- 1Department of Ophthalmology, Peking University Third Hospital, Beijing Key Laboratory of Restoration of Damaged Ocular Nerve, Beijing, China
- 2Cixi Institute of BioMedical Engineering, Ningbo Institute of Materials Technology and Engineering, Chinese Academy of Sciences, Ningbo, China
Purpose: To evaluate the retinal microvascular alteration after implantable collamer lens (ICL) implantation in moderate to high myopia patients using quantitative optical coherence tomography angiography (OCTA).
Methods: This prospective cohort study included 50 eyes of 25 patients with preoperative spherical equivalent ≥ −3.00 D. Patients underwent bilateral ICL implantation at the Department of Ophthalmology, Peking University Third Hospital, from November 2018 to July 2019. OCTA was used to image the superficial and deep retinal capillary plexuses before ICL implantation surgery and at 3 months follow-up.
Results: There was no significant difference in the microvascular density within each annular zone and all quadrantal zones of the superficial and deep layers found in myopia patients before and after ICL surgery.
Conclusion: Levels of microvascular density in retinal capillary plexuses were stable, as detected by the OCTA, showing the high security of ICL implantation, which would not leave adverse effects on retinal microvasculature in myopia patients.
1 Introduction
With the increase in educational pressure and limited time outdoors, myopia has become the most common vision problem (Morgan et al., 2018). Recent epidemiological studies indicated a prevalence of myopia as high as 80%–90% in young adults in East Asia (Foster and Jiang, 2014; Wu et al., 2016). It is estimated that, in 2050, half of the world’s population will be affected with myopia and 10% of people will be at a relevant risk of becoming blind as a result of high myopia (Holden et al., 2016; Hopf and Pfeiffer, 2017). With the elongation of the eyeball that occurs with the progression of myopia, the retinal microvascular decrease was observed in the myopia subjects (Yang et al., 2016; Al-Sheikh et al., 2017; Li et al., 2017).
Since the introduction of the Implantable Collamer Lens (ICL; Staar Surgical, Nidau, Switzerland) in 1993, refractive surgery has entered a new era of myopia treatment (Assetto et al., 1996). Because it significantly increased best-corrected visual acuity (BCVA) while reducing caused higher-order aberrations and improving postoperative contrast sensitivity (Wang and Zhou, 2016), the ICL is most frequently used to correct high and extreme myopia. In addition, the ICL performs superbly in the treatment of low to moderate myopia (Kamiya et al., 2012; Dougherty and Priver, 2017; Kamiya et al., 2018).
However, multiple studies revealed that intraocular refractive surgery, such as cataract surgery, may lead to early retinal ischemia, hypoxia, or even retinal vasculitis (Alnawaiseh et al., 2018; Kim et al., 2018; Pilotto et al., 2019; Liu J et al., 2021). Retinal complications would cause a potential or substantial threat to patients’ vision. So, it is vital to monitor retinal microvascular alteration after intraocular refractive surgery. ICL implantation is also a kind of intraocular refractive surgery. However, it is still unknown whether the ICL implantation surgery will affect the retinal microvasculature of myopic eyes.
Optical coherence tomography angiography (OCTA) is a new, non-invasive imaging technique with wide application potential for retinal vascular disease (de Carlo et al., 2015; Gao et al., 2016; Wylegala, 2018). In 2006, Optical Coherence Angiography was first performed to visualize the vasculature in the human eyes (Makita et al., 2006). OCTA can produce high-resolution, three-dimensional images and measure the microvascular network in different layers of the retina structure without the use of contrast agents (Gao et al., 2016; Zhang Q et al., 2016). This study aimed to use OCTA to uncover potential retinal capillary network alterations induced by ICL implantation surgery.
2 Materials and methods
2.1 Participants
This study includes a total of 50 eyes from 25 participants with moderate and high myopia. All subjects underwent ICL implantation surgery at the Department of Ophthalmology, Peking University Third Hospital between November 2018 and July 2019. Inclusion criteria: 21–45 years old, binocular myopia, with a spherical equivalent of greater than −3.00 diopters (D), anterior chamber depth (ACD) ≥ 2.8 mm, corneal endothelial cell count (cECC) ≥ 2000 cells/mm2, SE remained unchanged for more than 1 year, unsatisfactory vision with contact lenses or spectacles. All patients included in this study had no history of intraocular surgery and showed no other ocular pathologies (uveitis, glaucoma, cataract, keratoconus, severe dry eye, etc.) or serious systemic diseases (diabetes, uncontrolled hypertension, severe hyperthyroidism, etc.).
The method of this study was approved by the Ethics Committee of Peking University Third Hospital (M2020240). In addition, this study was registered and approved on Clinical Trials.gov (NCT04443231). Each subject was given informed consent after an adequate study explanation.
Before surgery, each subject got a full ocular examination: The ACD (measured from the endothelium to the crystalline lens) was measured using anterior segment Optical Coherence Tomography (Visante-OCT; Carl Zeiss Meditec, Jena, Germany), the horizontal white-to-white (WTW) distance and axial length (AL) were measured by optical biometry (IOL Master 700; Carl Zeiss Meditec, Jena, Germany), cECC was obtained from each eye, using a corneal endothelial microscope (SP-2000; Topcon, Tokyo, Japan). Additionally, each eye was subjected to slit-lamp biomicroscopic examination, corneal topography, and funduscopic examination.
The size of the ICL was calculated with a STAAR sizing formula, based on the result of WTW and ACD. Myopia patients are planned for standard ICL implantation surgeries by the same surgeon (QH) under similar settings. Preoperatively, in all patients, 0.5% levofloxacin eye drops were used 3 days before the operation, four times daily and topical anesthesia (4% lidocaine) was administered 30 min before the operation. Through a 3.0-mm temporal corneal incision, the ICL was slowly inserted into the anterior chamber following the implantation of hyaluronic acid (ViscAid, Beijing, China), under visualization with OPMI Lumera 700 surgical microscope (Carl Zeiss Meditec, Germany), and the Toric ICL implantation surgery was completed with the help of the Callisto Eye System (Carl Zeiss Meditec, Germany). Any remaining viscosurgical device was washed out of the anterior chamber with the balanced salt solution. Antibiotic eye drops, steroidal eye drops, and artificial tear drops were used postoperatively.
Moreover, each patient’s eye was assessed for uncorrected visual acuity (UCVA), BCVA, intraocular pressure (IOP), and manifest refraction before surgery as well as 1 day, 1 week, 1 month, and 3 months afterward. For statistical analysis, the decimal Snellen evaluation of UCVA and BCVA was converted to the logarithm of the minimum angle of resolution (logMAR). With the aid of a non-contact tonometer (CT-80; Topcon, Tokyo, Japan), the IOP was measured. The central vault of the ICL (distance from the posterior surface of the ICL to the crystalline lens) was measured using OCT 3 months after surgery.
2.2 OCT angiography
After the ocular examination, AngioVue (Optovue, Fremont, CA, United States), was used to capture the OCTA images in all participants from 8:00 a.m. to 12:00 a.m. The system has an A-scan rate of 70 kHz scans per second, with a light source centered on 840 nm and a bandwidth of 45 nm. The scan area was centered on the fovea with a field of view of 6 mm × 6 mm. The resolution of the exported OCT images was 400 × 400 pixels and images with scan quality ≥6 were included for analysis. Automatic segmentation was performed by the software to generate images of the superficial retinal capillary plexus (SCP), and deep retinal capillary plexus (DCP). The SCP was segmented from 3 μm beneath the inner limiting membrane (ILM) to 15 μm beneath the inner plexiform layer (IPL), representing the outer boundary of the ILM to the outer boundary of the IPL. The DCP was segmented from 15 μm beneath the IPL to 70 μm beneath the IPL, representing the outer boundary of the IPL to the outer boundary of the outer plexiform layer (OPL) (Yang et al., 2016).
Due to the elongation of the eye, the magnification for imaging the fundus using fundus photography and OCT differs in the myopic eye (Li et al., 2017). As a result, Bennett’s formula (Figure 1) (Bennett et al., 1994) was used to correct magnification in photographs taken with highly myopic eyes. The correction formula of the image is:
Where t represents the actual scan length, p is the magnification factor determined by the OCTA imaging system, and s represents the original measurement value obtained from the OCTA. The formula of the correction factor q is:
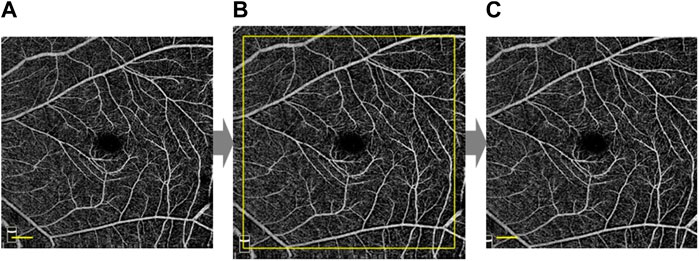
FIGURE 1. Magnification correction of OCTA high myopia image. According to Bennett’s formula, the original image (A) was magnified ×1.10 to obtain the magnified image (B) based on AL = 26.17mm, and then further cropped to the size of the original image (C). Scale bar: 600 μm. (A), (B), and (C) were based on the same OCTA image.
The AL is the axial length as mentioned above. The foveal avascular zone (FAZ) centroid was determined using Matlab (The Mathworks, Inc., Natick, MA, United States) and the image was skeletonized. Since large blood vessels in the deep retinal vascular plexus were considered to be projection artifacts of superficial blood vessels (Zhang M et al., 2016), our custom algorithm separated the vessels with diameters >30 μm in both the superficial and deep layers. The images were then converted to binary images. The partition method for the macular retinal region was shown in Figure 2.
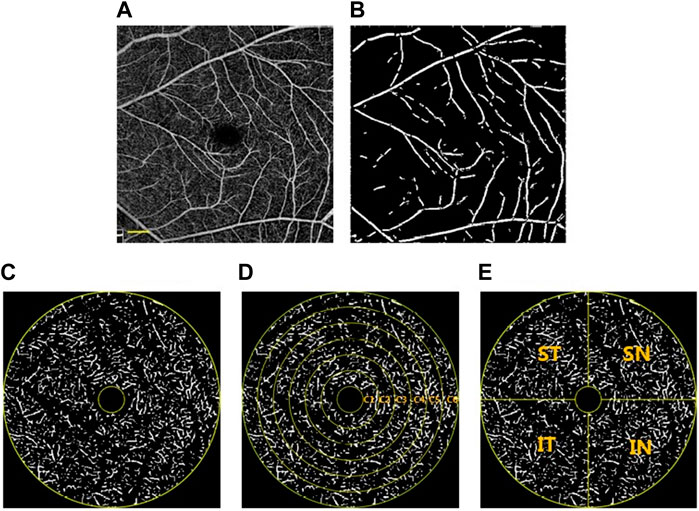
FIGURE 2. Image partitioning and processing methods. (A) OCTA image after magnification correction. The OCTA image was skeletonized and large blood vessels with a diameter> 30 μm were extracted (B) and divided. The annular zone with a diameter of 0.6–5 mm (C) was divided into 6 annuli (C1—C6) for analysis after the removal of the avascular zone (D). In addition, four quadrants centered on the fovea were generated (E). ST, superior temporal; SN, superior nasal; IN, inferior nasal; IT, inferior temporal. Scale bar: 600 μm. (A), (B), (C), (D), and (E) were based on the same OCTA image, which was the same sample as used for Figure 1.
Fractal dimension (FD) analysis was commonly used in the objective quantification of retinal capillary complexity (Ab Hamid et al., 2016). Photoshop was used to crop the image of each area separately, and then the box-counting method with FracLab 2.1 toolbox was used to quantitatively analyze the FD (representing blood vessel density) of each area. FracLab (Paris, France) is designed for digital image analysis and is a plug-in for Matlab.
2.3 Statistical analysis
The data were presented as the mean ± standard deviation (SD). The differences between the means were evaluated using independent sample t-tests (for patients preoperatively and postoperatively) and analyzed using SPSS Statistics 24 (SPSS Inc., Chicago, IL, United States). p < 0.05 was considered significantly different.
3 Result
The demographics of the enrolled subjects are summarized in Table 1. The mean patient age at the time of surgery was 27.0 ± 3.8 years (ranging from 21 to 36 years). The preoperative manifest refraction spherical equivalent (MRSE) was −8.50 ± 2.68 D (ranging from −3.50 D to −13.88 D). The preoperative manifest sphere was −7.95 ± −2.57 D (ranging from −3.00 D to −13.75 D). The preoperative manifest refractive cylinder was −1.16 ± 0.92 D (ranging from 0.00 to −3.25 D). The IOP was 14.60 ± 2.71 mmHg. AL was 26.63 ± 1.06 mm (ranging from 24.87 to 29.50 mm). WTW was 11.90 ± 0.31 mm (ranging from 11.3 mm to 12.7 mm). ACD was 3.26 ± 0.25 mm (ranging from 2.92 mm to 3.80 mm).
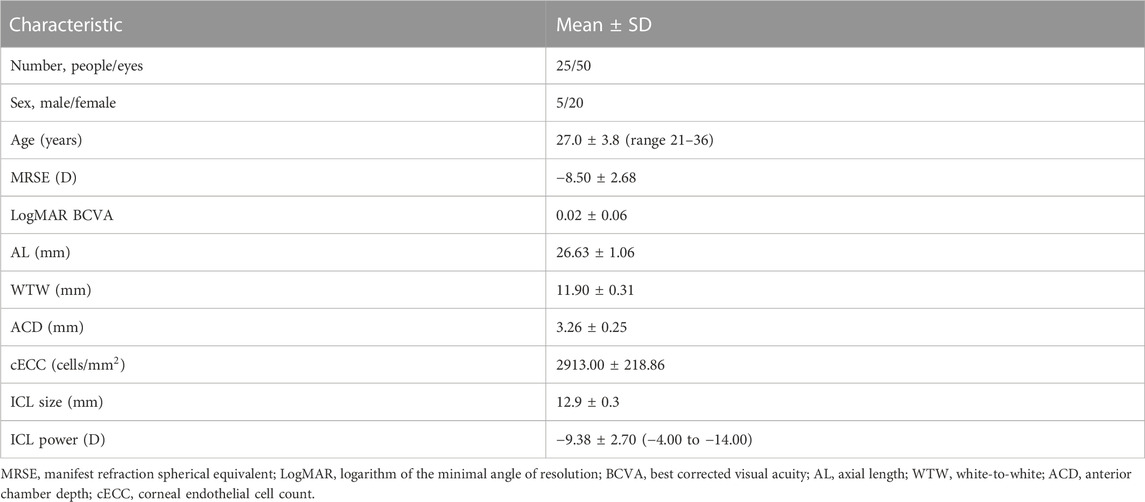
TABLE 1. Preoperative demographics of the myopia patients underwent implantable collamer lens implantation in this study.
All surgical procedures were uneventful, and no postoperative complications, such as cataract formation, pigment dispersion syndrome, pupillary block, or axis rotation, were seen throughout the observation period. Visual acuity improved for all patients on the first day after surgery. Three months postoperatively, 2% of eyes lost one line of vision and 98% of eyes maintained or gained BCVA (Figure 3A). The efficacy index was 1.11 ± 0.24 (preoperative BCVA: 0.01 ± 0.06 logMAR and postoperative UCVA: −0.02 ± 0.06 logMAR, p < 0.05; Figure 3B). The mean preoperative IOP and postoperative IOP (14.6 ± 2.7 versus 15.2 ± 3.2, p > 0.05; Figure 3C) were not significantly different. At 3 months postoperatively, 90% and 100% were within ±0.5 and 1.0 D of the attempted correction, respectively (Figure 3D). The time course changes in the manifest refraction were shown in Figure 3E. Changes in the manifest refraction from 1 day to 3 months were 0.02 ± 0.68 D. Three months postoperatively, the vault was 0.64 ± 0.20 mm.
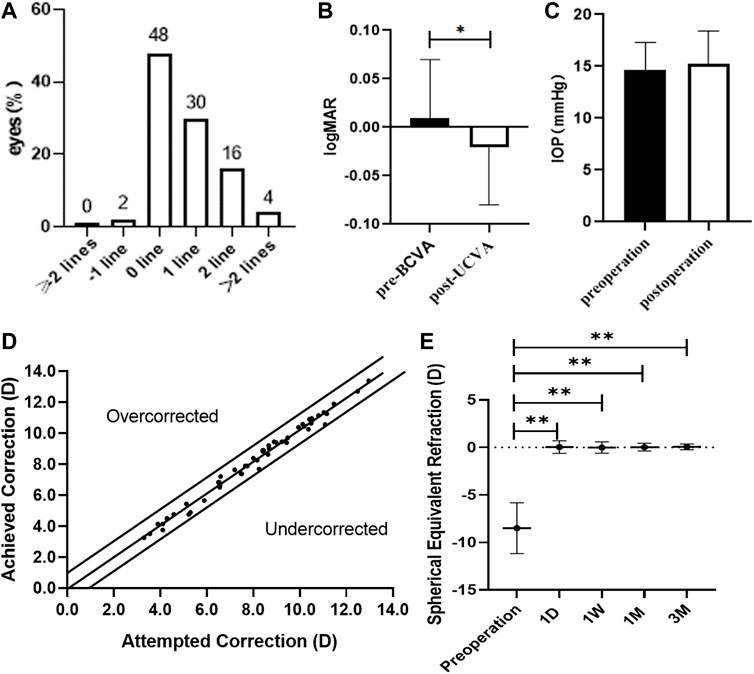
FIGURE 3. Clinical examinations of myopia patients after ICL implantation surgery. (A) Changes in Snellen lines of BCVA at 3 months after ICL implantation. (B) Changes between BCVA 3 months after ICL implantation and UCVA Preoperatively. (C) Changes in intraocular pressure 3 months after ICL implantation. (D) A scatter plot of the attempted versus the achieved manifest spherical equivalent correction 3 months after ICL implantation. (E) Time course of manifest spherical equivalent after ICL implantation. The asterisks indicate statistically significant differences between pre-surgery and post-surgery. D, day; W, week; M, month(s).
Preoperative versus postoperative retinal microvascular density for myopia patients, with p values for comparison. The total annular zone was divided into four quadrantal zones and six annular zones (bandwidth = 0.73 mm). No significant difference in the microvascular density within each annular zone and all quadrantal zones of the superficial and deep layers was found in myopia patients between pre-surgery and post-surgery (Figure 4).
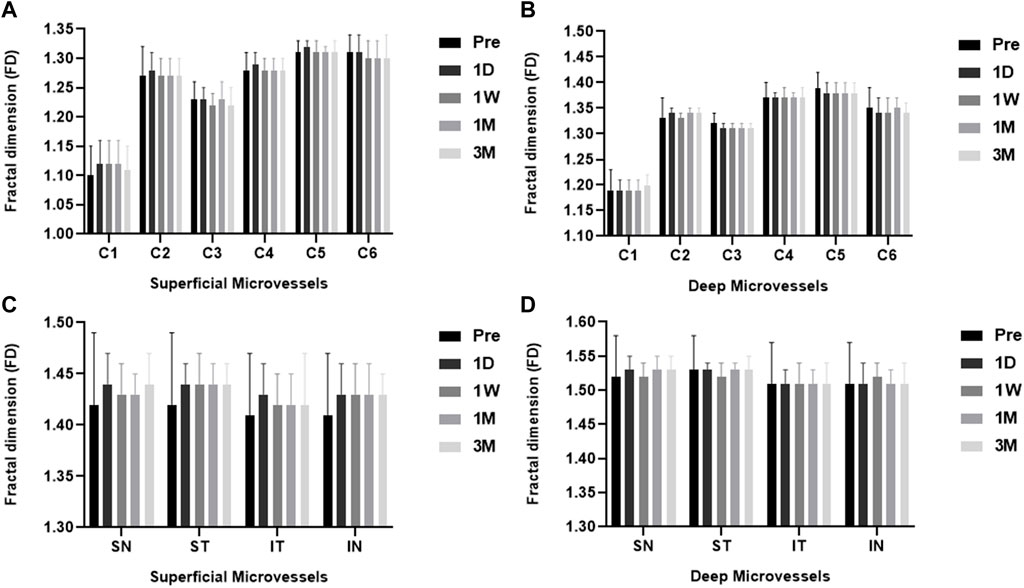
FIGURE 4. The FD (representing retinal microvascular density) of ICL patients pre- and post-surgery for each layer. Among the six individual annular zones (A, B), the microvascular density in the (A) superficial and (B) deep layers showed no significant difference in myopia patients pre- and post-surgery (all p > 0.05). The microvascular density was also not significantly different in all quadrantal zones of the superficial (C) and deep (D) layers in myopia patients pre-and post-surgery. (all p > 0.05). ST, superior temporal; SN, superior nasal; IN, inferior nasal; IT, inferior temporal.
4 Discussion
Myopia is one of the most prevalent eye disorders, and the epidemic of high myopia, in particular, is a serious hazard to public health, such as financial, psychological, quality of life, and direct and indirect risks of blindness. ICL implantation is one of the methods to treat myopia. According to our data, ICL implantation is a safe and effective treatment for both moderate and high myopia. No eye loses two or more lines of vision after ICL implantation. All eyes were within ±1.0 D of the attempted correction and both refractive status and IOP remained stable for 3 months after surgery. Its performance in safety, effectiveness, stability, and predictability is even better than the results of Sanders et al. (2004) due to the advancement of surgical techniques and the update of ICL.
Retinopathy is the most common complication of high myopia, which is a slowly progressive and sight-threatening condition. Several studies have investigated the retinal microvascular in patients with myopia, revealing the retinal microvascular network alterations in myopic eyes. The structural elongation of the eyeball mechanically stretches the retinal tissue, resulting in the straightening and narrowing of the microvessels and consequently the decrease of the retinal microvascular density and perfusion in myopic eyes (Li et al., 2017; Leng et al., 2018; Li et al., 2018; Milani et al., 2018; Gołębiewska et al., 2019; Guo et al., 2019). Jiang et al. (2021) found that the superficial and deep macular microvascular density in high myopia was significantly higher than that in non-high myopia by using OCTA; Liu M et al. (2021) also reached the same conclusion in a larger sample study and found a negative correlation between microvascular density and axial length.
OCT angiography, as an advanced imaging technique characterized by non-invasiveness, quantification, and reliability, which can detect blood flow signals in the retina, has superior advantages over traditional angiography techniques. OCTA is widely used in the evaluation and diagnosis of eye diseases, such as high myopia retinopathy (Grudzińska and Modrzejewska, 2018), glaucoma optic nerve damage (Rao et al., 2020), retinal vein occlusion (Hirano et al., 2021) and diabetic retinopathy (Sun et al., 2021). Using OCTA, images from different layers of the retina can be projected clearly due to its high resolution. In the present study, by using OCT angiography, superficial and deep retinal capillary density were measured in moderate and high myopia patients who underwent ICL implantation between pre-surgery and post-surgery. The OCTA provided the macular perfusion of a 6 mm × 6 mm area, we calculated the microvascular density in each region through the box-counting method after correcting for magnification. Our data showed that ICL implantation surgery would not leave adverse effects on the retinal capillary network.
Moreover, clinical evidence suggests that eye surgery would cause the alteration of retinal microcirculation (Alnawaiseh et al., 2018; Kim et al., 2018; Liu J et al., 2021). Pilotto et al. (2019) found that the perfusion of the retinal microvascular plexus in the deeper layers of the macula increased after uncomplicated cataract surgery, which may be related to the early postoperative local inflammatory response. Analogously, Chen et al. (2017) used a retinal oximeter to detect retinal oxygen desaturation due to retina oxygen deficiency after ICL implantation. ICL implantation surgery as a safe and effective refractive surgery plays an important role in correcting moderate and high myopia. However, due to the unusual anatomy and physiology of the retina in high myopia eyes, it deserves our attention for any microcirculation abnormality inherent to ICL implantation surgery. Using OCTA, ophthalmologists can easily assess patients’ retinal microvascular health and disease, which might be beneficial for pre-operative evaluation of ICL implantation surgery and the detection of postoperative complications.
There were a few limitations to this study. Since the levels of microvascular density in the retinal capillary plexuses after ICL surgery detected by OCTA were stable in this study, the sample size could not be calculated. Although we have included as many participants as possible in this study, the number of patients was relatively small and the follow-up period was only 3 months. In bigger populations and during longer follow-up periods, retinal microvascular change may be seen. Besides, because most of the patients who underwent ICL implantation were young people, this study did not include children and the elderly, whose preoperative retinal microcirculation is slightly different (Leng et al., 2018; Golebiewska et al., 2019), and might respond differently to ICL implantation surgery. Moreover, the OCTA scan area used in this study is 6 mm × 6 mm around the macula. Although it has a wider scan range, it has not achieved the best presentation on retinal microvasculature.
In conclusion, this study demonstrates that ICL implantation is an effective treatment for both moderate and high myopia in young patients, with excellent safety, predictability, and stability. Simultaneously, using OCTA, this research provides evidence that ICL implantation has no adverse effects on retinal microvascular. Further larger sample sizes and longer-term studies are warranted to confirm the conclusions presented herein.
Data availability statement
The raw data supporting the conclusion of this article will be made available by the authors, without undue reservation.
Ethics statement
The studies involving human participants were reviewed and approved by Ethics Committee of Peking University Third Hospital. The patients/participants provided their written informed consent to participate in this study.
Author contributions
The authors confirm contribution to the paper as follows: Study conception and design: CT, YZ, and HQ; data collection: CT, TS, and YL; analysis and interpretation of results: RL and JX; original draft preparation: CT; review and editing: YZ, HQ, and ZS. All authors reviewed the results and approved the final version of the manuscript.
Funding
The study was supported by National Natural Science Foundation of China (82171022, 81974128) and The Capital Health Research and Development of Special (2020-2-4097).
Acknowledgments
We thank all participants for their contribution to the present study.
Conflict of interest
The authors declare that the research was conducted in the absence of any commercial or financial relationships that could be construed as a potential conflict of interest.
Publisher’s note
All claims expressed in this article are solely those of the authors and do not necessarily represent those of their affiliated organizations, or those of the publisher, the editors and the reviewers. Any product that may be evaluated in this article, or claim that may be made by its manufacturer, is not guaranteed or endorsed by the publisher.
References
Ab Hamid, F., Che Azemin, M. Z., Salam, A., Aminuddin, A., Mohd Daud, N., and Zahari, I. (2016). Retinal vasculature fractal dimension measures vessel density. Curr. Eye Res. 41 (6), 823–831. doi:10.3109/02713683.2015.1056375
Al-Sheikh, M., Phasukkijwatana, N., Dolz-Marco, R., Rahimi, M., Iafe, N. A., Freund, K. B., et al. (2017). Quantitative OCT angiography of the retinal microvasculature and the choriocapillaris in myopic eyes. Invest Ophthalmol. Vis. Sci. 58 (4), 2063–2069. doi:10.1167/iovs.16-21289
Alnawaiseh, M., Müller, V., Lahme, L., Merté, R. L., and Eter, N. (2018). Changes in flow density measured using optical coherence tomography angiography after iStent insertion in combination with phacoemulsification in patients with open-angle glaucoma. J. Ophthalmol. 2018, 2890357. doi:10.1155/2018/2890357
Assetto, V., Benedetti, S., and Pesando, P. (1996). Collamer intraocular contact lens to correct high myopia. J. Cataract. Refract Surg. 22 (5), 551–556. doi:10.1016/s0886-3350(96)80007-1
Bennett, A. G., Rudnicka, A. R., and Edgar, D. F. (1994). Improvements on Littmann's method of determining the size of retinal features by fundus photography. Graefes Arch. Clin. Exp. Ophthalmol. 232 (6), 361–367. doi:10.1007/BF00175988
Chen, P., Cai, X., Xu, L., Zhang, J., Yang, Y., Gao, Q., et al. (2017). Assessing oxygen saturation in retinal vessels in high myopia patients pre- and post-implantable collamer lens implantation surgery. Acta Ophthalmol. 95 (6), 576–582. doi:10.1111/aos.13368
de Carlo, T. E., Romano, A., Waheed, N. K., and Duker, J. S. (2015). A review of optical coherence tomography angiography (OCTA). Int. J. Retina Vitr. 1, 5. doi:10.1186/s40942-015-0005-8
Dougherty, P. J., and Priver, T. (2017). Refractive outcomes and safety of the implantable collamer lens in young low-to-moderate myopes. Clin. Ophthalmol. 11, 273–277. doi:10.2147/opth.S120427
Foster, P. J., and Jiang, Y. (2014). Epidemiology of myopia. Eye (Lond) 28 (2), 202–208. doi:10.1038/eye.2013.280
Gao, S. S., Jia, Y., Zhang, M., Su, J. P., Liu, G., Hwang, T. S., et al. (2016). Optical coherence tomography angiography. Invest Ophthalmol. Vis. Sci. 57 (9), Oct27–36. doi:10.1167/iovs.15-19043
Gołębiewska, J., Biała-Gosek, K., Czeszyk, A., and Hautz, W. (2019). Optical coherence tomography angiography of superficial retinal vessel density and foveal avascular zone in myopic children. PLoS One 14 (7), e0219785. doi:10.1371/journal.pone.0219785
Grudzińska, E., and Modrzejewska, M. (2018). Modern diagnostic techniques for the assessment of ocular blood flow in myopia: Current state of knowledge. J. Ophthalmol. 2018, 4694789. doi:10.1155/2018/4694789
Guo, Y., Sung, M. S., and Park, S. W. (2019). Assessment of superficial retinal microvascular density in healthy myopia. Int. Ophthalmol. 39 (8), 1861–1870. doi:10.1007/s10792-018-1014-z
Hirano, Y., Suzuki, N., Tomiyasu, T., Kurobe, R., Yasuda, Y., Esaki, Y., et al. (2021). Multimodal imaging of microvascular abnormalities in retinal vein occlusion. J. Clin. Med. 10 (3), 405. doi:10.3390/jcm10030405
Holden, B. A., Fricke, T. R., Wilson, D. A., Jong, M., Naidoo, K. S., Sankaridurg, P., et al. (2016). Global prevalence of myopia and high myopia and temporal trends from 2000 through 2050. Ophthalmology 123 (5), 1036–1042. doi:10.1016/j.ophtha.2016.01.006
Hopf, S., and Pfeiffer, N. (2017). Epidemiology of myopia. Ophthalmologe 114 (1), 20–23. doi:10.1007/s00347-016-0361-2
Jiang, Y., Lou, S., Li, Y., Chen, Y., and Lu, T. C. (2021). High myopia and macular vascular density: An optical coherence tomography angiography study. BMC Ophthalmol. 21 (1), 407. doi:10.1186/s12886-021-02156-2
Kamiya, K., Igarashi, A., Shimizu, K., Matsumura, K., and Komatsu, M. (2012). Visual performance after posterior chamber phakic intraocular lens implantation and wavefront-guided laser in situ keratomileusis for low to moderate myopia. Am. J. Ophthalmol. 153 (6), 1178–1186. doi:10.1016/j.ajo.2011.12.005
Kamiya, K., Shimizu, K., Igarashi, A., Kitazawa, Y., Kojima, T., Nakamura, T., et al. (2018). Posterior chamber phakic intraocular lens implantation: Comparative, multicentre study in 351 eyes with low-to-moderate or high myopia. Br. J. Ophthalmol. 102 (2), 177–181. doi:10.1136/bjophthalmol-2017-310164
Kim, J. A., Kim, T. W., Lee, E. J., Girard, M. J. A., and Mari, J. M. (2018). Microvascular changes in peripapillary and optic nerve head tissues after trabeculectomy in primary open-angle glaucoma. Invest Ophthalmol. Vis. Sci. 59 (11), 4614–4621. doi:10.1167/iovs.18-25038
Li, M., Yang, Y., Jiang, H., Gregori, G., Roisman, L., Zheng, F., et al. (2017). Retinal microvascular network and microcirculation assessments in high myopia. Am. J. Ophthalmol. 174, 56–67. doi:10.1016/j.ajo.2016.10.018
Leng, Y., Tam, E. K., Falavarjani, K. G., and Tsui, I. (2018). Effect of age and myopia on retinal microvasculature. Ophthalmic Surg. Lasers Imaging Retina 49 (12), 925–931. doi:10.3928/23258160-20181203-03
Li, Y., Miara, H., Ouyang, P., and Jiang, B. (2018). The comparison of regional RNFL and fundus vasculature by OCTA in Chinese myopia population. J. Ophthalmol. 2018, 3490962. doi:10.1155/2018/3490962
Liu, J., Liu, Q., Yu, H., Xia, Y., Zhang, H., Geng, C., et al. (2021). Microvascular changes in macular area after phacoemulsification and its influencing factors assessed by optical coherence tomography angiography. Ther. Clin. Risk Manag. 17, 405–414. doi:10.2147/tcrm.S309679
Liu, M., Wang, P., Hu, X., Zhu, C., Yuan, Y., and Ke, B. (2021). Myopia-related stepwise and quadrant retinal microvascular alteration and its correlation with axial length. Eye (Lond) 35 (8), 2196–2205. doi:10.1038/s41433-020-01225-y
Makita, S., Hong, Y., Yamanari, M., Yatagai, T., and Yasuno, Y. (2006). Optical coherence angiography. Opt. Express 14 (17), 7821–7840. doi:10.1364/oe.14.007821
Milani, P., Montesano, G., Rossetti, L., Bergamini, F., and Pece, A. (2018). Vessel density, retinal thickness, and choriocapillaris vascular flow in myopic eyes on OCT angiography. Graefes Arch. Clin. Exp. Ophthalmol. 256 (8), 1419–1427. doi:10.1007/s00417-018-4012-y
Morgan, I. G., French, A. N., Ashby, R. S., Guo, X., Ding, X., He, M., et al. (2018). The epidemics of myopia: Aetiology and prevention. Prog. Retin Eye Res. 62, 134–149. doi:10.1016/j.preteyeres.2017.09.004
Pilotto, E., Leonardi, F., Stefanon, G., Longhin, E., Torresin, T., Deganello, D., et al. (2019). Early retinal and choroidal OCT and OCT angiography signs of inflammation after uncomplicated cataract surgery. Br. J. Ophthalmol. 103 (7), 1001–1007. doi:10.1136/bjophthalmol-2018-312461
Rao, H. L., Pradhan, Z. S., Suh, M. H., Moghimi, S., Mansouri, K., and Weinreb, R. N. (2020). Optical coherence tomography angiography in glaucoma. J. Glaucoma 29 (4), 312–321. doi:10.1097/ijg.0000000000001463
Sanders, D. R., Doney, K., and Poco, M. (2004). United States food and drug administration clinical trial of the implantable collamer lens (ICL) for moderate to high myopia: Three-year follow-up. Ophthalmology 111 (9), 1683–1692. doi:10.1016/j.ophtha.2004.03.026
Sun, Z., Yang, D., Tang, Z., Ng, D. S., and Cheung, C. Y. (2021). Optical coherence tomography angiography in diabetic retinopathy: An updated review. Eye (Lond) 35 (1), 149–161. doi:10.1038/s41433-020-01233-y
Wang, X., and Zhou, X. (2016). Update on treating high myopia with implantable collamer lenses. Asia Pac J. Ophthalmol. (Phila) 5 (6), 445–449. doi:10.1097/apo.0000000000000235
Wu, P. C., Huang, H. M., Yu, H. J., Fang, P. C., and Chen, C. T. (2016). Epidemiology of myopia. Asia Pac J. Ophthalmol. (Phila) 5 (6), 386–393. doi:10.1097/apo.0000000000000236
Wylegala, A. (2018). Principles of OCTA and applications in clinical neurology. Curr. Neurol. Neurosci. Rep. 18 (12), 96. doi:10.1007/s11910-018-0911-x
Yang, Y., Wang, J., Jiang, H., Yang, X., Feng, L., Hu, L., et al. (2016). Retinal microvasculature alteration in high myopia. Invest Ophthalmol. Vis. Sci. 57 (14), 6020–6030. doi:10.1167/iovs.16-19542
Zhang, M., Hwang, T. S., Campbell, J. P., Bailey, S. T., Wilson, D. J., Huang, D., et al. (2016). Projection-resolved optical coherence tomographic angiography. Biomed. Opt. Express 7 (3), 816–828. doi:10.1364/boe.7.000816
Keywords: implantable collamer lens implantation surgery, myopia, optical coherence tomography angiography, retinal microvasculature, vessel density, visual acuity
Citation: Tang C, Zhang Y, Sun T, Xie J, Liu Y, Liu R, Sun Z and Qi H (2023) Prospective clinical study of retinal microvascular alteration after ICL implantation. Front. Cell Dev. Biol. 11:1115822. doi: 10.3389/fcell.2023.1115822
Received: 04 December 2022; Accepted: 10 January 2023;
Published: 19 January 2023.
Edited by:
Yanwu Xu, Baidu, ChinaReviewed by:
Heng Li, Southern University of Science and Technology, ChinaGuoying Mu, Shandong Provincial Hospital affiliated to Shandong First Medical University, China
Yi Shao, Nanchang University, China
Copyright © 2023 Tang, Zhang, Sun, Xie, Liu, Liu, Sun and Qi. This is an open-access article distributed under the terms of the Creative Commons Attribution License (CC BY). The use, distribution or reproduction in other forums is permitted, provided the original author(s) and the copyright owner(s) are credited and that the original publication in this journal is cited, in accordance with accepted academic practice. No use, distribution or reproduction is permitted which does not comply with these terms.
*Correspondence: Hong Qi, ZG9jdG9ycWlob25nQGhvdG1haWwuY29t
†These authors have contributed equally to this work and share first authorship