- 1Sesoko Station, Tropical Biosphere Research Center, University of the Ryukyus, Nishihara, Japan
- 2Shimoda Marine Research Center, University of Tsukuba, Shimoda, Japan
- 3Department of Immunology, Graduate School of Biomedical and Health Sciences, Hiroshima University, Hiroshima, Japan
The reef-building coral Acropora is a broadcast spawning hermaphrodite including more than 110 species in the Indo-Pacific. In addition, many sympatric species show synchronous spawning. The released gametes need to mate with conspecifics in the mixture of the gametes of many species for their species boundaries. However, the mechanism underlying the species recognition of conspecifics at fertilization remains unknown. We hypothesized that rapid molecular evolution (positive selection) in genes encoding gamete-composing proteins generates polymorphic regions that recognize conspecifics in the mixture of gametes from many species. We identified gamete proteins of Acropora digitifera using mass spectrometry and screened the genes that support branch site models that set the “foreground” branches showing strict fertilization specificity. ADAM10, ADAM17, Integrin α9, and Tetraspanin4 supported branch-site model and had positively selected site(s) that produced polymorphic regions. Therefore, we prepared antibodies against the proteins of A. digitifera that contained positively selected site(s) to analyze their functions in fertilization. The ADAM10 antibody reacted only with egg proteins of A. digitifera, and immunohistochemistry showed ADAM10 localized around the egg surface. Moreover, the ADAM10 antibody inhibited only A. digitifera fertilization but not the relative synchronous spawning species A. papillare. This study indicates that ADAM10 has evolved to gain fertilization specificity during speciation and contributes to species boundaries in this multi-species, synchronous-spawning, and species-rich genus.
1 Introduction
In sessile animals such as coral, gamete recognition is a trait associated with mate choice. Species recognition by gametes is crucial for synchronous spawning species, especially species-rich genera (Knowlton, 2000; Carlisle and Swanson, 2021). Gamete recognition (species- and self-recognition) provides a mechanism for mate choice to distinguish self from non-self and species identity in hermaphroditic species that broadcast gamete during multispecies spawning events. Mate choice is imperative for maintaining species boundaries and the fitness of descendants (Gowaty et al., 2007; Harrison and Larson, 2014; Ihle et al., 2015). The mechanism(s) of species- and self-recognition are essential for species boundaries (Willis et al., 2006). However, how gamete fertilizes with conspecifics of the other colonies is still unknown in the Acropora corals.
The coral Acropora is a broadcast-spawning hermaphrodite. Their gametes are fertilized in the water column after the release of a small package filled with sperm and eggs called “gamete bundles.” In most species, gametes possess strict species recognition to mate with conspecifics after synchronous spawning among congeneric species (“multi-specific spawning”) (Willis et al., 2006; Baird et al., 2009). Although species recognition is a prerequisite for reproductive isolation and species boundary in Acropora spp., the mechanism of species recognition is still unclear.
In Acropora, synchronous spawning behaviors provoke a risk of hybridization, and therefore, specific fertilization pathways are required for their species boundary. For example, sperm may swim toward eggs (Morita et al., 2006), but the cascades from gamete interaction with conspecifics to membrane fusion are still unknown. In contrast, gamete recognition proteins leading to adhering conspecific gametes are identified. For example, gamete recognition protein “binding” in sea urchins contributes to species-specific adhering. The bindin is under positive selection (Zigler et al., 2005), and genotypes of “bindin” evolved to obtain fertilization efficiency depending on the sperm concentration (Levitan et al., 2007). In addition, the genotypes of the “bindin” changed rapidly according to the fertilization condition due to changes in sea urchin population number (Levitan and Stapper, 2009). Lysin in the sperm of the broadcast-spawning marine invertebrate, abalone, is also involved in the gamete species recognition (Vacquier and Lee, 1993). Lysin is also under positive selection (Lee et al., 1995; Galindo et al., 2003), and interacts with the egg protein VERL (Galindo et al., 2002; Aagaard et al., 2010). The VERL shows coevolution with lysin (Clark et al., 2009). However, gamete proteins involved in Acropora fertilization have not been fully studied.
The integrin family of proteins is involved in cell–cell adhesion (Hynes, 1987; Arnaout et al., 2007). In Acropora, its involvement in sperm and egg interactions (Iguchi et al., 2007) and its divergence in terms of sequences and expression (Knack et al., 2008) have been reported. The ADAM family of disintegrins and metalloproteases includes ADAM2 (a “fertilin”), which is implicated in sperm–egg binding via integrin—ADAM binding (Evans, 2001; Merc et al., 2021). However, the function of integrins and ADAM in the fertilization of coral have not yet been investigated. Although integrin β1 is partly involved in fertilization in Acropora, the involvement of integrins in fertilization in mammalian species with an internal fertilization system is controversial (Miller et al., 2000; He et al., 2003; Barraud-Lange et al., 2007). For example, antibodies against integrins suppress fertilization (Barraud-Lange et al., 2007). Fertilization occurs in eggs (without the ZP) lacking integrin α6β1 (Miller et al., 2000), and the removal of the ZP layer indicates that integrin β1-knockout mice are not sterile (He et al., 2003).
Another family of candidate proteins with a role in sperm—egg interaction is that of the transmembrane tetraspanins. In mammals, the tetraspanin family members participate in primary sperm binding, gamete fusion, and polyspermy blocking (Jankovicova et al., 2020). Of the tetraspanins, the cluster of differentiation (CD9) is essential in the mouse gamete fusion and fertilization (Kaji et al., 2000; Miyado et al., 2000). CD9 participates in the formation of integrin α6β1/tetraspanin clusters in the plasma membrane, which are required for gamete fusion (Ziyyat et al., 2006). The other tetraspanins play many roles, such as sperm-to-egg binding in the ZP (CD9, CD81, and CD151) (Yanagimachi, 1994; Jin et al., 2011) and polyspermy blocking at the plasma membrane (CD9 and CD81) (Talbot and Dandekar, 2003; Ravaux et al., 2018). However, the presence and role of tetraspanins in Acropora have not been studied.
Although these proteins in mammals are involved in gamete binding at fertilization, the functions of the proteins in the coral Acropora are unknown. Released gametes must mate with conspecifics within the mixture of heterospecifics. In other words, gamete proteins for species recognition must be polymorphic to recognize conspecifics.
The extracellular region of proteins potentially underlies species recognition due to its interaction with other proteins localizing on the gamete’s surface (Swanson and Vacquier, 2002; Palumbi, 2009; Carlisle and Swanson, 2021). The rapid evolution of the recognition sites is supposed to arise via a positive selection of specific codons in the extracellular region. The species-recognition sites are typically diverse, and many proteins are potentially involved in recognition. Taken together, the history of the species-recognition proteins reflects the speciation history of the coral Acropora.
In this study, gamete species-recognition proteins in the coral Acropora were explored. Proteins in sperm and eggs from A. digitifera were identified using mass spectrometry. The rates of molecular evolution of integrins, ADAMs, and tetraspanins from the identified proteins were analyzed to focus on the acquisition of species recognition. In the analyses, we set non-crossing species as those that acquired strict species recognition. Presumably, proteins in the non-crossing species have positively selected sites at the recognition region. The analyses indicated four proteins, ADAM10, ADAM17, tetraspanin 4, and integrin α9 that were candidates for the recognition proteins. The function(s) of the candidates in fertilization were investigated via antibody treatment. Although the positively selected sites in ADAM10 are supposed to be strong enough to be rigor species recognition in the more than 110 species in the coral Acropora, the inhibitory effect of the antibody was species-specific. Therefore, ADAM10 could be one of the gamete recognition proteins in the broadcast spawning coral Aropora.
2 Materials and methods
2.1 Coral
Acropora digitifera, A. austera, A. tenuis, and A. papillare were used for fertilization analyses. A. tenuis and A. austera spawn the same night, but the spawning time was earlier than the A. digitifera. A. papillare spawns closer to the A. digitifera and their gametes are compatible (Table 2). The other species, A. intermedia, A. florida, and A. donei, were used for RNA isolation and analysis of cDNA sequences of candidate genes. Published sequence data of 15 Acropora species were used, and species were re-sequenced when the registered sequences lacked parts of the open reading frames (ORFs). All colonies were collected at Sesoko Island, Okinawa Prefecture, Japan.
2.2 Mass spectrometry (MS) analyses to identify integrins in Acropora
Eggs and sperm of A. digitifera were collected after spawning according to the previously described methods (Morita et al., 2006), and proteins in the eggs or sperm were analyzed with liquid chromatography-tandem MS at the Kazusa DNA Research Center (Ibaraki, Japan). To identify proteins, the genome information of A. digitifera was used. Approximately 2 g of eggs or 100 mg of sperm were used for the analyses.
2.3 Isolation of orthologs of the integrin, tetraspanin, and ADAM families
Orthoscope v1.5.1 for Acropora (http://yurai.aori.u-tokyo.ac.jp/orthoscope/Acropora.html) (Inoue and Satoh, 2019) was used to isolate orthologs. To isolate CD9 orthologs in Acropora, we used a fasta file of CD9 from Homo sapiens (NM_001769.4).
Phylogenetic trees of isolated ORF sequences were constructed using RaxML with a rapid bootstrap and general time reversible-gamma model (Stamatakis, 2006), and the sequences were aligned using MAFFT v. 7 (multiple alignment program for amino acid or nucleotide sequences) (https://mafft.cbrc.jp/alignment/server/) (Katoh et al., 2019). The aligned phylip files and the maximum likelihood (ML) tree files were used for molecular evolutionary analyses with CodeML (Yang, 1997).
2.4 Molecular evolutionary analysis of candidate genes
The relative rates of synonymous and non-synonymous substitutions in Integrins, Tetraspanins, and ADAMs were calculated using CodeML in PAML (Yang, 1997). Complete ORFs of functional genes from the isolated sequences in Orthoscope were used in the analyses. The codon site model (Model 8 vs. 7) was used, and then confirmed comparison between Model8 and 8a (Supplementary Figure S1), and Bayes empirical Bayes (BEB) analyses were used to detect positively selected sites in the candidate genes (Swanson et al., 2003; Yang et al., 2005).
Branch site analyses (model 2a) were conducted in candidate proteins setting non-crossing species as foreground branches (Yang et al., 2005; Zhang et al., 2005) (Supplementary Figure S1). If the ML model included a category of sites with non-synonymous/synonymous mutations (dN/dS > 1), positive selection likely acted on those sites along that specific lineage. Based on previous studies (Hatta et al., 1999; Fukami et al., 2003; Suzuki et al., 2016), we set non-crossing species (A. digitifera, A. nasuta, A. accuminata, A. muricata, A. hyacinthus, and A. cytherea) as the foreground and crossing species (A. tenuis, A. yongei, A. intermedia, and A. florida) as the background. Several species where species-specificity has not yet been identified (such as A. selago, A. microphathalma, A. awi) were set as background branches. In the null model, dN/dS of positively selected sites in the foreground was constrained to one. A likelihood ratio test was conducted with one degree of freedom. If the branch site model was supported, positively selected sites calculated from BEB analyses were checked.
We also did branch site analyses with aBSREL (http://www.datamonkey.org/absrel) using phylip file (Smith et al., 2015). We set non-crossing species as foreground branches at sites and run the analyses.
2.5 Synthesis of cDNA for construction of expression vectors
Fragments of coral for RNA extraction were collected by snorkeling 3–5 months prior to the predicted spawning month in June. Total RNA was extracted from fresh or preserved coral fragments using TRIzol reagent (Thermo Fisher, Waltham, MA, United States); cDNA was synthesized from the total RNA using SuperScript IV (Thermo Fisher, Waltham, MA, United States) with oligo dT primers.
2.6 TA cloning candidate gene cDNA
TA cloning was performed to isolate several genes from A. papillare and A. donei, whose sequences were not indetified. First, target sequences were amplified with ExTaq (Takara, Ohtsu, Japan) using several primers (Supplementary Table S1). The PCR products were ligated into the pGEM-T Easy Vector (Promega, Madison, WI, United States), which was then transformed into JM109 competent cells (Takara, Ohtsu, Japan). Plasmids were extracted, and cycle sequencing reactions were conducted using ABI BigDye Terminator version 3.1 and Cycle Sequencing Kits with T7 or SP6 primers, followed by capillary electrophoresis in an ABI 3730xl sequencer (Applied Biosystems, Foster City, CA, United States).
2.7 Antibody generation
We generated antibodies against the genes that were positively selected in A. digitifera. Proteins were expressed with the expression vector, pColdPros2, purified, and then the expressed proteins were used as antigens. To construct expression vectors, primers were designed to cover positively selected sites. The target region was amplified using Primstar HS (Takara, Otsu, Japan) and ligated into the vector after restriction enzyme treatment (XhoI/EcoRI) using the DNA Ligation Kit - Mighty Mix (Takara, Otsu, Japan). The ligated plasmid was subcloned into DH5α cells, and the plasmid was isolated. The isolated plasmid was again transformed into BL21 cells, which were then cultured at 37°C in Luria Bertani medium containing ampicillin until reaching OD600 of 0.5, and the expression was induced at 15°C in the presence of 1 mM isopropyl β-D-1-thiogalactopyranoside (IPTG) for 24 h. Expressed proteins were solubilized with 8 M urea and 2 M thiourea and dialyzed against 5 M urea overnight. The supernatant of the extract was applied to TALON resin (Takara, Otsu, Japan). The resin was equilibrated with equilibration buffer (300 mM NaCl and 50 mM NaH2PO4, pH 7.4) and the proteins eluted with elution buffer (300 mM NaCl, 50 mM NaH2PO4, and 150 imidazole, pH 7.4). The purified proteins (3–5 mg) were dialyzed against phosphate-buffered saline, generating a polyclonal antibody. Antibody preparation was conducted at Biologica Co. (Nagoya, Japan). Antiserum was purified with Protein A, and IgG was eluted with 0.1 M glycine (pH 3.0). The eluted IgG with glycine was dialyzed with PBS. The IgG concentration was adjusted to 1.0 mg/mL with PBS.
2.8 Western blotting
Egg or sperm proteins were subjected to 7.5% or 10% acrylamide gel electrophoresis, and the separated proteins were transferred to polyvinylidene difluoride membranes. The membrane was then blocked with 5% (w/v) skim milk and Tris-base saline (TBS)-Tween (150 mM NaCl, 0.05% (v/v) Tween-20, 25 mM Tris-HCl, pH 7.4) overnight at 4°C. The first antibody reaction was carried out at 1/5,000–1/10,000 dilutions in the blocking solution for 1 h at room temperature (25–27°C), and the membrane was washed with TBS-Tween three times for 10 min each. Then, secondary antibody reactions of horseradish peroxidase (HRP)-labeled anti-rabbit immunoglobulin (Ig)G (Rockland, Limerick, PA, United States) were carried out at 1/20,000 dilution in the blocking solution for 1 h at room temperature. The membranes were again washed with TBS-Tween three times for 10 min each. Protein signals were detected using EzWestLumi Plus (ATTO, Tokyo, Japan).
2.9 Immunohistochemistry
Localizations of ADAM10 and Integrin α9 were examined 1 week before spawning according to the method of Morita et al. (2019). Fragments of the coral, A. digitifera, were fixed in Bouin’s solution. After the skeletons were dissolved, tissues were embedded in paraffin and cut into 5-μm-thick sections. The sections were washed with 0.1 M phosphate-buffered saline (PBS), soaked in methanol containing 3% H2O2 for 15 min, and washed with PBS. The H2O2-treated slides were blocked with goat serum from a Histofine kit (Nichirei, Tokyo, Japan) overnight at 4°C. After blocking, the slides were incubated with an antibody against ADAM10 (1/2000 dilution) in PBS containing 1% (w/v) BSA at 4°C for 4 h, and then washed with PBS for 5 min, three times each. The slides were incubated with biotinylated secondary antibody from the Histofine kit for 15 min at room temperature and then washed with PBS. Streptavidin-horseradish peroxidase (HRP) was applied to the slides for 15 min at room temperature and washed with PBS. Peroxidase activity was visualized with 3,3′-diaminobenzidine (DAB) in a Tris-HCl (pH 7.6) buffer containing H2O2.
2.10 Fertilization experiments
Fertilization trials were carried out with eggs and sperm after spawning of A. digitifera, A. austera, and A. papillare. Eggs were incubated with filtered seawater (SW) with one of the developed antibodies (10 µL antibody (1 mg/mL)/1 mL SW) for 10 min at room temperature, and sperm concentration was adjusted to 106 sperm/mL with filtered seawater. Fertilization was confirmed by observing for developed embryos after 2.5–3 h. Fertilization rates were calculated from ratios of developed embryos and the total number of eggs.
2.11 Statistical analysis
Pairwise comparisons using Wilcoxon rank sum test was used to determine significant differences among treatments in fertilization experiments (p < 0.05). Bonferroni correction was carried out for multiple comparisons. R v. 4.0.1 was used for the analysis (Team, 2020).
3 Results
3.1 Integrin, ADAM, and tetraspanin expression in Acropora eggs and sperm
Mass Spectrometry (MS) analyses showed that several integrin and ADAM proteins were present in the eggs and sperm (Table 1). Integrin β1 was detected in eggs, and variables of integrin α and β-like (integrin α-X, -V, -9, -PS1, and β-PS) were also found. However, integrins were not found in the sperm. Several ADAM proteins were found in both sperm (ADAM7 and 10) and eggs (ADAM 6, 10, 12, 17, and 18). On the other hand, ADAM10 was detected only in the eggs by western analyses (see below). In addition, bindin, lysin, and VERL contributing gamete species recognitions in the sea urchin and abalone were not found.
Tetraspanins were found in eggs (tetraspanin 3, 4, 7, and 33) and sperm (tetraspanin 4, 7, and CD63). CD9 was not detected in either eggs or sperm; CD9 of A. digitifera is not registered in GenBank (https://www.ncbi.nlm.nih.gov/gene/); CD9 could not be identified due to lack of the data. However, CD9 of Acropora was obtained from Orthoscope, and cDNA of CD9 in A. digitifera was isolated. We prepared an antibody from the cDNA of A. digitifera, and the antibody reaction was observed corresponding to its mass. Therefore, CD9 is plausibly present in Acropora eggs.
3.2 Molecular evolution of integrins, ADAMs, and tetraspanins
Codon site analysis suggested several genes were positively selected among congeneric Acropora (Table 1). Most species whose genome information is available to show synchronous spawning and gamete compatibility are also identified, except for several species (Table 2). For example, A. digitifera does not show crossing among species in the database for Orthoscope. Branch site analyses were conducted to specify the correlation between the substitution rates of the several limited amino acid sites and species-specific fertilization. Of the integrins, ADAMs, and tetraspanins, four genes (ADAM10, ADAM17, integrin α9, and tetraspanin 4) had positively selected sites during the acquisition of fertilization specificity (Table 1). In contrast, aBSREL analyses support branch analyses only in A. muricata in the tetraspanin 4 (Table 1). CD9 was subjected to strong purifying selection.
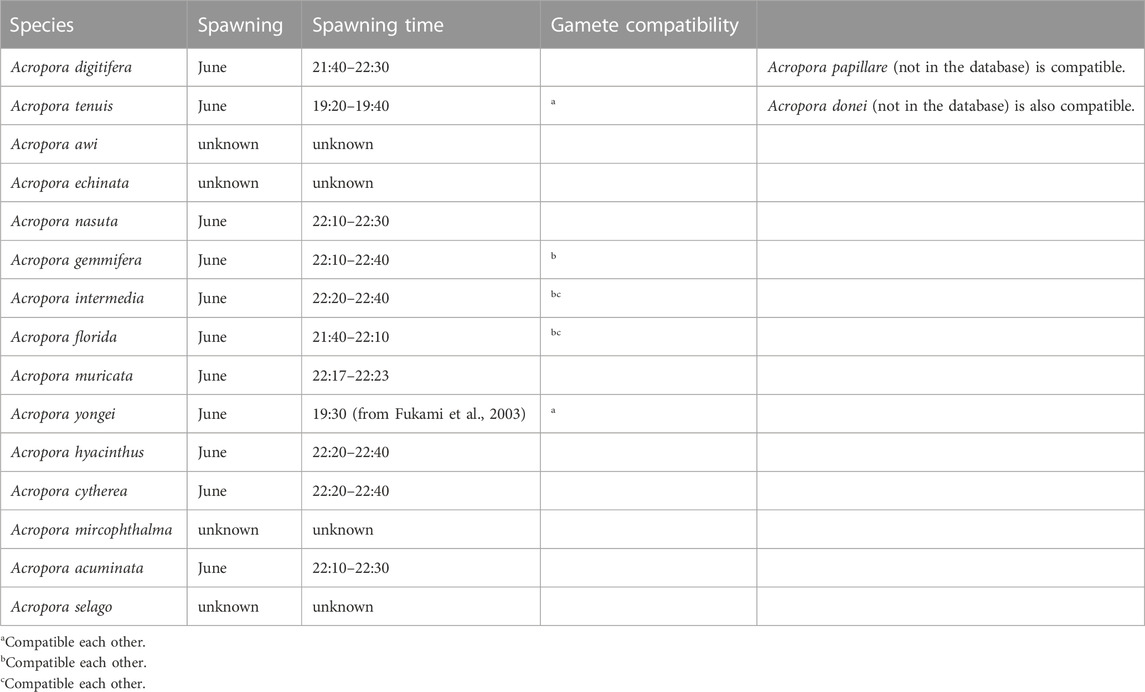
TABLE 2. Spawning synchronisms and gamete compatibility in the Acropora of the database of Orthoscope.
Two ADAM10 sequences were detected (XP_015778638.1, XP_015778639.1), but only one ADAM10 (XP_015778639.1) supported the branch-site model according to the species-specific fertilization mechanism. The loci of these two genes overlapped, but positive selection sites of ADAM10 (XP_015778639.1) were localized on a distinctive region of the genome (Figure 1).
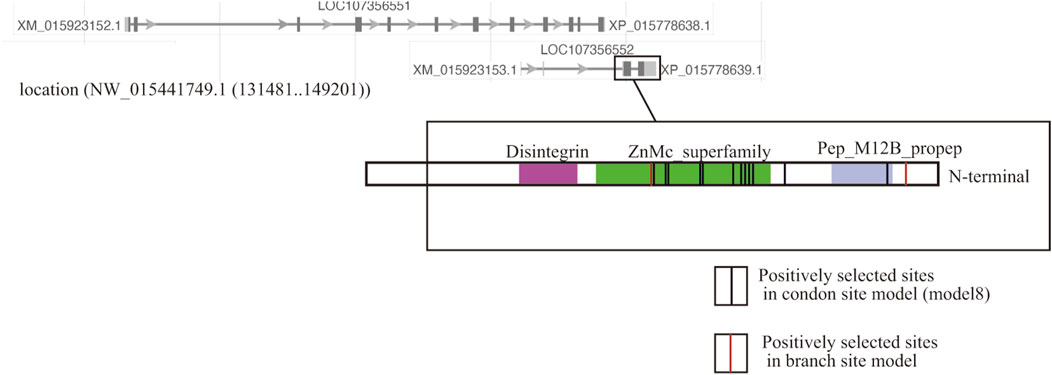
FIGURE 1. Localization of two copies of ADAM10 on the genome of the coral Acropora digitifera. Of the two ADAM10 sequences (XP_015778638.1, XP_015778639.1), the substitution rates of the several limited amino acid sites in one copy (XP_015778639.1) was accelerated. The red lines show codon sites in the Bayes empirical Bayes (BEB) analyses in the branch site model, and the black lines indicate the codon site model (Table 1).
3.3 Involvement of the branch-site supported integrins, ADAMs, and tetraspanins in fertilization
We developed antibodies against the proteins (integrin α9, ADAM10, ADAM17, and tetraspanin 4), for which the substitution rates of the several amino acid sites had been accelerated according to species-specific fertilization traits. We prepared antibodies against the antigen of each protein containing the positively selected sites (Figure 2A). Immunoblotting using each antibody suggested that ADAMs, integrins α9, and CD9 were present only in the eggs, but tetraspanin4 was localized in both sperm and eggs (Figure 2B). All of the bands corresponded the expected molecular mass from CDs sequences (ADAM10; 68 kDa, ADAM17; 94 kDa, Integrin α9; 110 kDa, tetraspanin 4; 26 kDa, CD9; 28 kDa). In anti-ADAM10 and integrin α9 antibodies, antibodies reacted only with proteins in A. digitifera eggs (Figure 2B). Immunohistochemistry also showed that ADAM10 was localized on the surface of the oocytes (Figure 2C). ADAM17 localized both oocytes and strong signal was found on the surface of the oocytes like ADAM10 and integrin α9. CD9 were mainly found in the oocytes but tetraspanin 4 were stained many tissues including oocytes.
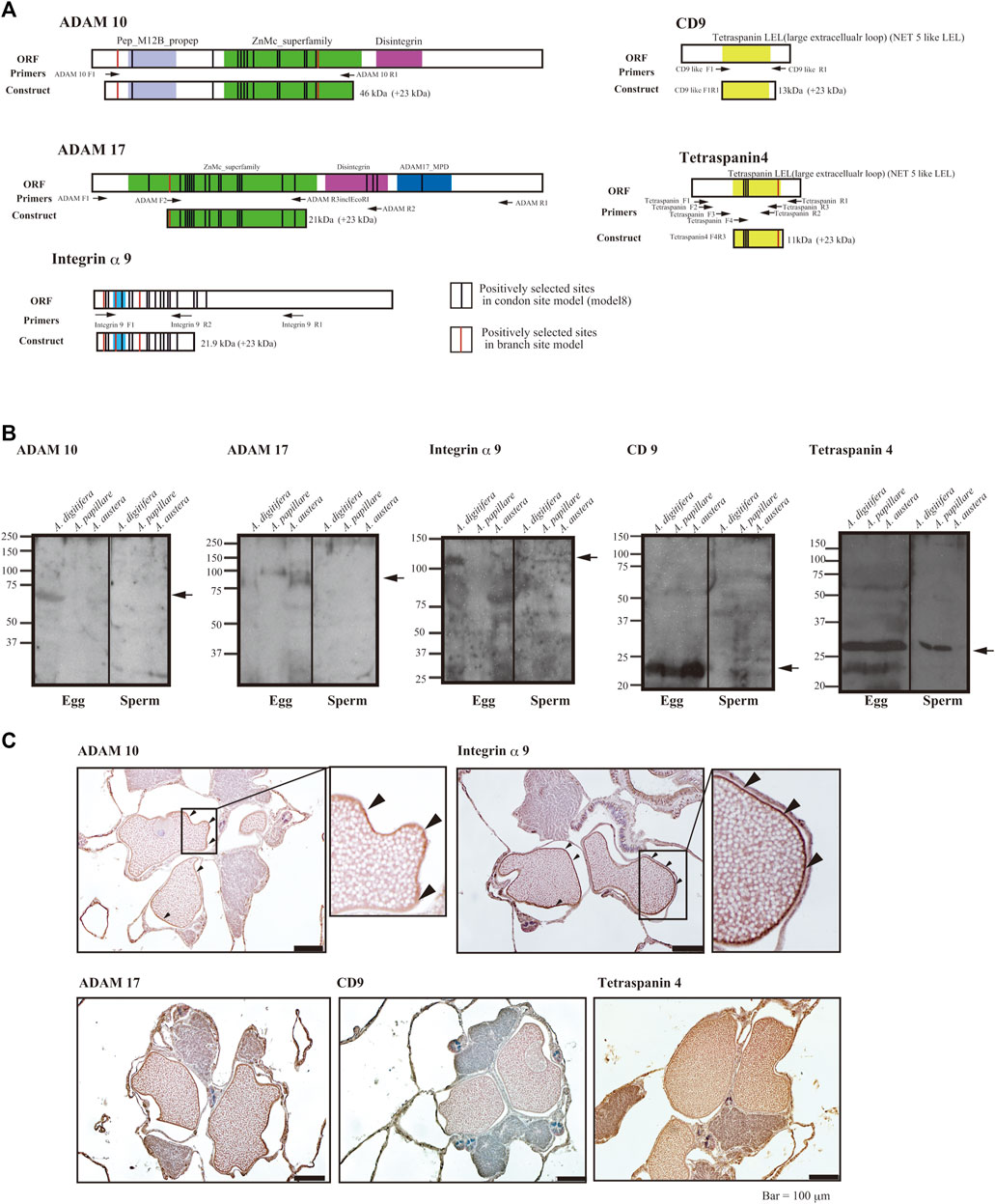
FIGURE 2. (A) Functional domains and positively selected sites of integrin α9, tetraspanins, ADAMs, and region(s) of antigen for antibody generation, (B) immunoblot analyses, and (C) immunohistology with the antibodies against sperm and eggs of the coral Acropora. (A) Functional domains of open reading frames, location of primers, and positively selected sites are indicated (red indicates the branch site model and black, the codon site model). Upper panels are hydropathy plots of deduced amino acid sequences. (B) Immunoblot analyses with eggs or sperm of A. digitifera, A. papillare, and A. austera. For tetraspanin 4 and CD9, 10% acrylamide gel was used to separate the proteins, and 7.5% gel was used for ADAMs and integrin α9. (C) Localization of ADAM10, ADAM17, Integrin α9, CD9, and Tetraspaning in about 1 week before the spawning of the coral A. digitifera. Arrowheads indicate the surface of oocytes. Bars = 100 μm.
Of the candidates that mediated species-specific fertilization, only antibodies against ADAM10 strongly inhibited fertilization in A. digitifera (Figure 3A; vs. control, p < 0.001). ADAM17 slightly inhibited fertilization in A. digitifera (Figure 3A; vs. control, p < 0.05). Other antibodies against integrin α9, tetraspanin4, and CD9 did not inhibit fertilization in any tested species, including A. papillare (Figure 3A; p > 0.05). The positively selected sites of ADAM10 in Acropora, including A. papillare, were different (Figure 3B). As a control, we did intercross experiments between A. digitifera and A. papillare, showing intercrossing but not fully compatible (Supplementary Figure S2A). In the A. austera, we could succeed with experiments only with one combination. We thus could not conclude anything from the results (Supplementary Figure S2B). However, the fertilization was not suppressed with antibodies except CD9. The gametes of all colonies did not show self-fertilization as a negative control (Figure 3A).
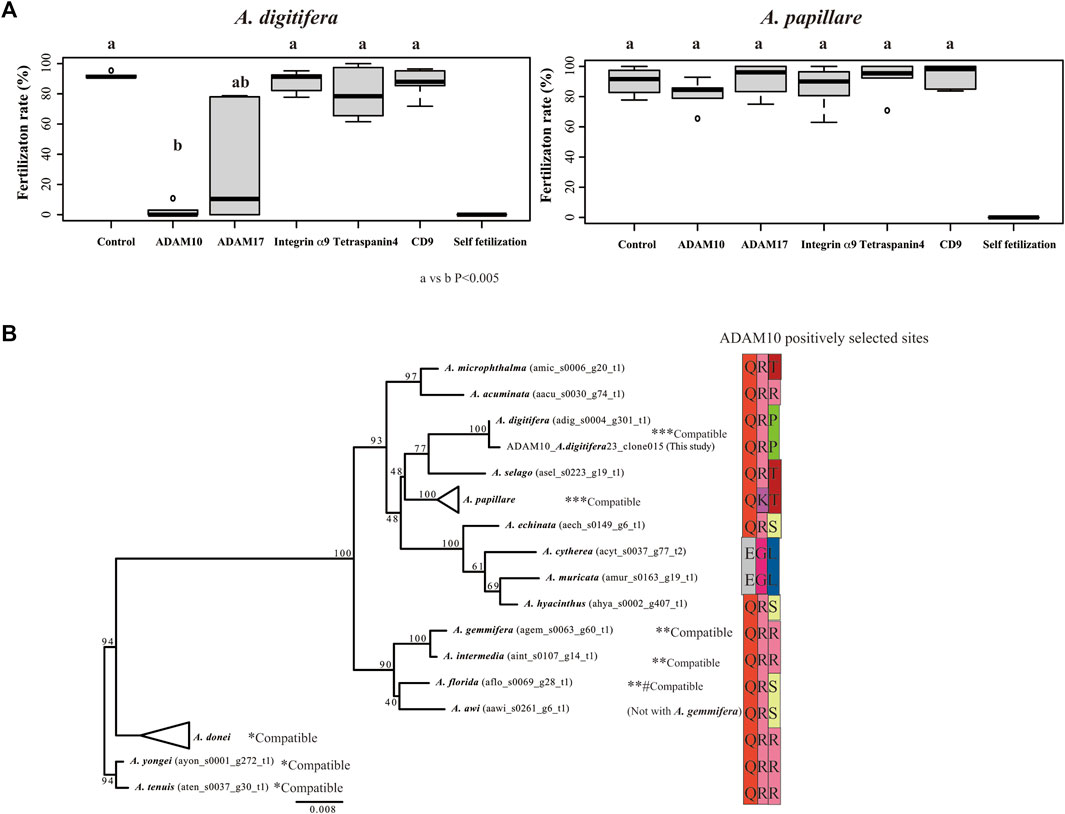
FIGURE 3. Fertilization ratio in the presence of antibodies against tetraspanins, integrin α9, and ADAMs. Fertilization in the presence of 10 μL of each antibody (1 mg/mL IgG) in 1 mL seawater (finally 10 μg/mL) was observed in gametes from Acropora digitifera and A. papillare. (A) Fertilization was conducted at 106 sperm/mL conditions, and the mixture of the gametes was finished within 2 h of spawning. Six crosses using gametes from three colonies of each species (N = 6; three colonies from both A. digitifera and A. papillare). Self-fertilization was used as a negative control. (A) or (B) indicates a significant difference (Wilcoxon rank sum test) (B) Maximum likelihood (ML) phylogenetic tree of ADAM10 and sequences of positively selected sites (branch site analyses) among species. Gamete-compatible species are indicated with *, **, or ***.
4 Discussion
In this study, we examined the roles of integrins, ADAMs, and tetraspanins in Acropora fertilization. As shown in mammals, the roles of ADAMs and integrins are controversial (e.g., Evans, 2001; He et al., 2003). Integrin β1 has been reported to be involved in Acropora fertilization (Iguchi et al., 2007). The other integrins, ADAMs, and tetraspanins were found in the gametes of A. digitifera. In the presence of antibodies against ADAM10, fertilization in A. digitifera was inhibited; ADAM10 may therefore be associated with fertilization in A. digitifera.
Although ADAM10 has not been reported to be involved in fertilization, our results suggest that ADAM10 is partly associated with gamete species recognition in Acropora. Fertilization-related ADAMs (ADAM2, ADAM9, ADAM12, ADAM15, and ADAM23) (Evans, 2001) interact with integrins (alpha4beta3, alpha9beta1) (Vjugina et al., 2009; Desiderio et al., 2010). The differences in the architecture of eggs between coral and mice suggest that the functions of ADAMs differ among species. For example, ADAMs and integrins are suggested to contribute to gamete interaction only when the ZP is present in the eggs (Evans et al., 1997). In Acropora, there is no ZP, and ADAM10 was found only in the eggs; the interacting integrins were not found in the sperm. Antibodies against integrin β1 slightly inhibit fertilization (Iguchi et al., 2007), and thus, the involvement of integrin β1 should be carefully considered. Overall, ADAM10 may interact with proteins other than integrins. Further studies are required to identify the protein(s) that interact with ADAM10.
The positively selected codon site in ADAM10 differs among species. Although the ADAM10 antibody specifically inhibited A. digitifera fertilization, only the two positively selected codon sites differed between A. papillare and A. digitifera. In addition, only two positively selected codon sites may be insufficient to determine their fertilization specificity for more than 20 synchronous species (Baird et al., 2021) or 110 extant species. Therefore, whether ADAM10 governs fertilization specificity among at least 20 synchronous spawning species is questionable. In addition, the feasibility of intercrossing between A. digitifera and A. papillare provokes the hypothesis that other gamete-composing proteins also play a role in species recognition.
Positive selections for species recognition might have arisen in many gamete protein genes in the Acropora spp. (Table 1; Morita et al., unpublished data). Specific codon sites are positively selected to generate polymorphic regions for recognition. Gamete compatibility is often congruent with rates of molecular evolution (Zigler et al., 2005). In addition, the risk of hybridization could influence the rates of molecular evolution. For example, the mixture of gametes from congeneric species could be associated with the risk of hybridization. The rates of codon evolution become slower when gamete interactions among heterospecifics are rare (Geyer et al., 2020).
The fertilization specificity and spawning synchronicity of several species (e.g., A. awi and A. echinata) used in this study are unknown. Thus, these species were not set as “foreground” for branch site analyses. For expedience, these species with unidentified fertilization specificity, such as A. awi and A. echinate, were set as background sequences. Due to the inclusion of these ambiguous species, the branch site analyses possibly underestimate the positively selected sites, and the robustness of the analyses needs to be considered carefully.
Coral is a basal animal, and its fertilization mechanisms are likely different from those in mammals, except for the plasma membrane fusion between sperm and egg. During the membrane fusion of sperm and eggs, CD9 functions as a strong determinant of fertilization in the mouse pathway (Miyado et al., 2008). Therefore, antibodies against CD9 were expected to suppress fertilization in all examined species. In contrast to our prediction, the CD9 antibody did not suppress fertilization. We developed an antibody against A. digitifera CD9 using its long extracellular loop (LEL) region, which is suggested to be involved in sperm–egg fusion (Umeda et al., 2020). It is also possible that antibodies against A. digitifera CD9 do not react with the functional site of CD9. In addition, small vesicles containing CD9 are released during membrane fusion between sperm and eggs in mice (Miyado et al., 2008; Barraud-Lange et al., 2012), but coral eggs are filled with wax esters (Harii et al., 2007), which are too rigid to form vesicles.
Another possibility is that CD9 does not underlie membrane fusion between sperm and eggs of the coral Acropora. The partner of CD9, EWI-2 protein with an Ig domain (Stipp et al., 2001), was not found in the eggs. Although EWI-2 protein has not been identified by MS analysis due to non-registration of EWI-2 cDNA, treatment with an anti-CD9 antibody against its LEL region was presumably insufficient to suppress the membrane fusion process if CD9 worked together with the EWI-2 complex. Our preliminary data found that proteins with IgG domains may be related to fertilization, but whether a protein–IgG complex interacts with CD9 and facilitates membrane fusion is unclear. To identify the differences between mammals and coral, the detailed pathway from sperm adhesion to membrane fusion should be investigated in Acropora.
The functions of the other tetraspanins are predicted to be different because differences in egg architecture are associated with differences in gamete adhesion pathways (e.g., Frolikova et al., 2019). Tetraspanins related to plasma membrane fusion (CD81 and CD151) were not found in the coral sperm. However, CD63, which functions as a primary adhesive of the cumulus cell layer in mammalian eggs, was found. In this study, we did not examine the role of CD63 because the positive selection of CD63 was not supported (Table 1). CD63 in mouse sperm is implicated in sperm–egg interactions via integrins (Frolikova et al., 2019). Functional investigation of other tetraspanins (including CD63) is needed to identify the evolution and differentiation of fertilization mechanisms through speciation.
Among the identified gamete proteins, the rapid evolution of integrin α9, ADAMs, and tetraspanin 4 is supported by molecular evolutionary analysis. Functional modifications might occur in these proteins, and their functions are potentially not limited to fertilization. In this study, although the immunostaining implies these proteins may be present in the many tissues in the corals, we did not investigate the localization of these proteins in other tissues. Therefore, their localization and roles in different tissues are still unclear. Their functions could be diverse and potentially useful in many tissues. Indeed, the ADAM10 and 17 localize many tissues and function in many aspects, such as the embryonic development process (Harrison et al., 2021). Although the function of integrins and ADAMs are questionable, integrins α9 forms complex with could interact with CD9 in the mouse (Zhu and Evans, 2002). Acropora has experienced climate change and is surviving. The rapid evolution of the proteins is presumably associated with the tolerance/resilience of the corals. However, further study is required.
In conclusion, we examined the roles of integrins, ADAMs, and tetraspanins in fertilization. As a result of this study, ADAM10 plausibly mediates species recognition. The ADAM10 antibody reacted only with A. digitifera, and thus, it still needs to be confirmed that ADAM10 governs species-specific fertilization in the other Acropora spp. In addition, positively selected sites in ADAM10 that arose during the acquisition of strict species recognition are limited. Overall, we predict more proteins are involved in species recognition, which is deeply associated with species boundaries in the coral Acropora.
Data availability statement
The datasets presented in this study can be found in online repositories. The names of the repository/repositories and accession number(s) can be found below: https://zenodo.org/deposit/6483451, 10.5281/zenodo.6483451.
Author contributions
MM conceived the study design, conducted all experiments, performed molecular evolutionary analyses, and wrote the paper. SK collected egg proteins for mass spectrometry analyses. KS and KI assisted in antibody preparation. All authors contributed critically to the drafts and gave final approval for publication.
Funding
This work was partly supported by the Project Foundation of the Tropical Biosphere Research Center of the University of the Ryukyus and JSPS KAKENHI (17K07414, 21H05304, and 22H02369 to MM; 21K15146 to SK).
Acknowledgments
We gratefully acknowledge the discursive inputs of Dr. K. Miyado and N. Kawano.
Conflict of interest
The authors declare that the research was conducted in the absence of any commercial or financial relationships that could be construed as a potential conflict of interest.
Publisher’s note
All claims expressed in this article are solely those of the authors and do not necessarily represent those of their affiliated organizations, or those of the publisher, the editors and the reviewers. Any product that may be evaluated in this article, or claim that may be made by its manufacturer, is not guaranteed or endorsed by the publisher.
Supplementary material
The Supplementary Material for this article can be found online at: https://www.frontiersin.org/articles/10.3389/fcell.2023.1171495/full#supplementary-material
References
Aagaard, J. E., Vacquier, V. D., Maccoss, M. J., and Swanson, W. J. (2010). ZP domain proteins in the abalone egg coat include a paralog of VERL under positive selection that binds lysin and 18-kDa sperm proteins. Mol. Biol. Evol. 27, 193–203. doi:10.1093/molbev/msp221
Arnaout, M. A., Goodman, S. L., and Xiong, J. P. (2007). Structure and mechanics of integrin-based cell adhesion. Curr. Opin. Cell Biol. 19, 495–507. doi:10.1016/j.ceb.2007.08.002
Baird, A., Guest, J., and Willis, B. (2009). Systematic and biogeographical patterns in the reproductive biology of scleractinian corals. Annu. Rev Ecol Evol Syst 40, 551–571. doi:10.1146/annurev.ecolsys.110308.120220
Baird, A. H., Guest, J. R., Edwards, A. J., Bauman, A. G., Bouwmeester, J., Mera, H., et al. (2021). An Indo-Pacific coral spawning database. Sci. Data 8, 35. doi:10.1038/s41597-020-00793-8
Barraud-Lange, V., Chalas Boissonnas, C., Serres, C., Auer, J., Schmitt, A., Lefevre, B., et al. (2012). Membrane transfer from oocyte to sperm occurs in two CD9-independent ways that do not supply the fertilising ability of Cd9-deleted oocytes. Reproduction 144, 53–66. doi:10.1530/REP-12-0040
Barraud-Lange, V., Naud-Barriant, N., Saffar, L., Gattegno, L., Ducot, B., Drillet, A. S., et al. (2007). Alpha6beta1 integrin expressed by sperm is determinant in mouse fertilization. BMC Dev. Biol. 7, 102. doi:10.1186/1471-213X-7-102
Carlisle, J. A., and Swanson, W. J. (2021). Molecular mechanisms and evolution of fertilization proteins. J. Exp. Zool. B Mol. Dev. Evol. 336, 652–665. doi:10.1002/jez.b.23004
Clark, N. L., Gasper, J., Sekino, M., Springer, S. A., Aquadro, C. F., and Swanson, W. J. (2009). Coevolution of interacting fertilization proteins. PLoS Genet. 5, e1000570. doi:10.1371/journal.pgen.1000570
Desiderio, U. V., Zhu, X., and Evans, J. P. (2010). ADAM2 interactions with mouse eggs and cell lines expressing α4/α9 (ITGA4/ITGA9) integrins: Implications for integrin-based adhesion and fertilization. PLoS One 5, e13744. doi:10.1371/journal.pone.0013744
Evans, J. P. (2001). Fertilin beta and other ADAMs as integrin ligands: Insights into cell adhesion and fertilization. Bioessays 23, 628–639. doi:10.1002/bies.1088
Evans, J. P., Schultz, R. M., and Kopf, G. S. (1997). Characterization of the binding of recombinant mouse sperm fertilin alpha subunit to mouse eggs: Evidence for function as a cell adhesion molecule in sperm-egg binding. Dev. Biol. 187, 94–106. doi:10.1006/dbio.1997.8612
Frolikova, M., Valaskova, E., Cerny, J., Lumeau, A., Sebkova, N., Palenikova, V., et al. (2019). Addressing the compartmentalization of specific integrin heterodimers in mouse sperm. Int. J. Mol. Sci. 20, 1004. doi:10.3390/ijms20051004
Fukami, H., Omori, M., Shimoike, K., Hayashibara, T., and Hatta, M. (2003). Ecological and genetic aspects of reproductive isolation by different spawning times in Acropora corals. Mar. Biol. 142, 679–684. doi:10.1007/s00227-002-1001-8
Galindo, B. E., Moy, G. W., Swanson, W. J., and Vacquier, V. D. (2002). Full-length sequence of VERL, the egg vitelline envelope receptor for abalone sperm lysin. Gene 288, 111–117. doi:10.1016/s0378-1119(02)00459-6
Galindo, B. E., Vacquier, V. D., and Swanson, W. J. (2003). Positive selection in the egg receptor for abalone sperm lysin. Proc. Natl. Acad. Sci. U. S. A. 100, 4639–4643. doi:10.1073/pnas.0830022100
Geyer, L. B., Zigler, K. S., Tiozzo, S., and Lessios, H. A. (2020). Slow evolution under purifying selection in the gamete recognition protein bindin of the sea urchin Diadema. Sci. Rep. 10, 9834. doi:10.1038/s41598-020-66390-2
Gowaty, P. A., Anderson, W. W., Bluhm, C. K., Drickamer, L. C., Kim, Y. K., and Moore, A. J. (2007). The hypothesis of reproductive compensation and its assumptions about mate preferences and offspring viability. Proc. Natl. Acad. Sci. U. S. A. 104, 15023–15027. doi:10.1073/pnas.0706622104
Harii, S., Nadaoka, K., Yamamoto, M., and Iwao, K. (2007). Temporal changes in settlement, lipid content and lipid composition of larvae of the spawning hermatypic coral Acropora tenuis. Mar. Ecol. Prog. Ser. 346, 89–96. doi:10.3354/meps07114
Harrison, N., Koo, C. Z., and Tomlinson, M. G. (2021). Regulation of ADAM10 by the TspanC8 family of tetraspanins and their therapeutic potential. Int. J. Mol. Sci. 22, 6707. doi:10.3390/ijms22136707
Harrison, R. G., and Larson, E. L. (2014). Hybridization, introgression, and the nature of species boundaries. J. Hered. 105 (1), 795–809. doi:10.1093/jhered/esu033
Hatta, M., Fukami, H., Wang, W., Omori, M., Shimoike, K., Hayashibara, T., et al. (1999). Reproductive and genetic evidence for a reticulate evolutionary history of mass-spawning corals. Mol. Biol. Evol. 16, 1607–1613. doi:10.1093/oxfordjournals.molbev.a026073
He, Z., Brakebusch, C., Fässler, R., Kreidberg, J. A., Primakoff, , and Myles, D. G. (2003). None of the integrins known to be present on the mouse egg or to be ADAM receptors are essential for sperm–egg binding and fusion. Dev. Biol. 254, 226–237. doi:10.1016/s0012-1606(02)00043-x
Hynes, R. O. (1987). Integrins: A family of cell surface receptors. Cell 48, 549–554. doi:10.1016/0092-8674(87)90233-9
Iguchi, A., Marquez, L. M., Knack, B., Shinzato, C., Van Oppen, M. J., Willis, B. L., et al. (2007). Apparent involvement of a beta1 type integrin in coral fertilization. Mar. Biotechnol. (NY) 9, 760–765. doi:10.1007/s10126-007-9026-0
Ihle, M., Kempenaers, B., and Forstmeier, W. (2015). Fitness benefits of mate choice for compatibility in a socially monogamous species. PLoS Biol. 13, e1002248. doi:10.1371/journal.pbio.1002248
Inoue, J., and Satoh, N. (2019). Orthoscope: An automatic web tool for phylogenetically inferring bilaterian orthogroups with user-selected taxa. Mol. Biol. Evol. 36, 621–631. doi:10.1093/molbev/msy226
Jankovicova, J., Neuerova, Z., Secova, P., Bartokova, M., Bubenickova, F., Komrskova, K., et al. (2020). Tetraspanins in mammalian reproduction: Spermatozoa, oocytes and embryos. Med. Microbiol. Immunol. 209, 407–425. doi:10.1007/s00430-020-00676-0
Jin, M., Fujiwara, E., Kakiuchi, Y., Okabe, M., Satouh, Y., Baba, S. A., et al. (2011). Most fertilizing mouse spermatozoa begin their acrosome reaction before contact with the zona pellucida during in vitro fertilization. Proc. Natl. Acad. Sci. U. S. A. 108, 4892–4896. doi:10.1073/pnas.1018202108
Kaji, K., Oda, S., Shikano, T., Ohnuki, T., Uematsu, Y., Sakagami, J., et al. (2000). The gamete fusion process is defective in eggs of Cd9-deficient mice. Nat. Genet. 24, 279–282. doi:10.1038/73502
Katoh, K., Rozewicki, J., and Yamada, K. D. (2019). MAFFT online service: Multiple sequence alignment, interactive sequence choice and visualization. Brief. Bioinform 20, 1160–1166. doi:10.1093/bib/bbx108
Knack, B. A., Iguchi, A., Shinzato, C., Hayward, D. C., Ball, E. E., and Miller, D. J. (2008). Unexpected diversity of cnidarian integrins: Expression during coral gastrulation. BMC Evol. Biol. 8, 136. doi:10.1186/1471-2148-8-136
Knowlton, N. (2000). Molecular genetic analyses of species boundaries in the sea. Hydrobiologia 420, 73–90. doi:10.1023/a:1003933603879
Lee, Y. H., Ota, T., and Vacquier, V. D. (1995). Positive selection is a general phenomenon in the evolution of abalone sperm lysin. Mol. Biol. Evol. 12, 231–238. doi:10.1093/oxfordjournals.molbev.a040200
Levitan, D. R., and Stapper, A. P. (2009). Simultaneous positive and negative frequency-dependent selection on sperm bindin, a gamete recognition protein in the sea urchin strongylocentrotus purpuratus. Evolution 64, 785–797. doi:10.1111/j.1558-5646.2009.00850.x
Levitan, D. R., Terhorst, C. P., and Fogarty, N. D. (2007). The risk of polyspermy in three congeneric sea urchins and its implications for gametic incompatibility and reproductive isolation. Evolution 61, 2007–2014. doi:10.1111/j.1558-5646.2007.00150.x
Merc, V., Frolikova, M., and Komrskova, K. (2021). Role of integrins in sperm activation and fertilization. Int. J. Mol. Sci. 22, 11809. [Online]. doi:10.3390/ijms222111809
Miller, B. J., Georges-Labouesse, E., Primakoff, P., and Myles, D. G. (2000). Normal fertilization occurs with eggs lacking the integrin alpha6beta1 and is CD9-dependent. J. Cell Biol. 149, 1289–1296. doi:10.1083/jcb.149.6.1289
Miyado, K., Yamada, G., Yamada, S., Hasuwa, H., Nakamura, Y., Ryu, F., et al. (2000). Requirement of CD9 on the egg plasma membrane for fertilization. Science 287, 321–324. doi:10.1126/science.287.5451.321
Miyado, K., Yoshida, K., Yamagata, K., Sakakibara, K., Okabe, M., Wang, X., et al. (2008). The fusing ability of sperm is bestowed by CD9-containing vesicles released from eggs in mice. Proc. Natl. Acad. Sci. U. S. A. 105, 12921–12926. doi:10.1073/pnas.0710608105
Morita, M., Kitanobo, S., Nozu, R., Iwao, K., Fukami, H., and Isomura, N. (2019). Reproductive strategies in the intercrossing corals Acropora donei and A. tenuis to prevent hybridization. Coral Reefs 38, 1211–1223. doi:10.1007/s00338-019-01839-z
Morita, M., Nishikawa, A., Nakajima, A., Iguchi, A., Sakai, K., Takemura, A., et al. (2006). Eggs regulate sperm flagellar motility initiation, chemotaxis and inhibition in the coral Acropora digitifera, A. gemmifera and A. tenuis. J. Exp. Biol. 209, 4574–4579. doi:10.1242/jeb.02500
Palumbi, S. R. (2009). Speciation and the evolution of gamete recognition genes: Pattern and process. Heredity 102, 66–76. doi:10.1038/hdy.2008.104
Ravaux, B., Favier, S., Perez, E., and Gourier, C. (2018). Egg CD9 protein tides correlated with sperm oscillations tune the gamete fusion ability in mammal. J. Mol. Cell Biol. 10, 494–502. doi:10.1093/jmcb/mjy005
Smith, M. D., Wertheim, J. O., Weaver, S., Murrell, B., Scheffler, K., and Kosakovsky Pond, S. L. (2015). Less is more: An adaptive branch-site random effects model for efficient detection of episodic diversifying selection. Mol. Biol. Evol. 32, 1342–1353. doi:10.1093/molbev/msv022
Stamatakis, A. (2006). RAxML-VI-HPC: Maximum likelihood-based phylogenetic analyses with thousands of taxa and mixed models. Bioinformatics 22, 2688–2690. doi:10.1093/bioinformatics/btl446
Stipp, C. S., Kolesnikova, T. V., and Hemler, M. E. (2001). EWI-2 is a major CD9 and CD81 partner and member of a novel Ig protein subfamily. J. Biol. Chem. 276, 40545–40554. doi:10.1074/jbc.M107338200
Suzuki, G., Keshavmurthy, S., Hayashibara, T., Wallace, C. C., Shirayama, Y., Chen, C. A., et al. (2016). Genetic evidence of peripheral isolation and low diversity in marginal populations of the Acropora hyacinthus complex. Coral Reefs 35, 1419–1432. doi:10.1007/s00338-016-1484-2
Swanson, W. J., Nielsen, R., and Yang, Q. (2003). Pervasive adaptive evolution in mammalian fertilization proteins. Mol. Biol. Evol. 20, 18–20. doi:10.1093/oxfordjournals.molbev.a004233
Swanson, W. J., and Vacquier, V. D. (2002). The rapid evolution of reproductive proteins. Nat. Rev. Genet. 3, 137–144. doi:10.1038/nrg733
Talbot, P., and Dandekar, P. (2003). Perivitelline space: Does it play a role in blocking polyspermy in mammals? Microsc. Res. Tech. 61, 349–357. doi:10.1002/jemt.10348
Team, R. C. (2020). R: A language and environment for statistical computing. Vienna, Austria: R Foundation for Statistical Computing.
Umeda, R., Satouh, Y., Takemoto, M., Nakada-Nakura, Y., Liu, K., Yokoyama, T., et al. (2020). Structural insights into tetraspanin CD9 function. Nat. Commun. 11, 1606. doi:10.1038/s41467-020-15459-7
Vacquier, V. D., and Lee, Y. H. (1993). Abalone sperm lysin: Unusual mode of evolution of a gamete recognition protein. Zygote 1, 181–196. doi:10.1017/s0967199400001465
Vjugina, U., Zhu, X., Oh, E., Bracero, N. J., and Evans, J. P. (2009). Reduction of mouse egg surface integrin alpha9 subunit (ITGA9) reduces the egg's ability to support sperm-egg binding and fusion. Biol. Reprod. 80, 833–841. doi:10.1095/biolreprod.108.075275
Willis, B. L., Van Oppen, M. J. H., Miller, D. J., Vollmer, S. V., and Ayre, D. J. (2006). The role of hybridization in the evolution of reef corals. Ann. Rev. Ecol. Evol. Syst. 37, 489–517. doi:10.1146/annurev.ecolsys.37.091305.110136
Yanagimachi, R. (1994). “Mammalian fertilization,” in The physiology of reproduction. Editors E. Knobil, and J. D. Neill (NEW YORK: Raven Press), 189–317.
Yang, Z. (1997). Paml: A program package for phylogenetic analysis by maximum likelihood. Bioinformatics 13, 555–556. doi:10.1093/bioinformatics/13.5.555
Yang, Z., Wong, W. S., and Nielsen, R. (2005). Bayes empirical bayes inference of amino acid sites under positive selection. Mol. Biol. Evol. 22, 1107–1118. doi:10.1093/molbev/msi097
Zhang, J., Nielsen, R., and Yang, Z. (2005). Evaluation of an improved branch-site likelihood method for detecting positive selection at the molecular level. Mol. Biol. Evol. 22, 2472–2479. doi:10.1093/molbev/msi237
Zhu, X., and Evans, J. P. (2002). Analysis of the roles of RGD-binding integrins, alpha(4)/alpha(9) integrins, alpha(6) integrins, and CD9 in the interaction of the fertilin beta (ADAM2) disintegrin domain with the mouse egg membrane. Biol. Reprod. 66, 1193–1202. doi:10.1095/biolreprod66.4.1193
Zigler, K. S., Mccartney, M. A., Levitan, D. R., and Lessios, H. A. (2005). Sea urchin bindin divergence predicts gamete compatibility. Evolution 59, 2399–2404. doi:10.1554/05-098.1
Ziyyat, A., Rubinstein, E., Monier-Gavelle, F., Barraud, V., Kulski, O., Prenant, M., et al. (2006). CD9 controls the formation of clusters that contain tetraspanins and the integrin alpha 6 beta 1, which are involved in human and mouse gamete fusion. J. Cell Sci. 119, 416–424. doi:10.1242/jcs.02730
Keywords: Acropora coral, ADAM10, positive selection, fertilization, species recognition, synchronous spawning
Citation: Morita M, Kitanobo S, Ohki S, Shiba K and Inaba K (2023) Positive selection on ADAM10 builds species recognition in the synchronous spawning coral Acropora. Front. Cell Dev. Biol. 11:1171495. doi: 10.3389/fcell.2023.1171495
Received: 22 February 2023; Accepted: 10 April 2023;
Published: 20 April 2023.
Edited by:
Takuji Ishikawa, Tohoku University, JapanReviewed by:
Ryusaku Deguchi, Miyagi University of Education, JapanJohn H. Henson, Dickinson College, United States
Copyright © 2023 Morita, Kitanobo, Ohki, Shiba and Inaba. This is an open-access article distributed under the terms of the Creative Commons Attribution License (CC BY). The use, distribution or reproduction in other forums is permitted, provided the original author(s) and the copyright owner(s) are credited and that the original publication in this journal is cited, in accordance with accepted academic practice. No use, distribution or reproduction is permitted which does not comply with these terms.
*Correspondence: Masaya Morita, bW9yaXRhQGxhYi51LXJ5dWt5dS5hYy5qcA==