- 1Centro de Investigaciones Endocrinológicas “Dr. César Bergadá” (CEDIE), CONICET—FEI—División de Endocrinología, Hospital de Niños Ricardo Gutiérrez, Buenos Aires, Argentina
- 2Instituto de Investigaciones Biomédicas (INBIOMED), Facultad de Medicina CONICET- Universidad de Buenos Aires, Buenos Aires, Argentina
- 3Biostatistics Shared Resource, Knight Cancer Institute, Oregon Health and Science University, Portland, OR, United States
- 4Departamento de Biología Celular e Histología, Facultad de Medicina-Universidad de Buenos Aires Buenos, Buenos Aires, Argentina
The epidermal growth factor receptor (EGFR) signaling pathway is one of the main pathways responsible for propagating the luteinizing hormone (LH) signal throughout the cumulus cells and the oocyte. Recently, we have proposed the C-C motif chemokine receptor 2 (CCR2) and its main ligand (monocyte chemoattractant protein-1, MCP1) as novel mediators of the ovulatory cascade. Our previous results demonstrate that the gonadotropins (GNT), amphiregulin (AREG), and prostaglandin E2 (PGE2) stimulation of periovulatory gene mRNA levels occurs, at least in part, through the CCR2/MCP1 pathway, proposing the CCR2 receptor as a novel mediator of the ovulatory cascade in a feline model. For that purpose, feline cumulus-oocyte complexes (COCs) were cultured in the presence or absence of an EGFR inhibitor, recombinant chemokine MCP1, and gonadotropins [as an inducer of cumulus-oocyte expansion (C-OE), and oocyte maturation] to further assess the mRNA expression of periovulatory key genes, C-OE, oocyte nuclear maturation, and steroid hormone production. We observed that MCP1 was able to revert the inhibition of AREG mRNA expression by an EGFR inhibitor within the feline COC. In accordance, the confocal analysis showed that the GNT-stimulated hyaluronic acid (HA) synthesis, blocked by the EGFR inhibitor, was recovered by the addition of recombinant MCP1 in the C-OE culture media. Also, MCP1 was able to revert the inhibition of progesterone (P4) production by EGFR inhibitor in the C-OE culture media. Regarding oocyte nuclear maturation, recombinant MCP1 could also revert the inhibition triggered by the EGFR inhibitor, leading to a recovery in the percentage of metaphase II (MII)-stage oocytes. In conclusion, our results confirm the chemokine receptor CCR2 as a novel intermediate in the ovulatory cascade and demonstrate that the EGFR/AREG and the CCR2/MCP1 signaling pathways play critical roles in regulating feline C-OE and oocyte nuclear maturation, with CCR2/MCP1 signaling pathway being downstream EGFR/AREG pathway within the ovulatory cascade.
Introduction
The domestic cat (Felis catus) is a valuable model to study oocyte biology along with diverse infertility syndromes in women due to the highly conserved reproductive mechanisms between human and feline species (Wildt et al., 2010). Interestingly, cat oocytes share several characteristics with human oocytes (Combelles et al., 2002; Comizzoli et al., 2003) in contrast to typical laboratory mouse models, such as the diameter of the oocyte proper and the germinal vesicle (GV), the time to reach the MII-stage of meiosis in culture, and a nuclear configuration with a small nucleolus and fibrillar chromatin. In addition to the potential to expand knowledge of feline reproduction, the use of this model system has the advantage of providing an excellent surrogate for understanding events involving human COC that are necessary for fertility. In our laboratory, we have established two different feline culture systems, showing that the culture of feline antral follicles and/or their COCs was a robust and valuable system to study follicular development, steroidogenesis, periovulatory events, as well as follicle and COC biology in general (Rojo et al., 2015; Rojo et al., 2018; Jaworski et al., 2020).
Before ovulation, the LH surge triggers key processes for fertility, such as C-OE, resumption of oocyte meiosis, and rupture of the follicle wall. Oocyte nuclear maturation consists of the resumption and completion of the first meiotic division until arrest at the second meiotic metaphase, which is easily observed (Eppig and O’Brien, 1996). However, the oocyte only acquires full developmental competence when nuclear, as well as cytoplasmic maturation, are closely integrated. Cytoplasmic maturation encompasses the processes that enable the oocyte to be fertilized successfully and support embryo development but it is more difficult to assess (Zheng, 2007). Although these two programs can also proceed as independent processes to some extent (Sun and Moor, 1991). Meanwhile, the LH surge triggers another critical process known as C-OE. This process is a consequence of the loss in cell-to-cell contacts and an HA-rich extracellular matrix (ECM) being synthesized, mainly by HA synthase-2 (HAS2), resulting in a large increase in area or expansion of the cumulus granulosa cell layer that surrounds the oocyte (Chen et al., 1993; Fulop et al., 1997; Hess et al., 1999; Kimura et al., 2002; Salustri et al., 2004). This HA-rich-containing matrix is composed and stabilized by several plasmas- and follicle-derived components (Richards, 2005) including inter-alpha trypsin inhibitor (IαI) and associated heavy chains (HC1–3), proteoglycans such as versican, the tumor necrosis factor alpha-induced protein 6 (TNFAIP6), pentraxin 3 (PTX3), as well as certain members of the a-disintegrin and metalloproteinase with thrombospondin-like repeats (ADAMTS) family of proteases (Chen et al., 1992; Mukhopadhyay et al., 2001; Fulop et al., 2003; Russell et al., 2003; Salustri et al., 2004; Richards, 2005).
While some of the autocrine and paracrine factors important for the periovulatory events have been identified, the molecular mechanisms responsible for initiating such complex processes are not fully understood. During the periovulatory interval, the activation of a complex signaling network is essential to induce and guarantee LH-dependent processes since the expression of the LH receptor is mainly restricted to the mural granulosa cells (Peng et al., 1991). One of the main pathways responsible for propagating the LH signal throughout the cumulus cells and the oocyte is the system composed of the EGFR and its ligands (such as AREG, epiregulin (EREG), and betacellulin (BTC)) (Park et al., 2004; Richani and Gilchrist, 2018). Indeed, it was demonstrated that EGF-related factors mediate the gonadotrophin action through the induction of steroid and prostaglandin production (Jamnongjit et al., 2005; Shimada et al., 2006). On the other hand, we have recently proposed the chemokine receptor CCR2 and its main ligand (MCP1) as novel mediators of the ovulatory cascade, demonstrating that MCP1 increases the mRNA levels of key genes involved in the ovulatory cascade [HAS2, AREG, TNFAIP6, PTX3, and growth differentiation factor-9 (GDF9)] within the feline COC in vitro (Rojo et al., 2019). And interestingly, in our previous work when the CCR2 receptor was inhibited in the COC in vitro, the stimulation of mRNA levels of different periovulatory genes (AREG, HAS2, and TNFAIP6) triggered by recombinant AREG was repressed (Jaworski et al., 2020), suggesting that MCP1/CCR2 system may be downstream the EGF-related factors in the ovulatory cascade. Supporting this idea, studies on MCP1 stimulation by EGF-like ligands have been reported in other tissues (Zhu et al., 2007; Liu et al., 2008).
Both types of receptors—G protein-coupled receptors (GPCRs; such as CCR2) and receptor tyrosine kinases (RTKs; such as EGFR)—share common signaling intermediates in the pathway (Luttrell et al., 1999). Although an increasing number of studies show that cross-communication can be generalized to several GPCRs and diverse cell types, between GPCRs and EGFR (Eguchi et al., 1998), the biological significance of this crosstalk within the COC remains unknown, and thus further studies are warranted. Therefore, the aim of our study was to evaluate the participation and interactions in the ovulatory cascade of the EGFR/AREG and the CCR2/MCP1 signaling pathways, using the domestic cat as our model. For that purpose, feline COCs were cultured in the presence or absence of an EGFR inhibitor, recombinant chemokine MCP1, and GNTs (as an inducer of C-OE and oocyte maturation) to further assess the mRNA expression of periovulatory key genes (AREG, HAS2, TNFAIP6, and PTX3), C-OE, oocyte nuclear maturation, and steroid hormone production.
Materials and methods
Animals
Ovaries from adult female F. catus (n = 104) at different stages of the natural estrous cycle during the breeding season were used. The ovaries were donated following routine spaying procedures conducted at “Centro de Salud Animal de la Municipalidad de Merlo” (Provincia de Buenos Aires, Argentina). The extracted ovaries were immediately transported to the laboratory in a refrigerated physiological solution. Those that presented stigmas, as an indication of recent ovulation, or with cysts were discarded.
COCs isolation
Antral follicles (0.5–2 mm) were dissected from the ovary using 30-gauge needles under a stereoscopic microscope. They were punctured and their COCs were extracted in a minimum essential medium (MEM; Gibco) containing Hepes (25 mM, Gibco), L-glutamine (2 mM, Sigma-Aldrich), sodium pyruvate (1 mM, Sigma-Aldrich), penicillin/streptomycin (100 IU/mL—0.1 mg/mL, Sigma-Aldrich), and 0.3% Bovine Serum Albumin (BSA; Gibco), as previously reported (Rojo et al., 2018; Rojo et al., 2019; Jaworski et al., 2020). Only healthy COCs presenting an adequate oocyte diameter (>110 μm excluding zona pellucida) with homogeneous dark cytoplasm, complete corona radiata, and compact cumulus were collected.
COC culture for gene expression and cumulus-oocyte expansion (C-OE) analysis
Isolated COCs (n = 237) were randomly divided and cultured in a 4-well plate in the optimal medium for C-OE analysis [MEM containing Hepes, L-glutamine, sodium pyruvate, penicillin/streptomycin, and 1% fetal bovine serum (FBS)-charcoal/stripped] previously determined empirically by our laboratory (Rojo et al., 2018). COCs were cultured in groups under different treatments containing human recombinant FSH + LH (GNTs; 10 and 5 UI/mL respectively; Merck Serono; as an inducer of C-OE and oocyte maturation), human recombinant MCP1 (10 ng/mL, Life Technologies), or in the presence of a dual EGFR inhibitor (1 μM, Lapatinib, a drug commonly used in patients; LC Labs). The concentrations used for the different treatments are based on the laboratory’s previous results, as well as the manufacturer’s datasheet and literature. COCs were cultured for 3 h for gene expression (Three separate cultures were performed, n = 78 Total COCs) or 24 h for C-OE (Five additional cultures were performed, n = 159 Total COCs) at 38°C under 5% CO2 in the air under different treatments as follows: a- GNT: Media + FSH + LH, b- GNT + LAPATINIB: Media + FSH + LH + EGFR Inhibitor (LAPATINIB), c- GNT + LAPATINIB + MCP1: Media + FSH + LH + LAPATINIB + MCP1, d- LAPATINIB: Media + LAPATINIB, e− LAPATINIB + MCP1: Media + LAPATINIB + MCP1, f- MCP1: Media + MCP1. The culture time for gene expression corresponds to the period in which the expression of key genes involved in C-OE (such as HAS2, TNFAIP6, AREG, and PTX3) increases against GNTs stimulation in our culture system (Rojo et al., 2018; Rojo et al., 2019; Jaworski et al., 2020). Serum was included in the media for COC due to culture serum factors being a requirement for C-OE to occur (Richards, 2005). COCs were photographed at 0 and 24 h of culture, using a digital camera attached to an Olympus microscope. At the end of the gene expression culture (3 h), COCs were stored individually at −80°C for subsequent RNA extraction and quantitative RT-PCR (qRT-PCR). On the other hand, at the end of the C-OE culture COCs were fixed in 4% paraformaldehyde for 15 min at 37°C and stored in a washing buffer (1% BSA, 0.2% powder milk, 0.2% goat serum, 0.2% donkey serum, 0.1% Triton X-100, 0.1 M glycine in 1x PBS) at 4°C for further HA IF, and medium culture was stored at −80°C for determination of steroid hormone production.
An additional set of experiments was accomplished to evaluate the effects of recombinant AREG (10 and 100 ng/mL) on the mRNA expression of MCP1 and its receptor (CCR2). Healthy COCs (n = 17) isolated from antral follicles were randomly divided and cultured under different treatments using optimal conditions for gene expression as previously described (Jaworski et al., 2020) above.
COC culture for oocyte maturation
Dissected COCs (n = 306) were randomly distributed and cultured in the optimal medium for in vitro maturation (IVM) used in our laboratory (MEM containing L-glutamine, sodium pyruvate, penicillin/streptomycin, and BSA), as previously reported (Jaworski et al., 2020) with slightly modifications. Oocyte maturation culture was carried out without oil since the hydrophobic characteristic of the inhibitor containing Hepes (25 mM). COCs were cultured in groups for 28 h at 38°C under 5% CO2 in the air under the same different treatments as follows: a- GNT, b- GNT + LAPATINIB, c- GNT + LAPATINIB + MCP1, d- LAPATINIB, e− LAPATINIB + MCP1, and f- MCP1. At the end of culture, COCs were treated briefly with hyaluronidase (1 mg/mL in MEM) to dissociate the cumulus, and naked oocytes were fixed in 4% PFA for 10 min at 37°C for indirect immunofluorescence (IF) to assess oocyte nuclear maturation, as previously described (Peluffo et al., 2010; Jaworski et al., 2020). The oocytes were then stored in washing buffer at 4°C, and medium culture was stored at −80°C for measurement of steroid hormone production.
mRNA extraction and gene expression analysis by qRT-PCR
Total RNA was extracted from each COC (Two COCs from each group from each separate culture were extracted, total per group n = 6) using Absolutely RNA Nanoprep Kit (Agilent), as previously described (Rojo et al., 2018; Rojo et al., 2019; Jaworski et al., 2020). Briefly, DNase treatment was performed to obtain DNA-free total RNA, following the kit instructions. Afterward, 10–100 ng of RNA per sample were eluted in 12 µL of elution buffer (as measured by UV-spectrophotometer, NanoDrop 2000; Thermo Scientific). To synthesize single-stranded cDNA from total RNA, the High-Capacity cDNA Reverse Transcription Kit (Applied Biosystems) was used following the manufacturer’s instructions. The reverse transcription was performed for 2 h at 37°C using 10 μL of the RNA extracted from each COC, in a 20 μL reaction volume. The kit uses random primers as the cDNA priming method and the Multiscribe RT, a recombinant reverse transcriptase obtained from the Moloney Murine Leukemia Virus (MoMuLV). At the end of the reaction, reverse transcriptase was inactivated at 85°C for 5 min. After cDNA synthesis, qPCR for key genes within the ovulatory cascade (AREG, HAS2, TNFAIP6, and PTX3), as well as CCR2 and MCP1, was conducted as previously described using the same set of primers and TaqMan probes (Rojo et al., 2018; Rojo et al., 2019; Jaworski et al., 2020). The list of primers and hydrolysis probes (TaqMan, Thermo Scientific), with the accession numbers of each target sequence, are listed in Supplementary Table S1. Relative levels of target gene expression were normalized to ribosomal RNA protein 18S levels.
Analyses of C-OE by HA-IF and confocal microscopy
Qualitative, as well as quantitative analyses of C-OE, focused on the change in the COC area, which is widely considered the main manifestation of this process. Because morphological assessment of expansion does not discriminate between simple loss of cell-cell contacts/death during 2-D culture and true expansion, a methodology was developed to assess a molecular indicator of C-OE: HA synthesis. A fluorescence-based technique using HA binding protein (HABP) was employed to evaluate the formation and deposition of an HA-rich ECM in expanded COCs in vitro, originally developed for rhesus macaque samples (Peluffo et al., 2014) with slight modifications. Briefly, an Avidin-Biotin Blocking Kit (Vector Laboratories) was used to block the endogenous signal. Subsequently, COCs were incubated with biotinylated HABP (1:200 in washing buffer; Calbiochem, Merk) for 2 h at room temperature. COCs were then washed and incubated with Cy3-labeled streptavidin (1:500 in washing buffer; Invitrogen) for 1 h at room temperature to reveal the presence of HA in the samples. After the following washing, nuclear staining was detected using Hoechst 33342 (1:1,000 in 1x PBS; Invitrogen) for 10 min at room temperature. COCs were washed, mounted on slides with glycerol/PBS solution (1:1), and coverslips affixed with clear nail polish. COCs were analyzed by confocal microscopy (Olympus FLUOVIEW FV1000 confocal laser scanning microscope) using UPLFLN 40 ×1.3 OIL CS UV objective. Full Z-stack datasets were collected for each COC, with images taken every 0.5 μm.
Oocyte maturation and IF
To determine the nuclear maturation stage [GV-intact, germinal vesicle breakdown (GVBD), metaphase I (MI), and MII] in cat-denuded oocytes, IF was performed as previously described (Peluffo et al., 2010; Jaworski et al., 2020) due to cat oocytes having a dark appearance because of high intracellular concentration of lipids, making GV observation difficult under the optical microscope. Oocytes were briefly incubated with mouse alpha-tubulin clone DM1A (1:800 in washing buffer; Novus Biologicals) for 1 h at 37°C to detect spindle microtubules. Afterward, COCs were incubated with Alexa Fluor 488 donkey anti-mouse IgG (1:200 in washing buffer; Life Technologies) for 1 h at 37°C. DNA was labeled with Hoechst 33342 (1:1,000 in 1x PBS) for 10 min at room temperature. Oocytes were mounted on slides with glycerol/PBS solution (1:1) and coverslips affixed with clear nail polish. The maturation stage for each oocyte was analyzed and visualized using a fluorescent light microscope (Axio Imager M2, Carl Zeiss, Germany).
Hormonal levels in culture media
Levels of estradiol (E2) and P4 in the media were measured from the different groups at 24 h (C-OE media) or 28 h (maturation media) to assess the ability to produce steroids and response under different treatment conditions. E2 and P4 levels were analyzed and expressed per well or per COC. So, the value expressed per COC corresponds to the media of that group from 6 cultures, where the raw data from each well and from each culture is divided according to the number of COC present in each well since COCs we cultured in groups. The steroids content within the culture media was analyzed by the Endocrine Laboratory at CEDIE “Hospital de Niños Ricardo Gutiérrez” using a COBAS e411 analyzer, an electrochemiluminescence-based automatic clinical platform (Roche Diagnostics GmbH) (Freire et al., 2013; Rojo et al., 2015). Intra- and inter-assay coefficients of variation of all assays were less than 4%. The minimum detectable amount of the standard for these steroids is 10 and 50 pg/mL, respectively.
Statistical analysis
Statistical calculations were mainly performed using GraphPad Prism 5 software (GraphPad Software, Inc., San Diego, CA, United States) and SAS version 9.4 (SAS inc. NC, United States). One-Way ANOVA was used to analyze differences in gene expression, followed by Newman–Keuls for multiple comparisons with differences considered significant at p < 0.05. Generalized linear model, logistic regression, was used to evaluate a treatment effect on maturation to MII. The odds ratios (OR) and 95% confidence interval (CI) were calculated, and a p-value <0.05 was considered to be statistically significant at a significance level of 0.05.
Results
MCP1 was able to revert the inhibition of AREG mRNA by the EGFR inhibitor, but not HAS2 or PTX3 mRNA levels within the COC in vitro
To evaluate the participation and interactions in the ovulatory cascade of the EGFR/AREG and the CCR2/MCP1 signaling pathways, we first performed COC cultures to analyze the mRNA expression of periovulatory key genes under different treatments (Figure 1). The normalized mRNA expression assessed by qRT-PCR showed that the EGFR inhibitor was able to significantly (p < 0.05) prevent or interfere with the GNT stimulation of AREG (Panel A), HAS2 (Panel B), and PTX3 (Panels D) mRNA expression. Interestingly, MCP1 was able to revert (p < 0.05) the inhibition of AREG mRNA expression trigged by the EGFR inhibitor (Panel A). In contrast, MCP1 could not revert (p > 0.05) the inhibition of HAS2 and PTX3 mRNA levels (Panels B and D, respectively). Regarding the mRNA expression of TNFAIP6, no significant differences (p > 0.05) were observed among the GNT, GNT + LAPATINIB, and GNT + LAPATINIB + MCP1 groups.
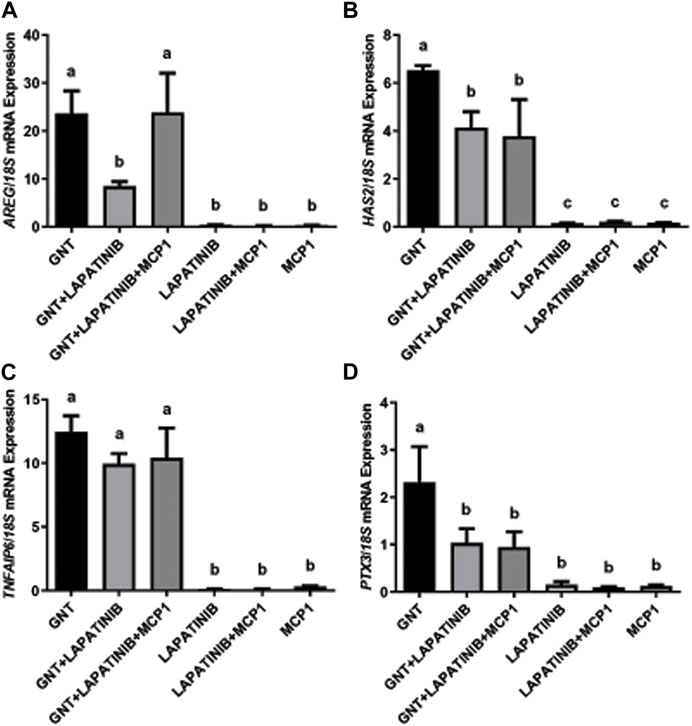
FIGURE 1. MCP1 reverted the inhibition of AREG mRNA by the EGFR inhibitor but not HAS2 or PTX3 in the feline COC in vitro. Normalized AREG (A), HAS2 (B), TNFAIP6 (C), and PTX3 (D) mRNA expression levels (mean ± SEM, n = 5–6 COCs per group) within the COC after 3 h of culture in the presence or absence of a positive stimulus (GNT), EGFR inhibitor (LAPATINIB), and/or the recombinant chemokine MCP1, assessed by qRT-PCR. 18S rRNA served as the invariant control for normalization. Different letters represent significant differences between groups (ANOVA; p < 0.05). AREG: amphiregulin, HAS2: hyaluronan synthase 2, TNFAIP6: tumor necrosis factor alpha-induced protein 6, PTX3: pentraxin 3.
In addition, as detailed in the M&M section, a subset of the COC culture was performed to assess the mRNA expression of MCP1 and its receptor (CCR2) in the presence of two different concentrations (10 and 100 ng/mL) of recombinant AREG (Figure 2), showing that AREG (10 ng/mL) significantly stimulated the mRNA levels of the feline chemokine MCP1 in comparison to the control (Panel A). Whereas AREG significantly increased the CCR2 mRNA levels at the higher concentration (100 ng/mL, Panel B).
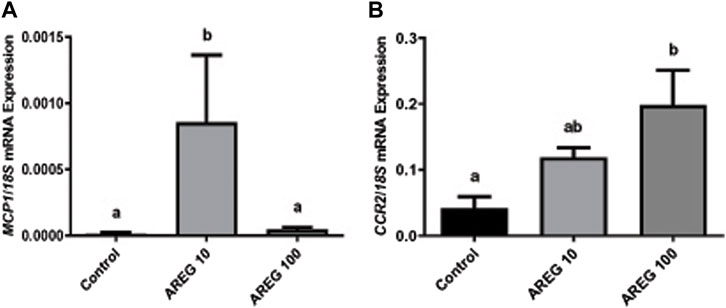
FIGURE 2. The mRNA expression of MCP1 and CCR2 significantly increased within the COC in the presence of recombinant AREG. Normalized MCP1 [Panel (A)], and CCR2 [Panel (B)], mRNA expression levels (mean ± SEM, n = 5–6 COCs per group) within the COC after 3 h of culture in the presence or absence of recombinant AREG (10 or 100 ng/mL), assessed by qRT-PCR. 18S rRNA served as the invariant control for normalization. Different letters represent significant differences between groups (ANOVA; p < 0.05). AREG: amphiregulin, MCP1: monocyte chemoattractant protein-1, CCR2: chemokine CC-motif receptor type 2.
MCP1 reverted the inhibition of GNT-stimulated HA synthesis triggered by the EGFR inhibitor within the expanded matrix in the COC in vitro
After evaluating the mRNA expression of periovulatory key genes, studies were conducted to evaluate the effect of the EGFR inhibitor on feline C-OE triggered by GNTs (as an inducer of C-OE), in the presence or absence of recombinant MCP1 in vitro. As a first approach, morphological features of C-OE were analyzed under the inverted microscope 24 h after culture (Figure 3) showing that the EGFR inhibitor blocked the C-OE in comparison to the GNT group. Interestingly, the addition of MCP1 seemed to restore the C-OE. To confirm these, we assessed the main molecular indicator of C-OE, the HA synthesis, by IF. Representative confocal images of feline COCs showing the formation and deposition of an HA-rich ECM in expanded COCs 24 h after culture under different conditions are depicted in Figure 4. IF performed in fixed COCs using a HA binding protein confirmed that GNT-stimulated HA synthesis within the expanded matrix in the COC (Panel A) was inhibited in the presence of the EGFR inhibitor (Panel B). In contrast, MCP1 could revert this effect, and HA was observed throughout the whole COC (Panel C). The LAPATINIB control group showed minimal HA-positive staining (Panel D). In contrast, all the groups containing the recombinant MCP1 consistently showed synthesis, and secretion of HA throughout and among all COCs analyzed (Panels C, E, F), being the group GNT + LAPATINIB + MCP1 the one with the strongest HA staining observed (Panel C). Supplementary Figure S1 depicts representative confocal microscopy images from three separate cultures of feline COC from C-OE culture under different treatments after HAIF staining.
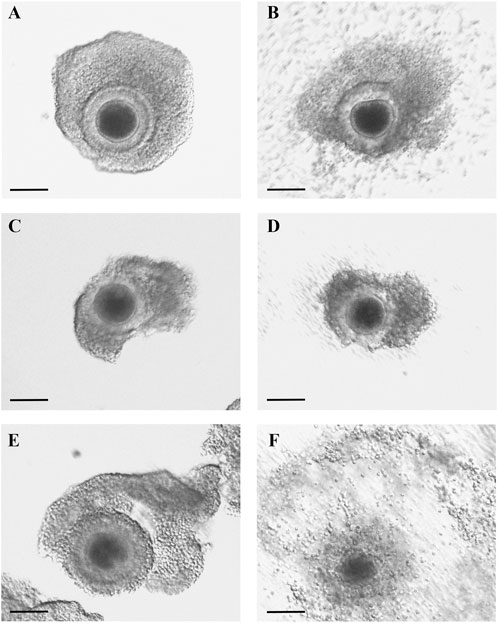
FIGURE 3. MCP1 restored C-OE inhibited by the EGFR inhibitor. Representative images of feline COCs from C-OE culture were taken prior to (A,C,E) and at the end of culture 24 h; (B,D,F). COCs were incubated (24 h) with GNT (A–B), GNT + LAPATINIB (C–D), GNT + LAPATINIB + MCP1 (E–F). Scale bars 100 μm.
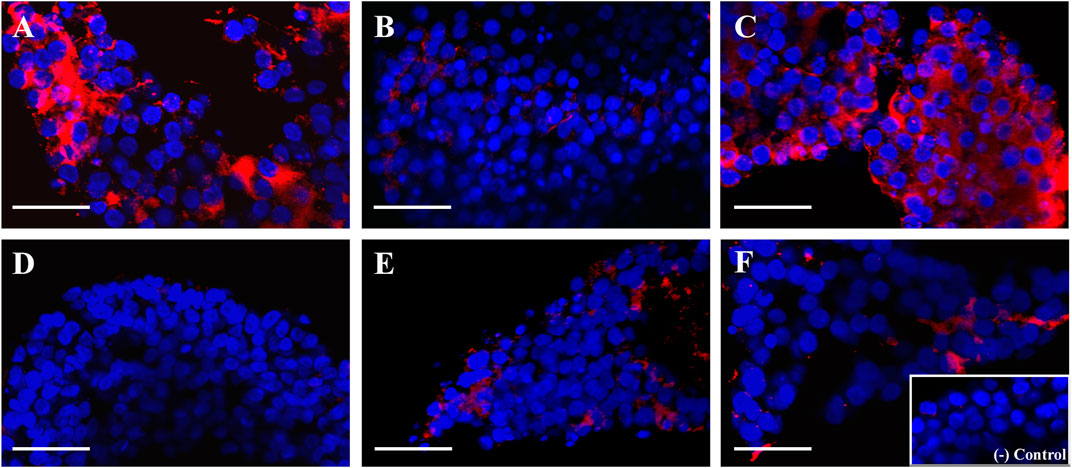
FIGURE 4. MCP1 restored the hyaluronic acid (HA) synthesis inhibited by the EGFR inhibitor. Representative confocal microscopy images (x 40) of feline COCs from C-OE culture under different treatments after HA IF staining. COCs were incubated (24 h) with GNT (A), GNT + LAPATINIB (B), GNT + LAPATINIB + MCP1 (C), LAPATINIB (D), LAPATINIB + MCP1 (E), MCP1 (F). (A–F) Nuclear staining is displayed in blue (Hoechst 33342), and HA in red (Cy3). No staining was observed in the negative control (in the absence of the HA binding protein, Insert). Scale bars 50 μm.
High percentage of feline MII-stage oocytes observed in the presence of GNT, MCP1, and the EGFR inhibitor
Oocyte maturation was measured as the percentage of oocytes that reached the MII stage after 28 h in culture under different treatment conditions. As expected, a high percentage of MII-stage oocytes was observed in the presence of GNT. Interestingly, the addition of the EGFR inhibitor to the media indeed significantly decreased (p = 0.0135) this stimulation (Figure 5), as expected based on other species. In contrast, the addition of MCP1 could revert this inhibition, leading to a recovery with an increased percentage of MII-stage oocytes and returning the percentage of MII-stage oocytes to a similar percentage obtained in the presence of GNT (GNT vs. GNT + LAPATINIB + MCP1, p = 0.7463).
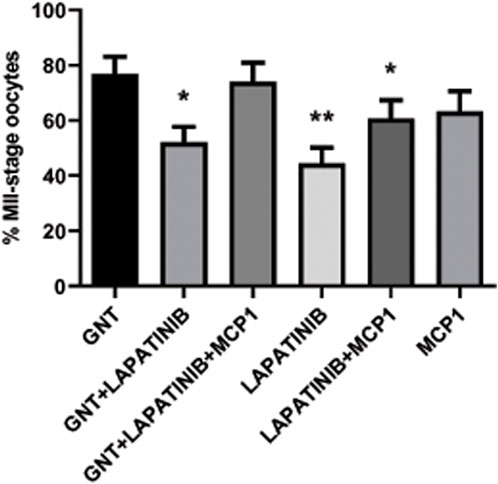
FIGURE 5. In vitro feline oocyte maturation under different treatment conditions. Proportion of metaphase II (MII) stage oocytes after 28 h in culture, in the presence or absence of a positive stimulus (GNT), a dual EGFR inhibitor (LAPATINIB), and/or recombinant chemokine MCP1, assessed by IF (expressed as mean percentage ±SEM, n = 306 total COCs). Generalized linear model, logistic regression, was used to evaluate a treatment effect on maturation to MII. * p-value <0.05 compared to GNT; ** p-value <0.001 compared to GNT.
The EGFR inhibitor alone did not affect oocyte maturation and the percentage of MII oocytes is significantly lower in comparison to the GNT (p = 0.0009). The percentage of MII oocytes within this group is like unstimulated COCs (spontaneous maturation) that usually represent around 30%–50%.
MCP1 restored in vitro P4 production inhibited by the EGFR inhibitor in C-OE cultures
To further study the possible effect and interactions of the EGFR/AREG and the CCR2/MCP1 signaling pathways, we assessed the E2 and P4 production by the COCs under different treatments. Levels of E2 and P4 measured in the culture media from the different groups are detailed in Table 1 (Panel A: C-OE media; Panel B: IVM media).
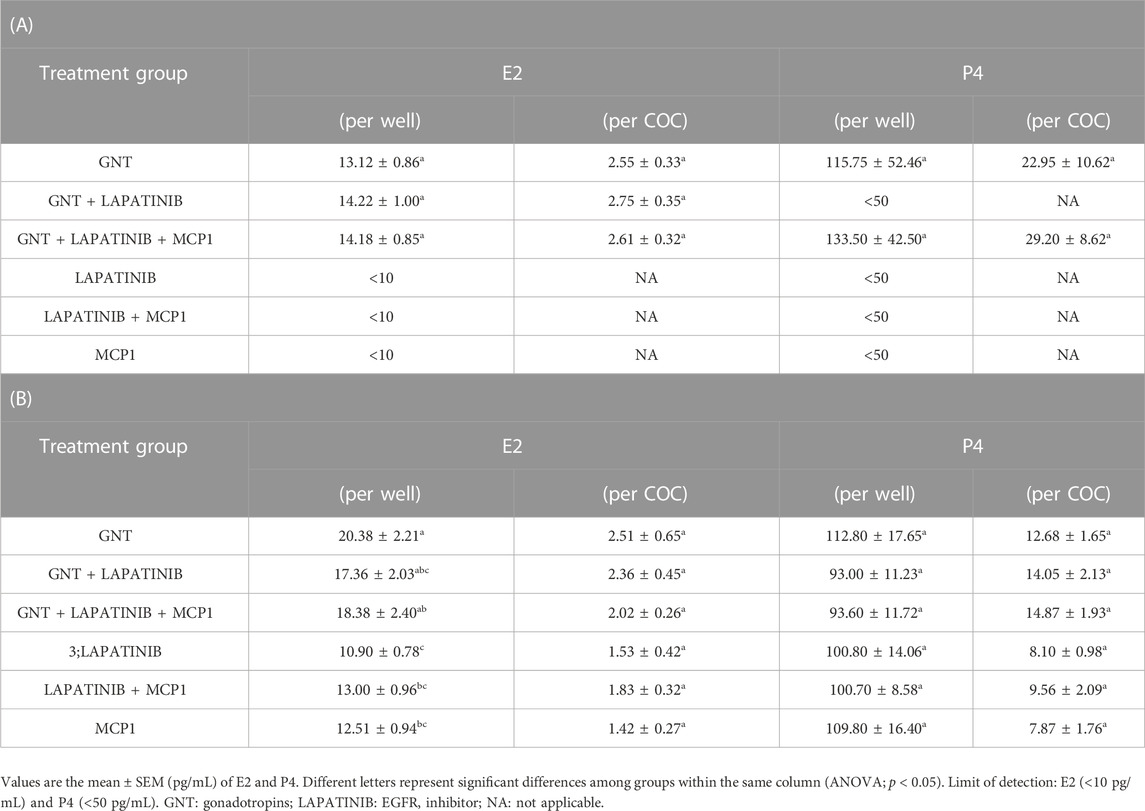
TABLE 1. Steroid levels present in the media of feline COC after 24 (Panel A: C-OE) or 28 (Panel B: IVM) hours in culture under the different treatments. E2 and P4 levels were analyzed and expressed per well or per COC (n = 6 experiments).
Steroids production at 24 h culturing the COCs within the C-OE media was detected only in groups containing GNTs (Panel A). EGFR inhibitor did not have a significant effect on E2 production. However, the presence of this inhibitor in the media blocked the P4 production stimulated by GNT, with no detectable levels observed within this group. But interestingly, MCP1 was able to restore the P4 production. Steroid data was analyzed (and expressed) by total levels detected per well but also, per COC considering that COCs were cultured in groups. Similar results were observed among groups when data were analyzed either per well or per COC.
Panel B shows the results from the IVM media. All samples from IVM media showed E2 and P4 levels with the highest concentrations observed within the GNT groups when analyzing per COC. In the IVM media, the EGFR inhibitor did not significantly affect the production of these steroids triggered by GNT (p > 0.05).
Discussion
The present study reveals critical roles and interactions of the EGFR/AREG and the CCR2/MCP1 signaling pathways in the ovulatory cascade. We observed that MCP1 was able to revert the inhibition of AREG mRNA expression by an EGFR inhibitor within the feline COC. In accordance, we also observed that the GNT-stimulated HA synthesis and P4 production, blocked by the EGFR inhibitor, was recovered by the addition of recombinant MCP1 in the C-OE culture media. Regarding oocyte nuclear maturation, recombinant MCP1 could also revert the inhibition triggered by the EGFR inhibitor, leading to a recovery in the percentage of MII-stage oocytes.
As a first approach, we studied the interactions between the EGFR/AREG and the CCR2/MCP1 signaling pathways within the mRNA expression of periovulatory key genes (AREG, HAS2, TNFAIP6, and PTX3). As expected, the EGFR inhibitor was able to prevent or interfere with the GNT stimulation of AREG, HAS2, and PTX3 mRNA expression, but not with TNFAIP6. Park et al. (2004) demonstrated that LH stimulation induced the transient and sequential expression of the EGF family members such as AREG, and these EGF-related growth factors act as paracrine mediators that propagate the LH signal throughout the follicle to promote periovulatory events in both oocytes and cumulus cells. Also, it was demonstrated by others that EGFR activity was essential for periovulatory events (such as oocyte maturation and cumulus expansion) using pharmacological inhibitors and genetic disruption to the EGF network (Ashkenazi et al., 2005; Hsieh et al., 2007). Thus, we expected that the inhibition of the EGFR within the feline COC would have a significant effect on the periovulatory genes, as observed. Surprisingly, MCP1 was only able to revert the inhibition of AREG mRNA triggered by the EGFR inhibitor. We previously demonstrated that a highly selective antagonist of CCR2 (the main receptor of MCP1) significantly repressed the stimulation of AREG, HAS2, and TNFAIP6 mRNA levels triggered by AREG within the feline COC (Jaworski et al., 2020), suggesting that CCR2/MCP1 system was downstream the EGF-related factors in the ovulatory cascade. On the other hand, we observed in the current study that AREG significantly stimulated the mRNA levels of the chemokine MCP1 and its receptor CCR2 within the feline COC. Considering that we observed that AREG stimulated directly CCR2 and MCP1 mRNA, and MCP1 stimulated AREG mRNA (Rojo et al., 2019), we could think about the existence of a positive loop within EGFR/AREG and CCR2/MCP1 pathways, that maybe guarantees and propagate the periovulatory process triggered by LH. With regard to the mechanism involved in regulating MCP1 expression by the EGFR pathway, it was reported that the EGFR signaling pathway can activate the PKC and MAPKs (Rabinovitz et al., 2004; Frijns et al., 2010), which will then induce the activation and translocation of nuclear factor (NF) κb to the nucleus. And it has been shown that induced expression of MCP1 is strongly dependent on the activation of the transcription factor NFκb (Ueda et al., 1994; Ueda et al., 1997).
Previously, our laboratory has demonstrated that a highly selective CCR2 antagonist significantly decreased the GNT stimulation of PTX3 mRNA expression within the feline COC; however, it could not inhibit the stimulation of PTX3 mRNA levels induced by AREG (Jaworski et al., 2020), indicating that PTX3 stimulation by AREG is independent of the CCR2/MCP1 pathway. In that manuscript, we proposed that CCR2/MCP1 could act by modulating PTX3 indirectly through the induction/stimulation of AREG. Mainly because PTX3 plays a role in cumulus matrix stability (Salustri et al., 2004), which may be a later event in the matrix formation, while HAS2 and TNFAIP6 play an earlier role. Thus, the current results, showing that MCP1 could not revert the inhibition, support this idea. On the other hand, HAS2 mRNA expression surprisingly was not recovered by the presence of the recombinant MCP1. Based on our previous work that showed that the CCR2 antagonist significantly decreased the stimulation of HAS2 mRNA levels triggered by AREG within the feline COC (Jaworski et al., 2020), we had expected that MCP1 was going to revert the significant decrease triggered by the EGFR inhibitor. Furthermore, in the present study, the addition of recombinant MCP1 reversed the reduction of the GNT-stimulated HA synthesis within the COC triggered by the EGFR inhibitor. One possible explanation for this discrepancy could be that in the groups containing GNT + LAPATINIB, and GNT + LAPATINIB + MCP1 there was high variability within the HAS2 mRNA expression. High variability in the HA-IF was also observed in the GNT + LAPATINIB group, where we noted samples with a total blockage of HA synthesis while others with some HA-positive staining. So, this variability in the response to the inhibitor within the different COCs could eventually explain this discrepancy. Interestingly, in all the groups with MCP1, the presence of HA was always detected among the cumulus cells. In agreement with these observations, MCP1 was shown to directly stimulate HAS2 mRNA within the feline COC (Rojo et al., 2019). Regarding TNFAIP6, as the EGFR inhibitor did not have a significant effect on its mRNA levels, no further analysis comparing the presence of MCP1 is worth doing. Why the EGFR inhibitor did not have a significant effect on TNFAIP6 mRNA could be explained by the fact that GNT stimulation of TNFAIP6 depends mainly on the PGE2 pathway (Ochsner et al., 2003).
EGFR is a member of the ErbB family of RTKs, which is composed of 4 receptors (named ErbB1-4) (Herbst, 2004), and all of them have been detected in the ovary (Richani and Gilchrist, 2018). For example, these 4 transcripts have been observed in human preovulatory follicle granulosa and cumulus cells following hCG stimulation (Zamah et al., 2010). Whereas three of them (Erb1-3) have been shown to be constitutively expressed in mouse granulosa and cumulus cells (Noma et al., 2011; Richani and Gilchrist, 2018). Since we used a dual EGFR inhibitor (Lapatinib), that also inhibits ErbB2, we cannot discard that the effect observed was exclusively from the EGFR (also known as ErbB1) inhibition. ErbB2, has no known ligand, but it can dimerize with other ErbB receptors (Citri and Yarden, 2006). For example, ErbB3 requires heterodimerization with ErbB2 to become active after its ligands (neuregulins, NGR, 1 and 2) bind since it lacks tyrosine kinase activity (Citri and Yarden, 2006). But even though the EGF family comprises 11 proteins with highly similar functional and structural properties (Schneider and Wolf, 2009), in the preovulatory follicle only some of these members seem to be relevant, mainly AREG. And since AREG signals by binding EGFR only, it is very likely that the repression in the periovulatory genes observed in the presence of the EGFR inhibitor is due to the blockage of EGFR activation. For example, in rhesus macaque, the mRNA expression of AREG and EREG significantly increased in the preovulatory follicle after an ovulatory stimulus, whereas the BTC mRNA was minimal and did not vary throughout the periovulatory interval (Xu et al., 2011). Moreover, the mRNA expression of other EGF family members was minimal or undetectable in the primate preovulatory follicle (Xu et al., 2011). Furthermore, one of the authors demonstrated a rapid and significant increase in the AREG protein content in the primate follicular fluid in agreement with the mRNA data (Peluffo et al., 2012). In humans, higher levels of the AREG protein content than EGF were observed in the follicular fluid of women undergoing IVF protocols, having a concentration of around 100 ng/mL in comparison with 5 pg/mL, respectively (Inoue et al., 2009). Moreover, EREG and BTC peptides have not been distinguished in human follicular fluid or conditioned media from mouse COC (Zamah et al., 2010; Richani et al., 2013). In the domestic cat, NRG2 and ErbB2 transcript expression were the transcripts observed from the EGF family in the preantral follicles, with a sequential decrease of NRG2 expression from primordial to secondary follicles (Kehoe et al., 2021). Thus, even though we cannot completely discard the involvement of ErbB2, the evidence supports that the effects observed by the EGFR inhibitor are likely due to the blockage of EGFR activation.
Regarding oocyte maturation, the EGFR inhibitor significantly decreased the percentage of MII-stage oocytes in comparison to the GNT group, as expected. Based on the literature, where several papers have reported positive effects of EGF on oocyte maturation in different species including; women, cats, dogs, cows, pigs, sheep, horses, and mice (Rieger et al., 1998; De La Fuente et al., 1999; Guler et al., 2000; Procházka et al., 2000; Lorenzo et al., 2001; Gomez et al., 2003; Merlo et al., 2005; Cui et al., 2006). Interestingly, MCP1 was able to restore it. This result is in accordance with our previous data that showed a stimulatory effect of MCP1 on meiosis (Jaworski et al., 2020), but it also confirmed that CCR2/MCP1 signaling pathway is downstream EGFR/AREG pathway within the ovulatory cascade. Activation of the CCR2 signaling includes Ca2+, PKC, MAPK, and Rho GTPase (Sozzani et al., 1991; Yen et al., 1997; Ashida et al., 2001; Jones et al., 2003). Interestingly, the chemokine MCP1 has been shown to also reduce the accumulation of cAMP following stimulation of adenylate cyclase with forskolin (O’Boyle et al., 2007). Thus, the binding of MCP1 to its receptor triggers two main events that play a critical role in the re-initiation of meiosis, such as inhibition of the adenylate cyclase and stimulation of calcium influx. On the other hand, it is important to emphasize that EGFR inhibitor did not provoke a complete blockage on the oocyte maturation stimulated by GNT, as it did with the synthesis of HA and the production of P4, supporting the idea that GNT stimulation triggered different pathways together with the EGFR. It is important to appreciate that during the ovulatory cascade, multiple signaling pathways are activated and required to trigger all the necessary events that in vitro are hard to mimic. Since the GNT + LAPATINIB + MCP1 group was one of the groups with the highest percentage of MII-stage oocytes, this treatment could provide an additional good option for IVM in fertility clinics. However, further studies are needed to assess the oocyte developmental outcome of this treatment in vitro. Especially, as Richani and Gilchrist highlighted, EGF-like peptide signaling in granulosa and cumulus cells was critical not only for oocyte meiotic maturation but also for this signaling network coordinated oocyte cytoplasmic maturation, influencing the developmental capacity of the oocyte (Richani and Gilchrist, 2018).
E2 and P4 levels were assessed and detected in the media from COCs cultured under different treatments in both culture systems (C-OE and IVM). Steroid production at 24 h from the C-OE media was detected only in groups containing GNTs. Interestingly, the presence of the EGFR inhibitor blocked the P4 production stimulated by GNT, with MCP1 being able to restore its production by the COCs. In contrast, EGFR inhibitor did not have a significant effect on E2 levels. Intriguingly, it was reported that EGF-related factors mediate the GNT action through the induction of steroid and prostaglandin production (Jamnongjit et al., 2005; Shimada et al., 2006). It is known that P4 is essential for the normal development of periovulatory events, especially for follicular rupture (Gaytan et al., 2004). Moreover, P4 produced by the COC seems to play a role in oocyte nuclear maturation (Shimada and Terada, 2002). In rhesus macaques, for example, a P4 agonist treatment can promote oocyte maturation to the MII-stage, even in the absence of the LH surge (Borman et al., 2004). And more importantly, Tian et al. (2017) demonstrated that P4 from cumulus cells promotes oocyte developmental potential in mice. So, it is not surprising that the EGFR inhibitor completely blocked its production by GNT as it is known to be a paracrine mediator of the LH surge. But it is interesting and novel that the chemokine MCP1 did revert that. P4 synthesis and release from the cumulus cells during the ovulatory cascade are believed to have another important action. Based on the sperm chemotaxis model, the spermatozoa can sense a gradient of P4 derived from the cumulus cells surrounding the oocyte (after ovulation) and swim toward the source of P4 (Villanueva-Diaz et al., 1995). Thus, our current results demonstrate the interaction between EGFR and CCR2 signaling pathways in the periovulatory process (including P4 production) and they support a direct role for chemokines like MCP1 in regulating events necessary to ovulate a fertilizable oocyte. In agreement with these observations, rodent studies suggest that chemokine signaling regulates the assembly of the cumulus extracellular matrix and thus fertilization (Tamba et al., 2008). Also, it was reported that when PGE2 binds to its receptor subtype 2 (PTGER2) its actions were mediated by some chemokines in mice (Yodoi et al., 2009).
Regarding the steroid production of COC during IVM, the inhibitor did not significantly affect the production of E2 or P4 by the COCs. Although the highest concentrations were observed within the GNT and GNT + LAPATINIB + MCP1 groups. In this work, we demonstrated that feline cumulus cells can produce E2 and P4 in response to a stimulus using either of our culture systems (C-OE and IVM), with detectable levels in the culture media. In the eighties, McNatty et al. (1980) has shown that human COCs from antral follicles were steroidogenically competent units (capable of synthesizing progesterone, androgens, and estrogens) with the capacity to modify the endocrine microenvironment of the follicle. P4 produced by the COC was reported to participate in the optimal milieu within the follicle (Shimada and Terada, 2002). Based on our results, we believe that cumulus cells previously incorporated the precursors for the synthesis of these steroid hormones; although dedifferentiation of cumulus cells to granulosa cells cannot be discarded. Discrepancies in E2 and P4 values between the C-OE and IVM culture systems may be due to the differences in time (28 h vs. 24 h), the presence of FBS-charcoal vs. BSA, or differences in concentrations of GNT that were optimal for each system.
In summary, our results confirm the chemokine receptor CCR2 as a novel intermediate in the ovulatory cascade and demonstrate that the EGFR/AREG and the CCR2/MCP1 signaling pathways play critical roles in regulating feline C-OE and oocyte nuclear maturation, with CCR2/MCP1 signaling pathway being downstream EGFR/AREG pathway within the ovulatory cascade. Considering that AREG stimulates directly CCR2 and MCP1 mRNA, and MCP1 stimulates AREG mRNA, it is likely the existence of a positive loop within EGFR/AREG and CCR2/MCP1 pathways, that may guarantee and propagate the periovulatory process triggered by LH. Figure 6 shows a scheme of the proposed interaction between CCR2 and EGFR pathways in the C-OE process and oocyte maturation. Therefore, a better understanding of these novel interactions within the COC during the ovulatory cascade could aid in increasing our understanding of events required for fertilization, thereby leading to the identification of possible causes of infertility and/or novel markers for oocyte quality that would improve IVM culture conditions and help in the identification of novel non-hormonal targets for contraception, as well as.
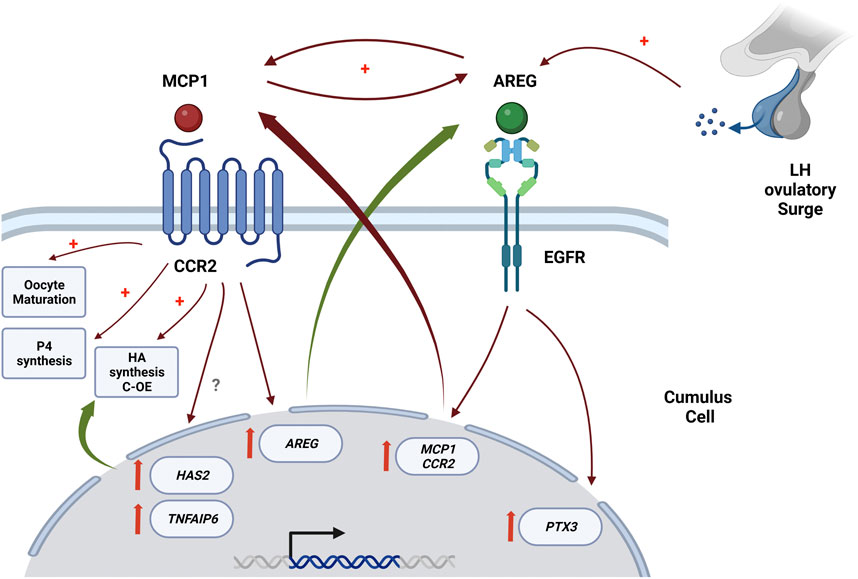
FIGURE 6. Proposed interaction between CCR2 and EGFR pathways in the C-OE process and oocyte maturation, with CCR2/MCP1 signaling pathway being downstream of the EGFR in the ovulatory cascade. This scheme is proposed based on mainly our current results in conjunction with some of our previously published results (Rojo et al., 2019; Jaworski et al., 2020). This figure was creating in QmlvcmVuZGVyLmNvbQ==.
Data availability statement
The original contributions presented in the study are included in the article/Supplementary Material, further inquiries can be directed to the corresponding author.
Ethics statement
Ethical review and approval was not required for the animal study because The study did not require the approval of the Animal Care and Use Committee because cat ovaries were collected at local routine spaying procedures as byproducts from owner-requested routine ovariohysterectomies. Written informed consent for participation was not obtained from the owners because The study did not require informed consent because cat ovaries were donated as byproducts/discarded material from routine spaying procedures.
Author contributions
JC contributed to the design and performance of the research, analyzed data, and contributed to the writing of the manuscript; MT and MB contributed to the design and performance of the research; BP contributed with the analysis of the data; GJ contributed with design of the research together with analyses of data; MP designed and performed research, analyzed data and wrote/corrected the manuscript. All the authors read and reviewed the manuscript.
Funding
This study was supported by PRESTAMO BID PICT 2020 No 01128 and PIP 2020 N° 0606.
Acknowledgments
Special thanks are due to DVMs Jimena Sosa and Maximiliano D. Collado from the “Centro de Salud Animal de la Municipalidad de Merlo” (Provincia de Buenos Aires, Argentina) for the donation of the feline ovaries. Also, we would like to thank Drs. Roxana Schillaci, Cecilia Mathó, and Ixiel Peluffo for their help with some reagents. We also want to thank German La lacona for his technical assistance with the confocal microscopy. Recombinant human FSH and LH (Merck Serono) were generously donated to the laboratory.
Conflict of interest
The authors declare that the research was conducted in the absence of any commercial or financial relationships that could be construed as a potential conflict of interest.
Publisher’s note
All claims expressed in this article are solely those of the authors and do not necessarily represent those of their affiliated organizations, or those of the publisher, the editors and the reviewers. Any product that may be evaluated in this article, or claim that may be made by its manufacturer, is not guaranteed or endorsed by the publisher.
Supplementary material
The Supplementary Material for this article can be found online at: https://www.frontiersin.org/articles/10.3389/fcell.2023.1161813/full#supplementary-material
References
Ashida, N., Arai, H., Yamasaki, M., and Kita, T. (2001). Distinct signaling pathways for MCP-1-dependent integrin activation and chemotaxis. J. Biol. Chem. 276, 16555–16560. doi:10.1074/jbc.M009068200
Ashkenazi, H., Cao, X., Motola, S., Popliker, M., Conti, M., and Tsafriri, A. (2005). Epidermal growth factor family members: Endogenous mediators of the ovulatory response. Endocrinology 146, 77–84. doi:10.1210/en.2004-0588
Baudhuin, L. M., Jiang, Y., Zaslavsky, A., Ishii, I., Chun, J., and Xu, Y. (2004). S1P3-mediated Akt activation and cross-talk with platelet-derived growth factor receptor (PDGFR). FASEB J. 18, 341–343. doi:10.1096/fj.03-0302fje
Borman, S. M., Chaffin, C. L., Schwinof, K. M., Stouffer, R. L., and Zelinski-Wooten, M. B. (2004). Progesterone promotes oocyte maturation, but not ovulation, in nonhuman primate follicles without a gonadotropin surge. Biol. Reprod. 71, 366–373. doi:10.1095/biolreprod.103.023390
Chen, L., Mao, S. J., and Larsen, W. J. (1992). Identification of a factor in fetal bovine serum that stabilizes the cumulus extracellular matrix. A role for a member of the inter-alpha-trypsin inhibitor family. J. Biol. Chem. 267, 12380–12386. doi:10.1016/s0021-9258(19)49851-7
Chen, L., Russell, P. T., and Larsen, W. J. (1993). Functional significance of cumulus expansion in the mouse: Roles for the preovulatory synthesis of hyaluronic acid within the cumulus mass. Mol. Reprod. Dev. 34, 87–93. doi:10.1002/mrd.1080340114
Citri, A., and Yarden, Y. (2006). EGF-ERBB signalling: Towards the systems level. Nat. Rev. Mol. Cell. Biol. 7, 505–516. doi:10.1038/nrm1962
Combelles, C. M. H., Cekleniak, N. A., Racowsky, C., and Albertini, D. F. (2002). Assessment of nuclear and cytoplasmic maturation in in-vitro matured human oocytes. Hum. Reprod. 17, 1006–1016. doi:10.1093/humrep/17.4.1006
Comizzoli, P., Wildt, D. E., and Pukazhenthi, B. S. (2003). Overcoming poor in vitro nuclear maturation and developmental competence of domestic cat oocytes during the non-breeding season. Reproduction 126, 809–816. doi:10.1530/rep.0.1260809
Cui, X. S., Jin, Y. X., Shen, X. H., Lee, J. Y., Lee, H. S., Yin, X. J., et al. (2006). Epidermal growth factor enhances meiotic resumption of canine oocytes in the presence of BSA. Theriogenology 66, 267–274. doi:10.1016/j.theriogenology.2005.11.011
Daub, H., Ulrich Weiss, F., Wallasch, C., and Ullrich, A. (1996). Role of transactivation of the EGF receptor in signalling by G-protein-coupled receptors. Nature 379, 557–560. doi:10.1038/379557a0
De La Fuente, R., O’Brien, M. J., and Eppig, J. J. (1999). Epidermal growth factor enhances preimplantation developmental competence of maturing mouse oocytes. Hum. Reprod. 14, 3060–3068. doi:10.10.93/humrep/14.12.3060
Eguchi, S., Numaguchi, K., Iwasaki, H., Matsumoto, T., Yamakawa, T., Utsunomiya, H., et al. (1998). Calcium-dependent epidermal growth factor receptor transactivation mediates the angiotensin II-induced mitogen-activated protein/kinase activation in vascular smooth muscle cells. J. Biol. Chem. 273, 8890–8896. doi:10.1074/jbc.273.15.8890
Eppig, J. J., and O’Brien, M. J. (1996). Development in vitro of mouse oocytes from primordial follicles. Biol. Reprod. 54, 197–207. doi:10.1095/biolreprod54.1.197
Fougerat, A., Smirnova, N. F., Gayral, S., Malet, N., Hirsch, E., Wymann, M. P., et al. (2012). Key role of PI3Kγ in monocyte chemotactic protein-1-mediated amplification of PDGF-induced aortic smooth muscle cell migration. Br. J. Pharmacol. 166, 1643–1653. doi:10.1111/j.1476-5381.2012.01866.x
Freire, A. V., Escobar, M. E., Gryngarten, M. G., Arcari, A. J., Ballerini, M. G., Bergada, I., et al. (2013). High diagnostic accuracy of subcutaneous Triptorelin test compared with GnRH test for diagnosing central precocious puberty in girls. Clin. Endocrinol. (Oxf). 78, 398–404. doi:10.1111/j.1365-2265.2012.04517.x
Frijns, E., Sachs, N., Kreft, M., Wilhelmsen, K., and Sonnenberg, A. (2010). EGF-induced MAPK signaling inhibits hemidesmosome formation through phosphorylation of the integrin {beta}4. J. Biol. Chem. 285, 37650–37662. doi:10.1074/jbc.M110.138818
Fulop, C., Salustri, A., and Hascall, V. C. (1997). Coding sequence of a hyaluronan synthase homologue expressed during expansion of the mouse cumulus-oocyte complex. Arch. Biochem. Biophys. 337, 261–266. doi:10.1006/abbi.1996.9793
Fulop, C., Szanto, S., Mukhopadhyay, D., Bardos, T., Kamath, R. V., Rugg, M. S., et al. (2003). Impaired cumulus mucification and female sterility in tumor necrosis factor-induced protein-6 deficient mice. Development 130, 2253–2261. doi:10.1242/dev.00422
Gaytan, M., Bellido, C., Morales, C., Gonzalez-Padilla, M., Sanchez-Criado, J. E., and Gaytan, F. (2004). Immature rats show ovulatory defects similar to those in adult rats lacking prostaglandin and progesterone actions. Reprod. Biol. Endocrinol. 2, 63–12. doi:10.1186/1477-7827-2-63
Gomez, M. C., Pope, E., Harris, R., Mikota, S., and Dresser, B. L. (2003). Development of in vitro matured, in vitro fertilized domestic cat embryos following cryopreservation, culture and transfer. Theriogenology 60, 239–251. doi:10.1016/s0093-691x(03)00004-9
Guler, A., Poulin, N., Mermillod, P., Terqui, M., and Cognie, Y. (2000). Effect of growth factors, EGF and IGF-I, and estradiol on in vitro maturation of sheep oocytes. Theriogenology 54, 209–218. doi:10.1016/s0093-691x(00)00342-3
Herbst, R. S. (2004). Review of epidermal growth factor receptor biology. Int. J. Radiat. Oncol. Biol. Phys. 59, S21–S26. doi:10.1016/j.ijrobp.2003.11.041
Hess, K. A., Chen, L., and Larsen, W. J. (1999). Inter-alpha-inhibitor binding to hyaluronan in the cumulus extracellular matrix is required for optimal ovulation and development of mouse oocytes. Biol. Reprod. 61, 436–443. doi:10.1095/biolreprod61.2.436
Hsieh, M., Lee, D., Panigone, S., Horner, K., Chen, R., Theologis, A., et al. (2007). Luteinizing hormone-dependent activation of the epidermal growth factor network is essential for ovulation. Mol. Cell. Biol. 27, 1914–1924. doi:10.1128/MCB.01919-06
Inoue, Y., Miyamoto, S., Fukami, T., Shirota, K., Yotsumoto, F., and Kawarabayashi, T. (2009). Amphiregulin is much more abundantly expressed than transforming growth factor-alpha and epidermal growth factor in human follicular fluid obtained from patients undergoing in vitro fertilization-embryo transfer. Fertil. Steril. 91, 1035–1041. doi:10.1016/j.fertnstert.2008.01.014
Jamnongjit, M., Gill, A., and Hammes, S. R. (2005). Epidermal growth factor receptor signaling is required for normal ovarian steroidogenesis and oocyte maturation. Proc. Natl. Acad. Sci. U. S. A. 102, 16257–16262. doi:10.1073/pnas.0508521102
Jaworski, J. P., Urrutia, M., Dascal, E., Jaita, G., and Peluffo, M. C. (2020). C-C motif chemokine receptor 2 as a novel intermediate in the ovulatory cascade. Mol. Hum. Reprod. 26, 289–300. doi:10.1093/molehr/gaaa020
Jones, G. E., Prigmore, E., Calvez, R., Hogan, C., Dunn, G. A., Hirsch, E., et al. (2003). Requirement for PI 3-kinase gamma in macrophage migration to MCP-1 and CSF-1. Exp. Cell. Res. 290, 120–131. doi:10.1016/s0014-4827(03)00318-5
Kehoe, S., Jewgenow, K., Johnston, P. R., Mbedi, S., and Braun, B. C. (2021). Signalling pathways and mechanistic cues highlighted by transcriptomic analysis of primordial, primary, and secondary ovarian follicles in domestic cat. Sci. Rep. 11, 2683. doi:10.1038/s41598-021-82051-4
Kimura, N., Konno, Y., Miyoshi, K., Matsumoto, H., and Sato, E. (2002). Expression of hyaluronan synthases and CD44 messenger RNAs in porcine cumulus-oocyte complexes during in vitro maturation. Biol. Reprod. 66, 707–717. doi:10.1095/biolreprod66.3.707
Liu, Z. X., Wang, J., Yu, L., Tang, K. L., Wang, X., and Li, S. (2008). [Effect of epidermal growth factor on transcription of MCP-1 in rat dental follicle cells]. Shanghai Kou Qiang Yi Xue 17, 212–215.
Lorenzo, P. L., Liu, I. K. M., Illera, J. C., Picazo, R. A., Carneiro, G. F., Illera, M. J., et al. (2001). Influence of epidermal growth factor on mammalian oocyte maturation via tyrosine-kinase pathway. J. Physiol. Biochem. 57, 15–22. doi:10.1007/BF03179809
Luttrell, L. M., Daaka, Y., and Lefkowitz, R. J. (1999). Regulation of tyrosine kinase cascades by G-protein-coupled receptors. Curr. Opin. Cell. Biol. 11, 177–183. doi:10.1016/s0955-0674(99)80023-4
McNatty, K. P., Moore Smith, D., Makris, A., Osathanondh, R., and Ryan, K. J. (1980). Steroidogenesis by the human oocyte-cumulus cell complex in vitro. Steroids 35, 643–651. doi:10.1016/0039-128x(80)90089-6
Merlo, B., Iacono, E., Zambelli, D., Prati, F., and Belluzzi, S. (2005). Effect of EGF on in vitro maturation of domestic cat oocytes. Theriogenology 63, 2032–2039. doi:10.1016/j.theriogenology.2004.09.045
Mukhopadhyay, D., Hascall, V. C., Day, A. J., Salustri, A., and Fulop, C. (2001). Two distinct populations of tumor necrosis factor-stimulated gene-6 protein in the extracellular matrix of expanded mouse cumulus cell-oocyte complexes. Arch. Biochem. Biophys. 394, 173–181. doi:10.1006/abbi.2001.2552
Noma, N., Kawashima, I., Fan, H. Y., Fujita, Y., Kawai, T., Tomoda, Y., et al. (2011). LH-induced neuregulin 1 (nrg1) type iii transcripts control granulosa cell differentiation and oocyte maturation. Mol. Endocrinol. 25, 104–116. doi:10.1210/me.2010-0225
O’Boyle, G., Brain, J. G., Kirby, J. A., and Ali, S. (2007). Chemokine-mediated inflammation: Identification of a possible regulatory role for CCR2. Mol. Immunol. 44, 1944–1953. doi:10.1016/j.molimm.2006.09.033
Ochsner, S. A., Russell, D. L., Day, A. J., Breyer, R. M., and Richards, J. S. (2003). Decreased expression of tumor necrosis factor-alpha-stimulated gene 6 in cumulus cells of the cyclooxygenase-2 and EP2 null mice. Endocrinology 144, 1008–1019. doi:10.1210/en.2002-220435
Park, J. Y., Su, Y. Q., Ariga, M., Law, E., Jin, S. L. C., and Conti, M. (2004). EGF-like growth factors as mediators of LH action in the ovulatory follicle. Science 303, 682–684. doi:10.1126/science.1092463
Peluffo, M. C., Barrett, S. L., Stouffer, R. L., Hennebold, J. D., and Zelinski, M. B. (2010). Cumulus-oocyte complexes from small antral follicles during the early follicular phase of menstrual cycles in rhesus monkeys yield oocytes that reinitiate meiosis and fertilize in vitro. Biol. Reprod. 83, 525–532. doi:10.1095/biolreprod.110.084418
Peluffo, M. C., Ting, A. Y., Zamah, A. M., Conti, M., Stouffer, R. L., Zelinski, M. B., et al. (2012). Amphiregulin promotes the maturation of oocytes isolated from the small antral follicles of the rhesus macaque. Hum. Reprod. 27, 2430–2437. doi:10.1093/humrep/des158
Peluffo, M. C., Stanley, J., Braeuer, N., Rotgeri, A., Fritzemeier, K. H., Fuhrmann, U., et al. (2014). A prostaglandin E2 receptor antagonist prevents pregnancies during a preclinical contraceptive trial with female macaques. Hum. Reprod. 29, 1400–1412. doi:10.1093/humrep/deu083
Peng, X. R., Hsueh, A. J. W., LaPolt, P. S., Bjersing, L., and Ny, T. (1991). Localization of luteinizing hormone receptor messenger ribonucleic acid expression in ovarian cell types during follicle development and ovulation. Endocrinology 129, 3200–3207. doi:10.1210/endo-129-6-3200
Procházka, R., Sršeň, V., Nagyová, E., Miyano, T., and Flechon, J. E. (2000). Developmental regulation of effect of epidermal growth factor on porcine oocyte-cumulus cell complexes: Nuclear maturation, expansion, and F-actin remodeling. Mol. Reprod. Dev. 56, 63–73. doi:10.1002/(SICI)1098-2795(200005)56:1<63::AID-MRD8>3.0.CO;2-D
Rabinovitz, I., Tsomo, L., and Mercurio, A. M. (2004). Protein kinase C-alpha phosphorylation of specific serines in the connecting segment of the beta 4 integrin regulates the dynamics of type II hemidesmosomes. Mol. Cell. Biol. 24, 4351–4360. doi:10.1128/MCB.24.10.4351-4360.2004
Richani, D., and Gilchrist, R. B. (2018). The epidermal growth factor network: Role in oocyte growth, maturation and developmental competence. Hum. Reprod. Update 24, 1–14. doi:10.1093/humupd/dmx029
Richani, D., Ritter, L. J., Thompson, J. G., and Gilchrist, R. B. (2013). Mode of oocyte maturation affects EGF-like peptide function and oocyte competence. Mol. Hum. Reprod. 19, 500–509. doi:10.1093/molehr/gat028
Richards, J. S. (2005). Ovulation: New factors that prepare the oocyte for fertilization. Mol. Cell. Endocrinol. 234, 75–79. doi:10.1016/j.mce.2005.01.004
Rieger, D., Luciano, A. M., Modina, S., Pocar, P., Lauria, A., and Gandolfi, F. (1998). The effects of epidermal growth factor and insulin-like growth factor I on the metabolic activity, nuclear maturation and subsequent development of cattle oocytes in vitro. J. Reprod. Fertil. 112, 123–130. doi:10.1530/jrf.0.1120123
Rojo, J. L., Linari, M., Musse, M. P., and Peluffo, M. C. (2015). Felis catus ovary as a model to study follicle biology in vitro. J. Assist. Reprod. Genet. 32, 1105–1111. doi:10.1007/s10815-015-0511-5
Rojo, J. L., Linari, M., Young, K. A., and Peluffo, M. C. (2018). Stromal-derived factor 1 directly promotes genes expressed within the ovulatory cascade in feline cumulus oocyte complexes. J. Assist. Reprod. Genet. 35, 785–792. doi:10.1007/s10815-018-1150-4
Rojo, J. L., Jaworski, J. P., and Peluffo, M. C. (2019). Direct role of the C-C motif chemokine receptor 2/monocyte chemoattractant protein 1 system in the feline cumulus oocyte complex. Biol. Reprod. 100, 1046–1056. doi:10.1093/biolre/ioy237
Russell, D. L., Doyle, K. M. H., Ochsner, S. A., Sandy, J. D., and Richards, J. A. S. (2003). Processing and localization of ADAMTS-1 and proteolytic cleavage of versican during cumulus matrix expansion and ovulation. J. Biol. Chem. 278, 42330–42339. doi:10.1074/jbc.M300519200
Salustri, A., Garlanda, C., Hirsch, E., De Acetis, M., Maccagno, A., Bottazzi, B., et al. (2004). PTX3 plays a key role in the organization of the cumulus oophorus extracellular matrix and in in vivo fertilization. Development 131, 1577–1586. doi:10.1242/dev.01056
Schneider, M. R., and Wolf, E. (2009). The epidermal growth factor receptor ligands at a glance. J. Cell. Physiol. 218, 460–466. doi:10.1002/jcp.21635
Shimada, M., and Terada, T. (2002). FSH and LH induce progesterone production and progesterone receptor synthesis in cumulus cells: A requirement for meiotic resumption in porcine oocytes. Mol. Hum. Reprod. 8, 612–618. doi:10.1093/molehr/8.7.612
Shimada, M., Hernandez-Gonzalez, I., Gonzalez-Robayna, I., and Richards, J. A. S. (2006). Paracrine and autocrine regulation of epidermal growth factor-like factors in cumulus oocyte complexes and granulosa cells: Key roles for prostaglandin synthase 2 and progesterone receptor. Mol. Endocrinol. 20, 1352–1365. doi:10.1210/me.2005-0504
Sozzani, S., Luini, W., Molino, M., Jilek, P., Bottazzi, B., Cerletti, C., et al. (1991). The signal transduction pathway involved in the migration induced by a monocyte chemotactic cytokine. J. Immunol. 147, 2215–2221. doi:10.4049/jimmunol.147.7.2215
Sun, F. Z., and Moor, R. M. (1991). Nuclear-cytoplasmic interactions during ovine oocyte maturation. Development 111, 171–180. doi:10.1242/dev.111.1.171
Tamba, S., Yodoi, R., Segi-Nishida, E., Ichikawa, A., Narumiya, S., and Sugimoto, Y. (2008). Timely interaction between prostaglandin and chemokine signaling is a prerequisite for successful fertilization. Proc. Natl. Acad. Sci. U. S. A. 105, 14539–14544. doi:10.1073/pnas.0805699105
Tian, X., Anthony, K., and Diaz, F. J. (2017). Transition metal chelator induces progesterone production in mouse cumulus-oocyte complexes and corpora lutea. Biol. Trace Elem. Res. 176, 374–383. doi:10.1007/s12011-016-0841-x
Ueda, A., Okuda, K., Ohno, S., Shirai, A., Igarashi, T., Matsunaga, K., et al. (1994). NF-kappa B and Sp1 regulate transcription of the human monocyte chemoattractant protein-1 gene. J. Immunol. 153, 2052–2063. doi:10.4049/jimmunol.153.5.2052
Ueda, A., Ishigatsubo, Y., Okubo, T., and Yoshimura, T. (1997). Transcriptional regulation of the human monocyte chemoattractant protein-1 gene: Cooperation of two NF-κB sites and NF-κB/Rel subunit specificity. J. Biol. Chem. 272, 31092–31099. doi:10.1074/jbc.272.49.31092
Vecchione, C., Patrucco, E., Marino, G., Barberis, L., Poulet, R., Aretini, A., et al. (2005). Protection from angiotensin II-mediated vasculotoxic and hypertensive response in mice lacking PI3Kgamma. J. Exp. Med. 201, 1217–1228. doi:10.1084/jem.20040995
Villanueva-Diaz, C., Arias-Martinez, J., Bermejo-Martinez, L., and Vadillo-Ortega, F. (1995). Progesterone induces human sperm chemotaxis. Fertil. Steril. 64, 1183–1188. doi:10.1016/s0015-0282(16)57982-5
Voisin, L., Foisy, S., Giasson, E., Lambert, C., Moreau, P., and Meloche, S. (2002). EGF receptor transactivation is obligatory for protein synthesis stimulation by G protein-coupled receptors. Am. J. Physiol. Cell. Physiol. 283, 446–455. doi:10.1152/ajpcell.00261.2001
Wildt, D. E., Comizzoli, P., Pukazhenthi, B., and Songsasen, N. (2010). Lessons from biodiversity–the value of nontraditional species to advance reproductive science, conservation, and human health. Mol. Reprod. Dev. 77, 397–409. doi:10.1002/mrd.21137
Xu, F., Stouffer, R. L., Muller, J., Hennebold, J. D., Wright, J. W., Bahar, A., et al. (2011). Dynamics of the transcriptome in the primate ovulatory follicle. Mol. Hum. Reprod. 17, 152–165. doi:10.1093/molehr/gaq089
Yen, H., Zhang, Y., Penfold, S., and Rollins, B. J. (1997). MCP-1-mediated chemotaxis requires activation of non-overlapping signal transduction pathways. J. Leukoc. Biol. 61, 529–532. doi:10.1002/jlb.61.4.529
Yodoi, R., Tamba, S., Morimoto, K., Segi-Nishida, E., Nishihara, M., Ichikawa, A., et al. (2009). RhoA/Rho kinase signaling in the cumulus mediates extracellular matrix assembly. Endocrinology 150, 3345–3352. doi:10.1210/en.2008-1449
Zamah, A. M., Hsieh, M., Chen, J., Vigne, J. L., Rosen, M. P., Cedars, M. I., et al. (2010). Human oocyte maturation is dependent on LH-stimulated accumulation of the epidermal growth factor-like growth factor, amphiregulin. Hum. Reprod. 25, 2569–2578. doi:10.1093/humrep/deq212
Zheng, P. (2007). Effects of in vitro maturation of monkey oocytes on their developmental capacity. Anim. Reprod. Sci. 98, 56–71. doi:10.1016/j.anireprosci.2006.10.005
Zhu, J., Jia, X., Xiao, G., Kang, Y., Partridge, N. C., and Qin, L. (2007). EGF-Like ligands stimulate osteoclastogenesis by regulating expression of osteoclast regulatory factors by osteoblasts: Implications for osteolytic bone metastases. J. Biol. Chem. 282, 26656–26665. doi:10.1074/jbc.M705064200
Keywords: epidermal growth factor receptor, C-C motif chemokine receptor 2, monocyte chemoattractant protein 1, cumulus-oocyte complex, feline, ovulatory cascade genes, cumulus-oocyte expansion, oocyte nuclear maturation
Citation: Conte JG, Tellechea ML, Park B, Ballerini MG, Jaita G and Peluffo MC (2023) Interaction between epidermal growth factor receptor and C-C motif chemokine receptor 2 in the ovulatory cascade. Front. Cell Dev. Biol. 11:1161813. doi: 10.3389/fcell.2023.1161813
Received: 08 February 2023; Accepted: 27 March 2023;
Published: 04 April 2023.
Edited by:
Jennifer R. Wood, University of Nebraska System, United StatesReviewed by:
Carl W. White, University of Western Australia, AustraliaSarah Moorey, The University of Tennessee, Knoxville, United States
Copyright © 2023 Conte, Tellechea, Park, Ballerini, Jaita and Peluffo. This is an open-access article distributed under the terms of the Creative Commons Attribution License (CC BY). The use, distribution or reproduction in other forums is permitted, provided the original author(s) and the copyright owner(s) are credited and that the original publication in this journal is cited, in accordance with accepted academic practice. No use, distribution or reproduction is permitted which does not comply with these terms.
*Correspondence: M. C. Peluffo, bXBlbHVmZm9AY2VkaWUub3JnLmFy