- 1Department of Obstetrics, Shenzhen Maternity and Child Healthcare Hospital, The First School of Clinical Medicine, Southern Medical University, Shenzhen, China
- 2Center for Energy Metabolism and Reproduction, Shenzhen Institute of Advanced Technology, Chinese Academy of Sciences, Shenzhen, China
- 3Shenzhen Institute of Advanced Technology, Chinese Academy of Sciences, Shenzhen, China
- 4Shenzhen Key Laboratory of Metabolic Health, Shenzhen, China
Preeclampsia, a clinical syndrome mainly characterized by hypertension and proteinuria, with a worldwide incidence of 3–8% and high maternal mortality, is a risk factor highly associated with maternal and offspring cardiovascular disease. However, the etiology and pathogenesis of preeclampsia are complicated and have not been fully elucidated. Obesity, immunological diseases and endocrine metabolic diseases are high-risk factors for the development of preeclampsia. Effective methods to treat preeclampsia are lacking, and termination of pregnancy remains the only curative treatment for preeclampsia. The pathogenesis of preeclampsia include poor placentation, uteroplacental malperfusion, oxidative stress, endoplasmic reticulum stress, dysregulated immune tolerance, vascular inflammation and endothelial cell dysfunction. The notion that placenta is the core factor in the pathogenesis of preeclampsia is still prevailing. G protein-coupled receptors, the largest family of membrane proteins in eukaryotes and the largest drug target family to date, exhibit diversity in structure and function. Among them, the secretin/adhesion (Class B) G protein-coupled receptors are essential drug targets for human diseases, such as endocrine diseases and cardiometabolic diseases. Given the great value of the secretin/adhesion (Class B) G protein-coupled receptors in the regulation of cardiovascular system function and the drug target exploration, we summarize the role of these receptors in placental development and preeclampsia, and outlined the relevant pathological mechanisms, thereby providing potential drug targets for preeclampsia treatment.
Introduction
Preeclampsia (PE) is a multisystem pregnancy disorder characterized by new-onset hypertension after 20 weeks of gestation and affects the functions of multiple organ (Brown et al., 2018; Chappell et al., 2021). PE influences around 3–8% of the pregnant women and remains a key cause of maternal mortality, bringing about at least 42,000 maternal deaths each year (Abalos et al., 2013; Say et al., 2014). PE seriously threatens maternal and fetal life safety, leading to many severe complications such as acute renal failure, intracranial hemorrage, fetal growth restriction (FGR), abnormal fetal heart development and still birth (Adu-Bonsaffoh et al., 2013; Brown et al., 2018; Hutcheon et al., 2011). Furthermore, PE can bring substantial long-term cardiovascular and endocrine metabolic risks both to the mother and the child (Bellamy et al., 2007; Kajantie et al., 2009; Mongraw-Chaffin et al., 2010; Tuovinen et al., 2012). The strong evidence has demonstrated that aspirin can prevent the development of PE (Duley et al., 2019; Jin, 2021). However, once PE occurs, the existing treatments, such as antispasmodic and antihypertensive agents, cannot prevent the progression of the disease. The termination pf pregnancy is the only effective treatment for PE, which may leads to the delivery of premature fetus or low birth weight fetus, with high healthcare costs (Chappell et al., 2021; Liu et al., 2009). Thus, it is necessary to explore new treatments for PE to reduce the risk and safely prolong pregnancy.
The pathophysiological mechanisms of PE have been studied for a long time. The placenta is the key factor responsible for the development of PE. All maternal complications share a common pathophysiological feature focusing on placental abnormalities (Burton et al., 2019). The pathogenesis of PE contains poor placentation, uteroplacental malperfusion and endothelial cell dysfunction (Brennan et al., 2014; Redman and Staff, 2015). However, due to the heterogeneity of PE and the diversity of its clinical manifestations, the immunological, genetic and environmental mechanisms of PE are still not fully understood, and there is no great breakthrough regarding the treatment for PE. Some research scholars advocate that PE should be reclassified using placenta-derived markers or new phenotypic combinations, which may assist in identifying high-risk patients, monitoring disease progression, and providing effective clinical interventions (Ferrazzi et al., 2018; Powers et al., 2012).
G protein-coupled receptors (GPCRs), the largest family of membrane proteins in eukaryotes, are involved in the regulation of almost all life processes and functions. GPCRs are the key factors for the occurrence and development of major diseases, including cardiometabolic diseases (Dorsam and Gutkind, 2007; Lappano and Maggiolini, 2011; Wang et al., 2018). Exploratory studies have revealed the essential functions of GPCRs in placental development and provided a sufficient theoretical basis that they can be used as potential targets for PE (Conrad, 2016; McGuane and Conrad, 2012; Quitterer and AbdAlla, 2021). Based on the classification by structure and phylogeny analysis of GPCRs, Class B GPCRs, which are structurally characterized by large extracellular regions, contain the following two families, secretin GPCRs and adhesion GPCRs. Secretin GPCRs are polypeptide hormone receptors that can mediate diverse physiological activities. Adhesion GPCRs are indispensable for human development, and their mutations are involved in all major tissues diseases. This review summarizes the pathophysiological mechanisms of PE and the biological role of secretin/adhesion (Class B) GPCRs in placental development and PE, aiming to find potential markers for the reclassification and treatment of PE.
Pathophysiology of PE: the two-stage placental model
The clinical symptoms of PE are immediately relieved once the placenta is delivered, suggesting that the placenta is crucial for the pathogenesis of PE. The two-stage placental model theory proposed by professor Redman is the most acceptable explanation for the pathogenesis of PE (Redman, 1991). In the first stage (preclinical and placental period), insufficient trophoblast infiltration causes the incomplete remodeling of the uterine spiral arteries, resulting in poor placentation and placental dysfunction. In the second stage (clinical and maternal disease period), placental ischemia, hypoxia and oxidative stress lead to the release of numerous inflammatory factors into the circulation, including soluble fms-like tyrosine kinase-1 (sFlt-1), soluble endoglin (sEng), trophoblast debris and reactive oxygen species (Levine et al., 2004; Maynard et al., 2003). These factors cause systemic vascular inflammation and extensive maternal endothelial cell dysfunction (Redman et al., 1999) and provoke diverse clinical manifestations such as maternal hypertension, proteinuria and FGR (Powe et al., 2011). This two-stage model is established on the assumption that poor placentation, predominantly leading to FGR, occurs in almost all PE cases (Avagliano et al., 2011). However, some of the late-onset PE patients with full delivery show unlimited neonatal growth, which suggests that poor placentation does not happens in all PE placenta (Xiong et al., 2002). The second placental cause of PE is uteroplacental malperfusion. The placenta capacity over the capacity of the uterus could compress the terminal villi and hinder intervillous perfusion, resulting in syncytiotrophoblast hypoxia and the subsequent placental dysfunction (Devisme et al., 2013). Increased syncytiotrophoblast apoptosis in the human full-term placenta may cause PE as well (Ishihara et al., 2002). Studies have shown that the angiogenesis-related factors secreted by syncytiotrophoblasts are expected to be circulating biomarkers for the diagnosis and prediction of PE (Droge et al., 2021). Excessive trophoblastic senescence increases placental cell stress, which may be a potential pathogenic factor for PE (Zaki et al., 2003; Zhang et al., 2021). Overall, syncytiotrophoblast stress is a common end point of both early-onset and late-onset PE pathways and is affected by maternal genetic, epigenetic and environmental factors. Syncytiotrophoblast stress signaling in the maternal circulation may be the most specific biomarker of PE (Redman et al., 2022). The two-stage model summarizes the pathophysiological mechanisms of PE into two stages (placental dysfunction and clinical manifestations) and three ways (poor placentation, dysfunctional uteroplacental perfusion and placental aging) (Figure 1). In addition, it incorporates a range of pathophysiological mechanisms, including dysregulated immune tolerance, vascular inflammation, endoplasmic reticulum stress and oxidative stress (Sheppard and Bonnar, 1976). Maternal and pregnancy risk factors, such as primiparity, obesity and chronic prepregnancy disorders, are also considered (Alnaes-Katjavivi et al., 2016; Egeland et al., 2016; Skjaerven et al., 2002; Tannetta et al., 2015).
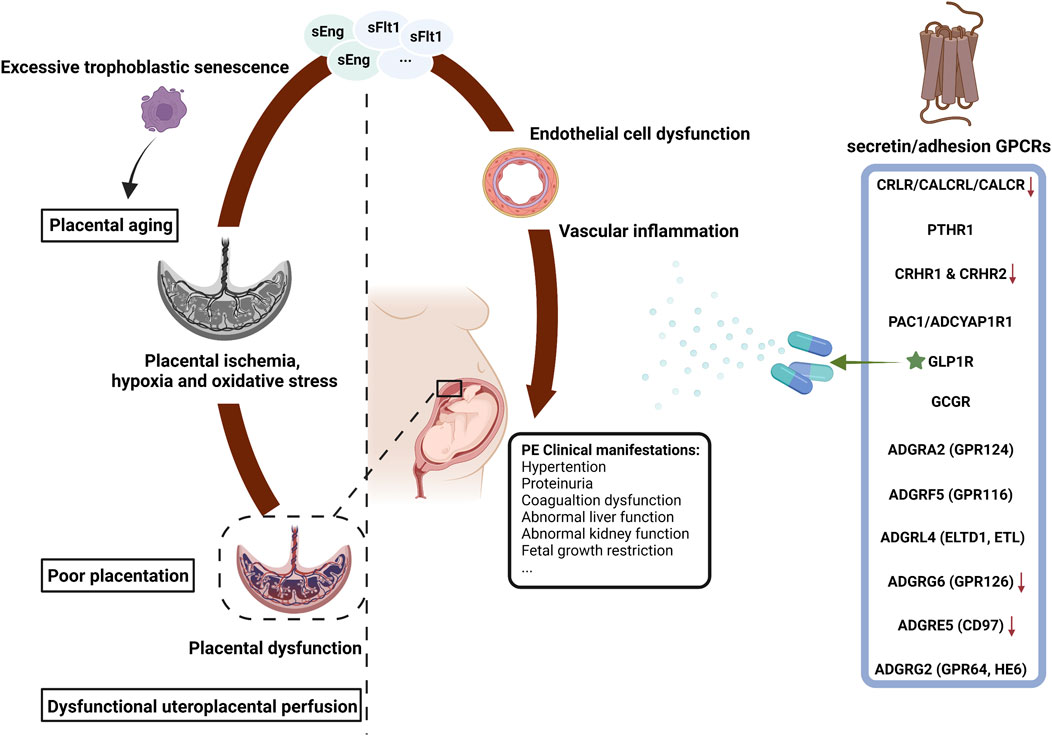
FIGURE 1. The secretin/adhesion GPCRs and the pathophysiology of preeclampsia. PE, preeclampsia; GPCRs, G protein-coupled receptors; sFlt-1, soluble fms-like tyrosine kinase 1; sEng, soluble endoglin; FGR, fetal growth restriction; ★, commercial drug; ↓, expressed at low levels in PE. The figure was created with BioRender.com.
Role of GPCRs in PE
GPCRs, a superfamily of seven-transmembrane receptors, more than 800 of which are encoded in the human genome, constitute the largest family of cell surface receptors in mammalian cells. GPCRs were initially divided into A–F systems according to the structural similarity of their receptor size, ligand interaction points and phylogeny. Their prototype members are as follows: Class A (rhodopsin receptors), Class B (secretin/adhesion receptors), Class C (metabotropic glutamate receptors), Class D (fungal mating pheromone receptors), Class E (cyclic AMP receptors) and Class F (frizzled/smoothened receptors) (Attwood and Findlay, 1994; Bockaert and Pin, 1999). Fredriksson et al. provided a GRAFS classification approach based on an overall phylogenetic analysis of human tracks that consists of the following five families: rhodopsin (Class A), secretin/adhesion (Class B), glutamate (Class C), frizzled (Class F) and taste 2 (Class T) (Fredriksson et al., 2003). GPCRs can recognize various different ligands or stimuli, such as hormones, neurotransmitters and light, to regulate key physiological processes. Abnormal GPCR signaling can lead to various diseases, such as diabetes (Riddy et al., 2018; Wu et al., 2021), cardiovascular diseases (Wang et al., 2018) and cancers (Dorsam and Gutkind, 2007; Lappano and Maggiolini, 2011). More than 40% of commercial drugs exert their efficacy through GPCRs. Most drugs target rhodopsin GPCRs because they have various ligands, but other GPCRs also possess distinct therapeutic potential, such as more than 34 drugs targeting secretin GPCRs and 21 drugs targeting glutamate GPCRs (Hutchings, 2020). Furthermore, research on drugs targeting GPCRs are still increasing (Zhou and Wild, 2019).
The GPCRs-targeted drugs such as GLP-1, which belongs to secretin GPCRs, have been used for the treament of cardiovascular diseases and endocrine diseases. The GPCR-mediated regulation of vascular tone and circulating blood volume plays crucial roles in the maintenance of blood pressure homeostasis. PE is inextricably linked to endocrine and cardiovascular diseases. Recently, it’s found GPCRs potentially contribute to maternal physiological adaptation to pregnancy and placental development. Several GPCRs such as calcitonin receptor-like receptor (CRLR) and angiotensin AT1/2 are potential therapeutic targets for PE. They can modulate systemic and/or uteroplacental vasodilation to alleviate hypertension in PE (Dong et al., 2006). Secretin and adhesion GPCRs, which belong to the class B GPCR family, present great potential in clinical use. Many studies are committed to investigate the fundamental role of Class B (secretin/adhesion) GPCRs in PE, so it is necessary to summarize the current research progress. A comprehensive summary of the researches in relation to Class B (secretin/adhesion) GPCRs in the field of placental development and PE is provided in the following part of this review (Table 1).
Secretin (Class B) GPCRs
The secretin family, a small part of GPCRs containing 15 members, has large extracellular domains that can bind to hormone and mainly regulates metabolism (Lagerstrom and Schioth, 2008).
Calcitonin receptor-like receptor (CRLR) is required for embryonic development (Chang and Hsu, 2013). Deficiency of CRLR causes extreme hydrops fetalis and embryonic death (Dackor et al., 2006; Mackie et al., 2018). CRLR is widely expressed in vascular endothelial cells of placental chorionic villi at the first trimester, human choriocarcinoma JAr cells and trophoblast HTR-8/svneo cells (Tsatsaris et al., 2002). It shows a spatiotemporal pattern in the female reproductive system of pregnant rats (Li et al., 2010). An analysis of mouse placenta obtained on E18 of pregnancy suggests that CRLR is predominantly expressed in trophoblast, syncytiotrophoblast and trophoblast giant cells (Chang and Hsu, 2013; Dong et al., 2003; Zhang et al., 2005). CRLR regulates trophoblast proliferation and differentiation in the implantation process (Tsatsaris et al., 2002). Existing research demonstrates that CRLR is decreasingly expressed at the uterus and umbilical artery tissues in pregnancy-induced hypertension patients (Makino et al., 2001) and at PE placenta (Dong et al., 2005). It also associates with PE in vascular remodeling (Chang and Hsu, 2013). The impairment of CRLR associated with calcitonin gene-related peptide (CGRP)-dependent vasodilation in PE (Dong et al., 2005) suggest their role in the control of human fetoplacental vascular tone. CGPR-mediated vascular dilation involves the activation of KATP channels, cAMP and nitric oxide pathway (Dong et al., 2004). The loss of CRLR associated receptor activity-modifying proteins (RAMPs) reduces the expression of parathyroid hormone 1 receptor (PTHR1) (Kadmiel et al., 2017). PTHR1 expresses differently depending on maternal hyperglycemia type. It’s highly expressed in the extravillous cytotrophoblasts and decidua tissues, regulating the human trophoblast-derived JEG-3 cell proliferation (Hellman et al., 1993), and committing to adverse pregnancy outcomes (Sirico et al., 2021).
Corticotropin releasing hormone (CRH) promotes embryo implantation (Makrigiannakis et al., 2001). It also control trophoblast invasion by downregulating the synthesis of carcinoembryonic antigen-related cell adhesion molecule 1 (CEACAM1) in extravillous trophoblast (EVT) cells (Bamberger et al., 2006). Abnormalities in trophoblast invasion may lead to abnormal placentation. The treatment of BeWo cells with exogenous CRH results in the elevation of cellular corticotropin releasing hormone receptor 1 (CRHR1) levels, which are significantly reduced in PE and intrauterine growth restriction (IUGR) (Chen et al., 2013; Karteris et al., 2003). CRHR1 and CRHR2 are expressed in placental trophoblasts, and they regulate estradiol and progesterone production as well as glucose transporters through distinct signaling pathways. They exert differential effects on 15 hydroxy prostaglandin dehydrogenase (PGDH) expression (Gao et al., 2007; Gao et al., 2008; Gao et al., 2012a; Gao et al., 2012b; Karteris et al., 1998). The deficiency of CRHR1 in uterine at early pregnancy implicates the pathogenesis of recurrent miscarriage, placenta accreta and PE (Kalantaridou et al., 2007).
The absence of the glucagon receptor (GCGR) gene during pregnancy leads to abnormal placentation and poor fetal growth, increasing occurrence rate of fetal and early postnatal death. The placenta affected by GCGR are characterized by extensive mineralization, fibrinoid necrosis, narrowing of the vascular channels and thickened interstitium associated with trophoblast hyperplasia. In addition, the lack of GCGR downregulates genes that control growth, vascularization and oxidative stress (Charron and Vuguin, 2015; Ouhilal et al., 2012). Regarding glucagon-like peptide receptors (GLPRs, including GLP1R and GLP2R), it’s found that the GLP1R agonist liraglutide can increase nitric oxide production and decrease blood pressures. They function partially through activating nitric oxide synthase (NOS) and thus serve as a potential therapeutic option for PE (Younes et al., 2020).
Pituitary adenylate cyclase activating polypeptide receptor 1 (PAC1), expressed in both human and mouse placenta, has spatiotemporal expression characteristics in decidual cells, chorionic vessels and stromal cells (Koh et al., 2003; Koh et al., 2005; Scaldaferri et al., 2000). Its antagonist PACAP6-38 can activate MAPK signaling in human cytotrophoblasts, which suggests the possible role in gestational maintenance and fetal growth (Reglodi et al., 2008). Drugs designed on the basis of secretin GPCR have been developed and applied for clinical use, especially in the treatment of metabolic diseases, such as diabetes. Given diabetes and obesity are risk factors for PE, the prospect of secretin GPCRs in therapy of PE could be speculated.
Adhesion (Class B) GPCRs
The adhesion family of GPCRs, a large branch with 33 members, is the second largest family of GPCRs separated from secretin GPCRs. The International Union of Basic and Clinical Pharmacology (IUPHAR) rename adhesion GPCRs as ADGRs followed a letter and a number to denote their subfamily and subtype, respectively (Hamann et al., 2015). Adhesion GPCRs are paid close attention due to its specific biological function and structure. Most adhesion GPCRs have long diverse N termini, and their N termini are rich in functional domains that can be found in other proteins, such as cadherins, lectins and immunoglobulins. It’s shown that the number and structure of these domains are essential for the specificity of receptor ligand binding interactions (Bjarnadottir et al., 2004; Purcell and Hall, 2018).
Several adhesion GPCRs participate in angiogenesis, a process that implicates in gestational physiology, placental development and the occurrence of PE (Masiero et al., 2013; Stehlik et al., 2004; Vallon and Essler, 2006; Wang et al., 2005). ADGRA2 (GPR124) deficiency leads to embryonic lethality due to the central nervous system (CNS)- specific angiogenesis and hemorrhage (Anderson et al., 2011; Chang et al., 2017; Cullen et al., 2011; Kuhnert et al., 2010). The loss of ADGRF5 (GPR116) and ADGRL4 (ELTD1, ETL) result in vascular remodeling defects (Lu et al., 2017). Both ADGRA2 (GPR124) and ADGRG6 (GPR126) are required for embryonic development (Chang et al., 2017; Waller-Evans et al., 2010). ADGRG6 (GPR126) has a strictly regulated expression pattern in mouse development (Moriguchi et al., 2004). A differential expression of ADGRG6 (GPR126) is found in IUGR placenta, which is correlated with placental angiogenesis (Majewska et al., 2019) and hypoxia in early pregnancy (Bogias et al., 2022). ADGRG6 (GPR126) mutant placenta shows a decreased expression of proteases associated with trophoblast invasion and maternal uterine vascular remodeling, leading to IUGR, PE and early miscarriage. Hence, ADGRG6 (GPR126) is essential in the trophoblast lineage for the promotion of spiral artery remodeling during placental development (Torregrosa-Carrion et al., 2021). Our research group previously analyzes the gene expression profiles at placentas in early-onset PE and late-onset PE. ADGRA2 (GPR124) is downregulated in early-onset PE and involve in cell surface receptor-related signaling (Liang et al., 2016). The specific role and mechanism of ADGRA2 (GPR124) in placental development and PE are still under investigation. ADGRE5 (CD97) is downregulated in PE placenta and promotes trophoblast invasion by targeting FOXC2 through PI3K/Akt/mTOR signaling pathway (Shen et al., 2020). ADGRG2 (GPR64, HE6) plays a crucial role in the decidualization of endometrial stromal cells (Yoo et al., 2017). Adhesion GPCRs are considered as suitable targets for therapy, but the ligands of most members have not been found. And there are no associated drugs are currently approved by the FDA.
Conclusion and perspectives
In summary, the Class B (secretin/adhesion) GPCRs play crucial roles in placental development and PE, suggesting that the class B (secretin/adhesion) GPCRs could serve as therapeutic targets in PE. However, most of the studies mainly focus on their expression level and associated phenotype, whereas the molecular mechanisms still need to be paid much more attention. Deep comprehension on the mechanisms is required to provide solid rationale for the application of Class B (secretin/adhesion) GPCR-targeted drugs into PE.
Author contributions
JZ and JN conceived the manuscript. AY, JZ and JN wrote and revised the manuscript. XG prepared the figure and the table. All authors contributed to the article and approved the submitted version.
Funding
This work was supported by the National Natural Science Foundation of China (81830041 and 82001521), Guangdong Basic and Applied Basic Research Foundation (2019A1515110340), Shenzhen Science and Technology Program (JCYJ20190812170005666) and Shenzhen Key Laboratory of Metabolic Health (Grant No. ZDSYS20210427152400001).
Conflict of interest
The authors declare that the research was conducted in the absence of any commercial or financial relationships that could be construed as a potential conflict of interest.
Publisher’s note
All claims expressed in this article are solely those of the authors and do not necessarily represent those of their affiliated organizations or those of the publisher, the editors and the reviewers. Any product that may be evaluated in this article or claim that may be made by its manufacturer is not guaranteed or endorsed by the publisher.
References
Abalos, E., Cuesta, C., Grosso, A. L., Chou, D., and Say, L. (2013). Global and regional estimates of preeclampsia and eclampsia: A systematic review. Eur. J. Obstetrics Gynecol. Reproductive Biol. 170 (1), 1–7. doi:10.1016/j.ejogrb.2013.05.005
Adu-Bonsaffoh, K., Oppong, S. A., Binlinla, G., and Obed, S. A. (2013). Maternal deaths attributable to hypertensive disorders in a tertiary hospital in Ghana. Int. J. Gynecol. Obstetrics 123 (2), 110–113. doi:10.1016/j.ijgo.2013.05.017
Alnaes-Katjavivi, P., Lyall, F., Roald, B., Redman, C. W., and Staff, A. C. (2016). Acute atherosis in vacuum suction biopsies of decidua basalis: An evidence based research definition. Placenta 37, 26–33. doi:10.1016/j.placenta.2015.10.020
Anderson, K. D., Pan, L., Yang, X. M., Hughes, V. C., Walls, J. R., Dominguez, M. G., et al. (2011). Angiogenic sprouting into neural tissue requires Gpr124, an orphan G protein-coupled receptor. Proc. Natl. Acad. Sci. U.S.A. 108 (7), 2807–2812. doi:10.1073/pnas.1019761108
Attwood, T. K., and Findlay, J. B. (1994). Fingerprinting G-protein-coupled receptors. Protein Eng. Des. Sel. 7 (2), 195–203. doi:10.1093/protein/7.2.195
Avagliano, L., Bulfamante, G. P., Morabito, A., and Marconi, A. M. (2011). Abnormal spiral artery remodelling in the decidual segment during pregnancy: From histology to clinical correlation. J. Clin. Pathol. 64 (12), 1064–1068. doi:10.1136/jclinpath-2011-200092
Bamberger, A. M., Minas, V., Kalantaridou, S. N., Radde, J., Sadeghian, H., Löning, T., et al. (2006). Corticotropin-releasing hormone modulates human trophoblast invasion through carcinoembryonic antigen-related cell adhesion molecule-1 regulation. Am. J. Pathology 168 (1), 141–150. doi:10.2353/ajpath.2006.050167
Bellamy, L., Casas, J. P., Hingorani, A. D., and Williams, D. J. (2007). Pre-eclampsia and risk of cardiovascular disease and cancer in later life: Systematic review and meta-analysis. BMJ 335 (7627), 974. doi:10.1136/bmj.39335.385301.BE
Bjarnadóttir, T. K., Fredriksson, R., Höglund, P. J., Gloriam, D. E., Lagerström, M. C., and Schiöth, H. B. (2004). The human and mouse repertoire of the adhesion family of G-protein-coupled receptors. Genomics 84 (1), 23–33. doi:10.1016/j.ygeno.2003.12.004
Bockaert, J., and Pin, J. P. (1999). Molecular tinkering of G protein-coupled receptors: An evolutionary success. EMBO J. 18 (7), 1723–1729. doi:10.1093/emboj/18.7.1723
Bogias, K. J., Pederson, S. M., Leemaqz, S., Smith, M. D., McAninch, D., Jankovic-Karasoulos, T., et al. (2022). Placental transcription profiling in 6-23 weeks' gestation reveals differential transcript usage in early development. Ijms 23 (9), 4506. doi:10.3390/ijms23094506
Brennan, L. J., Morton, J. S., and Davidge, S. T. (2014). Vascular dysfunction in preeclampsia. Microcirculation 21 (1), 4–14. doi:10.1111/micc.12079
Brown, M. A., Magee, L. A., Kenny, L. C., Karumanchi, S. A., McCarthy, F. P., Saito, S., et al. (2018). Hypertensive disorders of pregnancy. Hypertension 72 (1), 24–43. doi:10.1161/HYPERTENSIONAHA.117.10803
Burton, G. J., Redman, C. W., Roberts, J. M., and Moffett, A. (2019). Pre-eclampsia: Pathophysiology and clinical implications. BMJ 366, l2381. doi:10.1136/bmj.l2381
Chang, C. L., and Hsu, S. Y. (2013). Roles of CLR/RAMP receptor signaling in reproduction and development. Cpps 14 (5), 393–406. doi:10.2174/13892037113149990056
Chang, J., Mancuso, M. R., Maier, C., Liang, X., Yuki, K., Yang, L., et al. (2017). Gpr124 is essential for blood-brain barrier integrity in central nervous system disease. Nat. Med. 23 (4), 450–460. doi:10.1038/nm.4309
Chappell, L. C., Cluver, C. A., Kingdom, J., and Tong, S. (2021). Pre-eclampsia. Lancet 398 (10297), 341–354. doi:10.1016/S0140-6736(20)32335-7
Charron, M. J., and Vuguin, P. M. (2015). Lack of glucagon receptor signaling and its implications beyond glucose homeostasis. J. Endocrinol. 224 (3), R123–R130. doi:10.1530/JOE-14-0614
Chen, Y., Allars, M., Pan, X., Maiti, K., Angeli, G., Smith, R., et al. (2013). Effects of corticotrophin releasing hormone (CRH) on cell viability and differentiation in the human BeWo choriocarcinoma cell line: A potential syncytialisation inducer distinct from cyclic adenosine monophosphate (cAMP). Reprod. Biol. Endocrinol. 11, 30. doi:10.1186/1477-7827-11-30
Conrad, K. P. (2016). G-Protein-coupled receptors as potential drug candidates in preeclampsia: Targeting the relaxin/insulin-like family peptide receptor 1 for treatment and prevention. Hum. Reprod. Update 22 (5), 647–664. doi:10.1093/humupd/dmw021
Cullen, M., Elzarrad, M. K., Seaman, S., Zudaire, E., Stevens, J., Yang, M. Y., et al. (2011). GPR124, an orphan G protein-coupled receptor, is required for CNS-specific vascularization and establishment of the blood-brain barrier. Proc. Natl. Acad. Sci. U.S.A. 108 (14), 5759–5764. doi:10.1073/pnas.1017192108
Dackor, R. T., Fritz-Six, K., Dunworth, W. P., Gibbons, C. L., Smithies, O., and Caron, K. M. (2006). Hydrops fetalis, cardiovascular defects, and embryonic lethality in mice lacking the calcitonin receptor-like receptor gene. Mol. Cell. Biol. 26 (7), 2511–2518. doi:10.1128/MCB.26.7.2511-2518.2006
Devisme, L., Merlot, B., Ego, A., Houfflin-Debarge, V., Deruelle, P., and Subtil, D. (2013). A case-control study of placental lesions associated with pre-eclampsia. Int. J. Gynecol. Obstetrics 120 (2), 165–168. doi:10.1016/j.ijgo.2012.08.023
Dong, Y. L., Vegiraju, S., Chauhan, M., and Yallampalli, C. (2003). Expression of calcitonin gene-related peptide receptor components, calcitonin receptor-like receptor and receptor activity modifying protein 1, in the rat placenta during pregnancy and their cellular localization. Mol. Hum. Reprod. 9 (8), 481–490. doi:10.1093/molehr/gag058
Dong, Y. L., Vegiraju, S., Chauhan, M., Gangula, P. R., Hankins, G. D., Goodrum, L., et al. (2004). Involvement of calcitonin gene-related peptide in control of human fetoplacental vascular tone. Am. J. Physiology-Heart Circulatory Physiology 286 (1), H230–H239. doi:10.1152/ajpheart.00140.2003
Dong, Y. L., Green, K. E., Vegiragu, S., Hankins, G. D., Martin, E., Chauhan, M., et al. (2005). Evidence for decreased calcitonin gene-related peptide (CGRP) receptors and compromised responsiveness to CGRP of fetoplacental vessels in preeclamptic pregnancies. J. Clin. Endocrinol. Metabolism 90 (4), 2336–2343. doi:10.1210/jc.2004-1481
Dong, Y. L., Chauhan, M., Green, K. E., Vegiraju, S., Wang, H. Q., Hankins, G. D., et al. (2006). Circulating calcitonin gene-related peptide and its placental origins in normotensive and preeclamptic pregnancies. Am. J. Obstetrics Gynecol. 195 (6), 1657–1667. doi:10.1016/j.ajog.2006.04.006
Dorsam, R. T., and Gutkind, J. S. (2007). G-protein-coupled receptors and cancer. Nat. Rev. Cancer. 7 (2), 79–94. doi:10.1038/nrc2069
Dröge, L. A., Perschel, F. H., Stütz, N., Gafron, A., Frank, L., Busjahn, A., et al. (2021). Prediction of preeclampsia-related adverse outcomes with the sFlt-1 (soluble fms-like tyrosine kinase 1)/PlGF (placental growth factor)-ratio in the clinical routine. Hypertension 77 (2), 461–471. doi:10.1161/HYPERTENSIONAHA.120.15146
Duley, L., Meher, S., Hunter, K. E., Seidler, A. L., and Askie, L. M. (2019). Antiplatelet agents for preventing pre-eclampsia and its complications. Cochrane Database Syst. Rev. 2019 (10), CD004659. doi:10.1002/14651858.CD004659.pub3
Egeland, G. M., Klungsøyr, K., Øyen, N., Tell, G. S., Næss, O., and Skjærven, R. (2016). Preconception cardiovascular risk factor differences between gestational hypertension and preeclampsia. Hypertension 67 (6), 1173–1180. doi:10.1161/HYPERTENSIONAHA.116.07099
Ferrazzi, E., Stampalija, T., Monasta, L., Di Martino, D., Vonck, S., and Gyselaers, W. (2018). Maternal hemodynamics: A method to classify hypertensive disorders of pregnancy. Am. J. Obstetrics Gynecol. 218 (1), e1–124. doi:10.1016/j.ajog.2017.10.226
Fredriksson, R., Lagerström, M. C., Lundin, L. G., and Schiöth, H. B. (2003). The G-protein-coupled receptors in the human genome form five main families. Phylogenetic analysis, paralogon groups, and fingerprints. Mol. Pharmacol. 63 (6), 1256–1272. doi:10.1124/mol.63.6.1256
Gao, L., He, P., Sha, J., Liu, C., Dai, L., Hui, N., et al. (2007). Corticotropin-releasing hormone receptor type 1 and type 2 mediate differential effects on 15-hydroxy prostaglandin dehydrogenase expression in cultured human chorion trophoblasts. Endocrinology 148 (8), 3645–3654. doi:10.1210/en.2006-1212
Gao, L., Lu, C., Xu, C., Tao, Y., Cong, B., and Ni, X. (2008). Differential regulation of prostaglandin production mediated by corticotropin-releasing hormone receptor type 1 and type 2 in cultured human placental trophoblasts. Endocrinology 149 (6), 2866–2876. doi:10.1210/en.2007-1377
Gao, L., Lv, C., Xu, C., Li, Y., Cui, X., Gu, H., et al. (2012a). Differential regulation of glucose transporters mediated by CRH receptor type 1 and type 2 in human placental trophoblasts. Endocrinology 153 (3), 1464–1471. doi:10.1210/en.2011-1673
Gao, L., Tao, Y., Hu, T., Liu, W., Xu, C., Liu, J., et al. (2012b). Regulation of estradiol and progesterone production by CRH-R1 and -R2 is through divergent signaling pathways in cultured human placental trophoblasts. Endocrinology 153 (10), 4918–4928. doi:10.1210/en.2012-1453
Hellman, P., Hellman, B., Juhlin, C., Juppner, H., Rastad, J., Ridefelt, P., et al. (1993). Regulation of proliferation in JEG-3 cells by a 500-kDa Ca2+ sensor and parathyroid hormone-related protein. Archives Biochem. Biophysics 307 (2), 379–385. doi:10.1006/abbi.1993.1603
Hamann, J., Aust, G., Araç, D., Engel, F. B., Formstone, C., Fredriksson, R., et al. (2015). International union of basic and clinical Pharmacology. XCIV. Adhesion G protein-coupled receptors. Pharmacol. Rev. 67 (2), 338–367. doi:10.1124/pr.114.009647
Hutcheon, J. A., Lisonkova, S., and Joseph, K. S. (2011). Epidemiology of pre-eclampsia and the other hypertensive disorders of pregnancy. Best Pract. Res. Clin. Obstetrics Gynaecol. 25 (4), 391–403. doi:10.1016/j.bpobgyn.2011.01.006
Hutchings, C. J. (2020). A review of antibody-based therapeutics targeting G protein-coupled receptors: An update. Expert Opin. Biol. Ther. 20 (8), 925–935. doi:10.1080/14712598.2020.1745770
Ishihara, N., Matsuo, H., Murakoshi, H., Laoag-Fernandez, J. B., Samoto, T., and Maruo, T. (2002). Increased apoptosis in the syncytiotrophoblast in human term placentas complicated by either preeclampsia or intrauterine growth retardation. Am. J. Obstetrics Gynecol. 186 (1), 158–166. doi:10.1067/mob.2002.119176
Jin, J. (2021). Use of aspirin during pregnancy to prevent preeclampsia. JAMA 326 (12), 1222. doi:10.1001/jama.2021.15900
Kadmiel, M., Matson, B. C., Espenschied, S. T., Lenhart, P. M., and Caron, K. M. (2017). Loss of receptor activity-modifying protein 2 in mice causes placental dysfunction and alters PTH1R regulation. PLoS One 12 (7), e0181597. doi:10.1371/journal.pone.0181597
Kajantie, E., Eriksson, J. G., Osmond, C., Thornburg, K., and Barker, D. J. (2009). Pre-eclampsia is associated with increased risk of stroke in the adult offspring. Stroke 40 (4), 1176–1180. doi:10.1161/STROKEAHA.108.538025
Kalantaridou, S. N., Zoumakis, E., Makrigiannakis, A., Godoy, H., and Chrousos, G. P. (2007). The role of corticotropin-releasing hormone in blastocyst implantation and early fetal immunotolerance. Horm. Metab. Res. 39 (6), 474–477. doi:10.1055/s-2007-980190
Karteris, E., Grammatopoulos, D., Dai, Y., Olah, K. B., Ghobara, T. B., Easton, A., et al. (1998). The human placenta and fetal membranes express the corticotropin-releasing hormone receptor 1α (CRH-1α) and the CRH-C variant Receptor1. J. Clin. Endocrinol. Metabolism 83 (4), 1376–1379. doi:10.1210/jcem.83.4.4705
Karteris, E., Goumenou, A., Koumantakis, E., Hillhouse, E. W., and Grammatopoulos, D. K. (2003). Reduced expression of corticotropin-releasing hormone receptor type-1α in human preeclamptic and growth-restricted placentas. J. Clin. Endocrinol. Metab. 88 (1), 363–370. doi:10.1210/jc.2002-020375
Koh, P. O., Kwak, S. D., Kim, H. J., Roh, G., Kim, J. H., Kang, S. S., et al. (2003). Expression patterns of pituitary adenylate cyclase activating polypeptide and its type I receptor mRNAs in the rat placenta. Mol. Reprod. Dev. 64 (1), 27–31. doi:10.1002/mrd.10221
Koh, P. O., Won, C. K., Noh, H. S., Cho, G. J., and Choi, W. S. (2005). Expression of pituitary adenylate cyclase activating polypeptide and its type I receptor mRNAs in human placenta. J. Vet. Sci. 6 (1), 1–5. doi:10.4142/jvs.2005.6.1.1
Kuhnert, F., Mancuso, M. R., Shamloo, A., Wang, H. T., Choksi, V., Florek, M., et al. (2010). Essential regulation of CNS angiogenesis by the orphan G protein-coupled receptor GPR124. Science 330 (6006), 985–989. doi:10.1126/science.1196554
Lagerström, M. C., and Schiöth, H. B. (2008). Structural diversity of G protein-coupled receptors and significance for drug discovery. Nat. Rev. Drug Discov. 7 (4), 339–357. doi:10.1038/nrd2518
Lappano, R., and Maggiolini, M. (2011). G protein-coupled receptors: Novel targets for drug discovery in cancer. Nat. Rev. Drug Discov. 10 (1), 47–60. doi:10.1038/nrd3320
Levine, R. J., Maynard, S. E., Qian, C., Lim, K. H., England, L. J., Yu, K. F., et al. (2004). Circulating angiogenic factors and the risk of preeclampsia. N. Engl. J. Med. 350 (7), 672–683. doi:10.1056/NEJMoa031884
Li, L., Tang, F., and O, W. S. (2010). Coexpression of adrenomedullin and its receptor component proteins in the reproductive system of the rat during gestation. Reprod. Biol. Endocrinol. 8, 130. doi:10.1186/1477-7827-8-130
Liang, M., Niu, J., Zhang, L., Deng, H., Ma, J., Zhou, W., et al. (2016). Gene expression profiling reveals different molecular patterns in G-protein coupled receptor signaling pathways between early- and late-onset preeclampsia. Placenta 40, 52–59. doi:10.1016/j.placenta.2016.02.015
Liu, A., Wen, S. W., Bottomley, J., Walker, M. C., and Smith, G. (2009). Utilization of health care services of pregnant women complicated by preeclampsia in Ontario. Hypertens. Pregnancy 28 (1), 76–84. doi:10.1080/10641950802366252
Lu, S., Liu, S., Wietelmann, A., Kojonazarov, B., Atzberger, A., Tang, C., et al. (2017). Developmental vascular remodeling defects and postnatal kidney failure in mice lacking Gpr116 (Adgrf5) and Eltd1 (Adgrl4). PLoS One 12 (8), e0183166. doi:10.1371/journal.pone.0183166
Mackie, D. I., Al Mutairi, M. F., Davis, R. B., Kechele, D. O., Nielsen, N. R., Snyder, J. C., et al. (2018). HCALCRL mutation causes autosomal recessive nonimmune hydrops fetalis with lymphatic dysplasia. J. Exp. Med. 215 (9), 2339–2353. doi:10.1084/jem.20180528
Majewska, M., Lipka, A., Paukszto, L., Jastrzebski, J. P., Szeszko, K., Gowkielewicz, M., et al. (2019). Placenta transcriptome profiling in intrauterine growth restriction (IUGR). Ijms 20 (6), 1510. doi:10.3390/ijms20061510
Makino, Y., Shibata, K., Makino, I., Kangawa, K., and Kawarabayashi, T. (2001). Alteration of the adrenomedullin receptor components gene expression associated with the blood pressure in pregnancy-induced hypertension. J. Clin. Endocrinol. Metabolism 86 (10), 5079. doi:10.1210/jcem.86.10.8099
Makrigiannakis, A., Zoumakis, E., Kalantaridou, S., Coutifaris, C., Margioris, A. N., Coukos, G., et al. (2001). Corticotropin-releasing hormone promotes blastocyst implantation and early maternal tolerance. Nat. Immunol. 2 (11), 1018–1024. doi:10.1038/ni719
Masiero, M., Simões, F. C., Han, H. D., Snell, C., Peterkin, T., Bridges, E., et al. (2013). A core human primary tumor angiogenesis signature identifies the endothelial orphan receptor ELTD1 as a key regulator of angiogenesis. Cancer Cell 24 (2), 229–241. doi:10.1016/j.ccr.2013.06.004
Maynard, S. E., Min, J. Y., Merchan, J., Lim, K. H., Li, J., Mondal, S., et al. (2003). Excess placental soluble fms-like tyrosine kinase 1 (sFlt1) may contribute to endothelial dysfunction, hypertension, and proteinuria in preeclampsia. J. Clin. Invest. 111 (5), 649–658. doi:10.1172/JCI17189
McGuane, J., and Conrad, K. (2012). GPCRs as potential therapeutic targets in preeclampsia. Drug Discov. Today Dis. Models 9 (3), e119–e127. doi:10.1016/j.ddmod.2012.05.001
Mongraw-Chaffin, M. L., Cirillo, P. M., and Cohn, B. A. (2010). Preeclampsia and cardiovascular disease death. Hypertension 56 (1), 166–171. doi:10.1161/HYPERTENSIONAHA.110.150078
Moriguchi, T., Haraguchi, K., Ueda, N., Okada, M., Furuya, T., and Akiyama, T. (2004). DREG, a developmentally regulated G protein-coupled receptor containing two conserved proteolytic cleavage sites. Genes cells. 9 (6), 549–560. doi:10.1111/j.1356-9597.2004.00743.x
Ouhilal, S., Vuguin, P., Cui, L., Du, X. Q., Gelling, R. W., Reznik, S. E., et al. (2012). Hypoglycemia, hyperglucagonemia, and fetoplacental defects in glucagon receptor knockout mice: A role for glucagon action in pregnancy maintenance. Am. J. Physiology-Endocrinology Metabolism 302 (5), E522–E531. doi:10.1152/ajpendo.00420.2011
Powe, C. E., Levine, R. J., and Karumanchi, S. A. (2011). Preeclampsia, a disease of the maternal endothelium. Circulation 123 (24), 2856–2869. doi:10.1161/CIRCULATIONAHA.109.853127
Powers, R. W., Roberts, J. M., Plymire, D. A., Pucci, D., Datwyler, S. A., Laird, D. M., et al. (2012). Low placental growth factor Across pregnancy identifies a subset of women with preterm preeclampsia. Hypertension 60 (1), 239–246. doi:10.1161/HYPERTENSIONAHA.112.191213
Purcell, R. H., and Hall, R. A. (2018). Adhesion g Protein-Coupled receptors as drug targets. Annu. Rev. Pharmacol. Toxicol. 58, 429–449. doi:10.1146/annurev-pharmtox-010617-052933
Quitterer, U., and AbdAlla, S. (2021). Pathological AT1R-B2R protein aggregation and preeclampsia. Cells 10 (10), 2609. doi:10.3390/cells10102609
Redman, C. W. (1991). Pre-eclampsia and the placenta. Placenta 12 (4), 301–308. doi:10.1016/0143-4004(91)90339-h
Redman, C. W., Sacks, G. P., and Sargent, I. L. (1999). Preeclampsia: An excessive maternal inflammatory response to pregnancy. Am. J. Obstetrics Gynecol. 180 (2), 499–506. doi:10.1016/s0002-9378(99)70239-5
Redman, C., Staff, A. C., and Roberts, J. M. (2022). Syncytiotrophoblast stress in preeclampsia: The convergence point for multiple pathways. Am. J. Obstetrics Gynecol. 226, S907–S927. doi:10.1016/j.ajog.2020.09.047
Redman, C. W., and Staff, A. C. (2015). Preeclampsia, biomarkers, syncytiotrophoblast stress, and placental capacity. Am. J. Obstetrics Gynecol. 213 (4), e1–S9. S9-S11. doi:10.1016/j.ajog.2015.08.003
Reglodi, D., Borzsei, R., Bagoly, T., Boronkai, A., Racz, B., Tamas, A., et al. (2008). Agonistic behavior of PACAP6-38 on sensory nerve terminals and cytotrophoblast cells. J. Mol. Neurosci. 36 (1-3), 270–278. doi:10.1007/s12031-008-9089-z
Riddy, D. M., Delerive, P., Summers, R. J., Sexton, P. M., and Langmead, C. J. (2018). G Protein-Coupled receptors targeting insulin resistance, obesity, and type 2 diabetes mellitus. Pharmacol. Rev. 70 (1), 39–67. doi:10.1124/pr.117.014373
Say, L., Chou, D., Gemmill, A., Tunçalp, O., Moller, A. B., Daniels, J., et al. (2014). Global causes of maternal death: A WHO systematic analysis. Lancet Glob. Health 2 (6), e323–e333. doi:10.1016/S2214-109X(14)70227-X
Scaldaferri, M. L., Modesti, A., Palumbo, C., Ulisse, S., Fabbri, A., Piccione, E., et al. (2000). Pituitary adenylate cyclase-activating polypeptide (PACAP) and PACAP-receptor type 1 expression in rat and human Placenta1. Endocrinology 141 (3), 1158–1167. doi:10.1210/endo.141.3.7346
Shen, H., Jin, M., Gu, S., Wu, Y., Yang, M., and Hua, X. (2020). CD97 is decreased in preeclamptic placentas and promotes human trophoblast invasion through PI3K/Akt/mTOR signaling pathway. Reprod. Sci. 27 (8), 1553–1561. doi:10.1007/s43032-020-00183-w
Sheppard, B. L., and Bonnar, J. (1976). The ultrastructure of the arterial supply of the human placenta in pregnancy complicated by fetal growth retardation. BJOG:An Int. J. O&G 83 (12), 948–959. doi:10.1111/j.1471-0528.1976.tb00781.x
Sirico, A., Dell’Aquila, M., Tartaglione, L., Moresi, S., Farì, G., Pitocco, D., et al. (2021). PTH-rP and PTH-R1 expression in placentas from pregnancies complicated by gestational diabetes: New insights into the pathophysiology of hyperglycemia in pregnancy. Diagnostics 11 (8), 1356. doi:10.3390/diagnostics11081356
Skjærven, R., Wilcox, A. J., and Lie, R. T. (2002). The interval between pregnancies and the risk of preeclampsia. N. Engl. J. Med. 346 (1), 33–38. doi:10.1056/NEJMoa011379
Stehlik, C., Kroismayr, R., Dorfleutner, A., Binder, B. R., and Lipp, J. (2004). Vigr - a novel inducible adhesion family G-protein coupled receptor in endothelial cells. FEBS Lett. 569 (1-3), 149–155. doi:10.1016/j.febslet.2004.05.038
Tannetta, D. S., Hunt, K., Jones, C. I., Davidson, N., Coxon, C. H., Ferguson, D., et al. (2015). Syncytiotrophoblast extracellular Vesicles from pre-eclampsia placentas differentially affect platelet function. PLoS One 10 (11), e0142538. doi:10.1371/journal.pone.0142538
Torregrosa-Carrión, R., Piñeiro-Sabarís, R., Siguero-Álvarez, M., Grego-Bessa, J., Luna-Zurita, L., Fernandes, V. S., et al. (2021). Adhesion G protein-coupled receptor Gpr126/Adgrg6 is essential for placental development. Sci. Adv. 7 (46), j5445. doi:10.1126/sciadv.abj5445
Tsatsaris, V., Tarrade, A., Merviel, P., Garel, J. M., Segond, N., Jullienne, A., et al. (2002). Calcitonin gene-related peptide (CGRP) and CGRP receptor expression at the human implantation site. J. Clin. Endocrinol. Metabolism 87 (9), 4383–4390. doi:10.1210/jc.2002-020138
Tuovinen, S., Räikkönen, K., Pesonen, A. K., Lahti, M., Heinonen, K., Wahlbeck, K., et al. (2012). Hypertensive disorders in pregnancy and risk of severe mental disorders in the offspring in adulthood: The Helsinki Birth Cohort Study. J. Psychiatric Res. 46 (3), 303–310. doi:10.1016/j.jpsychires.2011.11.015
Vallon, M., and Essler, M. (2006). Proteolytically processed soluble tumor endothelial marker (TEM) 5 mediates endothelial cell survival during angiogenesis by linking integrin αvβ3 to glycosaminoglycans. J. Biol. Chem. 281 (45), 34179–34188. doi:10.1074/jbc.M605291200
Waller-Evans, H., Prömel, S., Langenhan, T., Dixon, J., Zahn, D., Colledge, W. H., et al. (2010). The orphan adhesion-GPCR GPR126 is required for embryonic development in the mouse. PLoS One 5 (11), e14047. doi:10.1371/journal.pone.0014047
Wang, T., Ward, Y., Tian, L., Lake, R., Guedez, L., Stetler-Stevenson, W. G., et al. (2005). CD97, an adhesion receptor on inflammatory cells, stimulates angiogenesis through binding integrin counterreceptors on endothelial cells. Blood 105 (7), 2836–2844. doi:10.1182/blood-2004-07-2878
Wang, J., Gareri, C., and Rockman, H. A. (2018). G-Protein-Coupled receptors in heart disease. Circ. Res. 123 (6), 716–735. doi:10.1161/CIRCRESAHA.118.311403
Wu, C. T., Hilgendorf, K. I., Bevacqua, R. J., Hang, Y., Demeter, J., Kim, S. K., et al. (2021). Discovery of ciliary G protein-coupled receptors regulating pancreatic islet insulin and glucagon secretion. Genes Dev. 35 (17-18), 1243–1255. doi:10.1101/gad.348261.121
Xiong, X., Demianczuk, N. N., Saunders, L. D., Wang, F. L., and Fraser, W. D. (2002). Impact of preeclampsia and gestational hypertension on birth weight by gestational age. Am. J. Epidemiol. 155 (3), 203–209. doi:10.1093/aje/155.3.203
Yoo, J. Y., Ahn, J. I., Kim, T. H., Yu, S., Ahn, J. Y., Lim, J. M., et al. (2017). G-protein coupled receptor 64 is required for decidualization of endometrial stromal cells. Sci. Rep. 7 (1), 5021. doi:10.1038/s41598-017-05165-8
Younes, S. T., Maeda, K. J., Sasser, J., and Ryan, M. J. (2020). The glucagon-like peptide 1 receptor agonist liraglutide attenuates placental ischemia-induced hypertension. Am. J. Physiology-Heart Circulatory Physiology 318 (1), H72–H77. doi:10.1152/ajpheart.00486.2019
Zaki, M., Greenwood, C., and Redman, C. W. (2003). The spontaneous reversal of pre-eclampsia associated with parvovirus-induced hydrops and the placental theory of pre-eclampsia: A case report. BJOG Intern. J. Obs Gyn 110 (12), 1125–1126. doi:10.1111/j.1471-0528.2003.03060.x
Zhang, X., Green, K. E., Yallampalli, C., and Dong, Y. L. (2005). Adrenomedullin enhances invasion by trophoblast cell Lines1. Biol. Reprod. 73 (4), 619–626. doi:10.1095/biolreprod.105.040436
Zhang, B., Kim, M. Y., Elliot, G., Zhou, Y., Zhao, G., Li, D., et al. (2021). Human placental cytotrophoblast epigenome dynamics over gestation and alterations in placental disease. Dev. Cell 56 (9), 1238–1252. doi:10.1016/j.devcel.2021.04.001
Keywords: preeclampsia (PE), pathogenesis, placenta, secretin GPCRs, adhesion GPCRs
Citation: Yin A, Guan X, Zhang JV and Niu J (2022) Focusing on the role of secretin/adhesion (Class B) G protein-coupled receptors in placental development and preeclampsia. Front. Cell Dev. Biol. 10:959239. doi: 10.3389/fcell.2022.959239
Received: 01 June 2022; Accepted: 25 July 2022;
Published: 14 September 2022.
Edited by:
Rujuan Zuo, Oslo University Hospital, NorwayReviewed by:
Qingliang Zheng, The Eighth Affiliated Hospital, Sun Yat-sen University, ChinaLinlin Sui, Dalian Medical University, China
Copyright © 2022 Yin, Guan, Zhang and Niu. This is an open-access article distributed under the terms of the Creative Commons Attribution License (CC BY). The use, distribution or reproduction in other forums is permitted, provided the original author(s) and the copyright owner(s) are credited and that the original publication in this journal is cited, in accordance with accepted academic practice. No use, distribution or reproduction is permitted which does not comply with these terms.
*Correspondence: Jian V. Zhang, amlhbi56aGFuZ0BzaWF0LmFjLmNu; Jianmin Niu, bmppYW5taW5AMTYzLmNvbQ==