- 1Department of Obstetrics and Gynecology, West China Second University Hospital, Sichuan University, Chengdu, China
- 2West China School of Medicine, Sichuan University, Chengdu, China
- 3Key Laboratory of Birth Defects and Related Diseases of Women and Children (Sichuan University), Ministry of Education, West China Second University Hospital, Sichuan University, Chengdu, China
Ferroptosis is a form of regulated cell death characterized by iron overload, overwhelming lipid peroxidation, and disruption of antioxidant systems. Emerging evidence suggests that ferroptosis is associated with pregnancy related diseases, such as spontaneous abortion, pre-eclampsia, gestational diabetes mellitus, intrahepatic cholestasis of pregnancy, and spontaneous preterm birth. According to these findings, inhibiting ferroptosis might be a potential option to treat pregnancy related diseases. This review summarizes the mechanisms and advances of ferroptosis, the pathogenic role of ferroptosis in pregnancy related diseases and the potential medicines for its treatment.
1 Introduction
Cells are the fundamental organizing unit of life. Cell death, is therefore of critical importance in diverse aspects of mammalian development and homeostasis. Ferroptosis is a form of regulated cell death, coined in 2012. Generally, it is mainly characterized by iron overload, overwhelming lipid peroxidation, and disruption of antioxidant systems, particularly depletion of glutathione peroxidase 4 (GPX4) (Long et al., 2022). Mounting evidence suggests that ferroptosis plays an important role in cancer (Lei et al., 2022), neurodegenerative diseases (Ryan et al., 2022), and ischemia/reperfusion injury, such as acute kidney injury (Fan et al., 2022), acute myocardial infarction (Miyamoto et al., 2022), and hepatic ischemia-reperfusion injury (Ye et al., 2022a), and autoimmune disease, like psoriasis (Zhou et al., 2022) and rheumatoid arthritis (Long et al., 2022), Serving as the maternal-fetal interface, placenta plays a central role in maternal and fetal health during pregnancy. Placenta insufficiency, however, is tightly associated with pregnancy related diseases (PRDs), such as pre-eclampsia, gestational diabetes mellitus (GDM), and intrahepatic cholestasis of pregnancy (ICP) (Cindrova-Davies and Sferruzzi-Perri, 2022). Recently, there has been a growing appreciation for the importance of ferroptosis in PRDs. In this review, we summarized the molecular mechanisms of ferroptosis, as well as its pathogenic role and potential medicines in PRDs (Figure 1; Figure 2).
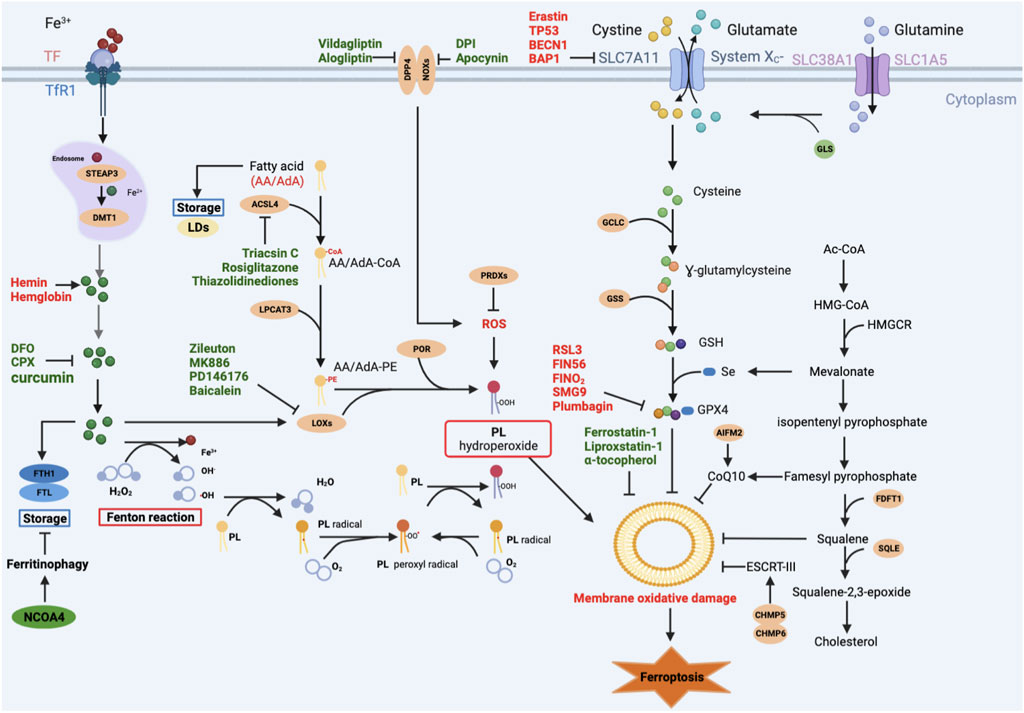
FIGURE 1. Main signaling pathways of ferroptosis. Ferroptosis can occur through three major pathways: 1) Iron overload: Fe2+ may directly generate excessive lipid reactive oxygen species (ROS) through the Fenton reaction, or Fe2+ acts as a cofactor of lipoxygenase (LOX) or prolyl hydroxylase, leading to lipid peroxidation and oxygen homeostasis. 2) Lipid peroxidation: ACSL4 catalyzes the ligation of CoA into free AA/AdA to form AA/AdA-CoA. AA/AdA-CoA are esterified into PE by LPCAT3 to form AA/AdA-PE. PE-AA/AdA-OOH are produced by the peroxidation of the AA/AdA-PE through non-enzymatically autoxidation (Fenton reaction) or enzyme-mediated pathways (LOXs). 3) Antioxidant systems: the SLC7A11-GSH-GPX4 axis, CoQ10 system, and other antioxidants like AIFM2, squalene. Red-colored: ferroptosis inducers; Green-colored: ferroptosis inhibitors.
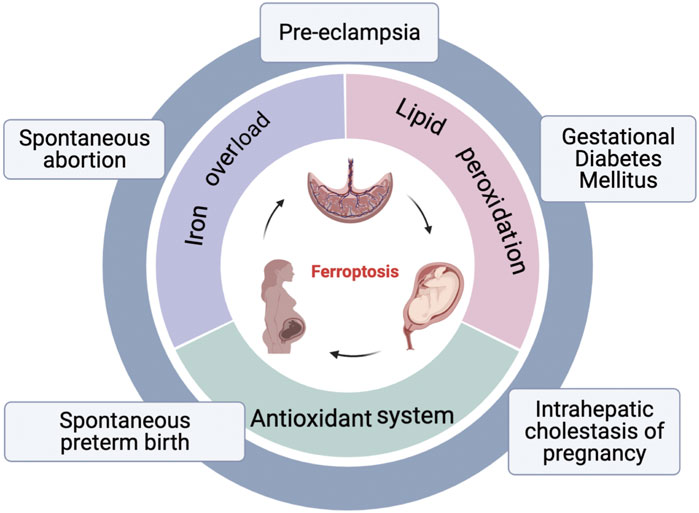
FIGURE 2. An overview of ferroptosis and pregnancy related diseases. Three hallmarks of ferroptosis: lipid peroxidation, iron overload, and disorder of antioxidant systems; Pregnancy related diseases associated with ferroptosis: spontaneous abortion, pre-eclampsia, gestational diabetes mellitus, intrahepatic cholestasis of pregnancy, spontaneous preterm birth.
2 Molecular mechanisms of ferroptosis
2.1 Lipid peroxidation
Mammalian lipid bilayers consist of up to 62% of unsaturated fatty acids of which 35% are polyunsaturated fatty acids (PUFAs) (Hulbert et al., 2002). However, PUFA is a double-edged sword. On the one hand, PUFAs are necessary for cell membrane to maintain its fluidity (Gill and Valivety, 1997) or deposit in lipid droplets (LDs) in order to produce metabolic energy in case of insufficient energy sources (Thiele and Spandl, 2008). On the other hand, PUFAs, especially arachidonic acid (AA) and adrenic acid (AdA), promote lipid peroxidation under various pathophysiological contexts (Doll et al., 2017). Acyl-CoA synthetase long chain family member 4 (ACSL4) (Küch et al., 2014) and lysophosphatidylcholine acyltransferase 3 (LPCAT3) (Hishikawa et al., 2008) are tightly linked to lipid peroxidation of AA/AdA. Firstly, ACSL4 catalyzes the ligation of CoA into free AA/AdA to form AA/AdA-CoA derivatives (Kagan et al., 2017). Then, AA/AdA-CoA are esterified into phosphatidylethanolamine (PE) by LPCAT3 to form arachidonic acid-phosphatidylethanolamines (AA/AdA-PE) (Hishikawa et al., 2008). Finally, toxic phospholipid hydroperoxides (PE-AA/AdA-OOH) are produced by the peroxidation of the AA/AdA-PE through non-enzymatically autoxidation or enzyme-mediated pathways (Dixon et al., 2012; Yang et al., 2016).
The non-enzymatic phospholipid (PL) autoxidation is iron-dependent lipid peroxidation. Hydroxyl radicals, produced by the interaction of Fe2+ and H2O2 (Fenton reaction), subtract hydrogen from lipid to form lipid radicals (L•) (Diggle, 2002). After that, the lipid radical combine with O2 to form a lipid peroxyl radical (LOO•), which then interacts with adjacent PUFAs to form lipid hydroperoxide (LOOH), and many electrophilic species such as malondialdehyde (MDA), and 4-hydroxynonenal (4HNE) (Rice-Evans and Burdon, 1993; Michalski et al., 2008).
Lipid peroxidation also occurs in enzyme-mediated processes. Lipoxygenases (LOXs), a dioxygenase containing non-heme iron, has six isoforms in humans: 15-LOX-1, 15-LOX-2, 12-LOX-1, 12-LOX-2, E3-LOX, and 5-LOX (Funk et al., 2002). Although the key role of LOXs in ferroptosis is still controversial, certain LOXs can catalyze the stereospecific addition of oxygen onto PUFAs (Kuhn et al., 2005), indicating LOXs may mediate ferroptosis. Indeed, LOX15, binding to the partner phosphatidylethanolamine-binding protein 1 (PEBP1), is of importance for erastin- or RSL3-induced ferroptosis (Wenzel et al., 2017). Furthermore, ferroptosis can be inhibited by some LOXs inhibitors, such as Zileuton, MK886, PD146176, Baicalein (Battista et al., 2012; Shah et al., 2018). However, some reported in vivo model of acute renal failure, 12/15- LOX deletion cannot eliminate the cell death of GPX4 knockout mouse (Friedmann Angeli et al., 2014; Brütsch et al., 2015). Therefore, the role of LOXs in ferroptosis should be further investigated. Other oxygenases, such as NADPH oxidases (NOXs) and cytochrome P450 oxidoreductase (POR), may also lead to ferroptosis. Apocynin and diphenyleneiodonium (DPI), two NOXs inhibitors, can directly mitigate ferroptotic cell death (Hou et al., 2019). Similarly, alogliptin and vildagliptin indirectly suppress NOXs activity mediated by dipeptidyl peptidase-4 (DPP-4), reducing lipid peroxidation (Zhang et al., 2022a). POR, identified through CRISPR/Cas9-mediated suppressor screening, can play a role in erastin-, FIN56-, ML210-, or Ras selective lethal small molecule 3 (RSL3)-induced ferroptosis, as well (Zou et al., 2020).
2.2 Iron in ferroptosis
Iron overload is a hallmark of ferroptosis. Iron drives ferroptosis mainly by two ways. Iron may directly generate excessive lipid reactive oxygen species (ROS) through the Fenton reaction (Conrad and Pratt, 2019). What’s more, Fe2+ acts as a cofactor of LOXs or prolyl hydroxylase (Doll and Conrad, 2017), which are enzymes responsible for lipid peroxidation and oxygen homeostasis (Kagan et al., 2017). Consequently, Fe2+ promotes the production of lipid ROS and contributes to ferroptosis indirectly. Therefore, iron metabolism, including iron uptake, transportation, utilization, may affect cell susceptibility to ferroptosis. Firstly, Fe3+ imports by binding to transferrin (TF), which can be recognized by transferrin receptor-1 (TfR1) in the cell membrane. And then, Fe3+ endocytosis in endosomes, where it is reduced to Fe2+ by six-transmembrane epithelial antigens of the prostate 3 (STEAP3). Finally, Fe2+ is transported to the cytosolic labile iron pool via divalent metal transporter 1 (DMT1) (El Hout et al., 2018). Fe2+ also comes from hemin and hemoglobin via the lysis of red blood cells, leading to ferroptosis (Kwon et al., 2015). It can be used in cellular processes or stored into ferritin, consisting of ferritin light chain (FTL) and ferritin heavy chain 1 (FTH1) (Ryu et al., 2017). But ferritin can be degraded by lysosomes through nuclear receptor coactivator 4 (NCOA4)-mediated ferritinophagy (Hou et al., 2016). Ferroportin (FPN1) is responsible for exporting iron (Donovan et al., 2000), resisting to ferroptosis.
2.3 Antioxidant systems
2.3.1 The SLC7A11-GSH-GPX4 axis
The SLC7A11-GSH-GPX4 axis is a classical signaling pathway of ferroptosis (Dixon et al., 2012). Glutathione peroxidase 4 (GPX4), a glutathione (GSH) -dependent selenoenzyme, functions as a phospholipid hydroperoxidase to reduce toxic PE-AA/AdA-OOH to the corresponding non-toxic phospholipid alcohol (PLOH), inhibiting ferroptosis (Ursini et al., 1985). However, the antioxidant activity of GPX4 demands the catalytic selenocysteine (Sec) residue at 46 (U46) and two electrons supplied mainly by GSH (Maiorino et al., 2018). Sec, encoded by the UGA codon, is a major form of selenium (Se) in the cell. Generally, it is present at active sites of enzymes, catalyzing redox reactions, thereby eliminating hydroperoxides (Santesmasses et al., 2020). In addition, Se can upregulate the expression of GPX4 through transcription factor AP-2 gamma (TFAP2C) and specificity protein 1 (SP1) (Alim et al., 2019). Truly, translation of GPX4 is attenuated in LRP8KO cells due to the limiting Se (Li et al., 2022a). GSH, as the reducing agent, is the substrate for the lipid repair function of GPX4, lowering the risk of ferroptosis. The biosynthesis of GSH is based on glutamate, glycine and cysteine, which is tightly correlated with its precursor cystine and system Xc− (Lu, 2013). The Xc− system is an antiporter on the cell membrane composed of SLC7A11 and SLC3A2, transporting glutamate outwards and cystine inwards at 1:1 ratio (Bannai, 1986). Thus, factors, directly or indirectly inhibiting GPX4, play a key role in inducing ferroptosis. Erastin inhibits its activity by binding to SLC7A11, reducing cystine import, thereby reducing GSH synthesis (Yang et al., 2014). Tumor suppressor genes TP53, BECN1, BAP1 downregulate the expression of SLC7A11 to induce ferroptosis (Jiang et al., 2015; Song et al., 2018; Zhang et al., 2018). However, the nuclear transcription factor 2 (Nrf2) upregulates the expression of SLC7A11 to inhibit ferroptosis (Chen et al., 2017). High calcium and phosphate can downregulate the expression of GPX4, inducing ferroptosis (Ye et al., 2022b). RSL3 can covalently bind to GPX4, resulting in increasing lipid peroxidation (Yang et al., 2014). GPX4 also can be degraded by some compounds, such as FIN56, FINO2, plumbagin, SMG9 (Gaschler et al., 2018; Han et al., 2021; Sun et al., 2021; Zhan et al., 2022). Together, the SLC7A11-GSH-GPX4 axis is of great significance for ferroptosis.
2.3.2 The FSP1 pathway
The ferroptosis suppressor protein 1 (FSP1)–NAD(P)H–coenzyme Q10 (CoQ10) pathway, acting in parallel to the SLC7A11-GSH-GPX4 pathway, is a potent suppressor of lipid peroxidation and ferroptosis (Bersuker et al., 2019; Doll et al., 2019). FSP1, known as apoptosis-inducing factor mitochondrial 2 (AIFM2), plays a crucial role in the non-mitochondrial CoQ antioxidant system (Wu et al., 2002). As members of the AIF family, FSP1 contains a short N-terminal hydrophobic sequence and a canonical flavin adenine dinucleotide (FAD)-dependent oxidoreductase domain, possessing NADH: ubiquinone oxidoreductase activity (Elguindy and Nakamaru-Ogiso, 2015). Ubiquinol, the reduced form of ubiquinone, known as CoQ10 traps lipid peroxyl radicals, thereby mediating lipid peroxidation. However, FSP1 catalyzes the catalyzing NADH: ubiquinone oxidoreductase reactions, reducing ubiquinol to CoQ10, which is a good radical-trapping antioxidant for lipid peroxides (Bersuker et al., 2019; Doll et al., 2019). Indeed, some reported that FSP1 inhibits ferroptosis in across hundreds of cancer cell lines and in mouse tumor models (Bersuker et al., 2019).
3 Ferroptosis inhibitors
Ferroptosis is associated with a great number of diseases. Multiple genes and signaling pathways, associated with lipid and iron metabolism, and antioxidant systems, play a role in inhibiting ferroptosis, providing new potential therapeutic drugs for these diseases (Table 1).
3.1 Inhibition of lipid peroxidation
PUFAs, substrates of lipid peroxidation, are responsible for ferroptosis. Selectively bis-allylic deuterated PUFA, suppressing ferroptosis induced by RSL3 and erastin (Yang et al., 2016), is a promising therapeutic strategy against ferroptosis. Additionally, blocking the process of PUFAs incorporation into phospholipid membranes can reduce lipid peroxidation, like thiazolidinediones, rosiglitazone and Triacsin C (Van Horn et al., 2005; Angeli et al., 2017; Doll et al., 2017), which inhibits ACSL4. There are many LOXs inhibitors, such as vitamin E, α-Tocopherol, baicalein, PD-146176 (Probst et al., 2017; Walters et al., 2018; Kenny et al., 2019; Hu et al., 2021a; Zhang et al., 2022b), stopping the process of LOX-mediated lipid peroxidation, thereby resisting ferroptosis. Ferrostatins and liproxstatins are classical ferroptosis inhibitor, depressing lipid peroxidation (Zilka et al., 2017).
3.2 Iron chelator
Fe2+ overload in intracellular iron pools, like endoplasmic reticulum (ER), may trigger ferroptosis (Tang et al., 2021). However, intracellular iron accumulation is linked with the transport of extracellular iron. Recently, Li et al. found eriodictyol can significantly decrease TfR1 and FTH, and increase FPN, leading to resisting ferroptosis (Li et al., 2022b). Furthermore, iron chelators, such as deferoxamine (DFO), ciclopirox (CPX), and curcumin (Rainey et al., 2019) can reduce the concentration of Fe2+ (Yang and Stockwell, 2008), suppressing ferroptosis.
3.3 Antioxidant systems
Antioxidant systems protecting cell from oxidative damage in ferroptosis are associated with multiple enzymes and proteins, including SLC7A11-GSH-GPX4 axis, CoQ10 system (Kuang et al., 2020).
3.3.1 The SLC7A11-GSH-GPX4 axis
The SLC7A11-GSH-GPX4 axis is the main ferroptosis prevention system. The induction of GPX4 synthesis is a classic pathway to suppress ferroptosis, relating to cysteine, system Xc-, Se, GSH. The β-mercaptoethanol may play a role in inhibiting ferroptosis by driving a highly efficient cystine/cysteine redox cycle (Sha et al., 2015). Cycloheximide is a potent inhibitor of ferroptosis, by increasing the concentration of GSH (Rashad et al., 2022). The XJB-5-131 alleviates I/R-induced renal injury and inflammation in mice by increasing the expression of GPX4 (Zhao et al., 2020). Similarly, 1,25(OH)2D3, astaxanthin, and echinatin can become resistant to ferroptosis by increasing GPX4 level (Cheng et al., 2021; Wang et al., 2022; Xu et al., 2022). At the same time, the 5-(tetradecyloxy)-2-furoic Acid (TOFA) was also found to be a potent suppressor of ferroptosis, through inhibiting the loss of GPX4 (Shimada et al., 2016). Quercetin, a natural polyphenol, could attenuate seizure-induced neuron ferroptosis in vivo and in vitro at via the SIRT1/Nrf2/SLC7A11/GPX4 axis (Xie et al., 2022).
3.3.2 CoQ10 System
The FSP1–NAD(P)H–CoQ10 pathway, acting in parallel to the GPX4 axis, is a powerful antioxidant system in membrane structures (Teran et al., 2018). Idebenone, an analog of CoQ10, prevents ferroptosis caused by FIN56 or RSL3 (Shimada et al., 2016). Likewise, farnesyl pyrophosphate, an upstream product of CoQ10 synthesis, suppresses FIN56-induced ferroptosis (Shimada et al., 2016).
3.3.3 Other antioxidants
AIFM2, promoting CHMP5- and CHMP6-mediated ESCRT-III membrane repair, results in blocking ferroptosis (Dai et al., 2020). As reported, some antioxidant proteins may also resist to ferroptotic cell death, like peroxiredoxins (Qi et al., 2019; Lovatt et al., 2020), thioredoxin (Llabani et al., 2019). Cytosolic Ca2+ overload was a key mediator of ferroptosis (Chen et al., 2020; Liu et al., 2022). Recently, researchers found BAPTA-AM, an intracellular Ca2+ chelator, could rescued ferroptosis in HK-2 cells (Liu et al., 2022). Therefore, limiting oxidative damage of ferroptosis has been a promising therapeutic strategy for PRDs.
4 Role of ferroptosis in pregnancy related diseases
Recently, basic research on ferroptosis in PRDs has gradually increased. Studies have indicated that placenta is susceptible to ferroptosis. Primarily, lipid peroxidation is frequent in placental injury (Schoots et al., 2018); Secondly, trophoblasts are abundant of iron: syncytiotrophoblasts extraordinarily highly expressed TfR1 (Seligman et al., 1979). Furthermore, Zrt- and Irt-like protein 8 (ZIP8) and Zrt- and Irt-like protein 14 (ZIP14), both of which play a roles in exporting iron from placental endosomal into the cytosol, are found at high levels in human placenta (Jenkitkasemwong et al., 2012). Three reviews described details on the role of iron and ferroptosis in the placenta (Ng et al., 2019; Beharier et al., 2021; Zaugg et al., 2022). Finally, decreased GPX4 levels have been associated with human placental dysfunction (Zhang et al., 2020). Therefore, fully understanding the role of ferroptosis in placenta dysfunction may provide new treatment options for PRDs, including spontaneous abortion, PE, GDM, ICP, and spontaneous preterm birth (Figure 2).
4.1 Pre-eclampsia
4.1.1 Lipid peroxidation and pre-eclampsia
PE plays a leading role in maternal morbidity and mortality (Leitao et al., 2022). Ferroptosis has been related to the pathogenesis of PE (Chen et al., 2022). There are mounting evidence suggesting lipid peroxidation, is a major contributor for the damage of PE. A single-cell transcriptomics of the human placenta analysis indicated LPCAT3 and Sat1 (spermidine/spermine N1-acetyltransferase 1) highly expressed in trophoblasts (Ng et al., 2019), both of which are related to ferroptosis (Dixon et al., 2015; Ou et al., 2016). Irwinda, R., et al. reported that the level of PUFAs significantly increased in PE patients (Irwinda et al., 2021; Liao et al., 2022). Furthermore, in rats model of PE, the concentration of MDA, the end product of lipid peroxidation, in the placenta has increased dramatically (Zhang et al., 2020). Similarly, the levels of MDA in plasma and placenta are significantly elevated in PE patients (Aydin et al., 2004). To summarize, these studies suggest that lipid peroxidation leading to ferroptosis could contribute to PE.
4.1.2 Iron and pre-eclampsia
Iron overload is also associated with PE. Researchers have confirmed the concentration of plasma iron is higher in PE pregnancy than that in normal pregnancy (Liu et al., 2019). Yang et al., 2022a. reported the differentially expressed ferroptosis-related genes (FRGs) in early-onset PE were mainly enriched in iron-related pathways, including FTH1, FTL. Importantly, iron is abundant in trophoblasts under physiological conditions or in the context of iron deficiency (Sangkhae et al., 2020). What’s more, the expression of FPN1 of trophoblasts decreased under hypoxic conditions (Zhang et al., 2020), leading to the intracellular accumulation of Fe2+. Consequently, trophoblasts are vulnerable to ferroptosis. Ferrostatin-1 (Fer-1), a ferroptosis inhibitor, decreased the mortality rate of trophoblasts (Beharier et al., 2020). Similarly, the ferroptosis inhibitor improved the PE symptoms in a rat model, with the reduction of MDA (Zhang et al., 2020). Thus, reducing the concentration of Fe2+ might be a good way for PE treatment.
4.1.3 The SLC7A11-GSH-GPX4 axis and pre-eclampsia
Disorder of antioxidant system mediates ferroptosis in PE. A microarray analysis identified that miRNA-30b-5pm, which is in charge of reducing the expression of SLC7A11, upregulated in PE placental tissues. Also, they found SLC7A11 and GPX4 were decreased in PE placental tissues via GSE10588 data set (Zhang et al., 2020). In accordance with other studies, the levels of SLC7A11, GSH and GPX4 declined while MDA levels were significantly increased (El-Khalik et al., 2022; D'Souza et al., 2016), indicating ferroptosis is involved in the pathogenesis of PE through the SLC7A11-GSH-GPX4 axis. A genome-wide methylome analysis found the expression of ATF3, suppressing the system Xc− by binding to the SLC7A11 promoter (Wang et al., 2020), is higher in PE placenta than the normal placenta (Ching et al., 2014). As a result, human trophoblasts are susceptible to ferroptosis by the depletion or inhibition of GPX4 (Kajiwara et al., 2022). Additionally, pannexin 1 (Panx1) and toll-like receptor 4 (TLR4), which had a negative correlation with SLC7A11, are demonstrated to induce ferroptosis in PE (El-Khalik et al., 2022). Conversely, anti-ferroptosis factors can protect trophoblasts against ferroptosis through the SLC7A11-GSH-GPX4 axis. The level of Nrf2, which is responsible for promoting transcriptions of SLC7A11 and GPX4 (Dong et al., 2020), is lower in PE rats (Ju et al., 2022). DJ-1 plays a protective role in the process of ferroptosis in PE via the Nrf2/GPX4 signaling pathway (Liao et al., 2022). These studies demonstrated that the SLC7A11-GSH-GPX4 axis plays a role in the pathogenesis of PE.
4.2 Gestational diabetes mellitus
4.2.1 Lipid peroxidation and gestational diabetes mellitus
Gestational diabetes mellitus (GDM) is common during pregnancy and is increasing in prevalence globally (Sweeting et al., 2022). The incidence of GDM ranges from 6.6% to 45.3% of pregnancies (Brown and Wyckoff, 2017) and one in six live births worldwide were complicated by GDM (Atlas, 2015). GDM is associated with long-lasting complications in the short and long term, such as macrosomia (Song et al., 2022), dystocia (Crowther et al., 2022), childhood obesity in the child (Choi et al., 2022), recurrence of GDM (Giuliani et al., 2022), developing type 2 diabetes (Vounzoulaki et al., 2020) and cardiovascular disease in the mother (Christensen et al., 2022). Emerging evidence suggests ferroptosis contributes to the pathogenesis of GDM (Han et al., 2020; Gautam et al., 2021; Zhang et al., 2022c; Hu et al., 2022; Zaugg et al., 2022). The insulin sensitivity shifts depending on the requirements of pregnancy, which is an important metabolic adaptation during healthy pregnancy (Di Cianni et al., 2003). However, excessive insulin resistance in GDM promotes endogenous glucose production and the breakdown of fat stores, increasing the levels of blood glucose and free fatty acid (FFA) (Phelps et al., 1981). Indeed, glucose metabolism disorder is often accompanied by lipid metabolism disorder in GDM (Parhofer, 2015). A study indicated that women with GDM had significantly higher triglyceride (TG) concentrations (Hu et al., 2021b). In vitro model, the death rate of trophoblasts significantly increased after the co-treatment of high lipid (HL) and high glucose (HG). Furthermore, it was found that HL and HG can induce GDM in pregnant rats, leading to the damage of rats’ placenta (He et al., 2021). The expression of ACSL4 significantly increased in placental tissues, as well (Zheng et al., 2022). Consequently, excessive FFA of GDM may cause an increase in the level of lipid peroxidation, resulting in ferroptosis.
4.2.2 Iron and gestational diabetes mellitus
Iron overload, leading to oxidative stress damage, could promote the pathogenesis of GDM (Gautam et al., 2021; Zhang et al., 2022c; Zaugg et al., 2022). As reported, both elevated plasma ferritin concentrations and iron supplementation in pregnant women having adequate iron stores are risk factors of GDM (Zhang et al., 2021). In GDM vivo model, the levels of iron deposition significantly increased (Zheng et al., 2022), inducing the production of ROS via the Fenton reaction. As a result, oxidative damage leads to the injury and ferroptosis of pancreatic β–cell in GDM (Gautam et al., 2021; Du et al., 2022). The SLC7A11-GSH-GPX4 axis also contributes to GDM. The serum lipid peroxidation was higher, while the serum GPX4 concentration was lower in GDM women (Mauri et al., 2021). In summary, mounting evidence may suggest that excessive iron, and reduced GPX4 levels, two hallmarks of ferroptosis, are associated with GDM. However, experiments testing this hypothesis are still lacking.
4.3 Intrahepatic cholestasis of pregnancy
Intrahepatic cholestasis of pregnancy (ICP) is a complication, most occurs in the third trimester, in 0.3%–15% of pregnancies in various populations (Wikström Shemer et al., 2013). It is characterized by pruritus, elevated serum bile acid levels and liver transaminases, leading to meconium-stained amniotic fluid, fetal distress, preterm birth, and stillbirth (Wikström Shemer et al., 2013). There is increasing evidence that oxidative stress induced by bile acids leads to the pathogenesis of ICP (Sanhal et al., 2018). ICP patients had significantly lower levels of Se and GPX4 than normal pregnancies (Reyes et al., 2000; Hu et al., 2015). Moreover, patients with ICP had significantly higher level of MDA (Zhu et al., 2019). Analysis of differentially expressed ferroptosis-related genes in ICP and healthy pregnant showed EGFR, mediating ferroptosis, was higher upregulated in human placenta (Fang and Fang, 2022). Modification of oxidative stress caused by ferroptosis might be a treatment target for ICP. Further research, particularly in vivo and in vitro experiments, is needed to characterize the association between ferroptosis and ICP.
4.4 Other pregnancy-related disease
Excessive ferroptosis occurred in spontaneous abortion rat model with low levels of GSH, GPX4 and increased levels of TFR1, ACSL4 and MDA (Meihe et al., 2021). Some evidence also indicated spontaneous preterm birth is related to ferroptosis (Beharier et al., 2020). But few studies reported that the exact mechanism of ferroptosis and spontaneous abortion and spontaneous preterm birth are still unclear.
5 Potential medicines for ferroptosis in pregnancy related diseases
Trophoblast ferroptosis may provide a useful therapeutic target for pregnancy-related diseases. Quercetin, as an antioxidant, can significantly promote trophoblast invasion during early pregnancy via significantly increasing GSH levels (Ebegboni et al., 2019). Additionally, quercetin has positive effects on pre-eclampsia rats induced by L-NAME (Yang et al., 2019a; Yang et al., 2022b). Iron chelators, deferoxamine and ferrostatin-1, were indicated to decrease the concentration of placenta MDA in the PE rat mode, thereby blocking trophoblast ferroptosis (Beharier et al., 2020; Zhang et al., 2020). Similarly, vitamin E plays a role in the preventing PE by mitigating lipid peroxidation in placenta (Raijmakers et al., 2004). Thiazolidinediones, inhibiting ACSL4 against ferroptosis, is also oral antidiabetic drug by sensitizing tissue to the effects of insulin (Pollex and Hutson, 2011). A recently published case series demonstrate thiazolidinediones is safe during pregnancy (Haddad et al., 2008), indicating its potential therapeutic role for GDM. Trophoblasts ferroptosis may contribute to ICP, while the low concentration of Se is related to the pathogenesis of ICP (Reyes et al., 2000). Thus, Se, upregulating the expression of GPX4, can protect placental trophoblasts against oxidative stress, particularly ICP (Habibi et al., 2021). CoQ10 is significantly decreased in patients with ICP (Martinefski et al., 2014). Furthermore, CoQ10 supplementation improves estradiol-induced cholestasis in rats. CoQ10 supplementation is very well tolerated and has no clinically relevant toxic side effects in humans (Hidaka et al., 2008; Martinefski et al., 2020). Therefore, it would be an alternative therapy for women with ICP. Lack of 1,25(OH)2D3 is related to PRDs, which may result from ferroptosis, such as spontaneous abortion, GDM and PE (Bespalova et al., 2019; Li et al., 2019; de Souza and Pisani, 2020). Vitamin D elevated the level of GSH, GPX4 and reduced MDA through activation of the Nrf2/HO-1 pathway to suppresses ferroptosis. Therefore, vitamin D supplementation may be a strategy to improve PRDs. Previous studies reported astaxanthin significantly reduced the content of MDA in preeclamptic rats and trophoblast cell line (Xuan et al., 2014; Xuan et al., 2016; Fu et al., 2021). Certainly, drug efficacy and safety are quite important for pregnant woman and fetus. Further research may shed light on potential targeting drugs for ferroptosis in PRDs.
6 Conclusions and perspectives
Ferroptosis is a form of regulated cell death involving lipid metabolism, iron metabolism and antioxidant system, regulated by multiple genes and signaling pathways. PRDs are mainly associated with placenta dysfunction due to trophoblasts injury and death. Recently, an increasing number of experimental studies are exploring role of ferroptosis in PRDs in order to provide new potential therapeutic drugs and therapeutic targets for it. However, there are numerous problems that have not been elucidated on the association between ferroptosis and pregnancy related diseases. Firstly, the exact molecular mechanism of transplacental iron transport is not clear, though much work has been done on it. Secondly, ferroptosis is a form of cell death that is associated with lots of signaling pathways, like hypoxia signaling (Zou et al., 2019), AMP-activated protein kinase signaling (Li et al., 2020), E-cadherin-NF2-Hippo-YAP pathway (Yang et al., 2019b), and NRF2-KEAP1 pathway (Anandhan et al., 2020). However, the regulation of ferroptosis in placenta also remains a pressing challenge. Finally, we still do not know whether ferroptosis of trophoblasts leads to PRDs, or it is the execution pathway of PRDs. Therefore, extensive investigation is needed to explore it.
Author contributions
JX and CM conceived and designed the work. JX, FZ, XW, and CM wrote and revised the manuscript. All authors contributed to the article, read, and approved the final manuscript.
Funding
This work was supported by the National Natural Science Foundation of China (grant number 82200084 and 81801485), the Sichuan Science and Technology Program (Grant Number 2023NSFSC1456), the Chinese Scholarship Council (grant number 202106240141), the Fundamental Research Funds for the Central Universities, and Sichuan University postdoctoral interdisciplinary Innovation Fund.
Conflict of interest
The authors declare that the research was conducted in the absence of any commercial or financial relationships that could be construed as a potential conflict of interest.
Publisher’s note
All claims expressed in this article are solely those of the authors and do not necessarily represent those of their affiliated organizations, or those of the publisher, the editors and the reviewers. Any product that may be evaluated in this article, or claim that may be made by its manufacturer, is not guaranteed or endorsed by the publisher.
Abbreviations
AA-CoA, arachidonic acid-CoA; AA-PE, arachidonic acid-phosphatidylethanolamine; AdA-CoA, adrenic acid-CoA; AdA-PE, adrenic acid-phosphatidylethanolamine; ACSL4, acyl-CoA synthetase longchain family member 4; AIFM2, apoptosis-inducing factor 2; CPX, ciclopirox; CHMP5, charged multivesicular body protein 5; CHMP6, charged multivesicular body protein 6; DFO, deferoxamine; DMT1, divalent metal transporter 1; ESCRT-III, endosomal sorting complexes required for transport-III; FTH1, ferritin heavy chain 1; FTL, ferritin light chain; GCLC, glutamate cysteine ligase, catalytic; GPX4, glutathione peroxidase 4; GSH, glutathione; GSS, glutathione synthetase; HMG-CoA, β-Hydroxy β-methylglutaryl-CoA; HMGCR,3-hydroxy-3-methylglutaryl-CoA reductase; LDs, Lipid droplets; LOX, lipoxygenase; LPCAT3, lysophosphatidylcholine acyltransferase 3; NCOA4, nuclear receptor coactivator 4; PL, phospholipid; RSL3, ras-selective lethal 3; ROS, reactive oxygen species; Se, selenium; STEAP3, six-transmembrane epithelial antigen of prostate 3; TF, transferrin; TfR1, transferrin receptor-1.
References
ACOG Practice Bulletin (2019). ACOG Practice Bulletin No. 202: Gestational hypertension and preeclampsia. Obstet. Gynecol. 133 (1), 1–e25. doi:10.1097/AOG.0000000000003018
Alim, I., Caulfield, J. T., Chen, Y., Swarup, V., Geschwind, D. H., Ivanova, E., et al. (2019). Selenium drives a transcriptional adaptive Program to block ferroptosis and treat stroke. Cell 177 (5), 1262–1279. doi:10.1016/j.cell.2019.03.032
American Diabetes Association (2018). 2. Classification and diagnosis of diabetes: Standards of medical care in diabetes-2018. Diabetes Care 41 (1), S13–S27. doi:10.2337/dc18-S002
Anandhan, A., Dodson, M., Schmidlin, C. J., Liu, P., and Zhang, D. D. (2020). Breakdown of an ironclad defense system: The critical role of NRF2 in mediating ferroptosis. Cell Chem. Biol. 27 (4), 436–447. doi:10.1016/j.chembiol.2020.03.011
Angeli, J. P. F., Shah, R., Pratt, D. A., and Conrad, M. (2017). Ferroptosis inhibition: Mechanisms and opportunities. Trends Pharmacol. Sci. 38 (5), 489–498. doi:10.1016/j.tips.2017.02.005
Atlas, D. (2015). IDF diabetes atlas 7th edn. Brussels, Belgium: International Diabetes Federation, 33.
Aydin, S., Benian, A., Madazli, R., Uludag, S., Uzun, H., and Kaya, S. (2004). Plasma malondialdehyde, superoxide dismutase, sE-selectin, fibronectin, endothelin-1 and nitric oxide levels in women with preeclampsia. Eur. J. Obstet. Gynecol. Reprod. Biol. 113 (1), 21–25. doi:10.1016/S0301-2115(03)00368-3
Bannai, S. (1986). Exchange of cystine and glutamate across plasma membrane of human fibroblasts. J. Biol. Chem. 261 (5), 2256–2263. doi:10.1016/s0021-9258(17)35926-4
Battista, N., Meloni, M. A., Bari, M., Mastrangelo, N., Galleri, G., Rapino, C., et al. (2012). 5-Lipoxygenase-dependent apoptosis of human lymphocytes in the international space station: Data from the ROALD experiment. FASEB J. 26 (5), 1791–1798. doi:10.1096/fj.11-199406
Beharier, O., Kajiwara, K., and Sadovsky, Y. (2021). Ferroptosis, trophoblast lipotoxic damage, and adverse pregnancy outcome. Placenta 108, 32–38. doi:10.1016/j.placenta.2021.03.007
Beharier, O., Tyurin, V. A., Goff, J. P., Guerrero-Santoro, J., Kajiwara, K., Chu, T., et al. (2020). PLA2G6 guards placental trophoblasts against ferroptotic injury. Proc. Natl. Acad. Sci. U. S. A. 117 (44), 27319–27328. doi:10.1073/pnas.2009201117
Bersuker, K., Hendricks, J. M., Li, Z., Magtanong, L., Ford, B., Tang, P. H., et al. (2019). The CoQ oxidoreductase FSP1 acts parallel to GPX4 to inhibit ferroptosis. Nature 575 (7784), 688–692. doi:10.1038/s41586-019-1705-2
Bespalova, O., Bakleicheva, M., Kovaleva, I., Tolibova, G., Tral, T., and Kogan, I. (2019). Expression of vitamin D and vitamin D receptor in chorionic villous in missed abortion. Gynecol. Endocrinol. 35 (1), 49–55. doi:10.1080/09513590.2019.1653563
Brown, F. M., and Wyckoff, J. (2017). Application of one-step IADPSG versus two-step diagnostic criteria for gestational diabetes in the real world: Impact on health services, clinical care, and outcomes. Curr. Diab. Rep. 17 (10), 85. doi:10.1007/s11892-017-0922-z
Brütsch, S. H., Wang, C. C., Li, L., Stender, H., Neziroglu, N., Richter, C., et al. (2015). Expression of inactive glutathione peroxidase 4 leads to embryonic lethality, and inactivation of the Alox15 gene does not rescue such knock-in mice. Antioxid. Redox Signal 22 (4), 281–293. doi:10.1089/ars.2014.5967
Chappell, L. C., Bell, J. L., Smith, A., Linsell, L., Juszczak, E., Dixon, P. H., et al. (2019). Ursodeoxycholic acid versus placebo in women with intrahepatic cholestasis of pregnancy (PITCHES): A randomised controlled trial. Lancet 394 (10201), 849–860. doi:10.1016/S0140-6736(19)31270-X
Chen, D., Tavana, O., Chu, B., Erber, L., Chen, Y., Baer, R., et al. (2017). NRF2 is a major target of ARF in p53-independent tumor suppression. Mol. Cell 68 (1), 224–232. doi:10.1016/j.molcel.2017.09.009
Chen, P., Wu, Q., Feng, J., Yan, L., Sun, Y., Liu, S., et al. (2020). Erianin, a novel dibenzyl compound in Dendrobium extract, inhibits lung cancer cell growth and migration via calcium/calmodulin-dependent ferroptosis. Signal Transduct. Target. Ther. 5 (1), 51. doi:10.1038/s41392-020-0149-3
Chen, Z., Kajiwara, K., and Sadovsky, Y. (2022). Ferroptosis and its emerging role in pre-eclampsia. Antioxidants Basel 11, 1282. doi:10.3390/antiox11071282
Cheng, K., Huang, Y., and Wang, C. (2021). 1,25(OH)(2)D(3) inhibited ferroptosis in zebrafish liver cells (ZFL) by regulating keap1-nrf2-GPx4 and NF-κB-hepcidin Axis. Int. J. Mol. Sci. 22, 11334. doi:10.3390/ijms222111334
Ching, T., Song, M. A., Tiirikainen, M., Molnar, J., Berry, M., Towner, D., et al. (2014). Genome-wide hypermethylation coupled with promoter hypomethylation in the chorioamniotic membranes of early onset pre-eclampsia. Mol. Hum. Reprod. 20 (9), 885–904. doi:10.1093/molehr/gau046
Choi, M. J., Yu, J., and Choi, J. (2022). Maternal pre-pregnancy obesity and gestational diabetes mellitus increase the risk of childhood obesity. Child. (Basel) 9, 928. doi:10.3390/children9070928
Christensen, M. H., Rubin, K. H., Petersen, T. G., Nohr, E. A., Vinter, C. A., Andersen, M. S., et al. (2022). Cardiovascular and metabolic morbidity in women with previous gestational diabetes mellitus: A nationwide register-based cohort study. Cardiovasc Diabetol. 21 (1), 179. doi:10.1186/s12933-022-01609-2
Cindrova-Davies, T., and Sferruzzi-Perri, A. N. (2022). Human placental development and function. Semin. Cell Dev. Biol. 131, 66–77. doi:10.1016/j.semcdb.2022.03.039
Conrad, M., and Pratt, D. A. (2019). The chemical basis of ferroptosis. Nat. Chem. Biol. 15 (12), 1137–1147. doi:10.1038/s41589-019-0408-1
Crowther, C. A., Samuel, D., Hughes, R., Tran, T., Brown, J., and Alsweiler, J. M. (2022). Tighter or less tight glycaemic targets for women with gestational diabetes mellitus for reducing maternal and perinatal morbidity: A stepped-wedge, cluster-randomised trial. PLoS Med. 19, e1004087. doi:10.1371/journal.pmed.1004087
D'Souza, V., Rani, A., Patil, V., Pisal, H., Randhir, K., Mehendale, S., et al. (2016). Increased oxidative stress from early pregnancy in women who develop preeclampsia. Clin. Exp. Hypertens. 38 (2), 225–232. doi:10.3109/10641963.2015.1081226
Dai, E., Zhang, W., Cong, D., Kang, R., Wang, J., and Tang, D. (2020). AIFM2 blocks ferroptosis independent of ubiquinol metabolism. Biochem. Biophys. Res. Commun. 523 (4), 966–971. doi:10.1016/j.bbrc.2020.01.066
de Souza, E. A., and Pisani, L. P. (2020). The relationship among vitamin D, TLR4 pathway and preeclampsia. Mol. Biol. Rep. 47 (8), 6259–6267. doi:10.1007/s11033-020-05644-8
Di Cianni, G., Miccoli, R., VoLpe, L., LenCioni, C., and Del Prato, S. (2003). Intermediate metabolism in normal pregnancy and in gestational diabetes. Diabetes Metab. Res. Rev. 19 (4), 259–270. doi:10.1002/dmrr.390
Diggle, C. P. (2002). In vitro studies on the relationship between polyunsaturated fatty acids and cancer: Tumour or tissue specific effects? Prog. Lipid Res. 41 (3), 240–253. doi:10.1016/s0163-7827(01)00025-x
Dixon, S. J., Lemberg, K. M., Lamprecht, M. R., Skouta, R., Zaitsev, E. M., Gleason, C. E., et al. (2012). Ferroptosis: An iron-dependent form of nonapoptotic cell death. Cell 149 (5), 1060–1072. doi:10.1016/j.cell.2012.03.042
Dixon, S. J., Winter, G. E., Musavi, L. S., Lee, E. D., Snijder, B., Rebsamen, M., et al. (2015). Human haploid cell genetics reveals roles for lipid metabolism genes in nonapoptotic cell death. ACS Chem. Biol. 10 (7), 1604–1609. doi:10.1021/acschembio.5b00245
Doll, S., and Conrad, M. (2017). Iron and ferroptosis: A still ill-defined liaison. IUBMB Life 69 (6), 423–434. doi:10.1002/iub.1616
Doll, S., Freitas, F. P., Shah, R., Aldrovandi, M., da Silva, M. C., Ingold, I., et al. (2019). FSP1 is a glutathione-independent ferroptosis suppressor. Nature 575 (7784), 693–698. doi:10.1038/s41586-019-1707-0
Doll, S., Proneth, B., Tyurina, Y. Y., Panzilius, E., Kobayashi, S., Ingold, I., et al. (2017). ACSL4 dictates ferroptosis sensitivity by shaping cellular lipid composition. Nat. Chem. Biol. 13 (1), 91–98. doi:10.1038/nchembio.2239
Dong, H., Qiang, Z., Chai, D., Peng, J., Xia, Y., Hu, R., et al. (2020). Nrf2 inhibits ferroptosis and protects against acute lung injury due to intestinal ischemia reperfusion via regulating SLC7A11 and HO-1. Aging 12 (13), 12943–12959. doi:10.18632/aging.103378
Donovan, A., Brownlie, A., Shepard, J., Pratt, S. J., Moynihan, J., et al. (2000). Positional cloning of zebrafish ferroportin1 identifies a conserved vertebrate iron exporter. Nature 403 (6771), 776–781. doi:10.1038/35001596
Du, G., Zhang, Q., Huang, X., and Wang, Y. (2022). Molecular mechanism of ferroptosis and its role in the occurrence and treatment of diabetes. Front. Genet. 13, 1018829. doi:10.3389/fgene.2022.1018829
Ebegboni, V. J., Balahmar, R. M., Dickenson, J. M., and Sivasubramaniam, S. D. (2019). The effects of flavonoids on human first trimester trophoblast spheroidal stem cell self-renewal, invasion and JNK/p38 MAPK activation: Understanding the cytoprotective effects of these phytonutrients against oxidative stress. Biochem. Pharmacol. 164, 289–298. doi:10.1016/j.bcp.2019.04.023
El Hout, M., Dos Santos, L., Hamai, A., and Mehrpour, M. (2018). A promising new approach to cancer therapy: Targeting iron metabolism in cancer stem cells. Semin. Cancer Biol. 53, 125–138. doi:10.1016/j.semcancer.2018.07.009
El-Khalik, S. R. A., Ibrahim, R. R., Ghafar, M. T. A., Shatat, D., and El-Deeb, O. S. (2022). Novel insights into the slc7a11-mediated ferroptosis signaling pathways in preeclampsia patients: Identifying pannexin 1 and toll-like receptor 4 as innovative prospective diagnostic biomarkers. J. Assist. Reprod. Genet. 39 (5), 1115–1124. doi:10.1007/s10815-022-02443-x
Elguindy, M. M., and Nakamaru-Ogiso, E. (2015). Apoptosis-inducing factor (AIF) and its family member protein, AMID, are rotenone-sensitive NADH:ubiquinone oxidoreductases (NDH-2). J. Biol. Chem. 290 (34), 20815–20826. doi:10.1074/jbc.M115.641498
Fan, X., Zhang, X., Liu, L. C., Zhang, S., Pelger, C. B., Lughmani, H. Y., et al. (2022). Hemopexin accumulates in kidneys and worsens acute kidney injury by causing hemoglobin deposition and exacerbation of iron toxicity in proximal tubules. Kidney Int. 102 (6), 1320–1330. doi:10.1016/j.kint.2022.07.024
Fang, Y., and Fang, D. (2022). Comprehensive analysis of placental gene-expression profiles and identification of EGFR-mediated autophagy and ferroptosis suppression in intrahepatic cholestasis of pregnancy. Gene 834, 146594. doi:10.1016/j.gene.2022.146594
Friedmann Angeli, J. P., Schneider, M., Proneth, B., Tyurina, Y. Y., Tyurin, V. A., Hammond, V. J., et al. (2014). Inactivation of the ferroptosis regulator Gpx4 triggers acute renal failure in mice. Nat. Cell Biol. 16 (12), 1180–1191. doi:10.1038/ncb3064
Fu, J. Y., Jing, Y., Xiao, Y. P., Wang, X. H., Guo, Y. W., and Zhu, Y. J. (2021). Astaxanthin inhibiting oxidative stress damage of placental trophoblast cells in vitro. Syst. Biol. Reprod. Med. 67 (1), 79–88. doi:10.1080/19396368.2020.1824031
Funk, C. D., Chen, X. S., Johnson, E. N., and Zhao, L. (2002). Lipoxygenase genes and their targeted disruption. Prostagl. Other Lipid Mediat 68-69, 303–312. doi:10.1016/s0090-6980(02)00036-9
Gaschler, M. M., Andia, A. A., Liu, H., Csuka, J. M., Hurlocker, B., Vaiana, C. A., et al. (2018). FINO2 initiates ferroptosis through GPX4 inactivation and iron oxidation. Nat. Chem. Biol. 14 (5), 507–515. doi:10.1038/s41589-018-0031-6
Gautam, S., Alam, F., Moin, S., Noor, N., and Arif, S. H. (2021). Role of ferritin and oxidative stress index in gestational diabetes mellitus. J. Diabetes Metab. Disord. 20 (2), 1615–1619. doi:10.1007/s40200-021-00911-2
Gill, I., and Valivety, R. (1997). Polyunsaturated fatty acids, Part 1: Occurrence, biological activities and applications. Trends Biotechnol. 15 (10), 401–409. doi:10.1016/S0167-7799(97)01076-7
Giuliani, C., Sciacca, L., Biase, N. D., Tumminia, A., Milluzzo, A., Faggiano, A., et al. (2022). Gestational diabetes mellitus pregnancy by pregnancy: Early, late and nonrecurrent GDM. Diabetes Res. Clin. Pract. 188, 109911. doi:10.1016/j.diabres.2022.109911
Habibi, N., Jankovic-Karasoulos, T., Leemaqz, S. Y. L., Francois, M., Zhou, S. J., Leifert, W. R., et al. (2021). Effect of iodine and selenium on proliferation, viability, and oxidative stress in HTR-8/SVneo placental cells. Biol. Trace Elem. Res. 199 (4), 1332–1344. doi:10.1007/s12011-020-02277-7
Haddad, G. F., Jodicke, C., Thomas, M. A., Williams, D. B., and Aubuchon, M. (2008). Case series of rosiglitazone used during the first trimester of pregnancy. Reprod. Toxicol. 26 (2), 183–184. doi:10.1016/j.reprotox.2008.08.001
Han, D., Jiang, L., Gu, X., Huang, S., Pang, J., Wu, Y., et al. (2020). SIRT3 deficiency is resistant to autophagy-dependent ferroptosis by inhibiting the AMPK/mTOR pathway and promoting GPX4 levels. J. Cell. Physiol. 235 (11), 8839–8851. doi:10.1002/jcp.29727
Han, L., Bai, L., Fang, X., Liu, J., Kang, R., Zhou, D., et al. (2021). SMG9 drives ferroptosis by directly inhibiting GPX4 degradation. Biochem. Biophys. Res. Commun. 567, 92–98. doi:10.1016/j.bbrc.2021.06.038
He, H., Liu, Y., and Sun, M. (2021). Nesfatin-1 alleviates high glucose/high lipid-induced injury of trophoblast cells during gestational diabetes mellitus. Bioengineered 12 (2), 12789–12799. doi:10.1080/21655979.2021.2001205
Hidaka, T., Fujii, K., Funahashi, I., Fukutomi, N., and Hosoe, K. (2008). Safety assessment of coenzyme Q10 (CoQ10). Biofactors 32 (1-4), 199–208. doi:10.1002/biof.5520320124
Hishikawa, D., Shindou, H., Kobayashi, S., Nakanishi, H., Taguchi, R., and Shimizu, T. (2008). Discovery of a lysophospholipid acyltransferase family essential for membrane asymmetry and diversity. Proc. Natl. Acad. Sci. U. S. A. 105 (8), 2830–2835. doi:10.1073/pnas.0712245105
Hou, L., Huang, R., Sun, F., Zhang, L., and Wang, Q. (2019). NADPH oxidase regulates paraquat and maneb-induced dopaminergic neurodegeneration through ferroptosis. Toxicology 417, 64–73. doi:10.1016/j.tox.2019.02.011
Hou, W., Xie, Y., Song, X., Sun, X., Lotze, M. T., Zeh, H. J., et al. (2016). Autophagy promotes ferroptosis by degradation of ferritin. Autophagy 12 (8), 1425–1428. doi:10.1080/15548627.2016.1187366
Hu, B., Li, D., Tang, D., Shangguan, Y., Cao, Y., Guo, R., et al. (2022). Integrated proteome and acetylome analyses unveil protein features of gestational diabetes mellitus and preeclampsia. Proteomics 22, e2200124. doi:10.1002/pmic.202200124
Hu, J., Gillies, C. L., Lin, S., Stewart, Z. A., Melford, S. E., Abrams, K. R., et al. (2021). Association of maternal lipid profile and gestational diabetes mellitus: A systematic review and meta-analysis of 292 studies and 97,880 women. EClinicalMedicine 34, 100830. doi:10.1016/j.eclinm.2021.100830
Hu, Q., Zhang, Y., Lou, H., Ou, Z., Liu, J., Duan, W., et al. (2021). GPX4 and vitamin E cooperatively protect hematopoietic stem and progenitor cells from lipid peroxidation and ferroptosis. Cell Death Dis. 12 (7), 706. doi:10.1038/s41419-021-04008-9
Hu, Y. Y., Liu, J. C., and Xing, A. Y. (2015). Oxidative stress markers in intrahepatic cholestasis of pregnancy: A prospective controlled study. Eur. Rev. Med. Pharmacol. Sci. 19 (17), 3181–3186.
Hulbert, A. J., Rana, T., and Couture, P. (2002). The acyl composition of mammalian phospholipids: An allometric analysis. Comp. Biochem. Physiol. B Biochem. Mol. Biol. 132 (3), 515–527. doi:10.1016/s1096-4959(02)00066-0
Irwinda, R., Hiksas, R., Siregar, A. A., Saroyo, Y. B., and Wibowo, N. (2021). Long-chain polyunsaturated fatty acid (LC-PUFA) status in severe preeclampsia and preterm birth: A cross sectional study. Sci. Rep. 11 (1), 14701. doi:10.1038/s41598-021-93846-w
Jenkitkasemwong, S., Wang, C. Y., Mackenzie, B., and Knutson, M. D. (2012). Physiologic implications of metal-ion transport by ZIP14 and ZIP8. Biometals 25 (4), 643–655. doi:10.1007/s10534-012-9526-x
Jiang, L., Kon, N., Li, T., Wang, S. J., Su, T., Hibshoosh, H., et al. (2015). Ferroptosis as a p53-mediated activity during tumour suppression. Nature 520 (7545), 57–62. doi:10.1038/nature14344
Ju, Y., Feng, Y., Hou, X., Wu, L., Yang, H., Zhang, H., et al. (2022). Combined apocyanin and aspirin treatment activates the PI3K/Nrf2/HO-1 signaling pathway and ameliorates preeclampsia symptoms in rats. Hypertens. Pregnancy 41 (1), 39–50. doi:10.1080/10641955.2021.2014518
Kagan, V. E., Mao, G., Qu, F., Angeli, J. P. F., Doll, S., Croix, C. S., et al. (2017). Oxidized arachidonic and adrenic PEs navigate cells to ferroptosis. Nat. Chem. Biol. 13 (1), 81–90. doi:10.1038/nchembio.2238
Kajiwara, K., Beharier, O., Chng, C. P., Goff, J. P., Ouyang, Y., St Croix, C. M., et al. (2022). Ferroptosis induces membrane blebbing in placental trophoblasts. J. Cell Sci. 135, jcs255737. doi:10.1242/jcs.255737
Kenny, E. M., Fidan, E., Yang, Q., Anthonymuthu, T. S., New, L. A., Meyer, E. A., et al. (2019). Ferroptosis contributes to neuronal death and functional outcome after traumatic brain injury. Crit. Care Med. 47 (3), 410–418. doi:10.1097/CCM.0000000000003555
Kuang, F., Liu, J., Tang, D., and Kang, R. (2020). Oxidative damage and antioxidant defense in ferroptosis. Front. Cell Dev. Biol. 8, 586578. doi:10.3389/fcell.2020.586578
Küch, E. M., Vellaramkalayil, R., Zhang, I., Lehnen, D., Brugger, B., Sreemmel, W., et al. (2014). Differentially localized acyl-CoA synthetase 4 isoenzymes mediate the metabolic channeling of fatty acids towards phosphatidylinositol. Biochim. Biophys. Acta 1841 (2), 227–239. doi:10.1016/j.bbalip.2013.10.018
Kuhn, H., Saam, J., Eibach, S., Holzhutter, H. G., Ivanov, I., and Walther, M. (2005). Structural biology of mammalian lipoxygenases: Enzymatic consequences of targeted alterations of the protein structure. Biochem. Biophys. Res. Commun. 338 (1), 93–101. doi:10.1016/j.bbrc.2005.08.238
Kwon, M. Y., Park, E., Lee, S. J., and Chung, S. W. (2015). Heme oxygenase-1 accelerates erastin-induced ferroptotic cell death. Oncotarget 6 (27), 24393–24403. doi:10.18632/oncotarget.5162
Lei, G., Zhuang, L., and Gan, B. (2022). Targeting ferroptosis as a vulnerability in cancer. Nat. Rev. Cancer 22 (7), 381–396. doi:10.1038/s41568-022-00459-0
Leitao, S., Manning, E., Greene, R. A., and Corcoran, P.Maternal Morbidity Advisory Group (2022). Maternal morbidity and mortality: An iceberg phenomenon. Bjog 129 (3), 402–411. doi:10.1111/1471-0528.16880
Li, C., Dong, X., Du, W., Shi, X., Chen, K., Zhang, W., et al. (2020). LKB1-AMPK axis negatively regulates ferroptosis by inhibiting fatty acid synthesis. Signal Transduct. Target. Ther. 5 (1), 187. doi:10.1038/s41392-020-00297-2
Li, G., Lin, L., Wang, Y. L., and Yang, H. (2019). 1,25(OH)2D3 protects trophoblasts against insulin resistance and inflammation via suppressing mTOR signaling. Reprod. Sci. 26 (2), 223–232. doi:10.1177/1933719118766253
Li, L., Li, W. J., Zheng, X. R., Liu, Q. L., Du, Q., Lai, Y. J., et al. (2022). Eriodictyol ameliorates cognitive dysfunction in APP/PS1 mice by inhibiting ferroptosis via vitamin D receptor-mediated Nrf2 activation. Mol. Med. 28 (1), 11. doi:10.1186/s10020-022-00442-3
Li, Z., Ferguson, L., Deol, K. K., Roberts, M. A., Magtanong, L., Hendricks, J. M., et al. (2022). Ribosome stalling during selenoprotein translation exposes a ferroptosis vulnerability. Nat. Chem. Biol. 18 (7), 751–761. doi:10.1038/s41589-022-01033-3
Liao, T., Xu, X., Ye, X., and Yan, J. (2022). DJ-1 upregulates the Nrf2/GPX4 signal pathway to inhibit trophoblast ferroptosis in the pathogenesis of preeclampsia. Sci. Rep. 12, 2934. doi:10.1038/s41598-022-07065-y
Liu, J. X., Chen, D., Li, M. X., and Hua, Y. (2019). Increased serum iron levels in pregnant women with preeclampsia: A meta-analysis of observational studies. J. Obstet. Gynaecol. 39 (1), 11–16. doi:10.1080/01443615.2018.1450368
Liu, Z., Ma, J., Zuo, X., Zhang, X., Hong, Y., Cai, S., et al. (2022). C5b-9 mediates ferroptosis of tubular epithelial cells in trichloroethylene-sensitization mice. Ecotoxicol. Environ. Saf. 244, 114020. doi:10.1016/j.ecoenv.2022.114020
Llabani, E., Hicklin, R. W., Lee, H. Y., Motika, S. E., Crawford, L. A., Weerapana, E., et al. (2019). Diverse compounds from pleuromutilin lead to a thioredoxin inhibitor and inducer of ferroptosis. Nat. Chem. 11 (6), 521–532. doi:10.1038/s41557-019-0261-6
Long, L., Guo, H., Chen, X., Liu, Y., Wang, R., Zheng, X., et al. (2022). Advancement in understanding the role of ferroptosis in rheumatoid arthritis. Front. Physiol. 13, 1036515. doi:10.3389/fphys.2022.1036515
Lovatt, M., Adnan, K., Kocaba, V., Dirisamer, M., Peh, G. S. L., and Mehta, J. S. (2020). Peroxiredoxin-1 regulates lipid peroxidation in corneal endothelial cells. Redox Biol. 30, 101417. doi:10.1016/j.redox.2019.101417
Lu, S. C. (2013). Glutathione synthesis. Biochim. Biophys. Acta 1830 (5), 3143–3153. doi:10.1016/j.bbagen.2012.09.008
Maiorino, M., Conrad, M., and Ursini, F. (2018). GPx4, lipid peroxidation, and cell death: Discoveries, rediscoveries, and open issues. Antioxid. Redox Signal. 29 (1), 61–74. doi:10.1089/ars.2017.7115
Martinefski, M. R., Rodriguez, M. R., Buontempo, F., Lucangioli, S. E., Bianciotti, L. G., and Tripodi, V. P. (2020). Coenzyme Q 10 supplementation: A potential therapeutic option for the treatment of intrahepatic cholestasis of pregnancy. Eur. J. Pharmacol. 882, 173270. doi:10.1016/j.ejphar.2020.173270
Martinefski, M. R., Scioscia, S., Contin, M. D., Samassa, P., Lucangioli, S. E., and Tripodi, V. P. (2014). A simple microHPLC-UV method for the simultaneous determination of retinol and α-tocopherol in human plasma. Application to intrahepatic cholestasis of pregnancy. Anal. Methods 6 (10), 3365–3369. doi:10.1039/c4ay00058g
Mauri, F., Schepkens, C., Lapouge, G., Drogat, B., Song, Y., Pastushenko, I., et al. (2021). NR2F2 controls malignant squamous cell carcinoma state by promoting stemness and invasion and repressing differentiation. Nat. Cancer 2 (11), 1152–1169. doi:10.1038/s43018-021-00287-5
Meihe, L., Shan, G., Minchao, K., Xiaoling, W., Peng, A., Xili, W., et al. (2021). The ferroptosis-NLRP1 inflammasome: The vicious cycle of an adverse pregnancy. Front. Cell Dev. Biol. 9, 707959. doi:10.3389/fcell.2021.707959
Michalski, M. C., Calzada, C., Makino, A., Michaud, S., and Guichardant, M. (2008). Oxidation products of polyunsaturated fatty acids in infant formulas compared to human milk–a preliminary study. Mol. Nutr. Food Res. 52 (12), 1478–1485. doi:10.1002/mnfr.200700451
Miyamoto, H. D., Ikeda, M., Ide, T., Tadokoro, T., Furusawa, S., Abe, K., et al. (2022). Iron overload via heme degradation in the endoplasmic reticulum triggers ferroptosis in myocardial ischemia-reperfusion injury. JACC Basic Transl. Sci. 7 (8), 800–819. doi:10.1016/j.jacbts.2022.03.012
Ng, S. W., Norwitz, S. G., and Norwitz, E. R. (2019). The impact of iron overload and ferroptosis on reproductive disorders in humans: Implications for preeclampsia. Int. J. Mol. Sci. 20, 3283. doi:10.3390/ijms20133283
Ou, Y., Wang, S. J., Li, D., Chu, B., and Gu, W. (2016). Activation of SAT1 engages polyamine metabolism with p53-mediated ferroptotic responses. Proc. Natl. Acad. Sci. U. S. A. 113, E6806–E6812. doi:10.1073/pnas.1607152113
Parhofer, K. G. (2015). Interaction between glucose and lipid metabolism: More than diabetic dyslipidemia. Diabetes Metab. J. 39 (5), 353–362. doi:10.4093/dmj.2015.39.5.353
Phelps, R. L., Metzger, B. E., and Freinkel, N. (1981). Carbohydrate metabolism in pregnancy. XVII. Diurnal profiles of plasma glucose, insulin, free fatty acids, triglycerides, cholesterol, and individual amino acids in late normal pregnancy. Am. J. Obstet. Gynecol. 140 (7), 730–736.
Pollex, E. K., and Hutson, J. R. (2011). Genetic polymorphisms in placental transporters: Implications for fetal drug exposure to oral antidiabetic agents. Expert Opin. Drug Metab. Toxicol. 7 (3), 325–339. doi:10.1517/17425255.2011.553188
Probst, L., Dachert, J., Schenk, B., and Fulda, S. (2017). Lipoxygenase inhibitors protect acute lymphoblastic leukemia cells from ferroptotic cell death. Biochem. Pharmacol. 140, 41–52. doi:10.1016/j.bcp.2017.06.112
Qi, W., Li, Z., Xia, L., Dai, J., Zhang, Q., Wu, C., et al. (2019). LncRNA GABPB1-AS1 and GABPB1 regulate oxidative stress during erastin-induced ferroptosis in HepG2 hepatocellular carcinoma cells. Sci. Rep. 9 (1), 16185. doi:10.1038/s41598-019-52837-8
Raefsky, S. M., Furman, R., Milne, G., Pollock, E., Axelsen, P., Mattson, M. P., et al. (2018). Deuterated polyunsaturated fatty acids reduce brain lipid peroxidation and hippocampal amyloid β-peptide levels, without discernable behavioral effects in an APP/PS1 mutant transgenic mouse model of Alzheimer's disease. Neurobiol. Aging 66, 165–176. doi:10.1016/j.neurobiolaging.2018.02.024
Raijmakers, M. T., Dechend, R., and Poston, L. (2004). Oxidative stress and preeclampsia: Rationale for antioxidant clinical trials. Hypertension 44 (4), 374–380. doi:10.1161/01.HYP.0000141085.98320.01
Rainey, N. E., Moustapha, A., Saric, A., Nicolas, G., Sureau, F., and Petit, P. X. (2019). Iron chelation by curcumin suppresses both curcumin-induced autophagy and cell death together with iron overload neoplastic transformation. Cell Death Discov. 5, 150. doi:10.1038/s41420-019-0234-y
Rashad, S., Byrne, S. R., Saigusa, D., Xiang, J., Zhou, Y., Zhang, L., et al. (2022). Codon usage and mRNA stability are translational determinants of cellular response to canonical ferroptosis inducers. Neuroscience 501, 103–130. doi:10.1016/j.neuroscience.2022.08.009
Reyes, H., Baez, M. E., Gonzalez, M. C., Hernandez, I., Palma, J., Ribalta, J., et al. (2000). Selenium, zinc and copper plasma levels in intrahepatic cholestasis of pregnancy, in normal pregnancies and in healthy individuals, in Chile. J. Hepatol. 32 (4), 542–549. doi:10.1016/s0168-8278(00)80214-7
Rice-Evans, C., and Burdon, R. (1993). Free radical-lipid interactions and their pathological consequences. Prog. Lipid Res. 32 (1), 71–110. doi:10.1016/0163-7827(93)90006-i
Ryan, S. K., Zelic, M., Han, Y., Teeple, E., Chen, L., Sadeghi, M., et al. (2022). Microglia ferroptosis is regulated by SEC24B and contributes to neurodegeneration. Nat. Neurosci. 26, 12–26. doi:10.1038/s41593-022-01221-3
Ryu, M. S., Zhang, D., Protchenko, O., Shakoury-Elizeh, M., and Philpott, C. C. (2017). PCBP1 and NCOA4 regulate erythroid iron storage and heme biosynthesis. J. Clin. Invest. 127 (5), 1786–1797. doi:10.1172/JCI90519
Sangkhae, V., Fisher, A. L., Wong, S., Koenig, M. D., Tussing-Humphreys, L., Chu, A., et al. (2020). Effects of maternal iron status on placental and fetal iron homeostasis. J. Clin. Invest. 130 (2), 625–640. doi:10.1172/JCI127341
Sanhal, C. Y., Daglar, K., Kara, O., Yilmaz, Z. V., Turkmen, G. G., Erel, O., et al. (2018). An alternative method for measuring oxidative stress in intrahepatic cholestasis of pregnancy: Thiol/disulphide homeostasis. J. Matern. Fetal Neonatal Med. 31 (11), 1477–1482. doi:10.1080/14767058.2017.1319922
Santesmasses, D., Mariotti, M., and Gladyshev, V. N. (2020). Tolerance to selenoprotein loss differs between human and mouse. Mol. Biol. Evol. 37 (2), 341–354. doi:10.1093/molbev/msz218
Schoots, M. H., Gordijn, S. J., Scherjon, S. A., van Goor, H., and Hillebrands, J. L. (2018). Oxidative stress in placental pathology. Placenta 69, 153–161. doi:10.1016/j.placenta.2018.03.003
Schreiber, R., Buchholz, B., Kraus, A., Schley, G., Scholz, J., Ousingsawat, J., et al. (2019). Lipid peroxidation drives renal cyst growth in vitro through activation of TMEM16A. J. Am. Soc. Nephrol. 30 (2), 228–242. doi:10.1681/ASN.2018010039
Seligman, P. A., Schleicher, R. B., and Allen, R. H. (1979). Isolation and characterization of the transferrin receptor from human placenta. J. Biol. Chem. 254 (20), 9943–9946. doi:10.1016/s0021-9258(19)86649-8
Sha, L. K., Sha, W., Kuchler, L., Daiber, A., Giegerich, A. K., Weigert, A., et al. (2015). Loss of Nrf2 in bone marrow-derived macrophages impairs antigen-driven CD8(+) T cell function by limiting GSH and Cys availability. Free Radic. Biol. Med. 83, 77–88. doi:10.1016/j.freeradbiomed.2015.02.004
Shah, R., Shchepinov, M. S., and Pratt, D. A. (2018). Resolving the role of lipoxygenases in the initiation and execution of ferroptosis. ACS Cent. Sci. 4 (3), 387–396. doi:10.1021/acscentsci.7b00589
Shimada, K., Skouta, R., Kaplan, A., Yang, W. S., Hayano, M., Dixon, S. J., et al. (2016). Global survey of cell death mechanisms reveals metabolic regulation of ferroptosis. Nat. Chem. Biol. 12 (7), 497–503. doi:10.1038/nchembio.2079
Song, X., Chen, L., Zhang, S., Liu, Y., Wei, J., Wang, T., et al. (2022). Gestational diabetes mellitus and high triglyceride levels mediate the association between pre-pregnancy overweight/obesity and macrosomia: A prospective cohort study in central China. Nutrients 14, 3347. doi:10.3390/nu14163347
Song, X., Zhu, S., Chen, P., Hou, W., Wen, Q., Liu, J., et al. (2018). AMPK-mediated BECN1 phosphorylation promotes ferroptosis by directly blocking system Xc- activity. Curr. Biol. 28 (15), 2388–2399. doi:10.1016/j.cub.2018.05.094
Sun, Y., Berleth, N., Wu, W., Schlutermann, D., Deitersen, J., Stuhldreier, F., et al. (2021). Fin56-induced ferroptosis is supported by autophagy-mediated GPX4 degradation and functions synergistically with mTOR inhibition to kill bladder cancer cells. Cell Death Dis. 12 (11), 1028. doi:10.1038/s41419-021-04306-2
Sweeting, A., Wong, J., Murphy, H. R., and Ross, G. P. (2022). A clinical update on gestational diabetes mellitus. Endocr. Rev. 43 (5), 763–793. doi:10.1210/endrev/bnac003
Tang, D., Chen, X., Kang, R., and Kroemer, G. (2021). Ferroptosis: Molecular mechanisms and health implications. Cell Res. 31 (2), 107–125. doi:10.1038/s41422-020-00441-1
Teran, E., Hernandez, I., Tana, L., Teran, S., Galaviz-Hernandez, C., Sosa-Macias, M., et al. (2018). Mitochondria and coenzyme Q10 in the pathogenesis of preeclampsia. Front. Physiol. 9, 1561. doi:10.3389/fphys.2018.01561
Thiele, C., and Spandl, J. (2008). Cell biology of lipid droplets. Curr. Opin. Cell Biol. 20 (4), 378–385. doi:10.1016/j.ceb.2008.05.009
Ursini, F., Maiorino, M., and Gregolin, C. (1985). The selenoenzyme phospholipid hydroperoxide glutathione peroxidase. Biochim. Biophys. Acta 839 (1), 62–70. doi:10.1016/0304-4165(85)90182-5
Van Horn, C. G., Caviglia, J. M., Li, L. O., Wang, S., Granger, D. A., and Coleman, R. A. (2005). Characterization of recombinant long-chain rat acyl-CoA synthetase isoforms 3 and 6: Identification of a novel variant of isoform 6. Biochemistry 44 (5), 1635–1642. doi:10.1021/bi047721l
Vounzoulaki, E., Khunti, K., Abner, S. C., Tan, B. K., Davies, M. J., and Gillies, C. L. (2020). Progression to type 2 diabetes in women with a known history of gestational diabetes: Systematic review and meta-analysis. Bmj 369, m1361. doi:10.1136/bmj.m1361
Walters, J. L. H., De Iuliis, G. N., Dun, M. D., Aitken, R. J., McLaughlin, E. A., Nixon, B., et al. (2018). Pharmacological inhibition of arachidonate 15-lipoxygenase protects human spermatozoa against oxidative stress. Biol. Reprod. 98 (6), 784–794. doi:10.1093/biolre/ioy058
Wang, L., Liu, Y., Du, T., Yang, H., Lei, L., Guo, M., et al. (2020). ATF3 promotes erastin-induced ferroptosis by suppressing system Xc. Cell Death Differ. 27 (2), 662–675. doi:10.1038/s41418-019-0380-z
Wang, X., Liu, Z., Peng, P., Gong, Z., Huang, J., and Peng, H. (2022). Astaxanthin attenuates osteoarthritis progression via inhibiting ferroptosis and regulating mitochondrial function in chondrocytes. Chem. Biol. Interact. 366, 110148. doi:10.1016/j.cbi.2022.110148
Wenzel, S. E., Tyurina, Y. Y., Zhao, J., St Croix, C. M., Dar, H. H., Mao, G., et al. (2017). PEBP1 wardens ferroptosis by enabling lipoxygenase generation of lipid death signals. Cell 171 (3), 628–641. doi:10.1016/j.cell.2017.09.044
Wikström Shemer, E., Marschall, H. U., Ludvigsson, J. F., and StephanssOn, O. (2013). Intrahepatic cholestasis of pregnancy and associated adverse pregnancy and fetal outcomes: A 12-year population-based cohort study. Bjog 120 (6), 717–723. doi:10.1111/1471-0528.12174
Wilcox, A. J., Weinberg, C. R., O'Connor, J. F., Baird, D. D., Schlatterer, J. P., Canfield, R. E., et al. (1988). Incidence of early loss of pregnancy. N. Engl. J. Med. 319 (4), 189–194. doi:10.1056/NEJM198807283190401
Wu, H., Luan, Y., Wang, H., Zhang, P., Liu, S., Wang, P., et al. (2022). Selenium inhibits ferroptosis and ameliorates autistic-like behaviors of BTBR mice by regulating the Nrf2/GPx4 pathway. Brain Res. Bull. 183, 38–48. doi:10.1016/j.brainresbull.2022.02.018
Wu, M., Xu, L. G., Li, X., Zhai, Z., and Shu, H. B. (2002). AMID, an apoptosis-inducing factor-homologous mitochondrion-associated protein, induces caspase-independent apoptosis. J. Biol. Chem. 277 (28), 25617–25623. doi:10.1074/jbc.M202285200
Xie, R., Zhao, W., Lowe, S., Bentley, R., Hu, G., Mei, H., et al. (2022). Quercetin alleviates kainic acid-induced seizure by inhibiting the Nrf2-mediated ferroptosis pathway. Free Radic. Biol. Med. 191, 212–226. doi:10.1016/j.freeradbiomed.2022.09.001
Xu, Z., You, Y., Tang, Q., Zeng, H., Zhao, T., Wang, J., et al. (2022). Echinatin mitigates sevoflurane-induced hippocampal neurotoxicity and cognitive deficits through mitigation of iron overload and oxidative stress. Pharm. Biol. 60 (1), 1915–1924. doi:10.1080/13880209.2022.2123941
Xuan, R. R., Niu, T. T., and Chen, H. M. (2016). Astaxanthin blocks preeclampsia progression by suppressing oxidative stress and inflammation. Mol. Med. Rep. 14 (3), 2697–2704. doi:10.3892/mmr.2016.5569
Xuan, R.-r., Wu, W., and Chen, H. M. (2014). Effect of astaxanthin on preeclampsia rat model. Yao Xue Xue Bao 49 (10), 1400–1405.
Yang, N., Wang, Q., Ding, B., Gong, Y., Wu, Y., Sun, J., et al. (2022). Expression profiles and functions of ferroptosis-related genes in the placental tissue samples of early- and late-onset preeclampsia patients. BMC Pregnancy Childbirth 22 (1), 87. doi:10.1186/s12884-022-04423-6
Yang, S., Song, L., Shi, X., Zhao, N., and Ma, Y. (2019). Ameliorative effects of pre-eclampsia by quercetin supplement to aspirin in a rat model induced by L-NAME. Biomed. Pharmacother. 116, 108969. doi:10.1016/j.biopha.2019.108969
Yang, S., Zhang, J., Chen, D., Ding, J., Zhang, Y., and Song, L. (2022). Quercetin supplement to aspirin attenuates lipopolysaccharide-induced pre-eclampsia-like impairments in rats through the NLRP3 inflammasome. Drugs R. D. 22 (4), 271–279. doi:10.1007/s40268-022-00402-6
Yang, W. H., Ding, C. K. C., Sun, T., Rupprecht, G., Lin, C. C., Hsu, D., et al. (2019). The hippo pathway effector TAZ regulates ferroptosis in renal cell carcinoma. Cell Rep. 28 (10), 2501–2508. doi:10.1016/j.celrep.2019.07.107
Yang, W. S., Kim, K. J., Gaschler, M. M., Patel, M., Shchepinov, M. S., and Stockwell, B. R. (2016). Peroxidation of polyunsaturated fatty acids by lipoxygenases drives ferroptosis. Proc. Natl. Acad. Sci. U. S. A. 113, E4966–E4975. doi:10.1073/pnas.1603244113
Yang, W. S., SriRamaratnam, R., Welsch, M. E., Shimada, K., Skouta, R., Viswanathan, V. S., et al. (2014). Regulation of ferroptotic cancer cell death by GPX4. Cell 156 (1-2), 317–331. doi:10.1016/j.cell.2013.12.010
Yang, W. S., and Stockwell, B. R. (2008). Synthetic lethal screening identifies compounds activating iron-dependent, nonapoptotic cell death in oncogenic-RAS-harboring cancer cells. Chem. Biol. 15 (3), 234–245. doi:10.1016/j.chembiol.2008.02.010
Ye, J., Peng, J., Liu, K., Zhang, T., and Huang, W. (2022). MCTR1 inhibits ferroptosis by promoting NRF2 expression to attenuate hepatic ischemia-reperfusion injury. Am. J. Physiol. Gastrointest. Liver Physiol. 323, G283–G293. doi:10.1152/ajpgi.00354.2021
Ye, Y., Chen, A., Li, L., Liang, Q., Wang, S., Dong, Q., et al. (2022). Repression of the antiporter SLC7A11/Glutathione/Glutathione Peroxidase 4 axis drives ferroptosis of vascular smooth muscle cells to facilitate vascular calcification. Kidney Int. 102 (6), 1259–1275. doi:10.1016/j.kint.2022.07.034
Zaugg, J., Solenthaler, F., and Albrecht, C. (2022). Materno-fetal iron transfer and the emerging role of ferroptosis pathways. Biochem. Pharmacol. 202, 115141. doi:10.1016/j.bcp.2022.115141
Zhan, S., Lu, L., Pan, S. S., Wei, X. Q., Miao, R. R., Liu, X. H., et al. (2022). Targeting NQO1/GPX4-mediated ferroptosis by plumbagin suppresses in vitro and in vivo glioma growth. Br. J. Cancer 127 (2), 364–376. doi:10.1038/s41416-022-01800-y
Zhang, H., He, Y., Wang, J. X., Chen, M. H., Xu, J. J., Jiang, M. H., et al. (2020). miR-30-5p-mediated ferroptosis of trophoblasts is implicated in the pathogenesis of preeclampsia. Redox Biol. 29, 101402. doi:10.1016/j.redox.2019.101402
Zhang, X., Wu, M., Zhong, C., Huang, L., Zhang, Y., Chen, R., et al. (2021). Association between maternal plasma ferritin concentration, iron supplement use, and the risk of gestational diabetes: A prospective cohort study. Am. J. Clin. Nutr. 114 (3), 1100–1106. doi:10.1093/ajcn/nqab162
Zhang, X., Wu, S., Guo, C., Guo, K., Hu, Z., Peng, J., et al. (2022). Vitamin E exerts neuroprotective effects in pentylenetetrazole kindling epilepsy via suppression of ferroptosis. Neurochem. Res. 47 (3), 739–747. doi:10.1007/s11064-021-03483-y
Zhang, Y., Lu, Y., and Jin, L. (2022). Iron metabolism and ferroptosis in physiological and pathological pregnancy. Int. J. Mol. Sci. 23, 9395. doi:10.3390/ijms23169395
Zhang, Y., Shi, J., Liu, X., Feng, L., Gong, Z., Koppula, P., et al. (2018). BAP1 links metabolic regulation of ferroptosis to tumour suppression. Nat. Cell Biol. 20 (10), 1181–1192. doi:10.1038/s41556-018-0178-0
Zhang, Y., Zhang, X., Wee Yong, V., and Xue, M. (2022). Vildagliptin improves neurological function by inhibiting apoptosis and ferroptosis following intracerebral hemorrhage in mice. Neurosci. Lett. 776, 136579. doi:10.1016/j.neulet.2022.136579
Zhao, Z., Wu, J., Xu, H., Zhou, C., Han, B., Zhu, H., et al. (2020). XJB-5-131 inhibited ferroptosis in tubular epithelial cells after ischemia-reperfusion injury. Cell Death Dis. 11 (8), 629. doi:10.1038/s41419-020-02871-6
Zheng, Y., Hu, Q., and Wu, J. (2022). Adiponectin ameliorates placental injury in gestational diabetes mice by correcting fatty acid oxidation/peroxide imbalance-induced ferroptosis via restoration of CPT-1 activity. Endocrine 75 (3), 781–793. doi:10.1007/s12020-021-02933-5
Zhou, Q., Yang, L., Li, T., Wang, K., Huang, X., Shi, J., et al. (2022). Mechanisms and inhibitors of ferroptosis in psoriasis. Front. Mol. Biosci. 9, 1019447. doi:10.3389/fmolb.2022.1019447
Zhu, P., Zhao, S. M., Li, Y. Z., Guo, H., Wang, L., and Tian, P. (2019). Correlation of lipid peroxidation and ATP enzyme on erythrocyte membrane with fetal distress in the uterus in patients with intrahepatic cholestasis of pregnancy. Eur. Rev. Med. Pharmacol. Sci. 23 (6), 2318–2324. doi:10.26355/eurrev_201903_17371
Zilka, O., Shah, R., Li, B., Friedmann Angeli, J. P., Griesser, M., Conrad, M., et al. (2017). On the mechanism of cytoprotection by ferrostatin-1 and liproxstatin-1 and the role of lipid peroxidation in ferroptotic cell death. ACS Cent. Sci. 3 (3), 232–243. doi:10.1021/acscentsci.7b00028
Zou, Y., Graham, E. T., Deik, A. A., Eaton, J. K., Wang, W., et al. (2020). Cytochrome P450 oxidoreductase contributes to phospholipid peroxidation in ferroptosis. Nat. Chem. Biol. 16 (3), 302–309. doi:10.1038/s41589-020-0472-6
Keywords: ferroptosis, molecular mechanisms, pregnancy related diseases, intrahepatic cholestasis of pregnancy (ICP), therapeutic potential
Citation: Xu J, Zhou F, Wang X and Mo C (2023) Role of ferroptosis in pregnancy related diseases and its therapeutic potential. Front. Cell Dev. Biol. 11:1083838. doi: 10.3389/fcell.2023.1083838
Received: 29 October 2022; Accepted: 20 February 2023;
Published: 08 March 2023.
Edited by:
Joseph Thomas Opferman, St. Jude Children’s Research Hospital, United StatesReviewed by:
Huizhen Zhang, Zhengzhou University, ChinaNathalie Le Floch, Université de Versailles Saint-Quentin-en-Yvelines, France
Copyright © 2023 Xu, Zhou, Wang and Mo. This is an open-access article distributed under the terms of the Creative Commons Attribution License (CC BY). The use, distribution or reproduction in other forums is permitted, provided the original author(s) and the copyright owner(s) are credited and that the original publication in this journal is cited, in accordance with accepted academic practice. No use, distribution or reproduction is permitted which does not comply with these terms.
*Correspondence: Chunheng Mo, Y2h1bmhlbmdtb0BnbWFpbC5jb20=; Xiaodong Wang, d2FuZ3hkX3NjdUBzaW5hLmNvbSYjeDAyMDBhOw==