- Université Paris-Saclay, CNRS, BioCIS, Orsay, France
Acanthamoeba castellanii is a widespread Free-Living Amoeba (FLA) that can cause severe ocular or cerebral infections in immunocompetent and immunocompromised patients, respectively, besides its capacity to transport diverse pathogens. During their life cycle, FLA can alternate between a vegetative form, called a trophozoite, and a latent and resistant form, called a cyst. This resistant form is characterized by the presence of a cell wall containing two layers, namely the ectocyst and the endocyst, mainly composed of cellulose and proteins. In the present work, we aimed to stimulate Acanthamoeba castellanii excystment by treating their cysts with a cellulolytic enzyme, i.e., cellulase, or two proteolytic enzymes, i.e., collagenase and pepsin. While 11 days were necessary to obtain total excystment in the control at 27°C, only 48 h were sufficient at the same temperature to obtain 100% trophozoites in the presence of 25 U/mL cellulase, 50 U/mL collagenase or 100 U/mL pepsin. Additionally, more than 96% amoebae have excysted after only 24 h with 7.5 U/mL cellulase at 30°C. Nevertheless, no effect of the three enzymes was observed on the excystment of Balamuthia mandrillaris and Vermamoeba vermiformis. Surprisingly, A. castellanii trophozoites excysted in the presence of cellulase displayed a markedly shorter doubling time at 7 h, in comparison to the control at 23 h. Likewise, trophozoites doubled their population in 9 h when both cellulose and cellulase were added to the medium, indicating that Acanthamoeba cyst wall degradation products promote their trophozoite proliferation. The analysis of cysts in epifluorescent microscopy using FITC-lectins and in electron microscopy revealed a disorganized endocyst and a reduction of the intercystic space area after cellulase treatment, implying that these cellular events are preliminary to trophozoite release during excystment. Further studies would be necessary to determine the signaling pathways involved during this amoebal differentiation process to identify new therapeutic targets for the development of anti-acanthamoebal drugs.
Introduction
Free-living amoebae (FLA) are unicellular eukaryotes widely distributed in natural or artificial environments such as soil, rivers, swimming pools, or drinking water reservoirs (Rodriguez-Zaragoza, 1994; Thomas and Ashbolt, 2011). These protozoa have the ability to live and multiply autonomously in nature, in opposition to parasitic amoebae, such as Entamoeba histolytica requiring a host to survive (Martinez and Visvesvara, 1997). They constitute a polyphyletic group including Amoebozoa, the most diverse and the only one exclusively composed of amoebae, Rhizaria, Discoba, Opisthokonta, and others (Burki et al., 2020; Shi et al., 2021). Among them, FLA belonging to the genera Acanthamoeba, Balamuthia, Naegleria, Sappinia and Vermamoeba (previously named Hartmannella) can become pathogenic to human and cause severe ocular or cerebral infections (Aitken et al., 1996; Centeno et al., 1996; Visvesvara et al., 2007). Especially, Acanthamoeba sp. is able to provoke amoebic keratitis which can be treated by a combination of chlorhexidine with polyhexamethylene biguanide or propamidine but leads to a treatment failure in around 40% of the cases (Randag et al., 2019; Scruggs et al., 2022). Importantly, this FLA can also give rise to granulomatous amoebic encephalitis for which no effective monotherapy is presently available. Despite the use of current therapies empirically associating a large cocktail of drugs, a high mortality is observed, above 90% of the cases (Visvesvara et al., 2007; Taravaud et al., 2021).
During its life cycle, Acanthamoeba sp. can alternate between two life stages: the trophozoite, a vegetative form able to reproduce, feed and move via cytoplasmic extensions called pseudopods, and the cyst, a dormant and resistant form allowing the amoeba to survive in hostile environmental conditions (Khan, 2006). The formation of mature cyst requires three main steps: 1) rounding and immobilization of the trophozoite form, 2) dehydration during which the cell loses most of its fluids and decreases in volume, and 3) the formation of the double-layered cyst wall with the arrangement of the outer layer, the ectocyst, before the fibrillar inner layer, the endocyst, providing cell protection (Bowers and Korn, 1969). Encystment is regulated by several signaling pathways such as cAMP synthesis, MAPK pathway, autophagy, cellulose synthesis, or DNA methylation involving diverse enzymes or proteins such as kinases, proteases, methyltransferases, acetyltransferases, deacetylases or heat shock proteins (Moon et al., 2007; Moon et al., 2009; Moon et al., 2011a; Moon et al., 2011b; Moon et al., 2012a; Moon et al., 2012b; Fouque et al., 2012; Moon and Kong, 2012; Song et al., 2012; Moon et al., 2013; Moon et al., 2016; Moon et al., 2017; Joo et al., 2020; Rolland et al., 2020; Wang et al., 2021). More recently, a time-resolved multi-omics analysis has revealed a rapid phospho-regulation of cytoskeleton and translation factors during encystment (Bernard et al., 2022). The cyst wall of A. castellanii is mainly composed of acid-resistant proteins and cellulose, this latter component being present in both the endocyst and the ectocyst, especially in the inner layer where it represents one third of the dry weight (Weisman, 1976; Chavez-Munguia et al., 2005; Dudley et al., 2009). Conversely, Acanthamoeba sp. excystment starts with a cytoplasmic bud protruding through an ostiole, corresponding to a cyst pore where both endo- and ectocyst layers are in contact, allowing emergence of the trophozoite from the empty cyst wall (Chambers and Thompson, 1972; Chavez-Munguia et al., 2005). Although the pathways involved in Acanthamoeba sp. excystment are currently not well known, this cellular process is typically accompanied by the secretion of depolymerizing enzymes such as cellulase or proteases (Krishna-Murti and Shukla, 1984). Some studies have reported a cysticidal effect of cellulase and a subtilisin-like serine protease treatment on Acanthamoeba sp. and Vermamoeba vermiformis, respectively (Fouque et al., 2015a; Ortilles et al., 2017; Lazuana et al., 2019). Moreover, in several Acanthamoeba species, treatments by cellulase and pancreatic protease can hydrolyze more than half of cyst wall carbohydrates and proteins, respectively (Barrett and Alexander, 1977). However, to the best of our knowledge, no study in the literature has described an influence of cellulase or protease treatment on FLA excystment.
The in vitro culture of FLA isolated from the environment requires media with a wide range of different components depending on the FLA genera (Schuster, 2002), giving a limitative diversity of amoebae since only environmental FLA that are able to grow in these media will be selected (Denet et al., 2017). Moreover, even after using a panel of different media, some environmental FLA, isolated in the cyst form, were not able to develop in culture in vitro, indicating that they are either dead, or viable but non-culturable (Taravaud et al., 2018). In order to facilitate the in vitro development of FLA cysts isolated from the environment and thus to determine their viability, we attempted in the present work to gently digest their cyst wall in order to stimulate trophozoite release from cysts, and therefore their proliferation in culture. The effect of different conditions of treatment by cellulolytic and proteolytic enzymes was thus analyzed on FLA excystment. The influence of cellulase was further investigated on Acanthamoeba castellanii trophozoite growth following their release from cysts. Cellulase activity was then studied on A. castellanii cysts at the cellular and ultrastructural levels in epifluorescence microscopy using lectin labeling and in transmission electron microscopy, respectively.
Materials and methods
Chemicals
All chemicals were purchased from Merck Laboratories (St-Quentin-Fallavier - France), unless otherwise stated. The enzymes used in this study were cellulase from Aspergillus niger, chitinase from Streptomyces griseus, collagenase type I from Clostridium histolyticum and pepsin from porcine gastric mucosa and were purchased from Merck Laboratories (St-Quentin-Fallavier - France). Fetal Bovine Serum (FBS) was provided by Fisher Scientific (Illkirch, France).
FLA culture
Acanthamoeba castellanii (ATCC 30010 strain) was grown axenically in PYG medium (ATCC medium 712) composed of 2% (w/v) peptone protein, 0.1% (w/v) yeast extract, 100 mM glucose, 4 mM MgSO4, 400 µM CaCl2, 3.4 mM sodium citrate, 2.5 mM Na2HPO4, 2.5 mM KH2PO4, 50 µM Fe (NH4)2(SO4)2, pH 6.5 (Magistrado-Coxen et al., 2019).
Vermamoeba vermiformis (ATCC 50237 strain) was cultured axenically in PYNFH medium (ATCC medium 1,034) containing 1% (w/v) bacto-peptone, 1% (w/v) yeast extract, 0.1% (w/v) RNA type VI from torula yeast, 34 µM folic acid, 1.5 µM hemin, 3.5 mM Na2HPO4, 2.7 mM KH2PO4, 10% (v/v) FBS, pH 6.5 (Fouque et al., 2015a).
Balamuthia mandrillaris (ATCC 50209 strain) was cultured in RPMI-1640 medium (Merck, Saint-Quentin-Fallavier, France) supplemented with 10% FBS and penicillin at 100 U/mL and streptomycin at 100 μg/ml (Fisher Scientific, Illkirch, France) in the presence of a monolayer of Vero cells (ATCC, Manassas, VA, USA), as previously described (Schuster and Visvesvara, 1996).
A. castellanii and V. vermiformis were grown at 27°C in the dark without shaking and B. mandrillaris was cultivated at 37°C with 5% of CO2 in the dark without shaking. All FLA were sub-cultured with a starting density at 5 × 104 amoebae/mL twice a week in these conditions.
Encystment
The encystment method was the same for the three FLA used in this study, namely Acanthamoeba castellanii, Vermamoeba vermiformis and Balamuthia mandrillaris, and was adapted from the method previously developed by Fouque et al., 2012. Briefly, 15 mL of a culture of FLA at 106 trophozoites/mL were centrifuged at room temperature at 3,000 g for 10 min, before resuspension and 3 washes in Neff buffer containing 0.1 M KCl, 8 mM MgSO4, 0.4 mM CaCl2, 1 mM NaHCO3, 20 mM Tris-HCl, pH 8.8 (Neff et al., 1964). Amoebae were then incubated in 15 mL of Neff buffer at 27°C for 5 days, and then further treated for 10 min at room temperature with 0.5% (w/v) SDS (Sodium Dodecyl Sulfate) to eliminate the remaining trophozoite and pseudocyst forms which are sensitive to SDS, unlike mature cysts. The selected mature cysts were then washed 3 times in PAS (Page’s Amoeba Saline) buffer (ATCC medium 1,323) containing 1 mM Na2HPO4, 1 mM KH2PO4, 16 µM MgSO4, 27 µM CaCl2, 2 mM NaCl, and then stored at 4°C in PAS buffer.
Excysment
Excystment experiments were performed in the presence or absence (control) of cellulase, collagenase and/or pepsin, with a starting density of 5 × 104 cysts/mL in 2 mL of FLA culture media, namely PYG for A. castellanii, PYNFH for V. vermiformis and complete RPMI-1640 for B. mandrillaris in a 24 well plate. The conditions of enzymatic treatments, in terms of enzyme concentrations, temperatures and incubation times, are described in the text. At the end of each treatment condition, the total number of cells, including cysts and trophozoites, was counted using a Malassez counting chamber (Fisher Scientific; France, Illkirch), then the culture was centrifuged at 3,000 g for 10 min at room temperature and treated with 0.5% (w/v) SDS for 10 min at room temperature to determine the density of mature cysts using a Nageotte counting chamber (Fisher Scientific; France, Illkirch). The number of trophozoites was then calculated by subtracting the number of mature cysts to the number of total cells. Cyst and trophozoite densities were further converted in percentages in comparison to total cells, corresponding to 100%, in order to determine their proportions in the total amoebal population.
Trophozoite growth
A. castellanii trophozoite growth was determined at 27°C, with a starting density of 5 × 104 ameobae/mL, in PYG medium supplemented with or without (control) 7.5 U/mL cellulase, 1 g/L, 2.5 g/L or 5 g/L cellulose, 50 mM, 100 mM or 150 mM glucose, 1 g/L, 2.5 g/L or 5 g/L cellulose with 7.5 U/mL cellulase, and 1 g/L, 2.5 g/L or 5 g/L cellulose with 7.5 U/mL cellulase pre-incubated 24 h at 27°C in cell-free PYG medium before adding trophozoites. The cell density was determined each day for a period of 13 days, to allow observation of stationary phase in trophozoite growth curve after excystment, by cell counting under light microscope using a Malassez chamber.
Fluorescence microscopy
The lectin labelling protocol was adapted from the method previously described by Elloway et al., 2004. Briefly, after an incubation of A. castellanii cysts at a density of 5 × 104 cysts/mL in the presence or absence (control) of cellulase at 7.5 U/mL at 27°C for 48h, amoebae were centrifuged at 3,000 g for 15 min at room temperature. The pellet was then washed three times in buffer A containing 10 mM HEPES pH 7.5, 100 μM CaCl2, 100 μM MnCl2 and 100 μM MgCl2, and further resuspended in 200 μL of buffer A. A volume of 100 μL of a FITC-lectin (Fluorescein-5-isothiocyanate-conjugated lectin) was further added to the amoeba suspension at a final concentration of 10 μg/ml. The FITC-lectins used in the present study were: Con A (Concanavalin A), GSL II (Griffonia Simplicifolia Lectin II), Jacalin, LCA (Lens Culinaris Agglutinin), LEL (Lycopersicon Esculentum Lectin), SBA (SoyBean Agglutinin), VVL (Vicia Villosa Lectin, also called VVA), WGA (Wheat Germ Agglutinin) or SWGA (Succinylated Wheat Germ Agglutinin; Eurobio, Les Ulis, France). After 30 min of labeling at 4°C in the dark, the amoebae were centrifuged at 3,000 g for 10 min at 4°C, washed 3 times and further resuspended in buffer A. The FITC-lectin-labelled cysts were then observed with an inverted epifluorescence microscope Leica Dmi8 (Leica, Nanterre, France) equipped with a Leica DFC9000 GT cooled monochrome camera (4.2 megapixels) and controlled by the Leica Application Suite software (LAS X 3.7.0.20979). Images were further processed in ImagesJ (version 1.8.0_172, Bethesda, MD, USA).
Transmission electron microscopy
The protocol of sample preparation for electron microscopy was adapted from Taravaud et al., 2017. After a treatment of A. castellanii cysts at a density of 5 × 104 cysts/mL in the presence or absence (control) of cellulase at 5 U/mL at 27°C for 48h, amoebae were washed 3 times with PAS buffer, centrifuged at 3,000 g for 15 min at room temperature and fixed 2 h at room temperature with fixative buffer containing 3% (v/v) glutaraldehyde, 1% (v/v) para-formaldehyde, 0.1 M cacodylate pH 7.5. Cells were washed 3 times with 0.1 M cacodylate buffer pH 7.5, then incubated for 1 h at room temperature in 1% (w/v) osmium tetroxide pH 7.5 and further rinsed 3 times in 0.1 M cacodylate pH 7.5. The first inclusion was performed by putting pellets in 2% Low Melting Point (LMP) agarose (Merck, Saint-Quentin-Fallavier, France) to obtain concentrated pellets in 1 mm3 cubes. Then the pellets were dehydrated in graded acetone series: 50% (2 × 1 min), 70% (2 × 5 min), 90% (2 × 15 min) and 100% (4 × 15 min). Embedding in epoxy resin (Low Viscosity Premix Kit Medium, Agar Scientific, Oxford instruments, Oxford, United Kingdom) was performed in graded series (50–100%) in 2 days. Blocs were polymerized for 24 h at 60°C. Ultrathin sections (80 nm) were cut with an ultramicrotome EM UC6 (Leica Microsystems, Nanterre, France), collected on formvar carbon-coated copper grids, and then stained for 15 min with 2% uranyl acetate (Merck, Saint-Quentin-Fallavier, France), washed 3 times with water and treated with 3% Reynolds lead citrate (Reynolds, 1963) for 7 min before observation. Grids were washed 3 times with water before examination under a JEM 1400 TEM operating at 120 kV (JEOL). TEM Images were acquired using a post-column high-resolution (9 megapixels) high-speed camera (RIO9; Gatan, AMATEK, Elancourt, France) and processed with Digital Micrograph software (GMS3, Gatan, AMATEK, Elancourt, France). Vesicle and intercystic space areas were measured by using the option “Polygon selections” in ImageJ software (version 1.8.0_172; USA, Bethesda, MD).
Statistical analyses
Non-parametric two-tailed Mann-Whitney test (p < 0.05) was performed for comparisons between groups. All statistical analyses were performed with GraphPad Prism (version 9.3.1; San Diego, CA, USA).
Results
The purpose of this work was to analyze the effect of a slight digestion of A. castellanii cysts by cellulase and proteases on their excystment, cellulose and proteins being the main components of Acanthamoeba sp. cyst walls (Weisman, 1976). As a cysticidal effect was previously observed with cellulase at concentrations of 500 U/mL and 1500 U/mL on Acanthamoeba sp. (Ortilles et al., 2017; Lazuana et al., 2019), we treated A. castellanii cysts with a range of lower concentrations, from 5 U/mL to 50 U/mL, in order to avoid cyst death. Different conditions of temperatures, from 27 to 37°C, and incubation times, from 24 to 72 h, were also evaluated in order to determine optimal conditions of treatment (Figure 1). At 24 h of treatment, at all temperatures analyzed, no excystment of A. castellanii was observed at 5 U/mL, as for the untreated control (Figure 1A). However, at the same incubation time with 7.5 U/mL cellulase, more than 96% of the amoebae were in the trophozoite form at 30°C, while no or very little effect was observed at the other temperatures (Figure 1A). Moreover, from 10 U/mL to 50 U/mL of cellulase more than 95% of the amoebae were in the trophozoite form at 24 h of treatment at all temperatures evaluated (Figure 1A). At 48 h of treatment, 100% of the amoebae were in the cyst form in the untreated control at 30°C, while 55% and more than 98% of the amoebae were in the trophozoite form at the lowest cellulase concentration of 5 U/mL and in the range from 7.5 U/mL to 50 U/mL, respectively (Figure 1B). However, at the same incubation time, at 27°C and 37°C, excystment started only at 7.5 U/mL, with 24–46% of trophozoite forms observed, and became considerable, with more than 82% of trophozoites, at higher concentrations (Figure 1B). Interestingly, no effect was observed on A. castellanii excystment with treatments in the same conditions of temperatures and incubation times for chitinase, a glycoside hydrolase acting on chitin, a polymer of N-acetyl-β-D-glucosamine distinct from the chains of D-glucose constituting cellulose (Supplementary Figure S1; Linder et al., 2002; Derda et al., 2009).
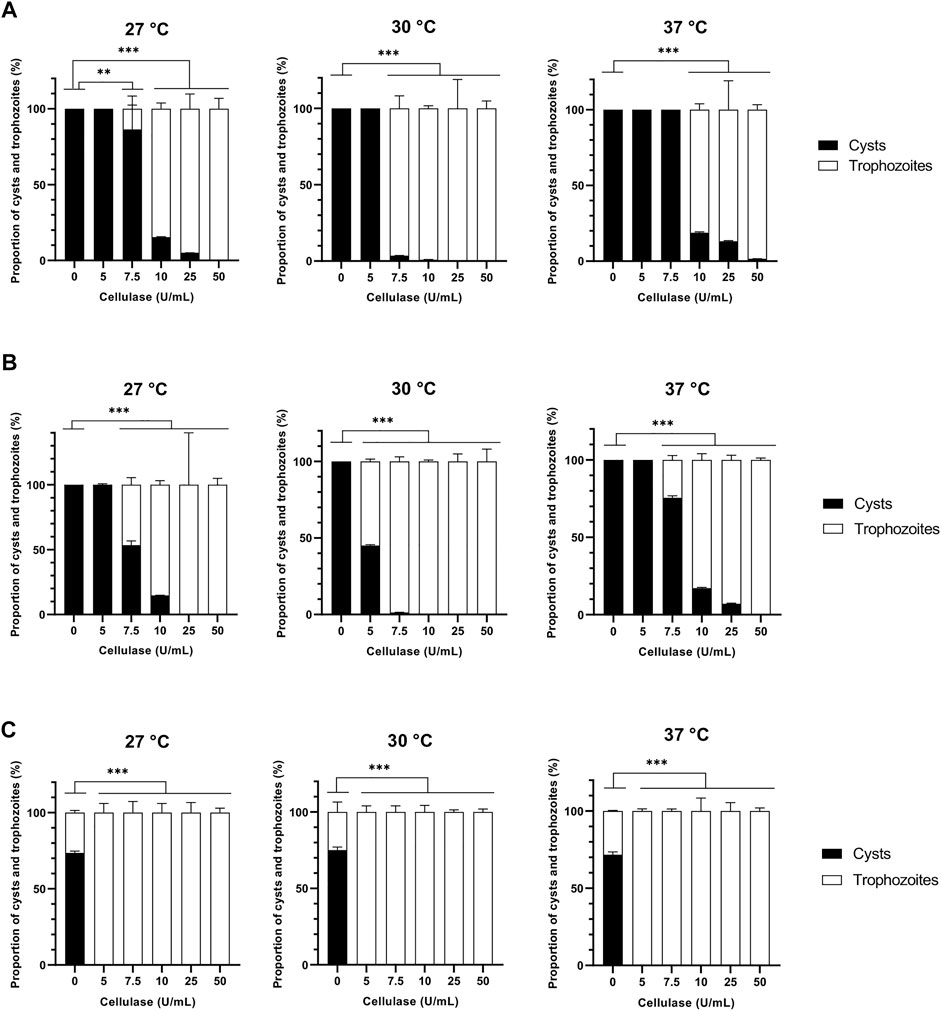
FIGURE 1. Effect of cellulase on Acanthamoeba castellanii excystment. Proportions of A. castellanii trophozoites and cysts observed following 24 h (A), 48 h (B) and 72 h (C) of cellulase treatment at 27, 30 and 37°C as a function of enzyme concentration. The results correspond to the mean of four independent experiments ±SD.
The proteolytic enzymes selected in this work were two proteases from distinct classes, namely collagenase and pepsin belonging to metalloproteases and aspartic proteases, respectively (Elsasser and Goettig, 2021). No effect was observed for both proteases on A. castellanii excystment at 24 h of treatment at all temperatures and concentrations used (Figure 1A, Figure 2A). After 48 h of collagenase treatment, at 27, 30 and 37°C, excystment was noticed from the lowest concentration of 5 U/mL with a proportion of 67% of trophozoites, which increased at 72–88% at 10 U/mL, and was above 90% from 25 U/mL to 100 U/mL (Figure 2B). After 48 h of pepsin treatment, at all temperatures used, 88%–92% of cysts were observed at 5 U/mL, and 60%–82% of trophozoites from the concentration of 25 U/mL (Figure 3B). Nonetheless, at the same treatment time, at 10 U/mL the proportion of excystment was different depending on the temperature: around half of the amoebae were already in the trophozoite form at 27°C and 30°C, while only 12% of this amoeba form were observed at 37°C (Figure 3B).
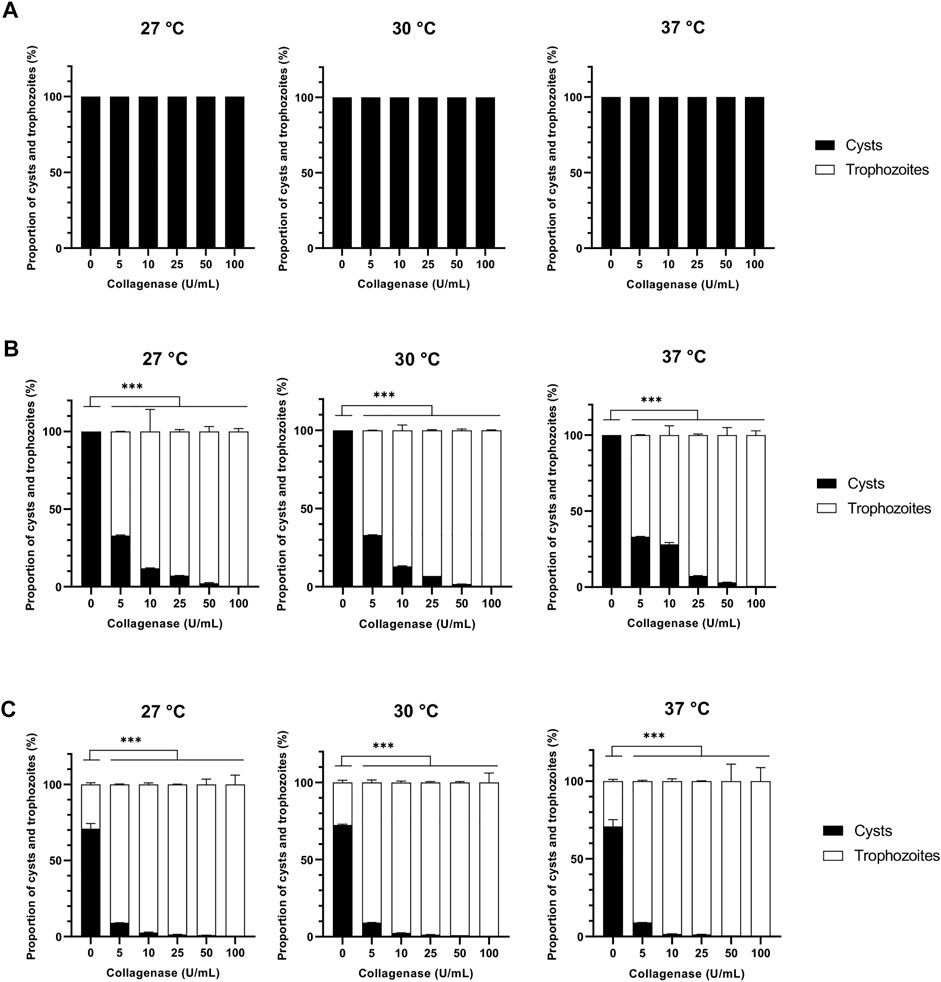
FIGURE 2. Effect of collagenase on Acanthamoeba castellanii excystment. Proportions of A. castellanii trophozoites and cysts observed following 24 h (A), 48 h (B) and 72 h (C) of collagenase treatment at 27, 30 and 37°C as a function of enzyme concentration. The results correspond to the mean of four independent experiments ±SD.
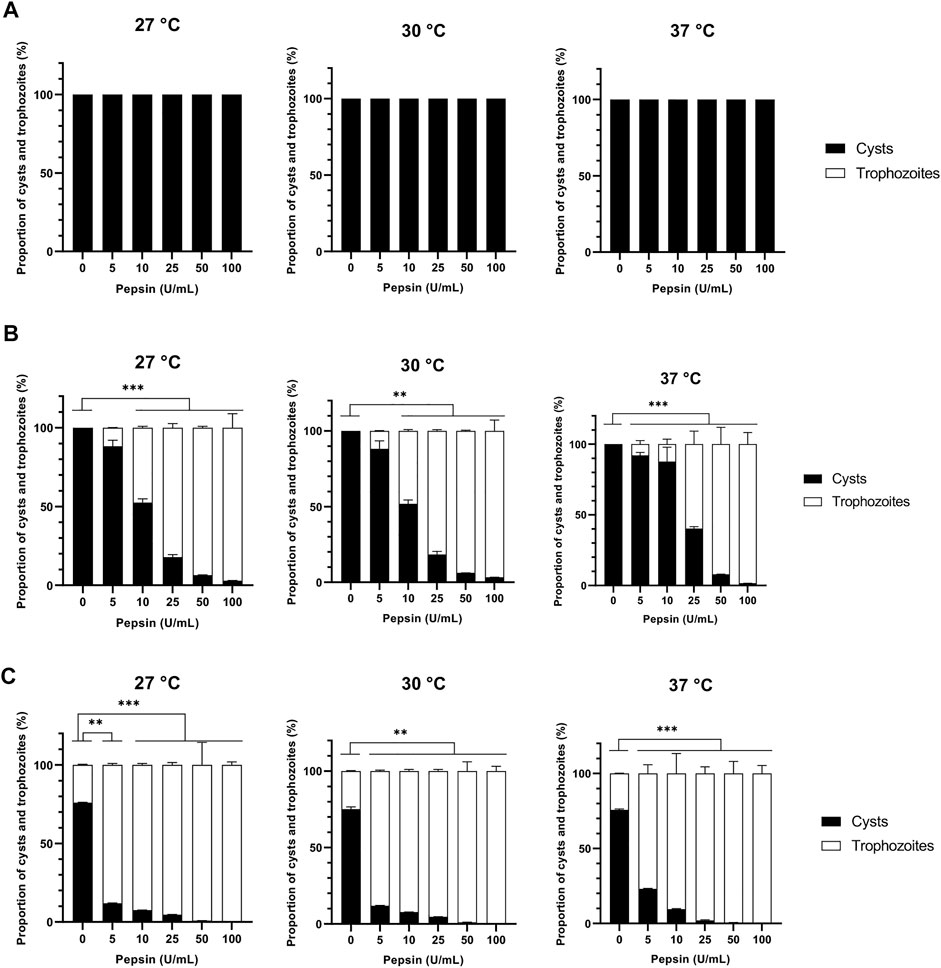
FIGURE 3. Effect of pepsin on Acanthamoeba castellanii excystment. Proportions of A. castellanii trophozoites and cysts observed following 24 h (A), 48 h (B) and 72 h (C) of pepsin treatment at 27, 30 and 37°C as a function of enzyme concentration. The results correspond to the mean of four independent experiments ±SD.
After 72 h of treatment, at 27°C, 30°C and 37°C, and all concentrations used for the 3 enzymes, more than 76%, above 90% and 100% of the pepsin-, collagenase- and cellulase-treated amoebae were in the trophozoite form, respectively, while only 27–36% of trophozoites was observed in the untreated control (Figure 1C, Figure 2C, Figure 3C). In the absence of treatment at 27°C, a total excystment, with 100% trophozoites and no cysts, was only observed after 11 days of incubation in culture medium (Figure 6B). These results show that the 3 enzymes used in the present study can accelerate considerably the cellular process of amoeba excystment, with the following optimal conditions to obtain more than 96% excysted trophozoites in the shortest period of time with the lowest enzyme concentration: 7.5 U/mL for 24 h at 30°C for cellulase, 50 U/mL for 48 h at 27°C, 30°C or 37°C for collagenase, and 100 U/mL for 48 h, also independently of the temperature, for pepsin (Table 1).
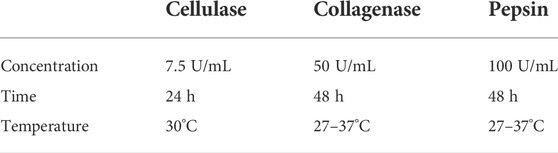
TABLE 1. Summary of the optimal conditions of enzyme treatment for A. castellanii excystment. Optimal condition is defined as the enzyme treatment condition where more than 96% amoebae have excysted in the shortest period of time with the lowest enzyme concentration.
Remarkably, no trophozoite of A. castellanii was observed after incubation of cysts at 30°C after up to 96 h in the presence of 100 U/mL pepsin in a culture medium at pH 2, corresponding to the optimal pH of this enzyme. These cysts did not further excyst and develop as a trophozoite culture after a passage in a regular culture medium without pepsin, in opposition to pepsin-untreated control at pH 2 which excysted and presented 100% of the trophozoite form after 18 days, corresponding to 7 days later than the untreated control at pH 6.5 (Supplementary Figure S2).
In addition, treatments with two by two associations of cellulase, collagenase and pepsin were further investigated in order to determine any supplemental effect on excystment kinetics (Figure 4). Cysts were therefore treated with enzyme associations at 30°C with individual enzyme concentrations at 5 U/mL and were further analyzed after 8 h, 24 h, and 48 h (Figure 4), as no excystment was observed in these conditions for the three enzymes after 24 h of treatment (Figure 1A, Figure 2A, Figure 3A). For all enzyme associations at 30°C, 100% cysts was observed after 8 h, and above 95% of trophozoites after 48 h (Figure 4). However, only 12–67% of trophozoites was observed for individual enzymatic treatments at this latter time of incubation (Figure 1B, Figure 2B, Figure 3B). Moreover, after 24 h at 30°C, while 100% cysts was observed when both proteases were associated, trophozoites started to be observed, with less than 10%, when cellulase was combined with either collagenase or pepsin (Figure 4). These data indicate that all enzyme associations, and especially the combination of cellulase with either collagenase or pepsin, have an additive effect on A. castellanii excystment kinetics.
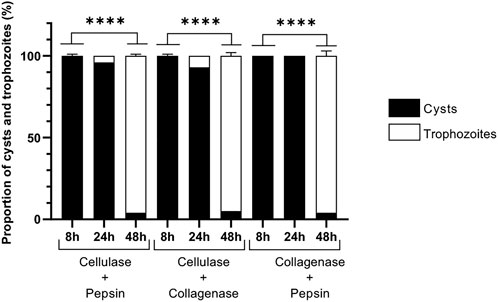
FIGURE 4. Effect of enzyme combinations on Acanthamoeba castellanii excystment. Proportions of A. castellanii trophozoites and cysts observed following 8, 24 and 48 h of treatment by enzyme associations at 30°C. In these associations, all enzyme concentrations were at 5 U/mL. The results correspond to the mean of three independent experiments ±SD.
The action of cellulase, collagenase and pepsin treatment was also investigated on the excystment of two other FLA, Vermamoeba vermiformis and Balamuthia mandrillaris, in the optimal conditions determined for A. castellanii (Figure 5). In these conditions, no effect was observed of all 3 enzymes on B. mandrillaris excystment, as only cysts were observed after 2 days of treatment (Figure 5). Likewise, on V. vermiformis, no trophozoite was observed with collagenase and pepsin (Figure 5). Moreover, these B. mandrillaris and V. vermiformis treated cysts were able to further excyst and grow in a culture medium, showing their viability (data not shown). However, V. vermiformis cysts incubated with cellulase were completely lysed after treatment (Figure 5).
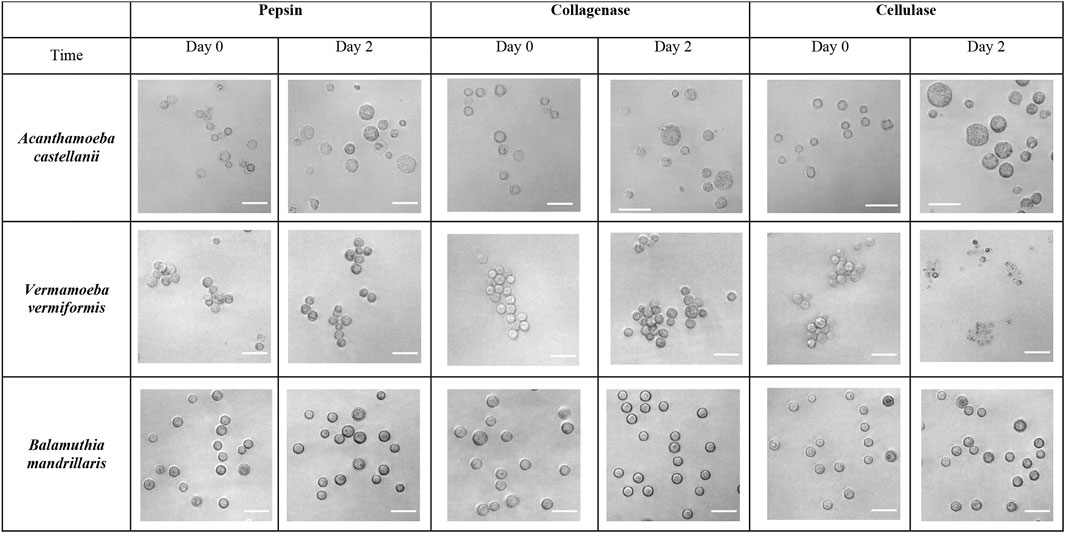
FIGURE 5. Effect of cellulase, collagenase and pepsin on the excystment of Acanthamoeba castellanii, Vermamoeba vermiformis, and Balamuthia mandrillaris. Phase contrast observation of A. castellanii, V. vermiformis, and B. mandrillaris before (Day 0) and after treatment of their cysts for 2 days at 30°C by 100 U/mL pepsin, 50 U/mL collagenase, or 7.5 U/mL cellulase. Scales bars = 20 µm.
Thereafter, the action of cellulase was investigated further as this enzyme showed a more rapid effect on A. castellanii excystment after 24 h of treatment, in comparison to both collagenase or pepsin (Figures 1–3). In particular, trophozoite growth was analyzed after excystment in the presence of 7.5 U/mL cellulase at 27°C, in comparison to the untreated control (Figures 6A,B). Intriguingly, while trophozoite stationary phase was obtained with a similar amoebal density at approximately 4.5 × 106 amoebae/mL in both conditions, this phase was reached at the 6th day of treatment in the presence of cellulase, with a very rapid doubling time of 7 h, and only at the 10th day in the untreated control, with a doubling time of only 23 h (Figures 6A,B). Additionally, after a passage following excystment in the presence or absence of cellulase, no growth difference was observed with trophozoites cultured with or without cellulase (Figures 6C,D). However, when non-freshly excysted, regularly passed, trophozoites were grown in the presence of cellulose and cellulase either pre-incubated for 24 h in the culture medium at 27°C to allow cellulose digestion before adding the amoebae (Figure 6F), or directly supplemented to the cells without pre-incubation (Figure 6E), the doubling time was likewise shorten at 9 h in both conditions, in comparison to the untreated control where 14 h were necessary to double the cell population. This growth stimulation effect was observed at all cellulose concentrations used, from 1 g/L to 5 g/L, in the presence of 7.5 U/mL cellulase (Figures 6E,F). Interestingly, trophozoite doubling time was also shorten at 9 h when the culture medium was supplemented with 50 mM glucose, but their growth was negligible when additive glucose concentration was increased at 100 mM (Figure 6H). Furthermore, trophozoite growth was similar to the control in the presence of 1 g/L and 2.5 g/L cellulose alone, while a very little development was observed with 5 g/L cellulose (Figure 6G).
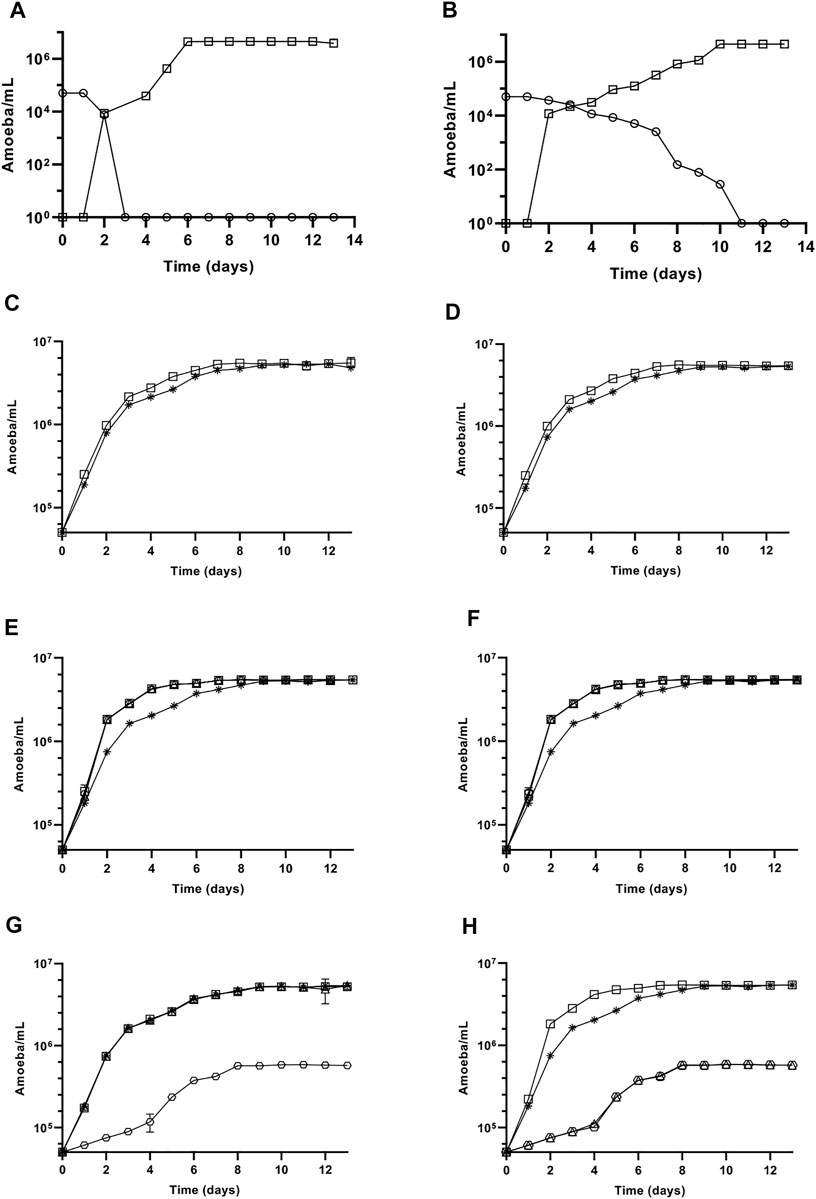
FIGURE 6. Influence of cellulase and cellulose on A. castellanii trophozoite growth at 27°C. (A,B) Cyst (○) excystment and trophozoite (□) growth kinetics in the presence (A) or absence (B) of 7.5 U/mL cellulase. (C,D) Growth kinetics of trophozoites in the presence (□) or absence (*) of 7.5 U/mL cellulase after a passage following their excystment in the presence (C) or absence (D) of 7.5 U/mL cellulase. (E,F) Growth kinetics of non-freshly excysted, regularly passed, trophozoites in PYG medium alone (*) or PYG medium supplemented with 7.5 U/mL cellulase and cellulose at 1 g/L (□), 2.5 g/L (△) or 5 g/L (○), either directly to the trophozoites (E), or pre-incubated 24 h at 27°C before adding the amoebae (F). (G,H) Growth kinetics of regularly passed trophozoites in PYG culture medium alone (*) or PYG medium supplemented with cellulose (G) at 1 g/L (□), 2.5 g/L (△), or 5 g/L (○), or with glucose (H) at 50 mM (□), 100 mM (△), or 150 mM (○). The results correspond to the mean of three independent experiments ±SD.
In order to analyze the distribution of glycopolymers in A. castellanii cyst walls following cellulase treatment in comparison to untreated control, a FITC-coupled lectin labeling was performed following treatment for 48 h at 27°C with 7.5 U/mL cellulase, where approximately 50% of trophozoites was observed (Figure 7). Nine different FITC-lectins previously described to have affinities to distinct monosaccharides were used in this study (Elloway et al., 2004; Figure 7). Among the nine lectins used, no loss or decrease of fluorescent signal intensity was observed after cellulase treatment. Moreover, the ectocyst of cellulase-treated A. castellanii cysts was similarly labeled in comparison to untreated control (Figure 7). However, while the endocyst was labeled in half of the cysts at best in the control, a tendancy to a decrease in the proportion of endocyst-labeled cysts was observed in cellulase-treated amoebae with all lectins (Supplementary Figure S3; Figure 7). Additionally, intracellular vesicles were revealed after cellulase treatment with GSL II, LCA and WGA and were labeled before and after treatment with SBA (Figure 7). Ostioles were also clearly labeled by Jacalin in untreated control, while they were not, or only partially, recognized by this lectin after treatment (Figure 7). In our conditions, trophozoites were not labeled by any of the lectin used, except for SWGA which revealed intracellular vesicles in this amoeba stage (Supplementary Figure S4). Furthermore, small dots were revealed in the intercystic space with WGA after cellulase treatment (Figure 7).
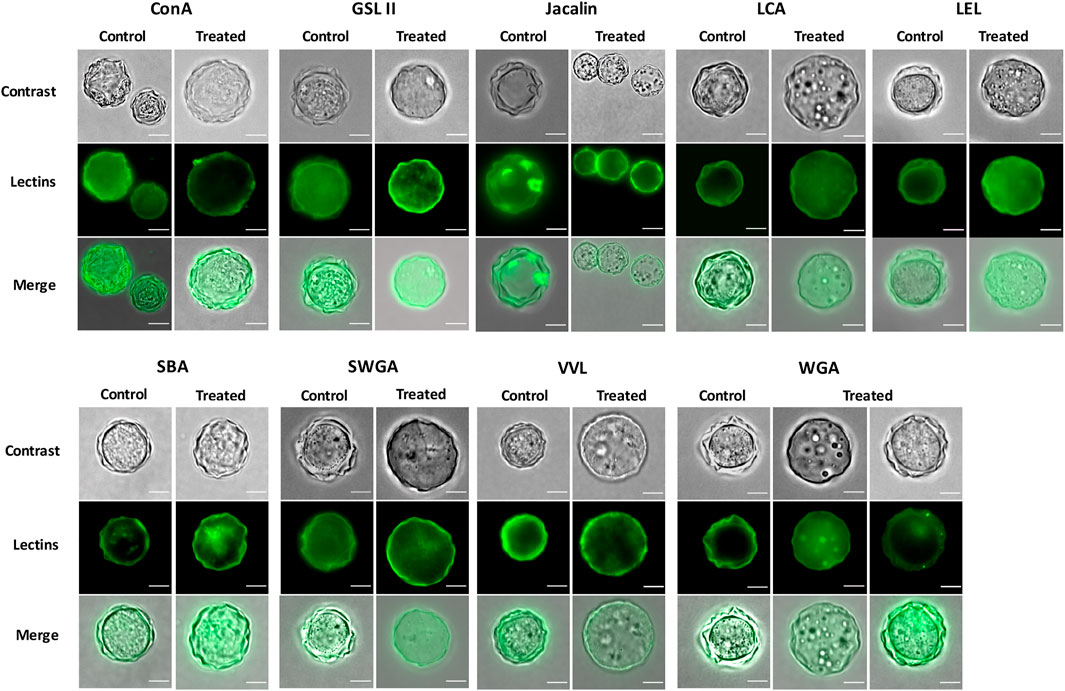
FIGURE 7. Influence of cellulase treatment on carbohydrate localization in A. castellanii cysts. After a treatment in the presence or absence (control) of 7.5 U/mL cellulase at 27°C for 48 h, carbohydrates were labeled by FITC-lectins (green). Phase contrast and merge images are shown on top and at the bottom of the FITC-lectin image, respectively. Nine lectins were used in this experiment: Con A, GSL II, Jacalin, LCA, LEL, SBA, SWGA, VVL and WGA. Scale bars = 10 µm.
At the ultrastructural level, the area of the space between the ectocyst and the endocyst, called the intercystic space, was significantly reduced in most of the cellulase-treated cysts, and was estimated to be twice smaller compared to untreated control (Figures 8A–D, Figures 8F–H). Moreover, the endocyst was either hardly visible (Figures 8C,D) or absent (Figures 8F,G) in the cellulase-treated cysts where the intercystic space area was reduced. Nevertheless, some cellulase-treated cysts presented a loose disorganized endocyst with the presence of fibrillar material within an intercystic space comparable in size to the one of the control (Figure 8E). Additionally, cellulase-treated cysts displayed electron-dense vesicles, probably lipidic vesicles, mostly at the periphery of the amoeba while they were distributed within the cytoplasm of untreated cells (Figures 8A,C,F). These vesicles were significantly smaller in cellulase-treated cysts in comparison to the control, with a reduction of approximately 25% of their area (Figure 8I). However, the number of lipidic vesicles per amoeba did not vary significantly between treated and untreated cysts (Figure 8J).
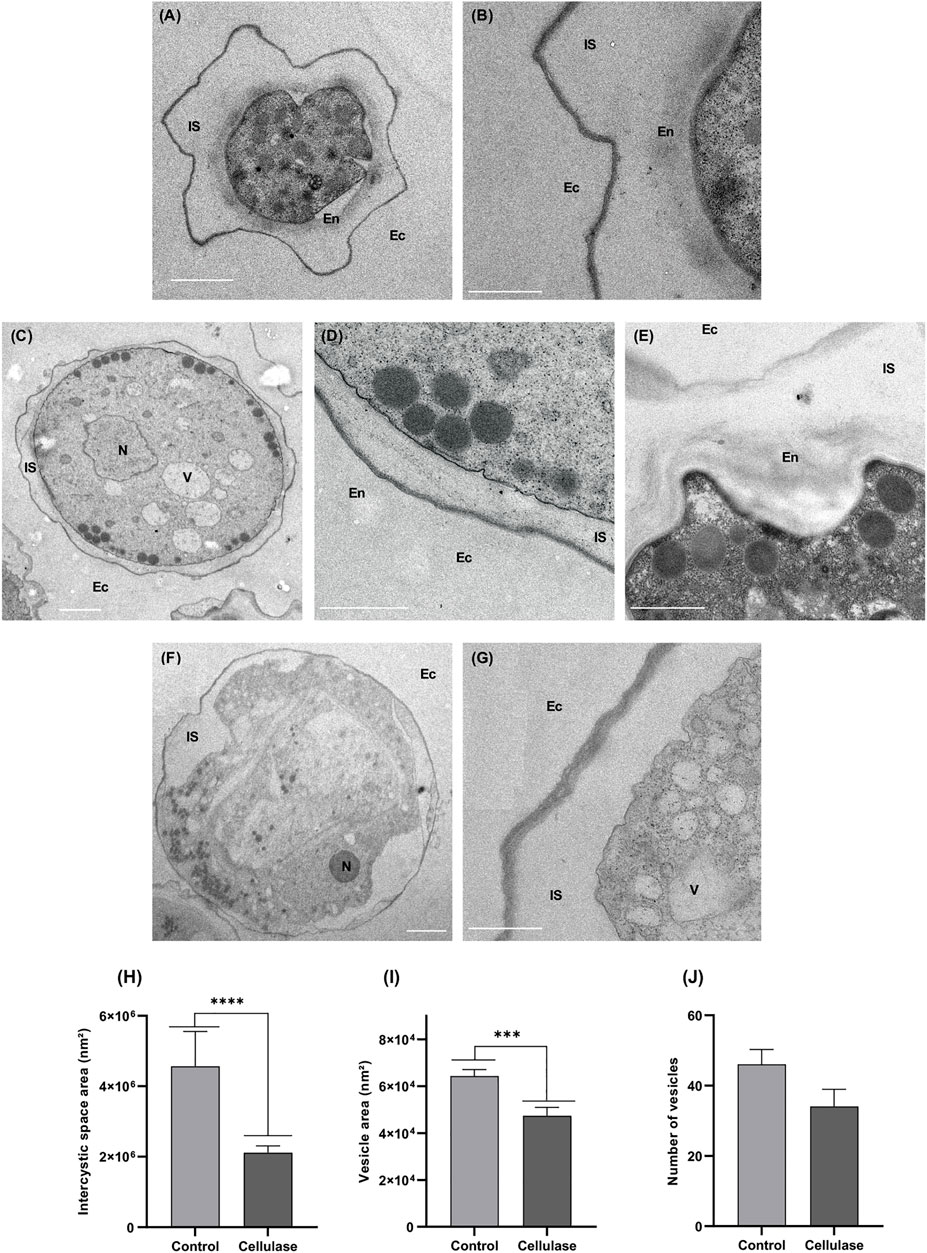
FIGURE 8. Analysis in transmission electron microscopy of A. castellanii cysts treated at 30°C for 48 h in the absence (A,B) or in the presence (C–G) of 5 U/mL cellulase. Treated cysts are characterized by a reduction of the intercystic space (C,D,F,G) in comparison to the control (A,B), with an endocyst either scarcely visualizable (C,D) or absent (F,G). Some treated cysts displayed a distance between endocyst and ectocyst comparable to the control, but with a disturbed endocyst and the presence of fibrillar material released within the intercystic space (E). In treated cysts, lipidic vesicles were mostly localized at the periphery of the amoeba (C,F), in opposition to the control (A). En, Endocyst; Ec, ectocyst; IS, Intercystic Space, N, Nucleus, V, Vacuole; White arrow: lipidic vesicle; Double black arrow: fibrillar material within the intercystic space. Scale bars represent 2 µm (A,F), and 1 µm (B,C,D,E,G). The intercystic space area (H) as well as the lipidic vesicle area (I) and number (J) were determined in cellulase-treated and untreated amoebae. The results correspond to the mean of 30 cells ±SEM. Three independent experiments were performed.
Discussion
As Acanthamoeba sp. cyst walls are mainly constituted of cellulose and proteins, we attempted in the present work to digest these components with cellulolytic or proteolytic enzymes with the aim to promote trophozoite excystment. Based on their sequence similarities, structural and catalytic properties, cellulases can be found in 11 Glycoside Hydrolase (GH) families while having similar substrate specificities (Lakhundi et al., 2015). Although the cellulase from Aspergillus niger used in the current study belongs to the glycoside hydrolase family 12, the cellulases of Acanthamoeba sp. have been identified to belong to glycoside hydrolase family 5 (Lakhundi et al., 2015). However, both glycoside hydrolase families 5 and 12 have been described to have similar structural and catalytic activities (Segato et al., 2014), showing that the cellulase of A. niger could mimic the activity of the counterpart encoded in A. castellanii. Moreover, based on their catalytic mechanism, proteases have been classified in 5 major different classes: cysteine, serine, threonine, aspartic and metallo-proteases (Lopez-Otin and Bond, 2008). While the two last classes use an activated water molecule as a nucleophile to attack protein peptide bond, in the other enzymes, the nucleophile is an amino acid directly located within the active site of the proteases, leading to a higher reactivity order and more efficient mechanism (Cuesta et al., 2020; Elsasser and Goettig, 2021). In Acanthamoeba sp., a subtilisin-like serine protease has been previously described to have a role in the excystment process (Dudley et al., 2008; Fouque et al., 2012). However, a treatment of V. vermiformis cysts by subtilisin-like protease with a concentration as low as 0.625 U/mL has been previously described to lead to high cysticidal activity (Fouque et al., 2015a). In order to avoid a cysticidal effect with protease treatment, we used in the current study lower efficient proteases belonging to the metallo- and aspartic protease class, namely collagenase and pepsin, respectively. Moreover, as both serine proteases and collagenases have been described to have a role in Acanthamoeba sp. pathogenicity (De Sousa-Carvalho et al., 2011), it was of interest to analyze also the influence of collagenase on A. castellanii excystment. In addition, pepsin was used in non-optimal conditions, at pH 6.5, which is distant from its optimal pH at 2 and where only a residual activity has been previously observed (Salelles et al., 2021), thus favoring a gentle digestion of the FLA cyst wall. Indeed, at pH 2, no excystment was observed in our conditions after an incubation of up to 18 days at 30°C in the presence of 100 U/mL pepsin, while the amoebae were able to excyst after a treatment in the same conditions at pH 2 without the enzyme, showing a cysticidal effect of pepsin at pH 2 on A. castellanii.
In order to avoid any toxic effect of enzyme treatment, cellulase was used with a range from 5 U/mL to 50 U/mL, much lower than the concentrations of 250 U/mL and 1500 U/mL previously described to cause less than 20% and less than 50% of cyst death, respectively (Lazuana et al., 2019), and proteases were used with a similar range, from 5 U/mL to 100 U/mL. As a result, in comparison to untreated A. castellanii cysts which need 11 days to completely excyst at 27°C, only 48 h were sufficient to give more than 96% of excystment at the same temperature in the presence of 50 U/mL of collagenase, 100 U/mL pepsin or 25 U/mL cellulase. These results show that, in these treatment conditions, cellulase, pepsin and collagenase can promote A. castellanii trophozoite release from cysts, with an acceleration of the excystment process of 8 days, corresponding to 73% of the excystment time, in comparison to untreated cysts. Moreover, at 30°C with 7.5 U/mL cellulase, a quasi-total excystment can be obtained after only 24 h, showing a temperature-dependent activity for this enzyme, which is less marked for collagenase and pepsin. The faster effect with smaller concentration of cellulase could be due to a higher specific activity of this enzyme in comparison to collagenase and pepsin in our conditions, and also to the high amount of cellulose present in Acanthamoeba sp. cyst wall (Weisman, 1976; Dudley et al., 2009). Moreover, the additive effect of the two by two associations of cellulase, collagenase and pepsin could be assigned to a complementary action of the enzymes on carbohydrates and proteins within the amoeba cyst wall leading to a faster excystment in comparison to cysts treated with individual enzymes: almost all amoebae have excysted after 48 h at 30°C with only 5 U/mL of each enzyme.
Remarkably, in the optimal conditions identified for A. castellanii, no effect of cellulase, collagenase or pepsin was observed on V. vermiformis or B. mandrillaris. This difference of activity could be ascribed to a distinct cell wall composition in these FLA. Indeed, the cyst wall of Hartmanella glebae, a FLA closely related to V. vermiformis, has been described to be composed of 64.7% proteins, and only 4.2% cellulose (Upadhyay et al., 1984). Moreover, the cyst wall of B. mandrillaris is presumably composed of a mixture of proteins and cellulose, but with a proportion that remains to be determined (Siddiqui et al., 2009a), although its carbohydrate composition has been identified with 20.9 mol% mannose and 79.1 mol% glucose (Siddiqui et al., 2009b). Because of differences of composition, the action of the enzymes could be prevented due to hindered sites of digestion, leading to an absence of activity on excystment. Conversely, some substrates could be easier to reach leading to an exacerbation of the enzyme effect, and thus to cyst lysis, as it was the case with cellulase on V. vermiformis in our study, indicating that cellulose has a crucial role in this FLA cyst wall integrity, even if it is present in minor proportion in this amoebal structure (Upadhyay et al., 1984).
Surprisingly, following their release from cysts, trophozoite growth was greatly stimulated in the presence of cellulase with a doubling time of 7 h, in comparison to untreated amoebae which doubled their population only every 23 h. However, no growth difference was observed on trophozoite growth after a passage in the presence or absence of cellulase following amoebae excystment, showing that cellulase did not have a direct effect on A. castellanii trophozoite proliferation. A hypothesis would be that cellulase added in the medium would help amoebae to digest their cyst wall to use it as a source of energy and stimulate trophozoite growth. When cellulase and cellulose were both added on trophozoites, either pre-incubated 24 h at 27°C prior to amoebae addition or directly added to the culture, doubling time was also markedly shortened to 9 h in comparison to untreated control, showing that the degradation product of cellulose can stimulate A. castellanii growth. Likewise, the addition of 50 mM glucose, the final product of cellulose degradation, to the culture medium stimulated A. castellanii growth with a doubling time of 9 h while no effect was observed with cellulose alone at 1 g/L and 2.5 g/L, and an inhibition of proliferation was obtained with higher concentrations of glucose, at 100 mM (equivalent to 18 g/L) and 250 mM (equivalent to 45 g/L), and cellulose at 5 g/L, showing a toxicity of these carbohydrates at these concentrations in the culture medium. Altogether, these results show that while cellulose or cellulase alone does not have any effect on amoeba growth, the product of cellulose degradation by cellulase promotes their proliferation. Therefore, following their excystment in the presence of cellulase, trophozoite growth is promoted by the degradation product of cellulose, mainly present in amoeba cyst wall, showing that the major carbohydrate resulting from cyst wall degradation after cellulase treatment, essentially glucose, can be used, at up to a supplemental concentration of 50 mM in the culture medium, as an energy source by trophozoites to promote their proliferation after excystment. Moreover, as empty cyst walls are no longer visible in culture following A. castellanii excystment in the absence of cellulase, it is conceivable that trophozoites would secrete their own cellulase during this cellular process allowing cyst wall degradation and amoebae proliferation, but with a much lower efficiency than in the presence of an additional cellulase. These data are in agreement with the studies previously describing the release of cellulase as well as proteases during Acanthamoeba culbertsoni excystment (Lasman 1975; Kaushal and Shukla, 1978a; Kaushal and Shukla, 1978b; Krishna-Murti and Shukla, 1984; Kaushal and Shukla, 1976). Moreover, as the monosaccharide composition of Acanthamoeba cyst wall is mainly constituted of glucose and galactose (Anwar et al., 2019), these carbohydrates could be released after cyst wall digestion during excystment and further used as an energy source or to synthesize glycoconjugates within the trophozoite. Likewise, in V. vermiformis, trophozoites have been described to digest its own cyst wall during excystment (Fouque et al., 2015b). Moreover, the difference of doubling times between trophozoites just after their release from cysts, at 23 h, and non-freshly excysted trophozoites, at 14 h, could be due to a difference of activity of their metabolism after excystment in comparison to regularly passed amoebae. Furthermore, the shorter doubling time of 14 h obtained in the present study with A. castellanii trophozoites in comparison to the one previously reported of 22 h (Taravaud et al., 2017), may be explained by presence of sodium citrate in the culture medium in the current work, which is recommended by ATCC and is included in diverse studies regarding Acanthamoeba sp. culture (Moon et al., 2018; Nakagawa et al., 2019; Li et al., 2020; Weber-Lima et al., 2020).
No effect was observed on A. castellanii excystment with chitinase in our study. However, only small chitinase concentrations, ranging from 0.1 to 2 U/mL, were possible to be used in our conditions, indicating a potential absence of significant activity in our study. The presence of chitin in Acanthamoeba cyst wall is currently controversial (Linder et al., 2002; Derda et al., 2009; Magistrado-Coxen et al., 2019). Nevertheless, several lectins used in the present study, i.e., WGA, SWGA and LEL, displayed a labeling of both ectocyst and endocyst layers in untreated cysts while they were previously described to have a better affinity for N-acetyl-β-D-glucosamine, the main component of chitin (Elloway et al., 2004). These results could be assigned to the ability of these lectins to bind to other carbohydrates in Acanthamoeba cyst wall (Immel et al., 2016), or to the presence of chitin in the cyst wall.
The FITC-lectin labeling revealed a tendancy to a decrease of endocyst recognition in cellulase-treated cysts, in comparison to untreated amoebae. This result was confirmed in electron microscopy where a disorganization of the endocyst was observed in cellulase-treated cysts. Moreover, the presence of fibrillar material in electron microscopy as well as WGA-labeled structures in epifluorescence microscopy within the intercystic space confirmed the endocyst breakdown following cellulase treatment. The deposition of fibrillar material within the intercystic space has also been observed during Acanthamoeba lugdunensis encystment (Garajova et al., 2019), indicating that the endocyst arrangement and disarrangement would originate from and lead to the deposition of fibrillar material within the intercystic space, respectively. Moreover, during encystment, both cellulose synthase and xylose isomerase have been reported to be involved in cyst wall formation (Aqeel et al., 2013; Bernard et al., 2022). In our study, besides the endocyst breakdown, a significant reduction of the intercystic space area was observed in the cellulase-treated cysts, probably due to a re-hydration of the amoeba upon excystment. Indeed, during A. castellanii encystment, a dehydration of the amoeba was previously described leading to a very dense cytoplasm in the cyst and a reduction of the cell volume (Bowers and Korn, 1969). In opposition to the dogma postulating that cysts are metabolically inactive, a recent study has reported that phosphate transport is more important in A. castellanii cysts than in trophozoites, and would be presumably used for anaerobic ATP synthesis and thus for energy metabolism in these resistant amoeba forms (Carvalho-Kelly et al., 2022), suggesting that amoebae in their cyst form can actively sense their environment to determine when the excystment process can be initiated. As some cellulase-treated cysts appeared with a disorganized endocyst, but with an intercystic space area comparable to the untreated control, it is probable that the endocyst would be disrupted prior to amoeba re-hydration and intercystic-space reduction during excystment. Following these cellular processes, the amoeba would emerge by a cytoplasmic bud through an ostiole in order to complete its excystment, as previously described (Chambers and Thompson, 1972; Chavez-Munguia et al., 2005). This A. castellanii excystment process is however different from V. vermiformis where no ostiole has been described in the cyst wall and which presumably relies on the digestion on its own cyst wall for trophozoite release (Fouque et al., 2015b).
Furthermore, some vesicules were revealed by GSLII, LCA and WGA labelings after cellulase treatment in epifluorescence microscopy, these three lectins presenting higher affinities for α/β-N-acetylgalactosamine, α-mannose and N-acetylglucosamine, respectively (Elloway et al., 2004). These carbohydrate-containing vesicles were however probably distinct from the electron dense vesicles, mainly composed of lipids, observed at the periphery of cellulase-treated cysts in electron microscopy. These peripheral lipidic vesicles were significantly smaller than the ones observed in the whole cytoplasm of the untreated control. Conversely, the volume of lipid vesicles, presumably storing energy for the amoeba, has been shown to increase during A. castellanii encystment (Bowers and Korn, 1969). Therefore, during the process of excystment promoted by cellulase treatment, lipid vesicles would localize at the periphery of the amoeba and their content would be partially discharged to be potentially used as a source of energy by the excysting trophozoite.
In conclusion, in the present work, we have determined optimal conditions of cellulase, collagenase and pepsin treatment to promote Acanthamoeba castellanii excystment, in terms of temperature, time of contact and enzyme concentration. To the best of our knowledge, this work has never been performed before. While these conditions could not be transposed to other FLA, an additional effect was observed by treating A. castellanii cysts with these enzymes associated two by two. Following excystment, trophozoite growth was also markedly stimulated in the presence of cellulase, presumably due to the digestion of the main carbohydrate component, namely cellulose, of Acanthamoeba sp. cyst wall. At the ultrastructural level, excystment stimulation by cellulase treatment was characterized by endocyst disarrangement and intercystic space area reduction, allowing the trophozoite to come out of the cyst. In future works, other treatment conditions have to be determined to stimulate excystment of other FLA. A more detailed study of these cyst differentiation pathways would then be necessary in order to identify therapeutic targets to further develop new anti-amoebal drugs.
Data availability statement
The raw data supporting the conclusions of this article will be made available by the authors, without undue reservation.
Author contributions
ZF-M performed the experiments, analyzed the data and wrote the initial manuscript; PL edited the manuscript; SP conceived the project and experiments, analyzed the data and wrote the article.
Funding
This work was funded by the Syndicat des Eaux d'Ile-De-France (grant N° 18HR2863) and has benefited from the facilities and expertise of platform Imagerie-Gif core facility supported by l’Agence Nationale de la Recherche (ANR-11-EQPX-0029/Morphoscope, ANR-10-INBS-04/FranceBioImaging, ANR-11-IDEX-0003-02/ Saclay Plant Sciences).
Acknowledgments
We thank Indira Dennemont, Marie-Nadine Hilarion and Sameh Obeid for their precious help and contribution.
Conflict of interest
The authors declare that the research was conducted in the absence of any commercial or financial relationships that could be construed as a potential conflict of interest.
Publisher’s note
All claims expressed in this article are solely those of the authors and do not necessarily represent those of their affiliated organizations, or those of the publisher, the editors and the reviewers. Any product that may be evaluated in this article, or claim that may be made by its manufacturer, is not guaranteed or endorsed by the publisher.
Supplementary material
The Supplementary Material for this article can be found online at: https://www.frontiersin.org/articles/10.3389/fcell.2022.982897/full#supplementary-material
References
Aitken, D., Hay, J., Kinnear, F. B., Kirkness, C. M., Lee, W. R., and Seal, D. V. (1996). Amebic keratitis in a wearer of disposable contact lenses due to a mixed Vahlkampfia and Hartmanella infection. Ophthalmology 103, 485–494. doi:10.1016/s0161-6420(96)30667-2
Anwar, A., Khan, N. A., and Siddiqui, R. (2019). Galactose as a novel target against Acanthamoeba cysts. PLoS Negl. Trop. Dis. 13, e0007385. doi:10.1371/journal.pntd.0007385
Aqeel, Y., Siddiqui, R., and Khan, N. A. (2013). Silencing of xylose isomerase and cellulose synthase by siRNA inhibits encystation in Acanthamoeba castellanii. Parasitol. Res. 112, 1221–1227. doi:10.1007/s00436-012-3254-6
Barrett, R. A., and Alexander, M. (1977). Resistance of cysts of amoebae to microbial decomposition. Appl. Environ. Microbiol. 33, 670–674. doi:10.1128/aem.33.3.670-674.1977
Bernard, C., Locard-Paulet, M., Noel, C., Duchateau, M., Giai-Gianetto, Q., Moumen, B., et al. (2022). A time-resolved multi-omics atlas of Acanthamoeba castellanii encystment. Nat. Commun. 13, 4104. doi:10.1038/s41467-022-31832-0
Bowers, B., and Korn, E. D. (1969). The fine structure of Acanthamoeba castellanii (Neff strain). II. Encystment. J. Cell Biol. 41, 786–805. doi:10.1083/jcb.41.3.786
Burki, F., Roger, A. J., Brown, M. W., and Simpson, A. G. B. (2020). The new tree of eukaryotes. Trends Ecol. Evol. 35, 43–55. doi:10.1016/j.tree.2019.08.008
Carvalho-Kelly, L. F., Dick, C. F., Rocco-Machado, N., Gomes-Vieira, A. L., Paes-Vieira, L., and Meyer-Fernandes, J. R. (2022). Anaerobic ATP synthesis pathways and inorganic phosphate transport and their possible roles in encystment in Acanthamoeba castellanii. Cell Biol. Int. 46, 1288–1298. doi:10.1002/cbin.11830
Centeno, M., Rivera, F., Cerva, L., Tsutsumi, V., Gallegos, E., Calderon, A., et al. (1996). Hartmannella vermiformis isolated from the cerebrospinal fluid of a young male patient with meningoencephalitis and bronchopneumonia. Arch. Med. Res. 27, 579–586.
Chambers, J. A., and Thompson, J. E. (1972). A scanning electron microscopic study of the excystment process of Acanthamoeba castellanii. Exp. Cell Res. 73, 415–421. doi:10.1016/0014-4827(72)90066-3
Chavez-Munguia, B., Omana-Molina, M., Gonzalez-Lazaro, M., Gonzalez-Robles, A., Bonilla, P., and Martinez-Palomo, A. (2005). Ultrastructural study of encystation and excystation in Acanthamoeba castellanii. J. Eukaryot. Microbiol. 52, 153–158. doi:10.1111/j.1550-7408.2005.04-3273.x
Cuesta, S. A., Mora, J. R., Zambrano, C. H., Torres, F. J., and Rincon, L. (2020). Comparative study of the nucleophilic attack step in the proteases catalytic activity: A theoretical study. Mol. Phys. 118, e1705412. doi:10.1080/00268976.2019.1705412
De Souza-Carvalho, F. R., Carrijo-Carvalho, L. C., Chudzinski-Tavassi, A. M., Foronda, A. S., and de Freitas, D. (2011). Serine-like proteolytic enzymes correlated with differential pathogenicity in patients with acute Acanthamoeba keratitis. Clin. Microbiol. Infect. 17, 603–609. doi:10.1111/j.1469-0691.2010.03252.x
Denet, E., Coupat-Goutaland, B., Nazaret, S., Pelandakis, M., and Favre-Bonte, S. (2017). Diversity of free-living amoebae in soils and their associated human opportunistic bacteria. Parasitol. Res. 116, 3151–3162. doi:10.1007/s00436-017-5632-6
Derda, M., Winieka-Krusnell, J., Linder, M. B., and Linder, E. (2009). Labeled Trichoderma reesei cellulase as a marker for Acanthamoeba cyst wall cellulose in infected tissues. Appl. Environ. Microbiol. 75, 6827–6830. doi:10.1128/AEM.01555-09
Dudley, R., Alsam, S., and Khan, N. A. (2008). The role of proteases in the differentiation of Acanthamoeba castellanii. FEMS Microbiol. Lett. 286, 9–15. doi:10.1111/j.1574-6968.2008.01249.x
Dudley, R., Jarroll, E. L., and Khan, N. A. (2009). Carbohydrate analysis of Acanthamoeba castellanii. Exp. Parasitol. 122, 338–343. doi:10.1016/j.exppara.2009.04.009
Elloway, E. A. G., Armstrong, R. A., Bird, R. A., Kelly, S. L., and Smith, S. N. (2004). Analysis of Acanthamoeba polyphaga surface carbohydrate exposure by FITC-lectin binding and fluorescence evaluation. J. Appl. Microbiol. 97, 1319–1325. doi:10.1111/j.1365-2672.2004.02430.x
Elsasser, B., and Goettig, P. (2021). Mechanisms of proteolytic enzymes and their inhibition in QM/MM studies. Int. J. Mol. Sci. 22, 3232. doi:10.3390/ijms22063232
Fouque, E., Hechard, Y., Hartemann, P., Humeau, P., and Trouilhe, M. C. (2015a). Sensitivity of Vermamoeba (Hartmanella) vermiformis cysts to conventional disinfectants and protease. J. Water Health 13, 302–310. doi:10.2166/wh.2014.154
Fouque, E., Trouilhe, M. C., Thomas, V., Hartemann, P., Rodier, M. H., and Hechard, Y. (2012). Cellular, biochemical, and molecular changes during encystment of free-living amoebae. Eukaryot. Cell 11, 382–387. doi:10.1128/EC.05301-11
Fouque, E., Yefimova, M., Trouilhe, M. C., Quellard, N., Fernandez, B., Rodier, M. H., et al. (2015b). Morphological study of the encystment and excystment of Vermamoeba vermiformis revealed original traits. J. Eukaryot. Microbiol. 62, 327–337. doi:10.1111/jeu.12185
Garajova, M., Mrva, M., Vaskovicova, N., Martinka, M., Melicherova, J., and Valigurova, A. (2019). Cellulose fibrils formation and organization of cytoskeleton during encystment are essential for Acanthamoeba cyst wall architecture. Sci. Rep. 9, 4466. doi:10.1038/s41598-019-41084-6
Immel, F., Broussard, C., Catherinet, B., Plasseraud, L., Alcaraz, G., Bundeleva, I., et al. (2016). The shell of the invasive bivalve species Dreissena polymorpha: Biochemical, elemental and textural investigations. PLoS One 11, e0154264. doi:10.1371/journal.pone.0154264
Joo, S. Y., Aung, J. M., Shin, M., Moon, E. K., Kong, H. H., Goo, Y. K., et al. (2020). The role of Acanthamoeba castellanii Sir2-like protein in the growth and encystation of Acanthamoeba. Parasit. Vectors 13, 368. doi:10.1186/s13071-020-04237-5
Kaushal, D. C., and Shukla, O. P. (1976). Release of certain extracellular enzymes during excystment of axenically produced cysts of Hartmanella culbertsoni. Indian J. Exp. Biol. 14, 498–499.
Kaushal, D. C., and Shukla, O. P. (1978b). Elaboration of proteases, cellulase & chitinase during excystment of Hartmanella culbertsoni. Indian J. Exp. Biol. 16, 1104–1106.
Kaushal, D. C., and Shukla, O. P. (1978a). Properties of an extracellular protease elaborated during excystment of Hartmanella culbertsoni. Indian J. Exp. Biol. 16, 1102–1104.
Khan, N. A. (2006). Acanthamoeba: Biology and increasing importance in human health. FEMS Microbiol. Rev. 30, 564–595. doi:10.1111/j.1574-6976.2006.00023.x
Krishna-Murti, C. R., and Shukla, O. P. (1984). Differentiation of pathogenic amoebae: Encystation and excystation of Acanthamoeba culbertsoni-a model. J. Biosci. 6, 475–489. doi:10.1007/BF02703900
Lakhundi, S., Siddiqui, R., and Khan, N. A. (2015). Cellulose degradation: A therapeutic strategy in the improved treatment of Acanthamoeba infections. Parasit. Vectors 8, 23. doi:10.1186/s13071-015-0642-7
Lasman, M. (1975). Glucosidase activity in Acanthamoeba (Mayorella) palestinensis. The effect of glucose and natural glucosides on α- and β-glucosidases. J. Protozool. 22, 435–437. doi:10.1111/j.1550-7408.1975.tb05201.x
Lazuana, T., Astuty, H., and Sari, I. P. (2019). Effect of cellulase enzyme treatment on cyst wall degradation of Acanthamoeba sp. J. Parasitol. Res. 2019, 8915314. doi:10.1155/2019/8915314
Li, P., Vassiliadis, P., Ong, S. Y., Bennett-Wood, V., Sugimoto, C., Yamagishi, J., et al. (2020). Legionella pneumophila infection rewires the Acanthamoeba castellanii transcriptome, highlighting a class of sirtuin genes. Front. Cell. Infect. Microbiol. 10, 428. doi:10.3389/fcimb.2020.00428
Linder, M., Winieka-Krusnell, J., and Linder, E. (2002). Use of recombinant cellulose-binding domains of Trichoderma reesei cellulase as selective immunocytochemical marker for cellulose in protozoa. Appl. Environ. Microbiol. 68, 2503–2508. doi:10.1128/aem.68.5.2503-2508.2002
Lopez-Otin, C., and Bond, J. S. (2008). Proteases: Multifunctional enzymes in life and disease. J. Biol. Chem. 283, 30433–30437. doi:10.1074/jbc.R800035200
Magistrado-Coxen, P., Aqeel, Y., Lopez, A., Haserick, J. R., Urbanowicz, B. R., Costello, C. E., et al. (2019). The most abundant cyst wall proteins of Acanthamoeba castellanii are lectins that bind cellulose and localize to distinct structures in developing and mature cyst walls. PLoS Negl. Trop. Dis. 13, e0007352. doi:10.1371/journal.pntd.0007352
Martinez, A. J., and Visvesvara, G. S. (1997). Free-living, amphizoic and opportunistic amebas. Brain Pathol. 7, 583–598. doi:10.1111/j.1750-3639.1997.tb01076.x
Moon, E. K., Choi, H. S., Kong, H. H., and Quan, F. S. (2018). Polyhexamethylene biguanide and chloroquine induce programmed cell death in Acanthamoeba castellanii. Exp. Parasitol. 191, 31–35. doi:10.1016/j.exppara.2018.06.002
Moon, E. K., Chung, D. I., Hong, Y. C., and Kong, H. H. (2011a). Atg3-mediated lipidation of Atg8 is involved in encystation of Acanthamoeba. Korean J. Parasitol. 49, 103–108. doi:10.3347/kjp.2011.49.2.103
Moon, E. K., Chung, D. I., Hong, Y. C., and Kong, H. H. (2009). Autophagy protein 8 mediating autophagosome in encysting Acanthamoeba. Mol. Biochem. Parasitol. 168, 43–48. doi:10.1016/j.molbiopara.2009.06.005
Moon, E. K., Chung, D. I., Hong, Y. C., and Kong, H. H. (2012a). Cysteine protease involving in autophagosomal degradation of mitochondria during encystation of Acanthamoeba. Mol. Biochem. Parasitol. 185, 121–126. doi:10.1016/j.molbiopara.2012.07.008
Moon, E. K., Chung, D. I., Hong, Y. C., and Kong, H. H. (2007). Differentially expressed genes of Acanthamoeba castellanii during encystation. Korean J. Parasitol. 45, 283–285. doi:10.3347/kjp.2007.45.4.283
Moon, E. K., Chung, D. I., Hong, Y. C., and Kong, H. H. (2011b). Expression levels of encystation mediating factors in fresh strain of Acanthamoeba castellanii cyst ESTs. Exp. Parasitol. 127, 811–816. doi:10.1016/j.exppara.2011.01.003
Moon, E. K., Chung, D. I., Hong, Y. C., and Kong, H. H. (2012b). Protein kinase C signaling molecules regulate encystation of Acanthamoeba. Exp. Parasitol. 132, 524–529. doi:10.1016/j.exppara.2012.07.008
Moon, E. K., Hong, Y. C., Chung, D. I., Goo, Y. K., and Kong, H. H. (2016). Identification of protein arginine methyltransferase 5 as a regulator for encystation of Acanthamoeba. Korean J. Parasitol. 54, 133–138. doi:10.3347/kjp.2016.54.2.133
Moon, E. K., Hong, Y. C., Chung, D. I., and Kong, H. H. (2013). Identification of Atg8 isoform in encysting Acanthamoeba. Korean J. Parasitol. 51, 497–502. doi:10.3347/kjp.2013.51.5.497
Moon, E. K., Hong, Y. C., Lee, H. A., Quan, F. S., and Kong, H. H. (2017). DNA methylation of gene expression in Acanthamoeba castellanii encystation. Korean J. Parasitol. 55, 115–120. doi:10.3347/kjp.2017.55.2.115
Moon, E. K., and Kong, H. H. (2012). Short-cut pathway to synthesize cellulose in encysting Acanthamoeba. Korean J. Parasitol. 50, 361–364. doi:10.3347/kjp.2012.50.4.361
Nakagawa, H., Koike, N., Ehara, T., Hattori, T., Narimatsu, A., Kumakura, S., et al. (2019). Corticosteroid eye drops instillation aggravates the development of Acanthamoeba keratitis in rabbit corneas inoculated with Acanthamoeba and bacteria. Sci. Rep. 9, 12821. doi:10.1038/s41598-019-49128-7
Neff, R. J., Ray, S. A., Benton, W. F., and Wilborn, M. (1964). Chapter 4 induction of synchronous encystment (differentiation) in Acanthamoeba sp. Methods Cell Biol. 1, 55–83. doi:10.1016/S0091-679X(08)62086-5
Ortilles, A., Belloc, J., Rubio, E., Fernandez, M. T., Benito, M., Cristobal, J. A., et al. (2017). In-vitro development of an effective treatment for Acanthamoeba keratitis. Int. J. Antimicrob. Agents 50, 325–333. doi:10.1016/j.ijantimicag.2017.03.033
Randag, A. C., van Rooij, J., van Goor, A. T., Ververk, S., Wisse, R. P. L., Saelens, I. E. Y., et al. (2019). The rising incidence of Acanthamoeba keratitis: A 7-year nationwide survey and clinical assessment of risk factors and functional outcomes. PLoS One 14, e0222092. doi:10.1371/journal.pone.0222092
Reynolds, E. S. (1963). The use of lead citrate at high pH as an electron-opaque stain in electron microscopy. J. Cell Biol. 17, 208–212. doi:10.1083/jcb.17.1.208
Rodriguez-Zaragoza, S. (1994). Ecology of free-living amoebae. Crit. Rev. Microbiol. 20, 225–241. doi:10.3109/10408419409114556
Rolland, S., Mengue, L., Noel, C., Crapart, S., Mercier, A., Aucher, W., et al. (2020). Encystment induces down-regulation of an acetyltransferase-like gene in Acanthamoeba castellanii. Pathogens 9, 321. doi:10.3390/pathogens9050321
Salelles, L., Floury, J., and Le Feunteun, S. (2021). Pepsin activity as a function of pH and digestion time on caseins and egg white proteins under static in vitro conditions. Food Funct. 12, 12468–12478. doi:10.1039/d1fo02453a
Schuster, F. L. (2002). Cultivation of pathogenic and opportunistic free-living amebas. Clin. Microbiol. Rev. 15, 342–354. doi:10.1128/CMR.15.3.342-354.2002
Schuster, F. L., and Visvesvara, G. S. (1996). Axenic growth and drug sensitivity studies of Balamuthia mandrillaris, an agent of amoebic meningoencephalitis in humans and other animals. J. Clin. Microbiol. 34, 385–388. doi:10.1128/jcm.34.2.385-388-1996
Scruggs, B. A., Quist, T. S., Zimmerman, M. B., Salinas, J. L., and Greiner, M. A. (2022). Risk factors, management, and outcomes of Acanthamoeba keratitis: A retrospective analysis of 110 cases. Am. J. Ophthalmol. Case Rep. 25, 101372. doi:10.1016/j.ajoc.2022.101372
Segato, F., Damasio, A. R. L., de Lucas, R. C., Squina, F. M., and Prade, R. A. (2014). Genomics review of holocellulose deconstruction by Aspergilli. Microbiol. Mol. Biol. Rev. 78, 588–613. doi:10.1128/MMBR.00019-14
Shi, Y., Queller, D. C., Tian, Y., Zhang, S., Yan, Q., He, Z., et al. (2021). The ecology and evolution of amoeba-bacterium interactions. Appl. Environ. Microbiol. 87, e01866–20. doi:10.1128/AEM.01866-20
Siddiqui, R., Jarroll, E. L., and Khan, N. A. (2009a). Balamuthia mandrillaris: Staining properties of cysts and trophozoites and the effect of 2, 6-dichlorobenzonitrile and calcofluor white on encystment. J. Eukaryot. Microbiol. 56, 136–141. doi:10.1111/j.1550-7408.2009.00402.x
Siddiqui, R., Khan, N. A., and Jarroll, E. L. (2009b). The cyst wall carbohydrate composition of Balamuthia mandrillaris. Parasitol. Res. 104, 1439–1443. doi:10.1007/s00436-009-1346-8
Song, S. M., Han, B. I., Moon, E. K., Lee, Y. R., Yu, H. S., Jha, B. K., et al. (2012). Autophagy protein 16-mediated autophagy is required for the encystation of Acanthamoeba castellanii. Mol. Biochem. Parasitol. 183, 158–165. doi:10.1016/j.molbiopara.2012.02.013
Taravaud, A., Ali, M., Lafosse, B., Nicolas, V., Feliers, C., Thibert, S., et al. (2018). Enrichment of free-living amoebae in biofilms developed at upper water levels in drinking water storage towers: An inter- and intra-seasonal study. Sci. Total Environ. 633, 157–166. doi:10.1016/j.scitotenv.2018.03.178
Taravaud, A., Fechtali-Moute, Z., Loiseau, P. M., and Pomel, S. (2021). Drugs used for the treatment of cerebral and disseminated infections caused by free-living amoebae. Clin. Transl. Sci. 14, 791–805. doi:10.1111/cts.12955
Taravaud, A., Loiseau, P. M., and Pomel, S. (2017). In vitro evaluation of antimicrobial agents on Acanthamoeba sp. and evidence of a natural resilience to amphotericin B. Int. J. Parasitol. Drugs Drug Resist. 7, 328–336. doi:10.1016/j.ijpddr.2017.09.002
Thomas, J. M., and Ashbolt, N. J. (2011). Do free-living amoebae in treated drinking water systems present an emerging health risk? Environ. Sci. Technol. 45, 860–869. doi:10.1021/es102876y
Upadhyay, J. M., Crow, S., and Cox, A. (1984). The cyst wall composition of Hartmanella glebae. Proc. Soc. Exp. Biol. Med. 175, 424–428. doi:10.3181/00379727-175-41815
Visvesvara, G. S., Moura, H., and Schuster, F. L. (2007). Pathogenic and opportunistic free-living amoebae: Acanthamoeba spp., Balamuthia mandrillaris, Naegleria fowleri, and Sappinia diploidea. FEMS Immunol. Med. Microbiol. 50, 1–26. doi:10.1111/j.1574-695X.2007.00232.x
Wang, N., Sun, H., Liu, D., Jiang, X., Zheng, M., Zhu, W., et al. (2021). Ac-HSP20 is associated with the infectivity and encystation of Acanthamoeba castellanii. Front. Microbiol. 11, 595080. doi:10.3389/fmicb.2020.595080
Weber-Lima, M. M., Prado-Costa, B., Becker-Finco, A., Costa, A. O., Billiald, P., Furst, C., et al. (2020). Acanthamoeba spp. monoclonal antibody against a CPA2 transporter: A promising molecular tool for acanthamoebiasis diagnosis and encystment study. Parasitology 147, 1678–1688. doi:10.1017/S0031182020001778
Keywords: Acanthamoeba, free-living amoeba, excystment, cellulase, protease, trophozoite growth
Citation: Fechtali-Moute Z, Loiseau PM and Pomel S (2022) Stimulation of Acanthamoeba castellanii excystment by enzyme treatment and consequences on trophozoite growth. Front. Cell Dev. Biol. 10:982897. doi: 10.3389/fcell.2022.982897
Received: 30 June 2022; Accepted: 17 August 2022;
Published: 12 September 2022.
Edited by:
Cecilia Giulivi, University of California, Davis, United StatesReviewed by:
Diego De Souza Gonçalves, Federal University of Rio de Janeiro, BrazilCatalina Dirney Alba Soto, University of Buenos Aires, Argentina
Copyright © 2022 Fechtali-Moute, Loiseau and Pomel. This is an open-access article distributed under the terms of the Creative Commons Attribution License (CC BY). The use, distribution or reproduction in other forums is permitted, provided the original author(s) and the copyright owner(s) are credited and that the original publication in this journal is cited, in accordance with accepted academic practice. No use, distribution or reproduction is permitted which does not comply with these terms.
*Correspondence: Sébastien Pomel, sebastien.pomel@universite-paris-saclay.fr