- 1Graduate School of Life Science and Engineering, Iryo Sosei University, Fukushima, Japan
- 2Research Institute of Innovative Medicine, Tokiwa Foundation, Fukushima, Japan
- 3School of Medicine, Fukushima Medical University, Fukushima, Japan
Androgen and androgen deprivation (castration) therapies, including androgen receptor antagonists, are clinically used to treat patients with prostate cancer. However, most hormone-dependent prostate cancer patients progress into a malignant state with loss of hormone-dependency, known as castration (drug)-resistant prostate cancer (CRPC), after prolong androgen-based treatments. Even in the CRPC state with irreversible malignancy, androgen receptor (AR) expression is detectable. An epigenetic transition to CRPC induced by the action of AR-mediated androgen could be speculated in the patients with prostate cancer. Androgen receptors belongs to the nuclear receptor superfamily with 48 members in humans, and acts as a ligand-dependent transcriptional factor, leading to local chromatin reorganization for ligand-dependent gene regulation. In this review, we discussed the transcriptional/epigenetic regulatory functions of AR, with emphasis on the clinical applications of AR ligands, AR protein co-regulators, and AR RNA coregulator (enhancer RNA), especially in chromatin reorganization, in patients with prostate cancer.
1 Introduction
Prostate cancer is a disease with several symptoms suffering over a million of men worldwide with death of over 0.3 million patients. However, the disease is curable if diagnosed early, with improved overall long-term survival in patients. The early developmental stage of prostate cancer is dependent on androgen. Therefore, hormone deprivation therapy is effective to attenuate cancer development, while adverse effects including hot flashes, anorexia and osteopenia are often seen. As standard treatments, pharmacological and surgical methods are used. Although hormone deprivation therapy is effective at the early stages, later most prostate cancer cases are transited into a hormone-independent prostate cancer state known as castration (drug)-resistant prostate cancer (CRPC) (Scher et al., 2012; Attard et al., 2016; Li et al., 2016c). Chemotherapy is clinically successful against CRPC; however, some CRPC patients develop metastatic CRPC (mCRPC), making effective treatment difficult to achieve. Thus, inhibiting the transition from a hormone-dependent to hormone-independent state is necessary to improve the quality of life (QOL) of patients. However, studies on strategies to inhibit the transition process are still preliminary, and the molecular mechanisms are yet to be elucidated. Recently, clinical studies have identified the importance of androgen signaling and the expression of androgen receptor (AR) in the transition from a hormone-dependent to hormone-independent state (Henshall et al., 2001; Sharifi, 2013; Antonarakis et al., 2014). In this review, we discussed the epigenetic function of AR in androgen signaling and its role in the transition process from hormone-dependent to hormone-independent prostate cancer.
1.1 The transition from hormone-dependent to castration (drug)-resistant prostate cancer (CRPC)
Androgen deprivation drugs, such as androgen antagonists and enzyme inhibitors of androgen biosynthesis, are effective against prostate cancer in the early stages (Scher et al., 2012; Attard et al., 2016; Li et al., 2016c). For example, the AR antagonists bicalutamide and flutamide were developed to antagonize endogenous androgen-induced activation of the gene regulatory function of AR, and have been clinically applied. Despite promising results, the efficacy of bicalutamide and flutamide is generally poor, and prolong used can cause drug resistance (Rice et al., 2019). To overcome this issue, a third-generation nonsteroidal antiandrogen (NSAA), darolutamide, has been developed (Crawford et al., 2019). Recently, other classes of androgen antagonists (enzalutamide) have been successfully developed, with better clinical outcomes in patients with CRPC than the AR antagonists (Scher et al., 2012). At the molecular level, enzalutamide anchors AR in cytosol even after AR binding, thereby blocking the action of endogenous androgens in prostate tumors (Nakazawa et al., 2014). Overall, enzalutamide treatment has been clinically more effective than the traditional bicalutamide and flutamide treatments in patients with CRPC and mCRPC (Li et al., 2016c). Unlike the AR antagonists, enzalutamide is an enzymatic inhibitor for p450 17A1 (CYP17) capable of irreversibly blocking steroid conversion in the biosynthesis pathway of active androgens in prostatic tumor cells (Li et al., 2016c). Although both enzalutamide and abiraterone have shown high efficacy against prostate cancer in clinical trials, they are unable to entirely eradicate prostate cancer (Romanel et al., 2015; Wang et al., 2021). Moreover, a radiopharmaceutical drug called pluvicto (lutetium Lu 177, vipivotide tetraxetan) has recently been evaluated with clinical success (Neels et al., 2021), indicating that new generation drugs with similar or distinct modes of action with the androgen deprivation drugs may be effective against prostate cancer.
1.2 Epigenetic transition during prostate cancer development
Despite the clinical application of new-generation drugs, some patients with hormone (androgens) sensitive prostate cancer (HSPC) become insensitive to androgen deprivation drugs after prolonged treatment. The transition from HSPC to CRPC is irreversible and is accompanied by alterations in gene expression profile, which may affect the chromatin landscape or follow the altered chromatin landscape (Malik et al., 2015; Cyrta et al., 2020). Over the years, the molecular mechanisms underlying cancer development and progression have been extensively studied. For example, several aberrant events in cellular signaling and DNA-templated biological processes, including transcription, DNA replication, and repair, have been identified in cancers, including prostate cancer (Gavande et al., 2016; Elsesy et al., 2020; Zhang et al., 2020). The DNA repair process includes specific histone modifications and chromatin remodeling at damaged chromatin regions, and DNA repair-induced epigenetic modifications have been observed in patients receiving radiotherapy (Clouaire et al., 2018). Moreover, a dysfunction in the regulation of DNA repair may occur during cancer development (Torgovnick and Schumacher, 2015).
Consistent with the role of DNA methylation in the inactivation of local chromatin state, DNA hypermethylation was observed in the promoters of the genes SOSTDC1 and FLT4, and was associated with prostate cancer development (Rauluseviciute et al., 2020). Previous studies have shown that DNA methyltransferases were upregulated and histone modifications were modulated in prostate cancers (Patra et al., 2002; Seligson et al., 2005; Rauluseviciute et al., 2020). Although the molecular basis for the local rearrangement of histone marks (aberrant profiles of histone methylation marks) is diverse and poorly understood, studies suggest that aberrant expressions and de novo genetic mutations in histone modifiers, such as LSD1 (an H3K4 methytransferase) and EZH1/2 (the sole H3K27 methytransferasess), may play a role (Metzger et al., 2005; Duan et al., 2020). Overall, these findings indicate that there are several alterations in epigenetic events and the related regulatory functions during prostate cancer development. Since the transition of prostate cancer into CRPC is irreversible and is associated with AR-mediating androgen signaling, we focused on the functions of AR and its co-regulators in prostate cancer development in this review.
1.3 Androgen signaling in prostate cancer development and drug resistance
The prostate is a part of the male reproductive system and requires androgen for tissue development and maintenance (Kawano et al., 2003; Matsumoto et al., 2013). In addition to the male reproductive organs, the brain, skeleton, adipose, and several organs are targets of androgen, and treatment with androgen antagonists can cause side effects, such as hot flashes, bone loss, and weight gain (Grossmann and Zajac, 2011; Nguyen et al., 2015). Androgen serves as a prime endocrine male steroid hormone, and active androgens specifically bind to AR, increasing the accumulation of activated AR in the nucleus to direct gene regulatory program in a spatial- and temporal-manner. Unlike estrogen, which has two types of nuclear receptors, androgens have only one receptor (AR) in androgen signaling (Kawano et al., 2003; Matsumoto et al., 2013). The important role of AR in androgen signaling is supported by clinical observations in patients that are genetically deficient in AR function and in transgenic mouse models (Matsumoto et al., 2013; Hayakawa et al., 2022).
Accumulating evidence indicates that androgen signaling and AR expression play important roles in prostate cancer, even after the transition into the CRPC state. For example, an increase in AR gene expression and mutations in cancer driver genes, such as PTEN, MYC, and TP53, are often observed in progressive CRPC (Takeda et al., 2018; Viswanathan et al., 2018). In breast cancer, ERα is a clinical standard marker, and its loss predicts a transition into a drug-resistant state (Kuukasjärvi et al., 1996). In contrast, AR expression is detected in prostatic tumors even in the advanced stages, indicating that a loss of AR expression is not a clinical marker for prostate cancer. However, the AR mRNA variant AR-V7 is known to appear only during transition to CRPC (Henshall et al., 2001; Sharifi, 2013; Antonarakis et al., 2014). Since the AR-V7 protein does not possess a ligand binding domain and is assumed to act as a constitutively active receptor (Figure 1), it was recently characterized as an AR repressor (Cato et al., 2019), indicating that its expression inhibits AR-mediated androgen signaling in CRPC.
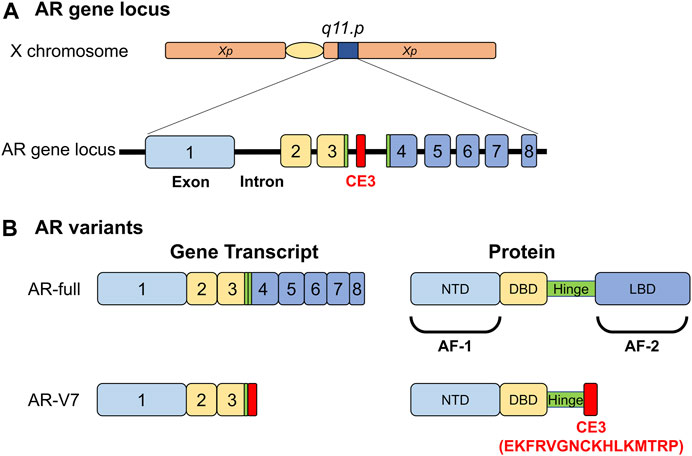
FIGURE 1. Functional structures of androgen receptor (AR) and its variant (AR-V7) selectively emerging in malignant prostatic tumors. (A) The human gene locus of the androgen receptor. CE; AR-V7- specific exon. (B) Functional structure of AR and AR-V7. NTD; N-terminal end: DBD; DNA-binding domain: LBD; Ligand-binding domain: AF-1; Autonomous transactivation function-1: AF-2; Autonomous transactivation function-2.
1.4 Androgen receptor function in androgen signaling
Androgen receptors are a pivotal factor in androgen signaling under physiological settings (Kawano et al., 2003; Matsumoto et al., 2013), as evidenced by phenotypic abnormalities in AR-deficient humans and mice (Kawano et al., 2003; Shiina et al., 2006; Matsumoto et al., 2013). Additionally, the importance of AR in the male external genitalia and reproductive organs, including the prostate, has been well illustrated in mouse lines with genetically disrupted AR gene (Kawano et al., 2003; Sato et al., 2004). Androgen receptors are important for developing and maintaining male reproductive organs in mammalians. Moreover, AR deficiency has been shown to affect other biological activities in humans and mice, including bone remodeling, energy consumption, and sexual behaviors (Hughes et al., 2012; Matsumoto et al., 2013). Accumulating evidence suggests that the androgen signaling pathway exerts its functions by mediating AR-related gene regulatory networks (genomic pathway); however, a non-genomic pathway via a cell membrane receptor has been hypothesized but is yet to be thoroughly studied. The active form of androgen circulating in mammalian serum is dihydrotestosterone (DHT), and the other forms serve as endogenous AR ligands with weak biological activities (Matsumoto et al., 2013; Bulun, 2014; Hayakawa et al., 2022). Most AR molecules are localized in the cytosol, but the binding of androgens translocates AR into the nucleus for gene regulation.
Androgen receptors belong to the nuclear receptor (NR) superfamily consisting of 48 members in humans and act as DNA-binding transcription regulatory factors (Figure 1) (Mangelsdorf et al., 1995; Hayakawa et al., 2022). Similar to other NR members, the AR protein is divided into functional domains from A to E, with the central C domain composed of two zinc finger motifs necessary for recognizing and binding to the target DNA sequence. The N-terminal A/B and E domains encompass autonomous activation functions (originally named as AF-1 and AF-2 domains) and are regarded as the docking sites for AR co-regulators (Hayakawa et al., 2022). In the absence of AR agonists, direct and stable DNA binding of AR homodimer is evident in the androgen response enhancer element (ARE) composed of 5′-AGAACANNNTGTTCT-3′ motif or on the related sequences. However, in vivo whole genome analyses using chromatin immunoprecipitation sequence (ChIP-seq) and other approaches have shown that AR binds with chromatic DNA sequences that often encompass half of the core motif (5′-AGAACA-3′) of ARE (Lupien et al., 2008). AR binding sites are highly overlapped and adjacent to the binding site of forkhead box A1 (FOXA1). A pioneer factor such as FOXA1 is believed to remodel chromatin locally for efficient DNA binding of AR (Figure 2) (Lupien et al., 2008; Hayakawa et al., 2022). The chromatin environment surrounding AR binding sites is diverse (Chen and Dent, 2014); hence, FOXA1 acts as a pioneer factor for AR-dependent activation of gene expression and facilitates AR DNA binding (Lupien et al., 2008).
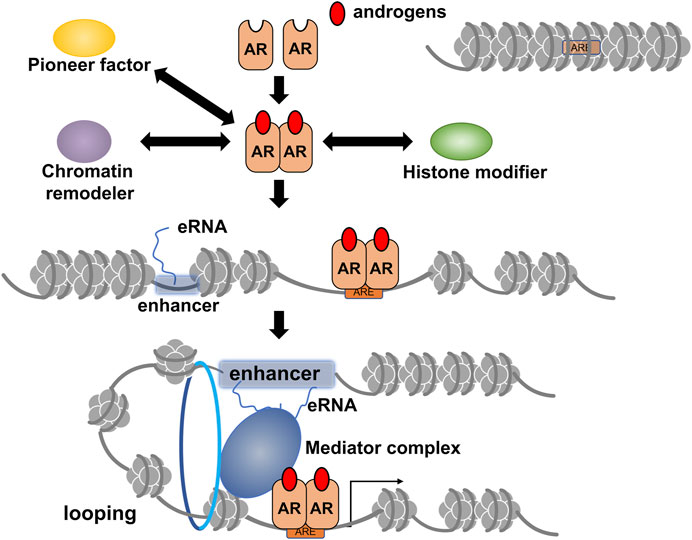
FIGURE 2. A schematic of ligand-dependent gene regulation by androgen receptor (AR) through epigenetic modifications and chromatin reorganization. The AR target site surrounding inactivated chromatin array is opened through chromatin remodeling by the activities of chromatin remodelers, histone modifiers, and pioneer factors, such as forkhead box A1 (FOXA1). Details of chromatin remodelers, histone modifiers, and transcriptional co-regulators involved in androgen-induced gene regulation by AR are described in Table 1. Formation of chromatin looping is induced for efficient transcription by activated AR, and enhancer RNA transcribed from potent enhancers, such as super-enhancer (SE), facilitates this process. Notably, it is currently unclear whether chromatin opening for AR DNA binding is associated with the eRNA-induced formation of chromatin looping.
1.5 Androgen/AR target genes
Canonical AR target genes, including KLK3 (coding PSA), SGK, and TIPARP (Bolton et al., 2007) are involved in exerting the biological actions of androgen. However, AR binding sites that have been successfully mapped by whole genome sequencing are mainly located in the intergenic regions, with more than 80% of the human genome harboring for non-coding RNAs (ncRNAs) (Cech and Steitz, 2014; Knoll et al., 2015). Some ncRNAs regulate gene expression at transcriptional and post-transcriptional levels. Among the several classes of ncRNAs, enhancer RNA (eRNAs) are transcribed by RNA polymerase II with high RNA turnovers from potent enhancers like super-enhancers (SEs) (Li et al., 2016b; Guan et al., 2018). Among enhancers facilitating AR-mediated transcription, a set of eRNAs was identified as androgen-inducible, and they promote androgen-induced expression of the AR target mRNAs by inducing chromatin looping for efficient transcription (Figure 2) (Hsieh et al., 2014; Nair et al., 2019; Sawada et al., 2021). Given the fact that the transcription of most ncRNAs is achieved using RNA polymerase II, the other classes of ncRNAs, such as long non-coding RNAs (lncRNAs), could be the targets of androgen-bound AR. Since 90% of the gene loci related to hereditary and chronic diseases are present in the ncRNA-coding regions of the human genome (Cech and Steitz, 2014; Knoll et al., 2015), these ncRNAs are believed to exhibit specific biological actions. Moreover, it is speculated that the biological activity of androgen is partly due to the functions of AR-regulated ncRNAs; however, further studies are necessary to validate this hypothesis.
1.6 AR co-regulators facilitate chromatin remodeling for gene regulation
Activated AR binds to chromatin through direct DNA binding or protein-protein interaction, depending on the chromatin environment (Kato et al., 2011; Hayakawa et al., 2022). If AR binding sites are within the activated chromatin-like euchromatic regions, rapid response to androgens in AR-mediated transcriptional regulation is achievable. Histone acetylation/deacetylation without dynamic chromatin reorganization is assumed to be sufficient for these transcriptional regulations (Hayakawa et al., 2022). In contrast, when the AR target sites are in the heterochromatinized regions, chromatin reorganization is required to facilitate AR binding using several AR co-regulators. FOXA1 binding motifs are often observed in the vicinity of AR binding sites. Since FOXA1 has an intrinsic function to remodel nucleosome arrays to facilitate AR binding (Figure 2), FOXA1 is regarded as a pioneer factor for AR-dependent activation of gene expression (Lupien et al., 2008). Two classes of AR co-regulators are involved in chromatin remodeling Table 1): histone modifiers and chromatin remodelers (Rosenfeld et al., 2006; Chen and Dent, 2014; Hayakawa et al., 2022). Both co-regulators are often present as large complexes of multiple subunits with protein motifs capable of recognizing modified histone residues. Direct and androgen-induced association of these co-regulators with AR has been eperimentally proven; moreover, the AR-coregulator complexes act as functional enzymatic units to modify histone marks (Rosenfeld et al., 2006; Kato et al., 2011; Chen and Dent, 2014). Reflecting the diversity of histone codes (histone modifications), several histone modifiers facilitating gene expression have been characterized (Rosenfeld et al., 2006; Kato et al., 2011; Chen and Dent, 2014), most of which are involved in the coregulation of AR function in androgen-regulated gene expression. Histone acetyltransferases (HATs) and histone deacetylases (HDACs) are considered effective histone modifiers for rapid gene regulation by AR (Rosenfeld et al., 2006; Kato et al., 2011). Apart from histone acetylation, a HAT (p300) was recruited to AR in an androgen-dependent manner, thereby acetylating AR to potentiate androgen-induced gene expression (Thompson et al., 2022). However, histone lysine methyltransferases (HKMTs) and histone demethylases (HDMs) act as pivotal AR co-regulators in instances where chromatin reorganization is requisite, consistent with the importance of methylated states of histone H3 K4, K9, K27 and K36 residues (Greer and Shi, 2012; Hayakawa et al., 2022). Additionally, several animal experiments have elucidated the roles of HKMTs and HDMs in prostate cancer development (Tong et al., 2016; Huang and Xu, 2017; Vatapalli et al., 2020). LSD1 was initially reported to act as an AR co-activator via demethylation of methylated H3K9 residue, along with upregulated expression of LSD1 in malignant prostate tumors (Metzger et al., 2005). However, the HDM activity of LSD1 for methylated H3K4 residue has been observed in other types of tumors (Hayami et al., 2011). Moreover, studies have shown a correlation between the expression levels of enzymes facilitating AR function in prostatic tumors and the malignancy of the tumor (Tong et al., 2016; Huang and Xu, 2017; Vatapalli et al., 2020). Accordingly, alterations in the expression levels and genetic mutations of the related enzymes were associated with life prognosis in prostate cancer patients (Tong et al., 2016; Huang and Xu, 2017; Vatapalli et al., 2020). Since the development of prostate cancer and the transition to the CRPC state are irreversible, the dynamic reorganization of the whole genome landscape is conceivable, and HKMTs and HDMs may regulate these epigenetic processes by redirecting histone code combinations.
Chromatin remodeling complexes conduct actual chromatin remodeling in an ATP-dependent manner (Chen and Dent, 2014). Hence, each complex contains ATPase as a critical driver for chromatin remodeling for gene regulation, although several complexes have distinct ATPases. Multi-faceted surfaces of the remodeler complexes may be advantageous in protein-protein interaction owing to multiple subunit assemblies (Kato et al., 2011; Hayakawa et al., 2022), enabling the complexes to stably and transiently associate with chromatin and other AR co-regulators (Figure 2). Among the remodeler complexes, direct interaction of AR with two types of SWI/SNF complexes has been reported (Ding et al., 2019; Cyrta et al., 2020). Recently, the pivotal role of this complex in AR-mediated prostatic tumor development was demonstrated using a compound (AU-15330) capable of selective degradation of SWI/SNF ATPases (Xiao et al., 2022). Treatment with AU-15330 induced selective proteolysis of the core subunits (SMARCA2 and SMARCA4) and promoted the dislodging of AR and FOXA1 from chromatin in prostate cancer cell lines and suppressed the growth of xenograft prostatic tumors in mice. Additionally, combined treatment with AU-15330 and a clinically used AR antagonist (enzalutamide) successfully reduced tumor volume within 3 months (Xiao et al., 2022). Overall, these results suggest that dynamic chromatin reorganization is involved in prostate tumor progression.
1.7 Enhancer RNA (eRNA) as AR co-regulator for gene regulation
Locally looped chromatin between the promoter region and enhancer(s) harboring AR binding sites in the target gene loci are important in initiating efficient transcription in response to androgens. Consistently, AR bindings have been identified in the multiple sites over the enhancers of the KLK3 and KLK4 (Hsieh et al., 2014; Sawada et al., 2021; Takayama et al., 2021). Recent findings suggest that ncRNAs transcribed from potent enhancers, such as SEs, assist in looping chromatin with the aid of mediator and co-cohesion complexes (Li et al., 2016b; Guan et al., 2018; Nair et al., 2019). Notably, a class of ncRNAs known as androgen-inducible eRNAs facilitates androgen-induced prostate-specific antigen [PSA (KLK3)] gene expression in human prostate cancer cell lines (Hsieh et al., 2014; Sawada et al., 2021). Moreover, androgen-inducible eRNAs have been reported to act as a trigger to form a massive transcription initiation complex with chromatin looping via a liquid-liquid phase separation (LLPS) state (Figure 2) (Takayama et al., 2021). The transcription initiation complex contains AR, eRNAs, mediator complex components, and HAT CBP/p300, indicating that the complex also serves as a histone modifier unit. Additionally, this vast complex may combine with a canonical transcription initiation complex consisting of a mediator complex, fundamental transcription factors, and RNA polymerase II. Overall, eRNA may be considered an RNA co-regulator for AR in addition to acting as a protein transcription co-regulator (Hsieh et al., 2014; Sawada et al., 2021; Takayama et al., 2021).
1.8 Mode of action of the clinically applied AR antagonists
Since prostate cancer is androgen-dependent at early stages, androgen-deprivation therapy is currently used in patients. The most common therapy is based on enzyme inhibitor treatments and AR antagonists (Sumanasuriya and De Bono, 2018), one of which is an inhibitor of a P450 enzyme (CYP17) converting precursors into steroids, leading to the deprivation of locally produced androgen in prostatic tumors (Matsumoto et al., 2013). These enzyme inhibitors are effective as chemical androgen inhibitors, with fewer side effects than other enzyme inhibitors and orchidectomy.
AR antagonists can be classified into two classes, with both classes exhibiting antagonistic actions against endogenous androgens in terms of activation of AR function. However, a recently developed AR antagonist called enzalutamide acts by blocking AR translocation from the cytosol to the nucleus, irrespective of AR binding, in a manner different from the mode of action of the canonical AR antagonists flutamide and bicalutamide (Scher et al., 2012; Sumanasuriya and De Bono, 2018). Thus, enzalutamide is regarded as a functional inhibitor of AR by blocking nuclear transport. The canonical AR antagonists are competitive in androgen binding and inhibitory for the transactivation function of AR. However, these antagonists are incapable of blocking nuclear transport coupled with ligand binding-induced alteration(s) in AR structure. The clinical outcomes and gene expression profiles of prostate cancer patients support the clinical benefits of these two types of AR antagonists (Sumanasuriya and De Bono, 2018; Rice et al., 2019). However, studies are yet to elucidate why prostatic tumors successfully treated with chemical therapy are prone to be malignant after specific periods (Pimenta et al., 2022).
1.9 Chromatin remodeling by AR synthetic ligands
CRPC often develops after androgen deprivation therapy, and the acquired hormone resistance is irreversible (Scher et al., 2012; Attard et al., 2016; Li et al., 2016c). Based on changes in gene expression profiles during the progression of prostate tumors (Wang et al., 2009; Cyrta et al., 2020), it is speculated that the transition to the CRPC state is highly associated with chromatin reorganization. Similar to other types of cancer, epigenetic regulators are involved in prostate cancer, and their malfunction has been well-documented (Gao and Alumkal, 2010; Thompson et al., 2022). Recently, the effect of a canonical AR antagonist (bicalutamide: Bic) in chromatin remodeling and expression profile in a human prostate cancer cell line (LNCaP cells) was examined (Sawada et al., 2022). ATAC-seq analysis showed that Bic-induced rearrangement pattern of the chromatin array was different from DHT-induced rearrangement (Figure 3), indicating that Bic is also effective in remodeling the chromatin array. Additionally, Bic acted as a transcriptional antagonist for AR function (Sawada et al., 2022). Although it is unclear if other AR antagonists are capable of remodeling the chromatin array, clinical studies have shown that these treatments modulate the chromatin landscape. In this respect, further studies of the epigenetic actions of the AR antagonists are necessary to improve the understanding of the molecular mechanism of the transition from a hormone-dependent to hormone-resistant state in prostatic tumors (Figure 4).
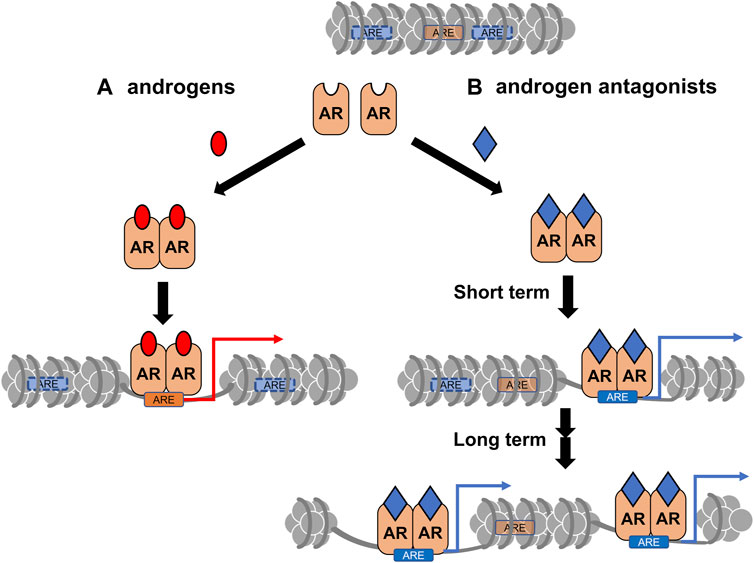
FIGURE 3. A schematic of differential chromatin remodeling by androgens vs. androgen receptor (AR) antagonists. (A) The canonical remodeling by AR-bound androgens (red). (B) The canonical target sites (ARE with orange) for AR-bound androgens could be closed by chromatin reorganization induced by the antagonist (blue) -bound AR. Cryptic AR target sites (ARE with blue) could be opened for AR binding.
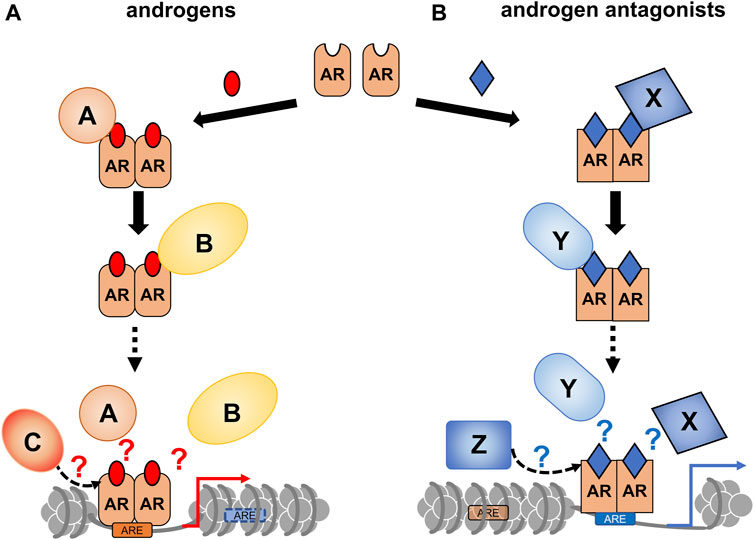
FIGURE 4. A schematic of androgen receptor (AR)-coregulator-mediated chromatin remodeling by ligand binding. A ligand type-specific set of AR co-regulators facilitate ligand type-specific chromatin reorganization. Associations of AR with co-regulators are differentially induced by AR ligands. In order to reorganize the chromatin environment, multiple co-regulators appear to be transiently and sequentially recruited for ligand-bound AR. (A) The canonical recruitment of co-regulators by androgen binding is depicted. (B) Androgen antagonists are potent to recruit non-canonical co-regulators, thereby leading atypical chromatin remodeling.
Author contributions
TS, YK, and TK prepared the figures and table. SK conceived and wrote the manuscript. YK and TK checked the citation.
Funding
This study was financially supported by a grant from the Tokiwa Foundation (SK) and the practical development projects by Fukushima prefecture for the Research Institute of Innovative Medicine, Tokiwa Foundation.
Acknowledgments
The authors thank all the past and present laboratory members who supported this study.
Conflict of interest
The authors declare that the research was conducted in the absence of any commercial or financial relationships that could be construed as a potential conflict of interest.
Publisher’s note
All claims expressed in this article are solely those of the authors and do not necessarily represent those of their affiliated organizations, or those of the publisher, the editors and the reviewers. Any product that may be evaluated in this article, or claim that may be made by its manufacturer, is not guaranteed or endorsed by the publisher.
References
Antonarakis, E. S., Lu, C., Wang, H., Luber, B., Nakazawa, M., Roeser, J. C., et al. (2014). AR-V7 and resistance to enzalutamide and abiraterone in prostate cancer. N. Engl. J. Med. 371, 1028–1038. doi:10.1056/NEJMoa1315815
Asangani, I. A., Ateeq, B., Cao, Q., Dodson, L., Pandhi, M., Kunju, L. P., et al. (2013). Characterization of the EZH2-MMSET histone methyltransferase regulatory axis in cancer. Mol. Cell. 49, 80–93. doi:10.1016/j.molcel.2012.10.008
Askew, E. B., Bai, S., Parris, A. B., Minges, J. T., and Wilson, E. M. (2017). Androgen receptor regulation by histone methyltransferase Suppressor of variegation 3-9 homolog 2 and Melanoma antigen-A11. Mol. Cell. Endocrinol. 443, 42–51. doi:10.1016/j.mce.2016.12.028
Attard, G., Parker, C., Eeles, R. A., Schröder, F., Tomlins, S. A., Tannock, I., et al. (2016). Prostate cancer. Lancet 387, 70–82. doi:10.1016/S0140-6736(14)61947-4
Augello, M. A., Liu, D., Deonarine, L. D., Robinson, B. D., Huang, D., Stelloo, S., et al. (2019). CHD1 loss alters AR binding at lineage-specific enhancers and modulates distinct transcriptional programs to drive prostate tumorigenesis. Cancer Cell. 35, 603–617. doi:10.1016/j.ccell.2019.03.001
Bali, P., Pranpat, M., Bradner, J., Balasis, M., Fiskus, W., Guo, F., et al. (2005). Inhibition of histone deacetylase 6 acetylates and disrupts the chaperone function of heat shock protein 90: A novel basis for antileukemia activity of histone deacetylase inhibitors. J. Biol. Chem. 280, 26729–26734. doi:10.1074/jbc.C500186200
Bolton, E. C., So, A. Y., Chaivorapol, C., Haqq, C. M., Li, H., and Yamamoto, K. R. (2007). Cell- and gene-specific regulation of primary target genes by the androgen receptor. Genes. Dev. 21, 2005–2017. doi:10.1101/gad.1564207
Bulun, S. E. (2014). Aromatase and estrogen receptor α deficiency. Fertil. Steril. 101, 323–329. doi:10.1016/j.fertnstert.2013.12.022
Cao, Z., Shi, X., Tian, F., Fang, Y., Wu, J. B., Mrdenovic, S., et al. (2021). KDM6B is an androgen regulated gene and plays oncogenic roles by demethylating H3K27me3 at cyclin D1 promoter in prostate cancer. Cell. Death Dis. 12, 2. doi:10.1038/s41419-020-03354-4
Cato, L., de Tribolet-Hardy, J., Lee, I., Rottenberg, J. T., Coleman, I., Melchers, D., et al. (2019). ARv7 represses tumor-suppressor genes in castration-resistant prostate cancer. Cancer Cell. 35, 401–413. doi:10.1016/j.ccell.2019.01.008
Cech, T. R., and Steitz, J. A. (2014). The noncoding RNA revolution-trashing old rules to forge new ones. Cell. 157, 77–94. doi:10.1016/j.cell.2014.03.008
Chen, T., and Dent, S. Y. R. (2014). Chromatin modifiers and remodellers: Regulators of cellular differentiation. Nat. Rev. Genet. 15, 93–106. doi:10.1038/nrg3607
Chen, L., Meng, S., Wang, H., Bali, P., Bai, W., Li, B., et al. (2005). Chemical ablation of androgen receptor in prostate cancer cells by the histone deacetylase inhibitor LAQ824. Mol. Cancer Ther. 4, 1311–1319. doi:10.1158/1535-7163.MCT-04-0287
Cho, S., Park, J. S., and Kang, Y.-K. (2014). AGO2 and SETDB1 cooperate in promoter-targeted transcriptional silencing of the androgen receptor gene. Nucleic Acids Res. 42, 13545–13556. doi:10.1093/nar/gku788
Clouaire, T., Rocher, V., Lashgari, A., Arnould, C., Aguirrebengoa, M., Biernacka, A., et al. (2018). Comprehensive mapping of histone modifications at DNA double-strand breaks deciphers repair pathway chromatin signatures. Mol. Cell. 72, 250–262. doi:10.1016/j.molcel.2018.08.020
Coffey, K., Rogerson, L., Ryan-Munden, C., Alkharaif, D., Stockley, J., Heer, R., et al. (2013). The lysine demethylase, KDM4B, is a key molecule in androgen receptor signalling and turnover. Nucleic Acids Res. 41, 4433–4446. doi:10.1093/nar/gkt106
Crawford, E. D., Heidenreich, A., Lawrentschuk, N., Tombal, B., Pompeo, A. C. L., Mendoza-Valdes, A., et al. (2019). Androgen-targeted therapy in men with prostate cancer: Evolving practice and future considerations. Prostate Cancer Prostatic Dis. 22, 24–38. doi:10.1038/s41391-018-0079-0
Cyrta, J., Augspach, A., De Filippo, M. R., Prandi, D., Thienger, P., Benelli, M., et al. (2020). Role of specialized composition of SWI/SNF complexes in prostate cancer lineage plasticity. Nat. Commun. 11, 5549. doi:10.1038/s41467-020-19328-1
Dai, Y., Ngo, D., Forman, L. W., Qin, D. C., Jacob, J., and Faller, D. V. (2007). Sirtuin 1 is required for antagonist-induced transcriptional repression of androgen-responsive genes by the androgen receptor. Mol. Endocrinol. 21, 1807–1821. doi:10.1210/me.2006-0467
Deng, X., Shao, G., Zhang, H.-T., Li, C., Zhang, D., Cheng, L., et al. (2017). Protein arginine methyltransferase 5 functions as an epigenetic activator of the androgen receptor to promote prostate cancer cell growth. Oncogene 36, 1223–1231. doi:10.1038/onc.2016.287
Ding, Y., Li, N., Dong, B., Guo, W., Wei, H., Chen, Q., et al. (2019). Chromatin remodeling ATPase BRG1 and PTEN are synthetic lethal in prostate cancer. J. Clin. Invest. 129, 759–773. doi:10.1172/JCI123557
Draker, R., Sarcinella, E., and Cheung, P. (2011). USP10 deubiquitylates the histone variant H2A.Z and both are required for androgen receptor-mediated gene activation. Nucleic Acids Res. 39, 3529–3542. doi:10.1093/nar/gkq1352
Duan, L., Rai, G., Roggero, C., Zhang, Q.-J., Wei, Q., Ma, S. H., et al. (2015). KDM4/JMJD2 histone demethylase inhibitors block prostate tumor growth by suppressing the expression of AR and BMYB-regulated genes. Chem. Biol. 22, 1185–1196. doi:10.1016/j.chembiol.2015.08.007
Duan, R., Du, W., and Guo, W. (2020). EZH2: A novel target for cancer treatment. J. Hematol. Oncol. 13, 104. doi:10.1186/s13045-020-00937-8
Elsesy, M. E., Oh-Hohenhorst, S. J., Löser, A., Oing, C., Mutiara, S., Köcher, S., et al. (2020). Second-generation antiandrogen therapy radiosensitizes prostate cancer regardless of castration state through inhibition of DNA double strand break repair. Cancers 12, 2467. doi:10.3390/cancers12092467
Ezponda, T., Popovic, R., Shah, M. Y., Martinez-Garcia, E., Zheng, Y., Min, D.-J., et al. (2013). The histone methyltransferase MMSET/WHSC1 activates TWIST1 to promote an epithelial-mesenchymal transition and invasive properties of prostate cancer. Oncogene 32, 2882–2890. doi:10.1038/onc.2012.297
Faus, H., Meyer, H.-A., Huber, M., Bahr, I., and Haendler, B. (2005). The ubiquitin-specific protease USP10 modulates androgen receptor function. Mol. Cell. Endocrinol. 245, 138–146. doi:10.1016/j.mce.2005.11.011
Fu, M., Wang, C., Reutens, A. T., Wang, J., Angeletti, R. H., Siconolfi-Baez, L., et al. (2000). p300 and p300/cAMP-response element-binding protein-associated factor acetylate the androgen receptor at sites governing hormone-dependent transactivation. J. Biol. Chem. 275, 20853–20860. doi:10.1074/jbc.M000660200
Fu, M., Rao, M., Wang, C., Sakamaki, T., Wang, J., Di Vizio, D., et al. (2003). Acetylation of androgen receptor enhances coactivator binding and promotes prostate cancer cell growth. Mol. Cell. Biol. 23, 8563–8575. doi:10.1128/mcb.23.23.8563-8575.2003
Fu, M., Liu, M., Sauve, A. A., Jiao, X., Zhang, X., Wu, X., et al. (2006). Hormonal control of androgen receptor function through SIRT1. Mol. Cell. Biol. 26, 8122–8135. doi:10.1128/MCB.00289-06
Gao, L., and Alumkal, J. (2010). Epigenetic regulation of androgen receptor signaling in prostate cancer. Epigenetics 5, 100–104. doi:10.4161/epi.5.2.10778
Gaughan, L., Logan, I. R., Cook, S., Neal, D. E., and Robson, C. N. (2002). Tip60 and histone deacetylase 1 regulate androgen receptor activity through changes to the acetylation status of the receptor. J. Biol. Chem. 277, 25904–25913. doi:10.1074/jbc.M203423200
Gaughan, L., Stockley, J., Wang, N., McCracken, S. R. C., Treumann, A., Armstrong, K., et al. (2011). Regulation of the androgen receptor by SET9-mediated methylation. Nucleic Acids Res. 39, 1266–1279. doi:10.1093/nar/gkq861
Gavande, N. S., VanderVere-Carozza, P. S., Hinshaw, H. D., Jalal, S. I., Sears, C. R., Pawelczak, K. S., et al. (2016). DNA repair targeted therapy: The past or future of cancer treatment? Pharmacol. Ther. 160, 65–83. doi:10.1016/j.pharmthera.2016.02.003
Gibbs, A., Schwartzman, J., Deng, V., and Alumkal, J. (2009). Sulforaphane destabilizes the androgen receptor in prostate cancer cells by inactivating histone deacetylase 6. Proc. Natl. Acad. Sci. U. S. A. 106, 16663–16668. doi:10.1073/pnas.0908908106
Giles, K. A., Gould, C. M., Achinger-Kawecka, J., Page, S. G., Kafer, G. R., Rogers, S., et al. (2021). BRG1 knockdown inhibits proliferation through multiple cellular pathways in prostate cancer. Clin. Epigenetics 13, 37. doi:10.1186/s13148-021-01023-7
Greer, E. L., and Shi, Y. (2012). Histone methylation: A dynamic mark in health, disease and inheritance. Nat. Rev. Genet. 13, 343–357. doi:10.1038/nrg3173
Grossmann, M., and Zajac, J. D. (2011). Management of side effects of androgen deprivation therapy. Endocrinol. Metab. Clin. North Am. 40, 655–671. doi:10.1016/j.ecl.2011.05.004
Guan, D., Xiong, Y., Borck, P. C., Jang, C., Doulias, P.-T., Papazyan, R., et al. (2018). Diet-induced circadian enhancer remodeling synchronizes opposing hepatic lipid metabolic processes. Cell. 174, 831–842. doi:10.1016/j.cell.2018.06.031
Hayakawa, A., Kurokawa, T., Kanemoto, Y., Sawada, T., Mori, J., and Kato, S. (2022). Skeletal and gene-regulatory functions of nuclear sex steroid hormone receptors. J. Bone Min. Metab. 40, 361–374. doi:10.1007/s00774-021-01306-2
Hayami, S., Kelly, J. D., Cho, H.-S., Yoshimatsu, M., Unoki, M., Tsunoda, T., et al. (2011). Overexpression of LSD1 contributes to human carcinogenesis through chromatin regulation in various cancers. Int. J. Cancer 128, 574–586. doi:10.1002/ijc.25349
Henshall, S. M., Quinn, D. I., Lee, C. S., Head, D. R., Golovsky, D., Brenner, P. C., et al. (2001). Altered expression of androgen receptor in the malignant epithelium and adjacent stroma is associated with early relapse in prostate cancer. Cancer Res. 61, 423–427.
Hong, Z., Xiang, Z., Zhang, P., Wu, Q., Xu, C., Wang, X., et al. (2021). Histone acetyltransferase 1 upregulates androgen receptor expression to modulate CRPC cell resistance to enzalutamide. Clin. Transl. Med. 11, e495. doi:10.1002/ctm2.495
Hsieh, C.-L., Fei, T., Chen, Y., Li, T., Gao, Y., Wang, X., et al. (2014). Enhancer RNAs participate in androgen receptor-driven looping that selectively enhances gene activation. Proc. Natl. Acad. Sci. U. S. A. 111, 7319–7324. doi:10.1073/pnas.1324151111
Huang, L., and Xu, A.-M. (2017). SET and MYND domain containing protein 3 in cancer. Am. J. Transl. Res. 9, 1–14.
Hughes, I. A., Davies, J. D., Bunch, T. I., Pasterski, V., Mastroyannopoulou, K., and MacDougall, J. (2012). Androgen insensitivity syndrome. Lancet 380, 1419–1428. doi:10.1016/S0140-6736(12)60071-3
Jääskeläinen, T., Makkonen, H., Visakorpi, T., Kim, J., Roeder, R. G., and Palvimo, J. J. (2012). Histone H2B ubiquitin ligases RNF20 and RNF40 in androgen signaling and prostate cancer cell growth. Mol. Cell. Endocrinol. 350, 87–98. doi:10.1016/j.mce.2011.11.025
Jaiswal, B., Agarwal, A., and Gupta, A. (2022). Lysine acetyltransferases and their role in AR signaling and prostate cancer. Front. Endocrinol. 13, 886594. doi:10.3389/fendo.2022.886594
Kahl, P., Gullotti, L., Heukamp, L. C., Wolf, S., Friedrichs, N., Vorreuther, R., et al. (2006). Androgen receptor coactivators lysine-specific histone demethylase 1 and four and a half LIM domain protein 2 predict risk of prostate cancer recurrence. Cancer Res. 66, 11341–11347. doi:10.1158/0008-5472.CAN-06-1570
Kang, Z., Jänne, O. A., and Palvimo, J. J. (2004). Coregulator recruitment and histone modifications in transcriptional regulation by the androgen receptor. Mol. Endocrinol. 18, 2633–2648. doi:10.1210/me.2004-0245
Kang, H.-B., Choi, Y., Lee, J. M., Choi, K.-C., Kim, H.-C., Yoo, J.-Y., et al. (2009). The histone methyltransferase, NSD2, enhances androgen receptor-mediated transcription. FEBS Lett. 583, 1880–1886. doi:10.1016/j.febslet.2009.05.038
Kato, S., Yokoyama, A., and Fujiki, R. (2011). Nuclear receptor coregulators merge transcriptional coregulation with epigenetic regulation. Trends biochem. Sci. 36, 272–281. doi:10.1016/j.tibs.2011.01.001
Kawano, H., Sato, T., Yamada, T., Matsumoto, T., Sekine, K., Watanabe, T., et al. (2003). Suppressive function of androgen receptor in bone resorption. Proc. Natl. Acad. Sci. U. S. A. 100, 9416–9421. doi:10.1073/pnas.1533500100
Kim, J.-Y., Banerjee, T., Vinckevicius, A., Luo, Q., Parker, J. B., Baker, M. R., et al. (2014). A role for WDR5 in integrating threonine 11 phosphorylation to lysine 4 methylation on histone H3 during androgen signaling and in prostate cancer. Mol. Cell. 54, 613–625. doi:10.1016/j.molcel.2014.03.043
Knoll, M., Lodish, H. F., and Sun, L. (2015). Long non-coding RNAs as regulators of the endocrine system. Nat. Rev. Endocrinol. 11, 151–160. doi:10.1038/nrendo.2014.229
Komura, K., Jeong, S. H., Hinohara, K., Qu, F., Wang, X., Hiraki, M., et al. (2016). Resistance to docetaxel in prostate cancer is associated with androgen receptor activation and loss of KDM5D expression. Proc. Natl. Acad. Sci. U. S. A. 113, 6259–6264. doi:10.1073/pnas.1600420113
Kuhns, K. J., Zhang, G., Wang, Z., and Liu, W. (2018). ARD1/NAA10 acetylation in prostate cancer. Exp. Mol. Med. 50, 1–8. doi:10.1038/s12276-018-0107-0
Kuukasjärvi, T., Kononen, J., Helin, H., Holli, K., and Isola, J. (1996). Loss of estrogen receptor in recurrent breast cancer is associated with poor response to endocrine therapy. J. Clin. Oncol. 14, 2584–2589. doi:10.1200/JCO.1996.14.9.2584
Lasko, L. M., Jakob, C. G., Edalji, R. P., Qiu, W., Montgomery, D., Digiammarino, E. L., et al. (2017). Discovery of a selective catalytic p300/CBP inhibitor that targets lineage-specific tumours. Nature 550, 128–132. doi:10.1038/nature24028
Lazaro-Camp, V. J., Salari, K., Meng, X., and Yang, S. (2021). SETDB1 in cancer: Overexpression and its therapeutic implications. Am. J. Cancer Res. 11, 1803–1827.
Lee, D. Y., Northrop, J. P., Kuo, M.-H., and Stallcup, M. R. (2006). Histone H3 lysine 9 methyltransferase G9a is a transcriptional coactivator for nuclear receptors. J. Biol. Chem. 281, 8476–8485. doi:10.1074/jbc.M511093200
Li, N., Dhar, S. S., Chen, T.-Y., Kan, P.-Y., Wei, Y., Kim, J.-H., et al. (2016a). JARID1D is a suppressor and prognostic marker of prostate cancer invasion and metastasis. Cancer Res. 76, 831–843. doi:10.1158/0008-5472.CAN-15-0906
Li, W., Notani, D., and Rosenfeld, M. G. (2016b). Enhancers as non-coding RNA transcription units: Recent insights and future perspectives. Nat. Rev. Genet. 17, 207–223. doi:10.1038/nrg.2016.4
Li, Z., Alyamani, M., Li, J., Rogacki, K., Abazeed, M., Upadhyay, S. K., et al. (2016c). Redirecting abiraterone metabolism to fine-tune prostate cancer anti-androgen therapy. Nature 533, 547–551. doi:10.1038/nature17954
Liu, C., Wang, C., Wang, K., Liu, L., Shen, Q., Yan, K., et al. (2013). SMYD3 as an oncogenic driver in prostate cancer by stimulation of androgen receptor transcription. J. Natl. Cancer Inst. 105, 1719–1728. doi:10.1093/jnci/djt304
Lu, D., Song, Y., Yu, Y., Wang, D., Liu, B., Chen, L., et al. (2021). KAT2A-mediated AR translocation into nucleus promotes abiraterone-resistance in castration-resistant prostate cancer. Cell. Death Dis. 12, 787. doi:10.1038/s41419-021-04077-w
Lupien, M., Eeckhoute, J., Meyer, C. A., Wang, Q., Zhang, Y., Li, W., et al. (2008). FoxA1 translates epigenetic signatures into enhancer-driven lineage-specific transcription. Cell. 132, 958–970. doi:10.1016/j.cell.2008.01.018
Malik, R., Khan, A. P., Asangani, I. A., Cieślik, M., Prensner, J. R., Wang, X., et al. (2015). Targeting the MLL complex in castration-resistant prostate cancer. Nat. Med. 21, 344–352. doi:10.1038/nm.3830
Mangelsdorf, D. J., Thummel, C., Beato, M., Herrlich, P., Schütz, G., Umesono, K., et al. (1995). The nuclear receptor superfamily: The second decade. Cell. 83, 835–839. doi:10.1016/0092-8674(95)90199-x
Matsumoto, T., Sakari, M., Okada, M., Yokoyama, A., Takahashi, S., Kouzmenko, A., et al. (2013). The androgen receptor in health and disease. Annu. Rev. Physiol. 75, 201–224. doi:10.1146/annurev-physiol-030212-183656
Menon, T., Yates, J. A., and Bochar, D. A. (2010). Regulation of androgen-responsive transcription by the chromatin remodeling factor CHD8. Mol. Endocrinol. 24, 1165–1174. doi:10.1210/me.2009-0421
Metzger, E., Wissmann, M., Yin, N., Müller, J. M., Schneider, R., Peters, A. H. F. M., et al. (2005). LSD1 demethylates repressive histone marks to promote androgen-receptor-dependent transcription. Nature 437, 436–439. doi:10.1038/nature04020
Nair, S. J., Yang, L., Meluzzi, D., Oh, S., Yang, F., Friedman, M. J., et al. (2019). Phase separation of ligand-activated enhancers licenses cooperative chromosomal enhancer assembly. Nat. Struct. Mol. Biol. 26, 193–203. doi:10.1038/s41594-019-0190-5
Nakazawa, M., Antonarakis, E. S., and Luo, J. (2014). Androgen receptor splice variants in the era of enzalutamide and abiraterone. Horm. Cancer 5, 265–273. doi:10.1007/s12672-014-0190-1
Neels, O. C., Kopka, K., Liolios, C., and Afshar-Oromieh, A. (2021). Radiolabeled PSMA inhibitors. Cancers 13, 6255. doi:10.3390/cancers13246255
Nguyen, P. L., Alibhai, S. M. H., Basaria, S., D’Amico, A. V., Kantoff, P. W., Keating, N. L., et al. (2015). Adverse effects of androgen deprivation therapy and strategies to mitigate them. Eur. Urol. 67, 825–836. doi:10.1016/j.eururo.2014.07.010
Park, S. H., Fong, K.-W., Mong, E., Martin, M. C., Schiltz, G. E., and Yu, J. (2021). Going beyond polycomb: EZH2 functions in prostate cancer. Oncogene 40, 5788–5798. doi:10.1038/s41388-021-01982-4
Patra, S. K., Patra, A., Zhao, H., and Dahiya, R. (2002). DNA methyltransferase and demethylase in human prostate cancer. Mol. Carcinog. 33, 163–171. doi:10.1002/mc.10033
Pimenta, R., Camargo, J. A., Candido, P., Ghazarian, V., Gonçalves, G. L., Guimarães, V. R., et al. (2022). Cholesterol triggers nuclear Co-association of androgen receptor, p160 steroid coactivators, and p300/CBP-associated factor leading to androgenic Axis transactivation in castration-resistant prostate cancer. Cell. Physiol. biochem. 56, 1–15. doi:10.33594/000000592
Rauluseviciute, I., Drabløs, F., and Rye, M. B. (2020). DNA hypermethylation associated with upregulated gene expression in prostate cancer demonstrates the diversity of epigenetic regulation. BMC Med. Genomics 13, 6. doi:10.1186/s12920-020-0657-6
Rice, M. A., Malhotra, S. V., and Stoyanova, T. (2019). Second-generation antiandrogens: From discovery to standard of care in castration resistant prostate cancer. Front. Oncol. 9, 801. doi:10.3389/fonc.2019.00801
Romanel, A., Gasi Tandefelt, D., Conteduca, V., Jayaram, A., Casiraghi, N., Wetterskog, D., et al. (2015). Plasma AR and abiraterone-resistant prostate cancer. Sci. Transl. Med. 7, 312re10. doi:10.1126/scitranslmed.aac9511
Rosenfeld, M. G., Lunyak, V. V., and Glass, C. K. (2006). Sensors and signals: A coactivator/corepressor/epigenetic code for integrating signal-dependent programs of transcriptional response. Genes. Dev. 20, 1405–1428. doi:10.1101/gad.1424806
Sato, T., Matsumoto, T., Kawano, H., Watanabe, T., Uematsu, Y., Sekine, K., et al. (2004). Brain masculinization requires androgen receptor function. Proc. Natl. Acad. Sci. U. S. A. 101, 1673–1678. doi:10.1073/pnas.0305303101
Sawada, T., Nishimura, K., Mori, J., Kanemoto, Y., Kouzmenko, A., Amano, R., et al. (2021). Androgen-dependent and DNA-binding-independent association of androgen receptor with chromatic regions coding androgen-induced noncoding RNAs. Biosci. Biotechnol. Biochem. 85, 2121–2130. doi:10.1093/bbb/zbab135
Sawada, T., Kanemoto, Y., Amano, R., Hayakawa, A., Kurokawa, T., Mori, J., et al. (2022). Antagonistic action of a synthetic androgen ligand mediated by chromatin remodeling in a human prostate cancer cell line. Biochem. Biophys. Res. Commun. 612, 110–118. doi:10.1016/j.bbrc.2022.04.109
Scher, H. I., Fizazi, K., Saad, F., Taplin, M.-E., Sternberg, C. N., Miller, K., et al. (2012). Increased survival with enzalutamide in prostate cancer after chemotherapy. N. Engl. J. Med. 367, 1187–1197. doi:10.1056/NEJMoa1207506
Schurter, B. T., Koh, S. S., Chen, D., Bunick, G. J., Harp, J. M., Hanson, B. L., et al. (2001). Methylation of histone H3 by coactivator-associated arginine methyltransferase 1. Biochemistry 40, 5747–5756. doi:10.1021/bi002631b
Seligson, D. B., Horvath, S., Shi, T., Yu, H., Tze, S., Grunstein, M., et al. (2005). Global histone modification patterns predict risk of prostate cancer recurrence. Nature 435, 1262–1266. doi:10.1038/nature03672
Sharifi, N. (2013). Mechanisms of androgen receptor activation in castration-resistant prostate cancer. Endocrinology 154, 4010–4017. doi:10.1210/en.2013-1466
Shiina, H., Matsumoto, T., Sato, T., Igarashi, K., Miyamoto, J., Takemasa, S., et al. (2006). Premature ovarian failure in androgen receptor-deficient mice. Proc. Natl. Acad. Sci. U. S. A. 103, 224–229. doi:10.1073/pnas.0506736102
Stein, J., Majores, M., Rohde, M., Lim, S., Schneider, S., Krappe, E., et al. (2014). KDM5C is overexpressed in prostate cancer and is a prognostic marker for prostate-specific antigen-relapse following radical prostatectomy. Am. J. Pathol. 184, 2430–2437. doi:10.1016/j.ajpath.2014.05.022
Sumanasuriya, S., and De Bono, J. (2018). Treatment of advanced prostate cancer-A review of current therapies and future promise. Cold Spring Harb. Perspect. Med. 8, a030635. doi:10.1101/cshperspect.a030635
Sun, A., Tawfik, O., Gayed, B., Thrasher, J. B., Hoestje, S., Li, C., et al. (2007). Aberrant expression of SWI/SNF catalytic subunits BRG1/BRM is associated with tumor development and increased invasiveness in prostate cancers. Prostate 67, 203–213. doi:10.1002/pros.20521
Takayama, K.-I., Kosaka, T., Suzuki, T., Hongo, H., Oya, M., Fujimura, T., et al. (2021). Subtype-specific collaborative transcription factor networks are promoted by OCT4 in the progression of prostate cancer. Nat. Commun. 12, 3766. doi:10.1038/s41467-021-23974-4
Takeda, D. Y., Spisák, S., Seo, J.-H., Bell, C., O’Connor, E., Korthauer, K., et al. (2018). A somatically acquired enhancer of the androgen receptor is a noncoding driver in advanced prostate cancer. Cell. 174, 422–432. doi:10.1016/j.cell.2018.05.037
Tan, K. N., Avery, V. M., and Carrasco-Pozo, C. (2020). Metabolic roles of androgen receptor and Tip60 in androgen-dependent prostate cancer. Int. J. Mol. Sci. 21, 6622. doi:10.3390/ijms21186622
Tang, S., Sethunath, V., Metaferia, N. Y., Nogueira, M. F., Gallant, D. S., Garner, E. R., et al. (2022). A genome-scale CRISPR screen reveals PRMT1 as a critical regulator of androgen receptor signaling in prostate cancer. Cell. Rep. 38, 110417. doi:10.1016/j.celrep.2022.110417
Thompson, D., Choo, N., Bolton, D. M., Lawrentschuk, N., Risbridger, G. P., Lawrence, M. G., et al. (2022). New approaches to targeting epigenetic regulation in prostate cancer. Curr. Opin. Urol. 32, 472–480. doi:10.1097/MOU.0000000000001027
Tong, D., Liu, Q., Liu, G., Yuan, W., Wang, L., Guo, Y., et al. (2016). The HIF/PHF8/AR axis promotes prostate cancer progression. Oncogenesis 5, e283. doi:10.1038/oncsis.2016.74
Torgovnick, A., and Schumacher, B. (2015). DNA repair mechanisms in cancer development and therapy. Front. Genet. 6, 157. doi:10.3389/fgene.2015.00157
Vatapalli, R., Sagar, V., Rodriguez, Y., Zhao, J. C., Unno, K., Pamarthy, S., et al. (2020). Histone methyltransferase DOT1L coordinates AR and MYC stability in prostate cancer. Nat. Commun. 11, 4153. doi:10.1038/s41467-020-18013-7
Viswanathan, S. R., Ha, G., Hoff, A. M., Wala, J. A., Carrot-Zhang, J., Whelan, C. W., et al. (2018). Structural alterations driving castration-resistant prostate cancer revealed by linked-read genome sequencing. Cell. 174, 433–447.e19. doi:10.1016/j.cell.2018.05.036
Wang, H., Huang, Z. Q., Xia, L., Feng, Q., Erdjument-Bromage, H., Strahl, B. D., et al. (2001). Methylation of histone H4 at arginine 3 facilitating transcriptional activation by nuclear hormone receptor. Science 293, 853–857. doi:10.1126/science.1060781
Wang, Q., Li, W., Zhang, Y., Yuan, X., Xu, K., Yu, J., et al. (2009). Androgen receptor regulates a distinct transcription program in androgen-independent prostate cancer. Cell. 138, 245–256. doi:10.1016/j.cell.2009.04.056
Wang, H.-J., Pochampalli, M., Wang, L.-Y., Zou, J. X., Li, P.-S., Hsu, S.-C., et al. (2019). KDM8/JMJD5 as a dual coactivator of AR and PKM2 integrates AR/EZH2 network and tumor metabolism in CRPC. Oncogene 38, 17–32. doi:10.1038/s41388-018-0414-x
Wang, Y., Chen, J., Wu, Z., Ding, W., Gao, S., Gao, Y., et al. (2021). Mechanisms of enzalutamide resistance in castration-resistant prostate cancer and therapeutic strategies to overcome it. Br. J. Pharmacol. 178, 239–261. doi:10.1111/bph.15300
Wissmann, M., Yin, N., Müller, J. M., Greschik, H., Fodor, B. D., Jenuwein, T., et al. (2007). Cooperative demethylation by JMJD2C and LSD1 promotes androgen receptor-dependent gene expression. Nat. Cell. Biol. 9, 347–353. doi:10.1038/ncb1546
Xiang, Y., Zhu, Z., Han, G., Ye, X., Xu, B., Peng, Z., et al. (2007). JARID1B is a histone H3 lysine 4 demethylase up-regulated in prostate cancer. Proc. Natl. Acad. Sci. U. S. A. 104, 19226–19231. doi:10.1073/pnas.0700735104
Xiao, L., Parolia, A., Qiao, Y., Bawa, P., Eyunni, S., Mannan, R., et al. (2022). Targeting SWI/SNF ATPases in enhancer-addicted prostate cancer. Nature 601, 434–439. doi:10.1038/s41586-021-04246-z
Xu, J., Wu, R.-C., and O’Malley, B. W. (2009a). Normal and cancer-related functions of the p160 steroid receptor co-activator (SRC) family. Nat. Rev. Cancer 9, 615–630. doi:10.1038/nrc2695
Xu, K., Shimelis, H., Linn, D. E., Jiang, R., Yang, X., Sun, F., et al. (2009b). Regulation of androgen receptor transcriptional activity and specificity by RNF6-induced ubiquitination. Cancer Cell. 15, 270–282. doi:10.1016/j.ccr.2009.02.021
Yamamoto, A., Hashimoto, Y., Kohri, K., Ogata, E., Kato, S., Ikeda, K., et al. (2000). Cyclin E as a coactivator of the androgen receptor. J. Cell. Biol. 150, 873–880. doi:10.1083/jcb.150.4.873
Yamane, K., Toumazou, C., Tsukada, Y.-I., Erdjument-Bromage, H., Tempst, P., Wong, J., et al. (2006). JHDM2A, a JmjC-containing H3K9 demethylase, facilitates transcription activation by androgen receptor. Cell. 125, 483–495. doi:10.1016/j.cell.2006.03.027
Yang, Y. A., and Yu, J. (2013). EZH2, an epigenetic driver of prostate cancer. Protein Cell. 4, 331–341. doi:10.1007/s13238-013-2093-2
Yıldırım-Buharalıoğlu, G. (2022). Lysine demethylase 6B regulates prostate cancer cell proliferation by controlling c-MYC expression. Mol. Pharmacol. 101, 106–119. doi:10.1124/molpharm.121.000372
Zhang, W., van Gent, D. C., Incrocci, L., van Weerden, W. M., and Nonnekens, J. (2020). Role of the DNA damage response in prostate cancer formation, progression and treatment. Prostate Cancer Prostatic Dis. 23, 24–37. doi:10.1038/s41391-019-0153-2
Keywords: epigenetic, prostate cancer, androgens, chromatin reorganization, genome
Citation: Sawada T, Kanemoto Y, Kurokawa T and Kato S (2023) The epigenetic function of androgen receptor in prostate cancer progression. Front. Cell Dev. Biol. 11:1083486. doi: 10.3389/fcell.2023.1083486
Received: 03 November 2022; Accepted: 07 March 2023;
Published: 21 March 2023.
Edited by:
Urszula Oleksiewicz, Poznan University of Medical Sciences, PolandReviewed by:
Zongwei Wang, Beth Israel Deaconess Medical Center and Harvard Medical School, United StatesXin Chen, Huazhong University of Science and Technology, China
Copyright © 2023 Sawada, Kanemoto, Kurokawa and Kato. This is an open-access article distributed under the terms of the Creative Commons Attribution License (CC BY). The use, distribution or reproduction in other forums is permitted, provided the original author(s) and the copyright owner(s) are credited and that the original publication in this journal is cited, in accordance with accepted academic practice. No use, distribution or reproduction is permitted which does not comply with these terms.
*Correspondence: Shigeaki Kato, shigeaki.kato@isu.ac.jp