- 1Laboratory of Odontogenesis and Osteogenesis, Institute of Animal Physiology and Genetics, v.v.i, Academy of Sciences, Brno, Czechia
- 2Department of Physiology, University of Veterinary Sciences, Brno, Czechia
- 3Institute of Cancer and Genomic Sciences, University of Birmingham, Birmingham, United Kingdom
- 4Department of Experimental Biology, Faculty of Science, Masaryk University, Brno, Czechia
- 5International Clinical Research Center, St. Anne’s University Hospital, Brno, Czechia
As the dentition forms and becomes functional, the alveolar bone is remodelled. Metalloproteinases are known to contribute to this process, but new regulators are emerging and their contextualization is challenging. This applies to Myb, a transcription factor recently reported to be involved in bone development and regeneration. The regulatory effect of Myb on Mmps expression has mostly been investigated in tumorigenesis, where Myb impacted the expression of Mmp1, Mmp2, Mmp7, and Mmp9. The aim of this investigation was to evaluate the regulatory influence of the Myb on Mmps gene expression, impacting osteogenesis and mandibular bone formation. For that purpose, knock-out mouse model was used. Gene expression of bone-related Mmps and the key osteoblastic transcription factors Runx2 and Sp7 was analysed in Myb knock-out mice mandibles at the survival limit. Out of the metalloproteinases under study, Mmp13 was significantly downregulated. The impact of Myb on the expression of Mmp13 was confirmed by the overexpression of Myb in calvarial-derived cells causing upregulation of Mmp13. Expression of Mmp13 in the context of other Mmps during mandibular/alveolar bone development was followed in vivo along with Myb, Sp7 and Runx2. The most significant changes were observed in the expression of Mmp9 and Mmp13. These MMPs and MYB were further localized in situ by immunohistochemistry and were identified in pre/osteoblastic cells as well as in pre/osteocytes. In conclusion, these results provide a comprehensive insight into the expression dynamics of bone related Mmps during mandibular/alveolar bone formation and point to Myb as another potential regulator of Mmp13.
1 Introduction
Matrix metalloproteinases (Mmps) are enzymes involved in many biological processes such as morphogenesis, angiogenesis, and wound healing, as well as related disorders including atheroma, arthritis, ulceration, and cancer (Visse and Nagase, 2003). Mmps have been recently recognized as biomarkers in several fields, including diagnosis or monitoring of treatment efficacy (Laronha and Caldeira, 2020). Mmps can be categorized into five types through bioinformatic analysis. Additionally, they can be subdivided based on substrate specificity, sequential similarity, domain organization structure, and biochemical properties, resulting in collagenases (incl. Mmps 1, 8, 13), gelatinases (incl. Mmps 2, 9), stromelysins (incl. Mmps 3, 10, 11), matrilysins (incl. Mmp 7), membrane-bound Mmps (incl. Mmp 14, 15, 16) and others (Page-McCaw et al., 2007; Young et al., 2019). Despite the relatively broad knowledge about Mmps, further research will be necessary to investigate the complexity of their regulation and activity. This applies also for osteogenesis, where Mmps play an important role in bone formation and remodelling (Fernandez-Patron et al., 2011). Notably, Mmp2, Mmp9, Mmp13, Mmp14 and Mmp16 have been reported as the most functionally important bone-associated Mmps in osteogenesis (Liang et al., 2016). Furthermore, Mmp2, Mmp9 and Mmp13 have also been emphasized in bone regeneration efforts (Khoswanto, 2023).
Several transcription factors known to regulate Mmps in bone have been identified, such as Sp7 and Runx2 (Zhang et al., 2012; Bruderer et al., 2014) but other potential candidates also exist. One of them is Myb, a protooncogene widely studied in cancerogenesis, haematopoiesis, and related disorders (Cicirò and Sala, 2021). Originally, Myb was associated with proliferating and undifferentiated cells but later it was identified also in differentiated cells (Ess et al., 1999; Soza-Ried et al., 2010). The latter case applies also for physiological chondrogenesis and osteogenesis. Myb was reported as a regulator of osteoclastogenesis via the system Rankl/Opg (Takanche et al., 2018; Kodric et al., 2019) and of the extracellular matrix (ECM) production (Matalova et al., 2011; Oralova et al., 2015; 2017).
Given that Mmps play a pivotal role in the remodelling of the bone extracellular matrix, and considering Myb regulates ECM factors, it is plausible that Mmps may be involved in the osteogenic effects by Myb. The background for such an expectation arises from observations made in cancer cells (Bhattarai et al., 2011; Knopfova et al., 2012; Xu et al., 2019), but have not yet been investigated in osteogenesis.
Osteogenesis includes a tight interplay among the key bone cell types: osteoblasts, osteocytes, and osteoclasts. Consequently, investigating osteogenesis in vivo is highly favoured. Therefore, we took advantage of the Myb knock-out mouse model to test the hypothesis that Myb could impact the expression of Mmps during bone formation and remodelling. Unfortunatelly, the survival of Myb knock-out is limited by the prenatal day 15 (Mucenski et al., 1991). At this point, long bones are only at the chondrogenic stage while the intramembranous mandibular/alveolar bone already contains all three bone cell types (reviewed in Svandova et al., 2020).
The analysis of Mmps mRNA expression (Mmps 1, 2, 3, 7, 8, 9, 10, 11, 12, 13, 14, 15 and 16) was performed on Myb knock-out mandibular samples and was followed by overexpression experiments in a calvaria-derived osteoblastic cells in vitro. Additionally, complex dynamics of Mmps expression at the onset of mandibular/alveolar bone formation (around E15) was provided to fill in missing gaps in mandibular/alveolar bone development characterization. The localization of MYB protein and selected MMPs (MMP9 and MMP13) in the forming mandibular/alveolar bone was performed by immunohistochemistry (IHC) to assess their temporo-spatial pattern.
2 Materials and methods
2.1 Animals
Wild type mice (strain CD1) were purchased from the Breeding Units of Masaryk University, Brno, Czech Republic. Heads corresponding to the embryonic days 13, 14, 15, 18 (E13, E14, E15, E18) and postnatal day (P2) were collected. The work with laboratory animals in the CR, including euthanasia, is regulated by Act No. 359/2012. According to the paragraph 3t), post mortem collection of organs and tissues is not considered as an experiment.
Myb-deficient mice (Mucenski et al., 1991 on a CB7BL/6 background) were maintained at the Institute of Cancer and Genomic Sciences, University of Birmingham, United Kingdom. Heads of wild type (WT) or knock-out (KO) embryos were collected prior to their survival limit at E15. The work with laboratory animals in Birmingham was performed under the terms of a UK Home Office licence according to the Animals (Scientific Procedures) Act, 1986.
2.2 Separation of the molar region
Mouse mandibles at E13, 14, 15, and 18 (CD1 strain) were dissected from freshly collected samples. The molar region together with the alveolar bone were removed from mandibles using micromanipulation (as described in Vesela et al., 2019). At least 3 embryos were used for each stage, and both molar regions (right and left sides) were dissected from each mandible. The tissues were lysed in the RLT buffer (Qiagen) with 1% 2-mercaptoethanol (Sigma-Aldrich, M3148-100 ML) and used for the subsequent isolation of RNA.
Mandibular molar regions of the wild type (WT) and Myb knock-out (KO) mouse embryos (survival limit E15) were dissected. At least 3 embryos were used for WT and KO with both molar regions (right and left sides). Dissected samples were prepared for RNA extraction and subsequent qRT-PCR analysis.
2.3 Cell line
Murine calvaria cells were obtained from the calvaria of neonatal CD1 mice 2 days after birth by sequential collagenase digestion at 37°C with minor changes in protocol described in Garcia et al. (2002). Samples from 12 mice were used for calvarial cell isolation to obtain a sufficient amount of cells. Briefly, for transfection experiments, the calvaria-derived cells were cultured until 80% confluence and the proliferation medium was replaced by a differentiation medium (α-MEM containing 10% FBS, 2 mM glutamine, 50 μg/mL ascorbic acid, and 10 mM β-glycerolphosphate). The culture medium was replaced every 3 days. The biological samples were collected at 24 h and 14 days for investigation.
2.4 Over expression of Myb in calvaria cells
The murine calvaria cells were transfected with the Myb-expressing vector (pcDNA3-mcMYB) or control vector (pcDNA-3), as was described earlier in Oralova et al., 2015; Oralova et al., 2017. The plasmids were added at day 0 and 7, respectively in the concentration of 100 ng DNA per 10 µL of cells using the FuGENE HD 6 transfection reagent (Cat. No. E2311, Promega, United States). The medium was changed every 2–4 days, cultures were collected at 24 h and 14 days.
2.5 qRT-PCR
Total RNA was extracted from the molar part region, calvaria and IDG-SW3 cells using the RNeasy Mini Kit (Qiagen, Germany). RNA concentration and purity were assessed using a NanoDrop and cDNA was synthesized using the reverse Master Mix (Generi Biotech, Czech Republic). For cDNA synthesis, 500 ng of RNA was used, and biological replicates of each sample were diluted to a concentration of 100 ng/μL before performing a qRT-PCR reaction. The qRT-PCR was performed in a final reaction volume of 10 μL containing the one-step Ideal PCR Master Mix (Generi Biotech, Czech Republic) using Light-Cycler 96 (Roche, Switzerland) with preheating to 95 °C for 10 min. This was followed by 40 cycles of 95 C/15 s and 62.5°C/1 min with mRNA probes for Mmp1a, Mmp1b, Mmp2, Mmp3, Mmp7, Mmp8, Mmp9, Mmp10, Mmp11, Mmp12, Mmp13, Mmp14, Mmp15, Mmp16, Myb, Runx2 and Sp7 (Mouse Mmp1a, Mm00473485_m1; Mmp1b, Mm00473493_g1; Mmp2, Mm00439498_m1; Mmp3, Mm00440295_m1; Mmp7, Mm00487724_m1; Mmp8, Mm00439509_m1; Mmp9, Mm00442991; Mmp10, Mm01168399_m1; Mmp11, Mm00485048_m1; Mmp12, Mm00500554_m1; Mmp13, Mm00439491_m1; Mmp14, Mm00485054_m1; Mmp15, Mm00485062_m1; Mmp16, Mm01210646_m1; Myb, Mm00501741_m1; Runx2 Mm00501584_m1; Sp7 Mm00504574_m1 TaqMan Gene expression Assay, Thermo Fisher Scientific, United States). Expression levels were calculated using the ΔΔCT method, with normalization against β−actin RNA levels (mouse Actb, Mm02619580_g1, TaqMan Gene Expression Assay, Thermo Fisher Scientific, United States).
2.6 Histology
Mouse heads at E13, 14, 15, and 18 (CD1 strain) were dissected, fixed in 4% buffered paraformaldehyde, dehydrated in ethanol series, treated with xylene, and embedded in paraffin. Histological sections were processed for haematoxylin-eosin (H&E) staining and immunohistochemistry (IHC). Wild type (WT) and Myb knock-out (KO) mouse embryos were processed in the same way and prepared for histological analyses (haematoxylin-eosin; haematoxylin-eosin-alcian blue; Masson’s green trichrome; Weigert van Gieson; Von Kossa and Alizarin red).
2.7 Immunohistochemistry
Serial frontal histological sections (5 μm) were prepared for IHC staining. Citrate solution was used as a pre-treatment (5 min/98°C). Endogenous peroxidases were inhibited using 3% hydrogen peroxide for 5 min/RT. Blocking serum (Vectastain Elite ABC Kit, Rabbit Ig, PK-6101, Vector Laboratories, United States) was applied for 20 min/RT, primary antibodies against MYB (anti-MYB, 1:300, ab226470, Abcam), MMP9 (anti-MMP9, 1:100, PA5-13199, Thermo Scientific) and MMP13 (anti-MMP13, 1:100, ab39012, Abcam) were diluted in DAKO antibody diluent (Dako, S3022, North America) and applied for 1 h/RT. The secondary antibodies and avidin-biotin complex (Vectastain Elite ABC Kit, Rabbit Ig, PK-6101, Vector Laboratories, United States) were applied each for 30 min/RT. Tissue antigens were visualised by DAB (diaminobenzidine) chromogen substrate (Liquid DAB + Substrate, K3468, Dako North America, United States). Counterstaining was achieved using haematoxylin.
2.8 Myb-binding consensus sequences
The sequence of the Mmp13 promoter region was scanned for the presence of potential Myb-binding sites using a consensus motif of YAACT/(C)/GGYCR derived by selection of oligodeoxynucleotides bound to mouse Myb protein in vitro (Howe and Watson, 1991).
2.9 Statistical analysis
All results were expressed as mean ± standard deviations (SD) of four samples for each time point and compared using an unpaired t-test (unpaired t-test, two-tailed, significance level = 0.05) and one-way ANOVA (One-way analysis of variance, Tukey test: Compare all pairs of columns, Significant level = 0.05). Differences were considered as significant at p < 0.05; p < 0.01; p < 0.001 and are indicated by *; **; *** symbols, respectively.
3 Results
3.1 Expression of bone-associated Mmps, Sp7, and Runx2 in the Myb-deficient mandible
First, the expression profile of bone-related Mmps was compared in Myb-deficient and wild type mouse mandibles (prior to the survival limit around the E15). Among investigated Mmps, Mmp13 was significantly downregulated.
The expression of all Mmps (Mmp1a, Mmp1b, Mmp2, Mmp3, Mmp7, Mmp8, Mmp9, Mmp10, Mmp11, Mmp12, Mmp13, Mmp14, Mmp15, and Mmp16) were detected in wild-type versus Myb knock-out embryos using qPCR. Transcripts of Mmp1a, Mmp1b, Mmp3, Mmp7, Mmp8, Mmp10, and Mmp12 were not detected. The expression of Mmp2, Mmp9, Mmp11, Mmp14, Mmp15, and Mmp16 was not significantly altered in Myb knock-out tissues compared to the wild-type (shown in Figures 1A–C,E–I). Mmp13 expression was significantly decreased in Myb mutant embryos (0.1-fold change compared to wild type, shown in Figure 1D).
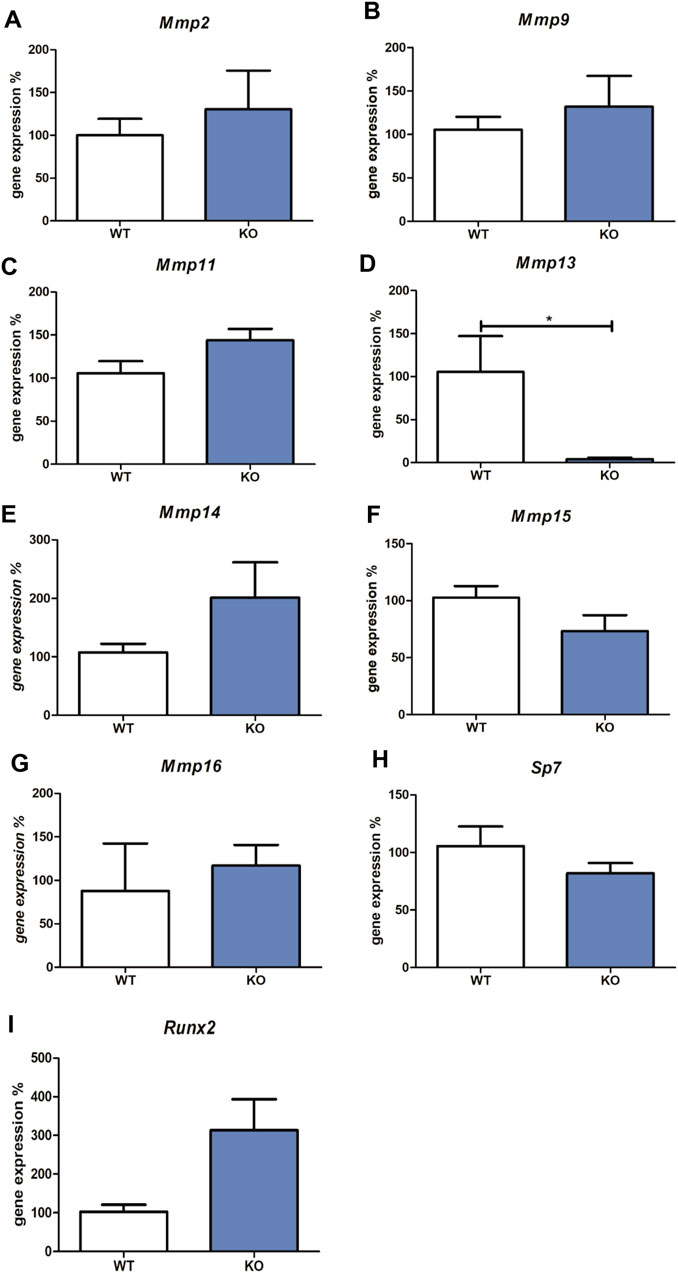
FIGURE 1. Expression of Mmp2, Mmp9, Mmp11, Mmp13, Mmp14, Mmp15, Mmp16, Sp7 and Runx2 mRNAs. The graph shows expression of mRNAs corresponding to Mmp2 (Panel A), Mmp9 (Panel B), Mmp11 (Panel C), Mmp13 (Panel D), Mmp14 (Panel E), Mmp15 (Panel F), Mmp16 (Panel G), Sp7 (Panel H), and Runx2 (Panel I) in tissues derived from wild type (WT) compared to Myb deficient mice (KO), detected by qRT-PCR. Data are expressed as mean ± SD (n—4 per group and statistically significant differences are highlighted (unpaired t-test, two-tailed, *p < 0.05).
Since the most well-known regulators of Mmp13 expression are Sp7 and Runx2, expression levels of these two factors were analysed in Myb-deficient versus wild type samples. Osteoblastic Sp7 and Runx2 transcription factors mRNA levels were not significantly altered in Myb knock-out tissues compared to the wild-type (shown in Figures 1H,I).
Histological analysis of the mandibular/alveolar bone in Myb-deficient mice did not show major differences (Supplementary Figure S1), however Myb-deficient mice tend to be smaller than their littermates.
3.2 Myb over expression in osteoblastic cells upregulates Mmp13 transcription
At the survival limit of the Myb-deficient mice, osteoblasts are the major population of cells within the mandible. Therefore, osteoblastic cells of intramembranous origin (isolated from calva, described in Material and Methods) were used to confirm the opposite effect of Myb on Mmp13 expression. Over expression was performed in two experimental settings (Supplementary Figure S1A,B). The immediate effect of Myb over expression was evaluated after 24 h, the long-term effect after 14 days.
The Mmp13 expression significantly increased in both cases, 24 h (1.4-fold change compared to control, shown in Figure 2A) and 14 days (2.8-fold change compared to control, shown in Figure 2B). The expression of osteoblastic Sp7 (Figure 2C) and Runx2 (Figure 2D) transcription factors was not significantly altered in the Myb over expressing calvaria cell line.
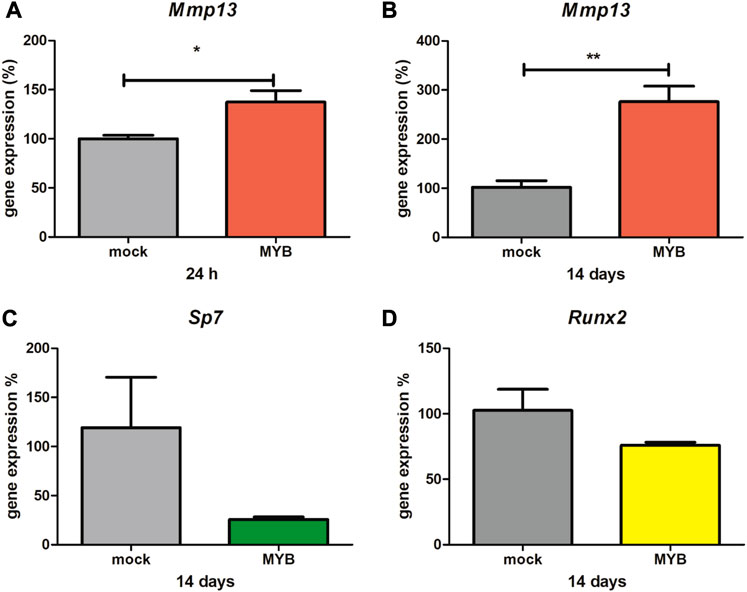
FIGURE 2. Mmp13, Sp7 and Runx2 expression in overexpressed calvaria cell line. The graph shows calvaria cells, treated by Myb vector (MYB) and empty vector (mock), expression of Mmp13 at 24 h (Panel A) and 14 days (Panel B), expression of Sp7 at 14 days (Panel C) and expression of Runx2 at 14 days (Panel D). Data are expressed as mean ± SD (n—4 per group) and statistically significant differences are highlighted (unpaired t-test, two-tailed, *p < 0.05; **p < 0.01).
Therefore, we looked for the presence of potential Myb-binding sequence motifs (MBS) in the Mmp13 promoter, using the consensus sequence YAACT/(C)/GGYCR (Supplementary Figure S2). Taking the YAAC element of the consensus motif as being essential, 2 sequences with a 7 out of 9 match can be seen, with a further three motifs with a 6 out of nine alignments.
3.3 The expression profile of Mmps, Myb, Sp7, and Runx2 in the molar part region during mouse prenatal development
In order to investigate the expression profile of bone-related Mmps, Sp7 and Runx2 in mandibular development in vivo, stages around the survival limit of the Myb knock-out mice were examined. The prenatal stages E13-E15 correspond to the onset of mandibular bone formation when osteoblasts, osteoclasts, and osteocytes keep appearing. By day 18, all three major cell populations become established.
qRT-PCR was used to provide the expression data for Mmp2, Mmp9, Mmp11, Mmp13, Mmp14, Mmp15, and Mmp16. As in the case of the Myb knock-out and corresponding wild type samples, expression of Mmp1a, Mmp1b, Mmp3, Mmp7, Mmp8, Mmp10, and Mmp12, was not detected in the molar area at any of the time points investigated.
Runx2, as a positive regulator of osteoblasts, is highly expressed at E14 (Figure 3A), when the first osteoblasts appear and then its expression decreases towards E18. Conversely, the expression of Sp7, the transcription factor of mature osteoblasts, increases along with the number of these cells (Figure 3B). Myb expression was at the same level at E13 and E14, rapidly increased at E15 and remains at a high level at E18 (Figure 3C). Mmp2, Mmp14, Mmp15, Mmp16 show a very similar pattern with increasing expression towards E15 and with a decreased level at E18 (Figures 3D–G). Mmp11 has a variable expression during mandibular/alveolar bone development, nevertheless, with significantly decreasing expression until E18 (Figure 3H). Expression of Mmp9 and Mmp13 dramatically increases throughout bone development at E18 (Figures 3I,J). The statistical significance (p < 0,05; p < 0,01; p < 0.001) of each expression change is indicated in the figures by symbols *, **, ***, respectively.
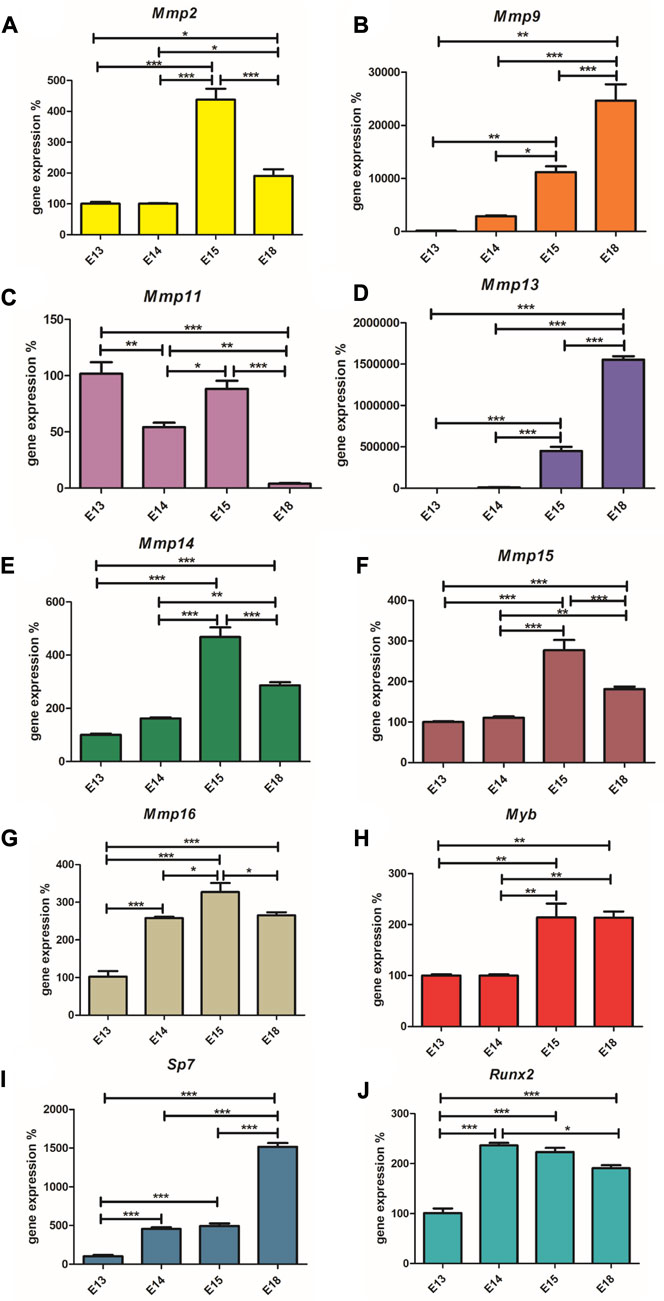
FIGURE 3. Expression (mRNA) of Runx2, Sp7, Myb, Mmp2, Mmp14, Mmp15, Mmp16, Mmp11, Mmp9, and Mmp13. The graph shows expression of mRNAs corresponding to Runx2 (Panel A), Sp7 (Panel B), Myb (Panel C), Mmp2 (Panel D), Mmp14 (Panel E), Mmp15 (Panel F), Mmp16 (Panel G), Mmp11 (Panel H), Mmp9 (Panel I) and Mmp13 (Panel J) during embryonic alveolar bone development detected by qPCR. Data are expressed as mean ± SD (n—4 per group) and statistically significant differences are highlighted (One-way ANOVA, Tukey test, *p < 0.05; **p < 0.01; ***p < 0.001).
3.4 Immunohistochemical localization of MYB and MMPs during mandibular/alveolar bone formation
To detect the protein expression of the investigated MYB and MMPs factors in situ, immunohistochemistry was applied.
The first structure of the mandibular/alveolar bone is morphologically visible at prenatal day 13, where the mesenchymal cells condense and differentiate into osteoblasts (Figure 4A1). MYB-positive nuclei were observed at this stage (Figure 4B1). At E14, MYB was localized in osteoblasts (Figure 4B2), and both osteoblasts and osteocytes were MYB-positive at E15 and E18 (Figure 4B3,B4).
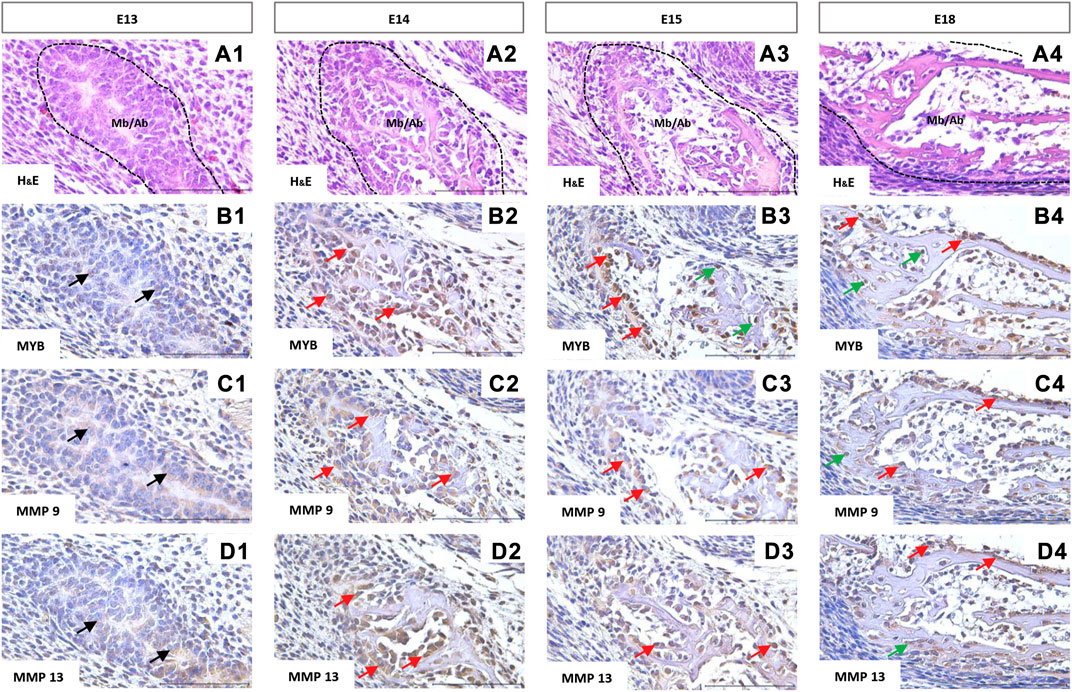
FIGURE 4. Immunohistochemical localization of MYB (B1–B4), MMP9 (C1–C4), and MMP13 (D1–D4) proteins in the forming mandibular/alveolar bone. Embryonic day (E) 13, 14, 15, 18. Haematoxylin and eosin (H&E) staining (A1–A4). Arrows point to the examples of positive cells: black = mesenchymal cells, red = osteoblasts, green = osteocytes. Scale bar =100 μm.
Matrix metalloproteinases 9 and 13 showed similar expression in histological sections. Both MMPs were expressed by the mesenchymal cells (Figure 4C1, 4D1) and were localized especially in the cell cytoplasm (Figures 4C,D), however positive nuclei were also observed in the case of MMP13 (Figure 4D2,D3,D4). At the stage E18, MMP9 and 13 positive osteocytes were found (Figure 4C4,D4).
4 Discussion
The comprehensive exploration of matrix metalloproteinases and mechanisms governing their multilevel regulation is challenging to understand tissue homeostasis (Gaffney et al., 2015). But, also to try to interconnect molecular biology, physiology, pathophysiology, and pharmacology in order to develop new selective therapeutic agents against various diseases (Vise and Nagase, 2003).
The Myb is essential for hematopoiesis (Soza-Ried et al., 2010) and its classical roles apply for undifferentiated and cancer cells (Cicirò and Sala, 2021). More recently, Myb has been linked to differentiated cells (Sandberg et al., 2005) including bone related ones (Matalova et al., 2011; Oralova et al., 2015; 2017). The challenge of analyzing Myb knock-out mice in osteogenesis is hindered by the prenatal lethality, by the day 15 in the mouse (Mucenski et al., 1991). This study capitalizes on Myb-deficient mice mandibles as a unique model to unravel the osteogenic potential of Myb transcription factor.
In vitro models are favoured in compliance with 3R principles to reduce or replace animal testing (Robinson et al., 2019). However, despite a rapid progress in the field (Owen and Reilly, 2018; de Wildt et al., 2019) there are no established in vitro models for bone remodelling (Ehnert et al., 2020). Therefore, to cover the cellular and extracellular complexity of the bone, animal models are extremely important particularly for translational research (Berridge, 2021). The mouse model is used for it is showing many similarities to humans, relatively short reproductive period, and financial availability. In addition, a wide spectrum of information is available at the genome, transcriptome, and proteome levels (Elefteriou and Yang, 2011).
This investigation provides data directly from the in vivo context of mandibular/alveolar bone formation. Metalloproteinases are essential for bone remodelling as well as homeostasis (Cicirò and Sala, 2021). Notably, the identification of some Mmps (Mmp1, Mmp2, Mmp7 and Mmp9) has been reported as Myb-regulated genes in cancer cells (Knopfova et al., 2012; Xu et al., 2019). At the stage of Myb knock-out lethality (by prenatal day 15), Mmps 2, 9, 11, 13, 14, 15 and 16 transcripts were detected in the mandibular samples. Out of these, expression of Mmp13 was significantly downregulated in the Myb knock-out mandibles. Mmp13, primarily secreted by chondrocytic and osteoblastic cells (Sasano et al., 2002), is the crucial collagenase that cleaves ECM collagens during bone and cartilage development (Stickens et al., 2004) especially collagen type I (Takaishi et al., 2008). As Mmp13 is important for bone remodelling (Tang et al., 2012), we can therefore speculate that the decreased Mmp13 expression in the Myb knock-out mice might reverberate within the bone tissue’s collagen type I breakdown. Interestingly, potential several Myb binding sites within the Col1a2 promoter suggests that it might be involved in the regulation of collagen type I production (reviewed in Jinnin, 2010). Mmp13 is well-known to be required for proper fracture healing (Nishimura et al., 2012; Tang et al., 2012). This may also apply for the intramembranous bone and could even be associated with the effect of Myb during dental implant osseointegration (Bhattarai et al., 2013). Mmp13 is an important marker of mature osteoblasts as demonstrated in the long bones (Zhang et al., 2012). Mmp13-deficient mice demonstrated abnormal bone phenotypes and increased trabecular bone volume (Stickens et al., 2004).
The precise regulation of Mmp13 expression in osteoblasts has not yet been completely elucidated. However previous studies have indicated that the transcription factors Runx2 and Sp7 (osterix) contribute to Mmp13 transcription (Zhang et al., 2012; Komori, 2018; Liu et al., 2020). Our investigation revealed that neither Runx2 nor Sp7 exhibited any significant alterations in the Myb-deficient mandible. This observation suggests the potential involvement of Myb as another regulator in bone development. This hypothesis gains support from the identification of Myb binding sites (MBS) within the Mmp13 promoter. Furthermore, overexpression of Myb in calvaria-derived cells confirmed that there were no significant changes in expression of Runx2 or Sp7, despite Mmp13 was upregulated. Notably, dysregulated overexpression or activation of Mmp13 has been associated with the excessive bone tissue breakdown and contribution to the pathogenesis, e.g., in the case of osteoarthritis (Hu and Ecker, 2021). Furthermore, the specific pattern of expression changes in Mmp13 could provide insights into the regulatory mechanism by which Myb influences Mmp13 expression. Thus, the increased in Mmp13 expression induced by Myb may occur through direct binding to the Mmp13 promoter region.
To contextualize the findings with mandibular/alveolar bone formation process, and the critical period of Myb knock-out lethality, different developmental stages of the mandible were investigated. From prenatal days 13–15 (E13-E15), the first osteoblasts appear (E13), followed by influx of osteoclasts (E14) and differentiated osteocytes (E15) (Alfaqeeh et al., 2013).
Along with Mmp 2, 9, 13, 14 and 16 identified in long bone development (Liang et al., 2016), Mmp11 and 15 mRNAs were detected in the mandibular samples. The other Mmps (Mmp 1,3,7,8,10,12) displayed negligible expression.
The expression profiles of Mmp 2, 14, 15 and 16 peaked around day 15. Mmp2 is involved in the formation and maintenance of the osteocyte channel network (Inoue et al., 2006) initiated around this stage. Remarkably, the expression pattern and levels of this metalloproteinase were identical with that of Mmp14. Mmp14 was reported as a novel target of PTH (and RANK/RANKL/OPG) signalling, which applies for osteoblasts as well as osteocytes (Delgado-Calle et al., 2018). Therefore, the elevation is likely to be connected with initiated osteocytogenesis and bone remodelling at the onset of mandibular/alveolar bone establishment. Not much is known about the function of Mmp15 in bone (Fatima et al., 2020). Mmp16 is present in osteoblasts and osteocytes (Haeusler et al., 2005) and is important for their viability (Shi et al., 2008). Thus, the elevation in Mmp16 could again correspond with the differentiation of first osteocytes at this stage.
Mmp11 was the only one among the metalloproteinases investigated that exhibited decreasing expression levels during the onset of alveolar bone formation. In general, Mmp11 plays a role in whole body metabolism and energy homeostasis (Cabral-Pacheco et al., 2020). Mmp11 has been associated with many physiological processes where ECM remodelling takes place, such as embryonic development (Klein and Bischoff, 2010). The physiological role of Mmp11 is unclear, but unlike the other Mmps investigated here, Mmp11 only weakly degrades structural proteins of the extracellular matrix (Reddy and Rani, 2017).
Mmp13 (relative gene expression compared to actin) unveiled the most striking expression changes within the developmental dynamics and also its relative levels (compared to other Mmps). Beyond its function in bone remodelling, Mmp13 plays multiple roles in osteogenesis (Nakatani and Partridge, 2017), including the formation of osteocytic processes and networks (Tang et al., 2012), and association with PTH pathways has been noted (Lee and Partridge, 2010). A pivotal study by Behonick et al. (2007) further underscores the significance of Mmp13 in bone physiology. Their research on Mmp13−/− mice revealed disrupted bone remodelling during the healing of stabilized fractures and cortical defects via intramembranous ossification. Specifically, the study found that Mmp13−/− mice had delayed bone healing and reduced bone formation compared to the control mice (Behonick et al., 2007). This demonstrated that Mmp13 is involved in the normal remodelling of bone and cartilage during adult skeletal repair, suggesting a direct involvement of Mmp13 in the initial stages of ECM degradation. Therefore, based on the broad impact of Mmp13 on both early but also on later osteogenesis, it is plausible to speculate that the consequence of Myb deficiency could extend to various stages of bone development. However, due to the prenatal lethality, this cannot be followed using the Myb knock-out mice analysed in the presented research.
Another Mmp with remarkable changes in expression dynamics during development was Mmp9. Mmp9 is critical for long bone development since it prevents accumulation of late hypertrophic chondrocytes in the growth plate (Liang et al., 2016). In early bone development, Mmp9 is expressed by osteoclasts (Reponen et al., 1994) appearing in the mandibular/alveolar bone at E14, when the expression of Mmp9 keeps a gradual increase towards E18. This increase also corresponds to the onset of angiogenesis within the bone region. Mmp9 has been demonstrated to participate in angiogenesis (Cackowski et al., 2010) and was investigated extensively also in cancerogenesis (Vu and Werb, 2000).
This study aimed to investigated the localization of Myb protein in osteoblasts, osteocytes and osteoclasts during the prenatal period of mandibular/alveolar bone formation/remodelling was provided. The first reports about MYB protein in alveolar bone development were provided by our team earlier (Matalova et al., 2011; Lungova et al., 2012). Notably, MMP9 and MMP13 proteins colocalized within the Myb-positive osteoblasts, osteocytes and osteoclasts. These two Mmps observed a coincidence with the most significantly increasing mRNA expression levels, as determined by RT-qPCR. This observed co-localization of MYB, MMP9, and MMP13 proteins provides additional support to the hypothesis about the Myb transcription factor being involved in the regulation of Mmps expression dynamics (Paiva and Granjeiro, 2017).
In the initial steps of alveolar bone development, metalloproteinases play intricate roles in regulating osteoblast/osteocyte differentiation, orchestrating bone formation and resorption, and facilitating osteoclast recruitment and migration via bloodstream (Omi and Mishina, 2022). This dynamic interplay is fundamental for establishing the alveolar bone as an integral part of the periodontal apparatus anchoring the tooth within the jaw and as such being subjected to regeneration therapies (Ward, 2022). Our findings are in agreement with the Myb contribution to osseointegration of dental implants as reported before (Bhattarai et al., 2013). The significant downregulation of Mmp13 expression in Myb-deficient mice suggests the possibility of direct Myb transcription factor binding within the Mmp13 promotor, intricately intertwined with processes of bone formation and resorption.
The recent investigations thus underscored the role of Mmps in the context of periodontium. The identification of novel components within the regulatory networks governing Mmps expression and functionality holds promise for enhancing our understanding of these processes and advancing strategies for the prevention and treatment of periodontal diseases (Checchi et al., 2020; Luchian et al., 2022).
It is worth mentioning that a number of pathologies are associated with impaired balance in bone remodelling due to altered expression/activation of Mmps, such as osteolysis, osteoarthritis, or osteoporosis (Paiva and Granjeiro, 2017). In cancerogenesis, upregulation of Mmp13 is associated with poor prognosis in oral squamous cell carcinoma (Vincent-Chong et al., 2014). Evidence about Mmp-13 in breast cancer-induced osteolysis indicates this Mmp as a promising therapeutic target for breast cancer bone metastasis (Kwon, 2023), where also Myb is involved as well (Knopfova et al., 2012).
In conclusion, our study has shed light on the impact of Myb on metalloproteinases, extending well beyond the immediate scope of our study. In this context, our study contributes to the broader scientific dialogue surrounding the intricate interplay between Myb and metalloproteinases in patho/physiological osteogenesis, opening research for further exploration and potential clinical applications. Therapeutic strategies aimed at regulating Mmp13 activity (Hu and Ecker, 2021), via the transcription factor Myb may be used for emerging treatments (Kou et al., 2021).
Data availability statement
The original contributions presented in the study are included in the article/Supplementary Materials, further inquiries can be directed to the corresponding author.
Ethics statement
The animal study was approved by the work with laboratory animals in the CR, including euthanasia, is regulated by Act No. 359/2012. According to the paragraph 3t), post mortem collection of organs and tissues is not considered as an experiment. The study was conducted in accordance with the local legislation and institutional requirements.
Author contributions
SV: acquisition of data, drafting manuscript, VO: methodology, acquisition of data, analysis and interpretation of data, writing—original draft, funding acquisition, MC: acquisition of data, analysis of Mmp13 promoter, writing—review and editing, JF: acquisition of data, analysis of Mmp13 promoter, writing—review and editing, LK: analysis of Mmp13 promoter, writing—review and editing, HL: writing—review and editing, PB: writing—review and editing, EM: conceptualization, writing—original draft, review and editing, supervision. All authors contributed to the article and approved the submitted version.
Funding
The research was supported by the Czech Science Foundation (GACR 19-15272Y). The CR-UK cooperation was supported by the Inter-Excellence Project Inter-COST/LTC20048 (www.msmt.cz) associated with CA18133 (www.cost.eu). SV was a holder of the IGA VETUNI Brno student grant 115/2019/FVL. LK was supported by the project National Institute for Cancer Research (Programme EXCELES, ID Project No. LX22NPO5102)—Funded by the European Union—Next Generation EU.
Conflict of interest
The authors declare that the research was conducted in the absence of any commercial or financial relationships that could be construed as a potential conflict of interest.
Publisher’s note
All claims expressed in this article are solely those of the authors and do not necessarily represent those of their affiliated organizations, or those of the publisher, the editors and the reviewers. Any product that may be evaluated in this article, or claim that may be made by its manufacturer, is not guaranteed or endorsed by the publisher.
Supplementary material
The Supplementary Material for this article can be found online at: https://www.frontiersin.org/articles/10.3389/fcell.2023.1168866/full#supplementary-material
SUPPLEMENTARY FIGURE S1 | Wild type (WT) and Myb knock-out (KO) mandibular/alveolar bone. The haematoxylin-eosin staining (H&E); haematoxylin-eosin-alcian blue staining (H&E/Alc); Masson’s green trichrome (MGT); Weigert van Gieson (WvG); Von Kossa (VKs) and Alizarin red (AlzR). Scale bar = 100 μm.
SUPPLEMENTARY FIGURE S2 | Expression of Myb in overexpressed calvaria cell line. The graph shows expression of RNAs corresponding to Myb in calvarial cells treated by Myb vector (MYB) and empty vector (mock) at 24 h (Supplementary Figure S1A) and 14 days (Supplementary Figure S1B). Data are expressed as mean ± SD (n—4 per group) and statistically significant differences are highlighted (unpaired t-test, two-tailed, ***p < 0.001).
SUPPLEMENTARY FIGURE S3 | Nucleotide sequence of Mmp13 promotor. Representation of the Mmp13 sequences upstream of the first exon (yellow), which contains the translation initiation codon (red). Sequences with a match to the YAACT/(C)/GGYCR consensus motif are highlighted, where cyan and pink represent 7/9 and 6/9 nucleotide matches, respectively.
References
Alfaqeeh, S. A., Gaete, M., and Tucker, A. S. (2013). Interactions of the tooth and bone during development. J. Dent. Res. 92 (12), 1129–1135. doi:10.1177/0022034513510321
Behonick, D. J., Xing, Z., Lieu, S., Buckley, J. M., Lotz, J. C., Marcucio, R. S., et al. (2007). Role of matrix metalloproteinase 13 in both endochondral and intramembranous ossification during skeletal regeneration.2 (11), e1150. doi:10.1371/journal.pone.0001150
Berridge, B. R. (2021). Animal Study Translation: the Other Reproducibility Challenge. J 62, 1–6. doi:10.1093/ilar/ilac005
Bhattarai, G., Lee, Y. H., Lee, M. H., and Yi, H. K. (2013). Gene delivery of c-myb increases bone formation surrounding oral implants. J. Dent. Res. 92 (9), 840–845. doi:10.1177/0022034513497753
Bhattarai, G., Lee, Y. H., Lee, N. H., Yun, J. S., Hwang, P. H., and Yi, H. K. (2011). c-myb mediates inflammatory reaction against oxidative stress in human breast cancer cell line, MCF-7. iochem. Funct. 29, 686–693. doi:10.1002/cbf.1808
Bruderer, M., Richards, R. G., Alini, M., and Stoddart, M. J. (2014). Role and regulation of runx2 in osteogenesis. Eur. 23 (28), 269–286. doi:10.22203/ecm.v028a19
Cabral-Pacheco, G. A., Garza-Veloz, I., Castruita-De la Rosa, C., Ramirez-Acuna, J. M., Perez-Romero, B. A., Guerrero-Rodriguez, J. F., et al. (2020). The roles of matrix metalloproteinases and their inhibitors in human diseases. Int. J. Mol. Sci. 21 (24), 9739. doi:10.3390/ijms21249739
Cackowski, F. C., Anderson, J. L., Patrene, K. D., Choksi, R. J., Shapiro, S. D., Windle, J. J., et al. (2010). Osteoclasts are important for bone angiogenesis. Blood 115 (1), 140–149. doi:10.1182/blood-2009-08-237628
Checchi, V., Maravic, T., Bellini, P., Generali, L., Consolo, U., Breschi, L., et al. (2020). The role of matrix metalloproteinases in periodontal disease. Int. J. Environ. Res. Public Health. 17 (14), 4923. doi:10.3390/ijerph17144923
Cicirò, Y., and Sala, A. (2021). MYB oncoproteins: emerging players and potential therapeutic targets in human cancer. Oncogenesis 10 (2), 19. doi:10.1038/s41389-021-00309-y
de Wildt, B. W. M., Ansari, S., Sommerdijk, N. A. J. M., Ito, K., Akiva, A., and Hofmann, S. (2019). From bone regeneration to three-dimensional in vitro models: issue engineering of organized bone extracellular matrix. Curr Opin Biomed Eng 10, 107–115. doi:10.1016/j.cobme.2019.05.005
Delgado-Calle, J., Hancock, B., Likine, E. F., Sato, A. Y., McAndrews, K., Sanudo, C., et al. (2018). MMP14 is a novel target of PTH signaling in osteocytes that controls resorption by regulating soluble RANKL production. FASEB J 32 (5), 2878–2890. doi:10.1096/fj.201700919RRR
Ehnert, S., Rinderknecht, H., Aspera-Werz, R. H., Haussling, V., and Nussler, A. K. (2020). Use of in vitro bone modls to screen for altered bone metabolism, osteopathies, and fracture healing: hallenges of complex models. Arch. Toxicol. 94 (12), 3937–3958. doi:10.1007/s00204-020-02906-z
Elefteriou, F., and Yang, X. (2011). Genetic mouse models for bone studies – strengths and limitations. Bone 49 (6), 1242–1254. doi:10.1016/j.bone.2011.08.021
Ess, K. C., Witte, D. P., Bascomb, C. P., and Aronow, B. J. (1999). Diverse developing mouse lineages exhibit high-level c-Myb expression in immature cells and loss of expression upon differentiation. Oncogene 18 (4), 1103–1111. doi:10.1038/sj.onc.1202387
Fatima, S., and Thakur, S. C. (2020). New insights into the role of matrix metalloproteinases. A M B 18 (7), 1448. doi:10.3390/ijms18071448
Fernandez-Patron, C., Kassiri, Z., and Leung, D. (2011). Modulation of Systemic Metabolism by MMP-2: from MMP-2 Deficiency in Mice to MMP-2 Deficiency in Patients. Compr. Physiol. 6 (4), 1935–1949. doi:10.1002/cphy.c160010
Gaffney, J., Solomonov, I., Zehorai, E., and Sagi, I. (2015). Multilevel regulation of matrix metalloproteinases in tissue homeostasis indicates their molecular specifity in vivo. Matrix Biol 44-46, 191–199. doi:10.1016/j.matbio.2015.01.012
Garcia, T., Roman-roman, S., Jackson, A., Theilhaber, J., Connolly, T., Spinella-jaegle, S., et al. (2002). Behavior of osteoblast, adipocyte, and myoblast markers in genome-wide expression analysis of mouse calvaria primery osteoblasts in vitro. Bone 31 (1), 205–211. doi:10.1016/s8756-3282(02)00781-0
Haeusler, G., Walter, I., Helmreich, M., and Egerbacher, M. (2005). Localization of matrix metalloproteinases, (MMPs) their tissue inhibitors, and vascular endothelial growth factor (VEGF) in growth plates of children and adolescents indicates a role for MMPs in human postnatal growth and skeletal maturation. Calcif. Tissue. Int. 76 (5), 326–335. doi:10.1007/s00223-004-0161-6
Howe, K. M., and Watson, R. J. (1991). Nucleotide preferences in sequence-specific recognition of DNA by c-myb protein. Nucl. Acids. Res. 19 (14), 3913–3919. doi:10.1093/nar/19.14.3913
Hu, Q., and Ecker, M. (2021). Overview of MMP-13 as a promising target for the treatment of osteoarthritis. Int J Mol Sci 22 (4), 1742. doi:10.3390/ijms22041742
Inoue, K., Mikuni-Takagaki, Y., Oikawa, K., Itoh, T., Inada, M., Noguchi, T., et al. (2006). A crucial role for matrix metalloproteinase 2 in osteocytic canalicular formation and bone metabolism. J. Biol. Chem. 281 (44), 33814–33824. doi:10.1074/jbc.M607290200
Jinnin, M. (2010). Mechanisms of skin fibrosis in systemic sclerosis. J 37 (1), 11–25. doi:10.1111/j.1346-8138.2009.00738.x
Khoswanto, Ch. (2023). Role of matrix metalloproteinases in bone regeneration: narrative review. J. Biol. Res. 13 (5), 539–543. doi:10.1016/j.jobcr.2023.06.002
Klein, T., and Bischoff, R. (2010). Physiology and pathophysiology of matrix metalloproteases. Acids 41 (2), 271–290. doi:10.1007/s00726-010-0689-x
Knopfova, L., Benes, P., Pekarcikova, L., Hermanova, M., Masarik, M., Pernicova, Z., et al. (2012). c-Myb regulates matrix metalloproteinases 1/9, and cathepsin D: implications for matrix-dependent breast cancer cell invasion and metastasis. Mol. Cancer. 11 (1), 15–15. doi:10.1186/1476-4598-11-15
Kodric, K., Zupan, J., Kranjc, T., Komadina, R., Mlakar, V., Marc, J., et al. (2019). Sex-determining region Y (SRY) attributes to gender differences in RANKL expression and incidence of osteoporosis. Exp. Mol. Med. 51 (8), 1–16. doi:10.1038/s12276-019-0294-3
Komori, T. (2018). Runx2, an inducer of osteoblast and chondrocyte differentiation. Histochem Biol 149 (4), 313–323. doi:10.1007/s00418-018-1640-6
Kou, L., Jiang, Y., Lin, X., Huang, H., Wang, J., Yao, Q., et al. (2021). Matrix Metalloproteinase Inspired Therapeutic Strategies for Bone Diseases. Curr. Pharm. Biotechnol. 22 (4), 451–467. doi:10.2174/1389201021666200630140735
Kwon, M. J. (2023). Matrix metalloproteinases as therapeutic targets in breast cancer. Front Oncol 19 (12), 1108695. doi:10.3389/fonc.2022.1108695
Laronha, H., and Caldeira, J. (2020). Structure and function of human matrix metalloproteinases. Cells 26 (5), 1076. doi:10.3390/cells9051076
Lee, M., and Partridge, N. C. (2010). Parathyroid hormone activation of matrix metalloproteinase-13 transcription requires the histone acetyltransferase activity of p300 and PCAF and p300-dependent acetylation of PCAF. J Biol Chem 285 (49), 38014–38022. doi:10.1074/jbc.M110.142141
Liang, H. P. H., Xu, J., Xue, M., and Jackson, C. J. (2016). Matrix metalloproteinases in bone development and pathology: current knowledge and potential clinical utility. Med 3, 93–102. doi:10.2147/MNM.S92187
Liu, Q., Li, M., Wang, S., Xiao, Z., Xiong, Y., and Wang, G. (2020). Recent advances of osterix transcription factor in osteoblast differentiation and bone formation. Front. Dev. Biol. 8, 601224. doi:10.3389/fcell.2020.601224
Luchian, I., Goriuc, A., Sandu, D., and Covasa, M. (2022). The role of matrix metalloproteinases (MMP-8, MMP-9, MMP-13) in periodontal and peri-implant pathological processes. Int J Mol Sci 23 (3), 1806. doi:10.3390/ijms23031806
Lungova, V., Buchtova, M., Janeckova, E., Tucker, A. S. S., Smarda, J., Matalova, E., et al. (2012). Localization of c-Myb in differentiated cells during postnatal molar and alveolar bone development. Eur. J. Sci. 120, 495–504. doi:10.1111/j.1600-0722.2012.01004.x
Matalova, E., Buchtova, M., Tucker, A. S., Bender, T. P., Janeckova, E., Lungova, V., et al. (2011). Expression and characterization of c-Myb in prenatal odontogenesis. Dev. Growth Differ. 53 (6), 793–803. doi:10.1111/j.1440-169X.2011.01287.x
Mucenski, M. L., McLain, K., Kier, A. B., Swerdlow, S. H., Schreiner, C. M., Miller, T. A., et al. (1991). A functional c-myb gene is required for normal murine fetal hepatic hematopoiesis. Cell. 65(4):677–689. doi:10.1016/0092-8674(91)90099-K
Nakatani, T., and Partridge, N. C. (2017). MEF2C interacts with c-FOS in PTH-Stimulated Mmp13 gene expression in osteoblastic cells. Endocrinology 158 (11), 3778–3791. doi:10.1210/en.2017-00159
Nishimura, R., Wakabayashi, M., Hata, K., Matsubara, T., Honma, S., Wakisaka, S., et al. (2012). Osterix regulates calcification and degradation of chondrogenic matrices through matrix metalloproteinase 13 (MMP13) expression in association with transcription factor Runx2 during endochondral ossification. J. Biol. Chem. 287 (40), 33179–33190. doi:10.1074/jbc.M111.337063
Omi, M., and Mishina, Y. (2022). Roles of osteoclasts in alveolar bone remodeling. Genesis 60 (8-9), e23490. doi:10.1002/dvg.23490
Oralova, V., Matalova, E., Janeckova, E., Krejci, E. D., Knopfova, L., Snajdr, P., et al. (2015). Role of c-Myb in chondrogenesis. Bone 76, 97–106. doi:10.1016/j.bone.2015.02.031
Oralova, V., Matalova, E., Killinger, M., Knopfova, L., Smarda, J., and Buchtová, M. (2017). Osteogenic Potential of the Transcription Factor c-MYB. Tissue Inter 100 (3), 311–322. doi:10.1007/s00223-016-0219-2
Owen, R., and Reilly, G. C. (2018). In vitro Models of Bone Remodelling and Associated Disorders. Front Bioeng Biotechnol 11 (6), 134. doi:10.3389/fbioe.2018.00134
Page-McCaw, A., Ewald, A. J., and Werb, Z. (2007). Matrix metalloproteinases and the regulation of tissue remodelling. Nat. Rev. Mol. Biol. 8 (3), 221–233. doi:10.1038/nrm2125
Paiva, K. B., and Granjeiro, J. M. (2017). Matrix metalloproteinases in bone resorption, remodeling, and repair. Prog. Mol. Biol. Transl. Sci. 148, 203–303. doi:10.1016/bs.pmbts.2017.05.001
Reddy, S. G., and Rani, S. H. (2017). Matrix Metalloproteases: potential Role in Type 2 Diabetic Nephropathy. Pathophysiol, 605–616. doi:10.1007/978-981-10-6141-7_25
Reponen, P., Sahlberg, C., Munaut, C., Thesleff, I., and Tryggvason, K. (1994). High expression of 92-kD type IV collagenase (gelatinase B) in the osteoclast lineage during mouse development. J. Biol. 124 (6), 1091–1102. doi:10.1083/jcb.124.6.1091
Robinson, N. B., Krieger, K., Khan, F. M., Huffman, W., Chang, M., Naik, A., et al. (2019). The current state of animal models in research: A review. Int. J. Surg. 72, 9–13. doi:10.1016/j.ijsu.2019.10.015
Stickens, D., Behonick, D. J., Ortega, N., Heyer, B., Hartenstein, B., Yu, Y., et al. (2004). Altered endochondral bone development in matrix metalloproteinase 13-deficient mice. Development 131 (23), 5883–5895. doi:10.1242/dev.01461
Sandberg, M. L., Sutton, S. E., Pletcher, M. T., Wilshire, T., Tarantino, L. M., Hogenesch, J. B., et al. (2005). C-Myb and p300 regulate hematopoietic stem cell proliferation and differentiation. Dev. 8 (2), 153–166. doi:10.1016/j.devcel.2004.12.015
Sasano, Y., Zhu, J. X., Tsubota, M., Takahashi, I., Onodera, K., Mizoguchi, I., et al. (2002). Gene expression of MMP8 and MMP13 during embryonic development of bone and cartilage in the rat mandible and hind limb. J. Histochem Cytochem. 50 (3), 325–332. doi:10.1177/002215540205000304
Shi, J., Son, M. Y., Yamada, S., Szabova, L., Kahan, S., Chrysovergis, K., et al. (2008). Membrane-type MMPs enable extracellular matrix permissiveness and mesenchymal cell proliferation during embryogenesis. Dev. Biol. 313 (1), 196–209. doi:10.1016/j.ydbio.2007.10.017
Soza-Ried, C., Hess, I., Netuschil, N., and Boehm, T. (2010). Essential role of c-myb in definitive hematopoiesis is evolutionarily conserved. PNAS 107 (40), 17304–17308. doi:10.1073/pnas.1004640107
Svandova, E., Peterkova, R., Matalova, E., and Lesot, H. (2020). Formation and developmental specification of the odontogenic and osteogenic mesenchymes. Front. Dev. Biol. 8, 640. doi:10.3389/fcell.2020.00640
Takaishi, H., Kimura, T., Dala, S., Okada, Y., and D´Armiento, J. (2008). Joint diseases and matrix metalloproteinases: A role for MMP-13. Curr Pharm Biotechnol 9 (1), 47–54. doi:10.2174/138920108783497659
Takanche, J. S., Kim, J. E., Kim, J. S., Lee, M. H., Jeon, J. G., Park, I. S., et al. (2018). Chitosan-gold nanoparticles mediated gene delivery of c-myb facilitates osseointegration of dental implants in ovariectomized rat. Artif. Cells. Biotechnol 46 (3), S807–S817. doi:10.1080/21691401.2018.1513940
Tang, S. Y., Herber, R. P., Ho, S. P., and Alliston, T. (2012). Matrix metalloproteinase–13 is required for osteocytic perilacunar remodeling and maintains bone fracture resistance. J. Bone Min. Res. 27 (9), 1936–1950. doi:10.1002/jbmr.1646
Vesela, B., Svandova, E., Bobek, J., Lesot, H., and Matalová, E. (2019). Osteogenic and angiogenic profiles of mandibular bone-forming cells. Front. Phys. 10, 124. doi:10.3389/fphys.2019.00124
Vincent-Chong, V. K., Salahshourifar, I., Karen-Ng, L. P., Siow, Y. M., Kallarakkal, T. G., Ramanathan, A., et al. (2014). Overexpression of MMP13 is associated with clinical outcomes and poor prognosis in oral squamous cell carcinoma. Sci World J 2014, 897523. doi:10.1155/2014/897523
Visse, R., and Nagase, H. (2003). Matrix metalloproteinases and tissue inhibitors of metalloproteinases: structure, function, and biochemistry. 92 (8), 827–839. doi:10.1161/01.RES.0000070112.80711.3D
Vu, J. L., and Werb, Z. (2000). Matrix metalloproteinases: effectors of development and normal physiology. Dev 14 (17), 2123–2133. doi:10.1101/gad.815400
Ward, E. (2022). A Review of Tissue Engineering for Periodontal Tissue Regeneration. J Vet Dent 39 (1), 49–62. doi:10.1177/08987564211065137
Xu, L. H., Zhao, F., Yang, W. W., Chen, C. W., Du, Z. H., Fu, M., et al. (2019). Myb promotes the growth and metastasis of salivary adenoid cystic carcinoma. Int J Oncol 54 (5), 1579–1590. doi:10.3892/ijo.2019.4754
Young, D. A., Barter, M. J., and Wilkinson, D. J. (2019). Recent advances in understanding the regulation of metalloproteinases. eCollection 18 (8), F1000 Faculty Rev-195. doi:10.12688/f1000research.17471.1
Keywords: metalloproteinases, MYB transcription factor, mandibular alveolar bone, development and remodelling, osteogenesis
Citation: Varadinkova S, Oralova V, Clarke M, Frampton J, Knopfova L, Lesot H, Bartos P and Matalova E (2023) Expression dynamics of metalloproteinases during mandibular bone formation: association with Myb transcription factor. Front. Cell Dev. Biol. 11:1168866. doi: 10.3389/fcell.2023.1168866
Received: 18 February 2023; Accepted: 18 August 2023;
Published: 28 August 2023.
Edited by:
Erika Kuchler, University of Regensburg, GermanyReviewed by:
Ricardo Reyes, University of La Laguna, SpainAndrea Ribeiro Lips Soares, University of the Region of Joinville, Brazil
Copyright © 2023 Varadinkova, Oralova, Clarke, Frampton, Knopfova, Lesot, Bartos and Matalova. This is an open-access article distributed under the terms of the Creative Commons Attribution License (CC BY). The use, distribution or reproduction in other forums is permitted, provided the original author(s) and the copyright owner(s) are credited and that the original publication in this journal is cited, in accordance with accepted academic practice. No use, distribution or reproduction is permitted which does not comply with these terms.
*Correspondence: V. Oralova, MjExODEyQG1haWwubXVuaS5jeg==
†These authors have contributed equally to this work and share first authorship