- 1Department of Genetics and Cell Biology, Basic Medical College, Qingdao University, Qingdao, China
- 2Department of Traditional Chinese Medicine, The People’s Hospital of Zhaoyuan City, Yantai, China
- 3Health Science Center, Qingdao University, Qingdao, China
- 4Department of Hematology, The Affiliated Hospital of Qingdao University, Qingdao, China
The annexin A (ANXA) protein family is a well-known tissue-specific multigene family that encodes Ca2+ phospholipid-binding proteins. A considerable amount of literature is available on the abnormal expression of ANXA proteins in various malignant diseases, including cancer, atherosclerosis and diabetes. As critical regulatory molecules in cancer, ANXA proteins play an essential role in cancer progression, proliferation, invasion and metastasis. Recent studies about their structure, biological properties and functions in different types of cancers are briefly summarised in this review. We further discuss the use of ANXA as new class of targets in the clinical diagnosis and treatment of cancer.
1 Introduction
ANXs are proteins that are encoded by a highly conserved multigene family and are widely found in animals, plants and protists (Geisow et al., 1987). In 1978, ANXs were given different names based on their different biochemical properties and were divided into five categories: A, B, C, D and E2. The ANXA protein family in humans contains 12 species, ANXA1– ANXA11 and ANXA13 (Croissant et al., 2021), all of which are small soluble proteins. They are uniquely expressed in specific tissues; for example, ANXA3 induces human umbilical vein endothelial cell migration and tubulogenesis (Park et al., 2005), ANXA4 is specifically expressed in cochlear and vestibular hair cells (Li et al., 2021a), while, while ANXA5 participates in the activation of immune T cells (Hu et al., 2020).
Tissue-specific ANXA proteins exist in different cancers, including hepatocellular carcinoma (Dong et al., 2014), gastric cancer (Wang et al., 2013), breast cancer (Sharma et al., 2012), ovarian cancer (Manai et al., 2020) and lung cancer (Zhou et al., 2021a). In addition, numerous studies have indicated that ANXA is expressed irregularly in disparate cancers. For instance, ANXA2 is elevated in breast cancer and stimulates fibrinolytic enzyme production, which results in angiogenesis and metastasis. In contrast, ANXA6 expression is downregulated in primary gastric cancer tissues and directly inactivates the Ras/MAPK signalling pathway (Wang et al., 2013) to inhibit gastric cancer growth. Aside from ANXA2 and ANXA6, other ANXA family proteins can be used as markers of tumour occurrence and development as they affect a variety of signal pathways, such as cancer cell invasion, migration, apoptosis and autophagy. Furthermore, some ANXA proteins cause drug resistance and are thus potential therapeutic targets for tumours. A discussion of ANXA and their inhibitors may provide better strategies for the early diagnosis, clinical treatment and prognosis of cancer.
2 The molecular structure of ANXA protein family
The ANXA protein family contains structural and biological features. Structurally, the C-terminal region is conserved, with most membrane-bound proteins consisting of four fragments of around 70 amino acids each, which are internally and intrinsically homologous to the membrane-bound proteins. An exception is the ANXA6 protein, which has eight core fragments that form a high alpha helix and a closely spaced disc-like structure (Figure 1) (Li et al., 2022).
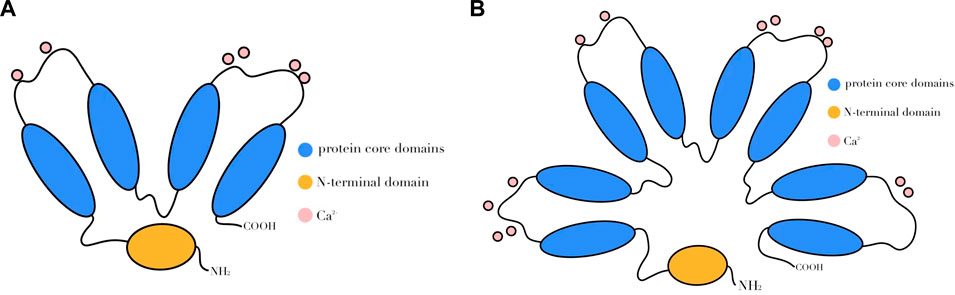
FIGURE 1. Schematic diagram of ANXA protein structure. ANXA consists of two regions, one of which is the C-terminus, the characteristic highly conserved core structural domain that usually consists of four repetitive sequences (A), while ANXA6 has eight repetitive sequences (B), each of which contains a calcium-binding motif. Another region is the N-terminal, where the amino acid arrangement order and length of each ANXA varies.
The biological functions of the superfamily members differ depending on the N-terminal domain, which is where most of their post-translational modifications occur (Wang et al., 2019). In biology, the ANXA protein family is a group of calcium-dependent phospholipid-binding proteins, so called because they only bind to phospholipid membranes with conformational changes in the presence of Ca2+12. This binding is, however, reversible. The removal of Ca2+ reveals the N-terminus hidden in the centre of the C-terminus (Rintala-Dempsey et al., 2008). The coordination of ANXA to calcium ions requires the involvement of water molecules to ensure the two ligands remain stable (Rintala-Dempsey et al., 2008). Besides Ca2+, the N-terminal of ANXA has multiple binding sites, including interacting partners. For instance, the S100 family, which comprises electron chiral Ca2+ binding proteins (Bharadwaj et al., 2013), functions to regulate cell proliferation, membrane transport and calcium homeostasis (Donato et al., 2013). The S100–ANXA interaction has been shown to play a role in membrane fusion by linking an S100 protein to two ANXA proteins on a phospholipid membrane (Rintala-Dempsey et al., 2008). Because of these different sequences in the N-terminal, ANXA members display distinctive properties and diverse functions. ANXA3 has a strong binding ability to phospholipids via the substitution of classical Trp-5 with alanine (Hofmann et al., 2000), while ANXA1 phosphorylates diverse protein residues via its N-terminal kinases characteristic (Rosengarth et al., 2001). Generally, these subtle changes do not affect their basic structure of ANXA proteins.
3 The role of ANXA protein family in cancer progression
3.1 The role of ANXA protein family in cell proliferation
The ANXA protein family can inhibit tumour proliferation by affecting cell membrane and cytoskeleton formation. In nasopharyngeal carcinoma, decreased ANXA1 leads to the upregulation of S100A9/vimentin, which increases tumour invasion by regulating the function of the cytoskeletal proteins (Xiao et al., 2017). When ANXA2 expression is effectively inhibited, the growth and motility of both the human colorectal cancer cell line CACO2 and human hepatocellular carcinoma cell line SMMC7721 are significantly reduced, and the motility-associated microstructures, such as pseudopods and filamentous pseudopods, as well as the polymerisation of microfilaments and microtubules, are significantly inhibited (He et al., 2019).
The members of the ANXA protein family play the opposing roles of promoting and inhibiting tumour proliferation through their participation in or activation of signalling pathways. The exceptions are ANXA5, ANXA6, ANXA10 and ANXA11, which play roles in several tumour types, while other ANXA members promote tumour cell proliferation (Grewal et al., 2010; Li et al., 2018a; Liu et al., 2019; Liu et al., 2020; Liu et al., 2021a; Zhou et al., 2021a; Zhou et al., 2021b; Gu et al., 2021; Huang et al., 2021; Ju et al., 2021; Wei and Zhu, 2021). In hepatocellular carcinoma, the overexpression of ANXA2 (Zhang et al., 2013), ANXA3 (Guo et al., 2021a), ANXA4 (Liu et al., 2017), ANXA5 (Sun et al., 2018) and ANXA11 (Liu et al., 2019) promotes proliferation because ANXA2–ANXA5 affect Wnt/β-catenin, MEK-ERK, PI3K/AKT-HIF-α and other pathways. It is worth mentioning that ANXA4 may be a key factor in hepatocellular carcinoma tumorigenesis (Liu et al., 2017), as downregulating ANXA4 expression can inhibit hepatocellular carcinoma proliferation and tumorigenesis in vitro and in vivo. Similarly, in lung cancer, the overexpression of ANXA8 activates the EGFR/AKT/mTOR signalling pathway to promote proliferation (Zhou et al., 2021a), while in thyroid cancer, increased ANXA1 promotes thyroid cancer proliferation by mediating IL-6/JAK2/STAT3 (Zhao et al., 2021). In contrast, the overexpression of the ANXA10 gene significantly inhibits HepG2 cell proliferation in vitro, and its downregulation inhibits papillary thyroid cancer proliferation; it acts directly on TSG101 by inactivating the MAPK/ERK signalling pathway (Wei and Zhu, 2021). In gastric cancer, different ANXA proteins show either proliferation promoting or inhibiting effects. For instance, ANXA2 directs the interaction of the protein YES1 and involves the EphA2-YES1-ANXA2 pathway (Mao et al., 2021), which promotes gastric cancer progression and metastasis, while ANXA6 (Wang et al., 2013) suppresses gastric cancer progression by inhibiting Ras/MAPK signalling. Moreover, increased ANXA5 inhibits cervical cancer proliferation by regulating the expression of Bcl-2 and Bax (Li et al., 2018a). A large number of studies have demonstrated that ANXA proteins are involved in the regulation of tumour proliferation, and these studies may provide new ideas and directions for research on tumour prevention and targeted therapies depending on the understanding of their role in the pathogenesis of malignant tumours (Figure 2).
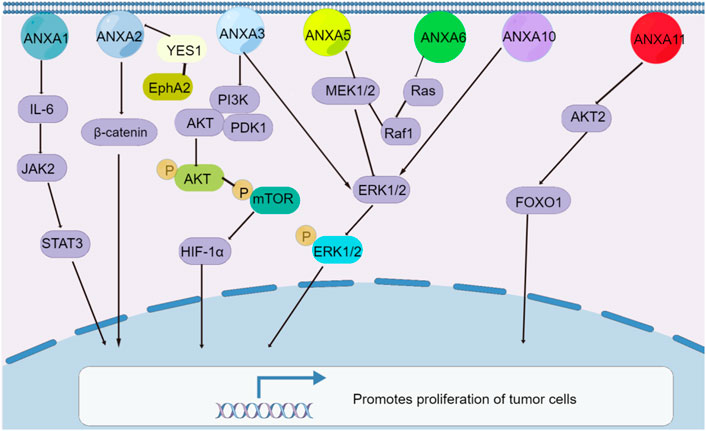
FIGURE 2. Signaling pathways of ANXA protein family regulating tumor cell proliferation. Except for ANXA6, the rest of the ANXA protein family was overexpressed and promoted the proliferation of tumor cells.
3.2 The role of the ANXA protein family in the cell cycle
The cell cycle is a complex process involving many regulatory proteins that guide cells through specific sequences of events that culminate in the production of two daughter cells (Schafer, 1998). The ANXA protein family plays critical roles in cell cycle regulation in a complex manner (Figure 3). ANXA1 promotes G0/G1 phase cyclic arrest in leukaemic K562 and U937 cells and promotes apoptosis in leukaemia (Tsai et al., 2013; Sabran et al., 2019). In one study, silencing ANXA1 in hypopharyngeal carcinoma not only significantly decreased the proportion of G0/G1 phase cells, but significantly increased the proportion of S phase cells, which enhanced cell activity and promoted cell cycle progression (Li et al., 2021b).
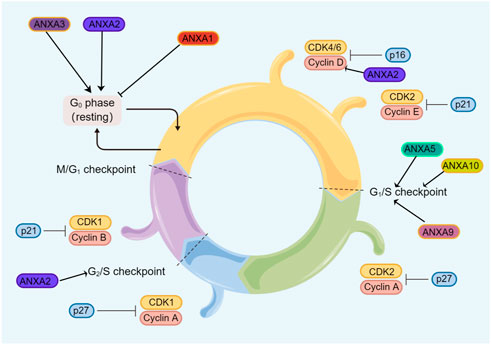
FIGURE 3. Regulation of the ANXA protein family in the cell cycle. Members of the ANXA protein family promotes tumor cell cycle progression.
ANXA2 regulates c-Myc expression by binding to the 5′UTR of its mRNA in a Ca2+-dependent manner at the two pseudoknots of the internal ribosome entry site. Thus, downstream cyclinD1, a cell cycle regulator protein, is affected, which leads to cell proliferation and a high proportion of S-phase cells (Strand et al., 2021; Sun and Zhang, 2021). The downregulation of ANXA2 stalls cells in the G1 phase or S-G2/M phase in gastric cancer (Sun et al., 2013) and glioblastoma multiforme (Maule et al., 2016), respectively. A study showed that the ANXA3 silencing group had a lower proliferation rate in breast cancer and a higher cell ratio in the G0/G1 compared to the control group (Li et al., 2018b). In oncogenic human papillomavirus-positive non-small cell lung cancer, ANXA4 is mediated by HPV16E7 and HPV16E6/E7, promotes cell proliferation and regulates the cell cycle and mitosis (Ciotti et al., 2009). Meanwhile, the combination of epigallocatechin gallate and quercetin enhance ANXA5 to effectively inhibit colorectal cancer arrest at the G1 stage (Xue et al., 2009; Al-Ghamdi et al., 2021). Furthermore, the overexpression of ANXA9 has been found to increase the migration of gastric cancer and form larger and more cell clones, which decrease in the G1 phase and increase in the S and G2 phases (Zhou et al., 2021b). ANXA10 is frequently found to be highly expressed in human oral cancer, and this high expression may promote G1 phase cell cycle progression by activating the ERK/MAPK signalling pathway, which in turn results in the reduced expression of member-dependent kinases of cell cycle proteins (Shimizu et al., 2012). To date, most studies have described ANXA protein family members as promoting the cell cycle in cancer, although little is known about the associated mechanisms. Future investigations regarding the impact of ANXA proteins on the regulation of the cell cycle would certainly inspire more exploration of their biological effects.
3.3 The role of ANXA protein family in invasion and metastasis
Invasion describes the occupation of adjacent normal tissues by cancer cells after leaving the tumour parent body, and metastasis is the spread of tumour cells from the primary site to distant organs (Hart and Fidler, 1980). Increasing evidence has elucidated the critical role of the ANXA protein family in cancer invasion and metastasis (Xu et al., 2015). Proteomics technology has revealed that ANXA1 mainly regulates the processes related to tumour cell skeletal remodelling and immune responses, thus affecting the invasive migration of cancer cells (Tu et al., 2017). Of particular interest, ANXA1 plays dual roles with regard to invasion and metastasis among different tumour cells (Liu et al., 2014; Swa et al., 2015). A study showed that the inhibition of ANXA1 in nasopharyngeal carcinoma increased the trauma-healing ability of the cells, and the activation of the ANXA1 gene decreased the trauma-healing rate by 70%. Conversely, in the mouse mammary tumour cell line 168FARN, the knockdown of ANXA1 inhibited the invasion and metastasis of breast cancer (Tu et al., 2017), with less expression of epithelial–mesenchymal transition (EMT) markers, wave proteins and myosin light-chain kinase.
In addition to ANXA1, the ANXA proteins ANXA2, ANXA9 and ANXA10 are associated with tumour cell adhesion, invasion and metastasis (Xu et al., 2015; Yu et al., 2018; Sun et al., 2019). Once ANXA2 binds to fibrinogen and tissue-type fibrinogen activators, it can activate metalloproteinase (MMPs), which will in turn disrupt the integrity of the extracellular matrix, resulting in tumour invasion and metastasis (Xu et al., 2015). Accordingly, in gastric cancer HGC-27 cells, the inhibition of ANXA2 was found to significantly reduce the migration and secretion of MMPs and inhibit cancer invasion and metastasis (Han et al., 2017). Similarly, Shi et al. (2020) Found that TAGLN2–ANXA2 interaction induces invasion and metastasis in hepatocellular carcinoma. In oesophageal cancer, the overexpression of ANXA2 promotes ESCC cell invasion in vitro and metastasis in vivo through the activation of the MYC-HIF-1α-VEGF cascade (Ma et al., 2018). More meaningfully, some clinical studies have shown the relationship between the expression levels of ANXA2 and the invasion and metastasis of prostate cancer. ANXA2 can therefore be used as a prognostic biomarker for aggressive prostate cancer (Tan et al., 2021). ANXA9 expression levels have been correlated with the depth of invasion and lymphatic metastasis of colorectal cancer (Yu et al., 2018). Patients with colorectal cancer and positive ANXA9 expression were found to have a poorer prognosis, which indicates that ANXA9 could be an independent risk factor for survival. In extrahepatic cholangiocarcinoma, ANXA10 can induce tumour proliferation, EMT facilitation and tumour metastasis (Sun et al., 2019). A large number of preclinical and clinical studies have demonstrated that most ANXAs exhibit obviously invasive and metastatic properties. This may provide an explanation for cancer survival via the broken host immune defence and distant cancer growth. Accordingly, inhibiting ANXA-associated targets may offer new approaches to mitigate cancer metastasis and improve patient survival.
3.4 The role of ANXA protein family in cell apoptosis
Apoptosis is an ordered and coordinated cellular process that occurs under physiological and pathological conditions (Wong, 2011). Evidence suggests that the ANXA protein family has a dual role in tumour cell apoptosis, namely, anti-apoptotic and pro-apoptotic activity (Figure 4) (Huang et al., 2008; Liu et al., 2012; Wei and Zhu, 2021). ANXA2 silencing significantly decreases the mRNA and protein expression of Bcl-1 and promotes apoptosis in osteosarcoma through the AKT signalling pathway and, in lung cancer, by the activity of p53 (Huang et al., 2008; Pan et al., 2018). The upregulation of ANXA4 enhances its interaction with NF-κB p50, activates the NF-κB signalling pathway, promotes cell cycle progression and inhibits apoptosis (Liu et al., 2020). ANXA7 knockdown reduces mRNA, the protein levels of LEPR and the intracellular signalling pathways of the ERK1/2, JAk2/STAT3 and PI3K-related proteins, thereby promoting apoptosis in hepatocellular carcinoma (Huang et al., 2021). While ANXA10 plays a diverse role in different tumours, in hepatocellular carcinoma, the overexpression of ANXA10 significantly promotes apoptosis. Conversely, in papillary thyroid cancer, the knockdown of ANXA10 promotes apoptosis by inhibiting the MAPK/ERK signalling pathway through the downregulation of TSG101 (Liu et al., 2012; Wei and Zhu, 2021). Although studies have shown the functional diversity of ANXA proteins in apoptosis, a deeper understanding of ANXA would make an important contribution to the field of cancer treatment by enhancing apoptosis.
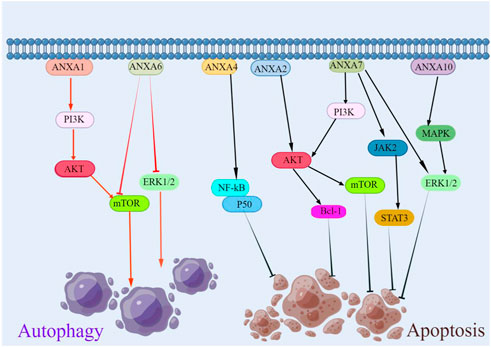
FIGURE 4. Regulation of ANXA protein family in apoptosis and autophagy. ANXA1 promoted cellular autophagy and ANXA6 inhibited cellular autophagy. In terms of apoptosis, except for ANXA10, which had a dual role, the rest of the ANXA protein family inhibited tumor cell apoptosis.
3.5 The role of ANXA protein family in autophagy
Autophagy is a catabolic process involving the lysosomal renewal of proteins and organelles to maintain cellular homeostasis and reduce metabolic stress (Lozy and Karantza, 2012). Autophagy is one of the most important programmed cell death mechanisms, and the ANXA protein family has a considerable impact on autophagy (Figure 4). ANXA regulates the formation of vesicular lipid membranes and promotes cellular cytokinesis (Xi et al., 2020). Respective members of the ANXA protein family have different effects on autophagy in various cancers. ANXA1 inhibits cellular autophagy and promotes tumour invasion and metastasis through PI3K/AKT signalling activation in nasopharyngeal carcinoma (Zhu et al., 2018). In contrast, autophagy induced by ANXA6 in cervical cancer may be related to the inhibition of the PI3K-AKT and ERK signalling pathways of mTOR activation (Sun et al., 2020). Elucidating the molecular mechanism of ANXA in autophagy may assist in identifying new therapeutic targets and developing novel treatment strategies.
3.6 Summary
There is a growing consensus that ANXA proteins play a role in cancer and that the ANXA protein family is involved in tumour cell proliferation, cell cycle regulation, invasive metastasis, apoptosis and autophagy in numerous cancers (Table 1). The consensus thus far is that ANXA proteins in tumour cells may be mediators of tumorigenesis, proliferation and metastasis, that is, ‘angels of life’ for some tumours and ‘demons of death’ for others. This summary therefore provides an exciting opportunity for the treatment of various cancers.
4 The role of ANXA protein family as a biomarker in cancer diagnosis
Previous studies have confirmed that the ANXA protein family is significantly associated with tumour development and can be used as a biomarker for cancer diagnoses. Through an in vitro study of 268 lung cancer patients, Rong et al. (2014) found that the expression of ANXA1 in the cancer tissues and serum of lung cancer patients was significantly higher than normal. Elevated serum ANXA1 was noted to closely correlate with the clinical manifestations in these patients. A large number of studies have also demonstrated that, in addition to lung cancer, ANXA1 expression is upregulated in a variety of cancers, including hepatocellular carcinoma (Zhuang et al., 2019), colorectal cancer (Liang and Li, 2021), pancreatic cancer (Novizio et al., 2021), melanoma (Delorme et al., 2021), and endometrial cancers (Aboulouard et al., 2021). By constructing a risk model, Liang and Li (2021) found that ANXA1 expression levels were associated with the level of immune infiltration in colorectal cancer. ANXA1 could therefore be used as a biomarker for colorectal cancer diagnoses and as an independent prognostic indicator for patients. Further, other ANXA protein family members have shown abnormal expression in many cancers. For example, the high expression of ANXA3 has been closely associated with upper tract urothelial carcinoma (Lu et al., 2014; Liu et al., 2021a), and patients with higher ANXA3 have been found to have a higher rate of postoperative recurrence (Liu et al., 2021a). Additionally, ANXA5 is significantly expressed in non-papillary bladder cancer (Wu et al., 2021), and lung cancer patients with high Cir–ANXA seven expression tend to have a poorer prognosis (Wang, 2021). Meanwhile, ANXA8 expression has been noted as significantly more enhanced in ovarian malignant tissues than benign tumours and normal ovarian tissues and is associated with a poor prognosis (Gou et al., 2019), and ANXA10 has recently been shown to be a prognostic biomarker for papillary thyroid cancer as well as a potential therapeutic target (Liu et al., 2021b). Similarly, ANXA13 expression is upregulated in colorectal cancer and promotes the invasion of cancer (Jiang et al., 2017). This suggests that ANXA has already been used as a diagnostic biomarker in a variety of cancers. More specifically, due to its unique structure, ANXA6 may act as either a tumour suppressor or a tumour promoter depending on the type and stage of cancer. ANXA6 expression has been reported to be upregulated in cancers such as pancreatic cancer (Leca et al., 2016), ovarian cancer (Noreen et al., 2020), female thyroid cancer (Lee et al., 2018), and esophageal adenocarcinoma (Zaidi et al., 2014), but downregulated in hepatocellular carcinoma (Meier et al., 2016), gastric cancer (Wang et al., 2013), breast cancer (Sakwe et al., 2011), cervical cancer (Lomnytska et al., 2010) and triple negative breast cancer (TNBC) (Korolkova et al., 2020). Such studies offer some important insights for the use of ANXA6 as a biomarker for the progression of these cancers (Table 2). In addition, ANXA1 expression is significantly increased in small cell lung cancer patients with bone metastases than without bone metastases (Chen et al., 2021). The elevation of ANXA1 has been detected in colorectal cancer patients with micro-metastases in the anterior lymph nodes than in matched individuals with normal lymph nodes (He et al., 2010). ANXA3 expression was also found to be higher in lung cancer patients with lymph node metastases than those without metastases. Accumulating evidence thus suggests that AXNA may be used as a potential biomarker for cancer metastasis (Liu et al., 2021a).
5 The role of ANXA protein family in cancer treatment
5.1 Role of ANXA protein family in therapy resistance
Therapy resistance is one of the fundamental reasons for cancer progression. It is primarily driven by tumour cells’ intrinsic mechanisms. Previous studies have shown that ANXA promotes therapy resistance to a large number of drugs, which suggests that ANXA plays an important role in treatment resistance. For example, ANXA4 was found to be overexpressed in paclitaxel-resistant lung cancer (Wei et al., 2012; Matsuzaki et al., 2014; Wei et al., 2015; Yao et al., 2016). In normal conditions, paclitaxel induces the translocation of ANXA4 to the cytoplasmic membrane and promotes apoptosis in cancer (Gaudio et al., 2013; Wei et al., 2015; Gaudio et al., 2016). However, ANXA4 can bind to the N-terminal part of the Fhit protein, a tumour suppressor gene, to form an ANXA4–Fhit complex, which specifically prevents cytoplasmic translocation and generates drug resistance (Huebner and Croce, 2001; Gaudio et al., 2013; Wei et al., 2015). Not only is the Fhit protein closely associated with ANXA4 and drug resistance, but also ATP7A. Toosendanin targets ANXA4/ATP7A to reduce the binding of ANXA4 to ATP7A and to mediate the sensitisation of non-small cell lung cancer to cisplatin (Zheng et al., 2018). Additionally, a vitro drug sensitivity assay demonstrated that the overexpression of ANXA3 significantly enhanced the resistance of hepatocellular carcinoma cells to cisplatin (Tong et al., 2015; Liu et al., 2021a), 5-fluorouracil (Pan et al., 2015; Tong et al., 2015; Liu et al., 2021a) and adriamycin (Liu et al., 2021a). A previous study found that the secretion of ANXA6-containing exosomes promoted paclitaxel resistance in breast cancer cells as well as cancer progression in a YAP1-dependent manner (Guo et al., 2021b). At the same time, reduced ANXA6 expression has been shown to sensitise TNBC cells to tyrosinase inhibitors (Korolkova et al., 2020), and Hsa-miR-105-1 expression can be downregulated in cisplatin-resistant ovarian cancer cells by targeting ANXA9 (Kou et al., 2021). The aforementioned information reveals a possible mechanism by which tumour cells become resistant to some anticancer drugs by affecting ANXA proteins.
Besides the traditional drugs mentioned above, some novel targeted drugs have been found to produce resistance by affecting ANXA proteins. Xiong et al. noted that upregulation of the ribonucleotide reductase M2 subunit (RRM2) causes resistance to sunitinib in renal cancer cells because it stabilises ANXA1 and activates the AKT pathway independently of its nucleotide reductase activity (Xiong et al., 2021). Spijkers-Hagelstein et al. found that ANXA2 was associated with glucocorticoid resistance in children with acute lymphoblastic leukaemia (ALL). The downregulation of ANXA2 expression significantly increased the sensitivity of ALL cells to glucocorticoids and prednisolone, and when ANXA2 was overexpressed in ALL cells, Src kinase activity was enhanced, which resulted in glucocorticoid resistance. Meanwhile, the downregulation of ANXA2 blocked this process, leading to increased sensitivity to prednisolone and thus improved therapeutic efficacy. Recently, ANXA2 has also been reported as a drug resistance-associated protein in ovarian cancer (Cruz et al., 2017). Furthermore, the knockdown of ANXA3 has been found to inhibit the resistance of hepatocellular carcinoma cells to sorafenib (Tong et al., 2018). The mechanism involved the overexpression of ANXA3 in sorafenib-resistant hepatocellular carcinoma cells, which inhibited PKCd/p38-associated apoptosis to promote cell survival. ANXA3 may therefore be important in the development of multidrug resistance in hepatocellular carcinoma patients. Although the mechanisms remain unclear, the anti-resistance function of ANXA sheds light on the utilisation of ANXA against drug resistance and potential improvements in treatment efficacy.
5.2 Role of ANXA protein family in modulation of therapy efficiency
As previously discussed, the ANXA protein family plays critical role in the development of cancer, so ANXA proteins have become viable new targets for cancer treatment. Natural compounds are one of the directions that may be used to effect the use of ANXA2 in treatment. For instance, the ginsenosides Rg5 and Rk1 can specifically bind to ANXA2. This interaction can inhibit NF-κB activity, downregulate apoptosis inhibitory proteins, activate caspases and promote apoptosis (Wang et al., 2018). Picrasidine, a plant alkaloid purified from the traditional Chinese medicine bitter ginseng, has been shown to achieve anti-tumour activity by targeting ANXA2 (Wang et al., 2017), and cicer arietinum lectin inhibits the EGFR -mediated signalling pathway by blocking the binding of ANXA2 and galactose lectin-3, with apoptosis as a consequence, and inhibiting cancer proliferation and migration (Shetty et al., 2016). A researcher designed a cationic lipid-directed nanoparticle, which is essentially an ANXA2 shRNA carrier, and found that it can suppress tumour growth by silencing ANXA2 (Andey et al., 2014). It has also been proposed that CBP12, a colorectal cancer binding peptide, can target ANXA2 with specific affinity and thus has the potential to become a novel targeted drug (Li et al., 2021c). In the meantime, Du et al. found that ANXA3 and HIF-1α act together to promote colon carcinogenesis, while HIF-1α siRNA can inhibit the expression of HIF-1α and ANXA3 in transplantation tumour tissues (Du et al., 2020), Liu et al. demonstrated that miR-1253 upregulates the expression of the pro-apoptosis-related proteins Bax and caspase-3 and downregulates the expression of the anti-apoptosis-related protein Bcl2 via the degradation of ANXA3 as its target, thereby promoting the apoptosis of cancer cells (Liu et al., 2021c).
To date, a negligible number of studies addressing this area of research have been published. Future research involving drugs that target ANXA will undoubtedly inspire the development of new strategies that enhance therapy efficiency.
5.3 The inhibitors of the ANXA protein family
Only a few studies are available on ANXA protein family inhibitors. The downregulation of ANXA expression mainly uses gene knockout and gene silencing. However, the identification of ANXA inhibitors would be beneficial as this would enable the targeting of ANXA proteins for cancer treatment. As described previously with respect to their structure, the functioning of ANXA proteins depends strictly on Ca2+, and some ion channel blockers exert an inhibitory effect on ANXA proteins (Kourie and Wood, 2000). In earlier studies, Pollard et al. found that concentrations of Ca2+ greater than 10 nM were able to block the synexin channel of ANXA7. While the non-activating Cd2+ channel inhibitor nifedipine required concentrations greater than 300 mM (Pollard and Rojas, 1988). Burns et al. pointed that the synexin channel of ANXA5 was sensitive to 0.2 nM La2+115,116. These ions and compounds inhibit ANXA function by blocking ion channels. Similarly, it was determined that benzothiazolecipine K201 could bind to ANXA5 and inhibit its Ca2+ channel activity because K201 was able to inhibit the hinge movement of the ANXA5 module in a metastable manner, thereby blocking Ca2+ movement in ANXA5 (Kaneko et al., 1997; Gerke and Moss, 2002). In a recent study, Aareskjold et al. synthesised RatRib120, a nuclease, with the ability to stably integrate with host DNA and enabled it to downregulate ANXA2 expression by targeting the ANXA2 mRNA (Aareskjold et al., 2019). In addition, ANXA8, which is highly expressed in patients with acute promyelocytic leukaemia, was found to be inhibited by all-trans-retinoic acid, an inhibitor that can help patients with symptom relief (Chang et al., 1992).
ANXA proteins characteristically bind to their receptors and relieve specific biological effects, so the inhibitors of ANXA receptors are key regulators of ANXA protein functions. n-t-Boc-Met-Leu-Phe is a classic ANXA1 receptor blocker (Liu et al., 2015; Vago et al., 2016; Bai et al., 2020) that competitively binds to the FRP2 receptor (Bai et al., 2020). In addition, due to the function of the interaction between ANXA and S100A10 in cancer, Reddy et al. screened 29 potential inhibitor compounds and determined that 2-[5-(4,6-dimethyl-pyrimidin-2-ylsulfanylmethyl)-4-furan-2-ylmethy l-4H-[1,2,4]triazol-3-ylsulfanyl]-N-substituted (R)-acetamide analogues and 3,4,5-trisubstituted-1,2,4-triazole analogues were the two inhibitors that attached to the N-terminal of ANXA2 (Reddy et al., 2014). This attachment not only disrupted the interaction between ANXA and S100A10, but also inhibited ANXA2 activity.
A recent study found that phenothiazine derivatives, especially trifluoperazine (TFP), are able to inhibit the repair function of ANXA proteins at the cell membrane. TFP delays the accumulation of ANXA2-GFP at the damaged plasma membrane, inhibits ANXA-mediated membrane curvature, affects the membrane-forming ability of ANXA and, to some extent, impairs ANXA proteins from binding to membrane patches (Table 3) (Heitmann et al., 2021). Not only inhibitors, but also their functions need deeper investigation. It remains an open question whether inhibitors are a potential target for cancer treatment and further, how to transfer this knowledge to clinical application.
6 Conclusion and future directions
Most of the numerous members of the ANXA protein family have been found to be associated with cancer, which is characterised by abnormal changes in the normal cellular pathway through the up- or downregulation of expression. Due to the individual characteristics of tumour cells and the various post-translational patterns of the ANXA protein family, members of the ANXA protein family trigger different tumorigenesis and metastasis, thus leading to variable outcomes. A more in-depth study of the ANXA protein family in the tumour microenvironment, with careful consideration of the timing and approach to target selection, may suggest more biologically meaningful clinical treatment strategies. In the early stages of cancer, high expression of ANXA proteins may promote metastasis. It is therefore important to consider the timing of the use of ANXA proteins as clinical prognostic biomarkers. The expression of ANXA proteins is significantly altered during cancer progression, so they can be used as diagnostic and prognostic biomarkers for certain cancers. Furthermore, targeting ANXA proteins has emerged as a promising new strategy for cancer therapy. ANXA proteins are also closely associated with multidrug resistance in many cancers, including cisplatin, paclitaxel and sorafenib resistance. If this could be a research direction and the sensitivity of cancer cells to drugs could be improved, it would undoubtedly be of significant help in cancer treatment. Currently, most of the drug studies involving ANXA proteins are still in the laboratory stage with only a few clinical applications. How to apply these findings to clinical treatment is thus one of the future challenges in cancer research. Basic research on the ANXA protein family is currently relatively shallow, and many members of this protein family have not been studied in an integrated manner with the aim of enhancing tumour prevention and treatment. Future research should focus on the role of the ANXA protein family in the tumour microenvironment and consider tumour-related macrophages, tumour-related fibroblasts and lymphatic metastasis to achieve the combination of cancer prevention and treatment. Moreover, such research should focus on drug screening, as well as drug resistance and its role in immune suppression mechanisms, and aim to achieve precise treatments.
Author contributions
HZ and TG contributed to the collection of data, preparation of the figures and tables, and writing of the manuscript. GC and GL contributed to data collection and preparation of Figure 1. QS and GL contributed to data collection and preparation of Figures 2, 3. FX, XD, and FY contributed to data collection and preparation of Figure 4 and Table 1. CC and DZ contributed to data collection and preparation of Tables 2, 3. SL, YL, and MW reviewed the manuscript. ZZ has revised the language and grammar of the article. LY and BL contributed to the establishment of the structure of this manuscript and acted as the supervisor. All authors read and approved the final manuscript.
Funding
This work was supported by National Natural Science Foundation of China (No.81803895) and Shandong Province Natural Science Foundation (ZR2021YQ57).
Acknowledgments
Figures 2, 3 and 4 were drawn by Figdraw (www.figdraw.com)
Conflict of interest
The authors declare that the research was conducted in the absence of any commercial or financial relationships that could be construed as a potential conflict of interest.
Publisher’s note
All claims expressed in this article are solely those of the authors and do not necessarily represent those of their affiliated organizations, or those of the publisher, the editors and the reviewers. Any product that may be evaluated in this article, or claim that may be made by its manufacturer, is not guaranteed or endorsed by the publisher.
Abbreviations
ALL, Acute lymphoblastic leukemia; ANXA, Annexin A; ANXs, Annexins; ATP, Adenosine triphosphate; Bcl-2, B-cell lymphoma-2; EGFR, Epidermal growth factor receptor; EMT, Epithelial-mesenchymal transition; ERK, Extracellular signal-regulated kinase; FHIT, Fragile histidine triad; GBM, Glioblastoma multiforme; HIF, Hypoxia Inducible Factor; HepG2, Human hepatocellular carcinomas; HPV, Human papillomavirus; IL-6, Interleukin-6; JAK2, Janus-family tyrosine kinase 2; MAPK, Mitogen-activated protein kinase; MEK, MAPK/ERK kinase; MMPs, Matrix metalloproteinases; MYC, Myelocytomatosis oncogene cellular homolog; PI3K, Phosphoinositide 3 kinase; AKT, AKT8 virus oncogene cellular homolog; RRM2, Ribonucleotide reductase M2 subunit; STAT3, Signal transducer and activator of transcription 3; TAGLN, Transgelin; NF-κB, Nuclear factor κ B; TFP, Trifluoperazine; NBC, Triple negative breast cancer; TSG, Tumor necrosis factor-stimulated gene; VEGF, Vascular endothelial growth factor.
References
Aareskjold, E., Grindheim, A. K., Hollås, H., Goris, M., Lillehaug, J. R., and Vedeler, A. (2019). Two tales of Annexin A2 knock-down: One of compensatory effects by antisense RNA and another of a highly active hairpin ribozyme. Biochem. Pharmacol. 166, 253–263. doi:10.1016/j.bcp.2019.05.028
Aboulouard, S., Wisztorski, M., Duhamel, M., Saudemont, P., Cardon, T., Narducci, F., et al. (2021). In-depth proteomics analysis of sentinel lymph nodes from individuals with endometrial cancer. Cell Rep. Med. 2 (6), 100318. doi:10.1016/j.xcrm.2021.100318
Al-Ghamdi, M. A., Al-Enazy, A., Huwait, E. A., Albukhari, A., Harakeh, S., and Moselhy, S. S. (2021). Enhancement of Annexin V in response to combination of epigallocatechin gallate and quercetin as a potent arrest the cell cycle of colorectal cancer. Braz. J. Biol. = Revista brasleira de Biol. 83, e248746. doi:10.1590/1519-6984.248746
Andey, T., Marepally, S., Patel, A., Jackson, T., Sarkar, S., O'Connell, M., et al. (2014). Cationic lipid guided short-hairpin RNA interference of annexin A2 attenuates tumor growth and metastasis in a mouse lung cancer stem cell model. J. Control. release official J. Control. Release Soc. 184, 67–78. doi:10.1016/j.jconrel.2014.03.049
Bai, F., Zhang, P., Fu, Y., Chen, H., Zhang, M., Huang, Q., et al. (2020). Targeting ANXA1 abrogates Treg-mediated immune suppression in triple-negative breast cancer. J. Immunother. Cancer 8 (1), e000169. doi:10.1136/jitc-2019-000169
Bharadwaj, A., Bydoun, M., Holloway, R., and Waisman, D. (2013). Annexin A2 heterotetramer: Structure and function. Int. J. Mol. Sci. 14 (3), 6259–6305. doi:10.3390/ijms14036259
Burns, A. L., Magendzo, K., Shirvan, A., Rojas, E., Alijani, M. R., et al. (1989). Calcium channel activity of purified human synexin and structure of the human synexin gene. Proc. Natl. Acad. Sci. U. S. A. 86 (10), 3798–3802. doi:10.1073/pnas.86.10.3798
Chang, K. S., Wang, G., Freireich, E. J., Daly, M., Naylor, S. L., Trujillo, J. M., et al. (1992). Specific expression of the annexin VIII gene in acute promyelocytic leukemia. Blood 79 (7), 1802–1810. doi:10.1182/blood.v79.7.1802.bloodjournal7971802
Chen, P., Min, J., Wu, H., Zhang, H., Wang, C., Tan, G., et al. (2021). Annexin A1 is a potential biomarker of bone metastasis in small cell lung cancer. Oncol. Lett. 21 (2), 141. doi:10.3892/ol.2020.12402
Ciotti, M., Marzano, V., Giuliani, L., Nuccetelli, M., D'Aguanno, S., Azzimonti, B., et al. (2009). Proteomic investigation in A549 lung cell line stably infected by HPV16E6/E7 oncogenes. Respir. Int. Rev. Thorac. Dis. 77 (4), 427–439. doi:10.1159/000176209
Croissant, C., Carmeille, R., Brévart, C., and Bouter, A. (2021). Annexins and membrane repair dysfunctions in muscular dystrophies. Int. J. Mol. Sci. 22 (10), 5276. doi:10.3390/ijms22105276
Cruz, I. N., Coley, H. M., Kramer, H. B., Madhuri, T. K., Safuwan, N. A. M., Angelino, A. R., et al. (2017). Proteomics analysis of ovarian cancer cell lines and tissues reveals drug resistance-associated proteins. Cancer Genomics Proteomics 14 (1), 35–51. doi:10.21873/cgp.20017
Delorme, S., Privat, M., Sonnier, N., Rouanet, J., Witkowski, T., Kossai, M., et al. (2021). New insight into the role of ANXA1 in melanoma progression: Involvement of stromal expression in dissemination. Am. J. Cancer Res. 11 (4), 1600–1615.
Donato, R., Cannon, B. R., Sorci, G., Riuzzi, F., Hsu, K., Weber, D. J., et al. (2013). Functions of S100 proteins. Curr. Mol. Med. 13 (1), 24–57. doi:10.2174/156652413804486214
Dong, Z., Yao, M., Zhang, H., Wang, L., Huang, H., Yan, M., et al. (2014). Inhibition of Annexin A2 gene transcription is a promising molecular target for hepatoma cell proliferation and metastasis. Oncol. Lett. 7 (1), 28–34. doi:10.3892/ol.2013.1663
Du, K., Ren, J., Fu, Z., Wu, X., Zheng, J., and Li, X. (2020). ANXA3 is upregulated by hypoxia-inducible factor 1-alpha and promotes colon cancer growth. Transl. Cancer Res. 9 (12), 7440–7449. doi:10.21037/tcr-20-994
Gaudio, E., Paduano, F., Ngankeu, A., Ortuso, F., Lovat, F., Pinton, S., et al. (2016). A Fhit-mimetic peptide suppresses annexin A4-mediated chemoresistance to paclitaxel in lung cancer cells. Oncotarget 7 (21), 29927–29936. doi:10.18632/oncotarget.9179
Gaudio, E., Paduano, F., Spizzo, R., Ngankeu, A., Zanesi, N., Gaspari, M., et al. (2013). Fhit delocalizes annexin a4 from plasma membrane to cytosol and sensitizes lung cancer cells to paclitaxel. PLoS One 8 (11), e78610. doi:10.1371/journal.pone.0078610
Geisow, M. J., Walker, J. H., Boustead, C., and Taylor, W. (1987). Annexins--new family of Ca2+-regulated-phospholipid binding protein. Biosci. Rep. 7 (4), 289–298. doi:10.1007/BF01121450
Gerke, V., and Moss, S. E. (2002). Annexins: From structure to function. Physiol. Rev. 82 (2), 331–371. doi:10.1152/physrev.00030.2001
Gou, R., Zhu, L., Zheng, M., Guo, Q., Hu, Y., Li, X., et al. (2019). Annexin A8 can serve as potential prognostic biomarker and therapeutic target for ovarian cancer: Based on the comprehensive analysis of annexins. J. Transl. Med. 17 (1), 275. doi:10.1186/s12967-019-2023-z
Grewal, T., Koese, M., Rentero, C., and Enrich, C. (2010). Annexin A6-regulator of the EGFR/Ras signalling pathway and cholesterol homeostasis. Int. J. Biochem. Cell Biol. 42 (5), 580–584. doi:10.1016/j.biocel.2009.12.020
Gu, Z. Y., Miao, X. Y., Ma, L. C., Gao, J. J., Gong, Y. P., and Li, C. L. (2021). Maintenance of cellular annexin A1 level is essential for PI3K/AKT/mTOR-mediated proliferation of pancreatic beta cells. J. Biol. Regul. Homeost. Agents 35 (3), 1011–1019. doi:10.23812/20-417-A
Guo, C., Li, N., Dong, C., Wang, L., Li, Z., Liu, Q., et al. (2021). 33-kDa ANXA3 isoform contributes to hepatocarcinogenesis via modulating ERK, PI3K/Akt-HIF and intrinsic apoptosis pathways. J. Adv. Res. 30, 85–102. doi:10.1016/j.jare.2020.11.003
Guo, Z., Guo, A., and Zhou, C. (2021). Breast cancer stem cell-derived ANXA6-containing exosomes sustain paclitaxel resistance and cancer aggressiveness in breast cancer. Front. Cell Dev. Biol. 9, 718721. doi:10.3389/fcell.2021.718721
Han, F., Shrestha, S., Huang, H., Lv, H. Y., Nie, C., Lin, L., et al. (2017). Expression of annexin II in gastric carcinoma and its role in gastric cancer metastasis. World J. Gastroenterol. 23 (38), 7009–7015. doi:10.3748/wjg.v23.i38.7009
Hart, I. R., and Fidler, I. J. (1980). Cancer invasion and metastasis. Q. Rev. Biol. 55 (2), 121–142. doi:10.1086/411730
He, H., Xiao, L., Cheng, S., Yang, Q., Li, J., Hou, Y., et al. (2019). Annexin A2 enhances the progression of colorectal cancer and hepatocarcinoma via cytoskeleton structural rearrangements. Microsc. Microanal. official J. Microsc. Soc. Am. Microbeam Analysis Soc. Microsc. Soc. Can. 25 (4), 950–960. doi:10.1017/S1431927619000679
He, Z. Y., Wen, H., Shi, C. B., and Wang, J. (2010). Up-regulation of hnRNP A1, Ezrin, tubulin β-2C and Annexin A1 in sentinel lymph nodes of colorectal cancer. World J. Gastroenterol. 16 (37), 4670–4676. doi:10.3748/wjg.v16.i37.4670
Heitmann, A. S. B., Zanjani, A. A. H., Klenow, M. B., Mularski, A., Sonder, S. L., Lund, F. W., et al. (2021). Phenothiazines alter plasma membrane properties and sensitize cancer cells to injury by inhibiting annexin-mediated repair. J. Biol. Chem. 297 (2), 101012. doi:10.1016/j.jbc.2021.101012
Hofmann, A., Raguénès-Nicol, C., Favier-Perron, B., HubeR, R., Russo-Marie, F., et al. (2000). The annexin A3-membrane interaction is modulated by an N-terminal tryptophan. Biochemistry 39 (26), 7712–7721. doi:10.1021/bi992359+
Hu, Z., Li, L., Zhu, B., Huang, Y., Wang, X., Lin, X., et al. (2020). Annexin A5 is essential for PKCθ translocation during T-cell activation. J. Biol. Chem. 295 (41), 14214–14221. doi:10.1074/jbc.RA120.015143
Huang, H., Zhang, J., Ling, F., Huang, Y., Yang, M., Zhang, Y., et al. (2021). Leptin Receptor (LEPR) promotes proliferation, migration, and invasion and inhibits apoptosis in hepatocellular carcinoma by regulating ANXA7. Cancer Cell Int. 21 (1), 4. doi:10.1186/s12935-020-01641-w
Huang, Y., Jin, Y., Yan, C. H., Yu, Y., Bai, J., Chen, F., et al. (2008). Involvement of Annexin A2 in p53 induced apoptosis in lung cancer. Mol. Cell Biochem. 309 (1-2), 117–123. doi:10.1007/s11010-007-9649-5
Huebner, K., and Croce, C. M. (2001). FRA3B and other common fragile sites: The weakest links. Nat. Rev. Cancer 1 (3), 214–221. doi:10.1038/35106058
Jiang, G., Wang, P., Wang, W., Li, W., Dai, L., and Chen, K. (2017). Annexin A13 promotes tumor cell invasion in vitro and is associated with metastasis in human colorectal cancer. Oncotarget 8 (13), 21663–21673. doi:10.18632/oncotarget.15523
Ju, Y., Yuan, B., Wu, W., Zhao, J., and Shi, X. (2021). CircRNA ANXA2 promotes lung cancer proliferation and metastasis by upregulating PDPK1 expression. J. Oncol. 2021, 4526609. doi:10.1155/2021/4526609
Kaneko, N., Ago, H., Matsuda, R., Inagaki, E., and Miyano, M. (1997). Crystal structure of annexin V with its ligand K-201 as a calcium channel activity inhibitor. J. Mol. Biol. 274 (1), 16–20. doi:10.1006/jmbi.1997.1375
Korolkova, O. Y., Widatalla, S. E., Williams, S. D., Whalen, D. S., Beasley, H. K., Ochieng, J., et al. (2020). Diverse roles of annexin A6 in triple-negative breast cancer diagnosis, prognosis and EGFR-targeted therapies. Cells 9 (8), 1855. doi:10.3390/cells9081855
Kou, X., Ding, H., Li, L., and Chao, H. (2021). Hsa-miR-105-1 regulates cisplatin-resistance in ovarian carcinoma cells by targeting ANXA9. Anal. Cell. Pathol. Amst. 2021, 6662486. doi:10.1155/2021/6662486
Kourie, J. I., and Wood, H. B. (2000). Biophysical and molecular properties of annexin-formed channels. Prog. biophysics Mol. Biol. 73 (2-4), 91–134. doi:10.1016/s0079-6107(00)00003-1
Leca, J., Martinez, S., Lac, S., Nigri, J., Secq, V., Rubis, M., et al. (2016). Cancer-associated fibroblast-derived annexin A6+ extracellular vesicles support pancreatic cancer aggressiveness. J. Clin. Invest. 126 (11), 4140–4156. doi:10.1172/JCI87734
Lee, H. S., Kang, Y., Tae, K., Bae, G. U., Park, J. Y., Cho, Y. H., et al. (2018). Proteomic biomarkers for bisphenol A-early exposure and women's thyroid cancer. Cancer Res. Treat. 50 (1), 111–117. doi:10.4143/crt.2017.001
Li, J., Zhou, T., Liu, L., Ju, Y. C., Chen, Y. T., Tan, Z. R., et al. (2018). The regulatory role of Annexin 3 in a nude mouse bearing a subcutaneous xenograft of MDA-MB-231 human breast carcinoma. Pathol. Res. Pract. 214 (10), 1719–1725. doi:10.1016/j.prp.2018.09.009
Li, L., Wang, Z., Lu, T., Li, Y., Pan, M., Yu, D., et al. (2021). Expression and functional relevance of ANXA1 in hypopharyngeal carcinoma with lymph node metastasis. Onco Targets Ther. 14, 1387–1399. doi:10.2147/OTT.S292287
Li, N., Xi, Y., Du, H., Zhou, H., and Xu, Z. (2021). Annexin A4 is dispensable for hair cell development and function. Front. Cell Dev. Biol. 9, 680155. doi:10.3389/fcell.2021.680155
Li, X., Ma, W., Wang, X., Ci, Y., and Zhao, Y. (2018). Annexin A5 overexpression might suppress proliferation and metastasis of human uterine cervical carcinoma cells. Cancer Biomark. 23 (1), 23–32. doi:10.3233/CBM-171040
Li, Y. Z., Wang, Y. Y., Huang, L., Zhao, Y. Y., Chen, L. H., and Zhang, C. (2022). Annexin A protein family in atherosclerosis. Clin. Chim. Acta 531, 406–417. doi:10.1016/j.cca.2022.05.009
Li, Z., Yu, L., Hu, B., Chen, L., Jv, M., Wang, L., et al. (2021). Advances in cancer treatment: A new therapeutic target, annexin A2. J. Cancer 12 (12), 3587–3596. doi:10.7150/jca.55173
Liang, Z., and Li, X. (2021). Identification of ANXA1 as a potential prognostic biomarker and correlating with immune infiltrates in colorectal cancer. Autoimmunity 54 (2), 76–87. doi:10.1080/08916934.2021.1887148
Liu, A., Huang, W., Zeng, G., Ma, X., Zhou, X., Wang, Y., et al. (2014). Expression of the Annexin A1 gene is associated with suppression of growth, invasion and metastasis of nasopharyngeal carcinoma. Mol. Med. Rep. 10 (6), 3059–3067. doi:10.3892/mmr.2014.2656
Liu, C., Li, N., Liu, G., and Feng, X. (2021). Annexin A3 and cancer. Oncol. Lett. 22 (6), 834. doi:10.3892/ol.2021.13095
Liu, J., Wang, H., Zheng, M., Deng, L., Zhang, X., and Lin, B. (2020). p53 and ANXA4/NF-κB p50 complexes regulate cell proliferation, apoptosis and tumor progression in ovarian clear cell carcinoma. Int. J. Mol. Med. 46 (6), 2102–2114. doi:10.3892/ijmm.2020.4757
Liu, J. H., Feng, D., Zhang, Y. F., Shang, Y., Wu, Y., Li, X. F., et al. (2015). Chloral hydrate preconditioning protects against ischemic stroke via upregulating annexin A1. CNS Neurosci. Ther. 21 (9), 718–726. doi:10.1111/cns.12435
Liu, Q., Wang, S., Pei, G., Yang, Y., Min, X., Huang, Y., et al. (2021). Impact analysis of miR-1253 on lung cancer progression through targeted regulation of ANXA3. Cancer Manag. Res. 13, 1767–1776. doi:10.2147/CMAR.S251679
Liu, X., Peng, X., Hu, Z., Zhao, Q., He, J., Li, J., et al. (2012). Effects of over-expression of ANXA10 gene on proliferation and apoptosis of hepatocellular carcinoma cell line HepG2. J. Huazhong Univ. Sci. Technol. 32 (5), 669–674. doi:10.1007/s11596-012-1015-5
Liu, X., Yang, M., Guo, Y., and Lu, X. (2021). Annexin A10 is a novel prognostic biomarker of papillary thyroid cancer. Ir. J. Med. Sci. 190 (1), 59–65. doi:10.1007/s11845-020-02263-x
Liu, Y. Y., Ge, C., Tian, H., Jiang, J. Y., Zhao, F. Y., Li, H., et al. (2017). The transcription factor Ikaros inhibits cell proliferation by downregulating ANXA4 expression in hepatocellular carcinoma. Am. J. Cancer Res. 7 (6), 1285–1297.
Liu, Z., Wang, Y., Wang, L., Yao, B., Sun, L., Liu, R., et al. (2019). Long non-coding RNA AGAP2-AS1, functioning as a competitive endogenous RNA, upregulates ANXA11 expression by sponging miR-16-5p and promotes proliferation and metastasis in hepatocellular carcinoma. J. Exp. Clin. Cancer Res. 38 (1), 194. doi:10.1186/s13046-019-1188-x
Lomnytska, M. I., Becker, S., Hellman, K., Hellstrom, A. C., Souchelnytskyi, S., Mints, M., et al. (2010). Diagnostic protein marker patterns in squamous cervical cancer. Proteomics. Clin. Appl. 4 (1), 17–31. doi:10.1002/prca.200900086
Lozy, F., and Karantza, V. (2012). Autophagy and cancer cell metabolism. Seminars Cell and Dev. Biol. 23 (4), 395–401. doi:10.1016/j.semcdb.2012.01.005
Lu, C. M., Lin, J. J., Huang, H. H., Ko, Y. C., Hsu, J. L., Chen, J. C., et al. (2014). A panel of tumor markers, calreticulin, annexin A2, and annexin A3 in upper tract urothelial carcinoma identified by proteomic and immunological analysis. BMC Cancer 14, 363. doi:10.1186/1471-2407-14-363
Ma, S., Lu, C. C., Yang, L. Y., Wang, J. J., Wang, B. S., Cai, H. Q., et al. (2018). ANXA2 promotes esophageal cancer progression by activating MYC-HIF1A-VEGF axis. J. Exp. Clin. Cancer Res. 37 (1), 183. doi:10.1186/s13046-018-0851-y
Manai, M., Doghri, R., Finetti, P., Mrad, K., Bouabsa, R., et al. (2020). Overexpression of annexin A1 is an independent predictor of longer overall survival in epithelial ovarian cancer. Vivo 34 (1), 177–184. doi:10.21873/invivo.11759
Mao, L., Yuan, W., Cai, K., Lai, C., Huang, C., Xu, Y., et al. (2021). EphA2-YES1-ANXA2 pathway promotes gastric cancer progression and metastasis. Oncogene 40 (20), 3610–3623. doi:10.1038/s41388-021-01786-6
Matsuzaki, S., Serada, S., Morimoto, A., Ueda, Y., Yoshino, K., Kimura, T., et al. (2014). Annexin A4 is a promising therapeutic target for the treatment of platinum-resistant cancers. Expert Opin. Ther. Targets 18 (4), 403–414. doi:10.1517/14728222.2014.882323
Maule, F., Bresolin, S., Rampazzo, E., Boso, D., Della Puppa, A., Esposito, G., et al. (2016). Annexin 2A sustains glioblastoma cell dissemination and proliferation. Oncotarget 7 (34), 54632–54649. doi:10.18632/oncotarget.10565
Meier, E. M., Rein-Fischboeck, L., Pohl, R., Wanninger, J., Hoy, A. J., Grewal, T., et al. (2016). Annexin A6 protein is downregulated in human hepatocellular carcinoma. Mol. Cell Biochem. 418 (1-2), 81–90. doi:10.1007/s11010-016-2735-9
Moss, S. E., and Morgan, R. O. (2004). The annexins. Genome Biol. 5 (4), 219. doi:10.1186/gb-2004-5-4-219
Noreen, S., Gardner, Q. A., Fatima, I., Sadaf, S., and Akhtar, M. W. (2020). Upregulated expression of calcium-dependent annexin A6: A potential biomarker of ovarian carcinoma. Proteomics. Clin. Appl. 14 (2), e1900078. doi:10.1002/prca.201900078
Novizio, N., Belvedere, R., Pessolano, E., et al. (2020). ANXA1 contained in EVs regulates macrophage polarization in tumor microenvironment and promotes pancreatic cancer progression and metastasis. Int. J. Mol. Sci. 2021 (20), 22. doi:10.3390/ijms222011018
Pan, B. L., Tong, Z. W., Wu, L., Pan, L., Li, J. E., Huang, Y. G., et al. (2018). Effects of MicroRNA-206 on osteosarcoma cell proliferation, apoptosis, migration and invasion by targeting ANXA2 through the AKT signaling pathway. Cell Physiol. Biochem. 45 (4), 1410–1422. doi:10.1159/000487567
Pan, Q. Z., Pan, K., Weng, D. S., Zhao, J. J., Zhang, X. F., Wang, D. D., et al. (2015). Annexin A3 promotes tumorigenesis and resistance to chemotherapy in hepatocellular carcinoma. Mol. Carcinog. 54 (8), 598–607. doi:10.1002/mc.22126
Park, J. E., Lee, D. H., Lee, J. A., Park, S. G., Kim, N. S., Park, B. C., et al. (2005). Annexin A3 is a potential angiogenic mediator. Biochem. Biophys. Res. Commun. 337 (4), 1283–1287. doi:10.1016/j.bbrc.2005.10.004
Pollard, H. B., and Rojas, E. (1988). Ca2+-activated synexin forms highly selective, voltage-gated Ca2+ channels in phosphatidylserine bilayer membranes. Proc. Natl. Acad. Sci. U. S. A. 85 (9), 2974–2978. doi:10.1073/pnas.85.9.2974
Reddy, T. R., Li, C., Guo, X., Fischer, P. M., and Dekker, L. V. (2014). Design, synthesis and SAR exploration of tri-substituted 1,2,4-triazoles as inhibitors of the annexin A2-S100A10 protein interaction. Bioorg Med. Chem. 22 (19), 5378–5391. doi:10.1016/j.bmc.2014.07.043
Rintala-Dempsey, A. C., Rezvanpour, A., and Shaw, G. S. (2008). S100-annexin complexes-structural insights. Febs J. 275 (20), 4956–4966. doi:10.1111/j.1742-4658.2008.06654.x
Rojas, E., Pollard, H. B., Haigler, H. T., Parra, C., and Burns, A. L. (1990). Calcium-activated endonexin II forms calcium channels across acidic phospholipid bilayer membranes. J. Biol. Chem. 265 (34), 21207–21215. doi:10.1016/s0021-9258(17)45347-6
Rong, B., Zhao, C., Liu, H., Ming, Z., Cai, X., Gao, W., et al. (2014). Elevated serum annexin A1 as potential diagnostic marker for lung cancer: A retrospective case-control study. Am. J. Transl. Res. 6 (5), 558–569.
Rosengarth, A., Gerke, V., and Luecke, H. (2001). X-ray structure of full-length annexin 1 and implications for membrane aggregation. J. Mol. Biol. 306 (3), 489–498. doi:10.1006/jmbi.2000.4423
Sabran, A., Kumolosasi, E., and Jantan, I. (2019). Effects of annexin A1 on apoptosis and cell cycle arrest in human leukemic cell lines. Acta Pharm. Zagreb. Croat. 69 (1), 75–86. doi:10.2478/acph-2019-0005
Sakwe, A. M., Koumangoye, R., Guillory, B., and Ochieng, J. (2011). Annexin A6 contributes to the invasiveness of breast carcinoma cells by influencing the organization and localization of functional focal adhesions. Exp. Cell Res. 317 (6), 823–837. doi:10.1016/j.yexcr.2010.12.008
Schafer, K. A. (1998). The cell cycle: A review. Veterinary pathol. 35 (6), 461–478. doi:10.1177/030098589803500601
Sharma, M., Blackman, M. R., and Sharma, M. C. (2012). Antibody-directed neutralization of annexin II (ANX II) inhibits neoangiogenesis and human breast tumor growth in a xenograft model. Exp. Mol. Pathol. 92 (1), 175–184. doi:10.1016/j.yexmp.2011.10.003
Shetty, P., Bargale, A., Patil, B. R., Mohan, R., Dinesh, U. S., Vishwanatha, J. K., et al. (2016). Cell surface interaction of annexin A2 and galectin-3 modulates epidermal growth factor receptor signaling in Her-2 negative breast cancer cells. Mol. Cell Biochem. 411 (1-2), 221–233. doi:10.1007/s11010-015-2584-y
Shi, J., Ren, M., She, X., Zhang, Z., Zhao, Y., Han, Y., et al. (2020). Transgelin-2 contributes to proliferation and progression of hepatocellular carcinoma via regulating Annexin A2. Biochem. Biophys. Res. Commun. 523 (3), 632–638. doi:10.1016/j.bbrc.2020.01.028
Shimizu, T., Kasamatsu, A., Yamamoto, A., Koike, K., Ishige, S., Takatori, H., et al. (2012). Annexin A10 in human oral cancer: Biomarker for tumoral growth via G1/S transition by targeting MAPK signaling pathways. PLoS One 7 (9), e45510. doi:10.1371/journal.pone.0045510
Strand, E., Hollås, H., Sakya, S. A., Romanyuk, S., Saraste, M. E. V., Grindheim, A. K., et al. (2021). Annexin A2 binds the internal ribosomal entry site of c-myc mRNA and regulates its translation. RNA Biol. 18 (1), 337–354. doi:10.1080/15476286.2021.1947648
Sun, M. Y., Xing, R. H., Gao, X. J., Yu, X., He, H. M., Gao, N., et al. (2013). ANXA2 regulates the behavior of SGC-7901 cells. Asian Pac J. Cancer Prev. 14 (10), 6007–6012. doi:10.7314/apjcp.2013.14.10.6007
Sun, R., Liu, Z., Qiu, B., Chen, T., Li, Z., Zhang, X., et al. (2019). Annexin10 promotes extrahepatic cholangiocarcinoma metastasis by facilitating EMT via PLA2G4A/PGE2/STAT3 pathway. EBioMedicine 47, 142–155. doi:10.1016/j.ebiom.2019.08.062
Sun, T., and Zhang, J. (2021). ETV4 mediates the Wnt/β-catenin pathway through transcriptional activation of ANXA2 to promote Hepatitis B virus-associated liver hepatocellular carcinoma progression. J. Biochem. 170 (5), 663–673. doi:10.1093/jb/mvab088
Sun, X., Liu, S., Wang, J., Wei, B., Guo, C., Chen, C., et al. (2018). Annexin A5 regulates hepatocarcinoma malignancy via CRKI/II-DOCK180-RAC1 integrin and MEK-ERK pathways. Cell Death Dis. 9 (6), 637. doi:10.1038/s41419-018-0685-8
Sun, X., Shu, Y., Xu, M., Jiang, J., Wang, L., Wang, J., et al. (2020). ANXA6 suppresses the tumorigenesis of cervical cancer through autophagy induction. Clin. Transl. Med. 10 (6), e208. doi:10.1002/ctm2.208
Swa, H. L., Shaik, A. A., Lim, L. H., and Gunaratne, J. (2015). Mass spectrometry based quantitative proteomics and integrative network analysis accentuates modulating roles of annexin-1 in mammary tumorigenesis. Proteomics 15 (2-3), 408–418. doi:10.1002/pmic.201400175
Tan, S. H., Young, D., Chen, Y., Kuo, H. C., Srinivasan, A., Dobi, A., et al. (2021). Prognostic features of Annexin A2 expression in prostate cancer. Pathology 53 (2), 205–213. doi:10.1016/j.pathol.2020.07.006
Tong, M., Che, N., Zhou, L., Luk, S. T., Kau, P. W., Chai, S., et al. (2018). Efficacy of annexin A3 blockade in sensitizing hepatocellular carcinoma to sorafenib and regorafenib. J. Hepatol. 69 (4), 826–839. doi:10.1016/j.jhep.2018.05.034
Tong, M., Fung, T. M., Luk, S. T., Ng, K. Y., Lee, T. K., Lin, C. H., et al. (2015). ANXA3/JNK signaling promotes self-renewal and tumor growth, and its blockade provides a therapeutic target for hepatocellular carcinoma. Stem Cell Rep. 5 (1), 45–59. doi:10.1016/j.stemcr.2015.05.013
Tsai, W. H., Lai, S. L., Li, I. T., Chien, H. Y., Shih, C. H., Kou, Y. R., et al. (2013). Annexin A1 mediates the anti-adhesive effects of the dexamethasone-treated promyelocytic leukemic cells. J. Cell Biochem. 114 (3), 551–557. doi:10.1002/jcb.24394
Tu, Y., Johnstone, C. N., and Stewart, A. G. (2017). Annexin A1 influences in breast cancer: Controversies on contributions to tumour, host and immunoediting processes. Pharmacol. Res. 119, 278–288. doi:10.1016/j.phrs.2017.02.011
Vago, J. P., Tavares, L. P., Sugimoto, M. A., Lima, G. L. N., Galvao, I., de Caux, T. R., et al. (2016). Proresolving actions of synthetic and natural protease inhibitors are mediated by annexin A1. J. Immunol. 196 (4), 1922–1932. doi:10.4049/jimmunol.1500886
Wang, D., Cao, Y., Zheng, L., Lv, D., Chen, L., Xing, X., et al. (2017). Identification of Annexin A2 as a target protein for plant alkaloid matrine. Chem. Commun. Camb. Engl. 53 (36), 5020–5023. doi:10.1039/c7cc02227a
Wang, T., Zhu, L., Liu, H., Yu, G., and Guo, Y. (2013). Picroside II protects SH-SY5Y cells from autophagy and apoptosis following oxygen glucose deprivation/reoxygen injury by inhibiting JNK signal pathway. Anat. Rec. 302 (12), 2245, doi:10.1002/ar.24214
Wang, X., Zhang, S., Zhang, J., Lam, E., Liu, X., Sun, J., et al. (2013). Annexin A6 is down-regulated through promoter methylation in gastric cancer. Am. J. Transl. Res. 5 (5), 555–562.
Wang, Y. (2021). circ-ANXA7 facilitates lung adenocarcinoma progression via miR-331/LAD1 axis. Cancer Cell Int. 21 (1), 85. doi:10.1186/s12935-021-01791-5
Wang, Y. S., Li, H., Li, Y., Zhu, H., and Jin, Y. H. (2018). Identification of natural compounds targeting Annexin A2 with an anti-cancer effect. Protein and Cell 9 (6), 568–579. doi:10.1007/s13238-018-0513-z
Wei, B., Guo, C., Liu, S., and Sun, M. Z. (2015). Annexin A4 and cancer. Clin. Chim. Acta 447, 72–78. doi:10.1016/j.cca.2015.05.016
Wei, R., Zhang, Y., Shen, L., Jiang, W., Li, C., Zhong, M., et al. (2012). Comparative proteomic and radiobiological analyses in human lung adenocarcinoma cells. Mol. Cell Biochem. 359 (1-2), 151–159. doi:10.1007/s11010-011-1008-x
Wei, T., and Zhu, X. (2021). Knockdown of ANXA10 inhibits proliferation and promotes apoptosis of papillary thyroid carcinoma cells by down-regulating TSG101 thereby inactivating the MAPK/ERK signaling pathway. J. bioenergetics Biomembr. 53 (4), 429–440. doi:10.1007/s10863-021-09902-7
Wong, R. S. (2011). Apoptosis in cancer: From pathogenesis to treatment. J. Exp. Clin. Cancer Res. 30 (1), 87. doi:10.1186/1756-9966-30-87
Wu, W., Jia, G., Chen, L., Liu, H., and Xia, S. (2021). Analysis of the expression and prognostic value of annexin family proteins in bladder cancer. Front. Genet. 12, 731625. doi:10.3389/fgene.2021.731625
Xi, Y., Ju, R., and Wang, Y. (2020). Roles of Annexin A protein family in autophagy regulation and therapy. Biomed. Pharmacother. 130, 110591. doi:10.1016/j.biopha.2020.110591
Xiao, Y., Ouyang, C., Huang, W., Tang, Y., Fu, W., and Cheng, A. (2017). Annexin A1 can inhibit the in vitro invasive ability of nasopharyngeal carcinoma cells possibly through Annexin A1/S100A9/Vimentin interaction. PLoS One 12 (3), e0174383. doi:10.1371/journal.pone.0174383
Xiong, W., Zhang, B., Zhu, L., Yi, L., and Jin, X. (2021). RRM2 regulates sensitivity to sunitinib and PD-1 blockade in renal cancer by stabilizing ANXA1 and activating the AKT pathway. Adv. Sci. (Weinh) 8 (18), e2100881. doi:10.1002/advs.202100881
Xu, X. H., Pan, W., Kang, L. H., Feng, H., and Song, Y. Q. (2015). Association of annexin A2 with cancer development (Review). Oncol. Rep. 33 (5), 2121–2128. doi:10.3892/or.2015.3837
Xue, G., Hao, L. Q., Ding, F. X., Mei, Q., Huang, J. J., Fu, C. G., et al. (2009). Expression of annexin a5 is associated with higher tumor stage and poor prognosis in colorectal adenocarcinomas. J. Clin. Gastroenterol. 43 (9), 831–837. doi:10.1097/MCG.0b013e31819cc731
Yao, H., Sun, C., Hu, Z., and Wang, W. (2016). The role of annexin A4 in cancer. Front. Biosci. (Landmark Ed. 21 (5), 949–957. doi:10.2741/4432
Yu, S., Bian, H., Gao, X., and Gui, L. (2018). Annexin A9 promotes invasion and metastasis of colorectal cancer and predicts poor prognosis. Int. J. Mol. Med. 41 (4), 2185–2192. doi:10.3892/ijmm.2018.3432
Zaidi, A. H., Gopalakrishnan, V., Kasi, P. M., Zeng, X., Malhotra, U., Balasubramanian, J., et al. (2014). Evaluation of a 4-protein serum biomarker panel-biglycan, annexin-A6, myeloperoxidase, and protein S100-A9 (B-AMP)-for the detection of esophageal adenocarcinoma. Cancer 120 (24), 3902–3913. doi:10.1002/cncr.28963
Zhang, H. J., Yao, D. F., Yao, M., Huang, H., Wang, L., Yan, M. J., et al. (2013). Annexin A2 silencing inhibits invasion, migration, and tumorigenic potential of hepatoma cells. World J. Gastroenterol. 19 (24), 3792–3801. doi:10.3748/wjg.v19.i24.3792
Zhao, X., Ma, W., Li, X., Li, J., et al. (2021). ANXA1 enhances tumor proliferation and migration by regulating epithelial-mesenchymal transition and IL-6/JAK2/STAT3 pathway in papillary thyroid carcinoma. J. Cancer 12 (5), 1295–1306. doi:10.7150/jca.52171
Zheng, M. D., Wang, N. D., Li, X. L., Yan, J., Tang, J. H., Zhao, X. H., et al. (2018). Toosendanin mediates cisplatin sensitization through targeting Annexin A4/ATP7A in non-small cell lung cancer cells. J. Nat. Med. 72 (3), 724–733. doi:10.1007/s11418-018-1211-0
Zhou, G. Z., Sun, Y. H., Shi, Y. Y., Zhang, Q., Zhang, L., Cui, L. Q., et al. (2021). ANXA8 regulates proliferation of human non-small lung cancer cells A549 via EGFR-AKT-mTOR signaling pathway. Mol. Biol. 55 (5), 870–880. doi:10.31857/S0026898421050116
Zhou, Y., Qiu, C., Wang, T., Tao, L., Zhang, Z., and Yao, J. (2021). High expression of annexin A9 promotes cell proliferation and migration in gastric cancer via the TGF-β signaling pathway. J. Environ. pathology, Toxicol. Oncol. official organ Int. Soc. Environ. Toxicol. Cancer 40 (3), 87–94. doi:10.1615/JEnvironPatholToxicolOncol.2021038527
Zhu, J. F., Huang, W., Yi, H. M., Xiao, T., Li, J. Y., Feng, J., et al. (2018). Annexin A1-suppressed autophagy promotes nasopharyngeal carcinoma cell invasion and metastasis by PI3K/AKT signaling activation. Cell Death Dis. 9 (12), 1154. doi:10.1038/s41419-018-1204-7
Keywords: annexin a protein family, structure, cancer progression, biomarker, treatment, inhibitor
Citation: Zhang H, Zhang Z, Guo T, Chen G, Liu G, Song Q, Li G, Xu F, Dong X, Yang F, Cao C, Zhong D, Li S, Li Y, Wang M, Li B and Yang L (2023) Annexin A protein family: Focusing on the occurrence, progression and treatment of cancer. Front. Cell Dev. Biol. 11:1141331. doi: 10.3389/fcell.2023.1141331
Received: 10 January 2023; Accepted: 23 February 2023;
Published: 03 March 2023.
Edited by:
Masahide Takahashi, Fujita Health University, JapanReviewed by:
Liang Weng, Xiangya Hospital, Central South University, ChinaMotoshi Suzuki, Fujita Health University, Japan
Copyright © 2023 Zhang, Zhang, Guo, Chen, Liu, Song, Li, Xu, Dong, Yang, Cao, Zhong, Li, Li, Wang, Li and Yang. This is an open-access article distributed under the terms of the Creative Commons Attribution License (CC BY). The use, distribution or reproduction in other forums is permitted, provided the original author(s) and the copyright owner(s) are credited and that the original publication in this journal is cited, in accordance with accepted academic practice. No use, distribution or reproduction is permitted which does not comply with these terms.
*Correspondence: Lina Yang, lina_young@163.com; Bing Li, libing_516@qdu.edu.cn
†These authors have contributed equally to this work