- 1Department of Rehabilitation Medicine and Institute of Rehabilitation Medicine, West China Hospital, Sichuan University, Chengdu, Sichuan, China
- 2Key Laboratory of Rehabilitation Medicine in Sichuan Province, Chengdu, Sichuan, China
- 3Department of Rehabilitation, Chengdu Fifth People’s Hospital, Chengdu, Sichuan, China
- 4National Clinical Research Center for Geriatrics, West China Hospital, Sichuan University, Chengdu, Sichuan, China
- 5Aging and Geriatric Mechanism Laboratory, West China Hospital, Sichuan University, Chengdu, Sichuan, China
The treatment of cardiovascular and cerebrovascular diseases have undergone major advances in recent decades, allowing for a more effective prevention of cardiovascular and cerebrovascular events. However, cardiac and cerebral atherothrombotic complications still account for substantial morbidity and mortality worldwide. Novel therapeutic strategies are critical to improve patient outcomes following cardiovascular diseases. miRNAs are small non-coding RNAs, that regulate gene expression. Here, we discuss the role of miR-182 in regulating myocardial proliferation, migration, hypoxia, ischemia, apoptosis and hypertrophy in atherosclerosis, CAD, MI, I/R injury, organ transplant, cardiac hypertrophy, hypertension, heart failure, congenital heart disease and cardiotoxicity. Besides, we also summarize the current progress of miR-182 therapeutics in clinical development and discuss challenges that will need to be overcome to enter the clinic for patients with cardiac disease.
Introduction
An estimated 17.9 million people died from cardiovascular diseases (CVDs) in 2019, accounting for 32% of all global deaths. Among these deaths, 85% were due to heart attack and stroke (WHO, 2019). CVDs are disorders of the heart and blood vessels including coronary heart diseases, cerebrovascular diseases and other conditions. Early detection of CVDs is crucial to reduce the mortality rate associated with cardiovascular diseases. Despite the fact that early revascularization therapy and drug treatment promote cardiac repair after ischemia and improve the survival rate of individuals with myocardial infarction (MI), some patients still experience heart failure (HF) (Parikh et al., 2009). Identifying factors for more effective prevention and early diagnosis remains a challenge. The mechanism and risk factors that intervene in disease occurrence and development need to be better explored.
miRNAs are single stranded, small (approx. 22 nucleotides), and endogenous non-coding RNA (ncRNA) molecules (Kim et al., 2009), which bind to the 3′-UTR of target messenger RNAs (mRNAs) causing the suppression of gene expression at both the mRNA and translational levels (Bartel, 2004). The identification of miRNAs has opened a new window to an important area of biology that was previously unexplored, but it also has important implications in human development and disease. miRNAs are active participants in a broad array of physiological homeostasis and pathological statuses (Saliminejad et al., 2019) (Rupaimoole and Slack, 2017). It has been estimated that miRNAs may be involved in the regulation of 30% of the protein-coding genes in the human genome (Sasso et al., 2022). miRNA-based regulation plays an important role in some crucial biological and cellular processes, such as differentiation, development, proliferation, apoptosis, metabolism and signal transduction pathways (Osman, 2012). However, a single miRNA can bind to hundreds of target mRNAs depending on target specificity and individual mRNAs can also be regulated by many different miRNAs (Bartel, 2004) (Fabian et al., 2010). Thus, their mechanism of action varies depending on the type of organs and cells, and sometimes even depending on the different stages of the disease (Winbanks et al., 2014) (Hata, 2013) (McClelland and Kantharidis, 2014). The multifunctionality of miRNAs has been extensively investigated in a range of diseases, including cardiovascular and cerebrovascular disorders (Beermann et al., 2016) (Lee and Dutta, 2009) (Ono et al., 2011).
miRNAs can be grouped into families based on sequence conservation within the seed region (nucleotides 2–8) but may on different chromosomes (Bernardo et al., 2012). microRNA-182 (miR-182) is a miRNA that belongs to the miR-183/96/182 cluster and highly conserved in vertebrates. They are located within genes positioned within a short distance (∼10 kb) on human chromosome 7q31-34 and mouse chromosome 6q A3, which are generated from a single primary transcript and have similar expression patterns (Xu et al., 2007). miR-182 is composed of two homologs miR-182-3p and miR-182-5p. It is highly expressed in mouse retina and inner ear hair cells, which has been identified in various cells such as osteoblasts, lymphocytes, and adipocyte (Stittrich et al., 2010) (Kim et al., 2012) (Friedman et al., 2009) (Dong et al., 2020). Currently, miR-182 is closely associated with the normal differentiation of sensory organs, the immune system, lymphatic vessels, bone, memory formation, the development of tumors, autoimmune diseases, depression, and metabolic diseases (Friedman et al., 2009; Dai et al., 2010; Saus et al., 2010; Stittrich et al., 2010; Kim et al., 2012; Yu et al., 2012; Griggs et al., 2013; Zhang et al., 2013; Kiesow et al., 2015). And particularly, upregulated blood levels of miR-182 have been shown to be associated with prognosis in patients with chronic heart failure (Cakmak et al., 2015). New nano system which can efficiently deliver inhibitor of miR-182 into hearts can significantly suppress cardiac hypertrophy (Zhi et al., 2019). Additionally, the regulatory connections of ncRNAs, represented by miRNAs, long non-coding RNAs (lncRNAs), and circular RNAs (circRNAs), have been widely demonstrated to be involved in the regulation of gene expression and to affect multiple biological processes in cardiovascular and cerebrovascular diseases. Some ncRNAs, such as lncRNA SNHG16 (Wang et al., 2020), lncRNA UCA1 (Chen et al., 2022), circRNA_0008028 (Shi et al., 2022), and circ_002664 (Liu et al., 2020), have been reported to act as significant regulators in cardiovascular and cerebrovascular diseases by targeting miR-182. Some recent studies have also described the role of miR-182 in cardiovascular and cerebrovascular diseases (see Table 1). Therefore, this review primarily focuses on miR-182 to discuss its role in cardiovascular and cerebrovascular diseases, and to explore the possibility of miR-182 as a potential diagnostic and therapeutic target for clinical treatment (Figure 1).
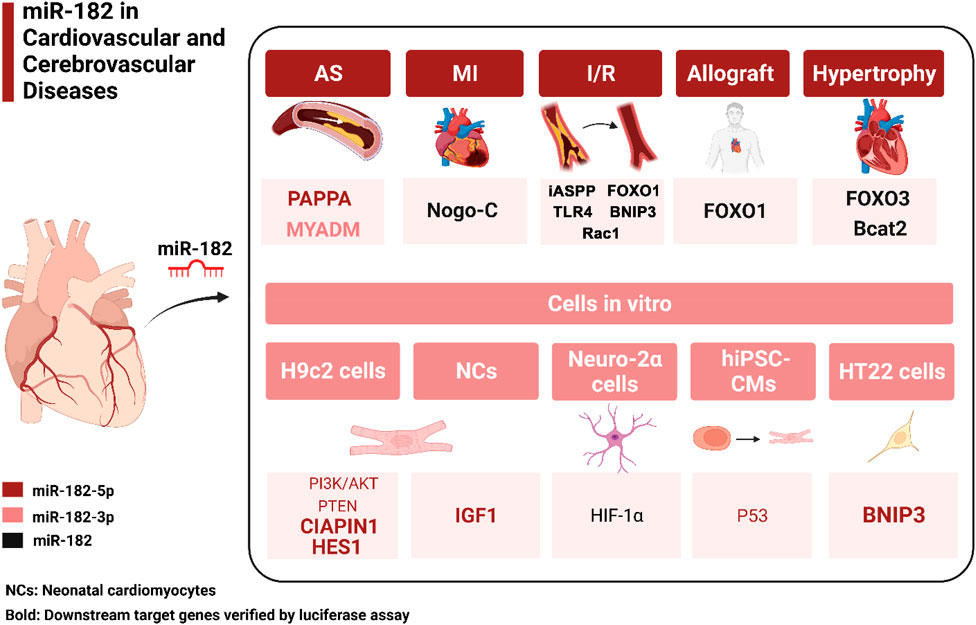
FIGURE 1. The target genes of miR-182 in different pathogenesis of cardiovascular and cerebrovascular diseases. Red, pink and black of downstream target genes represent the known miRNA-mRNA associations from miR-182-5p, miR-182-3p and miR-182, respectively. Bold indicates downstream target genes verified by luciferase assay. AS, atherosclerosis; MI, myocardial infarction; I/R, ischemia/reperfusion; PAPPA, pregnancy associated plasma protein A; MYADM, myeloid-associated differentiation marker; BNIP3, BCL2 Interacting Protein three; TLR4,Toll-like receptor four; FOXO1/3, forkhead box protein O1/3, CIAPIN1, cytokine-induced apoptosis inhibitor one; HES1, hairy and enhancer of split-1; iASPP: inhibitory member of the ASPP family; IGF1, insulin-like growth factor 1; HIF-1α, hypoxia-inducible factor; NCs, neonatal cardiomyocytes; hiPSC-CMs, human-induced pluripotent stem cell-derived cardiomyocytes; HT22, mouse hippocampal neuronal cells.
miR-182 in atherosclerosis and coronary artery disease
Coronary artery disease (CAD) caused by atherosclerosis (AS) is a leading cause of death and morbidity worldwide (Knuuti et al., 2019) (Frostegård, 2013). The proliferation, apoptosis, migration, and differentiation of vascular smooth muscle cells (VSMCs) play crucial roles in the pathogenesis of AS (Bennett et al., 2016). The role of oxidized low-density lipoprotein (ox-LDL) in the formation of foam cells derived from VSMCs, which act as one of the main risk factors for AS, has been extensively studied (Kattoor et al., 2019). In addition, asymmetrical dimethylarginine (ADMA), an endogenous inhibitor of nitric oxide synthase, is also directly affects VSMCs by influencing migration, apoptosis, and proliferation (Zhou et al., 2014). Studies have shown that miRNAs are involved in the detailed mechanism of how ox-LDL/ADMA induce VSMC phenotype change (Jin et al., 2020) (Sun et al., 2016). Of these, some clinical study have shown that dysregulation of circulating miR-182/miR-182–5p in patients with CAD or unprotected left main CAD by microarray profiling (Taurino et al., 2010) (Zhu et al., 2019). The expression of miR-182–5p was lower in the plasma of coronary atherosclerosis patients than in that of the healthy population. This approach may potentially provide a novel non-invasive diagnostic information and possible therapeutic targets in cardiovascular disease. miR-182–5p has been shown to inhibit the proliferation of vascular smooth muscle cells induced by ox-LDL by targeting pregnancy associated plasma protein A (PAPPA), further activating the NF-kB, PI3K/AKT and ERK signaling pathways (Jin et al., 2020). Furthermore, miR-182-3p was downregulated in ADMD-treated human aortic artery smooth muscle cells (SMCs) both in vitro and in vivo, and contributed to SMCs phenotype change by activating the ERK/MAP signaling pathway through upregulation of its direct target gene, myeloid-associated differentiation marker (MYADM) (Sun et al., 2016). Recently, researchers from the Peking Union Medical College have also described that the miR-182-3p/MYADM axis either inhibits pulmonary artery SMC proliferation via a KLF4/p21-dependent mechanism, or balances the BMP- and TGF-β signaling pathways in an SMC/EC-crosstalk-associated manner in pulmonary hypertension (Sun et al., 2020) (Bai et al., 2021). The ability of miR-182 in regulating multiple genes in signaling pathways makes it an attractive therapeutic target in AS and CAD.
miR-182 in myocardial infarction and ischemia‒reperfusion injury
Acute myocardial infarction (AMI) and subsequent ischemia/reperfusion (I/R) injury are major causes of death and disability worldwide (Yellon and Hausenloy, 2007). Timely and effective myocardial reperfusion using either thrombolytic therapy or percutaneous coronary intervention (PCI) is an efficacious treatment in clinical practice (Neri et al., 2017). However, the process of reperfusion can induce myocardial reperfusion injury, which accounts for half of the final injury in AMI and contributes to many clinical complications (Moens et al., 2005) (Hausenloy et al., 2013). Currently, the exact pathophysiological mechanisms of I/R injury are not fully known (Simonis et al., 2012). Recently, a large experimental of evidence suggests that miRNAs are promising candidates to modulate the molecular and cellular processes involved in MI and I/R, including miR-182. Several groups have reported miR-182 targets implicated in cardiac apoptosis, including Nogo-C (neurite outgrowth inhibitor protein C) and CIAPIN1 (cytokine-induced apoptosis inhibitor 1). miR-182 levels were increased in the OGD/R (oxygen and glucose deprivation followed by reperfusion) model of H9c2 cells (Ren et al., 2019), but decreased by activating Nogo-C-mediated cell apoptosis (Jia et al., 2016). By targeting CIAPIN1, inhibition of miR-182–5p could also protect H9C2 cells from hypoxia-induced apoptosis (Zhang et al., 2018b). In addition, miR-182–5p has been shown to involved in the protective effects of ganoderic acid A (GAA) on H9c2 cells proliferation and apoptosis via regulating the mRNA and protein levels of PTEN and the PI3K/AKT signaling pathway (Zhang X. et al., 2018). In particular, transplantation of mesenchymal stromal cells (MSCs) or their exosomes has gradually become an attractive candidate for cardiac inflammation modulation. miR-182 could act as a master regulator of macrophage polarization via targeting TLR4 for a novel mechanism of the beneficial effect of MSCs-derived exosome transplantation after MI (Zhao et al., 2019).
In addition to MI, I/R injury is also associated with a broad array of life-threatening medical conditions including organ transplant. Recent studies have observed that I/R injury causes dysregulation of miRNAs during organ transplants. Rancan et al. showed that the expression of miR-182 significantly increased and contribute to pulmonary artery hypertension vascular remodeling via targeting MYADM to regulate KLF4/p21 signaling pathway after I/R in pigs with lung auto-transplantation (Rancan et al., 2016). miR-182 has also been shown to regulate hepatic ischemia‒reperfusion injury by activating SIRT1 through downregulation of its direct target gene, XBP1 (Li et al., 2021). A monocentric retrospective study of 38 patients with the anamnesis of acute cellular rejection (ACR) of heart allografts reflected that miR-182 was statistically significantly dysregulated in endomyocardial biopsies (with, prior to and after ACR) by Next-Generation Sequencing (Nováková et al., 2019). These findings demonstrated that a group of microRNAs were discovered to be changed in endomyocardial biopsies during I/R injury, and a diagnostic score based on their relative levels was developed for I/R injury diagnosis. miR-182 is known to regulate FOXO1 expression (Roser et al., 2018). Wei et al. performed microarray miRNA profiling in a murine model of cardiac allograft rejection and subsequently found that miR-182 increased in allograft tissues, graft-infiltrating mononuclear cells and plasmas (Wei et al., 2012). Moreover, in their subsequent study, CD4 + T cells were the main cellular source of miR-182 during allograft rejection, and miR-182−/− mice showed augmented allograft survival via targeted modulation of FOXO1 expression (Wei et al., 2017). These studies suggest that miR-182 could also serve as a potential therapeutic target, which delineates new and strong mechanisms for patients following heart transplantation.
Furthermore, it is also becoming recognized that inhibiting or restoring specific miRNAs has the potential to regulate other non-coding RNAs. miR-182-5p may bind to the 3′-UTR of BNIP3 (Bcl-2/E1B 19 kDa-interacting protein 3) to repress its high expression after I/R, which prevents excessive cell death triggered by pathologic conditions (Jia et al., 2020) (Bellot et al., 2009). Deng et al. found that RT-qPCR analysis showed lncRNA SNHG14 was highly expressed and promoted OGD/R-induced neuronal injury by inducing excessive mitophagy via the miR-182-5p/BINP3 axis in HT22 cell cell model established by OGD/R (Deng et al., 2020). Of note, a small molecule inducer of miR-182, kenpaullone compound 5, suppressed cardiac cell death and improved heart function by downregulating BNIP3 in I/R-injury rats (Lee et al., 2016). Given these key roles and functions of miR-182 in cardiovascular disease, targeting miR-182 may regulate complex biological processes as a potential therapy for disease. However, issues such as immune stimulatory effects, mode of delivery and off-target effects pose a major challenge in bringing miRNA therapeutics into mainstream clinical practice. These concerns must be addressed in future studies.
miR-182 in cerebrovascular diseases
There are many similar pathobiology and clinical manifestations between ischemic stroke and myocardial ischemia. Among the various underlying mechanisms of I/R injury, adequate regulation of miRNA levels may play a critical role in the prevention and treatment of I/R. In an observational clinical study, it was observed that patients with acute cerebral infarction and cerebral hemorrhage had significantly lower expression of miR-126 and higher expression of miR-182 when compared to subjects in the control group. Patients with mild conditions or good prognosis had higher miR-126 expression and lower miR-182 expression than patients with severe conditions or poor prognosis, and the combination of miR-126 and miR-182 showed better prognosis accuracy (Qi et al., 2020). The analysis of microRNA expression detected by microarray showed that the expression of miR-182 was downregulated in the rat cerebral cortex in left common carotid artery ligation models (Cui and Yang, 2013). A similar finding was also observed in BV2 cells with an OGD model, which showed miR-182-5p mimics attenuates cerebral I/R injury by targeting TLR4 (Wang et al., 2018a). Conversely, miR-182 was selectively upregulated after ischemic preconditioning in mice by transient occlusion of the middle cerebral artery (Lee et al., 2010), and stroke volume and neurological score were significantly improved by pre-treatment with miR-182 antagomir (Alhadidi et al., 2022). Additionally, the expression of miR-182 was found to increase after permanent middle cerebral artery occlusion (pMCAO), and it exacerbated cerebral ischemia injury by targeting inhibitory member of the ASPP family (iASPP) or aggravated blood-brain barrier disruption by downregulating the mTOR/FOXO1 pathway in cerebral ischemia (Yi et al., 2017) (Zhang et al., 2020). The inconsistency with miR-182 expression level in patients with cerebrovascular diseases suggesting further work needs to be done to establish a greater degree of accuracy on this matter. The protective effects of propofol (PPF) and exosomes released from astrocytes induced by the OGD/R model with berberine pretreatment (BBR-exos) have been reported in cerebral I/R injury, and the expression of miR-182 is upregulated (Hu et al., 2021) (Ding et al., 2023). The reason for the protective effect of PPF is that downregulated lncRNA MALAT1 increases miR-182 expression and suppresses the expression of TLR4, while the reason for the protective effect of BBR-exos is that highly expressed miR-182-5p in BBR-exos inhibits neuroinflammation by targeting Rac1. These findings suggest that targeting miR-182 could be a new therapeutic approach to address cerebrovascular diseases.
miR-182 in cardiac hypertrophy and hypertension
Hypertension is one of the most prevalent chronic diseases (Mozaffarian et al., 2016). Maladaptive cardiac hypertrophy occurs as a consequence of persistent high blood pressure which is characterized by an enlargement of cardiomyocytes (CMs) and heart mass. Cardiac hypertrophy is a physiological response to physiological and pathological stimuli in hypertensive heart disease, MI, hypertrophic cardiomyopathy, and other CVDs. Persistent cardiac hypertrophy ultimately leads to eccentric heart dilation, HF and sudden death (Norton et al., 2002). For decades, a growing number of studies have suggested that previously unrecognized mechanisms, including non-coding RNAs, positively or negatively regulate cardiac hypertrophy and hypertension. The researchers discovered that hsa-miR-182-5p was associated with hypertension through the use of weighted gene co-expression network analysis (WGCNA), which helped to improve the understanding of the pathogenesis of hypertension (Li et al., 2020). Specifically, rno-miR-182 was upregulated and associated with remodeling in aging (12 months) spontaneously hypertensive rats (SHRs) when compared with young (3 months) SHRs (Wang et al., 2018b). miR-182 had a similar expression trend in myocardial hypertrophy associated with myocardial angiogenesis which was induced by placental growth factor (PlGF) in neonatal rat cardiomyocytes (NRCs) and mouse hearts. Their mechanism of action newly identified miR-182 target Bcat2 and regulate the expression of Akt/mTORC1 pathway (Li et al., 2016). Moreover, the expression of lncRNA SNHG16 was at a high level in the cardiac hypertrophic model induced by angiotensin II in neonatal mouse cardiomyocytes through repressed regulation of the miR-182-5p/IGF1 axis (Wang et al., 2020). New technologies such as cholesterol-containing nanocarriers, which can efficiently deliver inhibitor of miR-182 into hearts, have been developed. This nanosystem can significantly suppress cardiac hypertrophy in transverse aortic constriction (TAC) mice through miR-182 targeting FOXO3 and increasing its expression (Zhi et al., 2019).
miR-182 in heart failure
Heart failure (HF) is considered an epidemic disease in the modern world and results in different, parallel developing clinical signs and symptoms. These signs and symptoms sum up to an unspecific clinical picture; thus it is urgent to explore invasive and non-invasive diagnostic tools to obtain accurate diagnosis, treatment and prognosis (Tanai and Frantz, 2015). miR-182 was shown to be upregulated in the sera of HF patients (stable, acute decompensated), and which may be a potential prognostic marker by ROC analysis and Cox regression analysis (Cakmak et al., 2015) (Zhirov et al., 2019). miR-182 was found to be inversely correlated with left ventricular mass index in symptomatic HF patients with systolic dysfunction (Ikitimur et al., 2015). Previous studies indicated that a low circulating BDNF (brain-derived neurotrophic factor) level was linked with a poor prognosis in cardiovascular diseases (Montone et al., 2021) (Yılmaz, 2019). A recent study showed a novel correlation between BDNF and miR-182-5 have been described in HF patients. The expression of miR-182-5p was upregulated and the BDNF level was decreased in HF patients. Moreover, miR-182-5p combined with BDNF predicted prognosis for better prevention in HF by identifying and following risk groups (Fang et al., 2022). These clinical findings suggest that down-regulating the expression level of miR-182 may help improve ventricular remodeling and prognosis in patients with chronic HF, but further study is required for validation.
miR-182 in congenital heart disease and cardiotoxicity
Congenital heart disease (CHD) is one of the most common birth defects (Lage et al., 2012) (Diab et al., 2021). Genetic or chromosomal abnormalities, epigenetic factors, excessive alcohol consumption during pregnancy, the use of medications, and maternal viral infection in the first trimester of pregnancy are all risk factors for congenital heart disease in children (Diab et al., 2021). CHD is the leading non-infectious cause of mortality in newborns, especially cyanotic CHD (Rohit and Rajan, 2020). miR-182 was shown to be decreased in serum samples from patients with cyanotic CHD and overexpression of miR-182 in hypoxia-induced H9c2 cells that was associated with promoted cell proliferation and decreased apoptosis through targeting HES1 (Zhang et al., 2018c). Trichloroethylene (TCE) is a common environmental pollutant that is associated with congenital cardiac defects. TCE exposure induced heart defects and dysfunctions, and upregulated miR-182 in the hearts of zebrafish embryos (Huang et al., 2020). In addition, miR-182-5p was upregulated in human-induced pluripotent stem cell-derived cardiomyocytes (hiPSC-CMs) that were exposed to doxorubicin, etoposide or kinase inhibitors (Chaudhari et al., 2016) (Nemade et al., 2018) (Gryshkova et al., 2022). These observations provide new evidence for an unexpected and sensitive role of miR-182 as a cardiotoxicity biomarker for screening novel drugs and environmental cardiotoxicity.
Conclusion
Dysregulation of miR-182 contributes to the development of a variety of cardiovascular and cerebrovascular diseases, including atherosclerosis, CAD, MI, I/R injury, organ transplantation, cardiac hypertrophy, hypertension, heart failure, congenital heart disease and cardiotoxicity (Table 1). New insights into the involvement of miR-182 in the regulation of proliferation, migration, hypoxia, ischemia, hypertrophy and apoptosis have provided alternative ways to inhibit cardiac pathology with the potential for translation into the clinic. However, the same miR-182 members play different roles in different diseases and pathological processes. So, further researches on miR-182 in cardiovascular and cerebrovascular diseases are needed. Targeting miR-182 function with inhibitors or mimics has become a viable option for the modulation of proteins and other miRNAs that are dysregulated in cardiovascular and cerebrovascular diseases. However, the therapeutic application of miR-182 in cardiovascular diseases is still in its infancy. Most studies have only observed changes in the expression levels of miR-182 in the serum of CVD patients, mainly focusing on CAD and CHF patients, and there is a lack of relevant studies on the further intervention of miR-182. What’s more, some miR-182 family members have two sides in the role of cardiovascular and cerebrovascular diseases. For example, inducing miR-182 expression by kenpaullone compound 5 can suppress cardiac cell death and improved heart function (Lee et al., 2016). Nevertheless, miR-182 is also a regulator of cardiac hypertrophy. New nano system which can efficiently deliver inhibitor of miR-182 into hearts can significantly suppress cardiac hypertrophy (Zhi et al., 2019). Therefore, it is essential to identify the optimal balance between their harmful and beneficial effects to maximize their therapeutic potential while minimizing their negative impact. In addition, there have been few studies on the delivery system of miR-182, and it is necessary to find a suitable miR-182 vector that can target our specific cells for precision treatment. Meanwhile, there is a lack of studies on long-term effects. Current studies mostly focus on the short-term effects of miRNA, and there is not enough evidence to prove that miRNA can have a sustained therapeutic effect on CVD. Optimizing the stability of miR-182, improving delivery systems, controlling off-target effects and targeted drug delivery remain hurdles that need to be overcome for future development of therapeutic applications of miR-182 in CVD. Based on the aforementioned limitations, in order to provide new preventive and therapeutic targets for the treatment of cardiovascular and cerebrovascular diseases, more clinical trials translating animal experimental results need to be evaluated in future studies to evaluate the mechanism of the action of miR-182.
Author contributions
GP, LC, and QW designed and wrote the manuscript. YW, CH, and CF revised the manuscript. GP and LC drew the figures. CF and QW provided critical feedback and helped to shape the manuscript. All authors listed have made a substantial contribution to the work.
Funding
The study was funded by the National Key R&D Program of China (Grant No. 2020YFC2008502) and the 1·3·5 Project for Disciplines of Excellence, West China Hospital, Sichuan University.
Conflict of interest
The authors declare that the research was conducted in the absence of any commercial or financial relationships that could be construed as a potential conflict of interest.
Publisher’s note
All claims expressed in this article are solely those of the authors and do not necessarily represent those of their affiliated organizations, or those of the publisher, the editors and the reviewers. Any product that may be evaluated in this article, or claim that may be made by its manufacturer, is not guaranteed or endorsed by the publisher.
References
Alhadidi, Q. M., Xu, L., Sun, X., Althobaiti, Y. S., Almalki, A., Alsaab, H. O., et al. (2022). MiR-182 inhibition protects against experimental stroke in vivo and mitigates astrocyte injury and inflammation in vitro via modulation of cortactin activity. Neurochem. Res. 47 (12), 3682–3696. doi:10.1007/s11064-022-03718-6
Bai, Y., Wang, J., Chen, Y., Lv, T., Wang, X., Liu, C., et al. (2021). The miR-182/Myadm axis regulates hypoxia-induced pulmonary hypertension by balancing the BMP- and TGF-β-signalling pathways in an SMC/EC-crosstalk-associated manner. Basic Res. Cardiol. 116 (1), 53. doi:10.1007/s00395-021-00892-6
Bartel, D. P. (2004). MicroRNAs: Genomics, biogenesis, mechanism, and function. Cell 116 (2), 281–297. doi:10.1016/s0092-8674(04)00045-5
Beermann, J., Piccoli, M. T., Viereck, J., and Thum, T. (2016). Non-coding RNAs in development and disease: Background, mechanisms, and therapeutic approaches. Physiol. Rev. 96 (4), 1297–1325. doi:10.1152/physrev.00041.2015
Bellot, G., Garcia-Medina, R., Gounon, P., Chiche, J., Roux, D., Pouysségur, J., et al. (2009). Hypoxia-induced autophagy is mediated through hypoxia-inducible factor induction of BNIP3 and BNIP3L via their BH3 domains. Mol. Cell Biol. 29 (10), 2570–2581. doi:10.1128/mcb.00166-09
Bennett, M. R., Sinha, S., and Owens, G. K. (2016). Vascular smooth muscle cells in atherosclerosis. Circ. Res. 118 (4), 692–702. doi:10.1161/circresaha.115.306361
Bernardo, B. C., Charchar, F. J., Lin, R. C., and McMullen, J. R. (2012). A microRNA guide for clinicians and basic scientists: Background and experimental techniques. Heart Lung Circ. 21 (3), 131–142. doi:10.1016/j.hlc.2011.11.002
Cakmak, H. A., Coskunpinar, E., Ikitimur, B., Barman, H. A., Karadag, B., Tiryakioglu, N. O., et al. (2015). The prognostic value of circulating microRNAs in heart failure: Preliminary results from a genome-wide expression study. J. Cardiovasc. Med. 16 (6), 431–437. doi:10.2459/JCM.0000000000000233
Chaudhari, U., Nemade, H., Gaspar, J. A., Hescheler, J., Hengstler, J. G., and Sachinidis, A. (2016). MicroRNAs as early toxicity signatures of doxorubicin in human-induced pluripotent stem cell-derived cardiomyocytes. Arch. Toxicol. 90 (12), 3087–3098. doi:10.1007/s00204-016-1668-0
Chen, Y., Xue, J., Fang, D., and Tian, X. (2022). Clinical value and mechanism of long non-coding RNA UCA1 in acute respiratory distress syndrome induced by cardiopulmonary bypass. Heart Lung Circ. 32, 544–551. doi:10.1016/j.hlc.2022.10.008
Cui, H., and Yang, L. (2013). Analysis of microRNA expression detected by microarray of the cerebral cortex after hypoxic-ischemic brain injury. J. craniofacial Surg. 24 (6), 2147–2152. doi:10.1097/SCS.0b013e3182a243f3
Dai, R., Zhang, Y., Khan, D., Heid, B., Caudell, D., Crasta, O., et al. (2010). Identification of a common lupus disease-associated microRNA expression pattern in three different murine models of lupus. PLoS One 5 (12), e14302. doi:10.1371/journal.pone.0014302
Deng, Z., Ou, H., Ren, F., Guan, Y., Huan, Y., Cai, H., et al. (2020). LncRNA SNHG14 promotes OGD/R-induced neuron injury by inducing excessive mitophagy via miR-182-5p/BINP3 axis in HT22 mouse hippocampal neuronal cells. Biol. Res. 53 (1), 38. doi:10.1186/s40659-020-00304-4
Diab, N. S., Barish, S., Dong, W., Zhao, S., Allington, G., Yu, X., et al. (2021). Molecular genetics and complex inheritance of congenital heart disease. Genes (Basel) 12 (7), 1020. doi:10.3390/genes12071020
Ding, W., Gu, Q., Liu, M., Zou, J., Sun, J., and Zhu, J. (2023). Astrocytes-derived exosomes pre-treated by berberine inhibit neuroinflammation after stroke via miR-182-5p/Rac1 pathway. Int. Immunopharmacol. 118, 110047. doi:10.1016/j.intimp.2023.110047
Dong, M., Ye, Y., Chen, Z., Xiao, T., Liu, W., and Hu, F. (2020). MicroRNA 182 is a novel negative regulator of adipogenesis by targeting CCAAT/Enhancer-Binding protein α. Obes. (Silver Spring) 28 (8), 1467–1476. doi:10.1002/oby.22863
Fabian, M. R., Sonenberg, N., and Filipowicz, W. (2010). Regulation of mRNA translation and stability by microRNAs. Annu. Rev. Biochem. 79, 351–379. doi:10.1146/annurev-biochem-060308-103103
Fang, F., Zhang, X., Li, B., and Gan, S. (2022). miR-182-5p combined with brain-derived neurotrophic factor assists the diagnosis of chronic heart failure and predicts a poor prognosis. J. Cardiothorac. Surg. 17 (1), 88. doi:10.1186/s13019-022-01802-0
Friedman, L. M., Dror, A. A., Mor, E., Tenne, T., Toren, G., Satoh, T., et al. (2009). MicroRNAs are essential for development and function of inner ear hair cells in vertebrates. Proc. Natl. Acad. Sci. U. S. A. 106 (19), 7915–7920. doi:10.1073/pnas.0812446106
Frostegård, J. (2013). Immunity, atherosclerosis and cardiovascular disease. BMC Med. 11, 117. doi:10.1186/1741-7015-11-117
Griggs, E. M., Young, E. J., Rumbaugh, G., and Miller, C. A. (2013). MicroRNA-182 regulates amygdala-dependent memory formation. J. Neurosci. 33 (4), 1734–1740. doi:10.1523/jneurosci.2873-12.2013
Gryshkova, V., Lushbough, I., Palmer, J., Burrier, R., Delaunois, A., Donley, E., et al. (2022). microRNAs signatures as potential biomarkers of structural cardiotoxicity in human-induced pluripotent stem-cell derived cardiomyocytes. Arch. Toxicol. 96, 2033–2047. doi:10.1007/s00204-022-03280-8
Hata, A. (2013). Functions of MicroRNAs in cardiovascular biology and disease. Annu. Rev. Physiology 75 (1), 69–93. doi:10.1146/annurev-physiol-030212-183737
Hausenloy, D. J., Bøtker, H. E., Condorelli, G., Ferdinandy, P., Garcia-Dorado, D., Heusch, G., et al. (2013). Translating cardioprotection for patient benefit: Position paper from the working group of cellular biology of the heart of the European society of cardiology. Cardiovasc. Res. 98 (1), 7–27. doi:10.1093/cvr/cvt004
Hu, Y., Ye, C., Cheng, S., and Chen, J. (2021). Propofol downregulates lncRNA MALAT1 to alleviate cerebral ischemia-reperfusion injury. Inflammation 44 (6), 2580–2591. doi:10.1007/s10753-021-01525-9
Huang, Y., Jiang, B., Xia, Y., Wang, J., Ji, C., Tong, J., et al. (2020). Downregulation of miR-133a contributes to the cardiac developmental toxicity of trichloroethylene in zebrafish. Chemosphere 251, 126610. doi:10.1016/j.chemosphere.2020.126610
Ikitimur, B., Cakmak, H. A., Coskunpinar, E., Barman, H. A., and Vural, V. A. (2015). The relationship between circulating microRNAs and left ventricular mass in symptomatic heart failure patients with systolic dysfunction. Kardiol. Pol. 73 (9), 740–746. doi:10.5603/KP.a2015.0082
Jia, S., Qiao, X., Ye, J., Fang, X., Xu, C., Cao, Y., et al. (2016). Nogo-C regulates cardiomyocyte apoptosis during mouse myocardial infarction. Cell Death Dis. 7 (10), e2432. doi:10.1038/cddis.2016.331
Jia, X. N., Yin, S. D., Wei, Y., and Chen, L. (2020). MiR-182-5p inhibited proliferation and migration of ovarian cancer cells by targeting BNIP3. Eur. Rev. Med. Pharmacol. Sci. 24 (15), 7912. doi:10.26355/eurrev_202008_22446
Jin, C., Gao, S., Li, D., Shi, X., Hu, Z., Wang, C., et al. (2020). MiR-182-5p inhibits the proliferation of vascular smooth muscle cells induced by ox-LDL through targeting PAPPA. Int. Heart J. 61 (4), 822–830. doi:10.1536/ihj.19-708
Kattoor, A. J., Kanuri, S. H., and Mehta, J. L. (2019). Role of ox-LDL and LOX-1 in atherogenesis. Curr. Med. Chem. 26 (9), 1693–1700. doi:10.2174/0929867325666180508100950
Kiesow, K., Bennewitz, K., Miranda, L. G., Stoll, S. J., Hartenstein, B., Angel, P., et al. (2015). Junb controls lymphatic vascular development in zebrafish via miR-182. Sci. Rep. 5, 15007. doi:10.1038/srep15007
Kim, V. N., Han, J., and Siomi, M. C. (2009). Biogenesis of small RNAs in animals. Nat. Rev. Mol. Cell Biol. 10 (2), 126–139. doi:10.1038/nrm2632
Kim, K. M., Park, S. J., Jung, S. H., Kim, E. J., Jogeswar, G., Ajita, J., et al. (2012). miR-182 is a negative regulator of osteoblast proliferation, differentiation, and skeletogenesis through targeting FoxO1. J. Bone Min. Res. 27 (8), 1669–1679. doi:10.1002/jbmr.1604
Knuuti, J., Wijns, W., Saraste, A., Capodanno, D., Barbato, E., Funck-Brentano, C., et al. (2019). 2019 ESC Guidelines for the diagnosis and management of chronic coronary syndromes: The Task Force for the diagnosis and management of chronic coronary syndromes of the European Society of Cardiology (ESC). Eur. Heart J. 41 (3), 407–477. doi:10.1093/eurheartj/ehz425
Lage, K., Greenway, S. C., Rosenfeld, J. A., Wakimoto, H., Gorham, J. M., Segrè, A. V., et al. (2012). Genetic and environmental risk factors in congenital heart disease functionally converge in protein networks driving heart development. Proc. Natl. Acad. Sci. U. S. A. 109 (35), 14035–14040. doi:10.1073/pnas.1210730109
Lee, Y. S., and Dutta, A. (2009). MicroRNAs in cancer. Annu. Rev. Pathol. 4, 199–227. doi:10.1146/annurev.pathol.4.110807.092222
Lee, S. T., Chu, K., Jung, K. H., Yoon, H. J., Jeon, D., Kang, K. M., et al. (2010). MicroRNAs induced during ischemic preconditioning. Stroke 41 (8), 1646–1651. doi:10.1161/STROKEAHA.110.579649
Lee, S. Y., Lee, S., Choi, E., Ham, O., Lee, C. Y., Lee, J., et al. (2016). Small molecule-mediated up-regulation of microRNA targeting a key cell death modulator BNIP3 improves cardiac function following ischemic injury. Sci. Rep. 6, 23472. doi:10.1038/srep23472
Li, N., Hwangbo, C., Jaba, I. M., Zhang, J., Papangeli, I., Han, J., et al. (2016). miR-182 modulates myocardial hypertrophic response induced by angiogenesis in heart. Cell Mol. Life Sci. 6, 21228. doi:10.1038/srep21228
Li, Z., Chyr, J., Jia, Z., Wang, L., Hu, X., Wu, X., et al. (2020). Identification of hub genes associated with hypertension and their interaction with miRNA based on weighted gene coexpression network analysis (WGCNA) analysis. Med. Sci. Monit. 26, e923514. doi:10.12659/MSM.923514
Li, F., Zhang, L., Xue, H., Xuan, J., Rong, S., and Wang, K. (2021). SIRT1 alleviates hepatic ischemia-reperfusion injury via the miR-182-mediated XBP1/NLRP3 pathway. Mol. Ther. - Nucleic Acids 23, 1066–1077. doi:10.1016/j.omtn.2020.11.015
Liu, C., Xu, X., Huang, C., Zhang, L., Shang, D., Cai, W., et al. (2020). Circ_002664/miR-182-5p/Herpud1 pathway importantly contributes to OGD/R-induced neuronal cell apoptosis. Mol. Cell Probes 53, 101585. doi:10.1016/j.mcp.2020.101585
McClelland, A. D., and Kantharidis, P. (2014). microRNA in the development of diabetic complications. Clin. Sci. (Lond) 126 (2), 95–110. doi:10.1042/cs20130079
Moens, A. L., Claeys, M. J., Timmermans, J. P., and Vrints, C. J. (2005). Myocardial ischemia/reperfusion-injury, a clinical view on a complex pathophysiological process. Int. J. Cardiol. 100 (2), 179–190. doi:10.1016/j.ijcard.2004.04.013
Montone, R. A., Camilli, M., Del Buono, M. G., Russo, M., Rinaldi, R., Canonico, F., et al. (2021). Brain-derived neurotrophic factor in patients with acute coronary syndrome. Transl. Res. 231, 39–54. doi:10.1016/j.trsl.2020.11.006
Mozaffarian, D., Benjamin, E. J., Go, A. S., Arnett, D. K., Blaha, M. J., Cushman, M., et al. (2016). Executive summary: Heart disease and stroke statistics--2016 update: A report from the American heart association. Circulation 133 (4), 447–454. doi:10.1161/cir.0000000000000366
Nemade, H., Chaudhari, U., Acharya, A., Hescheler, J., Hengstler, J. G., Papadopoulos, S., et al. (2018). Cell death mechanisms of the anti-cancer drug etoposide on human cardiomyocytes isolated from pluripotent stem cells. BMC Cardiovasc Disord. 92 (4), 1507–1524. doi:10.1007/s00204-018-2170-7
Neri, M., Riezzo, I., Pascale, N., Pomara, C., and Turillazzi, E. (2017). Ischemia/reperfusion injury following acute myocardial infarction: A critical issue for clinicians and forensic pathologists. Mediat. Inflamm. 2017, 7018393. doi:10.1155/2017/7018393
Norton, G. R., Woodiwiss, A. J., Gaasch, W. H., Mela, T., Chung, E. S., Aurigemma, G. P., et al. (2002). Heart failure in pressure overload hypertrophy. The relative roles of ventricular remodeling and myocardial dysfunction. J. Am. Coll. Cardiol. 39 (4), 664–671. doi:10.1016/s0735-1097(01)01792-2
Nováková, T., Macháčková, T., Novák, J., Hude, P., Godava, J., Žampachová, V., et al. (2019). Identification of a diagnostic set of endomyocardial biopsy microRNAs for acute cellular rejection diagnostics in patients after heart transplantation using next-generation sequencing. Cells 8 (11), 1400. doi:10.3390/cells8111400
Ono, K., Kuwabara, Y., and Han, J. (2011). MicroRNAs and cardiovascular diseases. Febs J. 278 (10), 1619–1633. doi:10.1111/j.1742-4658.2011.08090.x
Osman, A. (2012). MicroRNAs in health and disease--basic science and clinical applications. Clin. Lab. 58 (5-6), 393–402.
Parikh, N. I., Gona, P., Larson, M. G., Fox, C. S., Benjamin, E. J., Murabito, J. M., et al. (2009). Long-term trends in myocardial infarction incidence and case fatality in the National Heart, Lung, and Blood Institute's Framingham Heart study. Circulation 119 (9), 1203–1210. doi:10.1161/circulationaha.108.825364
Qi, R., Liu, H., Liu, C., Xu, Y., and Liu, C. (2020). Expression and short-term prognostic value of miR-126 and miR-182 in patients with acute stroke. Exp. Ther. Med. 19 (1), 527–534. doi:10.3892/etm.2019.8227
Rancan, L., Simon, C., Marchal-Duval, E., Casanova, J., Paredes, S. D., Calvo, A., et al. (2016). Lidocaine administration controls micrornas alterations observed after lung ischemia-reperfusion injury. Anesth. Analgesia 123 (6), 1437–1447. doi:10.1213/ANE.0000000000001633
Ren, L., Chen, S., Liu, W., Hou, P., Sun, W., and Yan, H. (2019). Downregulation of long non-coding RNA nuclear enriched abundant transcript 1 promotes cell proliferation and inhibits cell apoptosis by targeting miR-193a in myocardial ischemia/reperfusion injury. BMC Cardiovasc. Disord. 19 (1), 192. doi:10.1186/s12872-019-1122-3
Rohit, M., and Rajan, P. (2020). Approach to cyanotic congenital heart disease in children. Indian J. Pediatr. 87 (5), 372–380. doi:10.1007/s12098-020-03274-3
Roser, A. E., Caldi Gomes, L., Halder, R., Jain, G., Maass, F., Tonges, L., et al. (2018). miR-182-5p and miR-183-5p act as GDNF mimics in dopaminergic midbrain neurons. Mol. Ther. - Nucleic Acids 11, 9–22. doi:10.1016/j.omtn.2018.01.005
Rupaimoole, R., and Slack, F. J. (2017). MicroRNA therapeutics: Towards a new era for the management of cancer and other diseases. Nat. Rev. Drug Discov. 16 (3), 203–222. doi:10.1038/nrd.2016.246
Saliminejad, K., Khorram Khorshid, H. R., Soleymani Fard, S., and Ghaffari, S. H. (2019). An overview of microRNAs: Biology, functions, therapeutics, and analysis methods. Biol. Funct. Ther. analysis methods 234 (5), 5451–5465. doi:10.1002/jcp.27486
Sasso, J. M., Ambrose, B. J. B., Tenchov, R., Datta, R. S., Basel, M. T., DeLong, R. K., et al. (2022). The progress and promise of RNA Medicine─An arsenal of targeted treatments. Prog. Promise RNA Medicine─An Arsenal Target. Treat. 65 (10), 6975–7015. doi:10.1021/acs.jmedchem.2c00024
Saus, E., Soria, V., Escaramís, G., Vivarelli, F., Crespo, J. M., Kagerbauer, B., et al. (2010). Genetic variants and abnormal processing of pre-miR-182, a circadian clock modulator, in major depression patients with late insomnia. Hum. Mol. Genet. 19 (20), 4017–4025. doi:10.1093/hmg/ddq316
Shi, L., Li, Y., Shi, M., Li, X., Li, G., Cen, J., et al. (2022). Hsa_circRNA_0008028 deficiency ameliorates high glucose-induced proliferation, calcification, and autophagy of vascular smooth muscle cells via miR-182-5p/TRIB3 Axis. Oxid. Med. Cell Longev. 2022, 5142381. doi:10.1155/2022/5142381
Simonis, G., Strasser, R. H., and Ebner, B. (2012). Reperfusion injury in acute myocardial infarction. Crit. Care 16 (2), A22. Epub 2012 Jun 7. doi:10.1186/cc11280
Stittrich, A. B., Haftmann, C., Sgouroudis, E., Kühl, A. A., Hegazy, A. N., Panse, I., et al. (2010). The microRNA miR-182 is induced by IL-2 and promotes clonal expansion of activated helper T lymphocytes. Nat. Immunol. 11 (11), 1057–1062. doi:10.1038/ni.1945
Sun, L., Bai, Y., Zhao, R., Sun, T., Cao, R., Wang, F., et al. (2016). Oncological miR-182-3p, a novel smooth muscle cell phenotype modulator, evidences from model rats and patients. Arteriosclerosis, Thrombosis, Vasc. Biol. 36 (7), 1386–1397. doi:10.1161/ATVBAHA.115.307412
Sun, L., Lin, P., Chen, Y., Yu, H., Ren, S., Wang, J., et al. (2020). miR-182-3p/Myadm contribute to pulmonary artery hypertension vascular remodeling via a KLF4/p21-dependent mechanism. Theranostics 10 (12), 5581–5599. doi:10.7150/thno.44687
Tanai, E., and Frantz, S. (2015). Pathophysiology of heart failure. Compr. Physiol. 6 (1), 187–214. doi:10.1002/cphy.c140055
Taurino, C., Miller, W. H., McBride, M. W., McClure, J. D., Khanin, R., Moreno, M. U., et al. (2010). Gene expression profiling in whole blood of patients with coronary artery disease. Clin. Sci. (Lond) 119 (8), 335–343. doi:10.1042/cs20100043
Wang, J., Xu, Z., Chen, X., Li, Y., Chen, C., Wang, C., et al. (2018a). MicroRNA-182-5p attenuates cerebral ischemia-reperfusion injury by targeting Toll-like receptor 4. Biochem. Biophys. Res. Commun. 505 (3), 677–684. doi:10.1016/j.bbrc.2018.09.165
Wang, J., Zhang, J., Ding, X., Wang, Y., Li, Z., Zhao, W., et al. (2018b). Differential microRNA expression profiles and bioinformatics analysis between young and aging spontaneously hypertensive rats. Int. J. Mol. Med. 41 (3), 1584–1594. doi:10.3892/ijmm.2018.3370
Wang, D., Lin, B., Zhang, W., and Wang, X. (2020). Up-regulation of SNHG16 induced by CTCF accelerates cardiac hypertrophy by targeting miR-182-5p/IGF1 axis. Cell Biol. Int. 44 (7), 1426–1435. doi:10.1002/cbin.11333
Wei, L., Wang, M., Qu, X., Mah, A., Xiong, X., Harris, A. G., et al. (2012). Differential expression of microRNAs during allograft rejection. Am. J. Transpl. 12 (5), 1113–1123. doi:10.1111/j.1600-6143.2011.03958.x
Wei, L., Kaul, V., Qu, X., Xiong, X., Lau, A. H., Iwai, N., et al. (2017). Absence of miR-182 augments cardiac allograft survival. Transplantation 101 (3), 524–530. doi:10.1097/tp.0000000000001345
WHO (2019). Global cardiovascular diseases report 2019. World Health Organization. Geneva Available at: https://www.who.int/news-room/fact-sheets/detail/cardiovascular-diseases-(cvds (Accessed June 4, 2022).
Winbanks, C. E., Ooi, J. Y., Nguyen, S. S., McMullen, J. R., and Bernardo, B. C. (2014). MicroRNAs differentially regulated in cardiac and skeletal muscle in health and disease: Potential drug targets? Clin. Exp. Pharmacol. Physiol. 41 (9), 727–737. doi:10.1111/1440-1681.12281
Xu, S., Witmer, P. D., Lumayag, S., Kovacs, B., and Valle, D. (2007). MicroRNA (miRNA) transcriptome of mouse retina and identification of a sensory organ-specific miRNA cluster. J. Biol. Chem. 282 (34), 25053–25066. doi:10.1074/jbc.M700501200
Yellon, D. M., and Hausenloy, D. J. (2007). Myocardial reperfusion injury. N. Engl. J. Med. 357 (11), 1121–1135. doi:10.1056/NEJMra071667
Yi, H., Huang, Y., Yang, F., Liu, W., He, S., and Hu, X. (2017). MicroRNA-182 aggravates cerebral ischemia injury by targeting inhibitory member of the ASPP family (iASPP). Arch. Biochem. Biophys. 620, 52–58. doi:10.1016/j.abb.2016.05.002
Yılmaz, M. B. (2019). Brain-derived neurotrophic factor in heart failure. Anatol. J. Cardiol. 22 (6), 317–318. doi:10.14744/AnatolJCardiol.2019.49393
Yu, B., Qian, T., Wang, Y., Zhou, S., Ding, G., Ding, F., et al. (2012). miR-182 inhibits Schwann cell proliferation and migration by targeting FGF9 and NTM, respectively at an early stage following sciatic nerve injury. Nucleic Acids Res. 40 (20), 10356–10365. doi:10.1093/nar/gks750
Zhang, Q. H., Sun, H. M., Zheng, R. Z., Li, Y. C., Zhang, Q., Cheng, P., et al. (2013). Meta-analysis of microRNA-183 family expression in human cancer studies comparing cancer tissues with noncancerous tissues. Gene 527 (1), 26–32. doi:10.1016/j.gene.2013.06.006
Zhang, X., Xiao, C., and Liu, H. (2018a). Ganoderic acid A protects rat H9c2 cardiomyocytes from hypoxia-induced injury via up-regulating miR-182-5p. Cell Physiol. Biochem. 50 (6), 2086–2096. doi:10.1159/000495053
Zhang, Y., Fang, J., and Ma, H. (2018b). Inhibition of miR-182-5p protects cardiomyocytes from hypoxia-induced apoptosis by targeting CIAPIN1. Biochem. Cell Biol. 96 (5), 646–654. doi:10.1139/bcb-2017-0224
Zhang, Y., Peng, B., and Han, Y. (2018c). MiR-182 alleviates the development of cyanotic congenital heart disease by suppressing HES1. Eur. J. Pharmacol. 836, 18–24. doi:10.1016/j.ejphar.2018.08.013
Zhang, T., Tian, C., Wu, J., Zhang, Y., Wang, J., Kong, Q., et al. (2020). MicroRNA-182 exacerbates blood-brain barrier (BBB) disruption by downregulating the mTOR/FOXO1 pathway in cerebral ischemia. Faseb J. 34 (10), 13762–13775. doi:10.1096/fj.201903092R
Zhao, J., Li, X., Hu, J., Chen, F., Qiao, S., Sun, X., et al. (2019). Mesenchymal stromal cell-derived exosomes attenuate myocardial ischaemia-reperfusion injury through miR-182-regulated macrophage polarization. Cardiovasc Res. 115 (7), 1205–1216. doi:10.1093/cvr/cvz040
Zhi, Y., Xu, C., Sui, D., Du, J., Xu, F. J., and Li, Y. (2019). Effective delivery of hypertrophic miRNA inhibitor by cholesterol-containing nanocarriers for preventing pressure overload induced cardiac hypertrophy. Hypertrophy 6 (11), 1900023. doi:10.1002/advs.201900023
Zhirov, I. V., Baulina, N. M., Nasonova, S. N., Osmak, G. Z., Matveyeva, N. A., Mindzaev, D. R., et al. (2019). [Full - transcriptome analysis of miRNA expression in mononuclear cells in patients with acute decompensation of chronic heart failure of various etiologies]. Ter. Arkh 91 (9), 62–67. doi:10.26442/00403660.2019.09.000294
Zhou, Y. M., Lan, X., Guo, H. B., Zhang, Y., Ma, L., and Cao, J. B. (2014). Rho/ROCK signal cascade mediates asymmetric dimethylarginine-induced vascular smooth muscle cells migration and phenotype change. Biomed. Res. Int. 2014, 683707. doi:10.1155/2014/683707
Keywords: cardiovascular and cerebrovascular diseases, miR-182, atherosclerosis, myocardial ischemia, ischemic stroke, heart failure, mesenchymal stromal cells, ischemia/reperfusion (I/R) injury
Citation: Pei G, Chen L, Wang Y, He C, Fu C and Wei Q (2023) Role of miR-182 in cardiovascular and cerebrovascular diseases. Front. Cell Dev. Biol. 11:1181515. doi: 10.3389/fcell.2023.1181515
Received: 07 March 2023; Accepted: 24 April 2023;
Published: 09 May 2023.
Edited by:
Achilleas D. Theocharis, University of Patras, GreeceReviewed by:
Bruno Moukette, Indiana University, Purdue University Indianapolis, United StatesYiwei Liu, Shanghai Children’s Medical Center, China
Copyright © 2023 Pei, Chen, Wang, He, Fu and Wei. This is an open-access article distributed under the terms of the Creative Commons Attribution License (CC BY). The use, distribution or reproduction in other forums is permitted, provided the original author(s) and the copyright owner(s) are credited and that the original publication in this journal is cited, in accordance with accepted academic practice. No use, distribution or reproduction is permitted which does not comply with these terms.
*Correspondence: Chenying Fu, ZmN5aW5nXzIwMDRAMTYzLmNvbQ==; Quan Wei, d2VpcXVhbkBzY3UuZWR1LmNu
†These authors have contributed equally to this work and share first authorship