- 1Anatomy and Embryology, University Medical Center Göttingen, Göttingen, Germany
- 2Department of Embryology, Faculty of Biology, Lomonosov Moscow State University, Moscow, Russia
- 3Koltzov Institute of Developmental Biology, Russian Academy of Sciences, Moscow, Russia
Development of visceral left–right asymmetry in bilateria is based on initial symmetry breaking followed by subsequent asymmetric molecular patterning. An important step is the left-sided expression of transcription factor pitx2 which is mediated by asymmetric expression of the nodal morphogen in the left lateral plate mesoderm of vertebrates. Processes leading to emergence of the asymmetric nodal domain differ depending on the mode of symmetry breaking. In Xenopus laevis and mouse embryos, the leftward fluid flow on the ventral surface of the left–right organizer leads through intermediate steps to enhanced activity of the nodal protein on the left side of the organizer and subsequent asymmetric nodal induction in the lateral plate mesoderm. In the chick embryo, asymmetric morphogenesis of axial organs leads to paraxial nodal asymmetry during the late gastrulation stage. Although it was shown that hedgehog signaling is required for initiation of the nodal expression, the mechanism of its asymmetry remains to be clarified. In this study, we established the activation of hedgehog signaling in early chick embryos to further study its role in the initiation of asymmetric nodal expression. Our data reveal that hedgehog signaling is sufficient to induce the nodal expression in competent domains of the chick embryo, while treatment of Xenopus embryos led to moderate nodal inhibition. We discuss the role of symmetry breaking and competence in the initiation of asymmetric gene expression.
Introduction
The position and shape of visceral organs of adult vertebrates do not display mirror symmetry deviating from the midline (Matsui and Bessho, 2012; Hamada and Tam, 2020). This left–right asymmetry is a fundamental morphological feature of all studied vertebrates and of many studied invertebrates (Tisler et al., 2016; Datar et al., 2017; Blum and Ott, 2018; Lebreton et al., 2018). The asymmetry displays a definite direction typical for the organism in the vast majority of cases (Afzelius and Stenram, 2006). This “normal” position, also called situs solitus, is based on asymmetric morphogenetic processes which are believed to be preceded by asymmetric gene expression. The key asymmetric “highway” is created by the left-sided expression of the TGF-beta ligand nodal and its downstream counterpart transcription factor pitx2 in the lateral plate mesoderm (Levin et al., 1995; Logan et al., 1998; Rebagliati et al., 1998; Campione et al., 1999; Patel et al., 1999; Blum et al., 2009; Grande and Patel, 2009). The asymmetry of the nodal expression is at the same time an early marker of successful symmetry breaking which is achieved in vertebrates by at least two different mechanisms. The so-called leftward flow is based on the rotation of long monocilia on the ventral surface of the epithelial progenitors of the axial mesoderm and operates in most model organisms such as X. laevis, Danio rerio, and Mus musculus (Essner et al., 2005; Nonaka et al., 2005; Schweickert et al., 2007), where it is suggested to be a symmetry breaking mechanism; also, earlier relevant asymmetries have been reported and suggested to break the symmetry, particularly in X. laevis (Lobikin et al., 2012; Vandenberg et al., 2013; Onjiko et al., 2016). The direction of the leftward flow is detected by sensory monocilia on the so-called somitic floor plate. This leads through intermediate steps to the left-sided activation of the paraxial secreted nodal protein, which in turn is responsible for its subsequent expression in the left lateral plate mesoderm (Schweickert et al., 2010; Maerker et al., 2021). However, the first signs of the molecular asymmetry in the chick and some mammals are seen already in the paraxial mesoderm in the form of the left-sided nodal domain (Tsikolia et al., 2012; Schroder et al., 2016), indicating divergence of symmetry-breaking mechanisms in vertebrates (Kajikawa et al., 2020). This suggestion is supported by both the absence of morphological structures enabling flow (Manner, 2001; Tsikolia et al., 2012; Stephen et al., 2014; Pieper et al., 2020) and the evolutionary loss of genes involved in the nodal activating network operating downstream of the leftward flow (Szenker-Ravi et al., 2022). Asymmetric nodal expression in the chick is preceded by a leftward node rotation at stage 4 (Cui et al., 2009; Gros et al., 2009), which is followed by asymmetric positioning of the axial mesoderm and the floor plate anlagen (Otto et al., 2014). As a result, the posterior part of the floor plate lies to the left of the notochord, whereby the expression of the sonic hedgehog (shh) morphogen in the floor plate is located directly above the prospective nodal domain in the left paraxial mesoderm, hence indicating a possible functional relationship. Sonic hedgehog is an early marker expressed in the organizer region and emerged in midline structures in various model organisms (Echelard et al., 1993; Roelink et al., 1994; Kremnyov et al., 2018) and is involved as a secreted morphogen in molecular patterning at different sites including neural tube and somitogenesis (Johnson et al., 1994; Ribes et al., 2010; Cohen et al., 2015). The activity of hedgehog signaling is required for initiation of the paraxial nodal expression in the chick as shown both by local anti-hedgehog antibody application (Pagan-Westphal and Tabin, 1998) and by global inhibition of hedgehog signaling by cyclopamine (Otto et al., 2014). Moreover, the nodal domain overlaps with the hedgehog receptor patched1 expression, which serves as an indirect reporter of hedgehog activity. Local implantation of shh expressing cells or of beads soaked with shh protein right to the node activates expression of nodal or its downstream effector pitx2 in the lateral plate mesoderm (LPM) at stage 9, indicating that shh is sufficient for nodal induction (Levin et al., 1995; Logan et al., 1998). However, the effect of ectopic shh on early paraxial nodal expression has not been studied yet. Moreover, hedgehog signaling undergoes complex regulation, and the presence of shh does not necessarily lead to an activated pathway. For example, the absence of shh and its downstream effector smoothened cause opposite effects in the mouse embryo (Tsukui et al., 1999; Tsiairis and McMahon, 2009), and phenotypic effects of knocking out of different hedgehog components on neural tube development are ambiguous (Motoyama et al., 2003; Iulianella et al., 2018). Hence, we aimed to test the effect of downstream signaling activation on the early left–right patterning and asymmetry by non-local activation of hedgehog signaling downstream of shh using the smoothened activator SAG. Furthermore, the global activator treatment enables addressing the competence of tissue. Our clear-cut results in the chick inspired us to examine the influence of hedgehog activation on nodal1 expression in the lateral plate mesoderm in X. laevis since the functional studies of hedgehog signaling indicated its non-conserved role in the left–right patterning (Tsiairis and McMahon, 2009; Zhu et al., 2020).
Materials and methods
Embryos
Fertilized chick eggs were incubated at 38°C under humidified conditions until reaching the desired stage (Hamburger and Hamilton, 1951; Pieper et al., 2020). For in vitro culture, embryos were processed as previously described (Sydow et al., 2017). After cultivation, blastoderms were prefixed in a fixative, excised, transferred into a petri dish, rinsed in Locke’s solution, and fixed in 4% PFA in phosphate-buffered saline (PBS) for 1 h.
X. laevis embryos were obtained by hormone-induced egg laying and in vitro fertilization using standard methods (Kay and Peng, 1992), de-jellied in 2% L-cysteine solution, pH 8, and then cultured in 0.1X MMR at 14–18°C. The embryos were staged according to the tables of normal development (Nieuwkoop and Faber, 1994). Before fixation in 4% PFA in phosphate-buffered saline (PBS), the embryos were liberated from the vitelline membrane with forceps. For further details, see Supporting Methods.
SAG treatment
In vivo activation of hedgehog signaling was performed with smoothened activator SAG (Bragina et al., 2010; Lewis and Krieg, 2014; Meinhardt et al., 2014; Todd and Fischer, 2015). SAG (Sigma-Aldrich, Taufkirchen) was dissolved in Millipore water to 10 mM (stock solution). The stock solution was diluted in PBS (for chicken) or 0.1X MMR (frog) to a final concentration of 250 and 100 µM, respectively, which were determined in pretests with different readouts. There, we also found that SAG retains its activity for 6 months if stored at −20°C. Control embryos were treated with 1% (Xenopus) or 2.5% (for chicken) Millipore water diluted in 0.1X MMR or PBS, respectively. Treatment of chick embryo was performed by application of 10 μl of the corresponding solution twice within 2 h to the ventral side of the embryos cultivated in a modified new culture as previously reported (Otto et al., 2014; Sydow et al., 2017).
In situ hybridization and cloning
In situ hybridization was performed according to previously published protocols (Harland, 1991; Weisheit et al., 2002). Digoxigenin-labeled mRNA probes for nodal and pitx2 were produced using the previously published plasmid DNAs (Levin et al., 1995; Logan et al., 1998). For further details, see Supplementary Methods.
Results
Stage 4 chick embryos were treated with control solution or smoothened agonist SAG and cultivated until stages 5–7. The control embryos show asymmetric nodal expression which at stage 5 reveals a strong narrow domain left to the notochord located in the paraxial mesoderm (Figure 1A), where advanced stage 6 expression is confined to both the left paraxial mesoderm and the lateral plate mesoderm (LPM), while these domains come close at the posterior level (Figures 1B, 2C, 2C'). From stage 7, the domain in the LPM extends and elongates especially toward the anterior pole (Figure 1C). The asymmetry of nodal expression is seen at all stages and is particularly strong in the LPM domain where it was never seen on the right side.
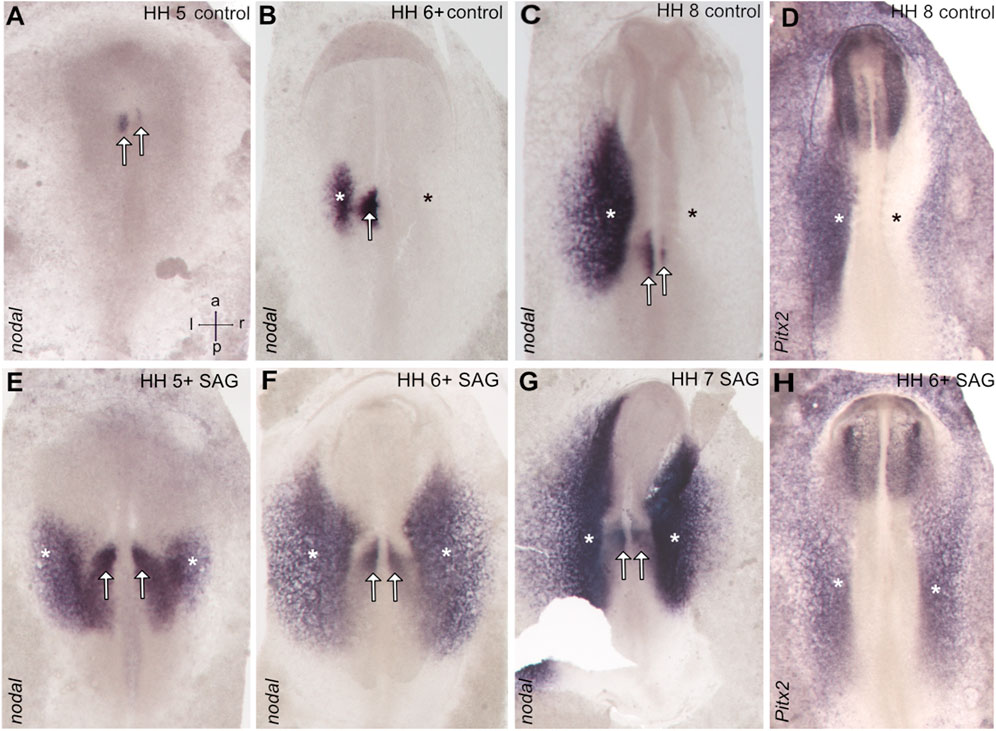
FIGURE 1. Symmetrical expression of markers of left–right patterning between stages 5 and 7 in the chick embryos treated with the activator of hedgehog signaling SAG. In control embryos, nodal expression is confined to the left-sided domains close to the posterior midline (paraxial domain) and in the area of the lateral plate mesoderm (A–C), while treated embryos reveal bilateral expression. (E–G) Similarly, pitx2 reveals in treated embryos bilateral expression in the lateral plate mesoderm. (H), while control embryos reveal left-sided expression in this domain (D). Asterisks indicate the position of the lateral plate mesoderm, arrows the paraxial domain, and intersecting arrows anatomical axes.
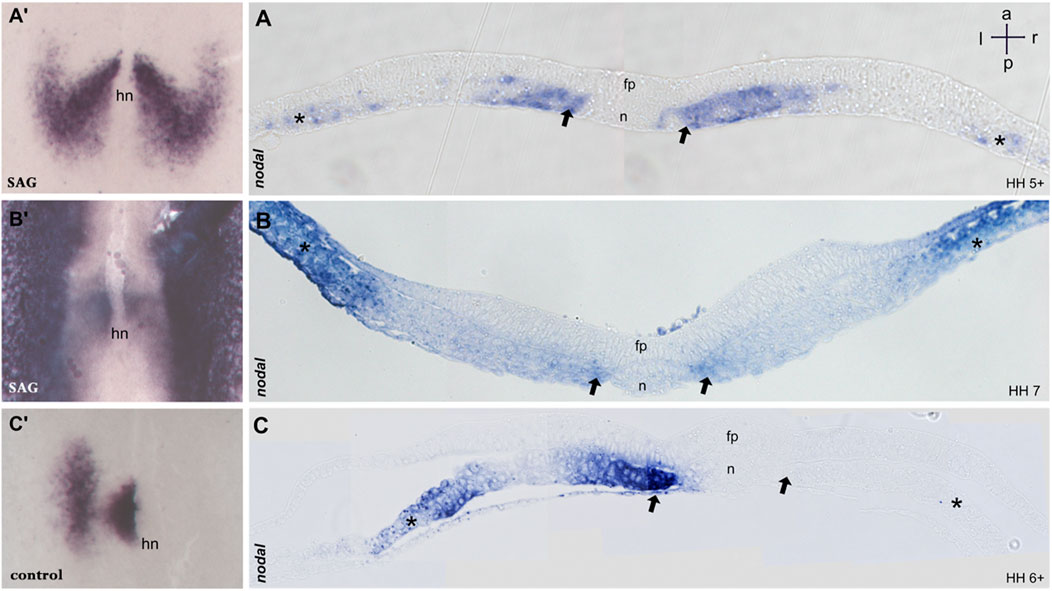
FIGURE 2. Sections of embryos treated with SAG. (A–C) 5 μm transversal sections of plastic (Technovit) embedded embryos at the level indicated in A′–C′, respectively. At stage 5, nodal expression is confined to the paraxial mesoderm and to mesodermal cells located laterally (A,A′). Stage 6 reveals four distinct domains confined to the paraxial mesoderm and lateral plate mesoderm on both sides (B,B′). Control embryos at stage 6 reveal expression in the left paraxial and lateral plate mesoderm (C,C′). n, notochord; fp, floor plate; hn, Hensen’s node; asterisk, lateral plate mesoderm; arrow, paraxial mesoderm. Intersecting arrows indicate anatomical axes.
Treated embryos develop without delay and form the notochord, head fold, and somites, while the overall phenotype was slightly widened. In situ hybridization reveals a strong and bilateral nodal expression. From advanced stage 5, the paraxial expression extends in the posterior and the lateral direction (Figures 1E, 2A′), forming a V-shaped domain. Sections reveal expression in emerging paraxial and lateral plate mesoderm (Figure 2A). At stages 6 and 7, nodal expression is confined to distinct elongated paraxial domains lateral to the notochord and to broad bilateral domains in the LPM (Figures 1F,G, 2B′,B), particularly at stage 6. Generally, in situ hybridization revealed strong symmetrization of the nodal domain (Supplementary Table S1A). We also analyzed the expression of nodal downstream effector pitx2. In control embryos, pitx2 expression at stages 7 and 8 reveals expression in extraembryonic tissue as well as in the left lateral plate mesoderm (Figure 1D). In treated embryos (Supplementary Table S1B), expression is confined particularly to the right lateral plate mesoderm (Figure 1H).
Furthermore, we treated stage 9 X. laevis embryos with SAG and analyzed nodal1 expression in the LPM at stage 24. Surprisingly, expression analysis indicates significant inhibition of nodal1 in the lateral plate mesoderm as compared to the control embryos (Figure 3).
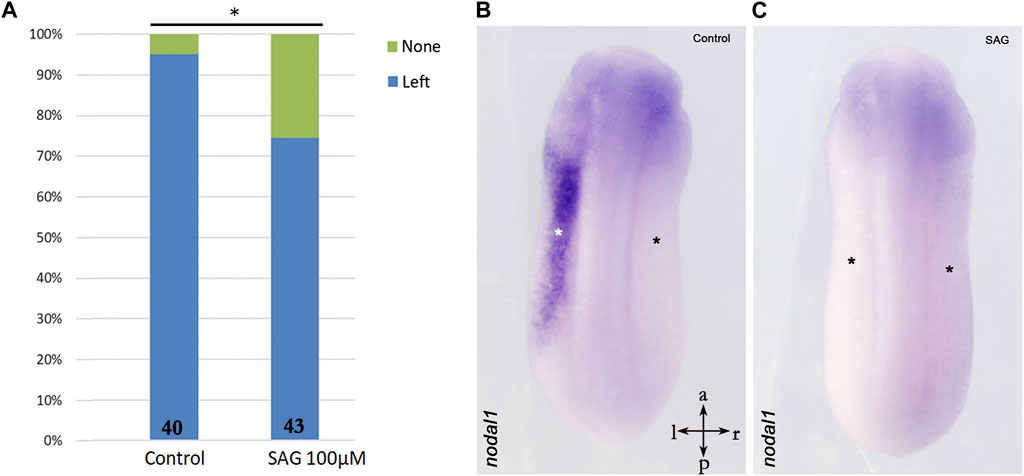
FIGURE 3. Inhibition of nodal1 expression in the lateral plate mesoderm of X. laevis embryos after treatment with an activator of hedgehog signaling. (A) Treatment with SAG leads to inhibition of nodal1 expression as compared to control embryos. (B) Example of left-sided nodal1 expression; (C) example of suppressed nodal1 expression. Intersecting arrows indicate anatomical axes. Numbers at the base of columns represent a number of analyzed embryos. *p < 0.05 (0.023) as compared to control and two-proportions z-test.
Asymmetrical cardiac looping is the first morphological sign of left–right asymmetry shared by all known model vertebrates (Manner, 2009; Lombardo et al., 2019). To study the influence of SAG on early morphological asymmetry, we treated chick embryos at stage 4 and let the embryos develop until stages 11–12. Treatment led to both randomizations of the cardiac looping and absent looping with a widened heart and severe deformities of the cardiac formation related to abnormal development of the intestinal portal, which included duplications of the heart tube (Figure 4).
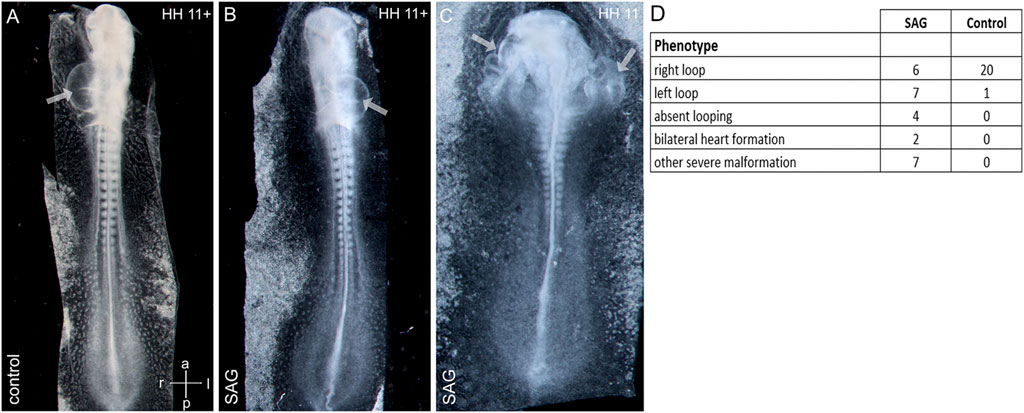
FIGURE 4. Abnormal cardiac development after treatment with an activator of hedgehog signaling SAG. (A–C): examples of right-sided (A), left-sided looping (B), and bilateral heart development (C) after SAG treatment. (D) Distribution of cardiac phenotypes in control and treated embryos. Arrows indicate heart tubes. Intersecting arrows indicate anatomical axes.
Discussion
The activity of sonic hedgehog has been proposed to be upstream of the asymmetric nodal expression in the chick as supported both by the spatial relationship between shh and nodal domains as well as by functional studies (cf. Supplementary Table S2) that indicate the involvement of shh or hedgehog signaling for nodal activation in the paraxial and LPM-domains (Levin et al., 1995; Pagan-Westphal and Tabin, 1998; Otto et al., 2014). The aim of our study was to test the spatio-temporal effects of global activation of hedgehog signaling in the framework of the early left–right patterning. Treatment with smoothened agonist SAG which activates hedgehog signaling caused additional nodal expression on the right side, hence mirroring the left-sided domain, elevating expression intensity and leading to decoupling of initiation of nodal in LPM from the beginning of somitogenesis. A medial sector of widened nodal expression at stage 5 after SAG treatment overlaps with the expression of the somitic mesoderm marker paraxis at stage 5 (not shown), indicating a nodal induction in the prospective somitic mesoderm. Right-sided nodal expression correlates with the right-sided expression of pitx2 in LPM. Furthermore, the SAG treatment interferes with cardiac development. While inhibition and local activation of hedgehog signaling lead to randomization of cardiac looping (Levin et al., 1997; Logan et al., 1998; Otto et al., 2014), the global activation leads to both randomization and severe deformations associated with disturbed closure of the intestinal portal. The latter may be explained by the involvement of shh, which is expressed in the endoderm (Narita et al., 1998), in the morphogenesis of the intestinal portal.
Our results argue in favor of a crucial role of competence in the nodal induction during chick left–right patterning: despite the treatment of the whole embryos with SAG, the ectopic nodal expression was confined to the paraxial and the lateral plate mesoderm. As suggested previously, the left paraxial nodal domain is most likely induced by shh from the floor plate above (Supplementary Figure S1). This induction, however, is specifically constrained: particularly, SAG-treated embryos reveal at the right side a competent domain mirroring the left domain. The concept of embryonic competence describes the ability or predisposition of the embryonic tissue to produce a specific response to a certain signal (Waddington, 1932; Waddington, 1936; Gurdon, 1987). The theoretical significance of competence lies in the fact that it points to the not yet discovered layer of interactions (Tsikolia, 2006). It may not only rely on the pattern of cellular receptivity on different levels but sensu lato also on specific transport and degradation of signaling molecules, enabling a local response to the global cue. The mechanism of predisposition of a certain mesodermal domain at the right side to respond to hedgehog activity with the nodal expression remains to be clarified: reported expression of the hedgehog pathway components gli or smoothened only partially overlaps with the prospective nodal expression domain. Further studies should clarify the basis of competence as well as the question of whether the lateral plate mesoderm in the chick is induced solely by a signal from the paraxial domain or possesses the ability to express nodal autonomously.
SAG has been used as a hedgehog activator in different models (Bragina et al., 2010; Meinhardt et al., 2014; Todd and Fischer, 2015) and was suggested to be highly specific (Lewis and Krieg, 2014). Although the question concerning the specificity of reported SAG effect on nodal expression cannot be definitively resolved here, the complementary effects of hedgehog suppression that led to efficient nodal inhibition and of proposed hedgehog activation by SAG which induced nodal, strongly support the specific involvement of hedgehog activation in the reported effect. Furthermore, the toxicity of SAG treatment until the organogenesis stages was low.
Activation of hedgehog signaling in our study led to different effects in chick and Xenopus embryos. This functional divergence is in line with previously published data from other organisms. In the sea urchin, the inhibition of hedgehog signaling by cyclopamine was shown to suppress both asymmetric nodal and pitx2 expression (Warner et al., 2016). In amphioxus, the downregulation of hedgehog signaling causes bilateral nodal expression (Hu et al., 2017), while the upregulation leads to bilateral expression of nodal antagonist DAND5 (Zhu et al., 2020). Interestingly, the asymmetric hedgehog activity in amphioxus was proposed to be caused by cilia-driven transport of hedgehog protein. Mouse embryos lacking smoothened or both shh and its relative Indian hedgehog (Ihh) do not express nodal in the lateral plate mesoderm (Tsiairis and McMahon, 2009). However, other studies reported bilateral symmetrical nodal expression in mouse embryos lacking shh alone (Tsukui et al., 1999), indicating a possible feedback loop between shh and Ihh in the mouse. Moreover, mouse embryos lacking both smoothened and hedgehog repressor Gli3 display a correct left-sided pitx2 expression, indicating a functional redundancy (Tsiairis and McMahon, 2009). Hence, detailed investigation of the exact mode of hedgehog activity in organisms with the leftward flow like Xenopus, mouse, or D. rerio can uncover unexpected levels of regulation.
To sum up, combined empirical evidence (cf. Supplementary Table S2) reveals that in the chick embryos, the hedgehog signaling is both necessary (Pagan-Westphal and Tabin, 1998; Otto et al., 2014) and sufficient (Levin et al., 1995 and this work) for initiation of nodal expression at stage 5 in the competent paraxial domain. We suggest that this interaction is local and the asymmetry of nodal is achieved by the left-sided position of the posterior floor plate (Supplementary Figure S1).
Furthermore, our data support the evolutionary divergence of shh-nodal interaction (Supplementary Figure S2) and provide an efficient model of stable symmetrization of nodal gene expression in the chick embryo.
Data availability statement
The original contributions presented in the study are included in the article/Supplementary Material; further enquiries can be directed to the corresponding author.
Ethics statement
The animal study was reviewed and approved by the Bioethics Committee of Koltsov Institute of Developmental Biology of the Russian Academy of Science.
Author contributions
MN: chicken data acquisition, data analysis, and writing the manuscript; NB chicken data acquisition; SK: Xenopus data acquisition, and conceptualization; NP Xenopus data acquisition and data analysis; and NT: project design, conceptualization, and writing the manuscript.
Funding
The work of SK was supported by the funding No. 0088-2021-0009 of the Koltzov Institute of Developmental Biology.
Acknowledgments
We thank Kirsten Falk-Stietenroth, Heike Faust, and Hans-Georg Sydow for their excellent technical assistance, Christoph Viebahn for fruitful discussions and Jörg Männer for advice in interpreting cardiac malformations. Cliff Tabin generously provided cDNA probes. We acknowledge support by the Open Access Publication Funds of the Göttingen University.
Conflict of interest
The authors declare that the research was conducted in the absence of any commercial or financial relationships that could be construed as a potential conflict of interest.
Publisher’s note
All claims expressed in this article are solely those of the authors and do not necessarily represent those of their affiliated organizations, or those of the publisher, the editors, and the reviewers. Any product that may be evaluated in this article, or claim that may be made by its manufacturer, is not guaranteed or endorsed by the publisher.
Supplementary material
The Supplementary Material for this article can be found online at: https://www.frontiersin.org/articles/10.3389/fcell.2022.957211/full#supplementary-material
SUPPLEMENTARY TABLE S1 | Left–right patterning in SAG-treated embryos and control: nodal and (A) pitx2 (B) expression.
SUPPLEMENTARY TABLE S2 | Hedgehog signaling in the chick left–right patterning: compilation of previous results concerning the role of hedgehog signaling in left–right patterning in comparison to the present report.
SUPPLEMENTARY FIGURE S1 | Proposed mechanism of molecular left‐right patterning as a consequence of asymmetric node morphogenesis in the chick. (A): Schematic dorsal view of chick embryo at early stage 5 after node rotation. (B,C): Transversal sections at levels shown in (A). The notochord is marked in red, the floor plate is labeled in turquoise and blue indicates the paraxial nodal domain. Dashed arrows in (A) indicate a shift of the node during notochord elongation and concomitant streak regression, black arrow in (C) indicates the proposed induction of the paraxial nodal expression by shh from the notochord, green arrow in (C) indicates the proposed subsequent induction of nodal expression in the prospective lateral plate mesoderm (lpm) by nodal ligand from the paraxial domain. A is modified version of Figure 7B published in (Kremnyov et al., 2018).
SUPPLEMENTARY FIGURE S2 | Hedgehog signaling and left‐right patterning in Deuterostomia. The red font is used for observed stimulating effect which is subdivided into “sufficient” and “required” (necessary) for nodal expression while the blue font is used for reported inhibition of nodal domain.
SUPPLEMENTARY DATA SHEET 1 | Supplementary Methods
References
Afzelius, B. A., and Stenram, U. (2006). Prevalence and genetics of immotile-cilia syndrome and left-handedness. Int. J. Dev. Biol. 50 (6), 571–573. doi:10.1387/ijdb.052132ba
Blum, M., Beyer, T., Weber, T., Vick, P., Andre, P., Bitzer, E., et al. (2009). Xenopus, an ideal model system to study vertebrate left-right asymmetry. Dev. Dyn. 238 (6), 1215–1225. doi:10.1002/dvdy.21855
Blum, M., and Ott, T. (2018). Animal left-right asymmetry. Curr. Biol. 28 (7), R301–R304. doi:10.1016/j.cub.2018.02.073
Bragina, O., Sergejeva, S., Serg, M., Zarkovsky, T., Maloverjan, A., Kogerman, P., et al. (2010). Smoothened agonist augments proliferation and survival of neural cells. Neurosci. Lett. 482 (2), 81–85. doi:10.1016/j.neulet.2010.06.068
Campione, M., Steinbeisser, H., Schweickert, A., Deissler, K., van BebberF., , Lowe, L. A., et al. (1999). The homeobox gene Pitx2: Mediator of asymmetric left-right signaling in vertebrate heart and gut looping. Development 126 (6), 1225–1234. doi:10.1242/dev.126.6.1225
Cohen, M., Kicheva, A., Ribeiro, A., Blassberg, R., Page, K. M., Barnes, C. P., et al. (2015). Ptch1 and Gli regulate Shh signalling dynamics via multiple mechanisms. Nat. Commun. 6, 6709. doi:10.1038/ncomms7709
Cui, C., Little, C. D., and Rongish, B. J. (2009). Rotation of organizer tissue contributes to left-right asymmetry. Anat. Rec. 292 (4), 557–561. doi:10.1002/ar.20872
Datar, A., Nandi, S., Julicher, F., and Grill, S. (2017). Left-right symmetry breaking during early development of C. elegans. Biophysical J. 112 (3), 434a. doi:10.1016/j.bpj.2016.11.2319
Echelard, Y., Epstein, D. J., St-Jacques, B., Shen, L., Mohler, J., McMahon, J. A., et al. (1993). Sonic hedgehog, a member of a family of putative signaling molecules, is implicated in the regulation of CNS polarity. Cell 75 (7), 1417–1430. doi:10.1016/0092-8674(93)90627-3
Essner, J. J., Amack, J. D., Nyholm, M. K., Harris, E. B., and Yost, H. J. (2005). Kupffer's vesicle is a ciliated organ of asymmetry in the zebrafish embryo that initiates left-right development of the brain, heart and gut. Development 132 (6), 1247–1260. doi:10.1242/dev.01663
Grande, C., and Patel, N. H. (2009). Nodal signalling is involved in left-right asymmetry in snails. Nature 457 (7232), 1007–1011. doi:10.1038/nature07603
Gros, J., Feistel, K., Viebahn, C., Blum, M., and Tabin, C. J. (2009). Cell movements at Hensen's node establish left/right asymmetric gene expression in the chick. Science 324 (5929), 941–944. doi:10.1126/science.1172478
Gurdon, J. B. (1987). Embryonic induction--molecular prospects. Development 99 (3), 285–306. doi:10.1242/dev.99.3.285
Hamada, H., and Tam, P. (2020). Diversity of left-right symmetry breaking strategy in animals. F1000Res 9, F1000. doi:10.12688/f1000research.21670.1
Hamburger, V., and Hamilton, H. L. (1951). A series of normal stages in the development of the chick embryo. J. Morphol. 88, 49–92. doi:10.1002/jmor.1050880104
Harland, R. M. (1991). In situ hybridization: An improved whole-mount method for Xenopus embryos. Methods Cell Biol. 36, 685–695. doi:10.1016/s0091-679x(08)60307-6
Hu, G., Li, G., Wang, H., and Wang, Y. (2017). Hedgehog participates in the establishment of left-right asymmetry during amphioxus development by controlling Cerberus expression. Development 144 (24), 4694–4703. doi:10.1242/dev.157172
Iulianella, A., Sakai, D., Kurosaka, H., and Trainor, P. A. (2018). Ventral neural patterning in the absence of a Shh activity gradient from the floorplate. Dev. Dyn. 247 (1), 170–184. doi:10.1002/dvdy.24590
Johnson, R. L., LaufEr, E., Riddle, R. D., and Tabin, C. (1994). Ectopic expression of Sonic hedgehog alters dorsal-ventral patterning of somites. Cell 79 (7), 1165–1173. doi:10.1016/0092-8674(94)90008-6
Kajikawa, E., Horo, U., Ide, T., Mizuno, K., Minegishi, K., Hara, Y., et al. (2020). Nodal paralogues underlie distinct mechanisms for visceral left-right asymmetry in reptiles and mammals. Nat. Ecol. Evol. 4 (2), 261–269. doi:10.1038/s41559-019-1072-2
Kay, B. K., and Peng, H. B. (1992). Xenopus laevis: Practical uses in cell and molecular biology. Academic Press.
Kremnyov, S., Henningfeld, K., Viebahn, C., and Tsikolia, N. (2018). Divergent axial morphogenesis and early shh expression in vertebrate prospective floor plate. Evodevo 9, 4. doi:10.1186/s13227-017-0090-x
Lebreton, G., Geminard, C., LaprazF., , PyrpaSSopouloS, S., Cerezo, D., SPeder, P., et al. (2018). Molecular to organismal chirality is induced by the conserved myosin 1D. Science 362 (6417), 949–952. doi:10.1126/science.aat8642
Levin, M., Johnson, R. L., Stern, C. D., KuehnM., , and Tabin, C. (1995). A molecular pathway determining left-right asymmetry in chick embryogenesis. Cell 82 (5), 803–814. doi:10.1016/0092-8674(95)90477-8
Levin, M., Pagan, S., Roberts, D. J., Cooke, J., Kuehn, M. R., and Tabin, C. J. (1997). Left/right patterning signals and the independent regulation of different aspects of situs in the chick embryo. Dev. Biol. 189 (1), 57–67. doi:10.1006/dbio.1997.8662
Lewis, C., and Krieg, P. A. (2014). Reagents for developmental regulation of Hedgehog signaling. Methods 66 (3), 390–397. doi:10.1016/j.ymeth.2013.08.022
Lobikin, M., Wang, G., Xu, J., Hsieh, Y. W., Chuang, C. F., Lemire, J. M., et al. (2012). Early, nonciliary role for microtubule proteins in left-right patterning is conserved across kingdoms. Proc. Natl. Acad. Sci. U. S. A. 109 (31), 12586–12591. doi:10.1073/pnas.1202659109
Logan, M., Pagan-Westphal, S. M., Smith, D. M., Paganessi, L., and Tabin, C. J. (1998). The transcription factor Pitx2 mediates situs-specific morphogenesis in response to left-right asymmetric signals. Cell 94 (3), 307–317. doi:10.1016/s0092-8674(00)81474-9
Lombardo, V. A., Heise, M., Moghtadaei, M., Bornhorst, D., Manner, J., and Abdelilah-Seyfried, S. (2019). Morphogenetic control of zebrafish cardiac looping by Bmp signaling. Development 146 (22), dev180091. doi:10.1242/dev.180091
Maerker, M., Getwan, M., Dowdle, M. E., McSheene, J. C., Gonzalez, V., Pelliccia, J. L., et al. (2021). Bicc1 and Dicer regulate left-right patterning through post-transcriptional control of the Nodal inhibitor Dand5. Nat. Commun. 12 (1), 5482. doi:10.1038/s41467-021-25464-z
Manner, J. (2001). Does an equivalent of the "ventral node" exist in chick embryos? A scanning electron microscopic study. Anat. Embryol. 203 (6), 481–490. doi:10.1007/s004290100183
Manner, J. (2009). The anatomy of cardiac looping: A step towards the understanding of the morphogenesis of several forms of congenital cardiac malformations. Clin. Anat. 22 (1), 21–35. doi:10.1002/ca.20652
Matsui, T., and Bessho, Y. (2012). Left-right asymmetry in zebrafish. Cell Mol. Life Sci. 69, 3069–3077. doi:10.1007/s00018-012-0985-6
Meinhardt, A., Eberle, D., Tazaki, A., Ranga, A., Niesche, M., Wilsch-Brauninger, M., et al. (2014). 3D reconstitution of the patterned neural tube from embryonic stem cells. Stem Cell Rep. 3 (6), 987–999. doi:10.1016/j.stemcr.2014.09.020
Motoyama, J., Milenkovic, L., Iwama, M., Shikata, Y., Scott, M. P., and Hui, C. c. (2003). Differential requirement for Gli2 and Gli3 in ventral neural cell fate specification. Dev. Biol. 259 (1), 150–161. doi:10.1016/s0012-1606(03)00159-3
Narita, T., Ishii, Y., Nohno, T., Noji, S., and YaSugi, S. (1998). Sonic hedgehog expression in developing chicken digestive organs is regulated by epithelial-mesenchymal interactions. Dev. Growth Differ. 40 (1), 67–74. doi:10.1046/j.1440-169x.1998.t01-5-00008.x
Nieuwkoop, P. D., and Faber, J. (1994). Normal table of Xenopus laevis (daudin). New York: Garland Publishing Inc.
Nonaka, S., Yoshiba, S., Watanabe, D., Ikeuchi, S., Goto, T., Marshall, W. F., et al. (2005). De novo formation of left-right asymmetry by posterior tilt of nodal cilia. PLoS Biol. 3 (8), e268. doi:10.1371/journal.pbio.0030268
Onjiko, R. M., Morris, S. E., Moody, S. A., and Nemes, P. (2016). Single-cell mass spectrometry with multi-solvent extraction identifies metabolic differences between left and right blastomeres in the 8-cell frog (Xenopus) embryo. Analyst 141 (12), 3648–3656. doi:10.1039/c6an00200e
Otto, A., Pieper, T., Viebahn, C., and Tsikolia, N. (2014). Early left-right asymmetries during axial morphogenesis in the chick embryo. Genesis 52 (6), 614–625. doi:10.1002/dvg.22773
Pagan-Westphal, S. M., and Tabin, C. J. (1998). The transfer of left-right positional information during chick embryogenesis. Cell 93 (1), 25–35. doi:10.1016/s0092-8674(00)81143-5
Patel, K., Isaac, A., and Cooke, J. (1999). Nodal signalling and the roles of the transcription factors SnR and Pitx2 in vertebrate left-right asymmetry. Curr. Biol. 9 (11), 609–612. doi:10.1016/s0960-9822(99)80267-x
Pieper, T., Carpaij, M., Reinermann, J., Surchev, L., Viebahn, C., and Tsikolia, N. (2020). Matrix-filled microcavities in the emerging avian left-right organizer. Dev. Dyn. 249 (4), 496–508. doi:10.1002/dvdy.133
Rebagliati, M. R., Toyama, R., FriCke, C., Haffter, P., and Dawid, I. B. (1998). Zebrafish nodal-related genes are implicated in axial patterning and establishing left-right asymmetry. Dev. Biol. 199 (2), 261–272. doi:10.1006/dbio.1998.8935
Ribes, V., Balaskas, N., Sasai, N., Cruz, C., Dessaud, E., Cayuso, J., et al. (2010). Distinct Sonic Hedgehog signaling dynamics specify floor plate and ventral neuronal progenitors in the vertebrate neural tube. Genes Dev. 24 (11), 1186–1200. doi:10.1101/gad.559910
Roelink, H., Augsburger, A., Heemskerk, J., Korzh, V., Norlin, S., Ruiz i AltAbA, A., et al. (1994). Floor plate and motor neuron induction by vhh-1, a vertebrate homolog of hedgehog expressed by the notochord. Cell 76 (4), 761–775. doi:10.1016/0092-8674(94)90514-2
Schroder, S. S., Tsikolia, N., Weizbauer, A., Hue, I., and Viebahn, C. (2016). Paraxial nodal expression reveals a novel conserved structure of the left-right organizer in four mammalian species. Cells Tissues Organs 201 (2), 77–87. doi:10.1159/000440951
Schweickert, A., Vick, P., Getwan, M., Weber, T., Schneider, I., Eberhardt, M., et al. (2010). The nodal inhibitor Coco is a critical target of leftward flow in Xenopus. Curr. Biol. 20 (8), 738–743. doi:10.1016/j.cub.2010.02.061
Schweickert, A., Weber, T., Beyer, T., Vick, P., Bogusch, S., Feistel, K., et al. (2007). Cilia-driven leftward flow determines laterality in Xenopus. Curr. Biol. 17 (1), 60–66. doi:10.1016/j.cub.2006.10.067
Stephen, L. A., Johnson, E. J., Davis, G. M., McTeir, L., Pinkham, J., Jaberi, N., et al. (2014). The chicken left right organizer has nonmotile cilia which are lost in a stage-dependent manner in the talpid(3) ciliopathy. Genesis 52 (6), 600–613. doi:10.1002/dvg.22775
Sydow, H. G., Pieper, T., Viebahn, C., and Tsikolia, N. (2017). An early chick embryo culture device for extended continuous observation. Methods Mol. Biol. 1650, 309–317. doi:10.1007/978-1-4939-7216-6_21
Szenker-Ravi, E., Ott, T., Khatoo, M., de Bellaing, A. M., Goh, W. X., Chong, Y. L., et al. (2022). Discovery of a genetic module essential for assigning left-right asymmetry in humans and ancestral vertebrates. Nat. Genet. 54 (1), 62–72. doi:10.1038/s41588-021-00970-4
Tisler, M., Wetzel, F., Mantino, S., Kremnyov, S., Thumberger, T., Schweickert, A., et al. (2016). Cilia are required for asymmetric nodal induction in the sea urchin embryo. BMC Dev. Biol. 16 (1), 28. doi:10.1186/s12861-016-0128-7
Todd, L., and Fischer, A. J. (2015). Hedgehog signaling stimulates the formation of proliferating Muller glia-derived progenitor cells in the chick retina. Development 142 (15), 2610–2622. doi:10.1242/dev.121616
Tsiairis, C. D., and McMahon, A. P. (2009). An Hh-dependent pathway in lateral plate mesoderm enables the generation of left/right asymmetry. Curr. Biol. 19 (22), 1912–1917. doi:10.1016/j.cub.2009.09.057
Tsikolia, N., Schroder, S., Schwartz, P., and Viebahn, C. (2012). Paraxial left-sided nodal expression and the start of left-right patterning in the early chick embryo. Differentiation. 84 (5), 380–391. doi:10.1016/j.diff.2012.09.001
Tsikolia, N. (2006). The role and limits of a gradient based explanation of morphogenesis: A theoretical consideration. Int. J. Dev. Biol. 50 (2-3), 333–340. doi:10.1387/ijdb.052053nt
Tsukui, T., Capdevila, J., Tamura, K., Ruiz-Lozano, P., Rodriguez-Esteban, C., Yonei-Tamura, S., et al. (1999). Multiple left-right asymmetry defects in Shh(-/-) mutant mice unveil a convergence of the shh and retinoic acid pathways in the control of Lefty-1. Proc. Natl. Acad. Sci. U. S. A. 96 (20), 11376–11381. doi:10.1073/pnas.96.20.11376
Vandenberg, L. N., Lemire, J. M., and Levin, M. (2013). Serotonin has early, cilia-independent roles in Xenopus left-right patterning. Dis. Model. Mech. 6 (1), 261–268. doi:10.1242/dmm.010256
Waddington, C. H. (1932). Experiments on the development of chick and duck embryos, cultivated in vitro. Philosophical Trans. R. Soc. Lond. Ser. B, Contain. Pap. a Biol. Character 221, 179–230. doi:10.1098/rstb.1932.0003
Waddington, C. H. (1936). The origin of competence for lens formation in the Amphibia. J. Exp. Biol. 13 (1), 86–91. doi:10.1242/jeb.13.1.86
Warner, J. F., Miranda, E. L., and McClay, D. R. (2016). Contribution of hedgehog signaling to the establishment of left-right asymmetry in the sea urchin. Dev. Biol. 411 (2), 314–324. doi:10.1016/j.ydbio.2016.02.008
Weisheit, G., Mertz, D., Schilling, K., and Viebahn, C. (2002). An efficient in situ hybridization protocol for multiple tissue sections and probes on miniaturized slides. Dev. Genes Evol. 212 (8), 403–406. doi:10.1007/s00427-002-0258-8
Keywords: left–right (LR) asymmetry, chick embryo, nodal, hedgehog signaling, heart looping, Xenopus laevis embryo, embryonic competence
Citation: Negretti MI, Böse N, Petri N, Kremnyov S and Tsikolia N (2022) Nodal asymmetry and hedgehog signaling during vertebrate left–right symmetry breaking. Front. Cell Dev. Biol. 10:957211. doi: 10.3389/fcell.2022.957211
Received: 30 May 2022; Accepted: 04 August 2022;
Published: 12 September 2022.
Edited by:
Myriam Roussigne, CNRS/Toulouse University UMR5077louse, FranceReviewed by:
Sally Ann Moody, George Washington University, United StatesMichael Levin, Tufts University, United States
Copyright © 2022 Negretti, Böse, Petri, Kremnyov and Tsikolia. This is an open-access article distributed under the terms of the Creative Commons Attribution License (CC BY). The use, distribution or reproduction in other forums is permitted, provided the original author(s) and the copyright owner(s) are credited and that the original publication in this journal is cited, in accordance with accepted academic practice. No use, distribution or reproduction is permitted which does not comply with these terms.
*Correspondence: Nikoloz Tsikolia, nikoloz.tsikolia@med.uni-goettingen.de