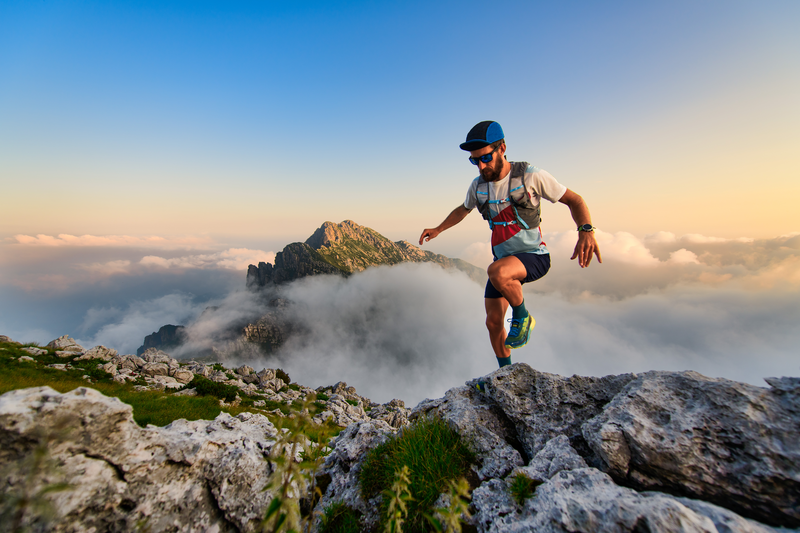
94% of researchers rate our articles as excellent or good
Learn more about the work of our research integrity team to safeguard the quality of each article we publish.
Find out more
EDITORIAL article
Front. Cell Dev. Biol. , 10 August 2022
Sec. Epigenomics and Epigenetics
Volume 10 - 2022 | https://doi.org/10.3389/fcell.2022.981075
This article is part of the Research Topic The Role of Interplay between Metabolism and Chromosomes in Tumorigenesis View all 14 articles
Editorial on the Research Topic
The role of interplay between metabolism and chromosomes intumorigenesis
Tumor is one of the biggest threats to human health. Genetic and epigenetic modifications in chromosome contribute to altered gene expression in tumor formation and development (Lee and Kim, 2022). In addition, altered metabolism such as activated glycolysis pathway is an essential feature of tumor to provide energy and structural resources for tumor cell proliferation (Altman et al., 2016). Previous studies have preliminarily confirmed the relationship between chromosome and metabolism, and the role of them in jointly promoting the development of tumor. For example, metabolites produced in tumor cells can affect enzyme activity of epigenetic modification to regulate gene expression (Miranda-Goncalves et al., 2018); some metabolites directly modify chromatin as substrates, resulting in chromatin structural abnormalities and modifications (Dai et al., 2020). In addition, genetic mutations associated with chromatin instability can lead to changes in enzyme activity resulting in the production of tumor-promoting metabolites (Kim and Yeom, 2018). Accumulation of harmful metabolites can trigger further mutations in chromosomes (Janke et al., 2015; Pavlova and Thompson, 2016; Pavlova et al., 2022). In our Research Topic, we further refined the relationship between chromosomes and metabolism from the following aspects, and clarified their cancer-promoting roles.
Non-coding RNA originates from chromosomes, and exerts cis-regulatory effects at the chromosomal level (Quinn and Chang, 2016). N6-methyladenosine (m6A) is the most abundant internal modification of RNA in eukaryotic cells, and has gained increasing attention in recent years. m6A can affect RNA function by affecting RNA processing, nuclear export and RNA translation to decay (Huang et al., 2020). In addition, m6A affects RNA stability and thus affect cell function. For example, in dendritic cell, CCR7 chemokine receptor upregulates long non-coding RNA Dpf3 via removing m6A modification to prevent RNA degradation. Dpf3 directly binds to and suppressed HIF-1α-dependent glycolysis (Liu et al., 2019). In our Research Topic, Liu et al. and Liang et al. reported that m6A modifications of long non-coding RNA DUXAP8 and LINC00106 both upregulate their stability, and then increase the migration, invasion, and metastasis characteristics of tumor cells and make tumor cells acquire chemotherapy-resistant properties. In addition, Liu et al. found that a glycolysis promoting gene SLC2A1 is closely associated with multiple m6A-related genes. These data suggested the close relationship between metabolism and pan-m6A modification system.
Competing endogenous RNAs (ceRNAs) are transcripts that can regulate each other at post-transcription level by competing for shared miRNAs (Qi et al., 2015). ceRNA network is an important way for non-coding RNAs to exert their functions (Tay et al., 2014). For example, HMGB1 mRNA has been found to promote the expression of RICTOR mRNA as the ceRNA through competitively binding with the miR-200 family, especially miR-429, then promotes glutamine metabolism and impedes immunotherapy by PD-L1+ exosomes activity (Wei et al., 2021). In our Research Topic, we also provide evidences that ceRNAs regulate tumor-related metabolism. The above mentioned long non-coding RNA DUXAP8, LINC00106 can both absorb microRNA to enhance the malignant phenotype of HCC cells. In addition, Lai et al. reported that LINC01572 and Wang et al. reported that LUCAT1 both act as ceRNAs to promote glycolysis in tumor cells. The above-mentioned glycolysis promoting gene SLC2A1 is also bio-informatic analyzed to be associated with ceRNA regulatory network. Li et al. reported that MTFR1 is a promoter of tumor progression and glycolysis by activating the AMPK/mTOR signalling pathway in LUAD. The MTFR1 negative regulator is miR-29c-3p, but the upstream ceRNA is not reported. We also published a review written by Xu et al. reported the role of non-coding RNA in cerebrospinal fluid. This review elucidates the brain tumorigenic mechanisms of non-coding RNAs in cerebrospinal fluid (mainly through m6A modification and ceRNA regulation), and concludes the research results of various non-coding RNAs as diagnostic, prognostic markers and therapeutic agents.
Altogether, the above articles describe that the m6A modification of non-coding RNAs to upregulate its stability, or through the downstream ceRNA network to activate glycolytic metabolism to play a tumor-promoting role.
Epigenetic regulated proteins such as histone modification readers and writers have many inhibitors/stimulators that regulate tumor metabolism by affecting epigenetics (Wimalasena et al., 2020). In our Research Topic, Qi et al. reported that Paris Saponin II inhibits human head and neck squamous cell carcinoma cell proliferation and metastasis by inhibiting the expression NOS3 and the nitric oxide metabolic pathway.
There are numerous examples of post-translational modifications regulating metabolism, such as protein phosphorylation, O-Glycosylation, methylation and crotonylation. A recent study reported that UCP1 is an important thermogenic protein in brown adipose tissue, and there are two key succinylation sites on UCP1 that are critical for UCP1 stability and activity. Loss of UCP1 succinylation results in impaired mitochondria respiration, defective mitophagy, and metabolic inflexibility (Wang et al., 2019). In our Research Topic, Wang et al. reported that ataxia-telangiectasia mutated deficiency firstly augments the phosphorylation of Mad1 Ser214, and promotes its heterodimerization with Mad2. Subsequently, Mad2 is phosphorylated at Ser195. This Mad2 phosphorylation decreases DNA damage repair capacity and is related to the radiotherapy resistance.
Glycolysis also has important effects on epigenetics. For example, a large amount of lactic acid is produced through glycolysis, and then protein lactylation occurs (Zhang et al., 2019). H3K18 lactylation elevates the expression of METTL3, which in turn leads to the activation of JAK/STAT signaling pathway, and further promotes cancer (Xiong et al., 2022). In our Research Topic, Shen et al. reported that in the hypoxic HCC microenvironment induced by glycolysis, ubiquitin E3 ligase ring finger protein RNF146 is transcriptionally activated by HIF-1α and HIF-2α. RNF146 promotes PTEN ubiquitination and degradation and stimulates AKT/mTOR pathway, thereby promotes HCC cell proliferation.
Markers simultaneously associated with epigenetics and metabolism are helpful for the treatment and evaluating prognosis of tumors. Existing markers include TET2, IDH1/2, DNMT3A recurrent mutations and so on (Nebbioso et al., 2018). Via metabolomic and transcriptomic analysis, Shan et al. found that 5-hydroxylysine and 1-methylnicotinamide are most likely to be keloid severity indicators.
In our Research Topic, a review written by Huo et al. details the relationship between epigenetic modification and metabolism in tumors. Firstly, epigenetic modifications such as deoxyribonucleic acid and ribonucleic acid methylation, non-coding ribonucleic acids and histone modifications regulate the metabolic remodeling of tumors, including glucose and lipid metabolism. In the contrary, intermediates produced by metabolism often participate in epigenetic regulation by serving as substrates or cofactors for epigenome-modifying enzymes. Therefore, the authors suggested that the metabolism-epigenome axis must be considered while approaching cancer biomarker studies.
Collectively, our Research Topic elaborated the influence of chromosomal substances such as non-coding RNA and its m6A modification, as well as post-translational modification of proteins on tumor glycolysis and other metabolism, and analyzed the influence of glycolysis on ubiquitination modification. Some epigenetic modified-related metabolic markers were found. Therefore, the close relationship between chromosome and metabolism is very important for the occurrence and development of tumors. In future research and clinical treatment, we need to explore the relationship between chromosome and metabolism in more detail.
LD, YL, and JW read the relevant literature and wrote the editorial. YL modified the grammar.
The authors declare that the research was conducted in the absence of any commercial or financial relationships that could be construed as a potential conflict of interest.
All claims expressed in this article are solely those of the authors and do not necessarily represent those of their affiliated organizations, or those of the publisher, the editors and the reviewers. Any product that may be evaluated in this article, or claim that may be made by its manufacturer, is not guaranteed or endorsed by the publisher.
Altman, B. J., Stine, Z. E., and Dang, C. V. (2016). From krebs to clinic: Glutamine metabolism to cancer therapy. Nat. Rev. Cancer 16, 749. doi:10.1038/nrc.2016.114
Dai, Z., Ramesh, V., and Locasale, J. W. (2020). The evolving metabolic landscape of chromatin biology and epigenetics. Nat. Rev. Genet. 21, 737–753. doi:10.1038/s41576-020-0270-8
Huang, H., Weng, H., and Chen, J. (2020). m(6 A modification in coding and non-coding RNAs: Roles and therapeutic implications in cancer. Cancer Cell 37, 270–288. doi:10.1016/j.ccell.2020.02.004
Janke, R., Dodson, A. E., and Rine, J. (2015). Metabolism and epigenetics. Annu. Rev. Cell Dev. Biol. 31, 473–496. doi:10.1146/annurev-cellbio-100814-125544
Kim, J. A., and Yeom, Y. I. (2018). Metabolic signaling to epigenetic alterations in cancer. Biomol. Ther. 26, 69–80. doi:10.4062/biomolther.2017.185
Lee, J. E., and Kim, M. Y. (2022). Cancer epigenetics: Past, present and future. Semin. Cancer Biol. 83, 4–14. doi:10.1016/j.semcancer.2021.03.025
Liu, J., Zhang, X., Chen, K., Cheng, Y., Liu, S., Xia, M., et al. (2019). CCR7 chemokine receptor-inducible lnc-dpf3 restrains dendritic cell migration by inhibiting HIF-1α-Mediated glycolysis. Immunity 50, 600–615. doi:10.1016/j.immuni.2019.01.021
Miranda-Goncalves, V., Lameirinhas, A., Henrique, R., and Jeronimo, C. (2018). Metabolism and epigenetic Interplay in cancer: Regulation and putative therapeutic targets. Front. Genet. 9, 427. doi:10.3389/fgene.2018.00427
Nebbioso, A., Tambaro, F. P., Dell'Aversana, C., and Altucci, L. (2018). Cancer epigenetics: Moving forward. PLoS Genet. 14, e1007362. doi:10.1371/journal.pgen.1007362
Pavlova, N. N., and Thompson, C. B. (2016). The emerging hallmarks of cancer metabolism. Cell Metab. 23, 27–47. doi:10.1016/j.cmet.2015.12.006
Pavlova, N. N., Zhu, J., and Thompson, C. B. (2022). The hallmarks of cancer metabolism: Still emerging. Cell Metab. 34, 355–377. doi:10.1016/j.cmet.2022.01.007
Qi, X., Zhang, D. H., Wu, N., Xiao, J. H., Wang, X., Ma, W., et al. (2015). ceRNA in cancer: possible functions and clinical implications. J. Med. Genet. 52, 710–718. doi:10.1136/jmedgenet-2015-103334
Quinn, J. J., and Chang, H. Y. (2016). Unique features of long non-coding RNA biogenesis and function. Nat. Rev. Genet. 17, 47–62. doi:10.1038/nrg.2015.10
Tay, Y., Rinn, J., and Pandolfi, P. P. (2014). The multilayered complexity of ceRNA crosstalk and competition. Nature 505, 344–352. doi:10.1038/nature12986
Wang, G., Meyer, J. G., Cai, W., Softic, S., Li, M. E., Verdin, E., et al. (2019). Regulation of UCP1 and mitochondrial metabolism in Brown adipose tissue by reversible succinylation. Mol. Cell 74, 844–857. doi:10.1016/j.molcel.2019.03.021
Wei, Y., Tang, X., Ren, Y., Yang, Y., Song, F., Fu, J., et al. (2021). An RNA-RNA crosstalk network involving HMGB1 and RICTOR facilitates hepatocellular carcinoma tumorigenesis by promoting glutamine metabolism and impedes immunotherapy by PD-L1+ exosomes activity. Signal Transduct. Target. Ther. 6, 421. doi:10.1038/s41392-021-00801-2
Wimalasena, V. K., Wang, T., Sigua, L. H., Durbin, A. D., and Qi, J. (2020). Using chemical epigenetics to target cancer. Mol. Cell 78, 1086–1095. doi:10.1016/j.molcel.2020.04.023
Xiong, J., He, J., Zhu, J., Pan, J., Liao, W., Ye, H., et al. (2022). Lactylation-driven METTL3-mediated RNA m(6)A modification promotes immunosuppression of tumor-infiltrating myeloid cells. Mol. Cell 82, 1660–1677 e10. doi:10.1016/j.molcel.2022.02.033
Keywords: non-coding RNA, post-translational modification, epigenetic regulation, glycolysis, m6A modification, cancer, ceRNA
Citation: Du L, Lu Y and Wang J (2022) Editorial: The role of interplay between metabolism and chromosomes in tumorigenesis. Front. Cell Dev. Biol. 10:981075. doi: 10.3389/fcell.2022.981075
Received: 29 June 2022; Accepted: 07 July 2022;
Published: 10 August 2022.
Edited and reviewed by:
Michael E. Symonds, University of Nottingham, United KingdomCopyright © 2022 Du, Lu and Wang. This is an open-access article distributed under the terms of the Creative Commons Attribution License (CC BY). The use, distribution or reproduction in other forums is permitted, provided the original author(s) and the copyright owner(s) are credited and that the original publication in this journal is cited, in accordance with accepted academic practice. No use, distribution or reproduction is permitted which does not comply with these terms.
*Correspondence: Lutao Du, bHV0YW9kdUBzZHUuZWR1LmNu; Yuanyuan Lu, bHV5dWFuMTFAZm1tdS5lZHUuY24=; Jiayi Wang, a2FyYWphbjJAMTYzLmNvbQ==
Disclaimer: All claims expressed in this article are solely those of the authors and do not necessarily represent those of their affiliated organizations, or those of the publisher, the editors and the reviewers. Any product that may be evaluated in this article or claim that may be made by its manufacturer is not guaranteed or endorsed by the publisher.
Research integrity at Frontiers
Learn more about the work of our research integrity team to safeguard the quality of each article we publish.