- 1Department of Neurobiology, School of Basic Medical Sciences, Peking University, Beijing, China
- 2Neuroscience Research Institute, Peking University, Beijing, China
- 3Key Laboratory for Neuroscience, Ministry of Education, National Health and Family Planning Commission, Peking University, Beijing, China
- 4Department of Integration of Chinese and Western Medicine, School of Basic Medical Sciences, Peking University, Beijing, China
- 5Institute for Anatomy and Cell Biology, Ulm University, Ulm, Germany
- 6Health Bureau of Kenli District, Dongying, China
- 7Autism Research Center, Peking University Health Science Center, Beijing, China
Introduction: Maternal immune activation (MIA) is closely related to the onset of autism-like behaviors in offspring, but the mechanism remains unclear. Maternal behaviors can influence offspring’s development and behaviors, as indicated in both human and animal studies. We hypothesized that abnormal maternal behaviors in MIA dams might be other factors leading to delayed development and abnormal behaviors in offspring.
Methods: To verify our hypothesis, we analyzed poly(I:C)-induced MIA dam’s postpartum maternal behavior and serum levels of several hormones related to maternal behavior. Pup’s developmental milestones and early social communication were recorded and evaluated in infancy. Other behavioral tests, including three-chamber test, self-grooming test, open field test, novel object recognition test, rotarod test and maximum grip test, were performed in adolescence of pups.
Results: Our results showed that MIA dams exhibit abnormal static nursing behavior but normal basic care and dynamic nursing behavior. The serum levels of testosterone and arginine vasopressin in MIA dams were significantly reduced compared with control dams. The developmental milestones, including pinna detachment, incisor eruption and eye opening, were significantly delayed in MIA offspring compared with control offspring, while the weight and early social communication showed no significant differences between the two groups. Behavioral tests performed in adolescence showed that only male MIA offspring display elevated self-grooming behaviors and reduced maximum grip.
Discussion: In conclusion, MIA dams display abnormal postpartum static nursing behavior concomitantly with reduced serum levels of testosterone and arginine vasopressin, possibly involving in the pathogenesis of delayed development and elevated self-grooming in male offspring. These findings hint that improving dam’s postpartum maternal behavior might be a potential regime to counteract delayed development and elevated self-grooming in male MIA offspring.
Introduction
Autism spectrum disorder (ASD) is a heterogeneous group of refractory neurodevelopmental disability. It is characterized by social dysfunction as well as repetitive and stereotyped behavior (American Psychiatric Association, 2013). According to an umbrella review of evidence, up to 67 environmental risk factors and 52 biomarkers have been evaluated in the pathogenesis of ASD (Kim et al., 2019). However, the mechanism of ASD remains unclear with few effective therapies due to the high clinical and genetic heterogeneity (Bhat et al., 2014; Bhandari et al., 2020). It is of great significance to explore the mechanism of ASD, which might pave the road toward novel strategies in therapy.
The prevalence and disability-adjusted life years of ASD have increased rapidly in countries with high socio-demographic index from 1990 to 2019 (Solmi et al., 2022). According to the latest data, the global prevalence of autism is approximately 1/100 (Zeidan et al., 2022), while in China it is up to 0.70% (Zhou et al., 2020). Coincidentally, numerous epidemiologic studies provide evidence that maternal viral infection is growing rapidly in developed countries (Cannon and Davis, 2005; Ludwig and Hengel, 2009; Bate et al., 2010; Basha et al., 2014; Taniguchi et al., 2014) and has significant association with autism (Atladottir et al., 2010b). Croen et al. (2019) found that women who had an infection accompanied by a fever during the second trimester are more likely to have children with ASD. Several meta-analyses of clinical studies also indicate that maternal infection during pregnancy confers an increase in risk for autism in offspring (Jiang et al., 2016; Tioleco et al., 2021). Taken together, we speculate that maternal viral infection might be one of the most important factors contributing to the dramatic increase of ASD morbidity.
Maternal viral infection can induce maternal immune activation (MIA), which affects fetal neurodevelopment via cytokine storm (Estes and McAllister, 2016). These cytokines are closely related to the onset of ASD. For example, the level of MCP-1 is significantly elevated in the amniotic fluid of ASD individuals (Abdallah et al., 2012). MCP1 is secreted by PDGFRβ vascular wall cell. It is firstly activated in central nervous system (CNS) responding to inflammation and in turn increases neuronal excitability by promoting excitatory synaptic transmission in glutamatergic neurons of multiple brain regions (Duan et al., 2018). Elevated levels of IFN-γ, IL-4 and IL-5 in maternal serum are associated with increased risk for ASD in offspring (Goines et al., 2011). Aberrant level of IL-17 has been reported in several rodent models of ASD (Thawley et al., 2022). Maternal IL-17a could promote abnormal cortical development and autism-like behaviors in mice offspring (Choi et al., 2016). Poly(I:C)-induced MIA activates integrated stress response (ISR) in male but not female mice offspring via an IL-17a-dependent manner, which reduced global mRNA translation and altered nascent proteome synthesis (Kalish et al., 2021).
Rodent MIA offspring is likely to be predisposed to ASD. Lee et al. (2021) found that lipopolysaccharide (LPS)-induced MIA male offspring showed social behavior deficits, anxiety-like and repetitive behavior, hypomyelination and abnormal microbiota profile. Mycobacterium tuberculosis-induced MIA mice offspring displayed increased grooming behavior (Manjeese et al., 2021). In addition, Atanasova et al. (2023) reported that MIA exacerbated ASD related alterations in Shank3-deficient mice, suggesting the synergistic effects of MIA and genetic factors in the pathogenesis of ASD. However, the effects of anti-inflammatory or antioxidant on ASD are controversial (Hafizi et al., 2019; Pangrazzi et al., 2020). Although antibiotic used on pregnant women may modify the influence of MIA on increasing the risk of ASD in child (Holingue et al., 2020), the incidence of ASD would be reduced by only 12%–17% if maternal infections could be prevented or safely treated in a timely manner (Tioleco et al., 2021), suggesting that MIA induced ASD in offspring through other mechanisms.
Maternal parenting behavior plays an important role in prosocial behavior development of children (Tang et al., 2022). Abnormal maternal parenting behavior is common in parents of ASD children and closely linked to the severity of ASD (Maljaars et al., 2014). Recently, Zambon et al. (2022) found that MIA disrupts hypothalamic neurocircuits of maternal care behavior, hinting that disrupted maternal care behavior might be involved in the pathogenesis of ASD induced by MIA. Maternal behaviors are regulated by multiple hormones. Estradiol, progesterone and prolactin are main peripheral hormones playing a synergistic role in the regulation of maternal behavior (Keller et al., 2019). Many neurochemical molecules, such as oxytocin (OXT) and arginine vasopressin (AVP), are also involved in regulating maternal behavior. OXT can mediate the formation of mother-infant connection and enhance maternal care (Mitre et al., 2016), while the main function of AVP is regulating maternal aggression (Bayerl and Bosch, 2019). Mothers of autistic children showed lower plasma levels of OXT and AVP as well as a higher plasma level of testosterone (Xu et al., 2013). In addition, increased corticosterone level in mother decreases neural response to baby’s crying (Laurent et al., 2011). Kirsten et al. (2013) found that prenatal exposure to LPS increases maternal serum corticosterone level, causing placental injury and increasing IL-1β level in adult rat offspring, which are relevant to autism.
Polyinosinic-polycytidylic acid [poly(I:C)] is a synthetic nucleotide dimer which has a great effect on inducing interferon (Traynor et al., 2004). In medical research, poly(I:C) is often used to mimic a viral infection (Schwarze et al., 2016). Exposure to poly(I:C) during mid-pregnancy in rats can be used as a model investigating MIA (Vorhees et al., 2012; McColl and Piquette-Miller, 2021). Importantly, this kind of animal model has been regarded as a preclinical model for neurodevelopmental disorders, such as autism and schizophrenia (Haddad et al., 2020).
In this study, we evaluated postpartum maternal behavior as well as serum levels of sex hormones, OXT, AVP and corticosterone in poly(I:C)-induced MIA dams. Developmental milestones and behaviors of MIA offspring were also recorded and analyzed.
Materials and methods
Animals
Male and female Sprague-Dawley (SD) rats (270 g–350 g) were obtained from the Department of Experimental Animal Sciences, Peking University Health Science Center. Animals were housed individually with free access to food and water under a 12–12 h light-dark cycle. The humidity was 50% ± 10% and temperature was 23°C ± 2°C. This study was carried out following USA National Institutes of Health Guide for the Care and Use of Laboratory Animals. The protocols were approved by Peking University Animal Care and Use Committee (LA2020228).
Construction of poly(I:C)-induced MIA rat model
We constructed the poly(I:C)-induced MIA rat model according to previous studies (Vorhees et al., 2012; McColl and Piquette-Miller, 2021). After acclimating for a week, 12-week-old female rats were paired with age-matched male rats. The day was considered embryonic day 0 (E 0) in the presence of a vaginal plug. Then pregnant rats were housed separately. On E 14, pregnant rats received a single intraperitoneal injection of either 8 mg/kg poly(I:C) (Sigma, P9582) or the same volume of 0.01M phosphate buffered saline (PBS). After weaning at postnatal day 21 (PND 21), offspring of the same group and sex but from different dams were randomly mixed with 4-5 per cage until the end of the experiments.
Experimental groups
The experiment was designed as 2 parts. In part I, 8 and 12 pregnant rats were subdivided into control group and MIA group respectively because poly(I:C) considerably increases the risk of resorption (Thaxton et al., 2013; Wang et al., 2015). We aimed to evaluate the postpartum maternal behavior and serum levels of several hormones related to maternal behavior in MIA dams. The litter size and the offspring’s early social communication were also evaluated in this part (see Figure 1A for experimental procedure). In part II, 5 and 3 pregnant rats were distributed into MIA group and control group, respectively. A total of 43 offspring (15 control males, 10 control females, 8 MIA males and 10 MIA females) were finally included in this part. We evaluated the litter size, developmental milestones and adolescent behavioral changes in MIA offspring. The serum IL-6 level of dams after poly(I:C) injection was also assessed in this part (see Figure 1B for experimental procedure).
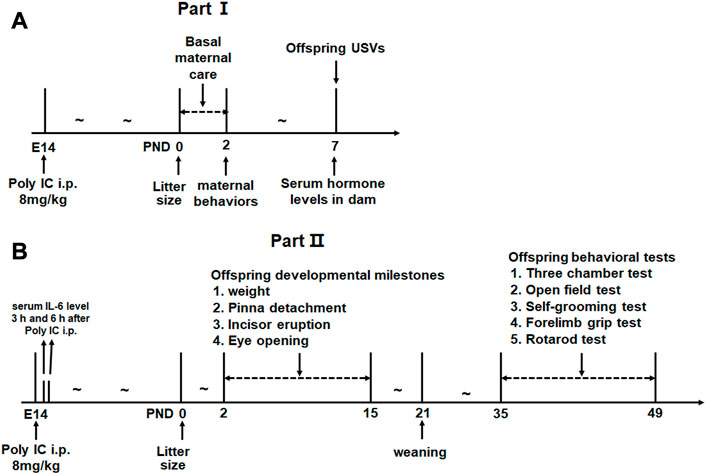
FIGURE 1. Experimental procedure. (A) Experimental design for Part I. (B) Experimental design for Part II.
Serum IL-6 level of dams after poly(I:C) injection
The serum IL-6 level of dams were used to validate the establishment of MIA model (Parker-Athill and Tan, 2010). The blood of dams in part Ⅱ was collected via caudal vein 3 h and 6 h after poly(I:C) injection. Samples were placed at room temperature for 20 min and centrifuged at 1,600 g for 15 min to separate serum. The concentration of serum IL-6 was assessed following the kit instruction (CUSABIO, CSB-E04640r, Wuhan, China).
Basal maternal care
The basal maternal care of dams was assessed as previously reported (Lonstein and Fleming, 2002). Cotton and tissue paper were given as nesting materials during the perinatal of female rats. The nests built by dams were scored by 2 experimenters who were blinded to the groups on PND 0, PND 1 and PND 2 (0 point: no nest, paper strips still scattered over entire floor of the cage; 1 point: poor nest, not all paper strips are used and the nest is flat; 2 point: fair nest, all paper is used but the nest is flat; 3 point: good nest, all paper is used and the nest wall is lower than 5 cm; 4 point: excellent nest, all paper is used and the nest wall is higher than 5 cm) and took the average. The nest score was the average of the 3-day scores. In addition, after delivery, the following indicators of offspring were recorded: with or without skin scar; attachment or removal of placenta; survival rate.
Maternal behavior
Maternal nurturing behaviors of dams were assessed as previously reported (Lonstein and Fleming, 2002). On PND 2, dams were separated from the pups for 30 min and placed individually in the home cage. Pups were placed on a heating pad (37°C). After separation, dams were removed from the cage transitorily and pups were quickly placed in the four corners of the home cage. Then we placed dams back to the home cage and videotaped for 30 min. The maternal care nursing behaviors mainly include static nursing and dynamic nursing. Static nursing includes latency and duration of retrieval and crouching the pups. Dynamic nursing consists of the duration of hovering above and licking the pups. All the maternal behaviors were evaluated by an observer blinded to grouping. In addition, we analyze the dam’s grooming time and nest building time (Berger et al., 2018).
Determination of serum testosterone, estradiol, progesterone, prolactin, corticosterone, oxytocin and AVP in dams
Serum levels of these hormones in dam were determined at PND 7. Blood was collected via decapitation and serum was separated. The levels of testosterone (CUSABIO, Wuhan, China), estradiol (CUSABIO, Wuhan, China), progesterone (CUSABIO, Wuhan, China), prolactin (CUSABIO, Wuhan, China), corticosterone (CUSABIO, Wuhan, China), oxytocin (Enzo life sciences, PA, USA) and AVP (Enzo life sciences, PA, USA) were assessed according to the kit instruction. All the 8 control dams in part II were included in this section. But among the 9 MIA dams, we failed to get blood in 1 MIA dam, and no enough serum was separated from another MIA dam. Finally, the serum hormone analytes had n = 8 per group except testosterone and corticosterone had n = 7 for the MIA group.
Offspring’s developmental milestones
We recorded the offspring’s developmental milestones from PND 2 to PND 15, including body weight, pinna detachment day, incisor eruption day and eye-opening day. For each group, the number of pups achieving these developmental goals was daily recorded.
Isolation-induced ultrasonic vocalizations (USVs)
Offspring’s early social communication was tested by isolation-induced USVs on PND 7 between 18:00 and 22:00 in a quiet environment with dim light. Offsprings were individually removed from the home cage and gently transferred to the test cage on a heating pad (37°C). USVs were recorded for 300 s for each pup and collected by an ultrasonic microphone (CM16/CMPA, Avisoft Bioacoustics, Berlin, Germany) hanging 25 cm above the cage floor. The connected amplifier (AUSG-116H, Avisoft Bioacoustics, Berlin, Germany) was set at a sampling frequency of 250 kHz with a 125 kHz low-pass filter. The recorded files were analyzed by Avisoft SASLab Pro (Version 4.52) using a fast Fourier transform (512 FFT-length, 100% frame size, hamming window, 50% time-window overlap). The number and duration of total USVs were recorded.
Offspring’s behavioral tests in adolescence
Adolescent offspring began behavioral tests on PND 35. Male and female offspring were performed three-chamber test and self-grooming test on PND 35 and PND 37, respectively. In order to explore the underlying mechanism of elevated stereotypic behavior in male offspring, we performed open field test, novel object recognition test, rotarod test and maximum grip test on PND 39, PND 43, PND 46 and PND 49, respectively, in male offspring since rodent self-grooming has a strong association with sensation, motor and memory (Kalueff et al., 2016). All the behavioral tests were conducted in this particular order.
Three-chamber test
Social preference and social novelty of offspring were evaluated on PND 35 by three-chamber test during the dark cycle (Dai et al., 2018). The three-chamber apparatus comprises three identical rectangular plexiglass chambers (40 cm × 34 cm × 24 cm). Each side chamber connected to the central chamber by a corridor. The subject rat was placed in the central chamber and explore freely for 5 min for habituation. Then two successive stages were followed. Stage I for social preference. An unfamiliar, weight and sex matched SD rat (Stranger 1) was locked in a wire cage and placed in one side chamber. An identical empty wire cage was placed in the other side chamber. The subject rat was placed in the central chamber and explored the three chambers for 10 min. Stage II for social novelty. Another unfamiliar, weight and sex matched SD rat (Stranger 2) was placed in the empty wire cage of the Stage I. The subject rat was then allowed to access to the three chambers freely for 10 min. During the experiment, the time spent in every chamber was automatically recorded. To minimize the impact from residual rat odors, the entire apparatus was thoroughly cleaned with 70% ethanol at the beginning of each trial.
Self-grooming test
The self-grooming test performed on PND 37 is used to measure the severity of stereotyped behavior in offspring. The rat was placed into an empty cage similar to the home cage and explored freely for 10 min. Then rat behavior was video-taped for 10 min and total self-grooming time was calculated by a researcher who was blinded to grouping (Song et al., 2019). Self-grooming behaviors include: 1) wiping nose, face, head and ears with forepaws; 2) licking body, anogenital area and tail (Kalueff et al., 2016).
Open field test
Anxiety behavior and spontaneous activity of male offspring were evaluated in open field test on PND 39. The subject rat was initially placed in the center of the acrylic box (100 cm × 100 cm × 40 cm) and explored freely for 10 min. Videos were processed by SMART software (v2.5.21, Panlab Harvard Apparatus). The total distances traveled in the open field represents spontaneous activity, while the time spent in the outer zone (the area ratio of center zone and outer zone is 1:3) represents anxiety behavior.
Novel object recognition test
Learning and memory ability of male offspring were evaluated by the novel object recognition test during the dark period on PND 43 under dim red illumination according to a previous study (Song et al., 2019). A subject rat was placed in the arena (60 cm × 40 cm × 40 cm) for 10 min of habituation on the first and second day (PND 41 and PND 42). On the third day (PND 43), the rat was allowed to explore two identical objects in the arena for 20 min. One hour later, one of the two objects was replaced by a new object (with similar size but different color and shape). The rat was placed into the arena again exploring freely for 10 min and videotaped. The object exploration time was calculated by a researcher blinded to grouping. The object exploration behavior was defined as the nose of the rat touching the object or being oriented toward the object within 2 cm (Wang et al., 2011).
Rotarod test
Male offspring’s motor ability was tested by a rotarod test. Subject rats were placed on the rotarod (Bioseb, France) for 5 min with a rotating speed of 4 rpm at the same time for 2 consecutive days (PND 44 and PND 45). At the same time of the third day (PND 46), subject rats were placed on the rotarod for 5 min with the rotating speed going from 4 rpm to 40 rpm. Every subject rat went through three repeated trials with an interval of 10 min. The latency to fall was recorded every time and the mean of three recordings was calculated.
Maximum grip test
The male offspring’s maximum grip strength was measured by maximum grip test. All the male pups were trained to be adapted to the maximum grip test 2 consecutive days (PND 47 and PND 48). We gently placed the pups on the grid of the dynamometer (Bioseb, France) and pulled their tails in the opposite direction. On PND 49, the maximum grip strength exerted by the pup before losing grip was recorded. We repeated 3 measurements on each pup, allowing a 30 s recovery time between each measurement. The mean of 3 measurements on each pup was calculated.
Statistical analysis
Data are presented as the mean ± SEM. Statistical analysis was conducted by unpaired t-test (quantitative data with normal distribution and equal variance) or Mann-Whitney U test (quantitative data with non-normal distribution). Shapiro-Wilk test was used to check normal distribution. Two-way ANOVA was used to analyze the pup weight. A paired t-test was used to determine within-group side preference in three-chamber test and object exploration time in novel object recognition test. Data were analyzed and graphed by GraphPad Prism software (version 8.0.1). A double-tailed p < 0.05 was considered statistically significant.
Results
Poly(I:C)-induced MIA dams showed acute elevated serum IL-6 level as well as reduced litter size
To validate the establishment of MIA model, we accessed the serum level of IL-6 in MIA dams 3 h and 6 h after poly(I:C) injection. The serum IL-6 level in MIA dam was significantly increased 3 h after poly(I:C) injection (Unpaired t-test, t = 4.059, df = 6, p = 0.0067, Figure 2A) but significantly decreased 6 h after poly(I:C) injection (Unpaired t-test, t = 3.032, df = 6, p = 0.0230, Figure 2A). In addition, we accessed the litter size of dams. The number of pups in MIA dams is significantly lower than that in control dams (Unpaired t-test, t = 3.204, df = 18, p = 0.0049, Figure 2B, part I; Unpaired t-test, t = 2.905, df = 6, p = 0.0272, Figure 2B, part II), which is consistent to previous studies (Kowash et al., 2019). This result indicated that poly(I:C) injection induced acute inflammation and reduced litter size in dams, suggesting that the MIA model construction is successful.
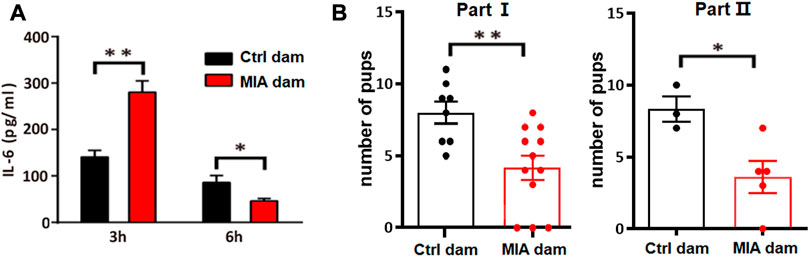
FIGURE 2. Model validation. (A) Serum level of IL-6 in dams 3 h or 6 h after Poly(I:C) injection. Ctrl dam, n = 3; MIA dam, n = 5; *p < 0.05 based on Unpaired t-test. **p < 0.01 based on Unpaired t-test. (B) Litter size of dams. Part I Ctrl dam, n = 8; MIA dam, n = 12; **p < 0.01 based on Unpaired t-test. Part II Ctrl dams, n = 3; MIA dams, n = 5; *p < 0.05 based on Unpaired t-test. Ctrl, control. MIA, maternal immune activation. Error bars in this figure represent mean ± SEM of the mean values of each experiment.
Poly(I:C)-induced MIA dams showed abnormal static nursing behavior but normal basic care and normal dynamic nursing behavior
We evaluated the postpartum maternal behavior of dams. The nesting score between the 2 groups showed no significant difference (Mann-Whitney U test, U = 33.5, p = 0.7529, Figure 3A). The placental adnexa tissue of all pups was cleaned and all the pups survived with no skin lesions (data not shown). These results suggested that the basal maternal care of MIA dams did not change compared with control dams. Regarding maternal behavior, the latency (Mann-Whitney U test, U = 25.5, p = 0.3356, Figure 3B) and duration (Unpaired t-test, t = 1.504, df = 15, p = 0.1534, Figure 3C) of retrieving the pups tended to be elevated in MIA group, while the latency of crouching the pups is significantly elevated (Mann-Whitney U test, U = 11, p = 0.0183, Figure 3D) and the duration of crouching the pups is significantly decreased in MIA group (Mann-Whitney U test, U = 15, p = 0.0480, Figure 3E). These results indicate that static nursing in MIA group is abnormal. The time spent in hovering above (Unpaired t-test, t = 0.3803, df = 15, p = 0.7091, Figure 3F) and licking (Unpaired t-test, t = 0.3257, df = 15, p = 0.7492, Figure 3G) the pups also showed no significant differences between control and MIA group, suggesting that the dynamic nursing did not change in MIA dams. We also evaluated the time spent in grooming (Unpaired t-test, t = 0.1559, df = 15, p = 0.8782, Figure 3H) and nest building (Mann-Whitney U test, U = 33, p = 0.8148, Figure 3I), which showed no significant differences between 2 groups.
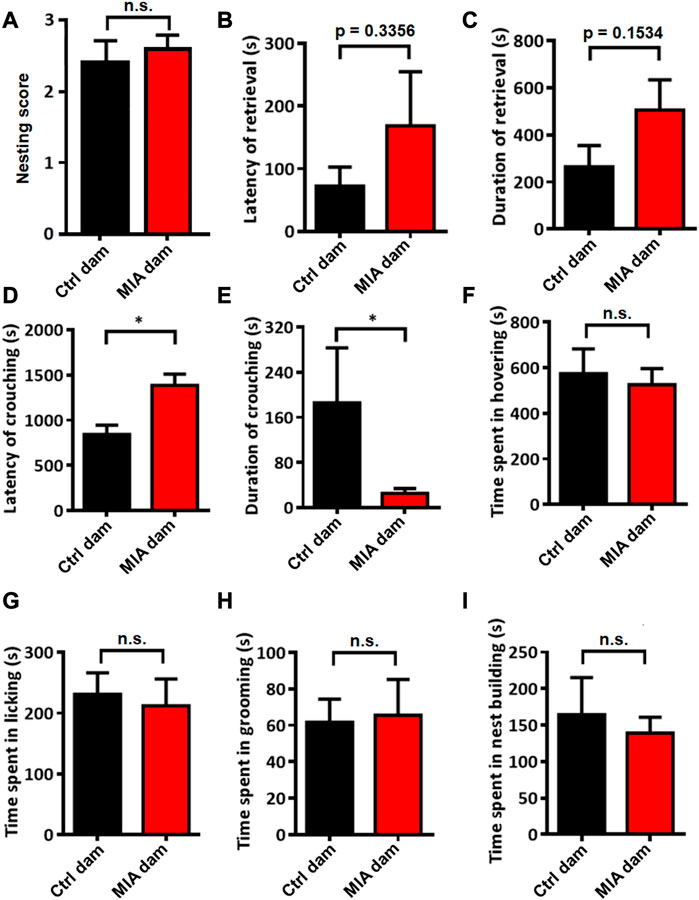
FIGURE 3. Postpartum maternal behavior in Ctrl and MIA dams. (A) Nesting score. Ctrl dam, n = 8; MIA dam, n = 9. Mann-Whitney U test. (B–E) Static nursing. Ctrl dam, n = 8; MIA dam, n = 9. (B) Latency of retrieval. p = 0.3356 based on Mann-Whitney U test. (C) Duration of retrieval. p = 0.1534 based on Unpaired t-test. (D) Latency of crouching. *p < 0.05 based on Mann-Whitney U test. (E) Duration of crouching. *p < 0.05 based on Mann-Whitney U test. (F–G) Dynamic nursing. Ctrl dam, n = 8; MIA dam, n = 9. (F) Time spent in hovering. Unpaired t-test. (G) Time spent in licking. Unpaired t-test. (H) Time spent in grooming. Ctrl dam, n = 8; MIA dam, n = 9. Unpaired t-test. (I) Time spent in nest building. Ctrl dam, n = 8; MIA dam, n = 9. Mann-Whitney U test. Ctrl, control. MIA, maternal immune activation. Error bars in this figure represent mean ± SEM of the mean values of each experiment.
Poly(I:C)-induced MIA dams showed reduced serum levels of testosterone and AVP
To evaluate the potential mechanisms of abnormal static nursing behavior in poly(I:C)-induced MIA dams, we evaluated the serum levels of several hormones related to maternal behavior. We found that the serum levels of testosterone (Mann-Whitney U test, U = 8, p = 0.0205, Figure 4B) and AVP (Unpaired t-test, t = 2.548, df = 14, p = 0.0232, Figure 4F) in MIA dams were significantly reduced compared with control dams. The serum levels of estradio (Unpaired t-test, t = 0.7375, df = 14, p = 0.4730, Figure 4A), progesterone (Unpaired t-test, t = 0.02518, df = 14, p = 0.9803, Figure 4C), prolactin (Unpaired t-test, t = 0.01691, df = 14, p = 0.9867, Figure 4D), oxytocin (Unpaired t-test, t = 0.2538, df = 14, p = 0.8033, Figure 4E) and corticosterone (Unpaired t-test, t = 0.4756, df = 13, p = 0.6422, Figure 4G) showed no significant differences between the 2 groups.
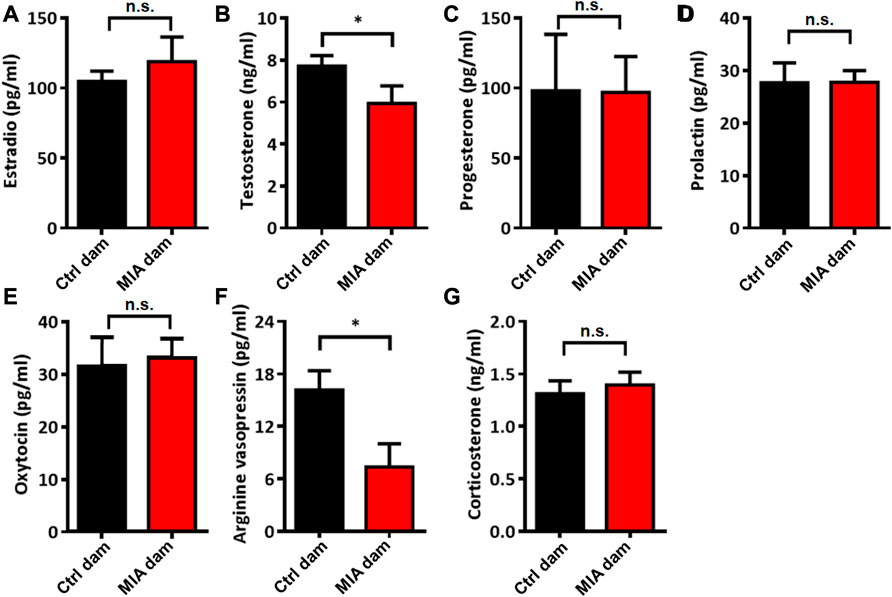
FIGURE 4. Serum levels of several hormones in dams on PND 7. (A) Estradio. Ctrl dam, n = 8; MIA dam, n = 8. Unpaired t-test. (B) Testosterone. Ctrl dam, n = 8; MIA dam, n = 7. *p < 0.05 based on Mann-Whitney U test (C) Progesterone. Ctrl dam, n = 8; MIA dam, n = 8. Unpaired t-test. (D) Prolaction. Ctrl dam, n = 8; MIA dam, n = 8. Unpaired t-test. (E) Oxytocin. Ctrl dam, n = 8; MIA dam, n = 8. Unpaired t-test. (F) Arginine vasopressin. Ctrl dam, n = 8; MIA dam, n = 8. *p < 0.05 based on Unpaired t-test. (G) Corticosterone. Ctrl dam, n = 8; MIA dam, n = 7. Unpaired t-test. Ctrl, control. MIA, maternal immune activation. Error bars in this figure represent mean ± SEM of the mean values of each experiment.
Poly(I:C)-induced MIA offspring showed delayed developmental milestones with normal weight and normal early communication
To evaluate the developmental milestones in poly(I:C)-induced MIA offspring, we recorded the pup’s weight, pinna detachment, incisor eruption and eye opening from PND 2 to PND 15. The pup’s weight showed no significant difference between the two groups (two-way ANOVA, df = 6, p = 0.9466, Figure 5A), while the day of pinna detachment (Mann-Whitney U test, U = 90, p < 0.001, Figure 5B), incisor eruption (Mann-Whitney U test, U = 108, p = 0.0018, Figure 5C) and eye opening (Mann-Whitney U test, U = 120, p = 0.0039, Figure 5D) in MIA offspring were significantly later than that in control offspring, suggesting that the developmental milestones were significantly delayed in MIA offspring. The early social communication in offspring was evaluated on PND7. The isolation-induced USVs showed that total USVs number (Mann-Whitney U test, U = 1490, p = 0.4257, Figure 5E) and total USVs duration (Mann-Whitney U test, U = 1431, p = 0.2601, Figure 5F) were comparable between MIA and control group, implying that MIA offspring had normal early social communication.
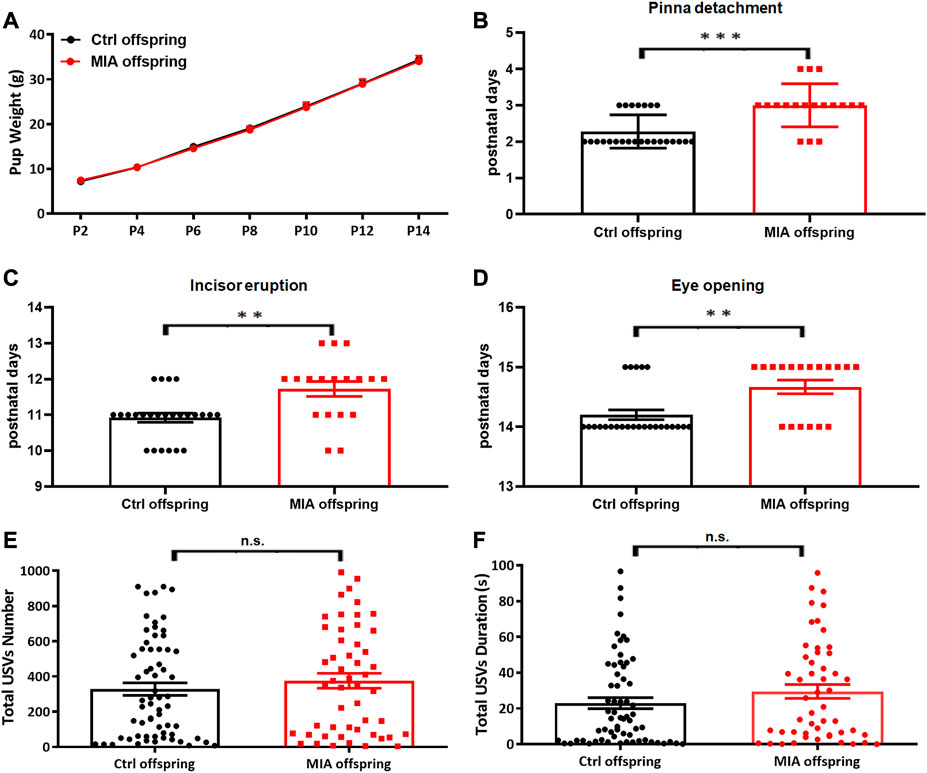
FIGURE 5. Offspring’s developmental milestones and early social communication. (A–D) Offspring’s developmental milestones. Ctrl offspring, n = 25; MIA offspring, n = 18. (A) Weight. Two-way ANOVA. (B) Pinna detachment. ***p < 0.001 based on Mann-Whitney U test. (C) Incisor eruption. **p < 0.01 based on Mann-Whitney U test. (D) Eye opening. **p < 0.01 based on Mann-Whitney U test. (E,F) Isolation-Induced USVs in offspring. Ctrl offspring, n = 64; MIA offspring, n = 51. (E) Total USVs number. Mann-Whitney U test. (F) Total USVs duration. Mann-Whitney U test. Ctrl, control. MIA, maternal immune activation. Error bars in this figure represent mean ± SEM of the mean values of each experiment.
Male MIA offspring showed elevated self-grooming behaviors and reduced maximum grip
In order to assess the autism-like behaviors in MIA offspring, we performed three-chamber test and self-grooming test on PND 35 and PND 37, respectively. In three-chamber test, at Stage I (social preference), both control and MIA offspring spent more time in the side with Stranger 1 than in the empty cage (paired t-test; control male, t = 18.73, df = 14, p < 0.001; control female, t = 15.25, df = 9, p < 0.001; MIA male, t = 10.10, df = 7, p < 0.001; MIA female, t = 9.358, df = 9, p < 0.001; Figures 6A, B), while at Stage II (social novelty), both control and MIA offspring spent more time in proximity to Stranger 2 than Stranger 1 (paired t-test; control male, t = 10.11, df = 14, p < 0.001; control female, t = 6.698, df = 9, p < 0.001; MIA male, t = 5.999, df = 7, p < 0.001; MIA female, t = 6.097, df = 9, p < 0.001; Figures 6C, D), showing that both male and female MIA offspring had normal social preference and social novelty. Self-grooming test showed that the self-grooming time was significantly elevated in male MIA offspring (Unpaired t-test, t = 2.581, df = 21, p = 0.0174, Figure 6E) but not changed in female MIA offspring (Unpaired t-test, t = 0.5895, df = 18, p = 0.5629, Figure 6F).
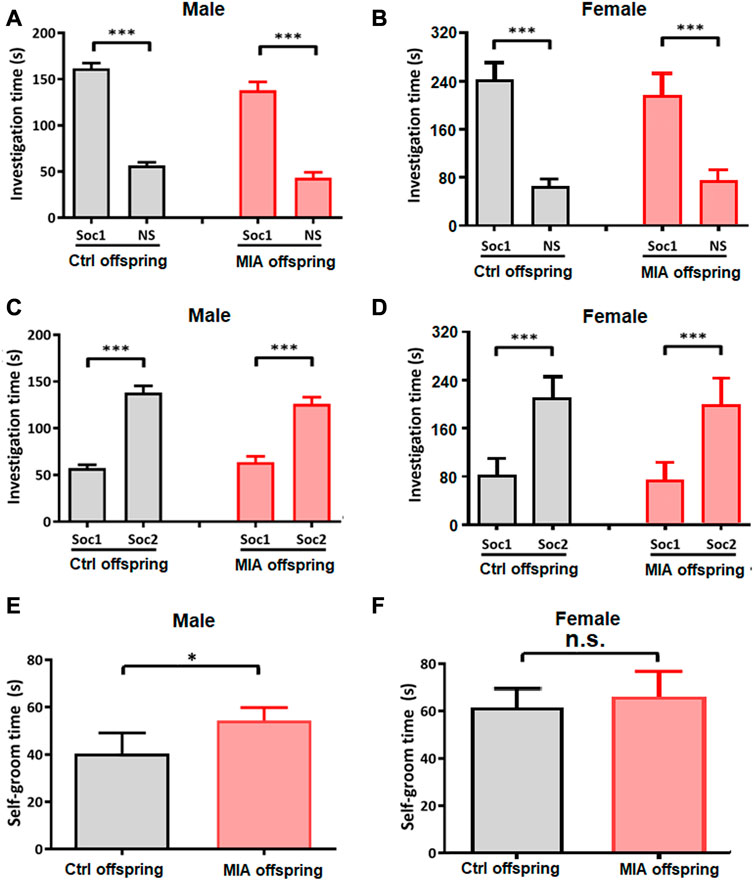
FIGURE 6. Offspring’s autism-like behaviors. (A–D) Three-chamber test. Ctrl males n = 15; Ctrl females n = 10; MIA males n = 8; MIA females n = 10. (A) Social preference of male offspring. ***p < 0.001 based on paired t-test. (B) Social preference of female offspring. ***p < 0.001 based on paired t-test. (C) Social novelty of male offspring. ***p < 0.001 based on paired t-test. (D) Social novelty of female offspring. ***p < 0.001 based on paired t-test. (E,F) Self-grooming test. Ctrl males n = 15; Ctrl females n = 10; MIA males n = 8; MIA females n = 10. (E) Self-grooming time of male offspring. *p < 0.05 based on Unpaired t-test. (F) Self-grooming time of female offspring. Unpaired t-test. Ctrl, control. MIA, maternal immune activation. Error bars in this figure represent mean ± SEM of the mean values of each experiment.
In order to explore the motor ability potentially related to elevated self-grooming behavior in male MIA offspring, open field test, novel object recognition test, rotarod test and maximum grip test were performed on PND 39, PND 43, PND 46 and PND 49, respectively. In open field test, MIA and control male offspring travelled comparable distance in the experimental arena (Unpaired t-test, t = 0.3804, df = 21, p = 0.7069, Figure 7A) and spent comparable time in the outer zone (Unpaired t-test, t = 0.6431, df = 21, p = 0.5260, Figure 7B), hinting that the anxiety behavior and spontaneous activity did not change in male MIA offspring. In novel object recognition test, both control and MIA male offspring spent more time exploring novel object than familiar object (paired t-test, Ctrl male, t = 7.326, df = 14, p < 0.001, MIA male, t = 4.391, df = 7, p = 0.0023, Figure 7C), showing that male MIA offspring had normal learning and memory ability. Rotarod test showed that the latency to fall from the rotarod showed no significant differences between control and MIA offspring (Unpaired t-test, t = 0.2923, df = 21, p = 0.7744, Figure 7D), suggesting that male offspring’s motor ability did not differ between the 2 groups. Maximum grip test showed that the maximum grip of male MIA offspring was significantly reduced (Mann-Whitney U test, U = 8, p = 0.0205, Figure 7E), implying that the muscle of male MIA offspring was weak.
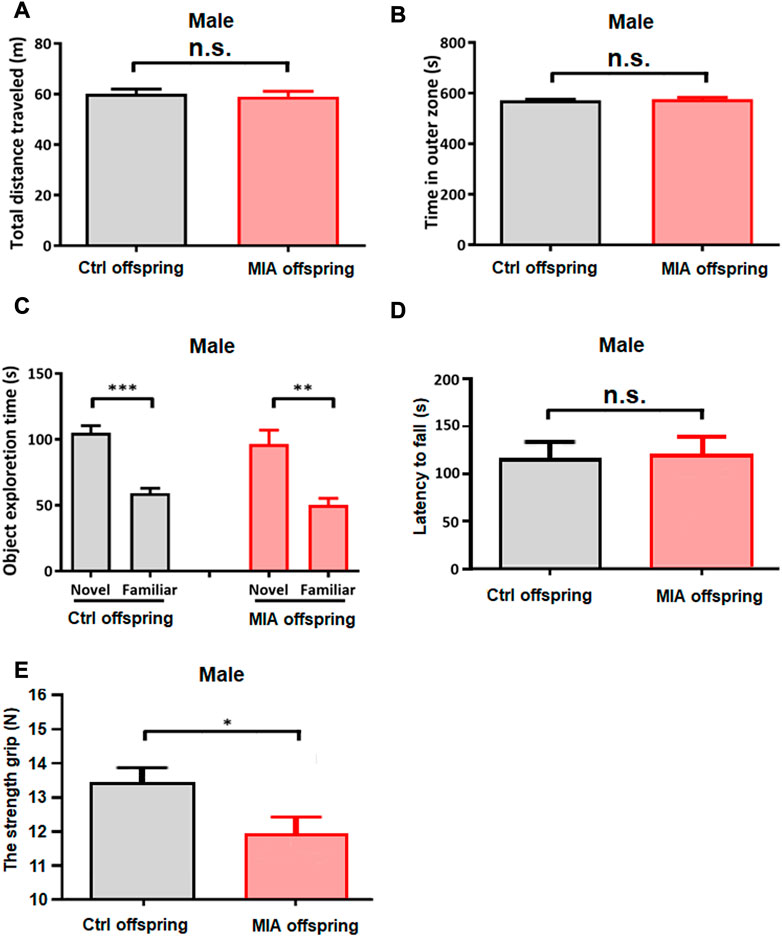
FIGURE 7. Male offspring’s other behavioral tests. (A,B) Open field test of male offspring. Ctrl males n = 15; MIA males n = 8. (A) Total distance traveled. Unpaired t-test. (B) Time spent in outer zone. Unpaired t-test. (C) Novel object recognition test of male offspring. Ctrl males n = 15; MIA males n = 8. **p < 0.01 based on paired t-test. ***p < 0.001 based on paired t-test. (D) Rotarod test of male offspring. Ctrl males n = 15; MIA males n = 8. Unpaired t-test. (E) Maximum grip test of male offspring. Ctrl males n = 13; MIA males n = 7. *p < 0.05 based on Mann-Whitney U test. Ctrl, control. MIA, maternal immune activation. Error bars in this figure represent mean ± SEM of the mean values of each experiment.
Discussion
In the current study, we established a poly(I:C)-induced MIA rat model to evaluate postpartum maternal behavior in MIA dams and autism-like behaviors in MIA offspring. MIA dams showed reduced litter size and abnormal static nursing behavior. The serum levels of testosterone and AVP significantly decreased in MIA dams. The early developmental milestones in MIA offspring were significantly delayed with no significant changes in weight and social communications. Both male and female MIA offspring have normal social preference and social novelty, while self-grooming behaviors are significantly elevated in male but not female MIA offspring. The strength grip of male MIA offspring was significantly reduced. Open field test and novel object recognition test showed no significant differences between the two groups. Our results hinted that abnormal maternal behavior in MIA dams might play an important role in the pathogenesis of delayed development and elevated self-grooming behaviors in male rat offspring.
MIA has been linked to an increased risk of ASD in offspring through affecting the development of the CNS via inflammatory molecules (Ciaranello and Ciaranello, 1995; Atladottir et al., 2010a). A recent study reported that MIA destroys hypothalamic neurocircuits of maternal care behavior (Zambon et al., 2022), which brings us a new clue that abnormal maternal behavior might be involved in the pathogenesis of autism-like behavior in MIA offspring. We found that MIA dams showed elevated latency and decreased duration of crouching the pups. The time spent in hovering above and licking pups as well as grooming and nest building time did not change between the 2 groups. These results are not consistent with a previous study reporting that poly(I:C)-induced MIA C3H/He mice dams showed reduced licking/grooming behaviors and elevated nesting building time (Berger et al., 2018). We speculate that behavioral and immunological effects of MIA on dams are strain-dependent. In addition, the litter size of MIA dams was significantly reduced compared with that of control dams. A previous study reported that reduction of litter size increased arched-back posture and licking pups in lactating rats (Enes-Marques and Giusti-Paiva, 2018), implying that litter size reduction might affect the results of postpartum maternal behavior. The influence of litter size should be eliminated in our future studies to make our conclusions more rigorous.
Furthermore, we evaluated serum levels of several hormones related to maternal behavior. We collected the serum of dams on PND 7 after pups’ early social communication was detected. Results showed that the serum levels of testosterone and AVP were significantly reduced in MIA dams. AVP has the twin peptide with OXT and plays a similar role in maternal behavior regulation (Bosch and Neumann, 2012) and aggression (Bosch and Neumann, 2012; Carter, 2017). Our previous clinical study reported that the plasma AVP level in mothers of ASD children tended to be lower than that of normal children (Zhang et al., 2016). It is interesting that the serum level of testosterone is significantly decreased in MIA dams, which is inconsistent with previous clinical findings, reporting that the serum level of testosterone is significantly elevated in mothers of ASD individuals (Ruta et al., 2011; Palomba et al., 2012; Xu et al., 2013). For there is still no direct evidence from the women experiencing inflammation, the reason of decrease of testosterone in MIA model needs more in-depth researches.
Self-grooming is a complex innate behavior frequently performed in rodents with an evolutionary conserved sequencing pattern. It is related with not only pattern of action but multiple motor abilities. Self-grooming behavior is regulated by multiple brain regions, including hypothalamus, striatum, neocortex, amygdala, brainstem and cerebellum (Kalueff et al., 2016). Elevated self-grooming behavior has been reported in many neurological and neuropsychiatric disorders, such as schizophrenia and ASD (American Psychiatric Association, 2013). In this study, we found that self-grooming behaviors were significantly elevated in male MIA offspring but not changed in females, indicating that MIA-induced autism-like behavior showed significant sex differences. The maximum grip of male MIA offspring was significantly reduced, implying that the muscle of male MIA offspring was weak. Haida et al. reported that male MIA mice offspring show reduced motor development and coordination deficits, as well as a significant decrease in the number of Purkinje cells in cerebellum and neurons in the motor cortex (Haida et al., 2019). In addition, reduced litter size can increase repetitive and stereotyped movements in offspring (de Novais et al., 2021). Similar results have also been reported in MIA mice offspring, showing that the autism-like behavior is more serious in male MIA offspring than that in females (Carlezon et al., 2019; Haida et al., 2019). It is well-acknowledged that the incidence of ASD is obviously male biased (Maenner et al., 2021). But the male-to-female ratio of ASD is heterogeneous, 6-16:1 in mild ASD population and 1-2:1 in severe ASD population (Fombonne, 2009; Jacquemont et al., 2014), suggesting that pregnancy infection is likely to cause mild ASD in offspring. Carlezon et al. reported in MIA offspring that the anti-inflammatory factors are decreased in males and increased in females (Carlezon et al., 2019), which to some extent explained the male biased morbidity of autism-like behavior induced by MIA. Taken together, we speculate that elevated self-grooming behavior in male MIA rat offspring is caused by multiple factors, including abnormal maternal behaviors, reduced litter size as well as immunological factors. The long-term effects of the maternal gestational environment or maternal behavior on pup phenotype need to be further evaluated.
However, results from different studies are heterogeneous. For example, Malkova et al. (2012) found that poly(I:C)-induced MIA mice offspring displayed reduced early social communication, decreased sociability and increased repetitive/stereotyped behaviors. Lee et al. (2021) constructed the MIA rat model by intraperitoneally injecting LPS on pregnant rats, finding that LPS-induced MIA rat offspring show reduced social ability and increased anxiety-like and repetitive behavior. Gzielo et al. (2021) constructed MIA rat model by intraperitoneal injection of 5 mg/kg poly(I:C) on PND 15, finding that male offspring showed deficits in social play behaviors, while elevated repetitive behaviors were found in both sexes. These phenomena are also appearing in the real world. During the COVID-19 pandemic, the potential effects of pregnancy SARS-CoV-2 infection on maternal and perinatal outcomes are controversial (Vergara-Merino et al., 2021). The outcomes of pregnancy and newborn can be affected by the symptoms and severity of COVID-19 as well as the infection time. Comparing with asymptomatic patients, symptomatic pregnant woman infected with SARS-CoV-2 are more likely to suffer from cesarean (Jenabi et al., 2022) or premature birth (Vimercati et al., 2022; Wilkinson et al., 2022). Fetuses born to symptomatic COVID-19 pregnant women have a significantly higher risk of suffering from low body weight comparing with those born to asymptomatic pregnant women (Jenabi et al., 2022). No significant relationship was found between asymptomatic pregnant women and fetal growth restriction (Narang et al., 2023). Fetuses born to SARS-CoV-2-infected women are more likely to suffer from neuromotor developmental disorders (Fajardo Martinez et al., 2023). They also had lower scores in communication, problem solving and personal-social domains (Cheng et al., 2021). Ayed et al. (2022) found that pregnant women infected by SARS-CoV-2 in the first and second pregnant trimesters were more likely to have children with developmental disorders than those infected in the third trimester of pregnancy. Wu T. et al. (2021) found that SARS-CoV-2 infection in the third pregnant trimester did not increase the risk of developmental disorders at the age of 3 months. We speculate that the dosage and the time window of poly(I:C) exposure can affect the offspring’s neural development and behavior in various degrees. Different strains of animals or people in different states might also respond differently to poly(I:C), LPS or virus. In addition, poly(I:C) from different companies have different properties (Kowash et al., 2019). These problems might be valuable lines of research direction in the future.
Limitations
This study has some limitations. Firstly, the number of dams was slightly small compared with similar studies (Lins et al., 2018; Gzielo et al., 2021). Secondly, as is described above, different methods constructing the MIA model may produce different results. Poly(I:C) is an analogue of viruses, not a virus. We speculated that MIA induced by poly(I:C) may be inconsistent with MIA induced by virus. A viral infection-induced MIA animal model, such as COVID-19 or cytomegalovirus infection animal model, may be closer to clinical practice. Thirdly, we did not access the serum level of IL-6 in Part Ⅰ. We consider that collecting blood from dams via caudal vein 3 h and 6 h after poly(I:C) injection on E 14 may affect the accuracy of basal maternal care and maternal behavior. The causal relationship among MIA, abnormal maternal behavior and autism-like behavior in offspring needs to be explored with more complicated model.
Conclusion
In general, our present study showed that poly(I:C)-induced MIA dams displayed abnormal static nursing behavior as well as reduced serum levels of testosterone and AVP, accompanied with delayed early development and elevated self-grooming behavior in male offspring. This is the first study systematically evaluating both dams’ maternal behavior and offspring’s autism-like behaviors in one model of MIA. More in-depth and detailed studies exploring the relationship between maternal behavior in dams and autism-like behaviors in offspring as well as the internal mechanisms will be carried out in our future research.
Data availability statement
The original contributions presented in the study are included in the article/supplementary material, further inquiries can be directed to the corresponding author.
Ethics statement
The animal study was reviewed and approved by Peking University Animal Care and Use Committee (LA2020228).
Author contributions
X-YL and RZ conceptualized and designed the study. SY, MY-J and F-JZ record offspring’s developmental milestones and tested isolation-induced USVs. Y-FX, M-JL, and J-NW performed all the behavioral tests in adolescent offspring. X-WW and W-WD performed all the behavioral tests in dams. H-HH and Z-RG performed ELISA. X-NY, and T-JS analyzed the data. YY-G wrote the manuscript. YZ, TB, and JS-Z reviewed and edited the manuscript and made valuable suggestions. All authors edited the manuscript.
Funding
This work was supported by Key Technological Projects of Guangdong Province (2018B030335001) and China University IUR Innovation Foundation (Dezhou) (2021DZ021).
Acknowledgments
We express our appreciation to all members of Laboratory Animal Center of Peking University Health Science Center for their assistance with maintenance of rats.
Conflict of interest
The authors declare that the research was conducted in the absence of any commercial or financial relationships that could be construed as a potential conflict of interest.
Publisher’s note
All claims expressed in this article are solely those of the authors and do not necessarily represent those of their affiliated organizations, or those of the publisher, the editors and the reviewers. Any product that may be evaluated in this article, or claim that may be made by its manufacturer, is not guaranteed or endorsed by the publisher.
References
Abdallah, M. W., Larsen, N., Grove, J., Norgaard-Pedersen, B., Thorsen, P., Mortensen, E. L., et al. (2012). Amniotic fluid chemokines and autism spectrum disorders: An exploratory study utilizing a Danish historic birth cohort. Brain Behav. Immun. 26 (1), 170–176. doi:10.1016/j.bbi.2011.09.003
American Psychiatric Association (2013). Diagnostic and statistical manual of mental disorders DSM-5. Washington, DC: American Psychiatric Association.
Atanasova, E., Arevalo, A. P., Graf, I., Zhang, R., Bockmann, J., Lutz, A. K., et al. (2023). Immune activation during pregnancy exacerbates ASD-related alterations in Shank3-deficient mice. Mol. Autism 14 (1), 1. doi:10.1186/s13229-022-00532-3
Atladottir, H. O., Thorsen, P., Ostergaard, L., Schendel, D. E., Lemcke, S., Abdallah, M., et al. (2010a). Maternal infection requiring hospitalization during pregnancy and autism spectrum disorders. J. Autism Dev. Disord. 40 (12), 1423–1430. doi:10.1007/s10803-010-1006-y
Atladottir, H. O., Thorsen, P., Schendel, D. E., Ostergaard, L., Lemcke, S., and Parner, E. T. (2010b). Association of hospitalization for infection in childhood with diagnosis of autism spectrum disorders: A Danish cohort study. Arch. Pediatr. Adolesc. Med. 164 (5), 470–477. doi:10.1001/archpediatrics.2010.9
Ayed, M., Embaireeg, A., Kartam, M., More, K., Alqallaf, M., AlNafisi, A., et al. (2022). Neurodevelopmental outcomes of infants born to mothers with SARS-CoV-2 infections during pregnancy: A national prospective study in Kuwait. BMC Pediatr. 22 (1), 319. doi:10.1186/s12887-022-03359-2
Basha, J., Iwasenko, J. M., Robertson, P., Craig, M. E., and Rawlinson, W. D. (2014). Congenital cytomegalovirus infection is associated with high maternal socio-economic status and corresponding low maternal cytomegalovirus seropositivity. J. Paediatr. Child. Health 50 (5), 368–372. doi:10.1111/jpc.12502
Bate, S. L., Dollard, S. C., and Cannon, M. J. (2010). Cytomegalovirus seroprevalence in the United States: The national health and nutrition examination surveys, 1988-2004. Clin. Infect. Dis. 50 (11), 1439–1447. doi:10.1086/652438
Bayerl, D. S., and Bosch, O. J. (2019). Brain vasopressin signaling modulates aspects of maternal behavior in lactating rats. Genes Brain Behav. 18 (1), e12517. doi:10.1111/gbb.12517
Berger, S., Ronovsky, M., Horvath, O., Berger, A., and Pollak, D. D. (2018). Impact of maternal immune activation on maternal care behavior, offspring emotionality and intergenerational transmission in C3H/He mice. Brain Behav. Immun. 70, 131–140. doi:10.1016/j.bbi.2018.02.008
Bhandari, R., Paliwal, J. K., and Kuhad, A. (2020). Neuropsychopathology of autism spectrum disorder: Complex interplay of genetic, epigenetic, and environmental factors. Adv. Neurobiol. 24, 97–141. doi:10.1007/978-3-030-30402-7_4
Bhat, S., Acharya, U. R., Adeli, H., Bairy, G. M., and Adeli, A. (2014). Autism: Cause factors, early diagnosis and therapies. Rev. Neurosci. 25 (6), 841–850. doi:10.1515/revneuro-2014-0056
Bosch, O. J., and Neumann, I. D. (2012). Both oxytocin and vasopressin are mediators of maternal care and aggression in rodents: From central release to sites of action. Hormones Behav. 61 (3), 293–303. doi:10.1016/j.yhbeh.2011.11.002
Cannon, M. J., and Davis, K. F. (2005). Washing our hands of the congenital cytomegalovirus disease epidemic. BMC Public Health 5, 70. doi:10.1186/1471-2458-5-70
Carlezon, W. A., Kim, W., Missig, G., Finger, B. C., Landino, S. M., Alexander, A. J., et al. (2019). Maternal and early postnatal immune activation produce sex-specific effects on autism-like behaviors and neuroimmune function in mice. Sci. Rep. 9 (1), 16928. doi:10.1038/s41598-019-53294-z
Carter, C. S. (2017). The oxytocin-vasopressin pathway in the context of love and fear. Front. Endocrinol. (Lausanne) 8, 356. doi:10.3389/fendo.2017.00356
Cheng, Y., Teng, H., Xiao, Y., Yao, M., Yin, J., and Sun, G. (2021). Impact of SARS-CoV-2 infection during pregnancy on infant neurobehavioral development: A case-control study. Front. Pediatr. 9, 762684. doi:10.3389/fped.2021.762684
Choi, G. B., Yim, Y. S., Wong, H., Kim, S., Kim, H., Kim, S. V., et al. (2016). The maternal interleukin-17a pathway in mice promotes autism-like phenotypes in offspring. Science 351 (6276), 933–939. doi:10.1126/science.aad0314
Ciaranello, A. L., and Ciaranello, R. D. (1995). The neurobiology of infantile autism. Annu Rev. Neurosci. 18, 101–128. doi:10.1146/annurev.ne.18.030195.000533
Croen, L. A., Qian, Y., Ashwood, P., Zerbo, O., Schendel, D., Pinto-Martin, J., et al. (2019). Infection and fever in pregnancy and autism spectrum disorders: Findings from the study to explore early development. Autism Res. 12 (10), 1551–1561. doi:10.1002/aur.2175
Dai, Y. C., Zhang, H. F., Schon, M., Bockers, T. M., Han, S. P., Han, J. S., et al. (2018). Neonatal oxytocin treatment ameliorates autistic-like behaviors and oxytocin deficiency in valproic acid-induced rat model of autism. Front. Cell Neurosci. 12, 355. doi:10.3389/fncel.2018.00355
de Novais, C. O., Batista, T. H., Ribeiro, A., Vitor-Vieira, F., Rojas, V. C. T., Ferri, B. G., et al. (2021). Maternal overweight induced by reduced litter size impairs the behavioral neurodevelopment of offspring. Life Sci. 277, 119611. doi:10.1016/j.lfs.2021.119611
Duan, L., Zhang, X. D., Miao, W. Y., Sun, Y. J., Xiong, G., Wu, Q., et al. (2018). PDGFRβ cells rapidly relay inflammatory signal from the circulatory system to neurons via chemokine CCL2. Neuron 100 (1), 183–200. doi:10.1016/j.neuron.2018.08.030
Enes-Marques, S., and Giusti-Paiva, A. (2018). Litter size reduction accentuates maternal care and alters behavioral and physiological phenotypes in rat adult offspring. J. Physiol. Sci. 68 (6), 789–798. doi:10.1007/s12576-018-0594-8
Estes, M. L., and McAllister, A. K. (2016). Maternal immune activation: Implications for neuropsychiatric disorders. Science 353 (6301), 772–777. doi:10.1126/science.aag3194
Fajardo Martinez, V., Zhang, D., Paiola, S., Mok, T., Cambou, M. C., Kerin, T., et al. (2023). Neuromotor repertoires in infants exposed to maternal COVID-19 during pregnancy: A cohort study. BMJ Open 13 (1), e069194. doi:10.1136/bmjopen-2022-069194
Fombonne, E. (2009). Epidemiology of pervasive developmental disorders. Pediatr. Res. 65 (6), 591–598. doi:10.1203/PDR.0b013e31819e7203
Goines, P. E., Croen, L. A., Braunschweig, D., Yoshida, C. K., Grether, J., Hansen, R., et al. (2011). Increased midgestational IFN-gamma, IL-4 and IL-5 in women bearing a child with autism: A case-control study. Mol. Autism 2, 13. doi:10.1186/2040-2392-2-13
Gzielo, K., Potasiewicz, A., Litwa, E., Piotrowska, D., Popik, P., and Nikiforuk, A. (2021). The effect of maternal immune activation on social play-induced ultrasonic vocalization in rats. Brain Sci. 11 (3), 344. doi:10.3390/brainsci11030344
Haddad, F. L., Patel, S. V., and Schmid, S. (2020). Maternal immune activation by poly I:C as a preclinical model for neurodevelopmental disorders: A focus on autism and schizophrenia. Neurosci. Biobehav Rev. 113, 546–567. doi:10.1016/j.neubiorev.2020.04.012
Hafizi, S., Tabatabaei, D., and Lai, M. C. (2019). Review of clinical studies targeting inflammatory pathways for individuals with autism. Front. Psychiatry 10, 849. doi:10.3389/fpsyt.2019.00849
Haida, O., Al Sagheer, T., Balbous, A., Francheteau, M., Matas, E., Soria, F., et al. (2019). Sex-dependent behavioral deficits and neuropathology in a maternal immune activation model of autism. Transl. Psychiatry 9 (1), 124. doi:10.1038/s41398-019-0457-y
Holingue, C., Brucato, M., Ladd-Acosta, C., Hong, X., Volk, H., Mueller, N. T., et al. (2020). Interaction between maternal immune activation and antibiotic use during pregnancy and child risk of autism spectrum disorder. Autism Res. 13 (12), 2230–2241. doi:10.1002/aur.2411
Jacquemont, S., Coe, B. P., Hersch, M., Duyzend, M. H., Krumm, N., Bergmann, S., et al. (2014). A higher mutational burden in females supports a "female protective model" in neurodevelopmental disorders. Am. J. Hum. Genet. 94 (3), 415–425. doi:10.1016/j.ajhg.2014.02.001
Jenabi, E., Bashirian, S., Khazaei, S., Masoumi, S. Z., Ghelichkhani, S., Goodarzi, F., et al. (2022). Pregnancy outcomes among symptomatic and asymptomatic women infected with COVID-19 in the west of Iran: A case-control study. J. Matern. Fetal Neonatal Med. 35 (24), 4695–4697. doi:10.1080/14767058.2020.1861599
Jiang, H. Y., Xu, L. L., Shao, L., Xia, R. M., Yu, Z. H., Ling, Z. X., et al. (2016). Maternal infection during pregnancy and risk of autism spectrum disorders: A systematic review and meta-analysis. Brain Behav. Immun. 58, 165–172. doi:10.1016/j.bbi.2016.06.005
Kalish, B. T., Kim, E., Finander, B., Duffy, E. E., Kim, H., Gilman, C. K., et al. (2021). Maternal immune activation in mice disrupts proteostasis in the fetal brain. Nat. Neurosci. 24 (2), 204–213. doi:10.1038/s41593-020-00762-9
Kalueff, A. V., Stewart, A. M., Song, C., Berridge, K. C., Graybiel, A. M., and Fentress, J. C. (2016). Neurobiology of rodent self-grooming and its value for translational neuroscience. Nat. Rev. Neurosci. 17 (1), 45–59. doi:10.1038/nrn.2015.8
Keller, M., Vandenberg, L. N., and Charlier, T. D. (2019). The parental brain and behavior: A target for endocrine disruption. Front. Neuroendocrinol. 54, 100765. doi:10.1016/j.yfrne.2019.100765
Kim, J. Y., Son, M. J., Son, C. Y., Radua, J., Eisenhut, M., Gressier, F., et al. (2019). Environmental risk factors and biomarkers for autism spectrum disorder: An umbrella review of the evidence. Lancet Psychiatry 6 (7), 590–600. doi:10.1016/S2215-0366(19)30181-6
Kirsten, T. B., Lippi, L. L., Bevilacqua, E., and Bernardi, M. M. (2013). LPS exposure increases maternal corticosterone levels, causes placental injury and increases IL-1Β levels in adult rat offspring: Relevance to autism. PLoS One 8 (12), e82244. doi:10.1371/journal.pone.0082244
Kowash, H. M., Potter, H. G., Edye, M. E., Prinssen, E. P., Bandinelli, S., Neill, J. C., et al. (2019). Poly(I:C) source, molecular weight and endotoxin contamination affect dam and prenatal outcomes, implications for models of maternal immune activation. Brain Behav. Immun. 82, 160–166. doi:10.1016/j.bbi.2019.08.006
Laurent, H. K., Stevens, A., and Ablow, J. C. (2011). Neural correlates of hypothalamic-pituitary-adrenal regulation of mothers with their infants. Biol. Psychiatry 70 (9), 826–832. doi:10.1016/j.biopsych.2011.06.011
Lee, G. A., Lin, Y. K., Lai, J. H., Lo, Y. C., Yang, Y. S. H., Ye, S. Y., et al. (2021). Maternal immune activation causes social behavior deficits and hypomyelination in male rat offspring with an autism-like microbiota profile. Brain Sci. 11 (8), 1085. doi:10.3390/brainsci11081085
Lins, B. R., Hurtubise, J. L., Roebuck, A. J., Marks, W. N., Zabder, N. K., Scott, G. A., et al. (2018). Prospective analysis of the effects of maternal immune activation on rat cytokines during pregnancy and behavior of the male offspring relevant to schizophrenia. eNeuro 5 (4), e0249. doi:10.1523/ENEURO.0249-18.2018
Lonstein, J. S., and Fleming, A. S. (2002). Parental behaviors in rats and mice. Curr. Protoc. Neurosci. Chapter 8, 15. doi:10.1002/0471142301.ns0815s17
Ludwig, A., and Hengel, H. (2009). Epidemiological impact and disease burden of congenital cytomegalovirus infection in Europe. Euro Surveill. 14 (9), 26–32. doi:10.2807/ESE.14.09.19140-EN
Maenner, M. J., Shaw, K. A., Bakian, A. V., Bilder, D. A., Durkin, M. S., Esler, A., et al. (2021). Prevalence and characteristics of autism spectrum disorder among children aged 8 Years - autism and developmental disabilities monitoring network, 11 sites, United States, 2018. MMWR Surveill. Summ. 70 (11), 1–16. doi:10.15585/mmwr.ss7011a1
Maljaars, J., Boonen, H., Lambrechts, G., Van Leeuwen, K., and Noens, I. (2014). Maternal parenting behavior and child behavior problems in families of children and adolescents with autism spectrum disorder. J. Autism Dev. Disord. 44 (3), 501–512. doi:10.1007/s10803-013-1894-8
Malkova, N. V., Yu, C. Z., Hsiao, E. Y., Moore, M. J., and Patterson, P. H. (2012). Maternal immune activation yields offspring displaying mouse versions of the three core symptoms of autism. Brain Behav. Immun. 26 (4), 607–616. doi:10.1016/j.bbi.2012.01.011
Manjeese, W., Mvubu, N. E., Steyn, A. J. C., and Mpofana, T. (2021). Mycobacterium tuberculosis-induced maternal immune activation promotes autism-like phenotype in infected mice offspring. Int. J. Environ. Res. Public Health 18 (9), 4513. doi:10.3390/ijerph18094513
McColl, E. R., and Piquette-Miller, M. (2021). Viral model of maternal immune activation alters placental AMPK and mTORC1 signaling in rats. Placenta 112, 36–44. doi:10.1016/j.placenta.2021.07.002
Mitre, M., Marlin, B. J., Schiavo, J. K., Morina, E., Norden, S. E., Hackett, T. A., et al. (2016). A distributed network for social cognition enriched for oxytocin receptors. J. Neurosci. 36 (8), 2517–2535. doi:10.1523/JNEUROSCI.2409-15.2016
Narang, K., Miller, M., Trinidad, C., Wick, M., Theiler, R., Weaver, A. L., et al. (2023). Impact of asymptomatic and mild COVID-19 infection on fetal growth during pregnancy. Eur. J. Obstet. Gynecol. Reprod. Biol. 281, 63–67. doi:10.1016/j.ejogrb.2022.12.020
Palomba, S., Marotta, R., Di Cello, A., Russo, T., Falbo, A., Orio, F., et al. (2012). Pervasive developmental disorders in children of hyperandrogenic women with polycystic ovary syndrome: A longitudinal case-control study. Clin. Endocrinol. (Oxf) 77 (6), 898–904. doi:10.1111/j.1365-2265.2012.04443.x
Pangrazzi, L., Balasco, L., and Bozzi, Y. (2020). Natural antioxidants: A novel therapeutic approach to autism spectrum disorders? Antioxidants (Basel) 9 (12), 1186. doi:10.3390/antiox9121186
Parker-Athill, E. C., and Tan, J. (2010). Maternal immune activation and autism spectrum disorder: interleukin-6 signaling as a key mechanistic pathway. Neurosignals 18 (2), 113–128. doi:10.1159/000319828
Ruta, L., Ingudomnukul, E., Taylor, K., Chakrabarti, B., and Baron-Cohen, S. (2011). Increased serum androstenedione in adults with autism spectrum conditions. Psychoneuroendocrinology 36 (8), 1154–1163. doi:10.1016/j.psyneuen.2011.02.007
Schwarze, J., Fitch, P. M., Heimweg, J., Errington, C., Matsuda, R., de Bruin, H. G., et al. (2016). Viral mimic poly-(I:C) attenuates airway epithelial T-cell suppressive capacity: Implications for asthma. Eur. Respir. J. 48 (6), 1785–1788. doi:10.1183/13993003.00841-2016
Solmi, M., Song, M., Yon, D. K., Lee, S. W., Fombonne, E., Kim, M. S., et al. (2022). Incidence, prevalence, and global burden of autism spectrum disorder from 1990 to 2019 across 204 countries. Mol. Psychiatry 27 (10), 4172–4180. doi:10.1038/s41380-022-01630-7
Song, T. J., Lan, X. Y., Wei, M. P., Zhai, F. J., Boeckers, T. M., Wang, J. N., et al. (2019). Altered behaviors and impaired synaptic function in a novel rat model with a complete Shank3 deletion. Front. Cell Neurosci. 13, 111. doi:10.3389/fncel.2019.00111
Tang, Y., Yang, Y., Chen, L., Liu, X., Deng, S., Dai, Y., et al. (2022). Association of prosocial behavior between mothers and their child with autism spectrum disorder: The mediating role of maternal parenting. J. Autism Dev. Disord. 15. doi:10.1007/s10803-022-05487-1
Taniguchi, K., Watanabe, N., Sato, A., Jwa, S. C., Suzuki, T., Yamanobe, Y., et al. (2014). Changes in cytomegalovirus seroprevalence in pregnant Japanese women-a 10-year single center study. J. Clin. Virol. 59 (3), 192–194. doi:10.1016/j.jcv.2013.12.013
Thawley, A. J., Veneziani, L. P., Rabelo-da-Ponte, F. D., Riederer, I., Mendes-da-Cruz, D. A., and Bambini-Junior, V. (2022). Aberrant IL-17 levels in rodent models of autism spectrum disorder: A systematic review. Front. Immunol. 13, 874064. doi:10.3389/fimmu.2022.874064
Thaxton, J. E., Nevers, T., Lippe, E. O., Blois, S. M., Saito, S., and Sharma, S. (2013). NKG2D blockade inhibits poly(I:C)-triggered fetal loss in wild type but not in IL-10-/- mice. J. Immunol. 190 (7), 3639–3647. doi:10.4049/jimmunol.1203488
Tioleco, N., Silberman, A. E., Stratigos, K., Banerjee-Basu, S., Spann, M. N., Whitaker, A. H., et al. (2021). Prenatal maternal infection and risk for autism in offspring: A meta-analysis. Autism Res. 14 (6), 1296–1316. doi:10.1002/aur.2499
Traynor, T. R., Majde, J. A., Bohnet, S. G., and Krueger, J. M. (2004). Intratracheal double-stranded RNA plus interferon-gamma: A model for analysis of the acute phase response to respiratory viral infections. Life Sci. 74 (20), 2563–2576. doi:10.1016/j.lfs.2003.10.010
Vergara-Merino, L., Meza, N., Couve-Perez, C., Carrasco, C., Ortiz-Munoz, L., Madrid, E., et al. (2021). Maternal and perinatal outcomes related to COVID-19 and pregnancy: An overview of systematic reviews. Acta Obstet. Gynecol. Scand. 100 (7), 1200–1218. doi:10.1111/aogs.14118
Vimercati, A., De Nola, R., Trerotoli, P., Metta, M. E., Cazzato, G., Resta, L., et al. (2022). COVID-19 infection in pregnancy: Obstetrical risk factors and neonatal outcomes-A monocentric, single-cohort study. Vaccines (Basel) 10 (2), 166. doi:10.3390/vaccines10020166
Vorhees, C. V., Graham, D. L., Braun, A. A., Schaefer, T. L., Skelton, M. R., Richtand, N. M., et al. (2012). Prenatal immune challenge in rats: Altered responses to dopaminergic and glutamatergic agents, prepulse inhibition of acoustic startle, and reduced route-based learning as a function of maternal body weight gain after prenatal exposure to poly IC. Synapse 66 (8), 725–737. doi:10.1002/syn.21561
Wang, J., Yin, T., Wen, Y., Tian, F., He, X., Zhou, D., et al. (2015). Potential effects of interferon regulatory factor 4 in a murine model of polyinosinic-polycytidylic acid-induced embryo resorption. Reprod. Fertil. Dev. 28 (10), 1631–1641. doi:10.1071/RD14499
Wang, X., McCoy, P. A., Rodriguiz, R. M., Pan, Y., Je, H. S., Roberts, A. C., et al. (2011). Synaptic dysfunction and abnormal behaviors in mice lacking major isoforms of Shank3. Hum. Mol. Genet. 20 (15), 3093–3108. doi:10.1093/hmg/ddr212
Wilkinson, M., Johnstone, E. D., Simcox, L. E., and Myers, J. E. (2022). The impact of COVID-19 on pregnancy outcomes in a diverse cohort in England. Sci. Rep. 12 (1), 942. doi:10.1038/s41598-022-04898-5
Wu, T., Chen, L., Wang, Y., Shi, H., Niu, J., Yin, X., et al. (2021b). Effects of SARS-CoV-2 infection during late pregnancy on early childhood development: A prospective cohort study. Front. Pediatr. 9, 750012. doi:10.3389/fped.2021.750012
Xu, X. J., Shou, X. J., Li, J., Jia, M. X., Zhang, J. S., Guo, Y., et al. (2013). Mothers of autistic children: Lower plasma levels of oxytocin and arg-vasopressin and a higher level of testosterone. PLoS One 8 (9), e74849. doi:10.1371/journal.pone.0074849
Zambon, A., Rico, L. C., Herman, M., Gundacker, A., Telalovic, A., Hartenberger, L. M., et al. (2022). Gestational immune activation disrupts hypothalamic neurocircuits of maternal care behavior. Mol. Psychiatry, 1–15. doi:10.1038/s41380-022-01602-x
Zeidan, J., Fombonne, E., Scorah, J., Ibrahim, A., Durkin, M. S., Saxena, S., et al. (2022). Global prevalence of autism: A systematic review update. Autism Res. 15 (5), 778–790. doi:10.1002/aur.2696
Zhang, H. F., Dai, Y. C., Wu, J., Jia, M. X., Zhang, J. S., Shou, X. J., et al. (2016). Plasma oxytocin and arginine-vasopressin levels in children with autism spectrum disorder in China: Associations with symptoms. Neurosci. Bull. 32 (5), 423–432. doi:10.1007/s12264-016-0046-5
Keywords: poly (I:C), maternal immune activation, autism-like behavior, maternal behavior, dam, offspring
Citation: Lan X-Y, Gu Y-Y, Li M-J, Song T-J, Zhai F-J, Zhang Y, Zhan J-S, Böckers TM, Yue X-N, Wang J-N, Yuan S, Jin M-Y, Xie Y-F, Dang W-W, Hong H-H, Guo Z-R, Wang X-W and Zhang R (2023) Poly(I:C)-induced maternal immune activation causes elevated self-grooming in male rat offspring: Involvement of abnormal postpartum static nursing in dam. Front. Cell Dev. Biol. 11:1054381. doi: 10.3389/fcell.2023.1054381
Received: 26 September 2022; Accepted: 06 March 2023;
Published: 16 March 2023.
Edited by:
Jun Wang, Hubei University of Technology, ChinaReviewed by:
Ewa Litwa, Maj Institute of Pharmacology, Polish Academy of Sciences, PolandXinchun Jin, Capital Medical University, China
Copyright © 2023 Lan, Gu, Li, Song, Zhai, Zhang, Zhan, Böckers, Yue, Wang, Yuan, Jin, Xie, Dang, Hong, Guo, Wang and Zhang. This is an open-access article distributed under the terms of the Creative Commons Attribution License (CC BY). The use, distribution or reproduction in other forums is permitted, provided the original author(s) and the copyright owner(s) are credited and that the original publication in this journal is cited, in accordance with accepted academic practice. No use, distribution or reproduction is permitted which does not comply with these terms.
*Correspondence: Rong Zhang, zhangrong@bjmu.edu.cn
†These authors have contributed equally to this work and share first authorship