- 1CIBER Enfermedades Cardiovasculares (CIBERCV), Madrid, Spain
- 2Molecular and Genetic Cardiovascular Pathophysiology Laboratory, Novel Mechanisms of Atherosclerosis Program, Centro Nacional de Investigaciones Cardiovasculares (CNIC), Madrid, Spain
Population aging and age-related cardiovascular disease (CVD) are becoming increasingly prevalent worldwide, generating a huge medical and socioeconomic burden. The complex regulation of aging and CVD and the interaction between these processes are crucially dependent on cellular stress responses. Interferon-stimulated gene-15 (ISG15) encodes a ubiquitin-like protein expressed in many vertebrate cell types that can be found both free and conjugated to lysine residues of target proteins via a post-translational process termed ISGylation. Deconjugation of ISG15 (deISGylation) is catalyzed by the ubiquitin-specific peptidase 18 (USP18). The ISG15 pathway has mostly been studied in the context of viral and bacterial infections and in cancer. This minireview summarizes current knowledge on the role of ISG15 in age-related telomere shortening, genomic instability, and DNA damage accumulation, as well as in hypertension, diabetes, and obesity, major CVD risk factors prevalent in the elderly population.
1 Introduction
Interferon-stimulated gene-15 (ISG15) encodes a ubiquitin-like protein expressed in many vertebrate cell types, including monocytes, lymphocytes, neutrophils, dendritic cells, natural killer (NK) cells, epithelial-derived cell lines, fibroblasts, vascular smooth muscle cells, endothelial cells, cardiomyocytes, and some tumor cells (Knight and Cordova, 1991; Zhang and Zhang, 2011; Colonne et al., 2011; Bogunovic et al., 2012; Rahnefeld et al., 2014; Tecalco Cruz and Mejia-Barreto, 2017; Albert et al., 2018; Gonzalez-Amor et al., 2022). Initially produced as a 17 kDa precursor, mature ISG15 is a 15 kDa protein with two ubiquitin-like domains: an N-terminal regulatory domain and a C-terminal conjugating domain (Chang et al., 2008) (Figure 1). ISG15 can be found as a free intracellular or extracellular protein or conjugated to target proteins through a reversible post-translational modification called ISGylation. Similar to ubiquitination, ISGylation of de novo synthesized proteins requires ATP-dependent ISG15 conjugation to lysine residues mediated by an enzymatic process involving E1 activating enzyme (UBE1L), E2 conjugating enzyme (UBE2L6), and E3 ligases (HERC5, EFP, HHARI) (Zhang and Zhang, 2011; Durfee and Huibregtse, 2012; Albert et al., 2018). Deconjugation of ISG15 (deISGylation) is catalyzed by the ubiquitin specific peptidase 18 (USP18) (Honke et al., 2016) (Figure 1). ISG15 and the enzymes involved in ISGylation are induced by several interferons (IFNs), mainly type I (IFNα and β) but also type II (IFNγ) and type III (IFNλ), as well as in response to tumor necrosis factor α (TNFα), lipopolysaccharides, and several infectious pathogens (Levy et al., 1990; Jeon et al., 2010; Zhang and Zhang, 2011; Chairatvit et al., 2012; MacParland et al., 2016; Albert et al., 2018; Lertsooksawat et al., 2019) (Figure 1). In addition, a feedback loop operates in humans where ISG15 stabilizes USP18, so that intracellular ISG15 deficiency provokes USP18 downregulation (Basters et al., 2018). Remarkably, USP18 can counteract type I IFN-induced responses by a mechanism independent of deISGylation which is mediated by the interaction of USP18 with type I IFN receptor, which in turn inhibits JAK-STAT-dependent signaling (Basters et al., 2018).
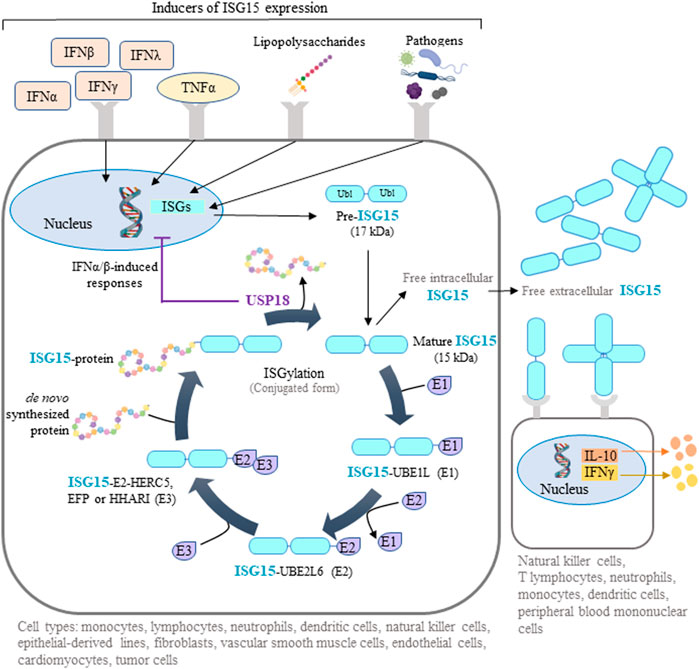
FIGURE 1. ISG15-mediated protein ISGylation. ISG15 is produced as a 17-kDa precursor with two ubiquitin-like domains in response to diverse stimuli, including interferons (IFNs), tumor necrosis factor α (TNFα), lipopolysaccharides, and several pathogens. Intracellular ISG15 exists either as a free protein or conjugated to de novo synthesized proteins through ISGylation. This post-translational process can be reversed by the action of ubiquitin specific peptidase 18 (USP18), a protease that also regulates IFN-mediated signaling. Free intracellular ISG15 can be secreted to act as a cytokine, causing the release of IFNγ and interleukin 10 (IL-10). Extracellular ISG15 can form dimers and multimers that modulate cytokine levels. Some illustrations were created using BioRender.com.
Intracellular ISG15 and ISGylation have been extensively studied in the context of viral and bacterial infections. In this context, they generally play a protective role, either directly, by counteracting pathogen activities such as cell entry, nucleic acid trafficking, viral replication and integration, and cell release, or indirectly, by regulating immune-cell IFN production (Kunzi and Pitha, 1996; Yuan and Krug, 2001; Lenschow et al., 2007; Guerra et al., 2008; Hsiang et al., 2009; Villarroya-Beltri et al., 2017). Surprisingly, humans with ISG15 deficiency do not show increased susceptibility to viral infection, possibly due to concomitant reduction of USP18 expression and USP18-dependent negative regulation of IFN-dependent signaling (Speer et al., 2016). Higher expression of ISG15 was found in female than in male HIV-1-infected patients (Chang et al., 2013), however we are unaware of any work reporting gender-dependent differences in ISG15 expression in basal conditions.
Extracellular ISG15 binds to surface receptors such as leukocyte-function associated antigen-1 on various cell types (neutrophils, monocytes, T lymphocytes, NK cells, dendritic cells, and peripheral blood mononuclear cells (PBMCs)), where it triggers the release of interleukins, including IFNγ (Dos Santos and Mansur, 2017; Ostvik et al., 2020) (Figure 1). Interestingly, individuals deficient for secreted, extracellular ISG15 are more susceptible to mycobacterial infection as a result of defective immune responses caused by reduced immune-cell IFN production (Bogunovic et al., 2012).
ISGylation also regulates key cellular processes involved in stress responses, such as proteasomal degradation of misfolded proteins, modulation of autophagy-dependent and lysosome-dependent degradation, downregulation of protein translation, inhibition of exosome secretion, attenuation of hypoxia, and modulation of cytoskeletal dynamics (Villarroya-Beltri et al., 2017; Dzimianski et al., 2019; Sandy et al., 2020) Due to these broad functions, it is unsurprising that ISG15 plays an important role in age-related diseases, including cancer (Zhang and Zhang, 2011), hypertension (Gonzalez-Amor et al., 2022), diabetes (Sun et al., 2019), and obesity (Wei et al., 2021; Yan et al., 2021) (see below).
Aging is a universal, inevitable, and multifactorial degenerative process associated with disability and increased risk of death due to major chronic diseases, including cardiovascular disease (CVD), the leading cause of morbimortality worldwide (Yazdanyar and Newman, 2009; Hamczyk et al., 2018; Galkin et al., 2019). Population aging and CVD prevalence have increased rapidly over the past decades in developed countries and in much of the developing world, creating an enormous medical and socioeconomic burden worldwide. The pace of biological aging (also called functional aging, denoting the decline over time in tissue and organismal function) is influenced by genetic and environmental factors (Hamczyk et al., 2020). Age-related deterioration has been proposed to result from genetic and molecular processes called “hallmarks of aging”, which include genomic instability, telomere attrition, epigenetic alterations, impaired proteostasis, dysregulated nutrient sensing, mitochondrial dysfunction, cell senescence, stem cell exhaustion, and altered intercellular communication (Lopez-Otin et al., 2013). This review discusses studies that link ISG15 to age-related telomere shortening, genomic instability, DNA damage accumulation, and three key CVD risk factors hypertension, diabetes, and obesity.
2 ISG15 and age-associated telomere attrition
Preservation of genome stability and integrity in eukaryotes requires functional telomeres. These specialized structures located at both chromosome ends contain multiple non-coding double-stranded repeats of a G-rich DNA sequence that ends in a short single-stranded sequence (Hoffmann et al., 2021). Cells in culture can divide until they reach the Hayflick limit, when they enter in a non-replicative state called senescence/mortality stage 1 (normal cells) or crisis/mortality stage 2 (cells expressing oncogenes) (Hayflick and Moorhead, 1961; Hayflick, 1965; Wei and Sedivy, 1999). Cellular aging and related growth arrest are associated with telomere attrition, chromosomal end-to-end fusions, and apoptosis and senescence when telomere length drops below a critical threshold; however, the order of events and whether telomere ablation is cause or consequence of the age-related alterations remains controversial (Hoffmann et al., 2021).
Telomere attrition can regulate gene expression long before the induction of cell-cycle arrest and DNA damage signaling by critically short telomeres (Robin et al., 2014). Genes in close proximity to telomeres are frequently silenced, a phenomenon known as the “telomere position effect”. In addition, loops formed by long telomeres can interact with and silence genes located as far as 10 Mb from the telomere ends, known as the “telomere position effect over long distances”. Upon telomere attrition, these loops become smaller and cover shorter chromosomal distances. Consequently, genes more distant from telomere ends become released from telomere-loop inhibition and can be expressed (Robin et al., 2014). ISG15 is located 1 Mb from the end of human chromosome 1p and was the first human gene reported to progressively increase its transcription with the loss of telomere looping upon telomere attrition (Lou et al., 2009; Zhang et al., 2021). This analysis showed that the number of interactions between the ISG15 locus and the chromosome 1p telomere structure is much higher in young cells than in old ones (Zhang et al., 2021). Free ISG15 expression and ISGylation both increase with telomere shortening in human cells independently of the expression of type I IFNs and of the transcription factor p53 (Lou et al., 2009). Moreover, independently of the telomere position effect, short and/or dysfunctional telomeres can lead to different cellular fates by activating the cyclic GMP-AMP synthase (cGAS)-stimulator of interferon genes (STING) pathway, which engages the autophagy machinery and leads to ISG15 expression and other IFN-stimulated genes (Nassour et al., 2019; Nassour et al., 2023). In patients with COVID-19, the cGAS-STING pathway is also a critical driver of pathological type I IFN responses, which are associated with upregulation of ISG15 and several pro-inflammatory cytokines in skin biopsies (Domizio et al., 2022).
This regulation of ISG15 expression may therefore promote tumor suppression before additional mechanisms of DNA repair are triggered, and impaired ISG15 upregulation due to telomere ablation could contribute to the initiation and progression of age-associated diseases (Lou et al., 2009; Robin et al., 2014).
3 ISG15 in the age-associated DNA damage response (DDR): p53 and PTEN
During both physiological and premature aging, the accumulation of senescent cells and the onset of age-related disorders are driven by genomic instability provoked by the cumulative action of endogenous and environmental factors and a defective DDR (Liu et al., 2005; Varela et al., 2005; Schumacher et al., 2008; Hoeijmakers, 2009; Cheedipudi et al., 2019; Chen et al., 2019; Wei and Ji, 2018; Di Micco et al., 2021; Schumacher et al., 2021; von Zglinicki et al., 2021). The role of ISG15 and ISGylation in the DDR has mostly been studied in the context of cancer. The ISG15/ISGylation system can be oncogenic or have tumor suppressor activity depending on the tissue affected, cancer stage, and specific cancer-related alterations in signalling pathways (Han et al., 2018; Kang et al., 2022). This complexity might be related to the cross-regulation between ISG15 and key tumor suppressors. One of the few identified and validated ISG15 substrates is the tumor suppressor p53, a pivotal transcription factor that coordinates the expression of many DDR effector genes that induce cell-cycle arrest, DNA repair, autophagy, senescence, and apoptosis (Sandy et al., 2020; Luo et al., 2022). ISG15 and p53 modulate each other at several levels. ISG15 and the ISGylation enzymes UBE1L (E1), UBE2L6 (E2), and EFP (E3) each have a p53-responsive element in their promoters, so their expression increases upon p53 activation (Park et al., 2016). Moreover, DNA damage induces the ISGylation of p53, increasing the transcription of target genes such as CDK1, BAX, and MDM2, as well as that of ISG15 and ISGylation factors (Park et al., 2016). ISGylation increases p53 activity and reduces the inhibitory activity of ΔNp63α (an alternative splice variant of the p53 family protein p63 that suppresses the trans-activity of other p53 family members), thus promoting cell growth arrest and tumor suppression (Jeon et al., 2012; Park et al., 2016). However, misfolded p53 can undergo proteasomal degradation upon ISGylation mediated by the ISG15 E3 ubiquitin ligase HERC5, and ISG15 deletion in normal cells causes accumulation of misfolded p53 and inhibits p53 activity (Huang et al., 2014). ISG15 and ISGylation therefore have potential as therapeutic targets for the fine-tuning of the p53-dependent DDR.
Another ISG15 substrate in the DDR pathway is the tumor suppressor phosphatase and tensin homolog (PTEN), which is lost in many cancer types (Lee et al., 2018; Alvarez-Garcia et al., 2019) and also plays important roles in diabetes (Li et al., 2017) and autism (Cummings et al., 2022). ISGylation reduces the cytoplasmic content of PTEN, while USP18-mediated deISGylation promotes PTEN protein stability and recovery of its cytoplasmic expression (Mustachio et al., 2017).
In summary, further studies are warranted to determine the role of ISG15/ISGylation in different cancer types, since its effect might be tumor suppressive or oncogenic depending on the context. Moreover, it is important to address the possible role of ISG15 pathway in the premature aging side effects triggered by anti-cancer therapies due to activation of DNA damage responses in healthy tissues.
4 ISG15 in age-related cardiovascular and cardiometabolic diseases
Most evidence for a role of ISG15 in CVD is related to viral infection. ISG15 activation is associated with coxsackievirus B3-induced myocarditis in mice (Maier et al., 2012), and cardiomyocyte ISG15 expression contributes significantly to the suppression of viral replication (Rahnefeld et al., 2014). Moreover, ISG15 suppresses viral infection in human cardiomyocytes, and patients with viral cardiomyopathy show conjugated ISG15 induction in the myocardium (Rahnefeld et al., 2014). These findings suggest that ISG15 activation in cardiomyocytes plays an important role in the fight against infectious pathogens, thereby diminishing inflammatory cardiomyopathy, heart failure, and mortality (Rahnefeld et al., 2014). However, the expression of ISG15 pathway components and several inflammatory cytokines is upregulated by cardiomyocyte-specific IkB kinase/NFκB activation, leading to cardiomyopathy and heart failure (Maier et al., 2012). Viruses and viral products can either activate or inhibit the NFκB cascade by direct or indirect binding to IKK subunits to enhance viral replication, evade the innate immune system, and establish an infection (Amaya et al., 2014). In a myocarditis model, cardiac innate immunity is partly mediated by lncRNA metastasis-associated lung adenocarcinoma transcript 1 (MALAT1) (Gast et al., 2016). In line with this finding, an analysis of apolipoprotein E-null mice with heterozygous MALAT1 deficiency revealed massive immune-system dysregulation and exaggerated atherosclerosis even when the mice were kept on a normal diet, and these features were associated with upregulated IFN signaling and Isg15 expression in splenocytes (Gast et al., 2019). Collectively, these results suggest that ISG15 expression can be both protective and deleterious for the cardiovascular system. In the following sections, we discuss studies that highlight an emerging role of the ISG15 pathway in hypertension, cardiac hypertrophy, diabetes, and obesity.
4.1 Hypertension and cardiac hypertrophy
Hypertension is a major risk factor for CVD, kidney disease, stroke, and diabetes (Fuchs and Whelton, 2020; Kim and Kim, 2022). Aging is associated with both hypertension and ISG15 activation. Although telomere length shows high interindividual variability, several population studies have shown an association between telomere ablation and hypertension (Fuster et al., 2007; Liu et al., 2019). For example, Demissie et al. demonstrated an association between hypertension and shorter leukocyte telomeres in men that appeared to be largely due to insulin resistance (Demissie et al., 2006), a disorder frequently associated with hypertension and age-associated diabetes (Saad et al., 2004; Sowers and Frohlich, 2004). Moreover, age-associated oxidative stress and chronic inflammation both contribute to telomere attrition and hypertension (Liu et al., 2019; Gavia-Garcia et al., 2021). However, these studies do not address whether telomere shortening is a cause or a consequence of hypertension.
A recent study of the ISG15 pathway in vascular pathophysiology found that Isg15 expression is increased in the aortas of hypertensive animals and in angiotensin II-treated vascular cells and macrophages (Gonzalez-Amor et al., 2022). The same study found that ISG15 expression in human peripheral blood mononuclear cells positively correlated with systolic and diastolic blood pressure and with carotid intima-media thickness and also found elevated ISG15 expression in human and mouse abdominal aortic aneurysms (Gonzalez-Amor et al., 2022). Moreover, Isg15-null mice were found to be protected against angiotensin II-induced hypertension, elastin remodeling, vascular stiffness, endothelial dysfunction, and expression of inflammatory and oxidative stress markers (Gonzalez-Amor et al., 2022). Conversely, excessive ISGylation in USP18C61A mice was associated with enhanced angiotensin II-induced hypertension, vascular fibrosis, inflammation, generation of reactive oxygen species (ROS), elastin breaks, and aortic dilation and rupture (Gonzalez-Amor et al., 2022). Importantly, treatment of angiotensin II-infused USP18C61A mice with the antioxidant tempol improved survival and reduced aneurysm formation and vascular remodeling (Gonzalez-Amor et al., 2022).
Hypertension is frequently associated with cardiac dilation and hypertrophy, and USP18 expression was found to be high both in dilated human hearts and in hypertrophic mice (Ying et al., 2016). USP18-null mice subjected to hypertrophic stimuli underwent exacerbated cardiac remodeling, and, conversely, cardiomyocyte-specific USP18 overexpression abrogated cardiac remodeling and dysfunction under the same pathological stimuli, a protective effect that was associated with inhibition of the transforming growth factor-β–activated kinase 1-p38/c-Jun N-terminal kinase 1/2 signaling cascade (Ying et al., 2016).
4.2 Type 2 diabetes mellitus (T2DM) and obesity
T2DM is considered a conventional risk factor for CVD and is associated with aging, obesity, hypertension, and hypercholesterolemia (Martin-Timon et al., 2014; Strain and Paldanius, 2018). Human studies have shown a positive correlation between plasma glucose, obesity, and Isg15 mRNA levels in peripheral blood mononuclear cells (Gonzalez-Amor et al., 2022). Moreover, mice fed a high-fat diet (HFD) show increased aortic mRNA expression of Isg15 and other inflammatory genes such as Ccl2, Il1b, and Tnfa (Ballesteros-Martinez et al., 2022). Using GEO data base query and related online analytical tools, Sun et al. identified ISG15 amongst five genes that were related to both T2M and ovarian cancer, and found that ISG15 expression in ovarian cancer biopsies significantly correlated with patient’s survival time (Sun et al., 2019). The authors concluded that genes and proteins involved in glycometabolism are related to ovarian cancer and may serve as potential therapeutic targets. In another study, the innate immune transcription factor IRF3 was shown to strongly repress thermogenic gene expression and oxygen consumption in adipocytes by upregulating ISG15, which ISGylates several glycolytic enzymes, thus decreasing their function and reducing lactate production (Yan et al., 2021). Moreover, Isg15-null mice had elevated energy expenditure and developed resistance to diet-induced obesity, which was attributed to lack of ISGylation of glycolytic enzymes in adipocytes (Yan et al., 2021).
A recent study demonstrated elevated expression of the ubiquitin/ISG15-conjugating enzyme UBE2L6 in white adipose tissue from obese mice and humans; moreover, HFD-fed mice with adipose tissue-specific Ube2l6 ablation had a reduced content of subcutaneous and visceral white adipose tissue, as well as attenuated glucose intolerance, insulin resistance, compensatory hyperinsulinemia, hypercholesterolemia, and hepatic steatosis (Wei et al., 2021). These effects of adipocyte-specific Ube2l6 were associated with the stabilization of adipose triglyceride lipase (Atgl) (Wei et al., 2021).
5 Discussion
The world population is aging rapidly, and age is the main risk factor for CVD. Consequently, CVD is the main cause of death in developed societies, where it presents a huge medical and socioeconomic burden. It is therefore of unparalleled importance to understand the cellular and molecular mechanisms governing aging and age-associated CVD. In this review, we have discussed studies that highlight a role of ISG15 as a key element in aging and age-associated CVD beyond its classical roles in the defense against pathogens and as a tumor suppressor. Accumulating evidence links ISG15 to well-characterized aging processes that play major roles in cellular stress responses, such DNA damage, defective DDR, telomere attrition, ROS production, and inflammation, as well as some CVD risk factors (Figure 2). Although targeting ISG15, USP18, and UBE2L6 has the potential to alleviate hypertension, T2DM, and age-associated cardiomyopathies, we are still a long way from understanding the specific consequences of ISGylation on validated substrates (Zhao et al., 2005) and how ISGylation is regulated in a context-specific manner, knowledge that will be essential for the development of ISGylation-targeted therapies.
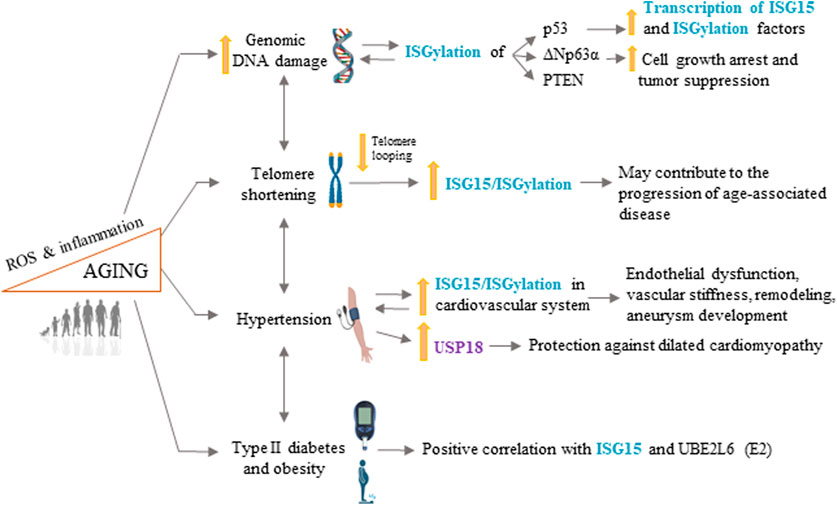
FIGURE 2. ISG15 in longevity and age-related cardiovascular disease. ISG15 is associated with several processes involved in aging and age-related cardiovascular disease, including increased genomic DNA damage, telomere shortening, hypertension, type II diabetes, and obesity. ROS, reactive oxygen species. Some illustrations were created using BioRender.com.
ISG15 is induced by IFNs and TNFα and can therefore be considered a pro-inflammatory gene. Chronic inflammation and accompanying oxidative stress are thought to contribute to different forms of age-associated CVD (Kofler et al., 2005; Vicenova et al., 2009; Karbach et al., 2014). However, the use of anti-inflammatory drugs or antioxidants to ameliorate CVD has yet to yield conclusive results (Mangge et al., 2014; Kosmas et al., 2019; Mirmiran et al., 2022). For example, treatment with anti-TNFα antibody (infliximab, ATTACH trial) or a soluble TNF receptor (etanercept, RECOVER and RENAISSANCE trials) has shown no effect or even deleterious effects in patients with heart failure (Coletta et al., 2002; Chung et al., 2003). In contrast, treatment of myocardial infarction patients with anti-interleukin-1β antibody (Canakinumab, CANTOS) produced a significant reduction in cardiovascular event rates without changing lipid levels, blood pressure, or incident hypertension (Ridker et al., 2017; Rothman et al., 2020). Future studies should explore the potential benefit of therapies targeting ISG15 and other ISGylation factors as a way to break the vicious cycle of ROS production and inflammation that leads to CVD. However, it may prove difficult to avoid undesired collateral effects of targeting ISG15. Several layers of difficulty are likely to impinge on any therapy targeting ISG15, given its multiple substrates and cellular functions (including pathogen defense and tumor suppression), its context-specific effects, and the complexity of the pro-inflammatory pathways in which it participates. Despite these challenges, further research on ISG15 regulation and functions are likely to shed light on the mechanisms that drive aging and age-associated CVD.
Author contributions
MG-A and BD reviewed the scientific literature related to the topic of this mini-review. VA provided funding. All authors participated in writing the manuscript and approved the final version of the manuscript.
Funding
VA lab is supported by Ministerio de Ciencia e Innovación (MICINN)/Agencia Estatal de Investigación (AEI) (grant PID2019-108489RB-I00/AEI/10.13039/501100011033) with cofund from Fondo Social Europeo “El FSE invierte en tu futuro”, the Progeria Research Foundation (grant PRF2019–77), Asociación Progeria Alexandra Peraut, Fundació Marató TV3 (grant 202033-31), and Sociedad Española de Cardiología (SEC/FEC-INV-BAS 22/005). MG-A is supported by the MCINN (post-doctoral contract FJC2021-047576-I). The CNIC is supported by the MICINN, the Instituto de Salud Carlos III, the Pro-CNIC Foundation, and is a Severo Ochoa Center of Excellence (grant CEX2020-001041-S funded by MICIN/AEI/10.13039/501100011033).
Acknowledgments
We thank Simon Bartlett for English editing.
Conflict of interest
The authors declare that the research was conducted in the absence of any commercial or financial relationships that could be construed as a potential conflict of interest.
Publisher’s note
All claims expressed in this article are solely those of the authors and do not necessarily represent those of their affiliated organizations, or those of the publisher, the editors and the reviewers. Any product that may be evaluated in this article, or claim that may be made by its manufacturer, is not guaranteed or endorsed by the publisher.
References
Albert, M., Becares, M., Falqui, M., Fernandez-Lozano, C., and Guerra, S. (2018). ISG15, a small molecule with huge implications: Regulation of mitochondrial homeostasis. Viruses 10 (11), 629. doi:10.3390/v10110629
Alvarez-Garcia, V., Tawil, Y., Wise, H. M., and Leslie, N. R. (2019). Mechanisms of PTEN loss in cancer: It's all about diversity. Semin. Cancer Biol. 59, 66–79. doi:10.1016/j.semcancer.2019.02.001
Amaya, M., Keck, F., Bailey, C., and Narayanan, A. (2014). The role of the IKK complex in viral infections. Pathog. Dis. 72 (1), 32–44. doi:10.1111/2049-632X.12210
Ballesteros-Martinez, C., Rodrigues-Diez, R., Beltran, L. M., Moreno-Carriles, R., Martinez-Martinez, E., Gonzalez-Amor, M., et al. (2022). Microsomal prostaglandin E synthase-1 is involved in the metabolic and cardiovascular alterations associated with obesity. Br. J. Pharmacol. 179 (11), 2733–2753. doi:10.1111/bph.15776
Basters, A., Knobeloch, K. P., and Fritz, G. (2018). USP18 - a multifunctional component in the interferon response. Biosci. Rep. 38 (6), 1. doi:10.1042/BSR20180250
Bogunovic, D., Byun, M., Durfee, L. A., Abhyankar, A., Sanal, O., Mansouri, D., et al. (2012). Mycobacterial disease and impaired IFN-gamma immunity in humans with inherited ISG15 deficiency. Science 337 (6102), 1684–1688. doi:10.1126/science.1224026
Chairatvit, K., Wongnoppavich, A., and Choonate, S. (2012). Up-regulation of interferon-stimulated gene15 and its conjugates by tumor necrosis factor-alpha via type I interferon-dependent and -independent pathways. Mol. Cell Biochem. 368 (1-2), 195–201. doi:10.1007/s11010-012-1360-5
Chang, J. J., Woods, M., Lindsay, R. J., Doyle, E. H., Griesbeck, M., Chan, E. S., et al. (2013). Higher expression of several interferon-stimulated genes in HIV-1-infected females after adjusting for the level of viral replication. J. Infect. Dis. 208 (5), 830–838. doi:10.1093/infdis/jit262
Chang, Y. G., Yan, X. Z., Xie, Y. Y., Gao, X. C., Song, A. X., Zhang, D. E., et al. (2008). Different roles for two ubiquitin-like domains of ISG15 in protein modification. J. Biol. Chem. 283 (19), 13370–13377. doi:10.1074/jbc.M800162200
Cheedipudi, S. M., Matkovich, S. J., Coarfa, C., Hu, X., Robertson, M. J., Sweet, M., et al. (2019). Genomic reorganization of lamin-associated domains in cardiac myocytes is associated with differential gene expression and DNA methylation in human dilated cardiomyopathy. Circ. Res. 124 (8), 1198–1213. doi:10.1161/CIRCRESAHA.118.314177
Chen, S. N., Lombardi, R., Karmouch, J., Tsai, J. Y., Czernuszewicz, G., Taylor, M. R. G., et al. (2019). DNA damage response/TP53 pathway is activated and contributes to the pathogenesis of dilated cardiomyopathy associated with LMNA (lamin A/C) mutations. Circ. Res. 124 (6), 856–873. doi:10.1161/CIRCRESAHA.118.314238
Chung, E. S., Packer, M., Lo, K. H., Fasanmade, A. A., and Willerson, J. T. (2003). Anti-TNF therapy against congestive heart failure InvestigatorsRandomized, double-blind, placebo-controlled, pilot trial of infliximab, a chimeric monoclonal antibody to tumor necrosis factor-alpha, in patients with moderate-to-severe heart failure: Results of the anti-TNF therapy against congestive heart failure (ATTACH) trial. Circulation 107 (25), 3133–3140. doi:10.1161/01.CIR.0000077913.60364.D2
Coletta, A. P., Clark, A. L., Banarjee, P., and Cleland, J. G. (2002). Clinical trials update: RENEWAL (RENAISSANCE and RECOVER) and ATTACH. Eur. J. Heart Fail 4 (4), 559–561. doi:10.1016/s1388-9842(02)00121-6
Colonne, P. M., Sahni, A., and Sahni, S. K. (2011). Rickettsia conorii infection stimulates the expression of ISG15 and ISG15 protease UBP43 in human microvascular endothelial cells. Biochem. Biophys. Res. Commun. 416 (1-2), 153–158. doi:10.1016/j.bbrc.2011.11.015
Cummings, K., Watkins, A., Jones, C., Dias, R., and Welham, A. (2022). Behavioural and psychological features of PTEN mutations: A systematic review of the literature and meta-analysis of the prevalence of autism spectrum disorder characteristics. J. Neurodev. Disord. 14 (1), 1. doi:10.1186/s11689-021-09406-w
Demissie, S., Levy, D., Benjamin, E. J., Cupples, L. A., Gardner, J. P., Herbert, A., et al. (2006). Insulin resistance, oxidative stress, hypertension, and leukocyte telomere length in men from the Framingham Heart Study. Aging Cell 5 (4), 325–330. doi:10.1111/j.1474-9726.2006.00224.x
Di Micco, R., Krizhanovsky, V., Baker, D., and d'Adda di Fagagna, F. (2021). Cellular senescence in ageing: From mechanisms to therapeutic opportunities. Nat. Rev. Mol. Cell Biol. 22 (2), 75–95. doi:10.1038/s41580-020-00314-w
Domizio, J. D., Gulen, M. F., Saidoune, F., Thacker, V. V., Yatim, A., Sharma, K., et al. (2022). The cGAS-STING pathway drives type I IFN immunopathology in COVID-19. Nature 603 (7899), 145–151. doi:10.1038/s41586-022-04421-w
Dos Santos, P. F., and Mansur, D. S. (2017). Beyond ISGlylation: Functions of free intracellular and extracellular ISG15. J. Interferon Cytokine Res. 37 (6), 246–253. doi:10.1089/jir.2016.0103
Durfee, L. A., and Huibregtse, J. M. (2012). The ISG15 conjugation system. Methods Mol. Biol. 832, 141–149. doi:10.1007/978-1-61779-474-2_9
Dzimianski, J. V., Scholte, F. E. M., Bergeron, E., and Pegan, S. D. (2019). ISG15: It's Complicated. J. Mol. Biol. 431 (21), 4203–4216. doi:10.1016/j.jmb.2019.03.013
Fuchs, F. D., and Whelton, P. K. (2020). High blood pressure and cardiovascular disease. Hypertension 75 (2), 285–292. doi:10.1161/HYPERTENSIONAHA.119.14240
Fuster, J. J., Diez, J., and Andres, V. (2007). Telomere dysfunction in hypertension. J. Hypertens. 25 (11), 2185–2192. doi:10.1097/HJH.0b013e3282ef6196
Galkin, F., Zhang, B., Dmitriev, S. E., and Gladyshev, V. N. (2019). Reversibility of irreversible aging. Ageing Res. Rev. 49, 104–114. doi:10.1016/j.arr.2018.11.008
Gast, M., Rauch, B. H., Nakagawa, S., Haghikia, A., Jasina, A., Haas, J., et al. (2019). Immune system-mediated atherosclerosis caused by deficiency of long non-coding RNA MALAT1 in ApoE-/-mice. Cardiovasc Res. 115 (2), 302–314. doi:10.1093/cvr/cvy202
Gast, M., Schroen, B., Voigt, A., Haas, J., Kuehl, U., Lassner, D., et al. (2016). Long noncoding RNA MALAT1-derived mascRNA is involved in cardiovascular innate immunity. J. Mol. Cell Biol. 8 (2), 178–181. doi:10.1093/jmcb/mjw003
Gavia-Garcia, G., Rosado-Perez, J., Arista-Ugalde, T. L., Aguiniga-Sanchez, I., Santiago-Osorio, E., and Mendoza-Nunez, V. M. (2021). Telomere length and oxidative stress and its relation with metabolic syndrome components in the aging. Biol. (Basel). 10 (4), 253. doi:10.3390/biology10040253
Gonzalez-Amor, M., Garcia-Redondo, A. B., Jorge, I., Zalba, G., Becares, M., Ruiz-Rodriguez, M. J., et al. (2022). Interferon-stimulated gene 15 pathway is a novel mediator of endothelial dysfunction and aneurysms development in angiotensin II infused mice through increased oxidative stress. Cardiovasc Res. 118 (16), 3250–3268. doi:10.1093/cvr/cvab321
Guerra, S., Caceres, A., Knobeloch, K. P., Horak, I., and Esteban, M. (2008). Vaccinia virus E3 protein prevents the antiviral action of ISG15. PLoS Pathog. 4 (7), e1000096. doi:10.1371/journal.ppat.1000096
Hamczyk, M. R., del Campo, L., and Andres, V. (2018). Aging in the cardiovascular system: Lessons from hutchinson-gilford Progeria syndrome. Annu. Rev. Physiol. 80, 27–48. doi:10.1146/annurev-physiol-021317-121454
Hamczyk, M. R., Nevado, R. M., Barettino, A., Fuster, V., and Andres, V. (2020). Biological versus chronological aging: JACC focus seminar. J. Am. Coll. Cardiol. 75 (8), 919–930. doi:10.1016/j.jacc.2019.11.062
Han, H. G., Moon, H. W., and Jeon, Y. J. (2018). ISG15 in cancer: Beyond ubiquitin-like protein. Cancer Lett. 438, 52–62. doi:10.1016/j.canlet.2018.09.007
Hayflick, L., and Moorhead, P. S. (1961). The serial cultivation of human diploid cell strains. Exp. Cell Res. 25, 585–621. doi:10.1016/0014-4827(61)90192-6
Hayflick, L. (1965). The limited in vitro lifetime of human diploid cell strains. Exp. Cell Res. 37, 614–636. doi:10.1016/0014-4827(65)90211-9
Hoeijmakers, J. H. (2009). DNA damage, aging, and cancer. N. Engl. J. Med. 361 (15), 1475–1485. doi:10.1056/NEJMra0804615
Hoffmann, J., Richardson, G., Haendeler, J., Altschmied, J., Andres, V., and Spyridopoulos, I. (2021). Telomerase as a therapeutic target in cardiovascular disease. Arterioscler. Thromb. Vasc. Biol. 41 (3), 1047–1061. doi:10.1161/ATVBAHA.120.315695
Honke, N., Shaabani, N., Zhang, D. E., Hardt, C., and Lang, K. S. (2016). Multiple functions of USP18. Cell Death Dis. 7 (11), e2444. doi:10.1038/cddis.2016.326
Hsiang, T. Y., Zhao, C., and Krug, R. M. (2009). Interferon-induced ISG15 conjugation inhibits influenza A virus gene expression and replication in human cells. J. Virol. 83 (12), 5971–5977. doi:10.1128/JVI.01667-08
Huang, Y. F., Wee, S., Gunaratne, J., Lane, D. P., and Bulavin, D. V. (2014). Isg15 controls p53 stability and functions. Cell Cycle 13 (14), 2200–2210. doi:10.4161/cc.29209
Jeon, Y. J., Jo, M. G., Yoo, H. M., Hong, S. H., Park, J. M., Ka, S. H., et al. (2012). Chemosensitivity is controlled by p63 modification with ubiquitin-like protein ISG15. J. Clin. Invest. 122 (7), 2622–2636. doi:10.1172/JCI61762
Jeon, Y. J., Yoo, H. M., and Chung, C. H. (2010). ISG15 and immune diseases. Biochim. Biophys. Acta 1802 (5), 485–496. doi:10.1016/j.bbadis.2010.02.006
Kang, J. A., Kim, Y. J., and Jeon, Y. J. (2022). The diverse repertoire of ISG15: More intricate than initially thought. Exp. Mol. Med. 54 (11), 1779–1792. doi:10.1038/s12276-022-00872-3
Karbach, S., Wenzel, P., Waisman, A., Munzel, T., and Daiber, A. (2014). eNOS uncoupling in cardiovascular diseases--the role of oxidative stress and inflammation. Curr. Pharm. Des. 20 (22), 3579–3594. doi:10.2174/13816128113196660748
Kim, H. J., and Kim, K. I. (2022). Blood pressure target in type 2 diabetes mellitus. Diabetes Metab. J. 46 (5), 667–674. doi:10.4093/dmj.2022.0215
Knight, E., and Cordova, B. (1991). IFN-induced 15-kDa protein is released from human lymphocytes and monocytes. J. Immunol. 146 (7), 2280–2284. doi:10.4049/jimmunol.146.7.2280
Kofler, S., Nickel, T., and Weis, M. (2005). Role of cytokines in cardiovascular diseases: A focus on endothelial responses to inflammation. Clin. Sci. (Lond). 108 (3), 205–213. doi:10.1042/CS20040174
Kosmas, C. E., Silverio, D., Sourlas, A., Montan, P. D., Guzman, E., and Garcia, M. J. (2019). Anti-inflammatory therapy for cardiovascular disease. Ann. Transl. Med. 7 (7), 147. doi:10.21037/atm.2019.02.34
Kunzi, M. S., and Pitha, P. M. (1996). Role of interferon-stimulated gene ISG-15 in the interferon-omega-mediated inhibition of human immunodeficiency virus replication. J. Interferon Cytokine Res. 16 (11), 919–927. doi:10.1089/jir.1996.16.919
Lee, Y. R., Chen, M., and Pandolfi, P. P. (2018). The functions and regulation of the PTEN tumour suppressor: New modes and prospects. Nat. Rev. Mol. Cell Biol. 19 (9), 547–562. doi:10.1038/s41580-018-0015-0
Lenschow, D. J., Lai, C., Frias-Staheli, N., Giannakopoulos, N. V., Lutz, A., Wolff, T., et al. (2007). IFN-stimulated gene 15 functions as a critical antiviral molecule against influenza, herpes, and Sindbis viruses. Proc. Natl. Acad. Sci. U. S. A. 104 (4), 1371–1376. doi:10.1073/pnas.0607038104
Lertsooksawat, W., Wongnoppavich, A., and Chairatvit, K. (2019). Up-regulation of interferon-stimulated gene 15 and its conjugation machinery, UbE1L and UbcH8 expression by tumor necrosis factor-alpha through p38 MAPK and JNK signaling pathways in human lung carcinoma. Mol. Cell Biochem. 462 (1-2), 51–59. doi:10.1007/s11010-019-03609-5
Levy, D. E., Lew, D. J., Decker, T., Kessler, D. S., and Darnell, J. E. (1990). Synergistic interaction between interferon-alpha and interferon-gamma through induced synthesis of one subunit of the transcription factor ISGF3. EMBO J. 9 (4), 1105–1111. doi:10.1002/j.1460-2075.1990.tb08216.x
Li, A., Qiu, M., Zhou, H., Wang, T., and Guo, W. P. T. E. N. (2017). PTEN, insulin resistance and cancer. Curr. Pharm. Des. 23 (25), 3667–3676. doi:10.2174/1381612823666170704124611
Liu, B., Wang, J., Chan, K. M., Tjia, W. M., Deng, W., Guan, X., et al. (2005). Genomic instability in laminopathy-based premature aging. Nat. Med. 11 (7), 780–785. doi:10.1038/nm1266
Liu, P., Zhang, Y., and Ma, L. (2019). Telomere length and associated factors in older adults with hypertension. J. Int. Med. Res. 47 (11), 5465–5474. doi:10.1177/0300060519882570
Lopez-Otin, C., Blasco, M. A., Partridge, L., Serrano, M., and Kroemer, G. (2013). The hallmarks of aging. Cell 153 (6), 1194–1217. doi:10.1016/j.cell.2013.05.039
Lou, Z., Wei, J., Riethman, H., Baur, J. A., Voglauer, R., Shay, J. W., et al. (2009). Telomere length regulates ISG15 expression in human cells. Aging (Albany NY) 1 (7), 608–621. doi:10.18632/aging.100066
Luo, Q., Sun, W., Wang, Y. F., Li, J., and Li, D. W. (2022). Association of p53 with neurodegeneration in Parkinson's disease. Park. Dis. 2022, 6600944. doi:10.1155/2022/6600944
MacParland, S. A., Ma, X. Z., Chen, L., Khattar, R., Cherepanov, V., Selzner, M., et al. (2016). Lipopolysaccharide and tumor necrosis factor alpha inhibit interferon signaling in hepatocytes by increasing ubiquitin-like protease 18 (USP18) expression. J. Virol. 90 (12), 5549–5560. doi:10.1128/JVI.02557-15
Maier, H. J., Schips, T. G., Wietelmann, A., Kruger, M., Brunner, C., Sauter, M., et al. (2012). Cardiomyocyte-specific IκB kinase (IKK)/NF-κB activation induces reversible inflammatory cardiomyopathy and heart failure. Proc. Natl. Acad. Sci. U. S. A. 109 (29), 11794–11799. doi:10.1073/pnas.1116584109
Mangge, H., Becker, K., Fuchs, D., and Gostner, J. M. (2014). Antioxidants, inflammation and cardiovascular disease. World J. Cardiol. 6 (6), 462–477. doi:10.4330/wjc.v6.i6.462
Martin-Timon, I., Sevillano-Collantes, C., Segura-Galindo, A., and Del Canizo-Gomez, F. J. (2014). Type 2 diabetes and cardiovascular disease: Have all risk factors the same strength? World J. Diabetes 5 (4), 444–470. doi:10.4239/wjd.v5.i4.444
Mirmiran, P., Hosseini-Esfahani, F., Esfandiar, Z., Hosseinpour-Niazi, S., and Azizi, F. (2022). Associations between dietary antioxidant intakes and cardiovascular disease. Sci. Rep. 12 (1), 1504. doi:10.1038/s41598-022-05632-x
Mustachio, L. M., Kawakami, M., Lu, Y., Rodriguez-Canales, J., Mino, B., Behrens, C., et al. (2017). The ISG15-specific protease USP18 regulates stability of PTEN. Oncotarget 8 (1), 3–14. doi:10.18632/oncotarget.13914
Nassour, J., Aguiar, L. G., Correia, A., Schmidt, T. T., Mainz, L., Przetocka, S., et al. (2023). Telomere-to-mitochondria signalling by ZBP1 mediates replicative crisis. Nature 614 (7949), 767–773. doi:10.1038/s41586-023-05710-8
Nassour, J., Radford, R., Correia, A., Fuste, J. M., Schoell, B., Jauch, A., et al. (2019). Autophagic cell death restricts chromosomal instability during replicative crisis. Nature 565 (7741), 659–663. doi:10.1038/s41586-019-0885-0
Ostvik, A. E., Svendsen, T. D., Granlund, A. V. B., Doseth, B., Skovdahl, H. K., Bakke, I., et al. (2020). Intestinal epithelial cells express immunomodulatory ISG15 during active ulcerative colitis and crohn's disease. J. Crohns Colitis 14 (7), 920–934. doi:10.1093/ecco-jcc/jjaa022
Park, J. H., Yang, S. W., Park, J. M., Ka, S. H., Kim, J. H., Kong, Y. Y., et al. (2016). Positive feedback regulation of p53 transactivity by DNA damage-induced ISG15 modification. Nat. Commun. 7, 12513. doi:10.1038/ncomms12513
Rahnefeld, A., Klingel, K., Schuermann, A., Diny, N. L., Althof, N., Lindner, A., et al. (2014). Ubiquitin-like protein ISG15 (interferon-stimulated gene of 15 kDa) in host defense against heart failure in a mouse model of virus-induced cardiomyopathy. Circulation 130 (18), 1589–1600. doi:10.1161/CIRCULATIONAHA.114.009847
Ridker, P. M., Everett, B. M., Thuren, T., MacFadyen, J. G., Chang, W. H., Ballantyne, C., et al. (2017). Antiinflammatory therapy with canakinumab for atherosclerotic disease. N. Engl. J. Med. 377 (12), 1119–1131. doi:10.1056/nejmoa1707914
Robin, J. D., Ludlow, A. T., Batten, K., Magdinier, F., Stadler, G., Wagner, K. R., et al. (2014). Telomere position effect: Regulation of gene expression with progressive telomere shortening over long distances. Genes Dev. 28 (22), 2464–2476. doi:10.1101/gad.251041.114
Rothman, A. M., MacFadyen, J., Thuren, T., Webb, A., Harrison, D. G., Guzik, T. J., et al. (2020). Effects of interleukin-1β inhibition on blood pressure, incident hypertension, and residual inflammatory risk: A secondary analysis of CANTOS. Hypertension 75 (2), 477–482. doi:10.1161/HYPERTENSIONAHA.119.13642
Saad, M. F., Rewers, M., Selby, J., Howard, G., Jinagouda, S., Fahmi, S., et al. (2004). Insulin resistance and hypertension: The insulin resistance atherosclerosis study. Hypertension 43 (6), 1324–1331. doi:10.1161/01.HYP.0000128019.19363.f9
Sandy, Z., da Costa, I. C., and Schmidt, C. K. (2020). More than meets the ISG15: Emerging roles in the DNA damage response and beyond. Biomolecules 10 (11), 1557. doi:10.3390/biom10111557
Schumacher, B., Garinis, G. A., and Hoeijmakers, J. H. (2008). Age to survive: DNA damage and aging. Trends Genet. 24 (2), 77–85. doi:10.1016/j.tig.2007.11.004
Schumacher, B., Pothof, J., Vijg, J., and Hoeijmakers, J. H. J. (2021). The central role of DNA damage in the ageing process. Nature 592 (7856), 695–703. doi:10.1038/s41586-021-03307-7
Sowers, J. R., and Frohlich, E. D. (2004). Insulin and insulin resistance: Impact on blood pressure and cardiovascular disease. Med. Clin. North Am. 88 (1), 63–82. doi:10.1016/s0025-7125(03)00128-7
Speer, S. D., Li, Z., Buta, S., Payelle-Brogard, B., Qian, L., Vigant, F., et al. (2016). ISG15 deficiency and increased viral resistance in humans but not mice. Nat. Commun. 7, 11496. doi:10.1038/ncomms11496
Strain, W. D., and Paldanius, P. M. (2018). Diabetes, cardiovascular disease and the microcirculation. Cardiovasc Diabetol. 17 (1), 57. doi:10.1186/s12933-018-0703-2
Sun, Y., Xiaoyan, H., Yun, L., Chaoqun, L., Jialing, W., Liu, Y., et al. (2019). Identification of key candidate genes and pathways for relationship between ovarian cancer and diabetes mellitus using bioinformatical analysis. Asian Pac J. Cancer Prev. 20 (1), 145–155. doi:10.31557/APJCP.2019.20.1.145
Tecalco Cruz, A. C., and Mejia-Barreto, K. (2017). Cell type-dependent regulation of free ISG15 levels and ISGylation. J. Cell Commun. Signal 11 (2), 127–135. doi:10.1007/s12079-017-0385-7
Varela, I., Cadinanos, J., Pendas, A. M., Gutierrez-Fernandez, A., Folgueras, A. R., Sanchez, L. M., et al. (2005). Accelerated ageing in mice deficient in Zmpste24 protease is linked to p53 signalling activation. Nature 437 (7058), 564–568. doi:10.1038/nature04019
Vicenova, B., Vopalensky, V., Burysek, L., and Pospisek, M. (2009). Emerging role of interleukin-1 in cardiovascular diseases. Physiol. Res. 58 (4), 481–498. doi:10.33549/physiolres.931673
Villarroya-Beltri, C., Guerra, S., and Sanchez-Madrid, F. (2017). ISGylation - a key to lock the cell gates for preventing the spread of threats. J. Cell Sci. 130 (18), 2961–2969. doi:10.1242/jcs.205468
von Zglinicki, T., Wan, T., and Miwa, S. (2021). Senescence in post-mitotic cells: A driver of aging? Antioxid. Redox Signal 34 (4), 308–323. doi:10.1089/ars.2020.8048
Wei, W., and Ji, S. (2018). Cellular senescence: Molecular mechanisms and pathogenicity. J. Cell Physiol. 233 (12), 9121–9135. doi:10.1002/jcp.26956
Wei, W., Li, Y., Li, Y., and Li, D. (2021). Adipose-specific knockout of ubiquitin-conjugating enzyme E2L6 (Ube2l6) reduces diet-induced obesity, insulin resistance, and hepatic steatosis. J. Pharmacol. Sci. 145 (4), 327–334. doi:10.1016/j.jphs.2020.12.008
Wei, W., and Sedivy, J. M. (1999). Differentiation between senescence (M1) and crisis (M2) in human fibroblast cultures. Exp. Cell Res. 253 (2), 519–522. doi:10.1006/excr.1999.4665
Yan, S., Kumari, M., Xiao, H., Jacobs, C., Kochumon, S., Jedrychowski, M., et al. (2021). IRF3 reduces adipose thermogenesis via ISG15-mediated reprogramming of glycolysis. J. Clin. Invest. 131 (7), e144888. doi:10.1172/JCI144888
Yazdanyar, A., and Newman, A. B. (2009). The burden of cardiovascular disease in the elderly: Morbidity, mortality, and costs. Clin. Geriatr. Med. 25 (4), 563–577. doi:10.1016/j.cger.2009.07.007
Ying, X., Zhao, Y., Yao, T., Yuan, A., Xu, L., Gao, L., et al. (2016). Novel protective role for ubiquitin-specific protease 18 in pathological cardiac remodeling. Hypertension 68 (5), 1160–1170. doi:10.1161/HYPERTENSIONAHA.116.07562
Yuan, W., and Krug, R. M. (2001). Influenza B virus NS1 protein inhibits conjugation of the interferon (IFN)-induced ubiquitin-like ISG15 protein. EMBO J. 20 (3), 362–371. doi:10.1093/emboj/20.3.362
Zhang, D., and Zhang, D. E. (2011). Interferon-stimulated gene 15 and the protein ISGylation system. J. Interferon Cytokine Res. 31 (1), 119–130. doi:10.1089/jir.2010.0110
Zhang, N., Li, Y., Lai, T. P., Shay, J. W., and Danuser, G. (2021). Imaging assay to probe the role of telomere length shortening on telomere-gene interactions in single cells. Chromosoma 130 (1), 61–73. doi:10.1007/s00412-020-00747-4
Keywords: ISG15, aging, telomere shortening, DNA damage response, hypertension, diabetes, obesity
Citation: González-Amor M, Dorado B and Andrés V (2023) Emerging roles of interferon-stimulated gene-15 in age-related telomere attrition, the DNA damage response, and cardiovascular disease. Front. Cell Dev. Biol. 11:1128594. doi: 10.3389/fcell.2023.1128594
Received: 20 December 2022; Accepted: 08 March 2023;
Published: 21 March 2023.
Edited by:
Hua Zhu, Wexner Medical Center, The Ohio State University, United StatesReviewed by:
Sivareddy Kotla, University of Texas MD Anderson Cancer Center, United StatesUsman Alizai, Wexner Medical Center, The Ohio State University, United States
Miguel Godinho Ferreira, Université Côte d’Azur, France
Copyright © 2023 González-Amor, Dorado and Andrés. This is an open-access article distributed under the terms of the Creative Commons Attribution License (CC BY). The use, distribution or reproduction in other forums is permitted, provided the original author(s) and the copyright owner(s) are credited and that the original publication in this journal is cited, in accordance with accepted academic practice. No use, distribution or reproduction is permitted which does not comply with these terms.
*Correspondence: Vicente Andrés, dmFuZHJlc0BjbmljLmVz