- 1Immunopatologia e Biomarcatori Oncologici, Dipartimento di Ricerca e Diagnostica Avanzata dei Tumori, CRO Aviano, National Cancer Institute, Istituto di Ricovero e Cura a Carattere Scientifico, IRCCS, Aviano, Italy
- 2Pediatric Hemato-Oncology Unit, Department of Women’s and Children’s Health, University of Padua, Padua, Italy
- 3AYA Oncology and Pediatric Radiotherapy Unit, Centro di Riferimento Oncologico IRCCS, Aviano, Italy
- 4Nuclear Medicine, IRCCS—Humanitas Research Hospital, Rozzano, MI, Italy
- 5Department of Pathology, San Bortolo Hospital, Vicenza, Italy
- 6Pediatric Hematology-Oncology Unit, Azienda Ospedaliera Universitaria, Ospedale Sant’Anna, Ferrara, Italy
Currently-available therapies for newly-diagnosed pediatric and adolescent patients with Hodgkin lymphoma result in >95% survival at 5 years. Long-term survivors may suffer from long-term treatment-related side effects, however, so the past 20 years have seen clinical trials for children and adolescents with HL gradually abandon the regimens used in adults in an effort to improve this situation. Narrower-field radiotherapy can reduce long-term toxicity while maintaining good tumor control. Various risk-adapted chemo-radiotherapy strategies have been used. Early assessment of tumor response with interim positron emission tomography and/or measuring metabolic tumor volume has been used both to limit RT in patients with favorable characteristics and to adopt more aggressive therapies in patients with a poor response. Most classical Hodgkin’s lymphoma relapses occur within 3 years of initial treatment, while relapses occurring 5 years or more after diagnosis are rare. As the outcome for patients with relapsed/refractory classical Hodgkin lymphoma remains unsatisfactory, new drugs have been proposed for its prevention or treatment. This review summarizes the important advances made in recent years in the management of pediatric and adolescent with classical Hodgkin lymphoma, and the novel targeted treatments for relapsed and refractory classical Hodgkin lymphoma.
Introduction: A model of Hodgkin lymphoma biology and biomarkers
Classical Hodgkin lymphoma (cHL) accounts for approximately 6%–7% of all pediatric cancers, with a peak incidence in adolescence and young adulthood. Reported incidence rates are: 29 myr in 15- to 19-year-olds; approximately 10/myr in 10- to 14-year-olds; 3.5/myr in children 5–9 years old; and 1/myr in infants up to 4 years old. Among Italian adolescents aged 15–19 years the incidence rate is 23.6/myr, which is twice the rate reported for the same age group in the United States and the rest of Europe (AIRTUM Working Group and CCM, 2013). HL is also associated with congenital immunodeficiency: it is estimated that 4.5% of HL cases are familial (McAulay and Jarrett, 2015).
There are four subtypes of cHL: nodular sclerosis (NSHL); mixed cell (MCHL); lymphocyte-rich (LRHL); and lymphocyte-depleted (LDHL) (Connors et al., 2020). NSHL is the most common of these subtypes. Patients with nodular lymphocyte-predominant HL (NLPHL) are classified as cases of non-cHL. Epstein-Barr virus (EBV) plays a role in the etiology of HL in approximately 30% of cases. The frequency of EBV positivity ranges from ∼75% in MCHL and LDHL to <20% in NSHL and LRHL (Massini et al., 2009).
Malignant cells are large and multinucleated, and derive from B lymphocytes. They are known as Hodgkin and Reed-Sternberg (HRS) cells. Figure 1 illustrates the model that the HRS cells originate from the postgerminal center. HRS cells are typically CD30+ and embedded in a tumor-promoting microenvironment (TME) rich in immune cells, where exhausted T cells, Th1 and Th2 T-helper (Th) cells, polarized regulatory T (Treg) cells, PD1+ T follicular helper (TFH) cells, lymphocyte-activating 3 (LAG3, CD223)-positive T cells, and various subpopulations of macrophages play a key role in tumor support (for more details, see the next section). Patients with NLPHL have scattered, large neoplastic B cells with multilobate nuclei (lymphocyte-predominant or popcorn cells) expressing a broad panel of B-related markers (CD19, CD20, CD79a, PAX5, OCT2, BOB1) within nodules dominated by mantle zone B cells and follicular dendritic cells (FDCs) (Connors et al., 2020).
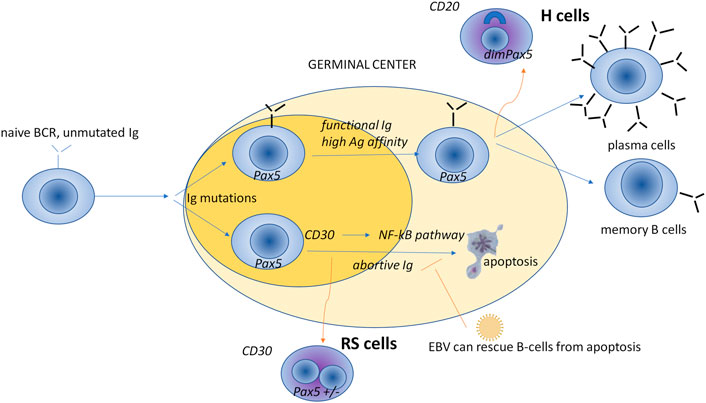
FIGURE 1. A model for the cellular germinal center origin of HRS cells. The origin of HRS cells has long been a matter of debate. Since the detection of clonal rearrangements of the immunoglobulin V gene in isolated HRS cells, it is now accepted that clonal B lymphocytes originate from HRS cells, with only a small proportion deriving from T lymphocytes. That said, HRS cells lose B-cell-specific genes (e.g., immunoglobulin genes and genes involved in the antigen presentation by MHC) and show characteristic positivity for nuclear paired box protein 5 (PAX5) (Khan et al., 2018) and CD30 antigen (Swerdlow et al., 2016; Weniger et al., 2018). PAX5 encodes a B-cell specific activator protein, a transcription factor expressed in the early but not in the late stages of B-cell differentiation. B-cell activation leads to the expression of the membrane receptor CD30, which regulates the apoptotic NF-kB pathway, and thus controls the load of the B-cell population (Küppers, 2009), B-cell receptor; dim, moderate expression; Hodgkin cells (H cells) of nodular lymphocyte-predominant HL; Ig, immunoglobulins; Reed-Sternberg (RS) cells of cHL.
HL is diagnosed on histological examination of an excisional biopsy of a suspect lymph node. Though not specific for HRS cells when considered separately, the relevant immunohistochemical markers include the expression of CD15+ (Lewis X antigen), CD30+ (TNFRSF8), PAX5+ (B-cell transcription factor) with a typical loss of B-cell antigens (e.g., CD19, CD20, CD79a), surface membrane immunoglobulin (Ig), and some B-cell transcription factors (Oct2 and Bob1). In contrast, tumor cells in NLPHL retain a complete B-cell program (CD19+, CD20+, and all transcription factors), but are CD30- and CD15-negative. In cases of cHL associated with EBV infection, tumor cells express a type II latency pattern associated with EBV-encoded RNA (EBER), latent membrane proteins (LMP) 1 and 2a, EBV nuclear antigen 1 (EBNA 1), and BamH1-A right frame 1 (BARF1) (De Re et al., 2020).
The overexpression of novel markers in cHL has been investigated both for diagnostic purposes and for novel targeted therapies (for more details, see the following sections). These include: integrin-associated protein (IAP) CD47 (Lopez-Pereira et al., 2020); transcription factor GATA3 activated by the NF-kB pathway; signal transducer and activator of transcription (STAT) 6; cluster of differentiation (CD) 83; transcription factor FOXO3A; Wilms tumor gene-1 (WT1); preferentially expressed antigen in melanoma (PRAME); and survivin (Dave et al., 2022).
Extracellular vesicles (EVs) formed inside endosomal compartments (i.e., exosomes), and carrying RNA, DNA, and proteins, are actively released from HRS cells and function as intercellular messengers. In HL, serum EV levels increase considerably. The EVs are rich in CD30 antigen (Hansen et al., 2014), also known as tumor necrosis factor receptor 8 (TNFR8), and exhibit functional protumoral effects. Hansen et al. (2014) showed that CD30-rich EVs stimulate the secretion of the tumorigenic chemokine interleukin-8 (IL-8) by eosinophil-like cells and primary granulocytes. CD30-rich EVs also facilitate the crosstalk between HRS cells and immune cells in the microenvironment, thus playing a part in the recruitment of distant immune cells with a key role in tumor growth. A recently-developed diagnostic analytic technique involves an aurum nanoparticle (AuNPs) with peroxidase activity capable of binding and ascertaining the concentration of CD30 antigen in circulating EVs for the purpose of monitoring cHL activity (Slyusarenko et al., 2022).
EVs released by HL cells also carry high levels of the metallopeptidase ADAM10 (Tosetti et al., 2018). Using the ADAM10 inhibitors LT4 and CAM29 to counteract ADAM10 shedding from HRS cells was found to restore the expression on the HRS cell surface of the major histocompatibility complex (MHC) Class I chain-related protein A (MICA) (Tosetti et al., 2018), a ligand for the activating receptor NKG2D present on NK and cytotoxic CD8+ T cells; and this resulted in the activation of cytotoxic T cells. ADAM10 also targets CD30, leading to the release of soluble CD30, which could interfere with anti-CD30 treatments.
EVs were also found involved in the crosstalk between the HRS and fibroblasts, leading to the production of highly-activated cancer fibroblasts that contribute to the tumor-promoting microenvironment (TME) (Dörsam et al., 2018). Plasma EVs encode proteins such as fibrinogen γ chain, complement C4B (an isotype of C4 protein), and transthyretin, which are more abundant in pediatric relapsed HL (Repetto et al., 2021).
Many miRNAs, such as miR21-5p, miR127-3p, let7a-5p, miR24-3p, and miR155-5p, are strongly enriched in the EVs of patients with cHL. In addition, miR-155-5p is related to disease progression, and miR155 responds to chemotherapy (van Eijndhoven et al., 2016). In an in vivo model of EBV-positive cHL, HRS cells were shown to release the EBV-encoded miRNA BamHI fragment A rightward transcript (BART), which induces the expression of immunosuppressive IL-10, TNF-α, and arginase-1 in macrophages, further supporting the development of HL (Higuchi et al., 2018). HRS cells may also transfer cell membrane fragments—including important proteins such as PD-L1, CD38, CD137, and CD30—to non-cancerous cells, a phenomenon known as trogocytosis and indicated as another mechanism contributing to the establishment of a TME (Zeng and Schwarz, 2020).
Genetic alterations in Hodgkin lymphoma
Patients have genetic alterations in their HRS cells (Table 1) that may also be present in their circulating mononuclear B cells, possibly due to telomere dysfunction (Buedts et al., 2021; Oki et al., 2015; M’kacher et al., 2018). The genetic basis for immune evasion and the anti-apoptotic signaling mediated by NF-κB and PI3K/AKT/mTOR pathways play a key role in cHL survival, while JAK/STAT signaling is known to sustain tumor growth. A reduced MHC Class I and/or Class II antigen expression and a reduced antigenic peptide/MHC complex presentation (Figure 2), which often correlates with worse prognosis, is reportedly common in HL, affecting from 40% to 70% of cases (Diepstra et al., 2007; Reichel et al., 2015).
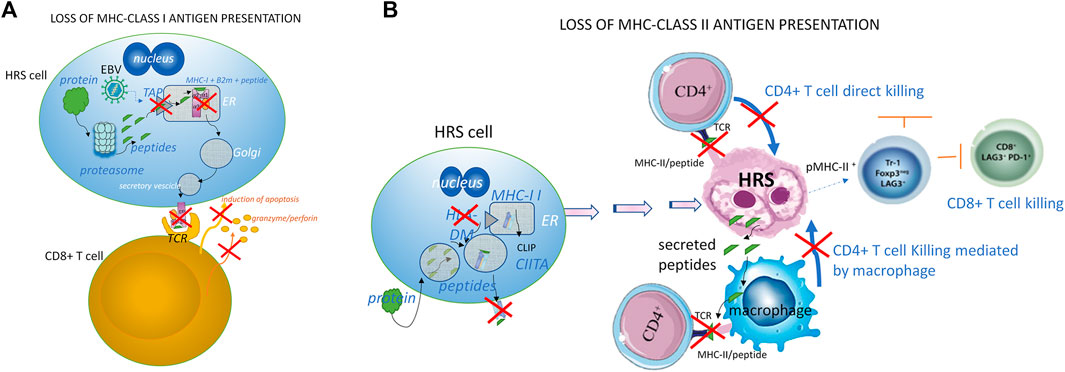
FIGURE 2. Loss of MHC class I (A) and class II (B) expression and MHC antigen presentation (A) A CD8+ cytotoxic T cell targets a cell expressing MHC-I/antigen complex after intracellular proteins have been digested by the proteasome into small peptides in Hodgkin and Reed-Sternberg cells, but the surface expression of the MHC-I/peptide complex is reduced due to transporters of peptides like processing (TAP) carriers and the beta 2 microglobulin (β2m) chain. Both the induction of apoptosis and the release of granzyme/perforin by CD8-T cells are consequently inhibited. In normal conditions, the peptides translocate into the endoplasmic reticulum (ER), allowing the antigen to bind to MHC-I. Then, after a passage in the Golgi structure and packaging in secretory vesicles, the MHC-I/peptide complex is fused with the plasma membrane and exposed to the surface of the target cell. The CD8+ T cell that recognizes and binds the MHC-I/peptide complex through its specific T-cell receptor (TCR) can induce the death of the target cell by activating the classical apoptotic signaling (Fas/caspase) pathway together with the release of the cytotoxic granzyme B and perforin from T-cell granules. In the case of tumor cells infected by EBV, the EBV BNLF2a, BGLF5, and BILF1 proteins produced during viral replication reduce the expression of MHC-I -antigen complexes, and thus contribute to the CD8+ T cell immune escape of the infected cells. (B) Direct and indirect killing of target cells by CD4+ T cells. MHC-II alpha and beta chains are normally assembled in the endoplasmic reticulum (ER). MHC-II binds the human leukocyte antigen DM (HLA-DM) to protect itself from endosomal degradation. HLA-DM releases the class II-associated invariant chain peptide (CLIP), replacing it with a sequence-specific peptide. The complex MHC-II/peptide (pMHC-II) goes to the cell surface, where MHC-II can present the peptide to the CD4+ T-cells. After the recognition of pMHC-II through its T-cell receptor (TCR), the CD4+ T-cell may kill the target cell directly by contact, or indirectly through the mediation of macrophages. Most HRS cells lack the HLA-DM, so the MHC-II is unprotected or unable to exchange the CLIP molecule with the antigenic peptide, and this leads to the absence of pMHC-II expression and a reduced tumor cell killing by CD4+ T-cells. The absence of pMHC-II expression is known to occur in about 40% of patients with cHL, and it is associated with poor treatment outcomes (Roemer et al., 2018). In other situations, HRS cells use pMHC-II to engage the immune suppressor lymphocyte-activation gene-3 (LAG3) receptor present on T cells, NK cells, plasmacytoid dendritic cells, and macrophages (Maruhashi et al., 2018; Triebel et al., 1990) in order to avoid immune recognition and exhaust cytotoxic T and NK cells. LAG-3 cooperates closely with PD-1 to alter regulator T cell homeostasis in cHL (Michot et al., 2021). Interestingly, CD4+ LAG3+ T cells have been found close to pMHC+ HRS cells, indicating that they play an important role in the development of the characteristic permissive microenvironment in cHL.
Clinical studies for the treatment of young patients with Hodgkin lymphoma
The standard of care for adults with relapsed cHL involves high-dose chemotherapy combined with autologous stem cell transplantation after conventional chemotherapy. At the end of 2019, the EuroNet Group published guidelines for pediatric and adolescent patients with recurrent or refractory cHL based on risk stratification, response-adapted therapy, and a limited use of transplantation (Daw et al., 2020). It recommended initially stratifying patients in one of two risk groups (low- and standard-risk). At tumor relapse, the two groups were differentiated in terms of: 1) time to relapse; 2) tumor stage before first-line treatment; and 3) tumor stage and tumor burden at relapse. A third (high-risk) group included non-responders when positron emission tomography (PET) was positive (i.e., Deauville score 4, 5) after two cycles of salvage chemotherapy.
Patients in the low-risk group were treated with conventional-dose salvage therapy and RT. The standard-risk group received conventional-dose salvage chemotherapy plus consolidation with high-dose chemotherapy followed by autologous stem cell transplantation. Patients in the high-risk group were candidates for autologous or allogeneic transplantation, or experimental therapies. There is currently no gold standard for choosing the best first-line and rescue therapies. Clinical trials conducted in the 2000s using different combinations of conventional chemotherapy, alone or followed by RT, in children and adolescents with low-risk, intermediate/high-risk, or relapsed HL are listed in Tables 2–4, respectively.
Consolidation RT improves progression-free survival in HL, but large irradiation fields are associated with an increased risk of secondary cancers and infertility, as well as heart and lung toxicities. Among these treatment-related complications, fertility issues have been paid particular attention in the setting of pediatric cancer care (given the patients’ young age), and fertility preservation is included in the discussion on the choice of treatment. In 2006, the American Society of Clinical Oncology (ASCO) published recommendations for fertility preservation in cancer patients. This document, and the update published in 2013, underscores the importance of discussing the risk of infertility associated with cancer therapies, and the options available for fertility preservation with patients as soon as possible. There are currently no effective ways to preserve fertility in prepubertal males with cancer, but most other patients can produce enough seminal fluid for cryopreservation before starting cancer therapy (Brannigan, 2014). Treatments may also adversely affect female reproductive organ function. In collaboration with the International Late Effects of Childhood Cancer Guideline Harmonization Group, the PanCareLIFE Consortium recently developed a practice guideline containing recommendations for fertility preservation in females with cancer up to 25 years of age (Mulder et al., 2021).
Fortunately, the use of smaller target volumes, combined with technological advances in treatment techniques, has led to the sparing of organs at risk without altering the efficient control of the tumor mass. Specifically, proton therapy has been proposed for treating mediastinal HL to significantly reduce the dose to organs at risk, such as cardiac substructures, and late toxicity (Loap et al., 2021). How to identify patients who may benefit from proton therapy is still being debated, however, as the slowness and complexity of technical aspects of the pretreatment setup and the cost of the instrument limit its routine use in clinical practice.
New target-specific biological drugs for cHL have been available since 2010, and have been used in combination with chemotherapy. Among them, brentuximab vedotin (Bv, SGN35), an anti-CD30 drug-conjugate antibody, and nivolumab, a monoclonal antibody (mAb) programmed cell death protein 1 (PD-1) inhibitor, were the first to be used in cases of relapsed/refractory cHL (Table 5). Clinical research on the specific clinical indications for their use, their association with traditional chemotherapy, and their potential long-term side effects is ongoing.
Bv conjugated with the antimitotic agent monomethylastatin E (MMAE), a cell cycle blocker that leads to tumor cell apoptosis, targets the tumor necrosis factor CD30+ in RS cells. A Phase I/II trial conducted in Europe and the United States showed a good safety profile and clinical benefits in 36 children with relapsed/refractory cHL (Locatelli et al., 2018). This therapy can also bridge to curative autologous and/or allogeneic stem cell transplantation in patients initially considered transplant-ineligible. Reported adverse events include: neutropenia (11%); fever (6%); increased gamma-glutamyl transferase levels (6%); and peripheral neuropathy (33%). The Food and Drug Administration (FDA) and the European Medicines Agency (EMA) approved Bv for use in adult patients with cHL if stem cell transplantation fails, or in patients not candidates for transplantation after ≥2 cycles of chemotherapy. Current therapies, including high-dose chemotherapy and stem cell transplantation, cure approximately 50% of children and young people with relapsed/refractory chronic HL. The Center for International Blood and Marrow Transplant Research (CIBMTR) reported a progression-free survival rate of 56%, and an overall survival of 73% in 671 patients (age ≤30 years).
A combination of Bv and the chemotherapy drug bendamustine showed a promising activity in a Phase II study conducted on adults with relapsed/recurrent HL (O’Connor et al., 2018). When administered before autologous stem cell transplantation, the overall response rate was 93%, with a 74% complete response and a favorable safety profile. Authorization is needed for its use in children, however. More recently, brentuximab was used in combination with various chemotherapeutic agents, with or without RT, in pediatric and young adults with newly-diagnosed, advanced-stage HL (NCT02979522, NCT02166463, and NCT01920932); the results are under evaluation.
In 2016, the EMA approved the use of nivolumab, a PD-1/PD-L1 immune checkpoint inhibitor that regulates T lymphocyte function in the TME, for the treatment of adults with relapsed/refractory cHL after autologous stem cell transplantation and Bv consolidation. CheckMate 744 (NCT02927769) was the first Phase II trial involving nivolumab, Bv, and bendamustine with response-adapted therapy for children, adolescents, and young adults at low or standard risk of relapse. Preliminary results for the standard-risk cohort were presented by the American Society of Clinical Oncology (ASCO) in 2020 (Cole et al., 2020). Of the 44 patients enrolled in the study, 31 were children, and 17 of them had refractory disease while 14 had recurrent cHL. The treatment was well tolerated and resulted in a complete metabolic response rate of 88%, making it an effective rescue therapy for relapsed/refractory cHL before stem cell transplantation.
Combination treatments with Bv plus PD/PD-L1 inhibitors have reportedly been tried in an effort to avoid RT, but they can induce immune-related adverse events. Bv is associated with peripheral neuropathy or severe pulmonary toxicity when combined with bleomycin, and PD/PD-L1 inhibitors may cause autoimmune toxicities. Further investigations in randomized trials are needed to assess the benefits and best uses of these agents as frontline treatments. The results of the ongoing Phase III trial (NCT03907488) comparing Nivo-AVD with Bv-AVD are awaited with particular interest (Castellino et al., 2020), though their use in pediatric patients has yet to be formally authorized.
The safety and efficacy of pembrolizumab, another PD-1 inhibitor, are under evaluation in the MK-3475/KEYNOTE-667 trial involving children and young adults with newly-diagnosed cHL and an inadequate response to chemotherapy. The results will be compare with the findings of the standard EuroNet-PHL-C2 study. The widespread use of immunotherapy has made it difficult to assess patients using this new therapeutic modality accurately, however. As PET often fails to differentiate pseudoprogression from true tumor progression in patients treated with immune complex inhibitors (ICIs) (Cheson et al., 2016; Aide et al., 2019), new criteria are needed to help clinicians assess response to ICIs, such as the iRECIST guidelines (Persigehl et al., 2020). A small number of patients also continue to show resistance to these new treatments, and the results are unsatisfactory, so it remains important to explore new strategies and targets for treating cHL.
Novel target drugs and treatment options in young patients with relapsed classic Hodgkin lymphoma
Table 5 gives a list of promising new targeted drugs. Alternative ICIs targeting PD-1/PD-L1 and other immune complexes that sustain an immunosuppressive TME have been discovered.
Sintilimab, camrelizumab, and tislelisumab all target PD-1. Ipilumumab targets the cytotoxic T lymphocyte-associated antigen-4 (CTLA-4) ligands CD80 (B7-1) and CD86 (B7-2), regulating the crosstalk between T cells and antigen-presenting cells (APC). Notably, CTLA-4+ cells, CD4+ Th1 polarized T cells, and T-reg (the main T-cell subsets in HL) are in close proximity to the CD68+ macrophages around HRS cells, and they are PD-1 negative (Patel et al., 2019). A Phase II trial is ongoing to verify the efficacy of dual PD-1 and CTLA-4 blockade with Bv (NCT01896999).
Relatlimab targets lymphocyte activation gene-3 (LAG-3; CD223 antigen). LAG-3 inhibits the function and expansion of both CD4 and CD8 T cells, serving as a marker of exhausted T cells during chronic antigen stimulation. LAG-3 is strongly expressed in T cells around the HRS cells, particularly in those lacking MHC-II expression (Gandhi et al., 2006), and in relapsed HL compared with cases in remission. The mechanism of T-cell inhibition is still poorly understood (Workman et al., 2002). The specific functional ligands of LAG-3 are still uncertain too, but it is now recognized that LAG-3, with its structural homology to the CD4 antigen (Triebel et al., 1990), preferentially recognizes specific MHC-II peptide complexes and consequently suppresses the function of specific CD4+ T cells (Maruhashi et al., 2022). Several other ligands for LAG-3 have been found, such as lymph node sinusoidal endothelial cell C-type lectin (LSECtin), galectin-3, and fibrinogen-like protein 1 (FGL1/FREP1), and their role in LAG3+ immune cell interactions is still under investigation (Chocarro et al., 2021; Wang et al., 2019).
SRF231 and TTI-621 block the interaction between CD47 (a “do not eat me” signal) and its ligand, the regulatory protein α (SIRPα), primarily expressed on phagocytic cells. Cases of cHL with a strong CD47 expression in HRS cells are associated with a shorter event-free survival (Ding et al., 2021; Gholiha et al., 2022).
More studies are needed on other immune checkpoint receptors that can downregulate immune cell function, such as T-cell immunoglobulin and mucin domain-containing protein 3 (TIM-3), T-cell immunoglobulin and ITIM domain (TIGIT), and V-domain Ig suppressor of T-cell activation (VISTA).
In recent years, there has been increased interest in immunotherapies that use chimeric antigen receptor (CAR) T cells (Bollard et al., 2014; Ramos et al., 2017; Ruella et al., 2017; Wang et al., 2017; Ramos et al., 2020; Ho et al., 2021; Gholiha et al., 2022; Meier et al., 2022). Unlike conventional immunotherapies, CAR-T cells use engineered receptors that modulate the structure and function of human T-cell receptors (TCRs). CAR-T cells consist of an extracellular antigen-binding domain ahowing antigen specificity, a transmembrane domain, and one or more intracellular signaling domains (Figure 3). CAR-T cells packaged in a viral vector are then transported onto T cells to transduce CAR-T cells on the cell membrane surface. The T cells can thus recognize and kill the cells that express the target antigen. The power advantage of CAR-T cells over conventional immunotherapies lies in that they do not depend on antigenic peptide/MHC complex presentation for their activation, and in their long-term persistence and efficacy in the organism. Epitope location and antigen density on the surface of cancer cells may influence CAR-T cell efficiency. In addition, research into functional intracellular signaling domains has gained importance in the scientific community because intracellular co-stimulatory domains modulate several important cellular pathways, such as cell differentiation and cell death, which may increase CAR-T efficacy. Early studies demonstrating the advantage of CAR-T cell therapy in relapsed HL involved CAR-T cells directed against HRS cells expressing the EBV LMP-1 antigen (Bollard et al., 2014). Then CAR-T cells were used for all types of refractory/relapsed HL, regardless of their EBV status, by targeting HRS-specific surface markers such as CD30 molecules (Wang et al., 2017; Ramos et al., 2020). Several preclinical and clinical studies are now ongoing to upgrade the potential efficacy of CAR-T cell treatments using several approaches. The focus is mainly on targeting several antigens associated with HRS or immunosuppressive cell interactions in the TME, or on prolonging and increasing the immune response by increasing the living CAR-T cell levels and/or modulating the T-cell death pathway as recently reviewed in (Meier et al., 2022).
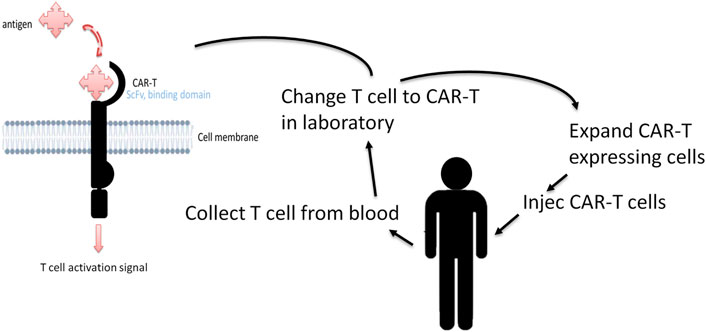
FIGURE 3. CAR-T structure and CAR-T cell therapy. The antigen-binding domain confers target specificity to CAR-T cells. This domain generally consists of variable heavy and light chains of monoclonal antibodies bound by a linker to form a single-chain variable fragment (scFv). The extracellular domain dictates the affinity and efficacy of the CAR-T cells and is independent of MHC-mediated antigen presentation. The difference in the intracellular co-stimulatory domains results in the modulation of different pathways like T cell differentiation and type of cell death. CAR-T treatment works by redirecting a patient’s own immune T cells, engineered in the laboratory, to directly identify and attack cancer cells.
Other targetable drug strategies, in mono- or combined therapies, include targeting HRS cell markers using antibody-toxin conjugates such as camidanlumab, or specific molecular pathways that are altered in HL, such as JAK (i.e., ruxolitinib, fedratinib, itacinib), or using mTOR inhibitors (i.e., everolimus) (Table 5). Alternatively, CD30− CD15− peripheral B cells may be the progenitors of the CD30+ HRS cells (Jones et al., 2009), and thus B cells might be potential targets of therapy.
Another approach to treating HL involves potentiating the effects of conventional chemotherapies with the aid of nuclear export (XPOI) inhibitors (i.e., Selinexor), epigenetic histone deacetylases (HDACs), and DNA methyltransferases (DNMTs), for instance (Table 5). One promising strategy is based on the use of hypomethylating agents in combination with ICIs. The rationale behind this approach stems from the discovery that MHC gene silencing can be reversed using hypomethylating drugs, resulting in a greater efficacy of cytotoxic T cells according to several studies (Falchi et al., 2016; Nie et al., 2019; Wang et al., 2021). Since EBV latency is partly controlled by DNA methylation, however, the use of hypomethylating agents has only been proposed for the EBV-negative cHL subtype. The combination of HDAC and ICIs has also shown efficacy and adequate tolerability.
Conclusion
Current research has led to the development of various strategies aimed at striking the best balance between supporting survival and reducing the long-term risk of toxicities related to therapies, including RT. The therapeutic strategies adopted for pediatric and young adults are consequently now quite different from those applied to adults and elderly patients with HL. It will be important to: 1) develop further studies on risk classification based not only on the classical parameters, but also using metabolic PET imaging; 2) identify patients eligible for proton therapy; and 3) discover new targeted drugs to include in more efficient combination treatment strategies. Studies on HRS cell biology and its close interaction with a multitude of cell subtypes in the TME could be useful for clarifying the molecular mechanisms that sustain the disease, and thereby reveal the best targets of treatment with a view to further reducing the risk of recurrence and long-term toxicity.
Author contributions
All authors listed have made a substantial, direct, and intellectual contribution to the work and approved it for publication.
Funding
This work was supported by Italian Ministry of Health, Ricerca Corrente and GOCNE ONLUS, Gruppo Oncologico Cooperativo North Est.
Conflict of interest
The authors declare that the research was conducted in the absence of any commercial or financial relationships that could be construed as a potential conflict of interest.
Publisher’s note
All claims expressed in this article are solely those of the authors and do not necessarily represent those of their affiliated organizations, or those of the publisher, the editors and the reviewers. Any product that may be evaluated in this article, or claim that may be made by its manufacturer, is not guaranteed or endorsed by the publisher.
References
Aide, N., Hicks, R. J., Le Tourneau, C., Lheureux, S., Fanti, S., and Lopci, E. (2019). FDG PET/CT for assessing tumour response to immunotherapy : Report on the EANM symposium on immune modulation and recent review of the literature. Eur. J. Nucl. Med. Mol. Imaging 46, 238–250. doi:10.1007/s00259-018-4171-4
Airtum Working Group, Ccm, (2013). Italian cancer figures, report 2012: Cancer in children and adolescents. Epidemiol. Prev. 37, 1–225.
Ansell, S. M., Maris, M. B., Lesokhin, A. M., Chen, R. W., Flinn, I. W., Sawas, A., et al. (2021). Phase I study of the CD47 blocker TTI-621 in patients with relapsed or refractory hematologic malignancies. Clin. Cancer Res. 27, 2190–2199. doi:10.1158/1078-0432.CCR-20-3706
Baetz, T., Belch, A., Couban, S., Imrie, K., Yau, J., Myers, R., et al. (2003). Gemcitabine, dexamethasone and cisplatin is an active and non-toxic chemotherapy regimen in relapsed or refractory Hodgkin’s disease: A phase II study by the national cancer institute of Canada clinical trials group. Ann. Oncol. 14, 1762–1767. doi:10.1093/annonc/mdg496
Balasubramanian, S. K., Azmi, A. S., and Maciejewski, J. (2022). Selective inhibition of nuclear export: A promising approach in the shifting treatment paradigms for hematological neoplasms. Leukemia 36, 601–612. doi:10.1038/s41375-021-01483-z
Bartlett, N. L., Niedzwiecki, D., Johnson, J. L., Friedberg, J. W., Johnson, K. B., van Besien, K., et al. (2007). Gemcitabine, vinorelbine, and pegylated liposomal doxorubicin (GVD), a salvage regimen in relapsed Hodgkin’s lymphoma: Calgb 59804. Ann. Oncol. 18, 1071–1079. doi:10.1093/annonc/mdm090
Ben Dhiab, M., Ziadi, S., Mestiri, S., Ben Gacem, R., Ksiaa, F., and Trimeche, M. (2015). DNA methylation patterns in EBV-positive and EBV-negative Hodgkin lymphomas. Cell. Oncol. 38, 453–462. doi:10.1007/s13402-015-0242-8
Boiocchi, M., De Re, V., Gloghini, A., Vaccher, E., Dolcetti, R., Marzotto, A., et al. (1993). High-incidence of monoclonal ebv episomes in hodgkins-disease and anaplastic large-cell ki-1-positive lymphomas in hiv-1-positive patients. Int. J. Cancer 54, 53–59. doi:10.1002/ijc.2910540110
Bollard, C. M., Gottschalk, S., Torrano, V., Diouf, O., Ku, S., Hazrat, Y., et al. (2014). Sustained complete responses in patients with lymphoma receiving autologous cytotoxic T lymphocytes targeting Epstein-Barr virus latent membrane proteins. J. Clin. Oncol. 32, 798–808. doi:10.1200/JCO.2013.51.5304
Borchmann, P., Goergen, H., Kobe, C., Lohri, A., Greil, R., Eichenauer, D. A., et al. (2017). PET-Guided treatment in patients with advanced-stage Hodgkin’s lymphoma (HD18): Final results of an open-label, international, randomised phase 3 trial by the German hodgkin study group. Lancet 390, 2790–2802. doi:10.1016/S0140-6736(17)32134-7
Brannigan, R. E. (2014). Risk of infertility in male survivors of childhood cancer. Lancet. Oncol. 15, 1181–1182. doi:10.1016/S1470-2045(14)70450-4
Buedts, L., Wlodarska, I., Finalet-Ferreiro, J., Gheysens, O., Dehaspe, L., Tousseyn, T., et al. (20212002). The landscape of copy number variations in classical hodgkin lymphoma: A joint KU leuven and LYSA study on cell-free DNA. Blood Adv. 5, 1991–2002. doi:10.1182/bloodadvances.2020003039
Burnelli, R., Fiumana, G., Rondelli, R., Pillon, M., Sala, A., Garaventa, A., et al. (2020). Comparison of Hodgkin’s lymphoma in children and adolescents. A twenty year experience with MH’96 and LH2004 AIEOP (Italian association of pediatric Hematology and Oncology) protocols. Cancers 12, 1620. doi:10.3390/cancers12061620
Burnelli, R., Rinieri, S., Rondelli, R., Todesco, A., Bianchi, M., Garaventa, A., et al. (2018). Long-term results of the AIEOP MH’96 childhood Hodgkin’s lymphoma trial and focus on significance of response to chemotherapy and its implication in low risk patients to avoid radiotherapy. Leuk. Lymphoma 59, 2612–2621. doi:10.1080/10428194.2018.1435872
Camus, V., Stamatoullas, A., Mareschal, S., Viailly, P-J., Sarafan-Vasseur, N., Bohers, E., et al. (2016). Detection and prognostic value of recurrent exportin 1 mutations in tumor and cell-free circulating DNA of patients with classical Hodgkin lymphoma. Haematologica 101, 1094–1101. doi:10.3324/haematol.2016.145102
Casagrande, N., Borghese, C., and Aldinucci, D. (2022). In classical Hodgkin lymphoma the combination of the CCR5 antagonist maraviroc with trabectedin synergizes, enhances DNA damage and decreases three-dimensional tumor-stroma heterospheroid viability. Haematologica 107, 287–291. doi:10.3324/haematol.2021.279389
Castellino, S. M., LeBlanc, M. L., Herrera, A. F., Parsons, S. K., Punnett, A., Hodgson, D. C., et al. (2020). An intergroup collaboration for advanced stage classical hodgkin lymphoma (cHL) in adolescents and young adults (AYA): Swog S1826. JCO 38, TPS8067. doi:10.1200/JCO.2020.38.15_suppl.TPS8067
Castellino, S. M., Parsons, S. K., and Kelly, K. M. (2019). Closing the survivorship gap in children and adolescents with Hodgkin lymphoma. Br. J. Haematol. 187, 573–587. doi:10.1111/bjh.16197
Cheson, B. D., Ansell, S., Schwartz, L., Gordon, L. I., Advani, R., Jacene, H. A., et al. (2016). Refinement of the Lugano Classification lymphoma response criteria in the era of immunomodulatory therapy. Blood 128, 2489–2496. doi:10.1182/blood-2016-05-718528
Chocarro, L., Blanco, E., Zuazo, M., Arasanz, H., Bocanegra, A., Fernández-Rubio, L., et al. (2021). Understanding LAG-3 signaling. Int. J. Mol. Sci. 22, 5282. doi:10.3390/ijms22105282
Cole, P. D., Mauz-Korholz, C., Mascarin, M., Michel, G., Cooper, S., Beishuizen, A., et al. Nivolumab and brentuximab vedotin (BV)-based, response-adapted treatment in children, adolescents, and young adults (CAYA) with standard-risk relapsed/refractory classical Hodgkin lymphoma (R/R cHL): Primary analysis. J. Clin. Oncol. (2020) 38: [Accessed April 19, 2022]8013, doi:10.1200/jco.2020.38.15_suppl.8013
Cole, P. D., McCarten, K. M., Pei, Q., Spira, M., Metzger, M. L., Drachtman, R. A., et al. (2018). Brentuximab vedotin with gemcitabine for paediatric and young adult patients with relapsed or refractory Hodgkin’s lymphoma (AHOD1221): A children’s Oncology group, multicentre single-arm, phase 1-2 trial. Lancet. Oncol. 19, 1229–1238. doi:10.1016/S1470-2045(18)30426-1
Connors, J. M., Cozen, W., Steidl, C., Carbone, A., Hoppe, R. T., Flechtner, H-H., et al. (2020). Hodgkin lymphoma. Nat. Rev. Dis. Prim. 6, 61. doi:10.1038/s41572-020-0189-6
Connors, J. M., Jurczak, W., Straus, D. J., Ansell, S. M., Kim, W. S., Gallamini, A., et al. (2018). Brentuximab vedotin with chemotherapy for stage III or IV Hodgkin’s lymphoma. N. Engl. J. Med. 378, 331–344. doi:10.1056/NEJMoa1708984
Dave, H., Terpilowski, M., Mai, M., Toner, K., Grant, M., Stanojevic, M., et al. (2022). Tumor-associated antigen-specific T cells with nivolumab are safe and persist in vivo in relapsed/refractory Hodgkin lymphoma. Blood Adv. 6, 473–485. doi:10.1182/bloodadvances.2021005343
Davis, K. L., Fox, E., Merchant, M. S., Reid, J. M., Kudgus, R. A., Liu, X., et al. (2020). Nivolumab in children and young adults with relapsed or refractory solid tumours or lymphoma (ADVL1412): A multicentre, open-label, single-arm, phase 1-2 trial. Lancet. Oncol. 21, 541–550. doi:10.1016/S1470-2045(20)30023-1
Daw, S., Hasenclever, D., Mascarin, M., Fernández-Teijeiro, A., Balwierz, W., Beishuizen, A., et al. (2020). Risk and response adapted treatment guidelines for managing first relapsed and refractory classical hodgkin lymphoma in children and young people. Recommendations from the EuroNet pediatric hodgkin lymphoma group. Hemasphere 4, e329. doi:10.1097/HS9.0000000000000329
De Re, V., Caggiari, L., De Zorzi, M., Fanotto, V., Miolo, G., Puglisi, F., et al. (2020). Epstein-Barr virus BART microRNAs in EBV- associated Hodgkin lymphoma and gastric cancer. Infect. Agent. Cancer 15, 42. doi:10.1186/s13027-020-00307-6
Desch, A-K., Hartung, K., Botzen, A., Brobeil, A., Rummel, M., Kurch, L., et al. (2020). Genotyping circulating tumor DNA of pediatric Hodgkin lymphoma. Leukemia 34, 151–166. doi:10.1038/s41375-019-0541-6
Diefenbach, C. S., Hong, F., Ambinder, R. F., Cohen, J. B., Robertson, M. J., David, K. A., et al. (2020). Ipilimumab, nivolumab, and brentuximab vedotin combination therapies in patients with relapsed or refractory hodgkin lymphoma: Phase 1 results of an open-label, multicentre, phase 1/2 trial. Lancet. Haematol. 7, e660–e670. doi:10.1016/S2352-3026(20)30221-0
Diepstra, A., van Imhoff, G. W., Karim-Kos, H. E., van den Berg, A., te Meerman, G. J., Niens, M., et al. (2007). HLA class II expression by Hodgkin Reed-Sternberg cells is an independent prognostic factor in classical Hodgkin’s lymphoma. J. Clin. Oncol. 25, 3101–3108. doi:10.1200/JCO.2006.10.0917
Ding, X-S., Mi, L., Song, Y-Q., Liu, W-P., Yu, H., Lin, N-J., et al. (2021). Relapsed/refractory classical hodgkin lymphoma effectively treated with low-dose decitabine plus tislelizumab: A case report. World J. Clin. Cases 9, 6041–6048. doi:10.12998/wjcc.v9.i21.6041
Dolcetti, R., Gloghini, A., Devita, S., Vaccher, E., De Re, V., Tirelli, U., et al. (1995). Characteristics of ebv-infected cells in hiv-related lymphadenopathy - implications for the pathogenesis of ebv-associated and ebv-unrelated lymphomas of hiv-seropositive individuals. Int. J. Cancer 63, 652–659. doi:10.1002/ijc.2910630509
Dörffel, W., Rühl, U., Lüders, H., Claviez, A., Albrecht, M., Bökkerink, J., et al. (2013). Treatment of children and adolescents with hodgkin lymphoma without radiotherapy for patients in complete remission after chemotherapy: Final results of the multinational trial GPOH-HD95. J. Clin. Oncol. 31, 1562–1568. doi:10.1200/JCO.2012.45.3266
Dörsam, B., Bösl, T., Reiners, K. S., Barnert, S., Schubert, R., Shatnyeva, O., et al. (2018). Hodgkin lymphoma-derived extracellular vesicles change the secretome of fibroblasts toward a CAF phenotype. Front. Immunol. 9, 1358. doi:10.3389/fimmu.2018.01358
El Halabi, L., Adam, J., Gravelle, P., Marty, V., Danu, A., Lazarovici, J., et al. (2021). Expression of the immune checkpoint regulators LAG-3 and TIM-3 in classical hodgkin lymphoma. Clin. Lymphoma Myeloma Leuk. 21, 257–266.e3. e3. doi:10.1016/j.clml.2020.11.009
Falchi, L., Sawas, A., Deng, C., Amengual, J. E., Colbourn, D. S., Lichtenstein, E. A., et al. (2016). High rate of complete responses to immune checkpoint inhibitors in patients with relapsed or refractory Hodgkin lymphoma previously exposed to epigenetic therapy. J. Hematol. Oncol. 9, 132. doi:10.1186/s13045-016-0363-1
Feng, Y., Chen, X., Cassady, K., Zou, Z., Yang, S., Wang, Z., et al. (2020). The role of mTOR inhibitors in hematologic disease: From bench to bedside. Front. Oncol. 10, 611690. doi:10.3389/fonc.2020.611690
Fisher, J. G., Walker, C. J., Doyle, A. D. P., Johnson, P. W. M., Forconi, F., Cragg, M. S., et al. (2021). Selinexor enhances NK cell activation against malignant B cells via downregulation of HLA-E. Front. Oncol. 11, 785635. doi:10.3389/fonc.2021.785635
Frisan, T., Sjoberg, J., Dolcetti, R., Boiocchi, M., De Re, V., Carbone, A., et al. (1995). Local suppression of epstein-barr-virus (ebv)-Specific cytotoxicity in biopsies of ebv-positive hodgkins-disease. Blood 86, 1493–1501. doi:10.1182/blood.V86.4.1493.bloodjournal8641493
Galinski, B., Alexander, T. B., Mitchell, D. A., Chatwin, H. V., Awah, C., Green, A. L., et al. (2021). Therapeutic targeting of exportin-1 in childhood cancer. Cancers 13, 6161. doi:10.3390/cancers13246161
Gandhi, M. K., Lambley, E., Duraiswamy, J., Dua, U., Smith, C., Elliott, S., et al. (2006). Expression of LAG-3 by tumor-infiltrating lymphocytes is coincident with the suppression of latent membrane antigen–specific CD8+ T-cell function in Hodgkin lymphoma patients. Blood 108, 2280–2289. doi:10.1182/blood-2006-04-015164
Geoerger, B., Kang, H. J., Yalon-Oren, M., Marshall, L. V., Vezina, C., Pappo, A., et al. (2020). Pembrolizumab in paediatric patients with advanced melanoma or a PD-L1-positive, advanced, relapsed, or refractory solid tumour or lymphoma (KEYNOTE-051): Interim analysis of an open-label, single-arm, phase 1-2 trial. Lancet. Oncol. 21, 121–133. doi:10.1016/S1470-2045(19)30671-0
Gholiha, A. R., Hollander, P., Löf, L., Glimelius, I., Hedstrom, G., Molin, D., et al. (2022). Checkpoint CD47 expression in classical Hodgkin lymphoma. Br. J. Haematol. 197, 580–589. doi:10.1111/bjh.18137
Gilmore, T. D., Kalaitzidis, D., Liang, M-C., and Starczynowski, D. T. (2004). The c-rel transcription factor and B-cell proliferation: A deal with the devil. Oncogene 23, 2275–2286. doi:10.1038/sj.onc.1207410
Gopal, A. K., Fanale, M. A., Moskowitz, C. H., Shustov, A. R., Mitra, S., Ye, W., et al. (2017). Phase II study of idelalisib, a selective inhibitor of PI3Kδ, for relapsed/refractory classical Hodgkin lymphoma. Ann. Oncol. 28, 1057–1063. doi:10.1093/annonc/mdx028
Hamadani, M., Collins, G. P., Caimi, P. F., Samaniego, F., Spira, A., Davies, A., et al. (2021). Camidanlumab tesirine in patients with relapsed or refractory lymphoma: A phase 1, open-label, multicentre, dose-escalation, dose-expansion study. Lancet. Haematol. 8, e433–e445. doi:10.1016/S2352-3026(21)00103-4
Hansen, H. P., Engels, H-M., Dams, M., Paes Leme, A. F., Pauletti, B. A., Simhadri, V. L., et al. (2014). Protrusion-guided extracellular vesicles mediate CD30 trans-signalling in the microenvironment of Hodgkin’s lymphoma. J. Pathol. 232, 405–414. doi:10.1002/path.4306
Higuchi, H., Yamakawa, N., Imadome, K-I., Yahata, T., Kotaki, R., Ogata, J., et al. (2018). Role of exosomes as a proinflammatory mediator in the development of EBV-associated lymphoma. Blood 131, 2552–2567. doi:10.1182/blood-2017-07-794529
Ho, C., Ruella, M., Levine, B. L., and Svoboda, J. (2021). Adoptive T-cell therapy for Hodgkin lymphoma. Blood Adv. 5, 4291–4302. doi:10.1182/bloodadvances.2021005304
Janik, J. E., Morris, J. C., O’Mahony, D., Pittaluga, S., Jaffe, E. S., Redon, C. E., et al. (2015). 90Y-daclizumab, an anti-CD25 monoclonal antibody, provided responses in 50% of patients with relapsed Hodgkin’s lymphoma. Proc. Natl. Acad. Sci. U. S. A. 112, 13045–13050. doi:10.1073/pnas.1516107112
Janku, F., Park, H., Call, S. G., Madwani, K., Oki, Y., Subbiah, V., et al. (2021). Safety and efficacy of vorinostat plus sirolimus or everolimus in patients with relapsed refractory hodgkin lymphoma. Clin. Cancer Res. 26, 5579–5587. doi:10.1158/1078-0432.CCR-20-1215
Johnston, P. B., Pinter-Brown, L. C., Warsi, G., White, K., and Ramchandren, R. (2018). Phase 2 study of everolimus for relapsed or refractory classical Hodgkin lymphoma. Exp. Hematol. Oncol. 7, 12. doi:10.1186/s40164-018-0103-z
Jones, R. J., Gocke, C. D., Kasamon, Y. L., Miller, C. B., Perkins, B., Barber, J. P., et al. (2009). Circulating clonotypic B cells in classic Hodgkin lymphoma. Blood 113, 5920–5926. doi:10.1182/blood-2008-11-189688
Kaplon, H., and Reichert, J. M. (2019). Antibodies to watch in 2019. mAbs 11, 219–238. doi:10.1080/19420862.2018.1556465
Keller, F. G., Nachman, J., Constine, L., Thomson, J., McCarten, K. M., Chen, L., et al. (2010). A phase III study for the treatment of children and adolescents with newly diagnosed low risk hodgkin lymphoma (HL). Blood 116, 767. doi:10.1182/blood.V116.21.767.767
Kelly, K. M., Sposto, R., Hutchinson, R., Massey, V., McCarten, K., Perkins, S., et al. (2011). BEACOPP chemotherapy is a highly effective regimen in children and adolescents with high-risk hodgkin lymphoma: A report from the children’s Oncology group. Blood 117, 2596–2603. doi:10.1182/blood-2010-05-285379
Khan, M. R., Ahmad, A., Kayani, N., and Minhas, K. (2018). Expression of PAX-5 in B cell hodgkin and non hodgkin lymphoma. Asian pac. J. Cancer Prev. 19, 3463–3466. doi:10.31557/APJCP.2018.19.12.3463
Kober-Hasslacher, M., and Schmidt-Supprian, M. (2019). The unsolved puzzle of c-rel in B cell lymphoma. Cancers 11, 941. doi:10.3390/cancers11070941
Küppers, R. (2009). The biology of Hodgkin’s lymphoma. Nat. Rev. Cancer 9, 15–27. doi:10.1038/nrc2542
Kuruvilla, J., Ramchandren, R., Santoro, A., Paszkiewicz-Kozik, E., Gasiorowski, R., Johnson, N. A., et al. (2021). Pembrolizumab versus brentuximab vedotin in relapsed or refractory classical hodgkin lymphoma (KEYNOTE-204): An interim analysis of a multicentre, randomised, open-label, phase 3 study. Lancet. Oncol. 22, 512–524. doi:10.1016/S1470-2045(21)00005-X
Landman-Parker, J., Wallace, H., Hasenclever, D., Balwierz, W., Karlen, J., Fossa, A., et al. (2016). First international inter-group study for classical hodgkin lymphoma in children and adolescents: euronet-phl-c1. report of the latest interim analysis. Haematologica 101, 35.
Loap, P., De Marzi, L., Mirandola, A., Dendale, R., Iannalfi, A., Vitolo, V., et al. (2021). Development and implementation of proton therapy for hodgkin lymphoma: Challenges and perspectives. Cancers 13, 3744. doi:10.3390/cancers13153744
Locatelli, F., Mauz-Koerholz, C., Neville, K., Llort, A., Beishuizen, A., Daw, S., et al. (2018). Brentuximab vedotin for paediatric relapsed or refractory Hodgkin’s lymphoma and anaplastic large-cell lymphoma: A multicentre, open-label, phase 1/2 study. Lancet. Haematol. 5, E450–E461. doi:10.1016/S2352-3026(18)30153-4
Lopez-Pereira, B., Fernandez-Velasco, A. A., Fernandez-Vega, I., Corte-Torres, D., Quiros, C., Villegas, J. A., et al. (2020). Expression of CD47 antigen in Reed-Sternberg cells as a new potential biomarker for classical Hodgkin lymphoma. Clin. Transl. Oncol. 22, 782–785. doi:10.1007/s12094-019-02171-2
Marks, L. J., Pei, Q., Bush, R., Buxton, A., Appel, B., Kelly, K. M., et al. (2018). Outcomes in intermediate-risk pediatric lymphocyte-predominant hodgkin lymphoma: A report from the children’s Oncology group. Pediatr. Blood Cancer 65, e27375. doi:10.1002/pbc.27375
Maruhashi, T., Okazaki, I-M., Sugiura, D., Takahashi, S., Maeda, T. K., Shimizu, K., et al. (2018) LAG-3 inhibits the activation of CD4(+) T cells that recognize stable pMHCII through its conformation-dependent recognition of pMHCII. Nat. Immunol. 19:1415–1426. doi:10.1038/s41590-018-0217-9
Maruhashi, T., Sugiura, D., Okazaki, I-M., Shimizu, K., Maeda, T. K., Ikubo, J., et al. Binding of LAG-3 to stable peptide-MHC class II limits T cell function and suppresses autoimmunity and anti-cancer immunity. Immunity (2022) 55:912–924.e8. doi:10.1016/j.immuni.2022.03.013
Massini, G., Siemer, D., and Hohaus, S. (2009). EBV in hodgkin lymphoma. Mediterr. J. Hematol. Infect. Dis. 1, e2009013. doi:10.4084/MJHID.2009.013
Mathas, S., Hinz, M., Anagnostopoulos, I., Krappmann, D., Lietz, A., Jundt, F., et al. (2002). Aberrantly expressed c-Jun and JunB are a hallmark of Hodgkin lymphoma cells, stimulate proliferation and synergize with NF-kappa B. EMBO J. 21, 4104–4113. doi:10.1093/emboj/cdf389
Mauz-Körholz, C., Hasenclever, D., Dörffel, W., Ruschke, K., Pelz, T., Voigt, A., et al. (2010). Procarbazine-free OEPA-COPDAC chemotherapy in boys and standard OPPA-COPP in girls have comparable effectiveness in pediatric Hodgkin’s lymphoma: The GPOH-HD-2002 study. J. Clin. Oncol. 28, 3680–3686. doi:10.1200/JCO.2009.26.9381
Mauz-Körholz, C., Landman-Parker, J., Balwierz, W., Ammann, R. A., Anderson, R. A., Attarbaschi, A., et al. (2022). Response-adapted omission of radiotherapy and comparison of consolidation chemotherapy in children and adolescents with intermediate-stage and advanced-stage classical Hodgkin lymphoma (EuroNet-PHL-C1): A titration study with an open-label, embedded, multinational, non-inferiority, randomised controlled trial. Lancet. Oncol. 23, 125–137. doi:10.1016/S1470-2045(21)00470-8
McAulay, K. A., and Jarrett, R. F. (2015). Human leukocyte antigens and genetic susceptibility to lymphoma. Tissue Antigens 86, 98–113. doi:10.1111/tan.12604
Meier, J. A., Savoldo, B., and Grover, N. S. (2022). The emerging role of CAR T cell therapy in relapsed/refractory hodgkin lymphoma. J. Pers. Med. 12, 197. doi:10.3390/jpm12020197
Michot, J-M., Mouraud, S., Adam, J., Lazarovici, J., Bigenwald, C., Rigaud, C., et al. (2021). CD8+ T lymphocytes immune depletion and LAG-3 overexpression in hodgkin lymphoma tumor microenvironment exposed to anti-PD-1 immunotherapy. Cancers 13, 5487. doi:10.3390/cancers13215487
M’kacher, R., Frenzel, M., Al Jawhari, M., Junker, S., Cuceu, C., Morat, L., et al. (2018). Establishment and characterization of a reliable xenograft model of hodgkin lymphoma suitable for the study of tumor origin and the design of new therapies. Cancers (Basel) 10, E414. doi:10.3390/cancers10110414
Moskowitz, C. H., Nimer, S. D., Zelenetz, A. D., Trippett, T., Hedrick, E. E., Filippa, D. A., et al. (2001). A 2-step comprehensive high-dose chemoradiotherapy second-line program for relapsed and refractory hodgkin disease: Analysis by intent to treat and development of a prognostic model. Blood 97, 616–623. doi:10.1182/blood.v97.3.616
Mulder, R. L., Font-Gonzalez, A., Green, D. M., Loeffen, E. A. H., Hudson, M. M., Loonen, J., et al. (2021). Fertility preservation for male patients with childhood, adolescent, and young adult cancer: Recommendations from the PanCareLIFE Consortium and the international late effects of childhood cancer guideline harmonization group. Lancet. Oncol. 22, e57–e67. doi:10.1016/S1470-2045(20)30582-9
Nagasaki, J., Togashi, Y., Sugawara, T., Itami, M., Yamauchi, N., Yuda, J., et al. (2020). The critical role of CD4+ T cells in PD-1 blockade against MHC-II-expressing tumors such as classic Hodgkin lymphoma. Blood Adv. 4, 4069–4082. doi:10.1182/bloodadvances.2020002098
Nie, J., Wang, C., Liu, Y., Yang, Q., Mei, Q., Dong, L., et al. (2019) Addition of low-dose decitabine to anti-PD-1 antibody camrelizumab in relapsed/refractory classical hodgkin lymphoma. J. Clin. Oncol. 37:1479–1489. doi:10.1200/JCO.18.02151
O’Connor, O. A., Lue, J. K., Sawas, A., Amengual, J. E., Deng, C., Kalac, M., et al. (2018). Brentuximab vedotin plus bendamustine in relapsed or refractory Hodgkin’s lymphoma: An international, multicentre, single-arm, phase 1-2 trial. Lancet. Oncol. 19, 257–266. doi:10.1016/S1470-2045(17)30912-9
Oki, Y., Neelapu, S. S., Fanale, M., Kwak, L. W., Fayad, L., Rodriguez, M. A., et al. (2015). Detection of classical Hodgkin lymphoma specific sequence in peripheral blood using a next-generation sequencing approach. Br. J. Haematol. 169, 689–693. doi:10.1111/bjh.13349
Patel, SS, Weirather, JL, Lipschitz, M, Lako, A, Chen, P-H, Griffin, GK, Armand, P, Shipp, MA, and Rodig, SJ. The microenvironmental niche in classic Hodgkin lymphoma is enriched for CTLA-4-positive T cells that are PD-1-negative. Blood (2019) 134:2059–2069. doi:10.1182/blood.2019002206
Pei, Y., and Robertson, E. S. (2020). The crosstalk of epigenetics and metabolism in herpesvirus infection. Viruses 12, E1377. doi:10.3390/v12121377
Peluso, M. O., Adam, A., Armet, C. M., Zhang, L., O’Connor, R. W., Lee, B. H., et al. (2020). The Fully human anti-CD47 antibody SRF231 exerts dual-mechanism antitumor activity via engagement of the activating receptor CD32a. J. Immunother. Cancer 8, e000413. doi:10.1136/jitc-2019-000413
Persigehl, T., Lennartz, S., and Schwartz, L. H. (2020). iRECIST: how to do it. Cancer Imaging 20, 2. doi:10.1186/s40644-019-0281-x
Ramos, C. A., Ballard, B., Zhang, H., Dakhova, O., Gee, A. P., Mei, Z., et al. (2017). Clinical and immunological responses after CD30-specific chimeric antigen receptor-redirected lymphocytes. J. Clin. Invest. 127, 3462–3471. doi:10.1172/JCI94306
Ramos, C. A., Grover, N. S., Beaven, A. W., Lulla, P. D., Wu, M-F., Ivanova, A., et al. (2020). Anti-CD30 CAR-T cell therapy in relapsed and refractory hodgkin lymphoma. J. Clin. Oncol. 38, 3794–3804. doi:10.1200/JCO.20.01342
Reichel, J., Chadburn, A., Rubinstein, P. G., Giulino-Roth, L., Tam, W., Liu, Y., et al. (2015). Flow sorting and exome sequencing reveal the oncogenome of primary Hodgkin and Reed-Sternberg cells. Blood 125, 1061–1072. doi:10.1182/blood-2014-11-610436
Rengstl, B., Newrzela, S., Heinrich, T., Weiser, C., Thalheimer, F. B., Schmid, F., et al. (2013). Incomplete cytokinesis and re-fusion of small mononucleated Hodgkin cells lead to giant multinucleated Reed-Sternberg cells. Proc. Natl. Acad. Sci. U. S. A. 110, 20729–20734. doi:10.1073/pnas.1312509110
Repetto, O., Lovisa, F., Elia, C., Enderle, D., Romanato, F., Buffardi, S., et al. (2021). Proteomic exploration of plasma exosomes and other small extracellular vesicles in pediatric hodgkin lymphoma: A potential source of biomarkers for relapse occurrence. Diagn. (Basel) 11, 917. doi:10.3390/diagnostics11060917
Roemer, M. G. M., Advani, R. H., Ligon, A. H., Natkunam, Y., Redd, R. A., Homer, H., et al. (2016). PD-L1 and PD-L2 genetic alterations define classical hodgkin lymphoma and predict outcome. J. Clin. Oncol. 34, 2690–2697. doi:10.1200/JCO.2016.66.4482
Roemer, M. G. M., Redd, R. A., Cader, F. Z., Pak, C. J., Abdelrahman, S., Ouyang, J., et al. (2018) Major histocompatibility complex class II and programmed death ligand 1 expression predict outcome after programmed death 1 blockade in classic hodgkin lymphoma. J. Clin. Oncol. 36, 942–950. doi:10.1200/JCO.2017.77.3994
Ruella, M., Klichinsky, M., Kenderian, S. S., Shestova, O., Ziober, A., Kraft, D. O., et al. (2017). Overcoming the immunosuppressive tumor microenvironment of Hodgkin lymphoma using chimeric antigen receptor T cells. Cancer Discov. 7, 1154–1167. doi:10.1158/2159-8290.CD-16-0850
Rui, L., Emre, N. C. T., Kruhlak, M. J., Chung, H-J., Steidl, C., Slack, G., et al. (2010). Cooperative epigenetic modulation by cancer amplicon genes. Cancer Cell 18, 590–605. doi:10.1016/j.ccr.2010.11.013
Salipante, S. J., Mealiffe, M. E., Wechsler, J., Krem, M. M., Liu, Y., Namkoong, S., et al. (2009). Mutations in a gene encoding a midbody kelch protein in familial and sporadic classical Hodgkin lymphoma lead to binucleated cells. Proc. Natl. Acad. Sci. U. S. A. 106, 14920–14925. doi:10.1073/pnas.0904231106
Santoro, A., Magagnoli, M., Spina, M., Pinotti, G., Siracusano, L., Michieli, M., et al. (2007). Ifosfamide, gemcitabine, and vinorelbine: A new induction regimen for refractory and relapsed Hodgkin’s lymphoma. Haematologica 92, 35–41. doi:10.3324/haematol.10661
Santoro, A., Mazza, R., Pulsoni, A., Re, A., Bonfichi, M., Zilioli, V. R., et al. (2016). Bendamustine in combination with gemcitabine and vinorelbine is an effective regimen as induction chemotherapy before autologous stem-cell transplantation for relapsed or refractory hodgkin lymphoma: Final results of a multicenter phase II study. J. Clin. Oncol. 34, 3293–3299. doi:10.1200/JCO.2016.66.4466
Schellong, G., Dörffel, W., Claviez, A., Körholz, D., Mann, G., Scheel-Walter, H-G., et al. (2005). Salvage therapy of progressive and recurrent Hodgkin’s disease: Results from a multicenter study of the pediatric DAL/GPOH-HD study group. J. Clin. Oncol. 23, 6181–6189. doi:10.1200/JCO.2005.07.930
Schneider, M., Schneider, S., Zühlke-Jenisch, R., Klapper, W., Sundström, C., Hartmann, S., et al. (2015). Alterations of the CD58 gene in classical Hodgkin lymphoma. Genes Chromosom. Cancer 54, 638–645. doi:10.1002/gcc.22276
Schwartz, C. L., Constine, L. S., Villaluna, D., London, W. B., Hutchison, R. E., Sposto, R., et al. (2009). A risk-adapted, response-based approach using ABVE-PC for children and adolescents with intermediate- and high-risk hodgkin lymphoma: The results of P9425. Blood 114, 2051–2059. doi:10.1182/blood-2008-10-184143
Schwarzer, R., and Jundt, F. (2011). Notch and NF-kappa B signaling pathways in the biology of classical hodgkin lymphoma. Curr. Mol. Med. 11, 236–245. doi:10.2174/156652411795243423
Sheikh, I., Nunez, C., McCall, D., Roth, M., and Cuglievan, B. (2022). Programmed cell death protein blockade with pembrolizumab for classical Hodgkin lymphoma after autologous stem cell transplantation in an adolescent patient. Pediatr. Blood Cancer 69, e29390. doi:10.1002/pbc.29390
Shi, S., Calhoun, H. C., Xia, F., Li, J., Le, L., and Li, W. X. (2006). JAK signaling globally counteracts heterochromatic gene silencing. Nat. Genet. 38, 1071–1076. doi:10.1038/ng1860
Siref, A., McCormack, C., Huang, Q., Lim, W., and Alkan, S. (2020). Diminished expression of 5hmc in Reed-Sternberg cells in classical Hodgkin lymphoma is a common epigenetic marker. Leuk. Res. 96, 106408. doi:10.1016/j.leukres.2020.106408
Slyusarenko, M., Shalaev, S., Valitova, A., Zabegina, L., Nikiforova, N., Nazarova, I., et al. (2022). AuNP aptasensor for hodgkin lymphoma monitoring. Biosens. (Basel) 12, 23. doi:10.3390/bios12010023
Song, H., Liu, X., Jiang, L., Li, F., Zhang, R., and Wang, P. (2021). Current status and prospects of camrelizumab, A humanized antibody against programmed cell death receptor 1. Recent Pat. anticancer. Drug Discov. 16, 312–332. doi:10.2174/1574892816666210208231744
Spina, V., Bruscaggin, A., Cuccaro, A., Martini, M., Di Trani, M., Forestieri, G., et al. (2018). Circulating tumor DNA reveals genetics, clonal evolution, and residual disease in classical Hodgkin lymphoma. Blood 131, 2413–2425. doi:10.1182/blood-2017-11-812073
Swerdlow, S. H., Campo, E., Pileri, S. A., Lee Harris, N., Stein, H., Siebert, R., et al. (2016). The 2016 revision of the World Health Organization classification of lymphoid neoplasms. Blood 127, 2375–2390. doi:10.1182/blood-2016-01-643569
Tebbi, C. K., Mendenhall, N. P., London, W. B., Williams, J. L., Hutchison, R. E., Fitzgerald, T. J., et al. (2012). Response-dependent and reduced treatment in lower risk hodgkin lymphoma in children and adolescents, results of P9426: A report from the children’s Oncology group. Pediatr. Blood Cancer 59, 1259–1265. doi:10.1002/pbc.24279
Tiacci, E., Ladewig, E., Schiavoni, G., Penson, A., Fortini, E., Pettirossi, V., et al. (2018). Pervasive mutations of JAK-STAT pathway genes in classical Hodgkin lymphoma. Blood 131, 2454–2465. doi:10.1182/blood-2017-11-814913
Tobin, J. W. D., Bednarska, K., Campbell, A., and Keane, C. (2021). PD-1 and LAG-3 checkpoint blockade: Potential avenues for therapy in B-cell lymphoma. Cells 10, 1152. doi:10.3390/cells10051152
Tosetti, F., Venè, R., Camodeca, C., Nuti, E., Rossello, A., D’Arrigo, C., et al. (2018). Specific ADAM10 inhibitors localize in exosome-like vesicles released by Hodgkin lymphoma and stromal cells and prevent sheddase activity carried to bystander cells. Oncoimmunology 7, e1421889. doi:10.1080/2162402X.2017.1421889
Traila, A., Dima, D., Achimas-Cadariu, P., and Micu, R. (2018). Fertility preservation in Hodgkin’s lymphoma patients that undergo targeted molecular therapies: An important step forward from the chemotherapy era. Cancer Manag. Res. 10, 1517–1526. doi:10.2147/CMAR.S154819
Triebel, F., Jitsukawa, S., Baixeras, E., Roman-Roman, S., Genevee, C., Viegas-Pequignot, E., et al. (1990). LAG-3, a novel lymphocyte activation gene closely related to CD4. J. Exp. Med. 171, 1393–1405. doi:10.1084/jem.171.5.1393
Van den Neste, E., Andre, M., Gastinne, T., Stamatoullas, A., Haioun, C., Belhabri, A., et al. (2018). A phase II study of the oral JAK1/JAK2 inhibitor ruxolitinib in advanced relapsed/refractory Hodgkin lymphoma. Haematologica 103, 840–848. doi:10.3324/haematol.2017.180554
van Eijndhoven, M. A., Zijlstra, J. M., Groenewegen, N. J., Drees, E. E., van Niele, S., Baglio, S. R., et al. (2016). Plasma vesicle miRNAs for therapy response monitoring in Hodgkin lymphoma patients. JCI Insight 1, e89631. doi:10.1172/jci.insight.89631
Veldman, J., Visser, L., Huberts-Kregel, M., Muller, N., Hepkema, B., van den Berg, A., et al. (2020). Rosetting T cells in Hodgkin lymphoma are activated by immunological synapse components HLA class II and CD58. Blood 136, 2437–2441. doi:10.1182/blood.2020005546
Wang, C., Liu, Y., Dong, L., Li, X., Yang, Q., Brock, M. V., et al. (2021). Efficacy of decitabine plus anti-PD-1 camrelizumab in patients with hodgkin lymphoma who progressed or relapsed after PD-1 blockade monotherapy. Clin. Cancer Res. 27, 2782–2791. doi:10.1158/1078-0432.CCR-21-0133
Wang, C-M., Wu, Z-Q., Wang, Y., Guo, Y-L., Dai, H-R., Wang, X-H., et al. (2017). Autologous T cells expressing CD30 chimeric antigen receptors for relapsed or refractory hodgkin lymphoma: An open-label phase I trial. Clin. Cancer Res. 23, 1156–1166. doi:10.1158/1078-0432.CCR-16-1365
Wang, J., Sanmamed, M. F., Datar, I., Su, T. T., Ji, L., Sun, J., et al. (2019). Fibrinogen-like protein 1 is a major immune inhibitory ligand of LAG-3. Cell 176, 334–347. doi:10.1016/j.cell.2018.11.010
Wang, J., Van Den Berg, D., Hwang, A. E., Weisenberger, D., Triche, T., Nathwani, B. N., et al. (2019). DNA methylation patterns of adult survivors of adolescent/young adult Hodgkin lymphoma compared to their unaffected monozygotic twin. Leuk. Lymphoma 60, 1429–1437. doi:10.1080/10428194.2018.1533128
Webermatthiesen, K., Deerberg, J., Poetsch, M., Grote, W., and Schlegelberger, B. (1995). Numerical chromosome aberrations are present within the CD30+ Hodgkin and Reed-Sternberg cells in 100% of analyzed cases of Hodgkin's disease [see comments]. Blood 86, 1464–1468. doi:10.1182/blood.V86.4.1464.bloodjournal8641464
Weniger, M. A., Tiacci, E., Schneider, S., Arnolds, J., Rüschenbaum, S., Duppach, J., et al. (2018). Human CD30+ B cells represent a unique subset related to Hodgkin lymphoma cells. J. Clin. Invest. 128, 2996–3007. doi:10.1172/JCI95993
Wienand, K., Chapuy, B., Stewart, C., Dunford, A. J., Wu, D., Kim, J., et al. (2019). Genomic analyses of flow-sorted Hodgkin Reed-Sternberg cells reveal complementary mechanisms of immune evasion. Blood Adv. 3, 4065–4080. doi:10.1182/bloodadvances.2019001012
Wolska-Washer, A., and Robak, T. (2019). Safety and tolerability of antibody-drug conjugates in cancer. Drug Saf. 42, 295–314. doi:10.1007/s40264-018-0775-7
Workman, C. J., Dugger, K. J., and Vignaw, D. a. A. (2002). Cutting edge: Molecular analysis of the negative regulatory function of lymphocyte activation gene-3. J. Immunol. 169, 5392–5395. doi:10.4049/jimmunol.169.10.5392
Younes, A., Santoro, A., Shipp, M., Zinzani, P. L., Timmerman, J. M., Ansell, S., et al. (2016). Nivolumab for classical Hodgkin’s lymphoma after failure of both autologous stem-cell transplantation and brentuximab vedotin: A multicentre, multicohort, single-arm phase 2 trial. Lancet. Oncol. 17, 1283–1294. doi:10.1016/S1470-2045(16)30167-X
Zeng, Q., and Schwarz, H. (2020). The role of trogocytosis in immune surveillance of Hodgkin lymphoma. Oncoimmunology 9, 1781334. doi:10.1080/2162402X.2020.1781334
Glossary
HL Hodgkin lymphoma
cHL classic Hodgkin lymphoma
RT radiotherapy
PET positron emission tomography
HRS Hodgkin and Reed-Sternberg
LAG-3 lymphocyte activation gene 3
NLPHL nodular lymphocyte-predominant HL
NSHL nodular sclerosis Hodgkin lymphoma
MCHL mixed cell Hodgkin lymphoma
LRHL lymphocyte-rich Hodgkin lymphoma
LDHL lymphocyte-depleted Hodgkin lymphoma
EBV Epstein-Barr virus
EBER EBV-encoded RNA
LMP latent membrane protein
BCR B-cell receptor
PAX5 nuclear-paired box protein 5
Ig immunoglobulin
CD cluster of differentiation
TNF tumor necrosis factor
MHC major histocompatibility complex
miRNA microRNA
AP-1 activator protein-1
Jak/STAT Janus kinase/signal transducers, and activators of transcription
mTOR mechanistic target of rapamycin kinase
NF- κ B nuclear factor kappa-light-chain-enhancer of activated B cells
PDL programmed cell death ligand
TCR T-cell receptor
mAb monoclonal antibody
MMAE anti-mitotic agent monomethylastatin E
Bv Brentuximab vedotin
ICI immune complex inhibitor
EMA European Medicines Agency
SIRPα signal regulatory protein α
TIM-3 T-cell immunoglobulin and mucin domain-containing protein 3
TIGIT T-cell immunoglobulin and ITIM domain
VISTA V-domain Ig suppressor of T-cell activation
HDAC histone deacetylase
DNMT DNA methyltransferase
Keywords: Hodgkin lymphoma, Epstein-Barr virus, chemotherapy, radiation, tumor target, adolescent
Citation: De Re V, Repetto O, Mussolin L, Brisotto G, Elia C, Lopci E, d’Amore ESG, Burnelli R and Mascarin M (2022) Promising drugs and treatment options for pediatric and adolescent patients with Hodgkin lymphoma. Front. Cell Dev. Biol. 10:965803. doi: 10.3389/fcell.2022.965803
Received: 10 June 2022; Accepted: 07 November 2022;
Published: 24 November 2022.
Edited by:
Shyamala Maheswaran, Massachusetts General Hospital and Harvard Medical School, United StatesReviewed by:
Maulik Vyas, Massachusetts General Hospital and Harvard Medical School, United StatesYu Zhao, Xuanwu Hospital, Capital Medical University, China
Copyright © 2022 De Re, Repetto, Mussolin, Brisotto, Elia, Lopci, d’Amore, Burnelli and Mascarin. This is an open-access article distributed under the terms of the Creative Commons Attribution License (CC BY). The use, distribution or reproduction in other forums is permitted, provided the original author(s) and the copyright owner(s) are credited and that the original publication in this journal is cited, in accordance with accepted academic practice. No use, distribution or reproduction is permitted which does not comply with these terms.
*Correspondence: Valli De Re, dmRlcmVAY3JvLml0; Maurizio Mascarin, bWFzY2FyaW5AY3JvLml0