- 1Xiangya School of Medicine, Central South University, Changsha, China
- 2Department of Orthopedics, Xiangya Hospital, Central South University, Changsha, China
- 3Department of Clinical Nursing, Xiangya Hospital, Central South University, Changsha, China
- 4Department of Orthopaedics, Shandong Provincial Hospital Affiliated to Shandong First Medical University, Jinan, China
- 5National Clinical Research Center for Geriatric Disorders, Xiangya Hospital, Central South University, Changsha, China
Osteoarthritis (OA) has remained a prevalent public health problem worldwide over the past decades. OA is a global challenge because its specific pathogenesis is unclear, and no effective disease-modifying drugs are currently available. Exosomes are small and single-membrane vesicles secreted via the formation of endocytic vesicles and multivesicular bodies (MVBs), which are eventually released when MVBs fuse with the plasma membrane. Exosomes contain various integral surface proteins derived from cells, intercellular proteins, DNAs, RNAs, amino acids, and metabolites. By transferring complex constituents and promoting macrophages to generate chemokines and proinflammatory cytokines, exosomes function in pathophysiological processes in OA, including local inflammation, cartilage calcification and degradation of osteoarthritic joints. Exosomes are also detected in synovial fluid and plasma, and their levels continuously change with OA progression. Thus, exosomes, specifically exosomal miRNAs and lncRNAs, potentially represent multicomponent diagnostic biomarkers for OA. Exosomes derived from various types of mesenchymal stem cells and other cell or tissue types affect angiogenesis, inflammation, and bone remodeling. These exosomes exhibit promising capabilities to restore OA cartilage, attenuate inflammation, and balance cartilage matrix formation and degradation, thus demonstrating therapeutic potential in OA. In combination with biocompatible and highly adhesive materials, such as hydrogels and cryogels, exosomes may facilitate cartilage tissue engineering therapies for OA. Based on numerous recent studies, we summarized the latent mechanisms and clinical value of exosomes in OA in this review.
Introduction
Osteoarthritis (OA) remains a leading joint disease in the aging population as well as the major cause of chronic pain and disability worldwide (Helmick et al., 2008; Hunter et al., 2014; Prieto-Alhambra et al., 2014). Whole joint structures deteriorate in this degenerative articular disorder, including synovium, ligaments, subchondral bone, articular cartilage and periarticular muscles (Brandt et al., 2006). Although the local cartilage damage is the fundamental pathological change of knee OA, the pathogenesis and epidemiology of OA are not completely clear, but multifactorial genetic, biological, and biomechanical factors are implicated. Current conservative treatments can only temporarily relieve osteoarthritic symptoms and partially delay the progression of OA (Crawford et al., 2013), and effective disease-modifying drugs are not available. Total knee arthroplasty (TKA) is a relatively effective treatment modality targeting advanced OA in clinical practice. However, the lifespan of prostheses is limited, and patient quality of life is inevitably affected (Kester et al., 2016). Therefore, studies are needed to further investigate definite mechanisms and effective therapeutic strategies.
Exosomes are extracellular vesicles (EVs) secreted by ubiquitous cells and carry various cellular constituents (Kalluri and LeBleu, 2020). Once released, exosomes are capable of remodeling the extracellular matrix and transmitting signaling molecules via the transport of biological cargo and the accumulation of specific cellular components. The diagnostic and therapeutic applications of exosomes in various diseases involving cardiovascular diseases, neurodegenerative diseases and cancer, are mainly attributed to their bioactive contents (Kalluri and LeBleu, 2020). Moreover, the inner cargo constituents of exosomes are available by means of biological fluid (liquid biopsy) sampling, which highlights their potential roles in the diagnosis of various diseases and in determining patient prognosis (Kalluri and LeBleu, 2020).
Exosomes have been detected in osteoarthritic synovial fluid and have emerged to play pivotal roles in the pathogenesis and therapy of OA (György et al., 2012; Headland et al., 2015). Different types of mesenchymal stem cell (MSC)-derived exosomes potentially serve as a novel approach for OA treatment based on the potent proliferation and differentiation abilities of multipotent stem cells (Asghar et al., 2020). Recent studies have demonstrated that MSC-derived exosomes alleviate knee OA and temporomandibular joint OA by alleviating inflammation and restoring matrix homeostasis (Zhang et al., 2019; Li et al., 2020). Various MSC-based therapies are in clinical trials for tissue regeneration of bone and cartilage, and exosomes derived from bone mesenchymal stem cells (BMSCs), synovial mesenchymal stem cells (SMSCs), adipose tissue mesenchymal stem cells (AMSCs), human embryonic stem cells (hESCs) and other stem cells may also function as potential therapeutic targets for OA (Ni et al., 2020). Additionally, the content of exosomes also dynamically varies during OA progression (Ruivo et al., 2017; Tai et al., 2018). Specifically, microRNAs (miRNAs), long noncoding RNAs (lncRNAs) and circular RNAs (circRNAs) can be transferred from MSCs to osteoarthritic cells through exosomes to treat OA. However, these studies are still in the primary research stage; thus, exosomes have not been applied in the clinical treatment of OA. MSC-Exos, as a new engineering technology, are gradually becoming a promising strategy for OA (Ni et al., 2020). In this review, we further shed light on the underlying biofunctions and therapeutic potential of exosomes in OA.
Exosomes
Biogenesis of exosomes
The biogenic process of exosome formation is initiated by a double invagination of the plasma membrane. Then, multivesicular bodies (MVBs) carrying intraluminal vesicles (ILVs) form and fuse with the cell membrane and are ultimately secreted as exosomes through exocytosis (Kalluri and LeBleu, 2020). Extracellular constituents and integral proteins on the cell surface access cells by means of endocytosis and invagination of the cell membrane to form early-sorting endosomes (ESEs). Subsequently, the ESEs combine with the endoplasmic reticulum (ER) and trans-Golgi network (TGN) or the constituents of the ER and TGN. Therefore, some ESEs serve as carriers of membrane and luminal constituents and subsequently form late-sorting endosomes (LSEs) (Kalluri, 2016; Hessvik and Llorente, 2018; van Niel et al., 2018; Willms et al., 2018; Mathieu et al., 2019; McAndrews and Kalluri, 2019). The ILVs are generated by the second invagination, and the cargo of exosomes is also modified simultaneously. Eventually, with the specific accumulation of ILVs, MVBs are either degraded by the lysosomes or transported to the cell membrane, contributing to the secretion of exosomes with a lipid bilayer orientation comparable to that of the cell membrane (Kahlert and Kalluri, 2013; van Niel et al., 2018). Various other specific vesicular activities within cells and other influencing factors, such as autophagy, culture systems, lysosomal pathways, Golgi apparatus–derived vesicle trafficking cell types, and genomic conditions, may affect the biogenic process as well (Mathieu et al., 2019).
Biofunctions of exosomes
Studies have demonstrated that exosomes exert vital functions in numerous biological activities, including angiogenesis, apoptosis, antigen presentation, intercellular signaling and inflammation, partially by utilizing the capability to transport RNA, enzymes, proteins, and lipids. Thus, exosomes influence the physiology and pathology of different diseases, such as atherosclerosis, diabetes-related cardiovascular disease, breast and pancreatic cancers, neurodegenerative diseases and OA (Gurunathan et al., 2019). The biofunctions of exosomes are mainly attributed to the inner composition and the phenotypical and molecular changes they exert on recipient cells via intercellular communication (Kalluri and LeBleu, 2020). For example, exosomes carrying tumor-specific RNAs, such as circulating miR-141 and miR-375, can be utilized for the diagnosis of prostate cancer (Mitchell et al., 2008; Silva et al., 2011). Serum exosomes loaded with proteoglycan glypican-1 (GP1) can serve as diagnostic markers for pancreatic cancer (Melo et al., 2015). Plasma exosomes isolated from the pleural effusions of lung cancer patients contain higher levels of CD151, CD171, and tetraspanin8, which represent promising biomarkers for lung cancer diagnosis (Li et al., 2021b). In addition, emerging studies have reported that by incorporating small molecules or nucleic acid drugs into exosomes and subsequently transporting them to specific types of cells or tissue, exosomes can be engineered for targeted drug delivery, providing new insights for cell-free therapies for a variety of diseases (Liang et al., 2021). However, the most significant role of exosomes involves use as a “vehicle” for intercellular communications, in which cargo contents are transferred to recipient cells (Lee et al., 2020). The cargo composition of exosomes is dependent on parent cells, however, regardless of the cell origin, exosomes carry some common components, such as heat shock proteins, tetraspanin, nucleic acids, membrane transportation and fusion proteins, biosynthesis-related proteins and lipids (Colombo et al., 2014; Choi et al., 2015). The biofunctions of exosomes in intercellular signaling can be made more complex due to the specific mechanisms and pathways involved in exosome uptake and the specific putative mechanisms of exosomes derived from distinct cell types. (Mulcahy et al., 2014; Mathieu et al., 2019).
In addition, exosomes play essential roles in inflammatory processes. Ridder et al. (Ridder et al., 2014) found that exosomes delivered mRNAs to recipient cells, and the process is facilitated by the stimulation of immune cells that produced exosomes of severe inflammation (peritonitis) or chronic inflammation (subcutaneous tumor). In another study, exosomes suppressed the immune response through the transfer of miRNAs, presenting of molecules to regulate the immune response, including programmed death-ligand 1 (PD-L1) and Fas ligand (FasL), on their surface (Chen et al., 2018). Moreover, circulating exosomes stimulate the release of proinflammatory cytokines, such as interleukin 6 (IL-6), tumor necrosis factor alpha (TNFα), granulocyte colony-stimulating factors, and chemokine C-C motif ligand 2 (CCL2) (Chow et al., 2014).
Exosomes also play vital roles in angiogenesis, which is associated with differentiation, neovascularization, promoted renewal of blood flow, and capillary network construction by loading a spectrum of miRNAs, including miR210, miR126, miR132, and miR21, from MSCs (Chen et al., 2010; Ma et al., 2018). In addition, exosomes also participate in apoptosis. Dying cells release apoptotic bodies as products of apoptotic cell disassembly, and the apoptotic bodies finally become one of the major types of EVs (Caruso and Poon, 2018). Sun et al. (2019) found that miR-664-5p transferred through BMSC-derived exosomes impaired the apoptotic death of ovarian granulosa cells by targeting p53. Tao et al. (2017a) adopted a dexamethasone (DEX)-treated cell model in vitro and methylprednisolone (MPS)-treated in vivo rat model and drew the conclusion that exosomes derived from human platelet-rich plasma prevented apoptosis through the AKT/BAD/BCL-2 signaling pathway.
Based on the abundant biofunctions of exosomes, exosomes may be applied in new and innovative approaches for the diagnosis and treatment of OA.
Exosomes in various diseases
Studies have confirmed that exosomes function in the emergence of metabolic diseases and cardiovascular health. Cancer cell-derived exosomes functionally induced the progression of cachexia and paraneoplastic syndrome by changing the metabolism of normal cells, including adipocytes and pancreatic islet cells. Platelet-derived exosomes function to reduce the expression level of the macrophage scavenger receptor CD36, thus reducing the intake of harmful cholesterol to influence atherosclerosis (Srikanthan et al., 2014). In addition, exosomes are related to some symbolic characteristics of cancer, which influence neoplasia, metastasis, paraneoplastic syndromes, tumor growth, and resistance to therapy (Hanahan and Weinberg, 2011). Moreover, exosomes may participate in detoxification and neuroprotective processes in neurodegenerative diseases by affecting the collection of unfolded and abnormally folded proteins in the brain and play roles in the clearance of misfolded proteins. Intercellular communication through exosomes including α-synuclein, amyloid β, and prions, leads to inflammatory signaling in Parkinson’s, Alzheimer’s, and Creutzfeldt-Jacob diseases (Li and Barres, 2018). In recent years, the emerging use of MSC-based therapies for OA also contributes to the paracrine secretion of nutritional factors (Toh et al., 2014), especially exosomes. Exosomes derived from MSCs safeguard bone and cartilage against damage in OA by enhancing the expression level of chondrocyte markers including type II collagen and aggrecan; inhibiting inflammatory markers (iNOS); suppressing apoptosis of chondrocytes; stimulating chondrocyte migration and proliferation (Zhu et al., 2017); and inhibiting the activation of macrophages (Cosenza et al., 2017). Therefore, exosomes may participate in the pathogenesis and progression of various diseases including OA.
Exosomes in OA pathogenesis
Exosomes play an essential role in biological processes in OA chondrocytes and relevant inflammatory cells (Tkach and Théry, 2016). Previous studies have revealed that T lymphocytes and macrophages are major inflammatory cells involved in OA (de Lange-Brokaar et al., 2012). Synovial macrophages are mainly located in the synovium lining layer and are stimulated in inflammatory conditions (Haywood et al., 2003). By releasing proinflammatory cytokines, growth factors and enzymes, macrophages participate in the stimulation of angiogenesis, recruitment of leukocytes and lymphocytes, fibroblast proliferation, and protease secretion, thus resulting in joint destruction (Griffin and Scanzello, 2019). Domenis et al. (2017) revealed that synovial fibroblast (SF)-derived exosomes from OA patients facilitated macrophages to generate an array of chemokines and proinflammatory cytokines, such as IL-1β (interleukin-1 beta), CCL (chemokine ligand) 8 (CCL8), CCL15 and CCL20, whereas the release of IL-16, IL-8 and CCL7 was downregulated, resulting in cartilage destruction and local inflammation in joints. However, the underlying mechanisms of how SF-derived exosomes facilitated macrophages to produce these chemokines have not been explored but require further study. Significantly, IL-1β, as an upstream cytokine involved in the local inflammation of OA, enhances the expression and secretion level of other proinflammatory cytokines, such as IL-8 and IL-6, and the production of proteases, mainly matrix metalloproteinases (MMPs). Furthermore, exosomes derived from IL-1β-induced SFB stimulated angiogenic activities, including migration and tube formation of human umbilical vein endothelial cells, are likely involved in the expression of OA-related genes stimulated by exosomes. This process is associated with the increased angiogenesis in osteoarthritic joints. However, the specific pathways involved are unclear (Kato et al., 2014). Moreover, M1 macrophages stimulated by exosomes derived from SF lead to the secretion of MMP12 and MMP7 and the suppression of MMP8 synthesis thus contributing to the degradation of extracellular matrix, in which exosomes may function via specific and alternative pathways. Nevertheless, the specific pathways involved should be further explored (Domenis et al., 2017). Therefore, via the stimulation of M1 macrophages to secrete critical molecules functioning in the inflammatory process and cartilage damage, SF-derived exosomes of inflammatory joints of patients with OA can consequently participate in disease development and trigger and contribute to the extension of the inflammatory process (Buzas et al., 2014; Malda et al., 2016).
Exosomes modulate the biological functions of osteoarthritic cells by transferring miRNAs and lncRNAs as well (Xie et al., 2020). Wu et al. (2019) used a destabilization of the medial meniscus (DMM)-induced OA mouse model and demonstrated that exosomes derived from infrapatellar fat pad (IPFP) MSCs protected articular cartilage from degeneration through relatively complex mechanisms in which exosomal miR-100-5p-modulated suppression of the mTOR-autophagy pathway is involved. In another study, Mao et al. (2018) showed that cartilage development and degradation were mediated by exosomal miR-92a-3p through the inhibition of WNT Family Member 5A (WNT5a) in chondrogenesis and pathogenesis of OA. Yan et al. (2021) utilized an SD rat cartilage defect model in vivo in the experiment and revealed that umbilical cord mesenchymal stem cell (UMSC)-derived exosomal lncRNA H19 promoted the migration of chondrocytes and matrix secretion, and suppressed apoptosis and senescence by acting as a competing endogenous RNA (ceRNA) against miR-29b-3p to increase FoxO3 in chondrocytes. Consequently, given variations in the levels of exosomal miRNAs, these miRNAs have potential to serve as biomarkers for OA diagnosis. In addition, exosomal miRNAs and lncRNAs play a significant role in improving osteochondral activities, demonstrating their therapeutic potential in OA.
Exosomes also contribute to the abnormal calcification and destruction of cartilage in OA. Abnormal calcification of cartilage is a significant pathological change of temporomandibular joint (TMJ) OA. Liu et al. (2022a) explored the connection between chondrocyte-derived exosomes and cartilage calcification and demonstrated that the secretion of exosomes in abnormal TMJ enhanced calcification in degenerative cartilage noted in TMJ OA.
Exosomes may serve as a novel regulatory strategy in OA pathogenesis via excessive catabolism and promotion of chondrocyte apoptosis (Kato et al., 2014). A recent study has found that by downregulating autophagy and p21 expression, vascular endothelial cell-derived exosomes restrained the capability of chondrocytes to defend against oxidative stress, thus increasing the cellular ROS content and contributing to apoptosis. These results indicate that vascular endothelial cell-derived exosomes facilitate OA pathogenesis and promote OA progression (Yang et al., 2021).
Clinical applications of exosomes in OA
Diagnostic potential of exosomes in OA
For the purpose of controlling the development of OA in a timely and effective manner, early-term diagnosis of OA is particularly important (Bhimani et al., 2018). Several biochemical markers for OA diagnosis have been proposed (Glyn-Jones et al., 2015). Currently, to determine the severity and development of OA, researchers mainly concentrate on biomarkers in plasma and synovial fluid that are associated with inflammatory and angiogenetic factors (Lipina et al., 2017). As novel biomarkers, exosomes are expected to be utilitarian as they carry distinct information from secreted cells, including miRNAs and lncRNAs. The expression of some molecules, including both exosomes and exosomal lncRNAs in the blood, is not altered in OA, indicating that OA is a local lesion and is limited to joint synovial fluid (Zhao and Xu, 2018). However, although the production of exosomes in synovial fluid is obviously increased in patients with OA compared with healthy people, no obvious distinction is observed between early-stage OA and advanced OA. To identify osteoarthritic stages of OA, Zhao et al. (Zhao and Xu, 2018) demonstrated that the expression level of synovial fluid-derived exosomal lncRNAs was increased in the primary stage of OA, indicating that lncRNAs are the underlying biomarkers for OA. Exosomal lncRNA prostate-specific transcript 1 (PCGEM1) showed appreciable differences in different stages of OA and was significantly elevated in advanced OA compared with primary OA as well as advanced OA compared with healthy controls, suggesting that lncRNA PCGEM1 from synovial fluid may represent a potent marker for identifying primary OA and advanced OA. In addition, still some studies have revealed that the expression of some exosomal miRNAs is modified in OA patients. Meng et al. (2018) showed that plasma exosomal miR-193b expression was obviously reduced in patients with OA compared with normal controls. In addition, circRNAs represent another family of noncoding RNAs with a closed ring structure in the cytoplasm or exosomes (Li et al., 2015). Studies have increasingly revealed that various circRNAs affect OA progression by interacting with cirRNA/miRNA/mRNA pathways, thereby influencing homeostasis. Therefore, circRNAs including hsa-circ-003213 in peripheral blood and hsa-circ-0104595 in synovial fluid, have the potential to be transported via bodily fluids and could serve as diagnostic biomarkers (Zhang et al., 2021b; Mao et al., 2021) (Table 1).
Therapeutic value of exosomes in OA
Exosomes and cartilage tissue repair
Cartilage is a specialized type of connective tissue consisting of collagen fibers, hyaluronic acid, proteoglycans and chondrocytes (Carballo et al., 2017). Effectual remodeling of impaired cartilage tissues is often limited by the typical avascular structure and restriction in interchanges of signaling molecules, adequate provision of oxygen and nutrients, and penetration of precursor cells (Krishnan and Grodzinsky, 2018). OA induces pathophysiological destruction of the cartilage tissue architecture. After the flaking and cracking of cartilage tissues at OA early stages, the matrix becomes calcified and expands. The matrix ultimately replaces the articular region in the local cartilage tissue, resulting in delamination and the exposure of subchondral bone tissues. Different paracrine signaling pathways in MSC populations induce cartilage tissue regeneration, in which exosomes act as important signaling messengers for intercellular communication and stimulation for cartilage tissue repair (Kim et al., 2020). However, the exact mechanism of physiological responses mediated by exosomes is complicated and involves the induction of chondrocyte proliferation, suppression of the apoptosis of cells in cartilage, downregulation of the levels of proinflammatory synovial cytokines, increased infiltration of M2 macrophages and the regulation of the immune response (Zhang et al., 2018).
Stem cell-derived exosomes in OA
Bone mesenchymal stem cell-derived exosomes
BMSC-Exos accelerate a series of pathophysiological processes, such as angiogenesis and osteogenesis (Zhang et al., 2020). BMSC-Exos considerably facilitate the repair of destroyed cartilage and subchondral bone (Asghar et al., 2020). In a study, Cosenza et al. (Cosenza et al., 2017) revealed that BMSC-Exos upregulated the expression of aggrecan and type II collagen while decreasing the expression levels of MMP-13, a disintegrin and metalloproteinase with thrombospondin motifs 5 (ADAMTS5) and iNOS. Moreover, exosomes from BMSCs treated with TGFβ3 markedly upregulated the expression levels of anabolic marker genes (ACAN, COL1, and COL2B) and downregulated the levels of catabolic and inflammatory marker genes (MMP-13, ADAMTS5, and iNOS) in osteoarthritic chondrocytes (Cosenza et al., 2017). BMSC exosomes also protect chondrocytes from IL-1β-induced apoptosis through the p38, ERK, and AKT pathways (Qi et al., 2019). In addition, chondrocyte proliferation and migration are also promoted by BMSC-Exos. One of the underlying mechanisms is that BMSC-Exos reversed the reduction of collagen type II, SOX9, aggrecanases, and proteoglycan four expression levels induced by OA (Zhou et al., 2020; Xu and Xu, 2021). Furthermore, BMSC-Exos modulate the activities of synovial fibroblasts and macrophages. PTGS2 is one of the target genes of miR-26a-5p, which is significantly upregulated in OA, thus inhibiting PTGS2 and exert a protective effect on OA. BMSC-derived exosomes deliver miR-26a-5p into synovial fibroblasts thus attenuating the impairment of synovial fibroblasts potentially by targeting PTGS2 (Jin et al., 2020) (Fukai et al., 2012). In addition, Raghu et al. (van den Bosch et al., 2016; Raghu et al., 2017) found that monocytes and microphage enhanced local inflammation and tissue damage in OA. BM-MSCs suppress the inflammation of the synovium by inducing the polarization of macrophages toward an anti-inflammatory phenotype (Schelbergen et al., 2014). This process is mediated by PGE2 detected in BMSC-Exos. Current studies have indicated that the production and contents of exosomes are regulated through the drug intervention or gene modification, which may alter the BMSC-Exo cellular effect on targeted cells. Thus exosomes may function as an appropriate therapeutic approach for OA (Gurunathan et al., 2019).
Adipose mesenchymal stem cell-derived exosomes
AMSCs are efficient in regenerating cartilage and regulating inflammatory reactions and are thus considered a preferable cell source for OA treatment (Lee et al., 2019). By modulating the local environment to make cartilage remodeling more advantageous via the paracrine secretion of nutritious molecules, AMSCs inhibit cartilage erosion and enhance joint function (Damia et al., 2018).
Tofiño-Vian et al. (2017) found that the paracrine effects of AMSCs were mediated by exosomes on osteoarthritic osteoblasts. Furthermore, AMSC-derived exosomes impaired the production of proinflammatory mediators in osteoarthritic chondrocytes and increased the levels of anti-inflammatory cytokines IL-10 and collagen II, which are specific to chondrocytes, among which the exosomal component annexin A1 may participate in the anti-inflammatory process (Tofiño-Vian et al., 2018). These results suggest that exosomes derived from AMSCs may become a prospective therapeutic modality for OA.
Synovial mesenchymal stem cell-derived exosomes
SMSCs are a type of articular MSCs found in the synovial fluid filling the joint cavity (Jo et al., 2014; Katare et al., 2014; Lee et al., 2015). SMSCs exhibit an increased ability to differentiate into chondrocytes, but a reduced ability for adipogenic, osteogenic and neurogenic differentiation than other types of MSCs (Xin et al., 2014; Burger et al., 2015). BMSCs and AMSCs have been used for the treatment of OA. However, as synovium and cartilage have the same origin during the development of synovial joints, synovial membrane-derived MSCs (SMMSCs) are especially suitable for cartilage (Archer et al., 2003; Koyama et al., 2008). Given advantageous chondrogenic differentiation capacity in vitro (Shirasawa et al., 2006; Kurth et al., 2007), transplantation of SMSCs has been used for OA treatment (Xu et al., 2021).
Studies by Koizumi et al. (Koizumi et al., 2016; Zhu et al., 2017) have shown that SMSC-derived exosomes significantly facilitated cartilage regeneration and suppressed OA development. Exploiting the WNT5a/WNT5b/YAP pathway, exosomes derived from SMSCs enhanced chondrocyte emigration and proliferations. Nevertheless, these SMSC exosomes inhibited the secretion of extracellular matrix (ECM). Tao et al. (2017b) used miR-140-5p to transfect SMSCs and derived exosomes from SMSCs or SMSC-140s. They found that exosomes treated with miR-140-5p (SMSC-140-Exos) facilitated chondrocyte proliferation and migrations without decreasing ECM secretion in vitro. Experiments in rat models demonstrated that SMMSC-derived exosomes modulated bone regeneration as well (Guo et al., 2016).
Embryonic mesenchymal stem cell-derived exosomes
Exosomes secreted by ESC-MSCs attenuate OA partially by regulating the equilibrium between the production and degeneration of cartilage matrix. Embryonic stem cell-induced mesenchymal stem cells (ESC-MSCs) enhanced Col II expression levels and decreased ADAMTS5 expression in the cartilage matrix (Wang et al., 2017). However, compared with traditional cellular therapies, MSC exosome therapies can be better controlled to reformulate and support different routes of administration. Weekly injection of human embryonic MSC exosomes into articular tissue promoted amelioration of critical-sized osteochondral damage and induced an orderly regeneration of cartilage and subchondral bone in an adult immunocompetent rat model (Zhang et al., 2016). Moreover, in the context of a lack of MHC class I/II proteins, human MSC exosomes can be applied in immunocompetent animals without the need for immunosuppression (Tan et al., 2014). Wang et al. (2017) also demonstrated that the development of cartilage damage in an OA model can be prevented by ESC-MSC-derived exosomes. This effect may be facilitated by direct contact and fusion between chondrocytes and exosomes, with a decrease in the matrix degradation enzyme ADAMTS5 and a balancing increase in the extracellular matrix protein collagen type II. Nevertheless, the mechanisms of EMSC-Exos in the treatment of OA need to be further explored.
Other stem cell-derived exosomes
Liu et al. (2022b) utilized an in vitro IL-1β-induced OA model and an in vivo rat KOA model and concluded that the proliferation and migration capacity of chondrocytes were promoted while apoptosis was effectively reduced after treatment with human urine-derived stem cell (hUSCs)-derived exosomes. Nevertheless, ECM degradation was also aggravated by destroying the constituents of ECM, including collagen II. However, after transfecting with miR-140 and increasing miR-140 expression, the proliferation and migration capability were further enhanced without destroying the ECM compared with hUSC-Exos. Therefore, intra-articular injection of hUSC-140-Exos has the potential to attenuate the development of early KOA and protect knee articular cartilage from further acute destruction through the regulation of ECM homeostasis and subchondral bone restoration.
Human induced pluripotent stem cells (iPSCs) are similar to embryonic stem cells in structure, self-regeneration, and specialization ability and are stimulated from somatic cells specific to patients (Kang et al., 2009; Hirschi et al., 2014). Through intra-articular administration of iPSCs-derived exosomes (iMSC-Exos) and SMMSC-Exos in a mouse model of OA induced by collagenase, Zhu et al. (Zhu et al., 2017) concluded that iMSC-Exos and SMMSC-Exos alleviated OA by stimulating chondrocyte movement and proliferation.
Compared with SMMSC-Exos, iMSC-Exos had superior therapeutic effects. iMSC-Exos probably function as a novel therapeutic target for OA because autologous iMSCs are theoretically inexhaustible, Compared with mature stem cells, such as BMSCs and AMSCs, amniotic fluid MSCs represent preferable exosome sources based on size and surface marker expression, and amniotic fluid MSCs are produced at higher levels from amniotic fluid cells (Tracy et al., 2019). Zavatti et al. (2020) conducted an experiment to study the effect of the secreted exosomes compared with their amniotic fluid stem cell (AFSC) source. These researchers utilized a MIA-induced animal model of osteoarthritis simulating a chronic and retrogressive process, where inflammation also participated and contributed to inversible joint degeneration and confirmed that by stimulating the movement and proliferation of repairing cells and enhancing the synthesis of cartilage matrix, AFSC-derived exosomes generated effective cartilage repair.
Yan et al. (Yan and Wu, 2020) used umbilical MSC (U-MSC) exosomes generated by typical two-dimensional (2D) tissue culture polystyrene flasks and 3D microgravity environment culture for the treatment of cartilage repair and demonstrated that the use of exosomes sourced from umbilical MSCs (U-MSC-Exos) also protected chondrocytes, activated cell proliferation, migration, and synthesis of the matrix, and reduced apoptosis. Moreover, exosomes derived from 3D-culture were more prolific and biologically active. In terms of sustaining the phenotypic stability of chondrocytes, 3D-Exos exhibited better capacity than 2D-Exos, which may partially contribute to a better capacity to promote the migration of chondrocytes and a prominent capacity in balancing matrix synthesis through the TGF-β1-dependent Smad2/3 signaling pathway. MSC-derived exosomes exhibit emerging potential as targets of especially acute examination, and several types of MSC derived exosomes have shown therapeutic potential in OA. However, comparisons between different MSC-derived exosomes are needed to optimize therapy utilizing MSC-derived exosomes (Tracy et al., 2019).
Above all, studies on exosomes derived from antler stem cells (ASCs) also provide new insights into novel therapeutic approaches for OA. Wang et al. (2019) detected genetic changes and gene expression in ruminant headgear and demonstrated that the great proliferation and differentiation potential of ASCs may be attributed to their origin from cranial neural crest cells. Lei et al. (2022) used an anterior cruciate ligament transection (ACLT) surgery-induced OA mouse model and found that ASC-derived exosomes attenuated human stem cell senescence and ameliorated cartilage degeneration. Consequently, ASC may represent an appropriate source for treatment based on exosomes.
Exosomes from other cells or tissues
The majority of current studies mainly concentrate on MSC-derived exosomes with respect to their diagnostic and therapeutic functions in OA. Nevertheless, exosomes derived from sources other than MSCs have therapeutic potential for OA. An in vivo study indicated that platelet-rich plasma (PRP)-derived exosomes increased proliferation and movement while decreasing apoptosis of osteoarthritic chondrocytes stimulated by IL-1β. Moreover, PRP-Exos activate the WNT/β-catenin signaling pathway and protect cartilage from degradation. Notably, the therapeutic outcome is even more satisfying than that of stimulated PRP (Liu et al., 2019).
Infrapatellar fat pad (IPFP)-derived exosomes have also exhibited potential utilization in the treatment of OA in DMM-induced OA models. Facilitated by exosomal miR-100-5p, which interacts with mammalian target of rapamycin (mTOR) and its downstream cofactors in chondrocytes, IPFP-Exos not only attenuated articular cartilage damage but also promoted gait function (Wu et al., 2019; Li et al., 2021a).
Zheng et al. (2019) demonstrated that exosomes derived from chondrocytes had effects on OA by regulating the metabolism of chondrocytes. They found that exosomes derived from chondrocytes delayed IL-1β-induced chondrocyte degeneration and restored the metabolism of damaged chondrocytes through the regeneration of the destroyed mitochondria via the supplementation of exosome proteins. Additionally, chondrocyte exosomes modulated the polarization of macrophages and increased the M2 phenotype to regulate immune reactivity in OA. An intracellular injection of exosomes also successfully mitigated the progression of OA, demonstrating the therapeutic potential of chondrocyte exosomes in an OA model.
Synovial fibroblast (SFC)-derived exosomes have also shown a great capability to reduce chondrocyte inflammation and cartilage destruction. An in vivo study by Zhou et al. revealed that SFCs-miRNA-126-3p-Exos maintained subchondral bone structure and suppressed synovial inflammation-mediated cartilage degeneration and articular cartilage chondrocyte apoptosis and inflammation in OA model rats (Zhou et al., 2021). These results indicate that SFC-miRNA-126-3p-Exos should be explored for therapeutic use in the treatment of OA.
Collectively, exosomes from stem cells including BMSCs, AMSCs, SMSCs, and ESCs, and other stem cells and other cells or tissues have shown powerful cartilage restoration ability and repressed OA progression in OA models. Still in the animal experiment stage, more research endeavors are desired to further clarify the specific mechanisms of exosomes in the treatment of OA and identify the most appropriate cell source (Table 2).
Exosomes and cartilage tissue engineering
Currently, osteochondral repair through encapsulating native cells such as chondrocytes and MSCs or growth factors by the injection of polymers generated from natural biomaterials or synthetic materials has been explored. However, there are still many limitations to its clinical application. For example, studies revealed that the autologous chondrocytes may be infective in elderly patients, and the long-lasting healing effects are also restricted by their short half-life property and the poor intracellular delivery of growth factors (Yang et al., 2017; Khorshidi and Karkhaneh, 2018; Chen et al., 2021). Exosomes with the bioactive constituents, including cytokines, growth factors, transcription factors, are secreted from the secreted cells, and are nonliving. Therefore, exosomes do not require in vitro maintenance, can easily integrate with target cells, and have been noted as a novel engineering strategy for osteochondral injuries in OA (Bei et al., 2021).
Modified exosomes can be derived from MSCs that are transfected with transcription factors to target different stages and phenotypes of OA. Liu et al. (2018) derived exosomes from lncRNA-KLF3-AS1-treated MSCs with enhanced KLF3-AS1 expression for the treatment of OA. They found that the ECM components Col2a1 and aggrecan were upregulated, whereas important inflammatory markers, such as MMP-13 and RUNX2, were reduced. These findings confirmed that the engineered exosomes facilitated chondrocyte proliferation and alleviated apoptosis.
Based on its soft-like quality, hydroscopicity, elastic mechanical function, and prominent biocompatibility, implantation of hydrogels is currently a major method to load various growth factors and stem cells for local cartilage repair (Taylor and Panhuis, 2016; Zhao et al., 2017; Murphy et al., 2020). To improve the poor adhesion of hydrogels to wet tissue, Zhang et al. (2021a) invented highly adhesive hydrogel combining alginate-dopamine, chondroitin sulfate, and regenerated silk fibroin, which significantly refined the bonding intensity to the wet surface. Relevant experiments based on chemokine signaling pathways and promoting BMSC differentiation into chondrocytes, the novel biomaterial showed therapeutic potential for OA. Hu et al. (2020) creatively combined laponite nanoclay with gelatin methacrylate (Gelma) hydrogel and confirmed that the poor strength and the lack of ordered structure in the cartilage tissue of the hydrogel can be remedied by nanoclay, ensuring a favorable condition for cell proliferation and differentiation. Recently, Shen et al. (2022) also fabricated a silk fibroin (SF) hydrogel that can be injected to support and maintain exosomes derived from hypoxia-pretreated mesenchymal stem cells for cartilage tissue engineering. The experiment revealed that the injectable silk hydrogel carrying articular chondrocytes and hypoxia-pretreated exosomes (H-Exos) was conducive to ameliorating cartilage degeneration as well as facilitating cartilage repair through the miR-205-5p/PTEN/AKT pathway, indicating a new therapeutic target for cartilage tissue engineering utilizing exosomes and articular chondrocytes loaded with SF hydrogel. Nevertheless, more studies are needed to further explore SF/H-Exos therapeutic effects without articular chondrocytes and whether the therapeutic strategy is viable in OA patients.
A series of studies confirmed that exosomes can be adsorbed over the cryogel surface. Nikhil et al. (Nikhil and Kumar, 2022) shed light on the promising role of exosomes combined with chitosan-gelatin-chondroitin (CGC) cryogel extract, which effectively promoted chondrocyte proliferation and migration. Notably, both exosomes and CGC cryogels facilitate chondrocyte proliferation alone and exert more potent positive effects when used in combination. Therefore, exosomes combined with cryogels can be potentially used as an alternative cartilage tissue engineering strategy for OA patients.
In addition to CGC and hydrogels, other biomaterials including titanium nanotubes and a delivery platform combined with exosomes, are also emerging (Wei et al., 2019; Swanson et al., 2020) (Table 3) (Figure 1)
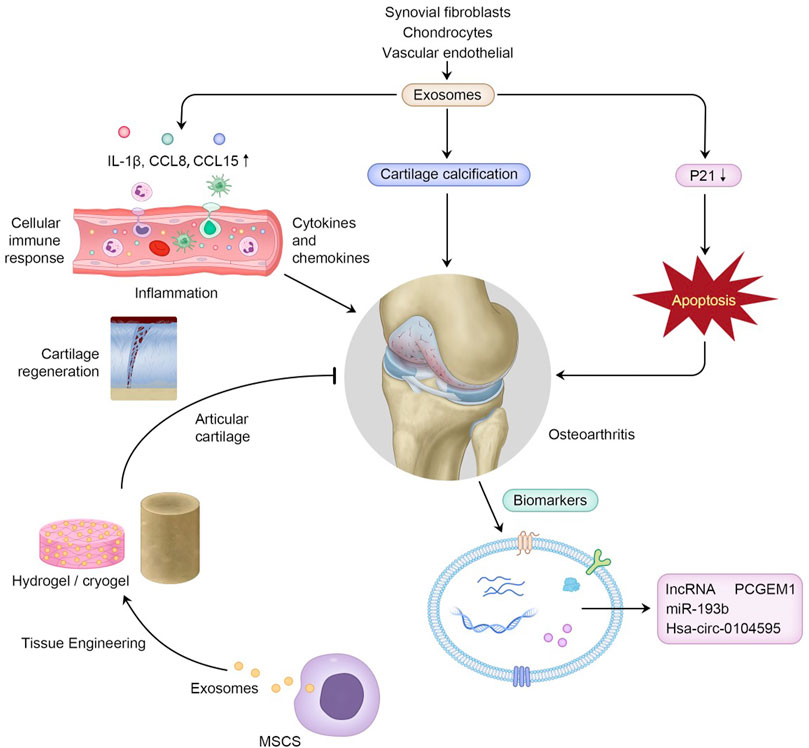
FIGURE 1. Exosomes in OA pathogenesis, and their clinical potential to serve as biomarkers in the diagnosis of OA and to function in cartilage tissue engineering combined with biomaterials.
Conclusion
As pivotal intercellular signaling messengers, exosomes provide an advanced strategy for the assessment of pathological cellular or tissue states in OA. Exosomes in biological fluids, such as synovial fluid and plasma, have shown great potential as novel biomarkers, as they contain specific nucleic acids (miRNAs, lncRNAs), proteins, lipids, amino acids, and metabolites derived from the selected cells and can steadily transfer vesicular substances. RNAs, especially circRNAs, have emerged as novel biomarkers for OA. Moreover, exosomes promote angiogenesis, bone remodeling and chondrocyte proliferation and migration while inhibiting osteoarthritic chondrocyte apoptosis and attenuating cartilage inflammation. Exosomes derived from different types of mesenchymal stem cells, especially BMSCs, AMSCs, SMSCs and EMSCs, and several types of other cells or tissues, including PRP, IPFP, chondrocytes, and SFCs, may ameliorate osteoarthritis as a disease-modifying osteoarthritis cell-free products through the stimulation of cartilage regeneration and inhibition of chondrocyte apoptosis. Although presenting studies reveal that exosome-derived MSCs exert better therapeutic effects, it remains a challenge for us to determine which source exhibits a preferable therapeutic role in OA. In addition, facilitated by biocompatible materials, including hydrogels and cryogels, exosomes exert increasing therapeutic effects by cartilage tissue engineering for OA. In the future, we expect that individualized treatment modalities based on exosomes will represent therapeutic strategy for OA.
Author contributions
YZ, S-BA, and W-FX conceived the idea of the study. L-YP, Y-LD, and Y-YZ searched, screened and arranged references. W-JF, DL, W-YW, and R-XY mainly drafted the manuscript. All authors critically revised the article for important intellectual content. All authors have read and approved the final manuscript.
Funding
This work was supported by National Natural Science Foundation of China (82102600), National Clinical Research Center for Geriatric Disorders (Xiangya Hospital, Grant No. 2021KFJJ02), National Clinical Research Center for Orthopedics, Sports Medicine and Rehabilitation (2021-NCRC-CXJJ-PY-40), Provincial Natural Science Foundation of Hunan (No. 2022SK2718), Provincial Key R&D Program of Hunan (S2020GCZDYF0312), Provincial Natural Science Foundation of Shandong (ZR202102210485), and the Independent Exploration and Innovation Project for Postgraduate Students of Central South University (2021zzts1037).
Conflict of interest
The authors declare that the research was conducted in the absence of any commercial or financial relationships that could be construed as a potential conflict of interest.
Publisher’s note
All claims expressed in this article are solely those of the authors and do not necessarily represent those of their affiliated organizations, or those of the publisher, the editors and the reviewers. Any product that may be evaluated in this article, or claim that may be made by its manufacturer, is not guaranteed or endorsed by the publisher.
References
Archer, C. W., Dowthwaite, G. P., and Francis-West, P. (2003). Development of synovial joints. Birth Defects Res. C Embryo Today 69 (2), 144–155. doi:10.1002/bdrc.10015
Asghar, S., Litherland, G. J., Lockhart, J. C., Goodyear, C. S., and Crilly, A. (2020). Exosomes in intercellular communication and implications for osteoarthritis. Rheumatol. Oxf. 59 (1), 57–68. doi:10.1093/rheumatology/kez462
Bei, H. P., Hung, P. M., Yeung, H. L., Wang, S., and Zhao, X. (2021). Bone-a-Petite: Engineering exosomes towards bone, osteochondral, and cartilage repair. Small 17 (50), e2101741. doi:10.1002/smll.202101741
Bhimani, R., Singh, P., and Bhimani, F. (2018). Rapidly progressive hip disease-A rare entity in Korean population. Int. J. Surg. Case Rep. 53, 486–489. doi:10.1016/j.ijscr.2018.11.055
Brandt, K. D., Radin, E. L., Dieppe, P. A., and van de Putte, L. (2006). Yet more evidence that osteoarthritis is not a cartilage disease. Ann. Rheum. Dis. 65 (10), 1261–1264. doi:10.1136/ard.2006.058347
Burger, D., Viñas, J. L., Akbari, S., Dehak, H., Knoll, W., Gutsol, A., et al. (2015). Human endothelial colony-forming cells protect against acute kidney injury: Role of exosomes. Am. J. Pathol. 185 (8), 2309–2323. doi:10.1016/j.ajpath.2015.04.010
Buzas, E. I., György, B., Nagy, G., Falus, A., and Gay, S. (2014). Emerging role of extracellular vesicles in inflammatory diseases. Nat. Rev. Rheumatol. 10 (6), 356–364. doi:10.1038/nrrheum.2014.19
Carballo, C. B., Nakagawa, Y., Sekiya, I., and Rodeo, S. A. (2017). Basic science of articular cartilage. Clin. Sports Med. 36 (3), 413–425. doi:10.1016/j.csm.2017.02.001
Caruso, S., and Poon, I. K. H. (2018). Apoptotic cell-derived extracellular vesicles: More than just debris. Front. Immunol. 9, 1486. doi:10.3389/fimmu.2018.01486
Chen, G., Huang, A. C., Zhang, W., Zhang, G., Wu, M., Xu, W., et al. (2018). Exosomal PD-L1 contributes to immunosuppression and is associated with anti-PD-1 response. Nature 560 (7718), 382–386. doi:10.1038/s41586-018-0392-8
Chen, T. S., Lai, R. C., Lee, M. M., Choo, A. B., Lee, C. N., Lim, S. K., et al. (2010). Mesenchymal stem cell secretes microparticles enriched in pre-microRNAs. Nucleic Acids Res. 38 (1), 215–224. doi:10.1093/nar/gkp857
Chen, Z., Zhang, Q., Li, H., Wei, Q., Zhao, X., Chen, F., et al. (2021). Elastin-like polypeptide modified silk fibroin porous scaffold promotes osteochondral repair. Bioact. Mat. 6 (3), 589–601. doi:10.1016/j.bioactmat.2020.09.003
Choi, D. S., Kim, D. K., Kim, Y. K., and Gho, Y. S. (2015). Proteomics of extracellular vesicles: Exosomes and ectosomes. Mass Spectrom. Rev. 34 (4), 474–490. doi:10.1002/mas.21420
Chow, A., Zhou, W., Liu, L., Fong, M. Y., Champer, J., Van Haute, D., et al. (2014). Macrophage immunomodulation by breast cancer-derived exosomes requires Toll-like receptor 2-mediated activation of NF-κB. Sci. Rep. 4, 5750. doi:10.1038/srep05750
Colombo, M., Raposo, G., and Théry, C. (2014). Biogenesis, secretion, and intercellular interactions of exosomes and other extracellular vesicles. Annu. Rev. Cell Dev. Biol. 30, 255–289. doi:10.1146/annurev-cellbio-101512-122326
Cosenza, S., Ruiz, M., Toupet, K., Jorgensen, C., and Noël, D. (2017). Mesenchymal stem cells derived exosomes and microparticles protect cartilage and bone from degradation in osteoarthritis. Sci. Rep. 7 (1), 16214. doi:10.1038/s41598-017-15376-8
Crawford, D. C., Miller, L. E., and Block, J. E. (2013). Conservative management of symptomatic knee osteoarthritis: A flawed strategy? Orthop. Rev. 5 (1), e2. doi:10.4081/or.2013.e2
Damia, E., Chicharro, D., Lopez, S., Cuervo, B., Rubio, M., Sopena, J. J., et al. (2018). Adipose-derived mesenchymal stem cells: Are they a good therapeutic strategy for osteoarthritis? Int. J. Mol. Sci. 19 (7), E1926. doi:10.3390/ijms19071926
de Lange-Brokaar, B. J., Ioan-Facsinay, A., van Osch, G. J., Zuurmond, A. M., Schoones, J., Toes, R. E., et al. (2012). Synovial inflammation, immune cells and their cytokines in osteoarthritis: A review. Osteoarthr. Cartil. 20 (12), 1484–1499. doi:10.1016/j.joca.2012.08.027
Domenis, R., Zanutel, R., Caponnetto, F., Toffoletto, B., Cifù, A., Pistis, C., et al. (2017). Characterization of the proinflammatory profile of synovial fluid-derived exosomes of patients with osteoarthritis. Mediat. Inflamm. 2017, 4814987. doi:10.1155/2017/4814987
Fukai, A., Kamekura, S., Chikazu, D., Nakagawa, T., Hirata, M., Saito, T., et al. (2012). Lack of a chondroprotective effect of cyclooxygenase 2 inhibition in a surgically induced model of osteoarthritis in mice. Arthritis Rheum. 64 (1), 198–203. doi:10.1002/art.33324
Glyn-Jones, S., Palmer, A. J., Agricola, R., Price, A. J., Vincent, T. L., Weinans, H., et al. (2015). Osteoarthritis. Lancet 386 (9991), 376–387. doi:10.1016/s0140-6736(14)60802-3
Griffin, T. M., and Scanzello, C. R. (2019). Innate inflammation and synovial macrophages in osteoarthritis pathophysiology. Clin. Exp. Rheumatol. 37 (Suppl. 1205), 57–63.
Guo, S. C., Tao, S. C., Yin, W. J., Qi, X., Sheng, J. G., Zhang, C. Q., et al. (2016). Exosomes from human synovial-derived mesenchymal stem cells prevent glucocorticoid-induced osteonecrosis of the femoral head in the rat. Int. J. Biol. Sci. 12 (10), 1262–1272. doi:10.7150/ijbs.16150
Gurunathan, S., Kang, M. H., Jeyaraj, M., Qasim, M., and Kim, J. H. (2019). The effects of apigenin-biosynthesized ultra-small platinum nanoparticles on the human monocytic THP-1 cell line. Cells 8 (4), E444. doi:10.3390/cells8050444
György, B., Szabó, T. G., Turiák, L., Wright, M., Herczeg, P., Lédeczi, Z., et al. (2012). Improved flow cytometric assessment reveals distinct microvesicle (cell-derived microparticle) signatures in joint diseases. PLoS One 7 (11), e49726. doi:10.1371/journal.pone.0049726
Hanahan, D., and Weinberg, R. A. (2011). Hallmarks of cancer: The next generation. Cell 144 (5), 646–674. doi:10.1016/j.cell.2011.02.013
Haywood, L., McWilliams, D. F., Pearson, C. I., Gill, S. E., Ganesan, A., Wilson, D., et al. (2003). Inflammation and angiogenesis in osteoarthritis. Arthritis Rheum. 48 (8), 2173–2177. doi:10.1002/art.11094
Headland, S. E., Jones, H. R., Norling, L. V., Kim, A., Souza, P. R., Corsiero, E., et al. (2015). Neutrophil-derived microvesicles enter cartilage and protect the joint in inflammatory arthritis. Sci. Transl. Med. 7 (315), 315ra190. doi:10.1126/scitranslmed.aac5608
Helmick, C. G., Felson, D. T., Lawrence, R. C., Gabriel, S., Hirsch, R., Kwoh, C. K., et al. (2008). Estimates of the prevalence of arthritis and other rheumatic conditions in the United States. Part I. Arthritis Rheum. 58 (1), 15–25. doi:10.1002/art.23177
Hessvik, N. P., and Llorente, A. (2018). Current knowledge on exosome biogenesis and release. Cell. Mol. Life Sci. 75 (2), 193–208. doi:10.1007/s00018-017-2595-9
Hirschi, K. K., Li, S., and Roy, K. (2014). Induced pluripotent stem cells for regenerative medicine. Annu. Rev. Biomed. Eng. 16, 277–294. doi:10.1146/annurev-bioeng-071813-105108
Hu, H., Dong, L., Bu, Z., Shen, Y., Luo, J., Zhang, H., et al. (2020). miR-23a-3p-abundant small extracellular vesicles released from Gelma/nanoclay hydrogel for cartilage regeneration. J. Extracell. Vesicles 9 (1), 1778883. doi:10.1080/20013078.2020.1778883
Hunter, D. J., Schofield, D., and Callander, E. (2014). The individual and socioeconomic impact of osteoarthritis. Nat. Rev. Rheumatol. 10 (7), 437–441. doi:10.1038/nrrheum.2014.44
Jin, Z., Ren, J., and Qi, S. (2020). Human bone mesenchymal stem cells-derived exosomes overexpressing microRNA-26a-5p alleviate osteoarthritis via down-regulation of PTGS2. Int. Immunopharmacol. 78, 105946. doi:10.1016/j.intimp.2019.105946
Jo, W., Kim, J., Yoon, J., Jeong, D., Cho, S., Jeong, H., et al. (2014). Large-scale generation of cell-derived nanovesicles. Nanoscale 6 (20), 12056–12064. doi:10.1039/c4nr02391a
Kahlert, C., and Kalluri, R. (2013). Exosomes in tumor microenvironment influence cancer progression and metastasis. J. Mol. Med. 91 (4), 431–437. doi:10.1007/s00109-013-1020-6
Kalluri, R., and LeBleu, V. S. (2020). The biology, function, and biomedical applications of exosomes. Science 367 (6478), eaau6977. doi:10.1126/science.aau6977
Kalluri, R. (2016). The biology and function of exosomes in cancer. J. Clin. Invest. 126 (4), 1208–1215. doi:10.1172/jci81135
Kang, L., Wang, J., Zhang, Y., Kou, Z., and Gao, S. (2009). iPS cells can support full-term development of tetraploid blastocyst-complemented embryos. Cell Stem Cell 5 (2), 135–138. doi:10.1016/j.stem.2009.07.001
Katare, R., Stroemer, P., Hicks, C., Stevanato, L., Patel, S., Corteling, R., et al. (2014). Clinical-grade human neural stem cells promote reparative neovascularization in mouse models of hindlimb ischemia. Arterioscler. Thromb. Vasc. Biol. 34 (2), 408–418. doi:10.1161/atvbaha.113.302592
Kato, T., Miyaki, S., Ishitobi, H., Nakamura, Y., Nakasa, T., Lotz, M. K., et al. (2014). Exosomes from IL-1β stimulated synovial fibroblasts induce osteoarthritic changes in articular chondrocytes. Arthritis Res. Ther. 16 (4), R163. doi:10.1186/ar4679
Kester, B. S., Minhas, S. V., Vigdorchik, J. M., and Schwarzkopf, R. (2016). Total knee arthroplasty for posttraumatic osteoarthritis: Is it time for a new classification? J. Arthroplasty 31 (8), 16491649–16491653. doi:10.1016/j.arth.2016.02.001
Khorshidi, S., and Karkhaneh, A. (2018). A review on gradient hydrogel/fiber scaffolds for osteochondral regeneration. J. Tissue Eng. Regen. Med. 12 (4), e1974–e1990. doi:10.1002/term.2628
Kim, Y. G., Choi, J., and Kim, K. (2020). Mesenchymal stem cell-derived exosomes for effective cartilage tissue repair and treatment of osteoarthritis. Biotechnol. J. 15 (12), e2000082. doi:10.1002/biot.202000082
Koizumi, K., Ebina, K., Hart, D. A., Hirao, M., Noguchi, T., Sugita, N., et al. (2016). Synovial mesenchymal stem cells from osteo- or rheumatoid arthritis joints exhibit good potential for cartilage repair using a scaffold-free tissue engineering approach. Osteoarthr. Cartil. 24 (8), 1413–1422. doi:10.1016/j.joca.2016.03.006
Koyama, E., Shibukawa, Y., Nagayama, M., Sugito, H., Young, B., Yuasa, T., et al. (2008). A distinct cohort of progenitor cells participates in synovial joint and articular cartilage formation during mouse limb skeletogenesis. Dev. Biol. 316 (1), 62–73. doi:10.1016/j.ydbio.2008.01.012
Krishnan, Y., and Grodzinsky, A. J. (2018). Cartilage diseases. Matrix Biol. 71-72, 51–69. doi:10.1016/j.matbio.2018.05.005
Kurth, T., Hedbom, E., Shintani, N., Sugimoto, M., Chen, F. H., Haspl, M., et al. (2007). Chondrogenic potential of human synovial mesenchymal stem cells in alginate. Osteoarthr. Cartil. 15 (10), 1178–1189. doi:10.1016/j.joca.2007.03.015
Lee, C., Carney, R. P., Hazari, S., Smith, Z. J., Knudson, A., Robertson, C. S., et al. (2015). 3D plasmonic nanobowl platform for the study of exosomes in solution. Nanoscale 7 (20), 9290–9297. doi:10.1039/c5nr01333j
Lee, W. S., Kim, H. J., Kim, K. I., Kim, G. B., and Jin, W. (2019). Intra-articular injection of autologous adipose tissue-derived mesenchymal stem cells for the treatment of knee osteoarthritis: A phase IIb, randomized, placebo-controlled clinical trial. Stem Cells Transl. Med. 8 (6), 504–511. doi:10.1002/sctm.18-0122
Lee, Y. H., Park, H. K., Auh, Q. S., Nah, H., Lee, J. S., Moon, H. J., et al. (2020). Emerging potential of exosomes in regenerative medicine for temporomandibular joint osteoarthritis. Int. J. Mol. Sci. 21 (4), E1541. doi:10.3390/ijms21041541
Lei, J., Jiang, X., Li, W., Ren, J., Wang, D., Ji, Z., et al. (2022). Exosomes from antler stem cells alleviate mesenchymal stem cell senescence and osteoarthritis. Protein Cell 13 (3), 220–226. doi:10.1007/s13238-021-00860-9
Li, D., Gupta, P., Sgaglione, N. A., and Grande, D. A. (2021a). Exosomes derived from non-classic sources for treatment of post-traumatic osteoarthritis and cartilage injury of the knee: In vivo review. J. Clin. Med. 10 (9), 2001. doi:10.3390/jcm10092001
Li, M. Y., Liu, L. Z., and Dong, M. (2021b). Progress on pivotal role and application of exosome in lung cancer carcinogenesis, diagnosis, therapy and prognosis. Mol. Cancer 20 (1), 22. doi:10.1186/s12943-021-01312-y
Li, Q., and Barres, B. A. (2018). Microglia and macrophages in brain homeostasis and disease. Nat. Rev. Immunol. 18 (4), 225–242. doi:10.1038/nri.2017.125
Li, Y., Zheng, Q., Bao, C., Li, S., Guo, W., Zhao, J., et al. (2015). Circular RNA is enriched and stable in exosomes: A promising biomarker for cancer diagnosis. Cell Res. 25 (8), 981–984. doi:10.1038/cr.2015.82
Li, Z., Li, M., Xu, P., Ma, J., and Zhang, R. (2020). Compositional variation and functional mechanism of exosomes in the articular microenvironment in knee osteoarthritis. Cell Transpl. 29, 963689720968495. doi:10.1177/0963689720968495
Liang, Y., Duan, L., Lu, J., and Xia, J. (2021). Engineering exosomes for targeted drug delivery. Theranostics 11 (7), 3183–3195. doi:10.7150/thno.52570
Lipina, M., Makarov, M., Makarov, S., and Novikov, A. (2017). The degree of cartilage degradation assessed by serum biomarker levels changes after arthroscopic knee synovectomy in rheumatoid arthritis patients. Int. Orthop. 41 (11), 2259–2264. doi:10.1007/s00264-017-3634-8
Liu, Q., Wang, R., Hou, S., He, F., Ma, Y., Ye, T., et al. (2022a). Chondrocyte-derived exosomes promote cartilage calcification in temporomandibular joint osteoarthritis. Arthritis Res. Ther. 24 (1), 44. doi:10.1186/s13075-022-02738-5
Liu, X., Wang, L., Ma, C., Wang, G., Zhang, Y., Sun, S., et al. (2019). Exosomes derived from platelet-rich plasma present a novel potential in alleviating knee osteoarthritis by promoting proliferation and inhibiting apoptosis of chondrocyte via Wnt/β-catenin signaling pathway. J. Orthop. Surg. Res. 14 (1), 470. doi:10.1186/s13018-019-1529-7
Liu, Y., Lin, L., Zou, R., Wen, C., Wang, Z., Lin, F., et al. (2018). MSC-derived exosomes promote proliferation and inhibit apoptosis of chondrocytes via lncRNA-KLF3-AS1/miR-206/GIT1 axis in osteoarthritis. Cell Cycle 17 (21-22), 2411–2422. doi:10.1080/15384101.2018.1526603
Liu, Y., Zeng, Y., Si, H. B., Tang, L., Xie, H. Q., Shen, B., et al. (2022b). Exosomes derived from human urine-derived stem cells overexpressing miR-140-5p alleviate knee osteoarthritis through downregulation of VEGFA in a rat model. Am. J. Sports Med. 50 (4), 1088–1105. doi:10.1177/03635465221073991
Ma, T., Chen, Y., Chen, Y., Meng, Q., Sun, J., Shao, L., et al. (2018). MicroRNA-132, delivered by mesenchymal stem cell-derived exosomes, promote angiogenesis in myocardial infarction. Stem Cells Int. 2018, 3290372. doi:10.1155/2018/3290372
Malda, J., Boere, J., van de Lest, C. H., van Weeren, P., and Wauben, M. H. (2016). Extracellular vesicles — New tool for joint repair and regeneration. Nat. Rev. Rheumatol. 12 (4), 243–249. doi:10.1038/nrrheum.2015.170
Mao, G., Zhang, Z., Hu, S., Zhang, Z., Chang, Z., Huang, Z., et al. (2018). Exosomes derived from miR-92a-3p-overexpressing human mesenchymal stem cells enhance chondrogenesis and suppress cartilage degradation via targeting WNT5A. Stem Cell Res. Ther. 9 (1), 247. doi:10.1186/s13287-018-1004-0
Mao, X., Cao, Y., Guo, Z., Wang, L., and Xiang, C. (2021). Biological roles and therapeutic potential of circular RNAs in osteoarthritis. Mol. Ther. Nucleic Acids 24, 856–867. doi:10.1016/j.omtn.2021.04.006
Mathieu, M., Martin-Jaular, L., Lavieu, G., and Théry, C. (2019). Specificities of secretion and uptake of exosomes and other extracellular vesicles for cell-to-cell communication. Nat. Cell Biol. 21 (1), 9–17. doi:10.1038/s41556-018-0250-9
McAndrews, K. M., and Kalluri, R. (2019). Mechanisms associated with biogenesis of exosomes in cancer. Mol. Cancer 18 (1), 52. doi:10.1186/s12943-019-0963-9
Melo, S. A., Luecke, L. B., Kahlert, C., Fernandez, A. F., Gammon, S. T., Kaye, J., et al. (2015). Glypican-1 identifies cancer exosomes and detects early pancreatic cancer. Nature 523 (7559), 177–182. doi:10.1038/nature14581
Meng, F., Li, Z., Zhang, Z., Yang, Z., Kang, Y., Zhao, X., et al. (2018). MicroRNA-193b-3p regulates chondrogenesis and chondrocyte metabolism by targeting HDAC3. Theranostics 8 (10), 2862–2883. doi:10.7150/thno.23547
Mitchell, P. S., Parkin, R. K., Kroh, E. M., Fritz, B. R., Wyman, S. K., Pogosova-Agadjanyan, E. L., et al. (2008). Circulating microRNAs as stable blood-based markers for cancer detection. Proc. Natl. Acad. Sci. U. S. A. 105 (30), 10513–10518. doi:10.1073/pnas.0804549105
Mulcahy, L. A., Pink, R. C., and Carter, D. R. (2014). Routes and mechanisms of extracellular vesicle uptake. J. Extracell. Vesicles 3, 24641. doi:10.3402/jev.v3.24641
Murphy, M. P., Koepke, L. S., Lopez, M. T., Tong, X., Ambrosi, T. H., Gulati, G. S., et al. (2020). Articular cartilage regeneration by activated skeletal stem cells. Nat. Med. 26 (10), 1583–1592. doi:10.1038/s41591-020-1013-2
Ni, Z., Zhou, S., Li, S., Kuang, L., Chen, H., Luo, X., et al. (2020). Exosomes: Roles and therapeutic potential in osteoarthritis. Bone Res. 8, 25. doi:10.1038/s41413-020-0100-9
Nikhil, A., and Kumar, A. (2022). Evaluating potential of tissue-engineered cryogels and chondrocyte derived exosomes in articular cartilage repair. Biotechnol. Bioeng. 119 (2), 605–625. doi:10.1002/bit.27982
Prieto-Alhambra, D., Judge, A., Javaid, M. K., Cooper, C., Diez-Perez, A., Arden, N. K., et al. (2014). Incidence and risk factors for clinically diagnosed knee, hip and hand osteoarthritis: Influences of age, gender and osteoarthritis affecting other joints. Ann. Rheum. Dis. 73 (9), 1659–1664. doi:10.1136/annrheumdis-2013-203355
Qi, H., Liu, D. P., Xiao, D. W., Tian, D. C., Su, Y. W., Jin, S. F., et al. (2019). Exosomes derived from mesenchymal stem cells inhibit mitochondrial dysfunction-induced apoptosis of chondrocytes via p38, ERK, and Akt pathways. Vitro Cell. Dev. Biol. Anim. 55 (3), 203–210. doi:10.1007/s11626-019-00330-x
Raghu, H., Lepus, C. M., Wang, Q., Wong, H. H., Lingampalli, N., Oliviero, F., et al. (2017). CCL2/CCR2, but not CCL5/CCR5, mediates monocyte recruitment, inflammation and cartilage destruction in osteoarthritis. Ann. Rheum. Dis. 76 (5), 914–922. doi:10.1136/annrheumdis-2016-210426
Ridder, K., Keller, S., Dams, M., Rupp, A. K., Schlaudraff, J., Del Turco, D., et al. (2014). Extracellular vesicle-mediated transfer of genetic information between the hematopoietic system and the brain in response to inflammation. PLoS Biol. 12 (6), e1001874. doi:10.1371/journal.pbio.1001874
Ruivo, C. F., Adem, B., Silva, M., and Melo, S. A. (2017). The biology of cancer exosomes: Insights and new perspectives. Cancer Res. 77 (23), 6480–6488. doi:10.1158/0008-5472.Can-17-0994
Schelbergen, R. F., van Dalen, S., ter Huurne, M., Roth, J., Vogl, T., Noël, D., et al. (2014). Treatment efficacy of adipose-derived stem cells in experimental osteoarthritis is driven by high synovial activation and reflected by S100A8/A9 serum levels. Osteoarthr. Cartil. 22 (8), 1158–1166. doi:10.1016/j.joca.2014.05.022
Shen, K., Duan, A., Cheng, J., Yuan, T., Zhou, J., Song, H., et al. (2022). Exosomes derived from hypoxia preconditioned mesenchymal stem cells laden in a silk hydrogel promote cartilage regeneration via the miR-205-5p/PTEN/AKT pathway. Acta Biomater. 143, 173–188. doi:10.1016/j.actbio.2022.02.026
Shirasawa, S., Sekiya, I., Sakaguchi, Y., Yagishita, K., Ichinose, S., Muneta, T., et al. (2006). In vitro chondrogenesis of human synovium-derived mesenchymal stem cells: Optimal condition and comparison with bone marrow-derived cells. J. Cell. Biochem. 97 (1), 84–97. doi:10.1002/jcb.20546
Silva, J., García, V., Zaballos, Á., Provencio, M., Lombardía, L., Almonacid, L., et al. (2011). Vesicle-related microRNAs in plasma of nonsmall cell lung cancer patients and correlation with survival. Eur. Respir. J. 37 (3), 617–623. doi:10.1183/09031936.00029610
Srikanthan, S., Li, W., Silverstein, R. L., and McIntyre, T. M. (2014). Exosome poly-ubiquitin inhibits platelet activation, downregulates CD36 and inhibits pro-atherothombotic cellular functions. J. Thromb. Haemost. 12 (11), 1906–1917. doi:10.1111/jth.12712
Sun, B., Ma, Y., Wang, F., Hu, L., and Sun, Y. (2019). miR-644-5p carried by bone mesenchymal stem cell-derived exosomes targets regulation of p53 to inhibit ovarian granulosa cell apoptosis. Stem Cell Res. Ther. 10 (1), 360. doi:10.1186/s13287-019-1442-3
Swanson, W. B., Zhang, Z., Xiu, K., Gong, T., Eberle, M., Wang, Z., et al. (2020). Scaffolds with controlled release of pro-mineralization exosomes to promote craniofacial bone healing without cell transplantation. Acta Biomater. 118, 215–232. doi:10.1016/j.actbio.2020.09.052
Tai, Y. L., Chen, K. C., Hsieh, J. T., and Shen, T. L. (2018). Exosomes in cancer development and clinical applications. Cancer Sci. 109 (8), 2364–2374. doi:10.1111/cas.13697
Tan, C. Y., Lai, R. C., Wong, W., Dan, Y. Y., Lim, S. K., Ho, H. K., et al. (2014). Mesenchymal stem cell-derived exosomes promote hepatic regeneration in drug-induced liver injury models. Stem Cell Res. Ther. 5 (3), 76. doi:10.1186/scrt465
Tao, S. C., Yuan, T., Rui, B. Y., Zhu, Z. Z., Guo, S. C., Zhang, C. Q., et al. (2017a). Exosomes derived from human platelet-rich plasma prevent apoptosis induced by glucocorticoid-associated endoplasmic reticulum stress in rat osteonecrosis of the femoral head via the Akt/Bad/Bcl-2 signal pathway. Theranostics 7 (3), 733–750. doi:10.7150/thno.17450
Tao, S. C., Yuan, T., Zhang, Y. L., Yin, W. J., Guo, S. C., Zhang, C. Q., et al. (2017b). Exosomes derived from miR-140-5p-overexpressing human synovial mesenchymal stem cells enhance cartilage tissue regeneration and prevent osteoarthritis of the knee in a rat model. Theranostics 7 (1), 180–195. doi:10.7150/thno.17133
Taylor, D. L., and Panhuis, M. (2016). Self-healing hydrogels. Adv. Mater 28 (41), 9060–9093. doi:10.1002/adma.201601613
Tkach, M., and Théry, C. (2016). Communication by extracellular vesicles: Where we are and where we need to go. Cell 164 (6), 1226–1232. doi:10.1016/j.cell.2016.01.043
Tofiño-Vian, M., Guillén, M. I., Pérez Del Caz, M. D., Castejón, M. A., and Alcaraz, M. J. (2017). Extracellular vesicles from adipose-derived mesenchymal stem cells downregulate senescence features in osteoarthritic osteoblasts. Oxid. Med. Cell. Longev. 2017, 7197598. doi:10.1155/2017/7197598
Tofiño-Vian, M., Guillén, M. I., Pérez Del Caz, M. D., Silvestre, A., and Alcaraz, M. J. (2018). Microvesicles from human adipose tissue-derived mesenchymal stem cells as a new protective strategy in osteoarthritic chondrocytes. Cell. Physiol. biochem. 47 (1), 11–25. doi:10.1159/000489739
Toh, W. S., Foldager, C. B., Pei, M., and Hui, J. H. (2014). Advances in mesenchymal stem cell-based strategies for cartilage repair and regeneration. Stem Cell Rev. Rep. 10 (5), 686–696. doi:10.1007/s12015-014-9526-z
Tracy, S. A., Ahmed, A., Tigges, J. C., Ericsson, M., Pal, A. K., Zurakowski, D., et al. (2019). A comparison of clinically relevant sources of mesenchymal stem cell-derived exosomes: Bone marrow and amniotic fluid. J. Pediatr. Surg. 54 (1), 86–90. doi:10.1016/j.jpedsurg.2018.10.020
van den Bosch, M. H., Blom, A. B., Schelbergen, R. F., Koenders, M. I., van de Loo, F. A., van den Berg, W. B., et al. (2016). Alarmin S100A9 induces proinflammatory and catabolic effects predominantly in the M1 macrophages of human osteoarthritic synovium. J. Rheumatol. 43 (10), 1874–1884. doi:10.3899/jrheum.160270
van Niel, G., D'Angelo, G., and Raposo, G. (2018). Shedding light on the cell biology of extracellular vesicles. Nat. Rev. Mol. Cell Biol. 19 (4), 213–228. doi:10.1038/nrm.2017.125
Wang, Y., Yu, D., Liu, Z., Zhou, F., Dai, J., Wu, B., et al. (2017). Exosomes from embryonic mesenchymal stem cells alleviate osteoarthritis through balancing synthesis and degradation of cartilage extracellular matrix. Stem Cell Res. Ther. 8 (1), 189. doi:10.1186/s13287-017-0632-0
Wang, Y., Zhang, C., Wang, N., Li, Z., Heller, R., Liu, R., et al. (2019). Genetic basis of ruminant headgear and rapid antler regeneration. Science 364 (6446), eaav6335. doi:10.1126/science.aav6335
Wei, F., Li, M., Crawford, R., Zhou, Y., and Xiao, Y. (2019). Exosome-integrated titanium oxide nanotubes for targeted bone regeneration. Acta Biomater. 86, 480–492. doi:10.1016/j.actbio.2019.01.006
Willms, E., Cabañas, C., Mäger, I., Wood, M. J. A., and Vader, P. (2018). Extracellular vesicle heterogeneity: Subpopulations, isolation techniques, and diverse functions in cancer progression. Front. Immunol. 9, 738. doi:10.3389/fimmu.2018.00738
Wu, J., Kuang, L., Chen, C., Yang, J., Zeng, W. N., Li, T., et al. (2019). miR-100-5p-abundant exosomes derived from infrapatellar fat pad MSCs protect articular cartilage and ameliorate gait abnormalities via inhibition of mTOR in osteoarthritis. Biomaterials 206, 87–100. doi:10.1016/j.biomaterials.2019.03.022
Xie, F., Liu, Y. L., Chen, X. Y., Li, Q., Zhong, J., Dai, B. Y., et al. (2020). Role of MicroRNA, LncRNA, and exosomes in the progression of osteoarthritis: A review of recent literature. Orthop. Surg. 12 (3), 708–716. doi:10.1111/os.12690
Xin, H., Li, Y., and Chopp, M. (2014). Exosomes/miRNAs as mediating cell-based therapy of stroke. Front. Cell. Neurosci. 8, 377. doi:10.3389/fncel.2014.00377
Xu, H., and Xu, B. (2021). BMSC-derived exosomes ameliorate osteoarthritis by inhibiting pyroptosis of cartilage via delivering miR-326 targeting HDAC3 and STAT1//NF-κB p65 to chondrocytes. Mediat. Inflamm. 2021, 9972805. doi:10.1155/2021/9972805
Xu, X., Liang, Y., Li, X., Ouyang, K., Wang, M., Cao, T., et al. (2021). Exosome-mediated delivery of kartogenin for chondrogenesis of synovial fluid-derived mesenchymal stem cells and cartilage regeneration. Biomaterials 269, 120539. doi:10.1016/j.biomaterials.2020.120539
Yan, L., Liu, G., and Wu, X. (2021). The umbilical cord mesenchymal stem cell-derived exosomal lncRNA H19 improves osteochondral activity through miR-29b-3p/FoxO3 axis. Clin. Transl. Med. 11 (1), e255. doi:10.1002/ctm2.255
Yan, L., and Wu, X. (2020). Exosomes produced from 3D cultures of umbilical cord mesenchymal stem cells in a hollow-fiber bioreactor show improved osteochondral regeneration activity. Cell Biol. Toxicol. 36 (2), 165–178. doi:10.1007/s10565-019-09504-5
Yang, J., Zhang, Y. S., Yue, K., and Khademhosseini, A. (2017). Cell-laden hydrogels for osteochondral and cartilage tissue engineering. Acta Biomater. 57, 1–25. doi:10.1016/j.actbio.2017.01.036
Yang, R. Z., Zheng, H. L., Xu, W. N., Zheng, X. F., Li, B., Jiang, L. S., et al. (2021). Vascular endothelial cell-secreted exosomes facilitate osteoarthritis pathogenesis by promoting chondrocyte apoptosis. Aging (Albany NY) 13 (3), 4647–4662. doi:10.18632/aging.202506
Zavatti, M., Beretti, F., Casciaro, F., Bertucci, E., and Maraldi, T. (2020). Comparison of the therapeutic effect of amniotic fluid stem cells and their exosomes on monoiodoacetate-induced animal model of osteoarthritis. Biofactors 46 (1), 106–117. doi:10.1002/biof.1576
Zhang, F. X., Liu, P., Ding, W., Meng, Q. B., Su, D. H., Zhang, Q. C., et al. (2021a). Injectable Mussel-Inspired highly adhesive hydrogel with exosomes for endogenous cell recruitment and cartilage defect regeneration. Biomaterials 278, 121169. doi:10.1016/j.biomaterials.2021.121169
Zhang, L., Jiao, G., Ren, S., Zhang, X., Li, C., Wu, W., et al. (2020). Exosomes from bone marrow mesenchymal stem cells enhance fracture healing through the promotion of osteogenesis and angiogenesis in a rat model of nonunion. Stem Cell Res. Ther. 11 (1), 38. doi:10.1186/s13287-020-1562-9
Zhang, S., Chu, W. C., Lai, R. C., Lim, S. K., Hui, J. H., Toh, W. S., et al. (2016). Exosomes derived from human embryonic mesenchymal stem cells promote osteochondral regeneration. Osteoarthr. Cartil. 24 (12), 2135–2140. doi:10.1016/j.joca.2016.06.022
Zhang, S., Chuah, S. J., Lai, R. C., Hui, J. H. P., Lim, S. K., Toh, W. S., et al. (2018). MSC exosomes mediate cartilage repair by enhancing proliferation, attenuating apoptosis and modulating immune reactivity. Biomaterials 156, 16–27. doi:10.1016/j.biomaterials.2017.11.028
Zhang, S., Teo, K. Y. W., Chuah, S. J., Lai, R. C., Lim, S. K., Toh, W. S., et al. (2019). MSC exosomes alleviate temporomandibular joint osteoarthritis by attenuating inflammation and restoring matrix homeostasis. Biomaterials 200, 35–47. doi:10.1016/j.biomaterials.2019.02.006
Zhang, W., Qi, L., Chen, R., He, J., Liu, Z., Wang, W., et al. (2021b). Circular RNAs in osteoarthritis: Indispensable regulators and novel strategies in clinical implications. Arthritis Res. Ther. 23 (1), 23. doi:10.1186/s13075-021-02420-2
Zhao, Y., and Xu, J. (2018). Synovial fluid-derived exosomal lncRNA PCGEM1 as biomarker for the different stages of osteoarthritis. Int. Orthop. 42 (12), 2865–2872. doi:10.1007/s00264-018-4093-6
Zhao, Z., Fang, R., Rong, Q., and Liu, M. (2017). Bioinspired nanocomposite hydrogels with highly ordered structures. Adv. Mat. 29 (45), 1703045. doi:10.1002/adma.201703045
Zheng, L., Wang, Y., Qiu, P., Xia, C., Fang, Y., Mei, S., et al. (2019). Primary chondrocyte exosomes mediate osteoarthritis progression by regulating mitochondrion and immune reactivity. Nanomedicine (Lond) 14 (24), 3193–3212. doi:10.2217/nnm-2018-0498
Zhou, X., Liang, H., Hu, X., An, J., Ding, S., Yu, S., et al. (2020). BMSC-derived exosomes from congenital polydactyly tissue alleviate osteoarthritis by promoting chondrocyte proliferation. Cell Death Discov. 6 (1), 142. doi:10.1038/s41420-020-00374-z
Zhou, Y., Ming, J., Li, Y., Li, B., Deng, M., Ma, Y., et al. (2021). Exosomes derived from miR-126-3p-overexpressing synovial fibroblasts suppress chondrocyte inflammation and cartilage degradation in a rat model of osteoarthritis. Cell Death Discov. 7 (1), 37. doi:10.1038/s41420-021-00418-y
Zhu, Y., Wang, Y., Zhao, B., Niu, X., Hu, B., Li, Q., et al. (2017). Comparison of exosomes secreted by induced pluripotent stem cell-derived mesenchymal stem cells and synovial membrane-derived mesenchymal stem cells for the treatment of osteoarthritis. Stem Cell Res. Ther. 8 (1), 64. doi:10.1186/s13287-017-0510-9
Keywords: exosomes, osteoarthritis, biomarkers, cartilage, cartilage tissue engineering
Citation: Fan W-J, Liu D, Pan L-Y, Wang W-Y, Ding Y-L, Zhang Y-Y, Ye R-X, Zhou Y, An S-B and Xiao W-F (2022) Exosomes in osteoarthritis: Updated insights on pathogenesis, diagnosis, and treatment. Front. Cell Dev. Biol. 10:949690. doi: 10.3389/fcell.2022.949690
Received: 21 May 2022; Accepted: 04 July 2022;
Published: 26 July 2022.
Edited by:
Zeyu Huang, Sichuan University, ChinaCopyright © 2022 Fan, Liu, Pan, Wang, Ding, Zhang, Ye, Zhou, An and Xiao. This is an open-access article distributed under the terms of the Creative Commons Attribution License (CC BY). The use, distribution or reproduction in other forums is permitted, provided the original author(s) and the copyright owner(s) are credited and that the original publication in this journal is cited, in accordance with accepted academic practice. No use, distribution or reproduction is permitted which does not comply with these terms.
*Correspondence: Yang Zhou, zhouyangcch@163.com; Sen-Bo An, ansenbo@sdfmu.edu.cn; Wen-Feng Xiao, xiaowenfeng@csu.edu.cn
†These authors have contributed equally to this work and share first authorship.