediates TGF-β-induced triple-negative breast cancer plasticity and chemoresistance. Oncogene 40, 2509–2523. doi:10.1038/s41388-021-01711-x
Jang, G. B., Hong, I. S., Kim, R. J., Lee, S. Y., Park, S. J., Lee, E. S., et al. (2015a). Wnt/β-catenin small-molecule inhibitor CWP232228 preferentially inhibits the growth of breast cancer stem-like cells. Cancer Res. 75, 1691–1702. doi:10.1158/0008-5472.CAN-14-2041
Jang, G. B., Kim, J. Y., Cho, S. D., Park, K. S., Jung, J. Y., Lee, H. Y., et al. (2015b). Blockade of Wnt/β-catenin signaling suppresses breast cancer metastasis by inhibiting CSC-like phenotype. Sci. Rep. 5, 12465. doi:10.1038/srep12465
Jeng, K. S., Jeng, C. J., Sheen, I. S., Wu, S. H., Lu, S. J., Wang, C. H., et al. (2018). Glioma-associated oncogene homolog inhibitors have the potential of suppressing cancer stem cells of breast cancer. Int. J. Mol. Sci. 19, 1375. doi:10.3390/ijms19051375
Jia, D., Yang, W., Li, L., Liu, H., Tan, Y., Ooi, S., et al. (2015). β-Catenin and NF-κB co-activation triggered by TLR3 stimulation facilitates stem cell-like phenotypes in breast cancer. Cell Death Differ. 22, 298–310. doi:10.1038/cdd.2014.145
Jiang, B., Zhu, H., Tang, L., Gao, T., Zhou, Y., Gong, F., et al. (2022). Apatinib inhibits stem properties and malignant biological behaviors of breast cancer stem cells by blocking wnt/β-catenin signal pathway through downregulating LncRNA ROR. Anticancer. Agents Med. Chem. 22, 1723–1734. doi:10.2174/1871520621666210412103849
Kang, L., Mao, J., Tao, Y., Song, B., Ma, W., Lu, Y., et al. (2015). MicroRNA-34a suppresses the breast cancer stem cell-like characteristics by downregulating Notch1 pathway. Cancer Sci. 106, 700–708. doi:10.1111/cas.12656
Karicheva, O., Rodriguez-Vargas, J. M., Wadier, N., Martin-Hernandez, K., Vauchelles, R., Magroun, N., et al. (2016). PARP3 controls TGFβ and ROS driven epithelial-to-mesenchymal transition and stemness by stimulating a TG2-Snail-E-cadherin axis. Oncotarget 7, 64109–64123. doi:10.18632/oncotarget.11627
Kasper, M., Jaks, V., Fiaschi, M., and Toftgård, R. (2009). Hedgehog signalling in breast cancer. Carcinogenesis 30, 903–911. doi:10.1093/carcin/bgp048
Kastrati, I., Delgado-Rivera, L., Georgieva, G., Thatcher, G. R. J., and Frasor, J. (2017). Synthesis and characterization of an aspirin-fumarate prodrug that inhibits NFκB activity and breast cancer stem cells. J. Vis. Exp. 2017, 54798. doi:10.3791/54798
Katoh, M. (2017). Canonical and non-canonical WNT signaling in cancer stem cells and their niches: Cellular heterogeneity, omics reprogramming, targeted therapy and tumor plasticity (Review). Int. J. Oncol. 51, 1357–1369. doi:10.3892/ijo.2017.4129
Katsuno, Y., Meyer, D. S., Zhang, Z., Shokat, K. M., Akhurst, R. J., Miyazono, K., et al. (2019). Chronic TGF-β exposure drives stabilized EMT, tumor stemness, and cancer drug resistance with vulnerability to bitopic mTOR inhibition. Sci. Signal. 12, eaau8544–36. doi:10.1126/scisignal.aau8544
Kendellen, M. F., Bradford, J. W., Lawrence, C. L., Clark, K. S., and Baldwin, A. S. (2014). Canonical and non-canonical NF-κB signaling promotes breast cancer tumor-initiating cells. Oncogene 33, 1297–1305. doi:10.1038/onc.2013.64
Kim, S. L., Choi, H. S., Kim, J. H., and Lee, D. S. (2020). The antiasthma medication ciclesonide suppresses breast cancer stem cells through inhibition of the glucocorticoid receptor signaling-dependent YAP pathway. Molecules 25, 6028. doi:10.3390/molecules25246028
Ko, Y. C., Choi, H. S., Liu, R., and Lee, D. S. (2021). Physalin a, 13,14-seco-16, 24-cyclo-steroid, inhibits stemness of breast cancer cells by regulation of hedgehog signaling pathway and yes-associated protein 1 (Yap1). Int. J. Mol. Sci. 22, 8718. doi:10.3390/ijms22168718
Kondratyev, M., Kreso, A., Hallett, R. M., Girgis-Gabardo, A., Barcelon, M. E., Ilieva, D., et al. (2012). Gamma-secretase inhibitors target tumor-initiating cells in a mouse model of ERBB2 breast cancer. Oncogene 31, 93–103. doi:10.1038/onc.2011.212
Kong, L., Guo, S., Liu, C., Zhao, Y., Feng, C., Liu, Y., et al. (2016). Overexpression of SDF-1 activates the NF-κB pathway to induce epithelial to mesenchymal transition and cancer stem cell-like phenotypes of breast cancer cells. Int. J. Oncol. 48, 1085–1094. doi:10.3892/ijo.2016.3343
Kong, Y., Ou, X., Li, X., Zeng, Y., Gao, G., Lyu, N., et al. (2020). LGR6 promotes tumor proliferation and metastasis through wnt/β-catenin signaling in triple-negative breast cancer. Mol. Ther. - Oncolytics 18, 351–359. doi:10.1016/j.omto.2020.06.020
Konge, J., Leteurtre, F., Goislard, M., Biard, D., Morel-Altmeyer, S., Vaurijoux, A., et al. (2018). Breast cancer stem cell-like cells generated during TGFβ-induced EMT are radioresistant. Oncotarget 9, 23519–23531. doi:10.18632/oncotarget.25240
Kontomanolis, E. N., Kalagasidou, S., Pouliliou, S., Anthoulaki, X., Georgiou, N., Papamanolis, V., et al. (2018). The notch pathway in breast cancer progression. Sci. World J. 2018, 2415489. doi:10.1155/2018/2415489
Kulkarni, M., Tan, T. Z., Sulaiman, N. B. S., Lamar, J. M., Bansal, P., Cui, J., et al. (2018). RUNX1 and RUNX3 protect against YAP-mediated EMT, stemness and shorter survival outcomes in breast cancer. Oncotarget 9, 14175–14192. doi:10.18632/oncotarget.24419
Kumar, S., Nandi, A., Singh, S., Regulapati, R., Li, N., Tobias, J. W., et al. (2021). Dll1+ quiescent tumor stem cells drive chemoresistance in breast cancer through NF-κB survival pathway. Nat. Commun. 12, 432. doi:10.1038/s41467-020-20664-5
Kundu, P., and Shankar, B. S. (2022). Macrophage induced ERK-TGF-β1 signaling in MCF7 breast cancer cells result in reversible cancer stem cell plasticity and epithelial mesenchymal transition. Biochim. Biophys. Acta - Gen. Subj. 1866, 130215. doi:10.1016/j.bbagen.2022.130215
Kurebayashi, J., Koike, Y., Ohta, Y., Saitoh, W., Yamashita, T., Kanomata, N., et al. (2017). Anti-cancer stem cell activity of a hedgehog inhibitor GANT61 in estrogen receptor-positive breast cancer cells. Cancer Sci. 108, 918–930. doi:10.1111/cas.13205
Lagadec, C., Vlashi, E., Alhiyari, Y., Phillips, T. M., Bochkur Dratver, M., and Pajonk, F. (2013). Radiation-induced notch signaling in breast cancer stem cells. Int. J. Radiat. Oncol. Biol. Phys. 87, 609–618. doi:10.1016/j.ijrobp.2013.06.2064
Li, B., Lu, Y., Yu, L., Han, X., Wang, H., Mao, J., et al. (2017). miR-221/222 promote cancer stem-like cell properties and tumor growth of breast cancer via targeting PTEN and sustained Akt/NF-κB/COX-2 activation. Chem. Biol. Interact. 277, 33–42. doi:10.1016/j.cbi.2017.08.014
Li, J., and Zhou, B. P. (2011). Activation of β-catenin and Akt pathways by Twist are critical for the maintenance of EMT associated cancer stem cell-like characters. BMC Cancer 11, 49. doi:10.1186/1471-2407-11-49
Li, S., Wu, H., Huang, X., Jian, Y., Kong, L., Xu, H., et al. (2021). BOP1 confers chemoresistance of triple-negative breast cancer by promoting CBP-mediated β-catenin acetylation. J. Pathol. 254, 265–278. doi:10.1002/path.5676
Li, X., Meng, Y., Xie, C., Zhu, J., Wang, X., Li, Y., et al. (2018a). Diallyl Trisulfide inhibits breast cancer stem cells via suppression of Wnt/β-catenin pathway. J. Cell. Biochem. 119, 4134–4141. doi:10.1002/jcb.26613
Li, X., Wang, X., Xie, C., Zhu, J., Meng, Y., Chen, Y., et al. (2018b). Sonic hedgehog and Wnt/β-catenin pathways mediate Curcumin inhibition of breast cancer stem cells. Anticancer. Drugs 29, 208–215. doi:10.1097/CAD.0000000000000584
Li, Y., Jiang, F., Chen, L., Yang, Y., Cao, S., Ye, Y., et al. (2015). Blockage of TGFβ-SMAD2 by demethylation-activated miR-148a is involved in caffeic acid-induced inhibition of cancer stem cell-like properties in vitro and in vivo. FEBS Open Bio 5, 466–475. doi:10.1016/j.fob.2015.05.009
Lim, J. R., Mouawad, J., Gorton, O. K., Bubb, W. A., and Kwan, A. H. (2021). Cancer stem cell characteristics and their potential as therapeutic targets. Med. Oncol. 38, 76. doi:10.1007/s12032-021-01524-8
Lin, C., Wang, L., Wang, H., Yang, L., Guo, H., and Wang, X. (2013). Tanshinone IIA inhibits breast cancer stem cells growth in vitro and in vivo through attenuation of IL-6/STAT3/NF-kB signaling pathways. J. Cell. Biochem. 114, 2061–2070. doi:10.1002/jcb.24553
Lindvall, C., Bu, W., Williams, B. O., and Li, Y. (2007). Wnt signaling, stem cells, and the cellular origin of breast cancer. Stem Cell Rev. 3, 157–168. doi:10.1007/s12015-007-0025-3
Liu, M., Sakamaki, T., Casimiro, M. C., Willmarth, N. E., Quong, A. A., Ju, X., et al. (2010). The canonical NF-kappaB pathway governs mammary tumorigenesis in transgenic mice and tumor stem cell expansion. Cancer Res. 70, 10464–10473. doi:10.1158/0008-5472.CAN-10-0732
Liu, R., Choi, H. S., Kim, S. L., Kim, J. H., Yun, B. S., and Lee, D. S. (2020a). 6-methoxymellein isolated from carrot (Daucus carota L) targets breast cancer stem cells by regulating NF-κB signaling. Molecules 25, 4374. doi:10.3390/molecules25194374
Liu, S., Dontu, G., Mantle, I. D., Patel, S., Ahn, N., Jackson, K. W., et al. (2006). Hedgehog signaling and bmi-1 regulate self-renewal of normal and malignant human mammary stem cells. Cancer Res. 66, 6063–6071. doi:10.1158/0008-5472.CAN-06-0054
Liu, W., Lu, X., Shi, P., Yang, G., Zhou, Z., Li, W., et al. (2020b). TNF-α increases breast cancer stem-like cells through up-regulating TAZ expression via the non-canonical NF-κB pathway. Sci. Rep. 10, 1804–1811. doi:10.1038/s41598-020-58642-y
Lo, P.-K., Kanojia, D., Liu, X., Singh, U. P., Berger, F. G., Wang, Q., et al. (2012). CD49f and CD61 identify Her2/neu-induced mammary tumor-initiating cells that are potentially derived from luminal progenitors and maintained by the integrin–TGFβ signaling. Oncogene 31, 2614–2626. doi:10.1038/onc.2011.439
Lu, Y., Ma, W., Mao, J., Yu, X., Hou, Z., Fan, S., et al. (2015). Salinomycin exerts anticancer effects on human breast carcinoma MCF-7 cancer stem cells via modulation of Hedgehog signaling. Chem. Biol. Interact. 228, 100–107. doi:10.1016/j.cbi.2014.12.002
Majumder, M., Xin, X., Liu, L., Tutunea-Fatan, E., Rodriguez-Torres, M., Vincent, K., et al. (2016). COX-2 induces breast cancer stem cells via EP4/PI3K/AKT/NOTCH/WNT Axis. Stem Cells 34, 2290–2305. doi:10.1002/stem.2426
Mak, K. K., Wu, A. T. H., Lee, W. H., Chang, T. C., Chiou, J. F., Wang, L. S., et al. (2013). Pterostilbene, a bioactive component of blueberries, suppresses the generation of breast cancer stem cells within tumor microenvironment and metastasis via modulating NF-κB/microRNA 448 circuit. Mol. Nutr. Food Res. 57, 1123–1134. doi:10.1002/mnfr.201200549
Mandal, S., Gamit, N., Varier, L., Dharmarajan, A., and Warrier, S. (2021). Inhibition of breast cancer stem-like cells by a triterpenoid, ursolic acid, via activation of Wnt antagonist, sFRP4 and suppression of miRNA-499a-5p. Life Sci. 265, 118854. doi:10.1016/j.lfs.2020.118854
Many, A. M., and Brown, A. M. C. (2014). Both canonical and non-canonical Wnt signaling independently promote stem cell growth in mammospheres. PLoS One 9, e101800–e101809. doi:10.1371/journal.pone.0101800
Maugeri-Saccà, M., and De Maria, R. (2016). Hippo pathway and breast cancer stem cells. Crit. Rev. Oncol. Hematol. 99, 115–122. doi:10.1016/j.critrevonc.2015.12.004
McGowan, P. M., Simedrea, C., Ribot, E. J., Foster, P. J., Palmieri, D., Steeg, P. S., et al. (2011). Notch1 inhibition alters the CD44hi/CD24lo population and reduces the formation of brain metastases from breast cancer. Mol. Cancer Res. 9, 834–844. doi:10.1158/1541-7786.MCR-10-0457
Memmi, E. M., Sanarico, A. G., Giacobbe, A., Peschiaroli, A., Frezza, V., Cicalese, A., et al. (2015). p63 sustains self-renewal of mammary cancer stem cells through regulation of Sonic Hedgehog signaling. Proc. Natl. Acad. Sci. U. S. A. 112, 3499–3504. doi:10.1073/pnas.1500762112
Miele, L., Golde, T., and Osborne, B. (2006). Notch signaling in cancer. Curr. Mol. Med. 6, 905–918. doi:10.2174/156652406779010830
Mikels, A. J., and Nusse, R. (2006). Purified Wnt5a protein activates or inhibits β-catenin–TCF signaling depending on receptor context. PLoS Biol. 4, e115. doi:10.1371/journal.pbio.0040115
Mishra, A. K., Parish, C. R., Wong, M. L., Licinio, J., and Blackburn, A. C. (2017). Leptin signals via TGFB1 to promote metastatic potential and stemness in breast cancer. PLoS One 12, e0178454. doi:10.1371/journal.pone.0178454
Nandy, S. B., Arumugam, A., Subramani, R., Pedroza, D., Hernandez, K., Saltzstein, E., et al. (2015). MicroRNA-125a influences breast cancer stem cells by targeting leukemia inhibitory factor receptor which regulates the hippo signaling pathway. Oncotarget 6, 17366–17378. doi:10.18632/oncotarget.3953
Nie, Z., Wang, C., Zhou, Z., Chen, C., Liu, R., and Wang, D. (2015). Transforming growth factor-beta increases breast cancer stem cell population partially through upregulating PMEPA1 expression. Acta Biochim. Biophys. Sin. (Shanghai). 48, 194–201. doi:10.1093/abbs/gmv130
Orlova, Z., Pruefer, F., Castro-Oropeza, R., Ordaz-Ramos, A., Zampedri, C., Maldonado, V., et al. (2019). IKKε regulates the breast cancer stem cell phenotype. Biochim. Biophys. Acta - Mol. Cell Res. 1866, 598–611. doi:10.1016/j.bbamcr.2019.01.002
Pal, D., Kolluru, V., Chandrasekaran, B., Baby, B. V., Aman, M., Suman, S., et al. (2017). Targeting aberrant expression of Notch-1 in ALDH+ cancer stem cells in breast cancer. Mol. Carcinog. 56, 1127–1136. doi:10.1002/mc.22579
Palafox, M., Ferrer, I., Pellegrini, P., Vila, S., Hernandez-Ortega, S., Urruticoechea, A., et al. (2012). RANK induces epithelial–mesenchymal transition and stemness in human mammary epithelial cells and promotes tumorigenesis and metastasis. Cancer Res. 72, 2879–2888. doi:10.1158/0008-5472.CAN-12-0044
Paramanantham, A., Kim, M. J., Jung, E. J., Kim, H. J., Chang, S. H., Jung, J. M., et al. (2020). Anthocyanins isolated from vitis coignetiae pulliat enhances cisplatin sensitivity in MCF-7 human breast cancer cells through inhibition of Akt and NF-κB activation. Molecules 25, 3623. doi:10.3390/molecules25163623
Park, S. Y., Kim, M. J., Park, S. A., Kim, J. S., Min, K. N., Kim, D. K., et al. (2015). Combinatorial TGF-β attenuation with paclitaxel inhibits the epithelial-to-mesenchymal transition and breast cancer stem-like cells. Oncotarget 6, 37526–37543. doi:10.18632/oncotarget.6063
Peng, G., Tian, Y., Lu, C., Guo, H., Zhao, X., Guo, Y., et al. (2014). Effects of notch-1 down-regulation on malignant behaviors of breast cancer stem cells. J. Huazhong Univ. Sci. Technol. Med. Sci. 34, 195–200. doi:10.1007/s11596-014-1258-4
Pfitzner, B. M., Branstetter, D., Loibl, S., Denkert, C., Lederer, B., Schmitt, W. D., et al. (2014). RANK expression as a prognostic and predictive marker in breast cancer. Breast Cancer Res. Treat. 145, 307–315. doi:10.1007/s10549-014-2955-1
Pratt, M. A. C., Tibbo, E., Robertson, S. J., Jansson, D., Hurst, K., Perez-Iratxeta, C., et al. (2009). The canonical NF-kappaB pathway is required for formation of luminal mammary neoplasias and is activated in the mammary progenitor population. Oncogene 28, 2710–2722. doi:10.1038/onc.2009.131
Qiu, M., Peng, Q., Jiang, I., Carroll, C., Han, G., Rymer, I., et al. (2013). Specific inhibition of Notch1 signaling enhances the antitumor efficacy of chemotherapy in triple negative breast cancer through reduction of cancer stem cells. Cancer Lett. 328, 261–270. doi:10.1016/j.canlet.2012.09.023
Qiu, T., Cao, J., Chen, W., Wang, J., Wang, Y., Zhao, L., et al. (2020). 24-Dehydrocholesterol reductase promotes the growth of breast cancer stem-like cells through the Hedgehog pathway. Cancer Sci. 111, 3653–3664. doi:10.1111/cas.14587
Ramamoorthy, P., Dandawate, P., Jensen, R. A., and Anant, S. (2021). Celastrol and triptolide suppress stemness in triple negative breast cancer: Notch as a therapeutic target for stem cells. Biomedicines 9, 482. doi:10.3390/biomedicines9050482
Renema, N., Navet, B., Heymann, M.-F., Lezot, F., and Heymann, D. (2016). RANK–RANKL signalling in cancer. Biosci. Rep. 36, e00366. doi:10.1042/BSR20160150
Riobo-Del Galdo, N. A., Montero, Á. L., and Wertheimer, E. V. (2019). Role of hedgehog signaling in breast cancer: Pathogenesis and therapeutics. Cells 8, 375. doi:10.3390/cells8040375
Rong, Z., Zhang, L., Li, Z., Xiao, Z., Duan, Y., Ren, X., et al. (2022). SIK2 maintains breast cancer stemness by phosphorylating LRP6 and activating Wnt/β-catenin signaling. Oncogene 41, 2390–2403. doi:10.1038/s41388-022-02259-0
Ruiz i Altaba, A., Mas, C., and Stecca, B. (2007). The Gli code: An information nexus regulating cell fate, stemness and cancer. Trends Cell Biol. 17, 438–447. doi:10.1016/j.tcb.2007.06.007
Saha, S., Mukherjee, S., Khan, P., Kajal, K., Mazumdar, M., Manna, A., et al. (2016). Aspirin suppresses the acquisition of chemoresistance in breast cancer by disrupting an NFκB-IL6 signaling axis responsible for the generation of cancer stem cells. Cancer Res. 76, 2000–2012. doi:10.1158/0008-5472.CAN-15-1360
Sakunrangsit, N., and Ketchart, W. (2019). Plumbagin inhibits cancer stem-like cells, angiogenesis and suppresses cell proliferation and invasion by targeting Wnt/β-catenin pathway in endocrine resistant breast cancer. Pharmacol. Res. 150, 104517. doi:10.1016/j.phrs.2019.104517
Séveno, C., Loussouarn, D., Bréchet, S., Campone, M., Juin, P., and Barillé-Nion, S. (2012). γ-Secretase inhibition promotes cell death, Noxa upregulation, and sensitization to BH3 mimetic ABT-737 in human breast cancer cells. Breast Cancer Res. 14, R96. doi:10.1186/bcr3214
Shamsian, A., Sepand, M. R., Kachousangi, M. J., Dara, T., Ostad, S. N., Atyabi, F., et al. (2020). Targeting tumorigenicity of breast cancer stem cells using saha/wnt-b catenin antagonist loaded onto protein corona of gold nanoparticles. Int. J. Nanomedicine 15, 4063–4078. doi:10.2147/IJN.S234636
Shi, P., Feng, J., and Chen, C. (2014). Hippo pathway in mammary gland development and breast cancer. Acta Biochim. Biophys. Sin. (Shanghai). 47, 53–59. doi:10.1093/abbs/gmu114
Siddharth, S., Goutam, K., Das, S., Nayak, A., Nayak, D., Sethy, C., et al. (2017). Nectin-4 is a breast cancer stem cell marker that induces WNT/β-catenin signaling via Pi3k/Akt axis. Int. J. Biochem. Cell Biol. 89, 85–94. doi:10.1016/j.biocel.2017.06.007
Simmons, M. J., Serra, R., Hermance, N., and Kelliher, M. A. (2012). NOTCH1 inhibition in vivo results in mammary tumor regression and reduced mammary tumorsphere-forming activity in vitro. Breast Cancer Res. 14, R126. doi:10.1186/bcr3321
Simões, B. M., O’Brien, C. S., Eyre, R., Silva, A., Yu, L., Sarmiento-Castro, A., et al. (2015). Anti-estrogen resistance in human breast tumors is driven by JAG1-NOTCH4-dependent cancer stem cell activity. Cell Rep. 12, 1968–1977. doi:10.1016/j.celrep.2015.08.050
Sims-Mourtada, J., Opdenaker, L. M., Davis, J., Arnold, K. M., and Flynn, D. (2015). Taxane-induced hedgehog signaling is linked to expansion of breast cancer stem-like populations after chemotherapy. Mol. Carcinog. 54, 1480–1493. doi:10.1002/mc.22225
Smith, S. M., and Cai, L. (2012). Cell specific CD44 expression in breast cancer requires the interaction of AP-1 and NFκB with a novel cis-element. PLoS One 7, e50867. doi:10.1371/journal.pone.0050867
Sulaiman, A., McGarry, S., Chilumula, S. C., Kandunuri, R., and Vinod, V. (2021). Clinically translatable approaches of inhibiting tgf-β to target cancer stem cells in tnbc. Biomedicines 9, 1386. doi:10.3390/biomedicines9101386
Sun, H. L., Men, J. R., Liu, H. Y., Liu, M. Y., and Zhang, H. S. (2020). FOXM1 facilitates breast cancer cell stemness and migration in YAP1-dependent manner. Arch. Biochem. Biophys. 685, 108349. doi:10.1016/j.abb.2020.108349
Sun, M., Zhang, N., Wang, X., Li, Y., Qi, W., Zhang, H., et al. (2016). Hedgehog pathway is involved in nitidine chloride induced inhibition of epithelial-mesenchymal transition and cancer stem cells-like properties in breast cancer cells. Cell Biosci. 6, 44–13. doi:10.1186/s13578-016-0104-8
Sun, X., He, Z., Guo, L., Wang, C., Lin, C., Ye, L., et al. (2021). ALG3 contributes to stemness and radioresistance through regulating glycosylation of TGF-β receptor II in breast cancer. J. Exp. Clin. Cancer Res. 40, 149. doi:10.1186/s13046-021-01932-8
Sun, Z., Zhang, C., Zou, X., Jiang, G., Xu, Z., Li, W., et al. (2015). Special AT-rich sequence-binding protein-1 participates in the maintenance of breast cancer stem cells through regulation of the Notch signaling pathway and expression of Snail1 and Twist1. Mol. Med. Rep. 11, 3235–3542. doi:10.3892/mmr.2015.3192
Suyama, K., Onishi, H., Imaizumi, A., Shinkai, K., Umebayashi, M., Kubo, M., et al. (2016). CD24 suppresses malignant phenotype by downregulation of SHH transcription through STAT1 inhibition in breast cancer cells. Cancer Lett. 374, 44–53. doi:10.1016/j.canlet.2015.12.013
Tanaka, H., Nakamura, M., Kameda, C., Kubo, M., Sato, N., Kuroki, S., et al. (2009). The Hedgehog signaling pathway plays an essential role in maintaining the CD44+CD24-/low subpopulation and the side population of breast cancer cells. Anticancer Res. 29, 2147–2157.
Tang, T., Guo, C., Xia, T., Zhang, R., Zen, K., Pan, Y., et al. (2019). LncCCAT1 promotes breast cancer stem cell function through activating WNT/β-catenin signaling. Theranostics 9, 7384–7402. doi:10.7150/thno.37892
Tang, W., Li, M., Qi, X., and Li, J. (2020). β1,4-Galactosyltransferase V modulates breast cancer stem cells through Wnt/β-catenin signaling pathway. Cancer Res. Treat. 52, 1084–1102. doi:10.4143/crt.2020.093
Ter Steege, E. J., and Bakker, E. R. M. (2021). The role of R-spondin proteins in cancer biology. Oncogene 40, 6469–6478. doi:10.1038/s41388-021-02059-y
Tian, J., Hachim, M. Y., Hachim, I. Y., Dai, M., Lo, C., Raffa, F. A, et al. (2017). Cyclooxygenase-2 regulates TGFβ-induced cancer stemness in triple-negative breast cancer. Sci. Rep. 7, 40258. doi:10.1038/srep40258
Tsubakihara, Y., Ohata, Y., Okita, Y., Younis, S., Eriksson, J., Sellin, M. E., et al. (2022). TGFβ selects for pro-stemness over pro-invasive phenotypes during cancer cell epithelial–mesenchymal transition. Mol. Oncol. 16, 2330–2354. doi:10.1002/1878-0261.13215
Tzavlaki, K., and Moustakas, A. (2020). TGF-Β signaling. Biomolecules 10, 487. doi:10.3390/biom10030487
van Amerongen, R., Mikels, A., and Nusse, R. (2008). Alternative wnt signaling is initiated by distinct receptors. Sci. Signal. 1, re9. doi:10.1126/scisignal.135re9
Vazquez-Santillan, K., Melendez-Zajgla, J., Jimenez-Hernandez, L. E., Gaytan-Cervantes, J., Muñoz-Galindo, L., Piña-Sanchez, P., et al. (2016). NF-kappaΒ-inducing kinase regulates stem cell phenotype in breast cancer. Sci. Rep. 6, 37340. doi:10.1038/srep37340
Vazquez-Santillan, K., Melendez-Zajgla, J., Jimenez-Hernandez, L., Martínez-Ruiz, G., and Maldonado, V. (2015). NF-κB signaling in cancer stem cells: A promising therapeutic target? Cell. Oncol. 38, 327–339. doi:10.1007/s13402-015-0236-6
Wang, B., Ye, Q., and Zou, C. (2020). Long non-coding rna thor enhances the stem cell-like traits of triple-negative breast cancer cells through activating β-catenin signaling. Med. Sci. Monit. 26, e923507–e923509. doi:10.12659/MSM.923507
Wang, L., Duan, W., Kang, L., Mao, J., Yu, X., Fan, S., et al. (2014a). Smoothened activates breast cancer stem-like cell and promotes tumorigenesis and metastasis of breast cancer. Biomed. Pharmacother. 68, 1099–1104. doi:10.1016/j.biopha.2014.09.012
Wang, L., Jin, Z., Master, R. P., Maharjan, C. K., Carelock, M. E., Reccoppa, T. B. A., et al. (2022). Breast cancer stem cells: Signaling pathways, cellular interactions, and therapeutic implications. Cancers (Basel). 14, 3287. doi:10.3390/cancers14133287
Wang, L., Tian, H., Yuan, J., Wu, H., Wu, J., and Zhu, X. (2015). Consort: Sam68 is directly regulated by MiR-204 and promotes the self-renewal potential of breast cancer cells by activating the wnt/beta-catenin signaling pathway. Med. (United States) 94, e2228. doi:10.1097/MD.0000000000002228
Wang, X., Zhang, N., Huo, Q., Sun, M., Dong, L., Zhang, Y., et al. (2014b). Huaier aqueous extract inhibits stem-like characteristics of MCF7 breast cancer cells via inactivation of hedgehog pathway. Tumor Biol. 35, 10805–10813. doi:10.1007/s13277-014-2390-2
Wang, Z., Li, B., Zhou, L., Yu, S., Su, Z., Song, J., et al. (2016). Prodigiosin inhibits Wnt/β-catenin signaling and exerts anticancer activity in breast cancer cells. Proc. Natl. Acad. Sci. U. S. A. 113, 13150–13155. doi:10.1073/pnas.1616336113
Wei, L., Liu, T. T., Wang, H. H., Hong, H. M., Yu, A. L., Feng, H. P., et al. (2011). Hsp27 participates in the maintenance of breast cancer stem cells through regulation of epithelial-mesenchymal transition and nuclear factor-ΚB. Breast Cancer Res. 13, R101–R113. doi:10.1186/bcr3042
Won, H. Y., Lee, J. Y., Shin, D. H., Park, J. H., Nam, J. S., Kim, H. C., et al. (2012). Loss of Mel-18 enhances breast cancer stem cell activity and tumorigenicity through activating Notch signaling mediated by the Wnt/TCF pathway. FASEB J. 26, 5002–5013. doi:10.1096/fj.12-209247
Wong, N. K. Y., Fuller, M., Sung, S., Wong, F., and Karsan, A. (2012). Heterogeneity of breast cancer stem cells as evidenced with Notch-dependent and Notch-independent populations. Cancer Med. 1, 105–113. doi:10.1002/cam4.18
Woosley, A. N., Dalton, A. C., Hussey, G. S., Howley, B. V., Mohanty, B. K., Grelet, S., et al. (2019). TGFβ promotes breast cancer stem cell self-renewal through an ILEI/LIFR signaling axis. Oncogene 38, 3794–3811. doi:10.1038/s41388-019-0703-z
Wu, F., Stutzman, A., and Mo, Y. Y. (2007). Notch signaling and its role in breast cancer. Front. Biosci. 12, 4370–4383. doi:10.2741/2394
Wu, Z., and Guan, K.-L. (2021). Hippo signaling in embryogenesis and development. Trends biochem. Sci. 46, 51–63. doi:10.1016/j.tibs.2020.08.008
Xie, W., Zhao, H., Wang, F., Wang, Y., He, Y., Wang, T., et al. (2021). A novel humanized Frizzled-7-targeting antibody enhances antitumor effects of Bevacizumab against triple-negative breast cancer via blocking Wnt/β-catenin signaling pathway. J. Exp. Clin. Cancer Res. 40, 30–18. doi:10.1186/s13046-020-01800-x
Xing, F., Okuda, H., Watabe, M., Kobayashi, A., Pai, S. K., Liu, W., et al. (2011). Hypoxia-induced Jagged2 promotes breast cancer metastasis and self-renewal of cancer stem-like cells. Oncogene 30, 4075–4086. doi:10.1038/onc.2011.122
Xu, C., Sun, X., Qin, S., Wang, H., Zheng, Z., Xu, S., et al. (2015). Let-7a regulates mammosphere formation capacity through Ras/NF-κB and Ras/MAPK/ERK pathway in breast cancer stem cells. Cell Cycle 14, 1686–1697. doi:10.1080/15384101.2015.1030547
Xu, X., Zhang, L., He, X., Zhang, P., Sun, C., Xu, X., et al. (2018). TGF-β plays a vital role in triple-negative breast cancer (TNBC) drug-resistance through regulating stemness, EMT and apoptosis. Biochem. Biophys. Res. Commun. 502, 160–165. doi:10.1016/j.bbrc.2018.05.139
Xu, X., Zhang, M., Xu, F., and Jiang, S. (2020). Wnt signaling in breast cancer: Biological mechanisms, challenges and opportunities. Mol. Cancer 19, 165. doi:10.1186/s12943-020-01276-5
Yadav, P., and Shankar, B. S. (2019). Radio resistance in breast cancer cells is mediated through TGF-β signalling, hybrid epithelial-mesenchymal phenotype and cancer stem cells. Biomed. Pharmacother. 111, 119–130. doi:10.1016/j.biopha.2018.12.055
Yamaguchi, N., Nakayama, Y., and Yamaguchi, N. (2017). Down-regulation of Forkhead box protein A1 (FOXA1) leads to cancer stem cell-like properties in tamoxifen-resistant breast cancer cells through induction of interleukin-6. J. Biol. Chem. 292, 8136–8148. doi:10.1074/jbc.M116.763276
Yamamoto, M., Taguchi, Y., Ito-Kureha, T., Semba, K., Yamaguchi, N., and Inoue, J. I. (2013). NF-κB non-cell-autonomously regulates cancer stem cell populations in the basal-like breast cancer subtype. Nat. Commun. 4, 2299. doi:10.1038/ncomms3299
Yan, X., He, Y., Yang, S., Zeng, T., Hua, Y., Bao, S., et al. (2022). A positive feedback loop: RAD18-YAP-TGF-β between triple-negative breast cancer and macrophages regulates cancer stemness and progression. Cell Death Discov. 8, 196. doi:10.1038/s41420-022-00968-9
Yan, Y., Liu, F., Han, L., Zhao, L., Chen, J., Olopade, O. I., et al. (2018). HIF-2α promotes conversion to a stem cell phenotype and induces chemoresistance in breast cancer cells by activating Wnt and Notch pathways. J. Exp. Clin. Cancer Res. 37, 256. doi:10.1186/s13046-018-0925-x
Yang, C. E., Lee, W. Y., Cheng, H. W., Chung, C. H., Mi, F. L., and Lin, C. W. (2019). The antipsychotic chlorpromazine suppresses YAP signaling, stemness properties, and drug resistance in breast cancer cells. Chem. Biol. Interact. 302, 28–35. doi:10.1016/j.cbi.2019.01.033
Yang, L., Xie, X., Tang, H., Kong, Y., Xie, X., Chen, J., et al. (2015). LGR5 promotes breast cancer progression and maintains stem-like cells through activation of wnt/β-catenin signaling. Stem Cells 33, 2913–2924. doi:10.1002/stem.2083
Yang, N., Zhou, T. C., Lei, X. X., Wang, C., Yan, M., Wang, Z. F., et al. (2016). Inhibition of sonic hedgehog signaling pathway by Thiazole Antibiotic Thiostrepton Attenuates the CD44+/CD24-stem-like population and sphere-forming capacity in triple-negative breast cancer. Cell. Physiol. biochem. 38, 1157–1170. doi:10.1159/000443066
Ye, G., Pan, R., Zhu, L., and Zhou, D. (2020). Circ_DCAF6 potentiates cell stemness and growth in breast cancer through GLI1-Hedgehog pathway. Exp. Mol. Pathol. 116, 104492. doi:10.1016/j.yexmp.2020.104492
Yeo, S. K., Wen, J., Chen, S., and Guan, J.-L. (2016). Autophagy differentially regulates distinct breast cancer stem-like cells in murine models via EGFR/Stat3 and tgfβ/smad signaling. Cancer Res. 76, 3397–3410. doi:10.1158/0008-5472.CAN-15-2946
Yin, P., Wang, W., Gao, J., Bai, Y., Wang, Z., Na, L., et al. (2020). Fzd2 contributes to breast cancer cell mesenchymal-like stemness and drug resistance. Oncol. Res. 28, 273–284. doi:10.3727/096504020X15783052025051
Yip, N. C., Fombon, I. S., Liu, P., Brown, S., Kannappan, V., Armesilla, A. L., et al. (2011). Disulfiram modulated ROS-MAPK and NFB pathways and targeted breast cancer cells with cancer stem cell-like properties. Br. J. Cancer 104, 1564–1574. doi:10.1038/bjc.2011.126
Yoldi, G., Pellegrini, P., Trinidad, E. M., Cordero, A., Gomez-Miragaya, J., Serra-Musach, J., et al. (2016). RANK signaling blockade reduces breast cancer recurrence by inducing tumor cell differentiation. Cancer Res. 76, 5857–5869. doi:10.1158/0008-5472.CAN-15-2745
Yu, J., Luo, Y., and Wen, Q. (2019). Nalbuphine suppresses breast cancer stem-like properties and epithelial-mesenchymal transition via the AKT-NFκB signaling pathway. J. Exp. Clin. Cancer Res. 38, 197. doi:10.1186/s13046-019-1184-1
Yue, Z., Yuan, Z., Zeng, L., Wang, Y., Lai, L., Li, J., et al. (2018). LGR4 modulates breast cancer initiation, metastasis, and cancer stem cells. FASEB J. 32, 2422–2437. doi:10.1096/fj.201700897R
Zakharchenko, O., Cojoc, M., Dubrovska, A., and Souchelnytskyi, S. (2013). A role of TGFß1 dependent 14-3-3σ phosphorylation at Ser69 and Ser74 in the regulation of gene transcription, stemness and radioresistance. PLoS One 8, e65163. doi:10.1371/journal.pone.0065163
Zhang, C., Gao, H., Li, C., Tu, J., Chen, Z., Su, W., et al. (2018). Tgfβ1 promotes breast cancer local invasion and liver metastasis by increasing the CD44high/CD24-subpopulation. Technol. Cancer Res. Treat. 17, 1533033818764497–11. doi:10.1177/1533033818764497
Zhang, H., Lang, T. Y., Zou, D. L., Zhou, L., Lou, M., Liu, J. S., et al. (2019). MiR-520b promotes breast cancer stemness through hippo/YAP signaling pathway. Onco. Targets. Ther. 12, 11691–11700. doi:10.2147/OTT.S236607
Zhang, T., Zhou, H., Wang, K., Wang, X., Wang, M., Zhao, W., et al. (2022). Role, molecular mechanism and the potential target of breast cancer stem cells in breast cancer development. Biomed. Pharmacother. 147, 112616. doi:10.1016/j.biopha.2022.112616
Zhang, W., Tan, W., Wu, X., Poustovoitov, M., Strasner, A., Li, W., et al. (2013). A NIK-IKKα module expands ErbB2-induced tumor-initiating cells by stimulating nuclear export of p27/kip1. Cancer Cell 23, 647–659. doi:10.1016/j.ccr.2013.03.012
Zhang, Y. E. (2017). Non-smad signaling pathways of the TGF-β family. Cold Spring Harb. Perspect. Biol. 9, a022129. doi:10.1101/cshperspect.a022129
Zhang, Y., Piao, B., Zhang, Y., Hua, B., Hou, W., Xu, W., et al. (2011). Oxymatrine diminishes the side population and inhibits the expression of β-catenin in MCF-7 breast cancer cells. Med. Oncol. 28, S99–107. doi:10.1007/s12032-010-9721-y
Zhao, D., Mo, Y., Li, M. T., Zou, S. W., Cheng, Z. L., Sun, Y. P., et al. (2014a). NOTCH-induced aldehyde dehydrogenase 1A1 deacetylation promotes breast cancer stem cells. J. Clin. Invest. 124, 5453–5465. doi:10.1172/JCI76611
Zhao, H., Tang, H., Xiao, Q., He, M., Zhao, L., Fu, Y., et al. (2016). The Hedgehog signaling pathway is associated with poor prognosis in breast cancer patients with the CD44+/CD24-phenotype. Mol. Med. Rep. 14, 5261–5270. doi:10.3892/mmr.2016.5856
Zhao, Z., Lu, P., Zhang, H., Xu, H., Gao, N., Li, M., et al. (2014b). Nestin positively regulates the Wnt/β-catenin pathway and the proliferation, survival and invasiveness of breast cancer stem cells. Breast Cancer Res. 16, 408–12. doi:10.1186/s13058-014-0408-8
Zhen, X., Choi, H. S., Kim, J. H., Kim, S. L., Liu, R., Yun, B. S., et al. (2020). Machilin D, a lignin derived from Saururus chinensis, suppresses breast cancer stem cells and inhibits NF-κB signaling. Biomolecules 10, 245–16. doi:10.3390/biom10020245
Zheng, A., Song, X., Zhang, L., Zhao, L., Mao, X., Wei, M., et al. (2019). Long non-coding RNA LUCAT1/miR-5582-3p/TCF7L2 axis regulates breast cancer stemness via Wnt/β-catenin pathway. J. Exp. Clin. Cancer Res. 38, 305–14. doi:10.1186/s13046-019-1315-8
Zheng, R., Wang, J., Wu, Q., Wang, Z., Ou, Y., Ma, L., et al. (2014). Expression of ALDH1 and TGFβ2 in benign and malignant breast tumors and their prognostic implications. Int. J. Clin. Exp. Pathol. 7, 4173–4183.
Zhou, J., Zhang, H., Gu, P., Bai, J., Margolick, J. B., and Zhang, Y. (2008). NF-kappaB pathway inhibitors preferentially inhibit breast cancer stem-like cells. Breast Cancer Res. Treat. 111, 419–427. doi:10.1007/s10549-007-9798-y
Zhu, L., Pan, R., Zhou, D., Ye, G., and Tan, W. (2019a). BCL11A enhances stemness and promotes progression by activating Wnt/β-catenin signaling in breast cancer. Cancer Manag. Res. 11, 2997–3007. doi:10.2147/CMAR.S199368
Zhu, R., Gires, O., Zhu, L., Liu, J., Li, J., Yang, H., et al. (2019b). TSPAN8 promotes cancer cell stemness via activation of sonic Hedgehog signaling. Nat. Commun. 10, 2863. doi:10.1038/s41467-019-10739-3
Zhu, T., Zheng, J., Zhuo, W., Pan, P., Li, M., Zhang, W., et al. (2021). ETV4 promotes breast cancer cell stemness by activating glycolysis and CXCR4-mediated sonic Hedgehog signaling. Cell Death Discov. 7, 126. doi:10.1038/s41420-021-00508-x
Keywords: cancer stem cells, pathways, self-renewal, breast cancer, therapy
Citation: Ordaz-Ramos A, Tellez-Jimenez O and Vazquez-Santillan K (2023) Signaling pathways governing the maintenance of breast cancer stem cells and their therapeutic implications. Front. Cell Dev. Biol. 11:1221175. doi: 10.3389/fcell.2023.1221175
Received: 11 May 2023; Accepted: 28 June 2023;
Published: 10 July 2023.
Edited by:
Gloria Bonuccelli, University of Salford, United KingdomReviewed by:
Alejandro Urtreger, University of Buenos Aires, ArgentinaCopyright © 2023 Ordaz-Ramos, Tellez-Jimenez and Vazquez-Santillan. This is an open-access article distributed under the terms of the Creative Commons Attribution License (CC BY). The use, distribution or reproduction in other forums is permitted, provided the original author(s) and the copyright owner(s) are credited and that the original publication in this journal is cited, in accordance with accepted academic practice. No use, distribution or reproduction is permitted which does not comply with these terms.
*Correspondence: Karla Vazquez-Santillan, a2l2YXpxdWV6QGlubWVnZW4uZ29iLm14
Disclaimer: All claims expressed in this article are solely those of the authors and do not necessarily represent those of their affiliated organizations, or those of the publisher, the editors and the reviewers. Any product that may be evaluated in this article or claim that may be made by its manufacturer is not guaranteed or endorsed by the publisher.
Research integrity at Frontiers
Learn more about the work of our research integrity team to safeguard the quality of each article we publish.