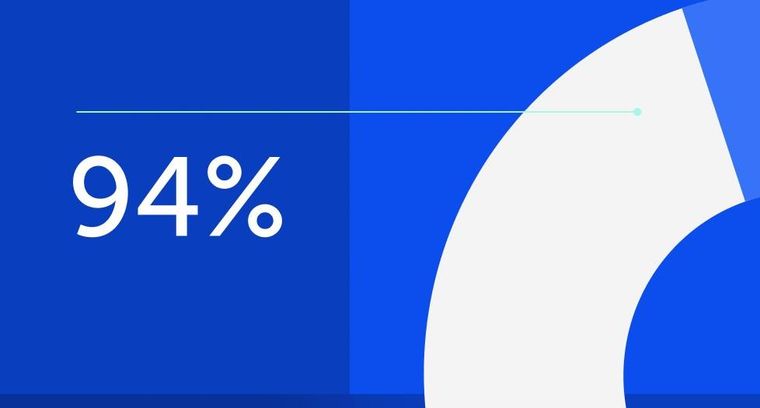
94% of researchers rate our articles as excellent or good
Learn more about the work of our research integrity team to safeguard the quality of each article we publish.
Find out more
ORIGINAL RESEARCH article
Front. Cell Dev. Biol., 02 September 2022
Sec. Cellular Biochemistry
Volume 10 - 2022 | https://doi.org/10.3389/fcell.2022.961675
This article is part of the Research TopicThe Role of Protein Post-Translational Modifications in Metabolic DisordersView all 7 articles
Ufmylation (UFM1 modification) is a newly identified ubiquitin-like modification system involved in numerous cellular processes. However, the regulatory mechanisms and biological functions of this modification remain mostly unknown. We have recently reported that Ufmylation family genes have frequent somatic copy number alterations in human cancer including melanoma, suggesting involvement of Ufmylation in skin function and disease. UFL1 is the only known Ufmylation E3-like ligase. In this study, we generated the skin-specific Ufl1 knockout mice and show that ablation of Ufl1 caused epidermal thickening, pigmentation and shortened life span. RNA-Seq analysis indicated that Ufl1 deletion resulted in upregulation of the genes involved in melanin biosynthesis. Mechanistically, we found that Endothelin-1 (ET-1) is a novel substrate of Ufmylation and this modification regulates ET-1 stability, and thereby deletion of Ufl1 upregulates the expression and secretion of ET-1, which in turn results in up-regulation of genes in melanin biosynthesis and skin pigmentation. Our findings establish the role of Ufl1 in skin pigmentation through Ufmylation modification of ET-1 and provide opportunities for therapeutic intervention of skin diseases.
Post-translational protein modification plays an important role in every aspect of cellular processes. Deficiency in protein modification is associated with numbers of human diseases (Themelis et al., 2005; Yang et al., 2016). Ubiquitination is the first and the one of the most prevalent post-translational protein modifications described so far. Besides Ubiquitination, several ubiquitin-like protein have been identified including SUMO, NEDD8, ISG15, Urm1, Atg8, Atg12, FAT10, and UFM1 (Hochstrasser, 2009). A growing number of studies have begun to unveil crucial revealed the role of NEDD8 (Zhou et al., 2016), ISG15 (Wolk et al., 2013; Malik et al., 2022), SUMO (Deyrieux et al., 2007; Zhou et al., 2018) in regulating skin development and diseases such as vitiligo, psoriasis, atopic dermatitis and inherited dermatological diseases. However, the biological function of the other ubiquitin-like proteins in the skin remains largely unknown.
UFM1 modification (Ufmylation) is a recently reported ubiquitin-like modification, which has one of each E1-like enzyme (Uba5), E2-like enzyme (Ufc1), E3-like ligase (Ufl1), and two UFM1 proteases UfSP1 and UfSP2 (Komatsu et al., 2004; Tatsumi et al., 2010). DDRGK1 (also known as UFM1-Binding Protein 1, UFBP1) is required for the maintenance of UFL1 ligase activity (Yoo et al., 2014). Dysfunction in the Ufmylation is implicated in numbers of human diseases (Azfer et al., 2006; Rubio et al., 2013; Hu et al., 2014; Yoo et al., 2014). E3-like ligase plays a key role in specific protein substrate recognition (Zheng & Shabek, 2017). Germline deletion of the Ufl1 gene in mice led to severe anemia and cytopenia or even embryonic lethality (Zhang et al., 2015). Cardiomyocyte-restricted Ufl1 knockout mice developed cardiomyopathy and heart failure (Li et al., 2018). Ablation of either Ufl1 or Ufbp1 led to significant loss of both Paneth and goblet cells and increased susceptibility to experimentally induced colitis (Cai et al., 2019). Ufl1 deficiency causes kidney atrophy associated with disruption of endoplasmic reticulum homeostasis (Zhou Y. et al, 2021). These recent findings underscore the importance of the Ufl1 in development and physiology. Nonetheless, the function of Ufl1 in skin remains uncharacterized.
The skin is the largest organ in the body and the first line of defense against the outside world (Grice & Segre, 2011). In addition, external color affects animal survival and reproduction, as pigmentation can provide camouflage, protect against photodamage, affect body temperature, and facilitate social interaction (Weiner et al., 2007). Skin pigmentation results from the synthesis of melanin in pigment-producing cells, melanocytes, with subsequent distribution and transport of pigment granules to adjacent keratinocytes (KCs), which are involved in regulation of the proliferation and differentiation of melanocytes. Endothelin-1 (ET-1), encoded by EDN1 gene, is a keratinocyte-derived factor that stimulate melanocytes located in the vicinity of keratinocytes by binding to endothelin receptors, which activates intracellular signaling cascades, and then regulates melanocyte proliferation and melanogenesis in the skin (Yada et al., 1991; Imokawa et al., 1992; Yohn et al., 1993; Hara et al., 1995). Moreover, it has been reported that addition of ET-1 to cultured human epidermal melanocytes results in increased expression of melanogenesis-related genes and tyrosinase activity (Imokawa et al., 1995). However, the underlying mechanism of ET-1 regulation in skin is poorly understood.
Our recent study shows that Ufmylation genes have frequent genomic alterations in tumors including melanoma (Zhou J. et al, 2021). To understand the role of Ufmylation in the skin, we generated skin Ufl1 knockout (CKO) mice and found that Ufl1 deletion results in epidermal thickening, pigmentation and shortened life span.
Generation of a transgenic mouse line bearing an Ufl1 conditional-knockout allele (Ufl1-floxed) described previously (Zhou Y. et al, 2021). To generate CKO mice, the Ufl1-floxed mice were crossed with KRT14Cre/+ mice. The primers used for PCR genotyping of the Ufl1-floxed mice and KRT14Cre/+ mice are described in Supplementary Table S1. All the mice used in this study were fed in Specific Pathogen Free (SPF) facilities. All animal experiments were performed according to the guidelines of the Animal Care and Use Committee of Hangzhou Normal University.
Morphologic changes in skin from both CTRL and CKO mice were determined by hematoxylin and eosin (HE) staining and Masson-Fontana staining. Skin tissue specimens were fixed overnight in 4% paraformaldehyde and embedded in paraffin. Three-micrometer-thick sections were generated and then immunohistochemically stained with antibodies against UFL1 (Bethyl Laboratories, A303-456A; dilution 1:100), Endothelin-1 (Proteintech, 12191-1-AP, dilution 1:100), or Ki-67 (Abcam, ab16667, dilution 1:200). The sections were scanned using an automated slide scanner to create high-resolution digital images (KFBIO). TUNEL staining was performed with a TUNEL detection kit (Recordbio Biological Technology) according to the manufacturer’s instructions. Images were acquired with an Eclipse C1 fluorescence microscope (NIKON).
HaCaT cells were were purchased from Fenghbio (Hunan, China) and cultured in Keratinocytes-SFM (1×) (Gibco, 10725-018). Human embryonic kidney 293T (HEK293T) cells were purchased from the American Type Culture Collection and maintained in DMEM with 10% FBS (Procell, CM-0109) and 1% penicillin-streptomycin. The following three siRNAs against Ufl1 was purchased from GenePharma; Ufl1-1: GGAACUUGUUAAUAGCGGA; Ufl1-2: GAGGAGUAAUUUUUACGGA; Ufl1-3: CUGCUACCCACUUCUUUAUTT. RNA interference was performed with Lipofectamine 3000 (Invitrogen) according to the manufacturer’s instructions. The sequence of shRNA targeting homo Ufl1 mRNA (NM_015323.5) is as follow: 5′-GCACGTATCCGTGGACTATTC-3’. Cycloheximide was purchased from Selleck; Toluidine Blue Staining Kit was purchased from Sangon Biotech.
ET-1 levels in serum and ear skin of mice were measured using an ELISA kit (mmbio, MM-0561M2, China) according to the manufacturer’s instructions.
The antibodies used in this study were anti-UFL1 (Bethy Laboratories, A303-456A, dilution 1:1000), anti-UFL1 (Sigma, HPA030559, dilution 1:1000), anti-Endothelin-1 (Proteintech, 12191-1-AP, dilution 1:1000), anti-HA (Cell Signaling Technology, 3597S, dilution 1:1000), anti-Flag (Cell Signaling Technology, 3177S, dilution 1:1000), anti-UFM1 (Abcam, ab109305, dilution 1:1000) and anti-GAPDH (HuaAn Biotechnology, M1310-2, dilution 1:5000).
Total RNA was extracted from ears of mice using Trizol reagent. The RNA integrity was assessed using the RNA Nano 6000 Assay Kit of the Bioanalyzer 2100 system (Agilent Technologies, CA, United States). Total RNA was used as input material for the RNA sample preparations. RNA-Seq libraries were prepared using the NEBNext® Ultra RNA Library Prep Kit (Illumina, San Diego, CA) according to the manufacturer’s protocols. The RNA-seq libraries were sequenced using Illumina Novaseq platform and 150 bp paired-end reads were generated. FeatureCounts v1.5.0-p3 was used to count the reads numbers mapped to each gene. Differential expression analysis of twoconditions/groups (two biological replicates per condition) was performed using the DESeq2 R package (1.20.0). Genes with an adjusted p-value<0.05 found by DESeq2 were assigned as differentially expressed. RNA sequencing technology was provided by Novogene (Beijing, China).
Total RNA was extracted with TRIzol reagent (Invitrogen, United States) according to the manufacturer’s instructions; cDNA was then prepared using 5×FastKing-RTsuperMix (TIANGEN). Quantitative real-time PCR (q-PCR) assays were performed using SYBR Green Supermix (Bio-Rad) with a CFX96 Real Time-PCR Detection System (Bio-Rad). The sequences of the primers used for q-PCR are described in Supplementary Table S2. Relative expression level of each transcript was normalized to murine beta-actin and GAPDH by using the 2 ^ (-delta delta Ct) method.
The in vivo Ufmylation assay has been described previously (Zhou Y. et al, 2021). Briefly, HEK293T cells were harvested after transfected with the appropriate constructs for 36 h. Cells were lysed by boiling in buffer (150 mM Tris-HCl (pH 8.0), 5% sodium dodecyl sulfate (SDS) and 30% glycerol) for 10 min. Cell lysates were diluted 20-fold with buffer A (50 mM Tris-HCl (pH 8.0), 150 mM NaCl, 0.5% NP-40 and 2 mM N-ethylmaleimide) and protease inhibitor cocktails (Roche). After incubation with anti-Flag M2 Affinity Gel (Sigma) overnight at 4°C, the immunoprecipitates were subjected to SDS polyacrylamide gel electrophoresis (SDS-PAGE) followed by western blot analysis.
GST-tagged ET-1 were ectopically expressed in BL21 (DE3) cells and purified using Glutathione Sepharose (GE Healthcare). In vitro Ufmylation assay was performed as described previously (Liu et al., 2020).
Western blot images were acquired with an MP 5000 VersaDoc imaging system (BioRad). GraphPad Prism 8 was used for all statistical analyses (GraphPad Software). Student’s t-test was performed for all quantitative data between different groups, and the statistical significance was labeled as *p < 0.05, **p < 0.01, ***p < 0.001, n.s. no significant. Each experiment was repeated independently with similar results.
We have previously generated mice carrying Ufl1 flox alleles (Zhou Y. et al, 2021), which were crossed with keratin 14 promoter-driven Cre transgenic (KRT14Cre/+) mice to achieve skin knockout of Ufl1 (Ufl1f/f KRT14Cre/+, hereafter referred to as CKO mice) (Figure 1A). Genomic insertion of the loxP sites in the Ufl1 gene and KRT14Cre/+ were confirmed by PCR (Figure 1B), and the expression of Ufl1 in the skin of CKO mice and CTRL mice (Ufl1f/+or Ufl1f/f, hereafter referred to as CTRL mice) were analyzed by q-PCR, western blotting, and immunohistochemical staining. These analyses demonstrated that Ufl1 was specifically deleted in the skin (Figures 1C–E), while it was expressed normally in other tissues (Supplementary Figure S1A). As expected, the global levels of UFM1-conjugated (Ufmylated) proteins were reduced in skin of CKO mice (Supplementary Figure S1B). These data indicated that skin-specific Ufl1-knockout mice were successfully generated.
FIGURE 1. Generation of Ufl1f/f KRT14Cre/+ mice. (A) Targeting strategy to generate Ufl1 conditional knockout mice. Ufl1f/f mice were crossed with KRT14Cre/+ transgenic mice to obtain conditional skin knockout mice. (B) PCR-based genotyping of wild-type and floxed alleles of Ufl1 and KRT14-Cre allele. (C) q-PCR analysis of Ufl1 gene expression in the skin of 3-month-old CTRL and CKO mice (n = 6). (D) Ufl1 expression was detected by Western blot in the skin of 3-month-old CTRL (Ufl1f/+, Ufl1f/f or Ufl1f/+ KRT14Cre/+), CKO (Ufl1f/f KRT14Cre/+) mice. (E) Immunohistochemical staining (IHC) of Ufl1 proteins in sections of ear skin from CTRL and CKO. ***p < 0.001, unpaired Student’s test. Error bars represent ± s.d. The scale bar represents 100 μm.
The CKO mice were born at a Mendelian ratio without apparent morphologically different compared with the CTRL mice. The analysis of skin permeability with toluidine blue staining revealed no difference between CTRL and CKO mice in neonates (birth day 0) (Supplementary Figure S2A). Notably, at 3 months of age, CKO mice showed a significantly reduced body weight and exhibited enhanced skin pigmentation at sites with little or no hair, such as the ears, tails and footspads (Figures 2A–C). Compared to CTRL mice, CKO mice had shortened lifespan (Supplementary Figure S2B), reduced body weight and showed a hunchback with flflaky skin, sparse hair and pigmentation at 16 months of age (Supplementary Figures S2C–E). Moreover, CKO mice exhibited increased epidermis thickness as assayed by HE staining in the ear and tail at 3 months old (Figures 2D,E). To examine whether absence of epidermal Ufl1 results in defective keratinocyte proliferation or apoptosis, we analyzed the epidermis of ears in 3 months mice. Results indicate that the absence of Ufl1 did not affect apoptosis of keratinocytes (KCs) in the epidermis as revealed by TUNEL staining, while the proliferation of keratinocytes was increased in the CKO mice, especially in the basal layer and hair follicle as assessed by Ki67 staining (Supplementary Figure S2F).
FIGURE 2. Loss of Ufl1 causes severe skin abnormalities in mice. (A) Images of mice with the indicated genotypes. (B) Body weight of mice in A. (C) Paws, tails and ears of the indicated mice as shown in A. (D,E) Tails and ears sections from CTRL and CKO mice were stained with hematoxylin and eosin (H&E) and epidermal thickness. (F,G) Masson-Fontana staining of the tail and ear sections of mice in C, and melanin quantification of the indicated tail and ear samples. Arrowheads mark the dermal-epidermal border in panels. SC, stratum corneum. ***p < 0.001, unpaired Student’s test. Error bars represent ± s.d. The scale bar represents 100 μm.
The color change observed in skin of CKO mice by Masson-Fontana staining suggests that Ufl1 may involve in melanogenesis. Consistent with the darkened coloration, we observed that melanin, which was normally located at dermis in CTRL mice, appeared at both the epidermis and dermis in CKO mice, specifically in hairless regions (Figures 2F,G). In addition, we observed that most of melanin was located in the basal layer in CKO mice, but it was also detected in suprabasal keratinocytes, and the melanin-positive cells extended into the stratum corneum, where they accumulated as dead, pigmented debris (the end product of keratinocyte differentiation). Whereas, no change in the color was observed in the dermis of CKO mice. These data suggest that Ufl1 plays a critical role in melanogenesis.
To understand the characteristic features of pigmentation in CKO mice, we performed RNA sequencing analysis (RNA-seq) in the CTRL and CKO ears at 3 months of age and the results indicated that the expression of genes belonging to enzymatic components of melanin biosynthesis including tyrosinase (Tyr), tyrosinase-related protein 1 (Tyrp1), melanocyte-Specific Transporter Protein (Oca2), dopachrome tautomerase (Dct) and Solute Carrier Family 45 Member 2 (Slc45a2), was markedly upregulated in ears of CKO mice (Figure 3A). These results were further confirmed by q-PCR analysis (Figure 3B). We also examined the expression of other genes involved in melanin synthesis, such as Mlana, Pmel and Trpm1, which are considered as biomarkers for melanoma (Wang et al., 2011; Brozyna et al., 2017; Zhang et al., 2021) and the results showed that these genes were significantly upregulated as well in CKO mice (Supplementary Figure S3). Furthermore, by using RNA-seq data from The Cancer Genome Atlas (TCGA) melanoma cohort (Tang et al., 2017), we found that the mRNA level of UFL1 was negatively correlated with those of well-known targets downstream from melanin biosynthesis pathway, including OCA2 and SLC45A2 (Figures 3C,D). Taken together, these results suggest that Ufl1 plays a vital role in melanin biosynthesis in vivo.
FIGURE 3. Global transcriptome analysis of ear. (A) Volcano plot of downregulated (blue) and upregulated (red) transcripts in CKO mice skin. Genes in melanin biosynthesis differentially expressed are marked by red lines. (B) q-PCR confirmation of mRNA expression of melanin biosynthesis-related genes. (C,D) Pearson’s correlation between Ufl1 and OCA2 or SLC45A2 in The Cancer Genome Atlas (TCGA) SKCM. Plots show the Spearman’s correlation and OCA2 or SLC45A2 with UFL1 mRNA level from RNA-seq data in TCGA melanoma calculated. R and p values are shown. *p < 0.05, ***p < 0.001, unpaired Student’s test. Error bars represent ± s.d.
Melanocytes in the epidermis contribute to human skin pigmentation by synthesizing the melanin, thereby neighboring keratinocytes receive and distribute it to upper layers of the skin. Endothelin-1 (ET-1) is a keratinocyte-derived factor that is involved in regulating the proliferation of melanoblasts and melanocytes, as well as the differentiation of melanocytes. To assess whether ET-1 is differentially expressed in the CTRL and CKO mice, we performed immunofluorescent staining with anti ET-1 antibody. As shown in Figure 4A, ET-1 fluorescence intensity was markedly increased in the epidermis of CKO mice. Consistently, we found that the protein levels of ET-1 dramatically upregulated in the CKO mice without significant changes in ET-1 messenger RNA levels (Figure 4B and Supplementary Figure S4A). Similar results were observed in Ufl1-depleted HaCaT cells (Figure 4C and Supplementary Figure S4B). Conversely, overexpression of Ufl1 in HaCaT keratinocyte cells resulted in down-regulated expression of ET-1 (Supplementary Figure S4C). We then evaluated secretion of ET-1 in mice plasma and ears by ELISA and found that the level of secreted ET-1 was upregulated in CKO mice (Figures 4D,E). These results demonstrate that the loss of Ufl1 led to upregulated ET-1 expression and secretion.
FIGURE 4. Ufl1 deficiency promote the expression and secretion of Endothelin-1 (ET-1). (A) Immunofluorescence staining for mice ears sections. The images showed ET-1 staining (green), and DAPI staining (blue) as a nuclear counterstain (upper panel). (B) Western blot analysis of ET-1 expression in the ears of 3-month-old CRTL and CKO mice (n = 3). (C) Western blot analysis of ET-1 expression in the HaCaT cells with Ufl1 depletion. si-RNA, small interfering RNA; NC, negative control. (D,E) ET-1secretion in the plasma and ears was analyzed by ELISA (n = 6). *p < 0.05, ***p < 0.001, unpaired Student’s test. Error bars represent ± s.d. The scale bar represents 100 μm.
To understand how deletion of Ufl1 resulted in upregulation of ET-1, we assessed whether ET-1 is a substrate of Ufmylation modification, given that Ufl1 is the only known E3 ligase of Ufmylation. Immunoprecipitation analysis indicated that UFL1, DDRGK1 and ET-1 were capable of binding to each other (Figure 5A and Supplementary Figure S4D). Furthermore, the in vitro pull-down assay with purified recombinant proteins (GST-ET-1, UFL1 and DDRGK1) showed that ET-1 directly interacted with UFL1 and DDRGK1 (Figure 5B). To determine whether ET-1 can be Ufmylated, we co-expressed ET-1 in HEK293T cells with the Ufmylation components UBA5, UFC1, UFL1, UFM1 and DDRGK1. The Ufmylation assays showed that wild-type UFM1 (UFM1WT) and an active form of UFM1 with an exposed carboxy (C)-terminal glycine 83 residues (UFM1∆C2) could conjugate to ET-1, but not an inactive form of UFM1 lacking the C-terminal glycine 83 residues (UFM1∆C3) (Figure 5C). In addition, the in vitro Ufmylation assay further demonstrated that ET-1 is a substrate of Ufmylation modification (Figure 5D). Moreover, we examined the effect of Ufmylation on ET-1 stability by cycloheximide chase assay and found that ET-1 stability was increased after depletion of UFL1 (Figure 5E). Thus, these data suggest that Ufl1 regulates ET-1 stability through Ufl1-mediated Ufmylation modification.
FIGURE 5. Ufl1 deletion affects Endothelin-1 synthesis. (A) Western blot analysis of the mutual interactions between Ufl1, DDRGK1 and ET-1 in HEK293T cells by co-immunoprecipitation. (B) In vitro binding assays between ET-1 and Ufl1 or DDRGK1. (C) Ufmylation of ET-1 was analyzed by western blot with anti-UFM1 antibody in HEK293T cells expressing the Ufmylation system components. (D) In vitro Ufmylation assay of ET-1. Us., UFMylation system components (consisting of UBA5, UFC1, UFL1, DDRGK1 and UFM1). (E) ET-1 stability was examined by western blot in UFL1-depletion HaCaT cells (sh-UFL1) compared to control HaCaT cells (sh-NC). The cells were treated with 100 µg ml−1 cycloheximide (CHX) for the indicated times. The graph represents quantification of the ET-1 protein levels.
In the present study, we showed that ablation of Ufl1 caused epidermal thickening, pigmentation and reduced life-span of animals. Our RNA-seq analysis indicated that the expression of genes of enzymatic components of melanin biosynthesis were significantly upregulated in CKO mice. We further identified ET-1 as a novel substrate of Ufmylation and showed that Ufl1 is involved in skin pigmentation by regulating ET-1 expression and secretion through Ufmylation modification.
Among the constituent cells present in the skin, KCs secrete a mass of mitogenic or melanogenesis factors that are recognized by their corresponding receptors on melanocytes. These KCs-derived cytokines or growth factors play essential roles in proliferation, differentiation and melanogenesis (Slominski et al., 2004). ET-1 releases from keratinocytes and binds to EDNRs on melanocytes, thereby transmitting signaling cascade to participate in the melanogenesis process. The hyperpigmentation observed in basal cell carcinoma (BCC) and seborrheic keratosis (SK) is associated with enhanced expression of keratinocyte-derived ET-1 (Teraki et al., 1996; Lan et al., 2005). Consistently, we found that pigmentation in CKO mice is associated with up-regulation of ET-1. It is known that increased ET-1 not only can cause hyperpigmentation, but also associated with atopic dermatitis (Aktar et al., 2015; Nakahara et al., 2018) and psoriasis (Bonifati et al., 1998), the well-known chronic pruritic dermatosis. Serum ET-1 levels have been reported to be elevated in patients with atopic dermatitis and psoriasis and correlate with disease severity. Interestingly, our RNA-seq analysis indicates that inflammation-related signaling pathways were upregulated in CKO mice, suggesting that Ufl1-deficient mice also have an inflammatory response (Supplementary Figure S5).
It is worth to mention that the KRT14-Cre is also expressed in dental epithelium and this may affect dental development. Indeed, we found that CKO mice exhibited tooth morphological abnormalities (including breaking, twisting, and lengthening). Thus, it is possible that reduced body weight observed in CKO mice may be due to their feeding difficulty because of dental abnormalities.
In summary, our study revealed the critical role of Ufl1 in skin pigmentation, which may provide insights for a better understanding of the mechanisms of pigmentary disorders and for therapeutic strategies.
The datasets presented in this study can be found in online repositories. The names of the repository/repositories and accession number(s) can be found in the article/Supplementary Material.
The animal study was reviewed and approved by the Animal Care and Use Committee of Hangzhou Normal University.
KW: investigation, validation, writing—Original draft. HX: Investigation, validation. Y-WW: investigation. JM: investigation. DL: investigation, validation. XZ: methodology: methodology. Y-SC and MW: investigation, conceptualization, funding acquisition, project administration, supervision, writing-review and editing.
This work was supported by grants from the National Natural Science Foundation of China (31730020), and the Hangzhou Science and Technology Bureau (20182014B01).
We thank all members of the Cong lab for their helpful discussion and comments.
The authors declare that the research was conducted in the absence of any commercial or financial relationships that could be construed as a potential conflict of interest.
All claims expressed in this article are solely those of the authors and do not necessarily represent those of their affiliated organizations, or those of the publisher, the editors and the reviewers. Any product that may be evaluated in this article, or claim that may be made by its manufacturer, is not guaranteed or endorsed by the publisher.
The Supplementary Material for this article can be found online at: https://www.frontiersin.org/articles/10.3389/fcell.2022.961675/full#supplementary-material
Aktar, M. K., Kido-Nakahara, M., Furue, M., and Nakahara, T. (2015). Mutual upregulation of endothelin-1 and IL-25 in atopic dermatitis. Allergy 70 (7), 846–854. doi:10.1111/all.12633
Azfer, A., Niu, J., Rogers, L. M., Adamski, F. M., and Kolattukudy, P. E. (2006). Activation of endoplasmic reticulum stress response during the development of ischemic heart disease. Am. J. Physiol. Heart Circ. Physiol. 291 (3), H1411–H1420. doi:10.1152/ajpheart.01378.2005
Bonifati, C., Mussi, A., Carducci, M., Pittarello, A., D'Auria, L., Venuti, A., et al. (1998). Endothelin-1 levels are increased in sera and lesional skin extracts of psoriatic patients and correlate with disease severity. Acta Derm. Venereol. 78 (1), 22–26. doi:10.1080/00015559850135779
Brozyna, A. A., Guo, H., Yang, S. E., Cornelius, L., Linette, G., Murphy, M., et al. (2017). TRPM1 (melastatin) expression is an independent predictor of overall survival in clinical AJCC stage I and II melanoma patients. J. Cutan. Pathol. 44 (4), 328–337. doi:10.1111/cup.12872
Cai, Y., Zhu, G., Liu, S., Pan, Z., Quintero, M., Poole, C. J., et al. (2019). Indispensable role of the Ubiquitin-fold modifier 1-specific E3 ligase in maintaining intestinal homeostasis and controlling gut inflammation. Cell Discov. 5, 7. doi:10.1038/s41421-018-0070-x
Deyrieux, A. F., Rosas-Acosta, G., Ozbun, M. A., and Wilson, V. G. (2007). Sumoylation dynamics during keratinocyte differentiation. J. Cell Sci. 120 (1), 125–136. doi:10.1242/jcs.03317
Grice, E. A., and Segre, J. A. (2011). The skin microbiome. Nat. Rev. Microbiol. 9 (4), 244–253. doi:10.1038/nrmicro2537
Hara, M., Yaar, M., and Gilchrest, B. A. (1995). Endothelin-1 of keratinocyte origin is a mediator of melanocyte dendricity. J. Invest. Dermatol. 105 (6), 744–748. doi:10.1111/1523-1747.ep12325522
Hochstrasser, M. (2009). Origin and function of ubiquitin-like proteins. Nature 458 (7237), 422–429. doi:10.1038/nature07958
Hu, X., Pang, Q., Shen, Q., Liu, H., He, J., Wang, J., et al. (2014). Ubiquitin-fold modifier 1 inhibits apoptosis by suppressing the endoplasmic reticulum stress response in Raw264.7 cells. Int. J. Mol. Med. 33 (6), 1539–1546. doi:10.3892/ijmm.2014.1728
Imokawa, G., Miyagishi, M., and Yada, Y. (1995). Endothelin-1 as a new melanogen: Coordinated expression of its gene and the tyrosinase gene in UVB-exposed human epidermis. J. Invest. Dermatol. 105 (1), 32–37. doi:10.1111/1523-1747.ep12312500
Imokawa, G., Yada, Y., and Miyagishi, M. (1992). Endothelins secreted from human keratinocytes are intrinsic mitogens for human melanocytes. J. Biol. Chem. 267 (34), 24675–24680. doi:10.1016/s0021-9258(18)35817-4
Komatsu, M., Chiba, T., Tatsumi, K., Iemura, S., Tanida, I., Okazaki, N., et al. (2004). A novel protein-conjugating system for Ufm1, a ubiquitin-fold modifier. EMBO J. 23 (9), 1977–1986. doi:10.1038/sj.emboj.7600205
Lan, C. C., Wu, C. S., Cheng, C. M., Yu, C. L., Chen, G. S., and Yu, H. S. (2005). Pigmentation in basal cell carcinoma involves enhanced endothelin-1 expression. Exp. Dermatol. 14 (7), 528–534. doi:10.1111/j.0906-6705.2005.00320.x
Li, J., Yue, G., Ma, W., Zhang, A., Zou, J., Cai, Y., et al. (2018). Ufm1-Specific ligase Ufl1 regulates endoplasmic reticulum homeostasis and protects against heart failure. Circ. Heart Fail. 11 (10), e004917. doi:10.1161/CIRCHEARTFAILURE.118.004917
Liu, J., Guan, D., Dong, M., Yang, J., Wei, H., Liang, Q., et al. (2020). UFMylation maintains tumour suppressor p53 stability by antagonizing its ubiquitination. Nat. Cell Biol. 22 (9), 1056–1063. doi:10.1038/s41556-020-0559-z
Malik, M. N. H., Waqas, S. F., Zeitvogel, J., Cheng, J., Geffers, R., Gouda, Z. A., et al. (2022). Congenital deficiency reveals critical role of ISG15 in skin homeostasis. J. Clin. Invest. 132 (3), e141573. doi:10.1172/JCI141573
Nakahara, T., Kido-Nakahara, M., Ohno, F., Ulzii, D., Chiba, T., Tsuji, G., et al. (2018). The pruritogenic mediator endothelin-1 shifts the dendritic cell-T-cell response toward Th17/Th1 polarization. Allergy 73 (2), 511–515. doi:10.1111/all.13322
Rubio, M. D., Wood, K., Haroutunian, V., and Meador-Woodruff, J. H. (2013). Dysfunction of the ubiquitin proteasome and ubiquitin-like systems in schizophrenia. Neuropsychopharmacology 38 (10), 1910–1920. doi:10.1038/npp.2013.84
Slominski, A., Tobin, D. J., Shibahara, S., and Wortsman, J. (2004). Melanin pigmentation in mammalian skin and its hormonal regulation. Physiol. Rev. 84 (4), 1155–1228. doi:10.1152/physrev.00044.2003
Tang, Z., Li, C., Kang, B., Gao, G., Li, C., and Zhang, Z. (2017). Gepia: A web server for cancer and normal gene expression profiling and interactive analyses. Nucleic Acids Res. 45 (W1), W98–W102. doi:10.1093/nar/gkx247
Tatsumi, K., Sou, Y. S., Tada, N., Nakamura, E., Iemura, S., Natsume, T., et al. (2010). A novel type of E3 ligase for the Ufm1 conjugation system. J. Biol. Chem. 285 (8), 5417–5427. doi:10.1074/jbc.M109.036814
Teraki, E., Tajima, S., Manaka, I., Kawashima, M., Miyagishi, M., and Imokawa, G. (1996). Role of endothelin-1 in hyperpigmentation in seborrhoeic keratosis. Br. J. Dermatol. 135 (6), 918–923. doi:10.1046/j.1365-2133.1996.d01-1095.x
Themelis, G. T., Trellopoulos, A. V., Tzanavaras, P. D., and Karlberg, B. (2005). Assay of the synthetic estrogen fosfestrol in pharmaceutical formulations using capillary electrophoresis. J. Pharm. Biomed. Anal. 39 (3-4), 559–563. doi:10.1016/j.jpba.2005.05.007
Wang, J., Jia, R., Yao, Y., Cun, B., Huang, X., Gu, P., et al. (2011). Differential expression of Mart-1 in human uveal melanoma cells. Mol. Med. Rep. 4 (5), 799–803. doi:10.3892/mmr.2011.504
Weiner, L., Han, R., Scicchitano, B. M., Li, J., Hasegawa, K., Grossi, M., et al. (2007). Dedicated epithelial recipient cells determine pigmentation patterns. Cell 130 (5), 932–942. doi:10.1016/j.cell.2007.07.024
Wolk, K., Witte, K., Witte, E., Raftery, M., Kokolakis, G., Philipp, S., et al. (2013). IL-29 is produced by T(H)17 cells and mediates the cutaneous antiviral competence in psoriasis. Sci. Transl. Med. 5, 204ra129. 204ra129. doi:10.1126/scitranslmed.3006245
Yada, Y., Higuchi, K., and Imokawa, G. (1991). Effects of endothelins on signal transduction and proliferation in human melanocytes. J. Biol. Chem. 266 (27), 18352–18357. doi:10.1016/s0021-9258(18)55277-7
Yang, A., Ha, S., Ahn, J., Kim, R., Kim, S., Lee, Y., et al. (2016). A chemical biology route to site-specific authentic protein modifications. Science 354 (6312), 623–626. doi:10.1126/science.aah4428
Yohn, J. J., Morelli, J. G., Walchak, S. J., Rundell, K. B., Norris, D. A., and Zamora, M. R. (1993). Cultured human keratinocytes synthesize and secrete endothelin-1. J. Invest. Dermatol. 100 (1), 23–26. doi:10.1111/1523-1747.ep12349932
Yoo, H. M., Kang, S. H., Kim, J. Y., Lee, J. E., Seong, M. W., Lee, S. W., et al. (2014). Modification of ASC1 by UFM1 is crucial for ERα transactivation and breast cancer development. Mol. Cell 56 (2), 261–274. doi:10.1016/j.molcel.2014.08.007
Zhang, M., Zhu, X., Zhang, Y., Cai, Y., Chen, J., Sivaprakasam, S., et al. (2015). RCAD/Ufl1, a Ufm1 E3 ligase, is essential for hematopoietic stem cell function and murine hematopoiesis. Cell Death Differ. 22 (12), 1922–1934. doi:10.1038/cdd.2015.51
Zhang, S., Chen, K., Liu, H., Jing, C., Zhang, X., Qu, C., et al. (2021). PMEL as a prognostic biomarker and negatively associated with immune infiltration in skin cutaneous melanoma (SKCM). J. Immunother. 44 (6), 214–223. doi:10.1097/CJI.0000000000000374
Zheng, N., and Shabek, N. (2017). Ubiquitin ligases: Structure, function, and regulation. Annu. Rev. Biochem. 86, 129–157. doi:10.1146/annurev-biochem-060815-014922
Zhou, J., Ma, X., Xu, L., Liang, Q., Mao, J., Liu, J., et al. (2021a). Genomic profiling of the UFMylation family genes identifies UFSP2 as a potential tumour suppressor in colon cancer. Clin. Transl. Med. 11 (12), e642. doi:10.1002/ctm2.642
Zhou, M., Lin, F., Xu, W., Jin, R., and Xu, A. (2018). Decreased SUMOylation of the retinoblastoma protein in keratinocytes during the pathogenesis of vitiligo. Mol. Med. Rep. 18 (3), 3469–3475. doi:10.3892/mmr.2018.9299
Zhou, X., Tan, M., Nyati, M. K., Zhao, Y., Wang, G., and Sun, Y. (2016). Blockage of neddylation modification stimulates tumor sphere formation in vitro and stem cell differentiation and wound healing in vivo. Proc. Natl. Acad. Sci. U. S. A. 113 (21), E2935–E2944. doi:10.1073/pnas.1522367113
Keywords: Ufmylation modification, Ufl1, Ufl1f/f KRT14Cre/+, pigmentation, endothelin-1
Citation: Wang K, Xu H-N, Wang Y-W, Mao J, Liu D, Zhu X-J, Cong Y-S and Wang M (2022) Ufl1 deficiency causes skin pigmentation by up-regulation of Endothelin-1. Front. Cell Dev. Biol. 10:961675. doi: 10.3389/fcell.2022.961675
Received: 05 June 2022; Accepted: 10 August 2022;
Published: 02 September 2022.
Edited by:
Geng Tian, Binzhou Medical University, ChinaReviewed by:
Yohan Oh, Hanyang University, South KoreaCopyright © 2022 Wang, Xu, Wang, Mao, Liu, Zhu, Cong and Wang. This is an open-access article distributed under the terms of the Creative Commons Attribution License (CC BY). The use, distribution or reproduction in other forums is permitted, provided the original author(s) and the copyright owner(s) are credited and that the original publication in this journal is cited, in accordance with accepted academic practice. No use, distribution or reproduction is permitted which does not comply with these terms.
*Correspondence: Yu-Sheng Cong, eXNjb25nQGh6bnUuZWR1LmNu; Miao Wang, bXdhbmdoem51QDEyNi5jb20=
Disclaimer: All claims expressed in this article are solely those of the authors and do not necessarily represent those of their affiliated organizations, or those of the publisher, the editors and the reviewers. Any product that may be evaluated in this article or claim that may be made by its manufacturer is not guaranteed or endorsed by the publisher.
Research integrity at Frontiers
Learn more about the work of our research integrity team to safeguard the quality of each article we publish.